- 1Department of Urology, Zhongnan Hospital of Wuhan University, Wuhan, China
- 2Wuhan Research Center for Infectious Diseases and Cancer, Chinese Academy of Medical Sciences, Wuhan, China
- 3Department of Biological Repositories, Zhongnan Hospital of Wuhan University, Wuhan, China
- 4President’s Office, Zhongnan Hospital of Wuhan University, Wuhan, China
- 5Human Genetic Resource Preservation Center of Hubei Province, Wuhan, China
Prostate cancer (PCa) is one of the most common male malignancies with frequent remote invasion and metastasis, leading to high mortality. Epithelial-mesenchymal transition (EMT) is a fundamental process in embryonic development and plays a key role in tumor proliferation, invasion and metastasis. Numerous long non-coding RNAs (lncRNAs) could regulate the occurrence and development of EMT through various complex molecular mechanisms involving multiple signaling pathways in PCa. Given the importance of EMT and lncRNAs in the progression of tumor metastasis, we recapitulate the research progress of EMT-related signaling pathways regulated by lncRNAs in PCa, including AR signaling, STAT3 signaling, Wnt/β-catenin signaling, PTEN/PI3K/AKT signaling, TGF-β/Smad and NF-κB signaling pathways. Furthermore, we summarize four modes of how lncRNAs participate in the EMT process of PCa via regulating relevant signaling pathways.
Introduction
Prostate cancer (PCa) is one of the most common malignancies in males worldwide and has become a global “killer” threatening the health of elderly men. The incidence and mortality of PCa rank second and fifth in men worldwide, respectively (Sung et al., 2021). Accounting for 27% of diagnoses and 11% deaths among all the male malignancies, PCa currently had the highest incidence rate and became the second most common cause of cancer-related death in the United States (Siegel et al., 2021; Siegel et al., 2022). Although the incidence of PCa in China is lower than that in American and African countries, the incidence and mortality have increased dramatically in the past 2 decades, and the annual increase ranks first among male malignant tumors (Sun et al., 2020). PCa is a hormone-dependent disease, therefore therapies targeting the androgen receptor (AR) signaling, especially the androgen deprivation therapy (ADT), become the main clinical theory for PCa patients (Ishikawa et al., 1989; Shore et al., 2020). Even though most PCa patients present a satisfying response to ADT for a while, the disease may finally develop into castration-resistant prostate cancer (CRPC) stage, characterized by resistance to ADT and aggressive metastases in a considerable proportion of patients (Galletti et al., 2017). The 5-year survival rate for localized PCa was 99.3%, while that for metastatic PCa decreased sharply to 32.3%, demonstrating that metastasis is the leading cause of PCa-related mortality (Heidenreich et al., 2014; Siegel et al., 2020).
For tumor metastasis, EMT is an important initiating factor driving this process and plays a critical role, which confers metastatic characteristics on cancer cells by increasing mobility and invasion (Nakazawa et al., 2017). EMT is a reversible process in which relatively stable epithelial cells lose cell polarity and intercellular adhesion, and transform into spindle-shaped mesenchymal cells with migration ability (Kalluri and Weinberg, 2009). During the process of EMT, the level of multiple epithelial cell markers would decrease, such as cytokeratins, laminin and E-cadherin, which lead to the loss of cell-to-cell adhesion. In contrast, mesenchymal markers, such as vimentin, N-cadherin, β-catenin and Snail protein, are up-regulated, thereby allowing the cells to migrate or metastasize to different organs (Odero-Marah et al., 2018). While EMT prevents cell apoptosis and senescence, it will also cause organ fibrosis and promote tumor development and metastasis (Bhangu et al., 2012).
LncRNAs are non-coding RNAs with length greater than 200 nucleotides and hundreds of them are dysregulated in human tumors with complex regulatory mechanisms (Yu and Shan, 2016). LncRNAs have been reported to regulate the development of EMT by targeting EMT-related inducible transcription factors such as Twist, Snail, Slug and Zeb (Peinado et al., 2007). Recent studies indicated that a variety of signaling pathways regulated by lncRNAs promoted EMT and led to tumor metastasis (Montano and Bushman, 2017; Yeh et al., 2019).
The metastasis of PCa, which leads to treatment failure and death of patients, is attributed to multi-system and multi-level pathological alterations affected by multiple factors, but the molecular mechanism is not yet fully understood (Hussain et al., 2020; Subudhi et al., 2020). In this review, we aimed to recapitulate the present studies of lncRNAs in PCa and their regulation in EMT process, as well as the signaling pathways regulated by EMT-related lncRNAs in PCa.
EMT and Tumor Metastasis
EMT occurs during different stages of embryonic development and can be classified into three distinct functional types based on their biological contexts, ranging from type I contributing to gastrulation and organ development found in normal embryological development to type III contributing to increased cancer cell invasiveness found in cancer progression and metastasis (Kalluri and Weinberg, 2009). EMT could be induced by a set of specific transcription factors including members of the Snail, ZEB and Twist families (Gheldof and Berx, 2013), which could directly regulate E-cadherin expression (Montanari et al., 2017). Supplementally, the downregulation of E-cadherin is regarded as a defining event when EMT occurred (Lo et al., 2017).
Previous studies have confirmed that the activation of EMT permitted cancer cells to acquire invasion and migration during cancer progression (Liao and Yang, 2017). A variety of molecular mechanisms regulate EMT directly or indirectly (Montanari et al., 2017; Hao et al., 2019). For example, the combination of growth factors or cytokines, such as transforming growth factor-β (TGF-β), epidermal growth factor (EGF) and insulin-like growth factor (IGF), with corresponding receptors participant in EMT via inducing downstream effectors (Maruyama, 2014). In addition, several signaling pathways including AR signaling pathway, PI3K/AKT pathway and Wnt/β-catenin pathway also play pivotal roles in orchestrating EMT and metastatic responses of PCa by cooperating to induce full EMT responses (Lamouille et al., 2014).
LncRNAs and Prostate Cancer
LncRNAs are non-coding RNAs with length greater than 200 nucleotides, which are key regulatory molecules in cells and can function via various paradigms (Wilusz et al., 2009). LncRNAs could be targeted to specific DNA sequences in cis or trans, and this feature gives them the ability to regulate tumor-related target genes at different levels, including epigenetic level, transcription level and post-transcriptional level (Marchese et al., 2017; Ni et al., 2019; Braga et al., 2020; Wang et al., 2020). Some lncRNAs are specifically transcribed and participate in the transduction mediated by special signaling pathways as signal molecules. In addition, lncRNAs are capable of folding into secondary and tertiary structures or sponging miRNA to perform more complex functions (Misawa et al., 2017). In recent years, many studies have shown that lncRNAs have a significant role as regulators of key cellular processes in cancers, including PCa (Prensner and Chinnaiyan, 2011; Liu et al., 2020; Hu C -Y et al., 2021; Tan et al., 2021).
LncRNAs may act as oncogenes or tumor suppressors in PCa and participate in the invasion and metastasis of PCa (Xu et al., 2019; Lu et al., 2021). Functionally, lncRNAs possess important application potential in the occurrence and development, early diagnosis, treatment and prognosis of PCa (Ramnarine et al., 2019; Morgan et al., 2021). An influential application of lncRNA in PCa diagnosis is the detection of PCA3 (Hessels and Schalken, 2009; Morgan et al., 2021), which could regulate the expression of AR signaling pathway and critical genes involved in the carcinogenesis and development of PCa, including EMT-related genes (Ferreira et al., 2012; Lemos et al., 2019; Ghafouri-Fard et al., 2022). LncRNAs are closely correlated with Gleason score, TNM stage and PSA kinetic parameters in PCa, which are recognized as the basis for treatment decision-making and prognosis. The expression of lncRNA TINCR was measured in 160 PCa specimens, and the results revealed that low expression of TINCR was strikingly associated with advanced clinical T stage, lymph node involvement, distant metastasis and high Gleason score (Dong et al., 2018). Besides, lncRNAs are often used as crucial biomarkers in the clinic for the prediction of PCa. For example, single nucleotide polymorphisms (SNPs) in lncRNA PCAT19 was used as a predictor of PCa risk variant based on decreased and increased levels of PCAT19-short and PCAT19-long (Hua et al., 2018). LncRNAs that contribute to tumor proliferation, invasion and metastasis in PCa and may function as oncogenes or tumor suppressors are shown in Table 1.
EMT-Related Signaling Pathways Regulated by lncRNAs in PCa
The tumorigenesis and development of PCa is a multi-channel, multi-link, multi-level and highly complex regulatory process, in which many signaling pathways, such as AR signaling pathway, STAT3 signaling pathway, Wnt/β-catenin signaling pathway, play diverse roles in the process of EMT mediated PCa metastasis (Fearon et al., 2012; Murata and Kang, 2018; Shorning et al., 2020). Recent studies have found that lncRNAs in PCa could participate in the regulation of key genes involved in the above EMT-related signaling pathways. In order to clarify the regulatory network between them, this review summarized the research progress of EMT-related signaling pathways regulated by lncRNAs in PCa in recent years.
AR Pathway
Androgen and AR are two main pathogenic factors of PCa. AR is a member of the nuclear receptor superfamily, which mainly exists in the nucleus and belongs to steroid receptors. Androgen is the main ligand of AR. When the ligand is lacking, AR is mainly distributed in the cytoplasm, binding to heat shock proteins and sustaining an inactive state (Husmann et al., 1990; Sar et al., 1990; Heery et al., 1997). When androgen enters the cell, AR will dissociate from heat shock protein, releasing ligand binding domain and spontaneously dimerizing. Then dimerized AR enters the nucleus and bind to ARE (Androgen Response Elements) on target genes to exert its transcription regulatory role.
The interaction network between AR and lncRNAs is multi-dimensional instead of unidirectional. AR is a transcription factor and there existed a positive feedback loop between AR and AR-induced lncRNAs in PCa cells. Zhang et al. (Zhang J et al., 2018) identified ARLNC1 (AR-regulated long non-coding RNA 1) as a target lncRNA of AR. They demonstrated that AR induced the expression of ARLNC1 and ARLNC1 stabilized AR via direct RNA-RNA interaction, and silencing ARLNC1 could reduce the global activity of AR signaling and the viability of PCa cells. Huntingtin-interacting protein 1 (HIP1) is an important AR regulator that could modulate the transcriptional activity of AR (Mills et al., 2005). Two research groups have reported similar positive feedback loops, mediated by HIP1, between AR and AR-induced lncRNAs. Shi et al. (Shi et al., 2021) and Li et al. (Mather et al., 2021) both treated LNCaP cells and VCap cells with 10 nM dihydrotestosterone, identifying both PCLN16 and PCAL7 could suppress the migration and proliferation of PCa cells. Functionally, they found PCLN16 and PCAL7 were transcriptionally induced by AR, and both the two lncRNAs could interact with HIP1 and reduce HIP1 degradation, advancing AR signaling via positive feedback loops.
Another important regulatory mechanism of the expression of AR protein is via ubiquitination or de-ubiquitination. The ubiquitination process of AR is mainly mediated by the E3 ubiquitin ligase MDM2, a well-known regulator of TP53. Previous studies reported that LncRNA LINC00675 and HOTAIR were markedly upregulated in androgen-insensitive PCa cell lines and CRPC patients, blocking the interaction of AR proteins with MDM2 and thereby preventing AR ubiquitination and protein degradation (Zhang et al., 2015; Yao et al., 2020). LncRNA PCBP1-AS1 (Zhang B et al., 2021) was reported to be able to stabilize AR via promoting the stability of USP22-AR complex by binding to AR NTD domain and enhancing AR de-ubiquitination. Importantly, targeting LINC00675, HOTAIR and PCBP1-AS1 all could effectively inhibit the growth and invasion ability of PCa cells and restore the drug sensitivity of Enzalutamide-resistant PCa cells, which offered distinct view for future clinical utilization.
Besides increasing the stability of AR protein, lncRNAs were also reported to affect the stability of AR mRNA and subsequent translation. Lnc-OPHN1-5, located closely to AR on chromosome X, was an AR-suppressor and could elevate Enzalutamide (Enz) sensitivity via inhibiting AR signaling (Zhang M et al., 2021). The study demonstrated that lnc-OPHN1-5 competed with hnRNPA1 to bind to the 3′UTR site of AR mRNA, not only decreasing the half-life period of AR mRNA but also blocking the enrichment of AR mRNA in the ribosome RNA 18S. PVT1 is a widely reported lncRNA in PCa which is over-expressed and related to an unwilling prognosis (Yang et al., 2017; Liu et al., 2021). Videria et al. (Videira et al., 2021) found that PVT1 may participate in an AR-dependent transcriptional repression program in PCa cells. They demonstrated that 160 genes repressed by AR were up-regulated and the epigenetic markers such as H3K27me3 and H3K27ac were remodeled when PVT1 was silenced. Therefore, they speculated that PVT1 may assist AR in repressing the expression of tumor suppressor genes via altering the epigenetic mode in PCa cells.
Taken together, the interaction network between AR and lncRNAs is multi-directional. AR can induce the transcription of certain lncRNAs, and lncRNAs could also regulate the expression abundance of AR at mRNA or protein level. Such reciprocal mode inside the AR-lncRNAs network may indicate us more potential methods to improve current anti-androgen therapies.
STAT3 Signaling Pathway
STAT3 is a member of the signal transducer and activator of transcription (STAT) family. STAT family has dual functions of signal transduction and transcriptional activation. In JAK-STAT signal pathway, JAK (Janus kinase) and STATs are intracellular and receptor binding proteins, which complete signal transduction from cytoplasm to nucleus (Darnell et al., 1994). Generally, STAT3 signaling pathway is activated by extracellular stimulus, especially diverse cytokines, including IL-6, IL-8, CXCL-5, and COX2, from the surrounding tumor micro-environment (Lou et al., 2000; Tong et al., 2017; Roca et al., 2018; Zheng P et al., 2018). While non-canonical regulation of STAT3 pathway always occurred inside cells involving the phosphorylation of Y705 site or S727 site and the ubiquitination of STAT3 (Bae et al., 2016; Wang et al., 2018). The continuously activated STAT3 signaling pathway plays an important role in tumorigenesis, including human PCa (Mora et al., 2002; Kroon et al., 2013; Schroeder et al., 2014). As reported by Nicholas et al.(Don-Doncow et al., 2017), 95% of 223 metastatic PCa samples were positive staining of p-STAT3 and the bone metastases presented an extremely obvious expression of p-STAT3 compared with lymph nodes or visceral metastases samples, suggesting the crucial role of active STAT3 in promoting the metastasis of PCa.
MAGI2-AS3 was a significantly reduced lncRNA in PCa samples, and it acted as the miR-424-5p sponge to suppress the progression of PCa by regulating COP1 expression and STAT3 signaling pathway activation (Wei et al., 2022). Jiang et al. (Jiang et al., 2021) reported that LINC00467 can promote PCa cell growth and metastasis via two methods. Firstly, silencing LINC00467 in tumor-associated lymphocytes (TAMs) promoted TAM polarization to the M2 subtype and inhibited the metastatic capacity of co-cultured PCa cells. Secondly, LINC00467 could promote PCa cell growth and metastasis via sponging miR-494-3p and subsequently activating the expression of STAT3. Zhang et al. found lncAMPC promoted the metastasis via a dual function in the cytoplasm and nucleus. They proved that cytoplastic lncAMPC could induce the expression of LIF by binding and suppressing the activity of miR637. While lncAMPC in the nucleus could remove histone H1.2 from the distal promoter region of LIFR and therefore up-regulated LIFR level. The axis of LIF/LIFR was a well-known inducer of JAK1-STAT3 signal (Nicola and Babon, 2015; Chan et al., 2019). Due to the up-regulated expression of LIF/LIFR axis under the dual stimulation of lncAMPC, JAK1-STAT3 signaling pathway was activated with the ensuing promoted expression of metastasis-associated genes, ultimately leading to the metastasis of PCa (Zhang et al., 2020). Zhu et al. also reported the lncRNA-activator role of STAT3 in PCa. STAT3 induced the expression of LINC00160, then LINC00160 bound to EZH2, leading to the hypermethylation of RCAN1, and therefore promoted the proliferation and metastasis of PCa cells (Zhu et al., 2022).
In conclusion, besides the canonical ceRNA interaction network of lncRNA-miRNA-mRNA, there also may exist a reciprocal interaction between lncRNAs and STAT3. As a transcription activator, STAT3 could induce the expression of certain lncRNAs, such as LINC00160, to epigenetically regulate the expression mode of down-stream genes. Besides, some lncRNAs could indirectly activate STAT3 pathway via regulate the expression of proteins up-stream of STAT3 signaling pathway, such as lncAMPC.
Wnt/β-Catenin Signaling Pathway
Wnt gene is the combined term of homologous genes Int and Wingless. The Wnt protein encoded and expressed by Wnt gene is a collection of secretory glycoprotein families including 19 Wnt protein members (Ring et al., 2014). Wnt signaling pathway is composed of transcription regulatory factors, functional proteins and enzymes, and β-Catenin is a key component of Wnt signaling pathway (Watanabe et al., 2004). β-Catenin is a multifunctional effector protein and its N-terminal and C-terminal have binding sites with glycogen synthase kinase-3 (GSK-3) and T-cell factor/lymphoid enhancing factor (TCF/LEF), respectively. It plays an important role in maintaining cell adhesion and the morphological structure of adjacent tissues. (Mao et al., 2014).
Canonical Wnt/β-Catenin pathway plays an important role in different stages of tumor development, including cancer cell proliferation, migration, invasion, tumorigenesis and metastasis. Normally, the cytoplastic β-Catenin complex consists of Axin, APC and CK1α and GSK-3β. The complex is encapsulated with E3 ubiquitin ligase β-TRCP and eventually degraded by the ubiquitin-proteome pathway (Jang et al., 2015). Wnt1 ligands (Wnt2, Wnt3, Wnt3a and wnt8a) bind to FZD receptor and LRP5/6 to initiate Wnt signaling pathway. Subsequently, the scattered proteins in the cytoplasm are phosphorylated and form a complex with Axin, which binds to GSK3β to block its activation (Liu et al., 2015). This combination further results in the breakdown of the degradation complex, leading to the accumulation of β-catenin in the cytoplasm. The accumulated β-catenin is transported to the nucleus, which is recognized as the main event of canonical Wnt pathway activation, and then interacts with TCF/LEF to form an activator complex, thereby initiating the transcription of Wnt/β-catenin signal transduction target genes, including c-Myc, Cyclin D1 and MMP-7 (Yoshida and Saya, 2014).
Noncoding RNA activated by DNA damage (NORAD) is a recently identified lncRNA that could promote PCa metastasis via regulating Wnt/β-catenin (Zhang Y et al., 2018). The ceRNA interaction of NORAD and miR-30a-5p promoted the growth and metastasis, while silencing the expression of NORAD exerted the opposite effect. AGAP2-AS1 (AGAP2 antisense RNA 1) is another lncRNA that was reported to activate Wnt/β-catenin pathway. Zhao et al. (Zhao et al., 2021) reported that AGAP2-AS1 could form a feedback loop with miR-628-5p/FOXP2 and promote proliferation and EMT progression of PCa by activating the Wnt signaling pathway.
Moreover, besides the canonical Wnt/β-Catenin pathway, the non-canonical Wnt pathway also participates in the EMT progression of PCa. As a non-canonical Wnt signaling pathway ligand, Wnt5a works with its receptor FZD2 and induces the expression of EMT-related genes in PCa. LncRNA MCM3AP-AS1 has been demonstrated abnormally up-regulated in PCa tissues and could up-regulate the expression of Wnt5a at mRNA and protein level via sponging miR-876-5p in PCa cells (Wu G et al., 2020).
PTEN/PI3K/AKT Signaling Pathway
The deficiency of PTEN (phosphatase and tensin homolog on chromosome 10) tumor suppressor and the oncogenic activation of PI3K (phosphatidylinositol-4,5-bisphosphate 3-kinase) signaling axis are among the most common altered signaling pathways in primary PCa that facilitate tumor occurrence, disease progression and therapeutic resistance (Shorning et al., 2020). Recent studies showed that nearly 15–20% PCa patients presented a loss of function of PTEN and the rate may reach 40–60% when the disease progress into the CRPC or metastatic stage (Yoshimoto et al., 2012; Leinonen et al., 2013; Jamaspishvili et al., 2018). PI3K is mainly composed of the regulatory subunit p85 and the catalytic subunit P110a and could specifically catalyze phosphatidylinositol. The difference between catalytic subunit P110 and related substrates can be divided into class I, II, and III subtypes. The study of type I PI3K showed that activated PI3K targeted PIP2 (phosphatidylinositol diphosphate), located on the plasma membrane, and then transformed into PIP3. Then PIP3, as the second messenger, binds to the downstream protein kinase B (AKT) containing PH domain and PDK1, prompting PDK1 to phosphorylate the Ser308 site of AKT and then activate AKT (Huang et al., 2014). Phosphorylated AKT can activate NF-κB, CREB and other transcription factors to activate anti-apoptotic genes to inhibit cell apoptosis and play a positive role in cell proliferation. Therefore, Akt is regarded as an important hub factor in PI3K/AKT signaling pathway. In human PCa, the phosphorylation of AKT at Ser473 has been reported to be an excellent indicator of the prognosis of PCa patients (Ayala et al., 2004) and the interaction of IGF-I/PI3K/AKT signaling pathway and AR signaling pathway promote the synthesis of PSA (Liu et al., 2011). Moreover, Lamin A/C protein could regulate the activation and inactivation of PTEN to promote the migration and invasion of PCa cells (Yan and Huang, 2019). Taken together, The dysregulated PTEN/PI3K/AKT signaling pathway can regulate the synthesis of various proteins and is involved in the proliferation and apoptosis, migration and differentiation of PCa cells (Wu et al., 2018).
LncRNA MBNL1-AS1 exerted a positive regulation of PTEN. As reported, MBNL1-AS1 is down-regulated in PCa cells, and the MBNL1-AS1/miR-181-5p/PTEN axis could suppress the proliferation and migration ability of PCa cells through the inhibitory effect of PTEN on AKT phosphorylation (Ding et al., 2021). LncRNA-ATB is a stimulator of EMT associated with ZEB1 and ZNF217 expression and regulates the EMT progression of PCa via activation of ERK and PI3K/AKT signaling pathways (Xu et al., 2016). In addition, Wu et al. (Wu et al., 2017) analyzed the differentially expressed lncRNAs in three pairs of PCa specimens and found that LINC01296, a novel identified highly expressed in PCa, was related to preoperative PSA (p = 0.002), lymph-node metastasis (p = 0.035), Gleason score (p = 0.001), tumor stage (p = 0.036) and recurrence-free survival of PCa patients. In vitro biological experiments have further proved that knockdown of LINC01296 can inhibit the proliferation, migration and invasion of PCa cells by inhibiting the activity of the PI3K/AKT/mTOR pathway. Another study (Sun et al., 2021) revealed that lncRNA DANCR exerted an oncogenic role in PCa cells via regulating the miR-185-5p/LASP1 axis and activated the PI3K/AKT/GSK3β pathway. Activated GSK3β promoted the transcriptional activity of Snail, leading to the elevated migration and invasion ability of PCa cells.
TGF-β/Smad Signaling Pathway
The TGF-β signaling pathway is a transmembrane signal transduction pathway widely existing in invertebrates and vertebrates. TGF-β belongs to a polypeptide superfamily with extensive and complex biological activity regulation. It plays an important role in the regulation of body tissue homeostasis and early embryonic development. The abnormal activation of TGF-β superfamily signaling pathway is related to a variety of diseases, including human cancers, fibrotic diseases, autoimmune diseases, etc. (Shangguan et al., 2012; Xue et al., 2014; Fennen et al., 2016). The Smad family is an important type of transcriptional regulator, and Smad2 and Smad3 are the key downstream factors of TGF-β. Activated TGF-β binds to TGF-β receptor on the cell membrane, catalyzes the phosphorylation and activation of Smad2 and Smad3, and then Smad2/3 and Smad4 bind to the nucleus and regulate target gene transcription (Park et al., 2015; Zhao et al., 2020). In the early stage of tumor, TGF-β signal can suppress tumor growth by inhibiting the secretion of cytokines and growth factors, and inducing apoptosis. When the tumor progresses to the pathological stage, due to the loss or mutation of heterozygous alleles, the inhibitory effect of TGF-β signal disappears, and it plays a role in promoting cancer (Oh and Joo, 2020). Specifically, TGF-β up-regulates the expression levels of VEGF and bFGF, and simultaneously induces the expression of MMP-2 and MMP-9. MMPs are enzymes that could promote the degradation of the vascular endothelial basement membrane and promote the neovascularization of solid tumors, which greatly benefits tumor distant metastasis. Studies have reported that the expression of TGF-β1 and Smad2 are higher in PCa tissues than that in prostate epithelial tissues. The high expression of TGF-β1 can induce cell metastasis, invasion and cause adverse effects on the overall survival of PCa patients (Atılgan et al., 2016).
LncRNA ANRIL was overexpressed in PCa, and knockdown of ANRIL significantly decreased the levels of TGF-β1 and p-Smad2 and inhibited the proliferation and migration of PCa cells (Zhao et al., 2018). The low expression of lncRNA DGCR5 acted as a predictor of poor survival in PCa. While over-expressing DGCR5 could decrease the stemness of PCa cells with the down-regulation of TGF-β1, and supplementing TGF-β1 could reverse the effect of over-expressing DGCR5 (Li B et al., 2019). Zhang et al. (Zhang H et al., 2019) reported that lncRNA MIR4435-2HG could promote the migration and invasion of PCa cells and the treatment of TGF-β inhibitor attenuated the enhancing effects of over-expression MIR4435-2HG in PCa cells. Distant bone metastasis is a well-recognized lethal event of advanced PCa patients. PCAT7, a bone metastasis-related lncRNA, could activate TGF-β/Smad signaling via elevating the protein level of TGFBR1 with sponging miR-324-5p and the activated TGF-β/Smad signaling in turn could induce the expression of PCAT7 by promoting the formation of a Smad3/Smad4/SP1 complex, which demonstrated a positive feedback loop in promoting the bone metastasis in PCa cells (Lang et al., 2020).
NF-κB Signaling Pathway
NF-κB signaling pathway is a widely reported pathway mainly involved in immune or inflammatory regulation. Canonical NF-κB signaling pathway consists of the several sequential events:the activation of IKK complex (containing catalytic subunits IKKα and IKKβ and regulatory subunit NEMO), phosphorylation of IκBα (activated by IKK complex), degradation of IκBα protein and release of NF-κB and the nuclear shuttle of NF-κB. The aberrant activation of NF-κB signaling pathway is also reported to participate in the alteration of diverse tumor phenotypes (Wang et al., 1996; Chen et al., 1999; Yamamoto and Gaynor, 2001; Karin and Greten, 2005). In human PCa, lncRNA DRAIC is reported to inhibit the progression of PCa via suppressing the activation of NF-κB (Saha et al., 2020). As reported, DRAIC obstruct the formation of IKK complex via directly interact with IKKα subunit and NEMO subunit, alleviating the phosphorylation of IκBα protein and the nuclear transportation of NF-κB. The inactivation of NF-κB signaling pathway by DRAIC could efficiently inhibit the growth and metastasis ability of human PCa cells. Moreover, Shang et al. (Shang et al., 2019) reported lncRNA PCAT1 could activate the NF-κB signaling pathway in CRPC cells. They firstly identified PCAT1 as an up-regulated and prognosis-related lncRNA in CRPC samples and found PCAT1 could compete with PHLPP to bind to the C-terminal tetratricopeptide repeat (TPR) domains of FKBP51 protein in an androgen-deprive environment. This competitive perturbation of FKBP51 and PHLPP not only abolished the suppression of AKT by PHLPP but also strengthened the stability of FKBP51/IKKα complex, resulting in the constant activation of NF-κB signaling pathway and finally the progression of PCa disease.
Other Signaling Pathways or Effective Proteins
The above is the main lncRNAs-regulated signaling pathways that were mostly reported to participate in the EMT process of human PCa cells, while researches about other signaling pathways, especially the pathways targeting EMT process, are not so abundant. Here, we selected the three most typical ones to make a brief discussion. The first one is NORAD. In addition to regulating Wnt/β-catenin signaling pathway, lncRNA NORAD also could regulate the release of extracellular vesicles (EVs) to promote the bone metastasis of PCa cells (Hu W et al., 2021). Via regulating miR-541-3p-PKM2 axis, NORAD could affect the release of EVs through phosphorylating SNAP-23 and promote the internalization of EVs by up-regulating the production of ATP in EVs. Elevated EVs containing PKM2 finally induced the bone metastasis of PCa cells. Secondly, NEAT1-1 functioned as a bridge to connect CYCLIN1 and CDK19 via a m6A dependent manner (Wen et al., 2020). The special complex promoted the phosphorylation of Pol II Ser2 and transcription of RUNX2 to induce distant metastasis of PCa cells. The third one is LINC00261, which perform a dual-role both in cytoplasm and in nucleus like lncAMPC (Mather et al., 2021). In the cytoplasm, LINC00261 promoted the expression of CBX2 via sponging miR-8485 through a ceRNA network. While in the nucleus, LINC00261 acted as a scaffold to induce SMAD-driven expression of the FOXA2. The hyper-activation axis of LINC00261-CBX2-FOXA2 coordinatively promoted the proliferation and metastasis of PCa cells. Other pathways or key factors that could interact with certain lncRNAs in PCa still deserve more interest for further exploration.
Discussion
In our review, we discuss the role of lncRNAs in participating in the EMT process in PCa via regulating multiple signaling pathways, including AR signaling pathway, STAT3 signaling pathway, Wnt/β-catenin signaling pathway, PTEN/PI3K/AKT signaling pathway, TGF-β/Smad pathway and NF-κB signaling pathway. Based on our discussion, there are four modes of how lncRNAs participate in the EMT process of PCa via regulating diverse signaling pathways: 1) Forming a ceRNA network via sponging miRNAs to alleviate the repression of downstream target genes and therefore activate or inactivate related signaling pathways, such as MAGI2-AS3 in regulating STAT3 signaling pathway and NORAD in regulating Wnt/β-catenin signaling pathway (shown in Figure 1); 2) Forming a positive feedback loop with key signaling effectors, such as the interaction between ARLNC1 with AR and PCAT7 with TGF-β/Smad signaling pathway (shown in Figure 2); 3) Epigenetically regulating the transcription of down-stream genes, for example, lncAMPC in regulating STAT3 signaling pathway (shown in Figure 3) or PVT1 in regulating AR signaling pathway; 4) Competing the binding site with regulator to promote the stability of key signaling effectors, namely HOTAIR in blocking the degradation of AR and PCAT1 in activating of NF-κB (shown in Figure 4).
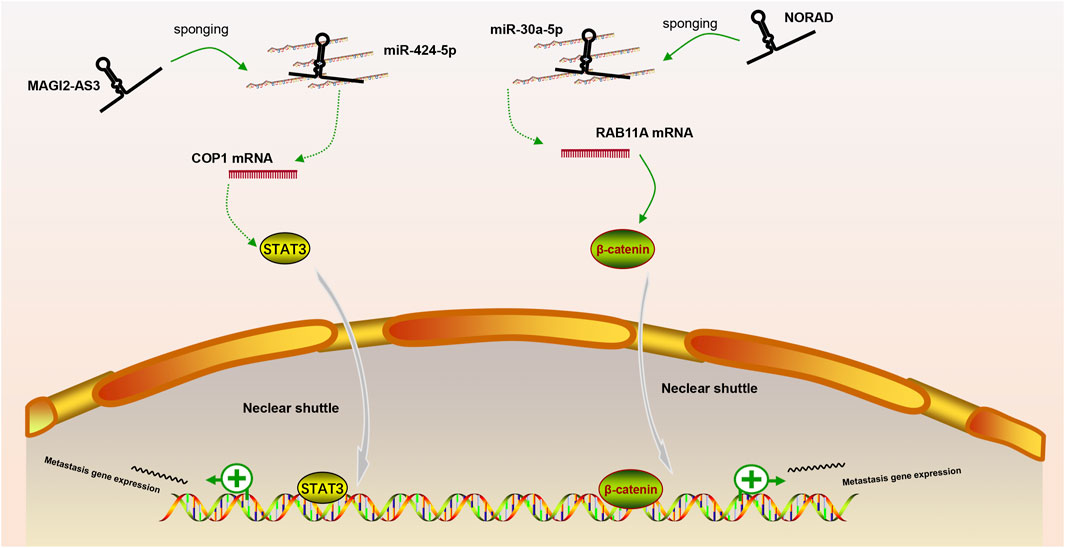
FIGURE 1. The ceRNA network of lncRNA in regulating downstream signaling pathway. MAGI2-AS3 and NORAD regulate STAT3 or Wnt/β-catenin signaling pathway via sponging miR-424-5p or miR-30a-5p, respectively.
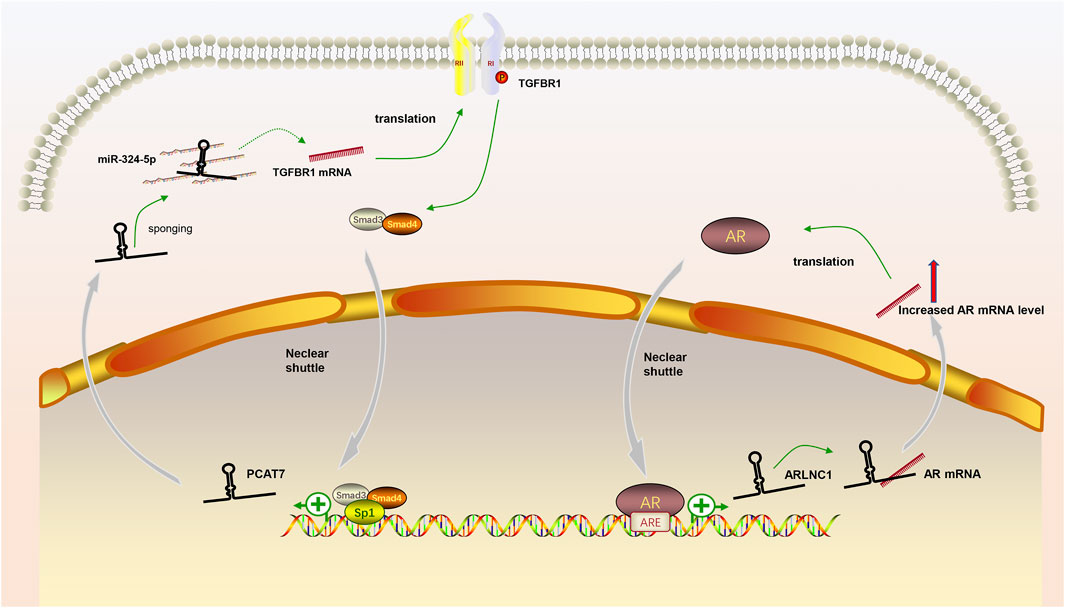
FIGURE 2. The positive feedback loop between lncRNAs and key signaling effectors. (1) AR induced the expression of lncRNA ARLNC1 and ARLNC1 increased the stability of AR mRNA to promote AR signaling pathway; (2) LncRNA PCAT7 increased the level of TGFBR1 and activated TGF-β/Smad signaling pathway promoted the expression of PCAT7 via the formation of Smad3/Smad4/SP1 complex.
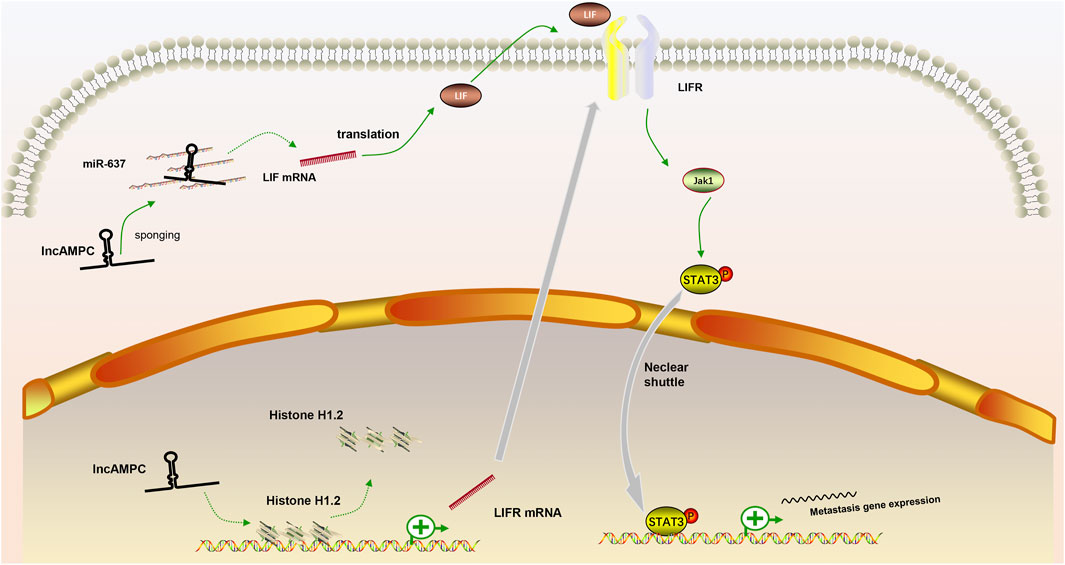
FIGURE 3. The positive feedback loop between lncRNAs and key signaling effectors. (1) In the cytoplasm, lncAMPC upregulated LIF expression by sponging miR-637; (2) In the nucleus, lncAMPC enhanced LIFR transcription by decoying histone H1.2 away from the upstream sequence of the LIFR gene; (3) The increased binding of LIF and LIFR stimulated the JAK1-STAT3 pathway to promote metastasis-associated gene expression.
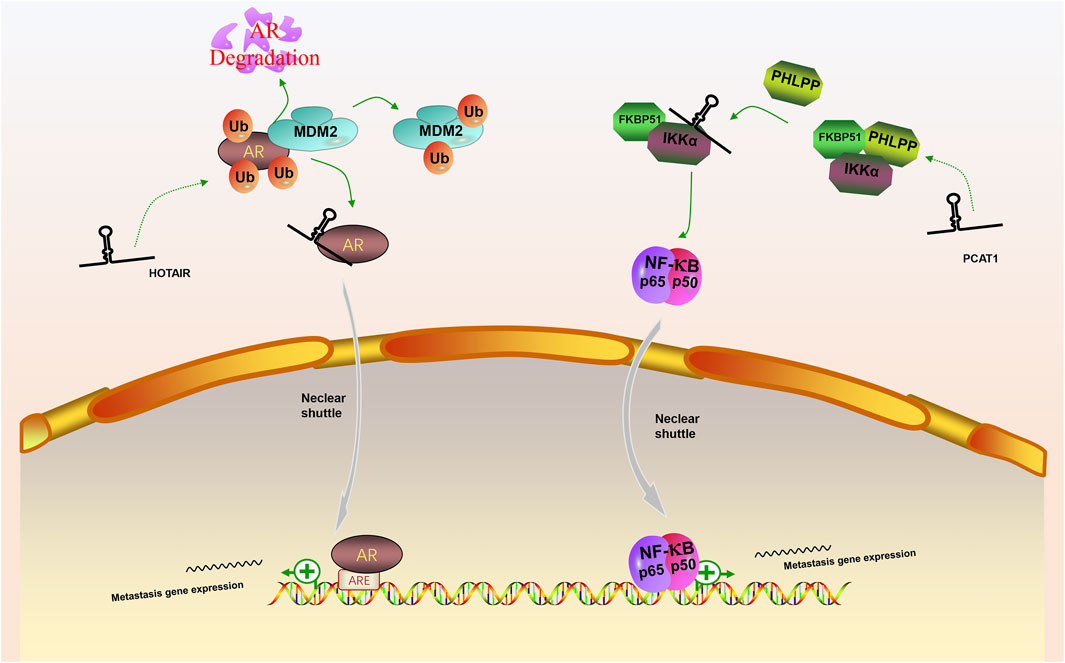
FIGURE 4. The competing integration of the binding site between lncRNAs with specific regulators to promote the stability of key signaling effectors. (1) LncRNA HOTAIR interfered the degradation of AR by MDM2 and promote the AR signaling pathway; (2) LncRNA PCAT1 activate NF-κB signaling pathway via abolishing the inhibitory effect of PHLPP on IKKα.
Moreover, considering that metastasis is the main lethal cause of PCa patients, we hope clinical doctors and researchers could benefit from our current literature review. From our standpoint, among the signaling pathways we discussed above, lncRNAs targeting AR signaling pathway are of much more values and here we raised several unsolved points that deserve further exploration. 1) Bioinformatic analysis or clinical researches about lncRNAs that could predict the metastasis-free survival (MFS) is absent. As distant metastasis is universally regarded as the terminal status of PCa patients, researches that are lack of quantitative analysis of MFS generally failed to evaluate the progress of PCa patients, since only MFS has been shown to be a surrogate endpoint for overall survival (OS) (Xie et al., 2020; Gharzai et al., 2021). 2) Researches about lncRNAs that targeting distant metastasis is deficient. The lncRNAs reported above are mainly differentially expressed in samples of public database, which neglecting the particularity of metastatic samples. 3) Researches about lncRNAs that could promote adjuvant therapies are scarce. Latest research demonstrated that radiotherapy combined with ADT help to improve MFS without compromising quality of life (Kishan et al., 2022). However, we find that researches focusing on the role of lncRNAs in promoting radiotherapy in PCa are very sparse. Therefore, we think these may be new directions for future researches.
Author Contributions
KQ, GW, DS, and HP organized the review study. DS, HP, CX, ZD, XT, and KQ collected and analyzed literature. All authors wrote the manuscript and approved the final version.
Funding
This work was supported by the Zhongnan Hospital of Wuhan University Science, Technology and Innovation Seed Fund (CXPY2020031), Hubei Provincial Natural Science Foundation (2021CFB453), Improvement Project for Theranostic Ability on Difficulty Miscellaneous Disease (Tumor) from National Health Commission of China (ZLYNXM202006), and the Non-profit Central Research Institute Fund of Chinese Academy of Medical Sciences (2020-PT320-004).
Conflict of Interest
The authors declare that the research was conducted in the absence of any commercial or financial relationships that could be construed as a potential conflict of interest.
Publisher’s Note
All claims expressed in this article are solely those of the authors and do not necessarily represent those of their affiliated organizations, or those of the publisher, the editors and the reviewers. Any product that may be evaluated in this article, or claim that may be made by its manufacturer, is not guaranteed or endorsed by the publisher.
Acknowledgments
We gratefully thank all members of Department of Biological Repositories, Zhongnan Hospital of Wuhan University for helpful discussions.
References
Atılgan, A. O., Özdemir, B. H., Akçay, E. Y., Demirkan, Ö. Ataol, Tekindal, M. A., and Özkardeş, H. (2016). Role of Tumor-Associated Macrophages in the Hexim1 and TGFβ/SMAD Pathway, and Their Influence on Progression of Prostatic Adenocarcinoma. Pathology - Res. Pract. 212 (2), 83–92. doi:10.1016/j.prp.2015.10.011
Ayala, G., Thompson, T., Yang, G., Frolov, A., Li, R., Scardino, P., et al. (2004). High Levels of Phosphorylated Form of Akt-1 in Prostate Cancer and Non-neoplastic Prostate Tissues Are Strong Predictors of Biochemical Recurrence. Clin. cancer Res. official J. Am. Assoc. Cancer Res. 10 (19), 6572–6578. doi:10.1158/1078-0432.ccr-04-0477
Bae, S. B., Cho, H. D., Oh, M.-H., Lee, J.-H., Jang, S.-H., Hong, S. A., et al. (2016). Expression of Programmed Death Receptor Ligand 1 with High Tumor-Infiltrating Lymphocytes Is Associated with Better Prognosis in Breast Cancer. J. Breast Cancer 19 (3), 242–251. doi:10.4048/jbc.2016.19.3.242
Bhangu, A., Wood, G., Mirnezami, A., Darzi, A., Tekkis, P., and Goldin, R. (2012). Epithelial Mesenchymal Transition in Colorectal Cancer: Seminal Role in Promoting Disease Progression and Resistance to Neoadjuvant Therapy. Surg. Oncol. 21 (4), 316–323. doi:10.1016/j.suronc.2012.08.003
Braga, E. A., Fridman, M. V., Moscovtsev, A. A., Filippova, E. A., Dmitriev, A. A., and Kushlinskii, N. E. (2020). LncRNAs in Ovarian Cancer Progression, Metastasis, and Main Pathways: ceRNA and Alternative Mechanisms. Ijms 21 (22), 8855. doi:10.3390/ijms21228855
Chan, L.-C., Li, C.-W., Xia, W., Hsu, J.-M., Lee, H.-H., Cha, J.-H., et al. (2019). IL-6/JAK1 Pathway Drives PD-L1 Y112 Phosphorylation to Promote Cancer Immune Evasion. J. Clin. Invest. 129 (8), 3324–3338. doi:10.1172/JCI126022
Chang, Z., Cui, J., and Song, Y. (2018). Long Noncoding RNA PVT1 Promotes EMT via Mediating microRNA-186 Targeting of Twist1 in Prostate Cancer. Gene 654, 36–42. doi:10.1016/j.gene.2018.02.036
Chen, F., Castranova, V., Shi, X., and Demers, L. M. (1999). New Insights into the Role of Nuclear Factor-kappaB, a Ubiquitous Transcription Factor in the Initiation of Diseases. Clin. Chem. 45 (1), 7–17. doi:10.1093/clinchem/45.1.7
Chen, Q.-H., Li, B., Liu, D.-G., Zhang, B., Yang, X., and Tu, Y.-L. (2020). LncRNA KCNQ1OT1 Sponges miR-15a to Promote Immune Evasion and Malignant Progression of Prostate Cancer via Up-Regulating PD-L1. Cancer Cell Int. 20, 394. doi:10.1186/s12935-020-01481-8
Dai, X., Liang, Z., Liu, L., Guo, K., Xu, S., and Wang, H. (2019). Silencing of MALAT1 Inhibits Migration and Invasion by Sponging miR-1-3p in P-rostate C-ancer C-ells. Mol. Med. Rep. 20 (4), 3499–3508. doi:10.3892/mmr.2019.10602
Darnell, J. E., Kerr, l. M., and Stark, G. R. (1994). Jak-STAT Pathways and Transcriptional Activation in Response to IFNs and Other Extracellular Signaling Proteins. Science 264 (5164), 1415–1421. doi:10.1126/science.8197455
Ding, X., Xu, X., He, X.-F., Yuan, Y., Chen, C., Shen, X.-Y., et al. (2021). Muscleblind-like 1 Antisense RNA 1 Inhibits Cell Proliferation, Invasion, and Migration of Prostate Cancer by Sponging miR-181a-5p and Regulating PTEN/PI3K/AKT/mTOR Signaling. Bioengineered 12 (1), 803–814. doi:10.1080/21655979.2021.1890383
Don-Doncow, N., Marginean, F., Coleman, I., Nelson, P. S., Ehrnström, R., Krzyzanowska, A., et al. (2017). Expression of STAT3 in Prostate Cancer Metastases. Eur. Urol. 71 (3), 313–316. doi:10.1016/j.eururo.2016.06.018
Dong, L., Ding, H., Li, Y., Xue, D., and Liu, Y. (2018). LncRNA TINCR Is Associated with Clinical Progression and Serves as Tumor Suppressive Role in Prostate Cancer. Cmar 10, 2799–2807. doi:10.2147/CMAR.S170526
Fearon, K. C. H., Glass, D. J., and Guttridge, D. C. (2012). Cancer Cachexia: Mediators, Signaling, and Metabolic Pathways. Cell Metab. 16 (2), 153–166. doi:10.1016/j.cmet.2012.06.011
Fennen, M., Pap, T., and Dankbar, B. (2016). Smad-dependent Mechanisms of Inflammatory Bone Destruction. Arthritis Res. Ther. 18 (1), 279. doi:10.1186/s13075-016-1187-7
Ferreira, L. B., Palumbo, A., de Mello, K. D., Sternberg, C., Caetano, M. S., de Oliveira, F. L., et al. (2012). PCA3 Noncoding RNA Is Involved in the Control of Prostate-Cancer Cell Survival and Modulates Androgen Receptor Signaling. BMC Cancer 12, 507. doi:10.1186/1471-2407-12-507
Galletti, G., Leach, B. I., Lam, L., and Tagawa, S. T. (2017). Mechanisms of Resistance to Systemic Therapy in Metastatic Castration-Resistant Prostate Cancer. Cancer Treat. Rev. 57, 16–27. doi:10.1016/j.ctrv.2017.04.008
Ghafouri-Fard, S., Khoshbakht, T., Hussen, B. M., Baniahmad, A., Taheri, M., and Rashnoo, F. (2022). A Review on the Role of PCA3 lncRNA in Carcinogenesis with an Especial Focus on Prostate Cancer. Pathology - Res. Pract., 231, 153800doi:doi:10.1016/j.prp.2022.153800
Gharzai, L. A., Jiang, R., Wallington, D., Jones, G., Birer, S., Jairath, N., et al. (2021). Intermediate Clinical Endpoints for Surrogacy in Localised Prostate Cancer: an Aggregate Meta-Analysis. Lancet Oncol. 22 (3), 402–410. doi:10.1016/S1470-2045(20)30730-0
Gheldof, A., and Berx, G. (2013). Cadherins and Epithelial-To-Mesenchymal Transition. Prog. Mol. Biol. Transl. Sci. 116, 317–336. doi:10.1016/B978-0-12-394311-8.00014-5
Han, Y., Hu, H., and Zhou, J. (2019). Knockdown of LncRNA SNHG7 Inhibited Epithelial-Mesenchymal Transition in Prostate Cancer Though miR-324-3p/WNT2B axis in vitro. Pathology - Res. Pract., 215(10), 152537doi:doi:10.1016/j.prp.2019.152537
Hao, S. D., Ma, J. X., Liu, Y., Liu, P. J., and Qin, Y. (2020). Long Non-coding TUG1 Accelerates Prostate Cancer Progression through Regulating miR-128-3p/YES1 axis. Eur. Rev. Med. Pharmacol. Sci. 24 (2), 619–632. doi:10.26355/eurrev_202001_20038
Hao, Y., Baker, D., and Ten Dijke, P. (2019). TGF-β-Mediated Epithelial-Mesenchymal Transition and Cancer Metastasis. Ijms 20 (11), 2767. doi:10.3390/ijms20112767
Heery, D. M., Kalkhoven, E., Hoare, S., and Parker, M. G. (1997). A Signature Motif in Transcriptional Co-activators Mediates Binding to Nuclear Receptors. Nature 387 (6634), 733–736. doi:10.1038/42750
Heidenreich, A., Bastian, P. J., Bellmunt, J., Bolla, M., Joniau, S., van der Kwast, T., et al. (2014). EAU Guidelines on Prostate Cancer. Part II: Treatment of Advanced, Relapsing, and Castration-Resistant Prostate Cancer. Eur. Urol. 65 (2), 467–479. doi:10.1016/j.eururo.2013.11.002
Hessels, D., and Schalken, J. A. (2009). The Use of PCA3 in the Diagnosis of Prostate Cancer. Nat. Rev. Urol. 6 (5), 255–261. doi:10.1038/nrurol.2009.40
Hu, C.-y., Chen, J., Qin, X.-h., You, P., Ma, J., Zhang, J., et al. (2021). Long Non‐coding RNA NORAD Promotes the Prostate Cancer Cell Extracellular Vesicle Release via microRNA-541-3p-Regulated PKM2 to Induce Bone Metastasis of Prostate Cancer. J. Exp. Clin. Cancer Res. 40 (1), 98. doi:10.1186/s13046-021-01891-0
Hu, W., Wang, Y., Fang, Z., He, W., and Li, S. (2021). Integrated Characterization of lncRNA-Immune Interactions in Prostate Cancer. Front. Cell Dev. Biol., 9, 641891doi:doi:10.3389/fcell.2021.641891
Hua, J. T., Ahmed, M., Guo, H., Zhang, Y., Chen, S., Soares, F., et al. (2018). Risk SNP-Mediated Promoter-Enhancer Switching Drives Prostate Cancer through lncRNA PCAT19. Cell 174 (3), 564–575. e18. doi:10.1016/j.cell.2018.06.014
Huang, H.-N., Lin, M.-C., Huang, W.-C., Chiang, Y.-C., and Kuo, K.-T. (2014). Loss of ARID1A Expression and its Relationship with PI3K-Akt Pathway Alterations and ZNF217 Amplification in Ovarian Clear Cell Carcinoma. Mod. Pathol. 27 (7), 983–990. doi:10.1038/modpathol.2013.216
Husmann, D. A., Wilson, C. M., McPhaul, M. J., Tilley, W. D., and Wilson, J. D. (1990). Antipeptide Antibodies to Two Distinct Regions of the Androgen Receptor Localize the Receptor Protein to the Nuclei of Target Cells in the Rat and Human Prostate*. Endocrinology 126 (5), 2359–2368. doi:10.1210/endo-126-5-2359
Hussain, M., Mateo, J., Fizazi, K., Saad, F., Shore, N., Sandhu, S., et al. (2020). Survival with Olaparib in Metastatic Castration-Resistant Prostate Cancer. N. Engl. J. Med. 383 (24), 2345–2357. doi:10.1056/NEJMoa2022485
Ishikawa, S., Soloway, M. S., Van der Zwaag, R., and Todd, B. (1989). Prognostic Factors in Survival Free of Progression after Androgen Deprivation Therapy for Treatment of Prostate Cancer. J. Urology 141 (5), 1139–1142. doi:10.1016/s0022-5347(17)41193-1
Jamaspishvili, T., Berman, D. M., Ross, A. E., Scher, H. I., De Marzo, A. M., Squire, J. A., et al. (2018). Clinical Implications of PTEN Loss in Prostate Cancer. Nat. Rev. Urol. 15 (4), 222–234. doi:10.1038/nrurol.2018.9
Jang, Y.-S., Sim, J. J., Ji, E., Jeong, K.-Y., and Kim, H. M. (2015). Investigation of Lactate Calcium Salt-Induced β-catenin Destabilization in Colorectal Cancer Cells. Life Sci. 139, 160–165. doi:10.1016/j.lfs.2015.08.012
Jiang, H., Deng, W., Zhu, K., Zeng, Z., Hu, B., Zhou, Z., et al. (2021). LINC00467 Promotes Prostate Cancer Progression via M2 Macrophage Polarization and the miR-494-3p/STAT3 Axis. Front. Oncol., 11, 661431doi:doi:10.3389/fonc.2021.661431
Jin, Y., Cui, Z., Li, X., Jin, X., and Peng, J. (2017). Upregulation of Long Non-coding RNA PlncRNA-1 Promotes Proliferation and Induces Epithelial-Mesenchymal Transition in Prostate Cancer. Oncotarget 8 (16), 26090–26099. doi:10.18632/oncotarget.15318
Kalluri, R., and Weinberg, R. A. (2009). The Basics of Epithelial-Mesenchymal Transition. J. Clin. Invest. 119 (6), 1420–1428. doi:10.1172/JCI39104
Karin, M., and Greten, F. R. (2005). NF-κB: Linking Inflammation and Immunity to Cancer Development and Progression. Nat. Rev. Immunol. 5 (10), 749–759. doi:10.1038/nri1703
Kishan, A. U., Wang, X., Sun, Y., Romero, T., Michalski, J. M., Ma, T. M., et al. (2022). High-dose Radiotherapy or Androgen Deprivation Therapy (HEAT) as Treatment Intensification for Localized Prostate Cancer: An Individual Patient-Data Network Meta-Analysis from the MARCAP Consortium. Eur. Urol. 82, 106–114. doi:10.1016/j.eururo.2022.04.003
Kroon, P., Berry, P. A., Stower, M. J., Rodrigues, G., Mann, V. M., Simms, M., et al. (2013). JAK-STAT Blockade Inhibits Tumor Initiation and Clonogenic Recovery of Prostate Cancer Stem-like Cells. Cancer Res. 73 (16), 5288–5298. doi:10.1158/0008-5472.CAN-13-0874
Lamouille, S., Xu, J., and Derynck, R. (2014). Molecular Mechanisms of Epithelial-Mesenchymal Transition. Nat. Rev. Mol. Cell Biol. 15 (3), 178–196. doi:10.1038/nrm3758
Lang, C., Dai, Y., Wu, Z., Yang, Q., He, S., Zhang, X., et al. (2020). SMAD3/SP1 Complex‐mediated Constitutive Active Loop between lncRNA PCAT7 and TGF‐β Signaling Promotes Prostate Cancer Bone Metastasis. Mol. Oncol. 14 (4), 808–828. doi:10.1002/1878-0261.12634
Leinonen, K. A., Saramäki, O. R., Furusato, B., Kimura, T., Takahashi, H., Egawa, S., et al. (2013). Loss of PTEN Is Associated with Aggressive Behavior in ERG-Positive Prostate Cancer. Cancer Epidemiol. Biomarkers Prev. 22 (12), 2333–2344. a Publication of the American Association For Cancer Research, Cosponsored by the American Society of Preventive Oncology. doi:10.1158/1055-9965.EPI-13-0333-T
Lemos, A. E. G., Ferreira, L. B., Batoreu, N. M., de Freitas, P. P., Bonamino, M. H., and Gimba, E. R. P. (2016). PCA3 Long Noncoding RNA Modulates the Expression of Key Cancer-Related Genes in LNCaP Prostate Cancer Cells. Tumor Biol. 37 (8), 11339–11348. doi:10.1007/s13277-016-5012-3
Lemos, A. E. G., Matos, A. d. R., Ferreira, L. B., and Gimba, E. R. P. (2019). The Long Non-coding RNA PCA3: an Update of its Functions and Clinical Applications as a Biomarker in Prostate Cancer. Oncotarget 10 (61), 6589–6603. doi:10.18632/oncotarget.27284
Li, B., Guo, Z., Liang, Q., Zhou, H., Luo, Y., He, S., et al. (2019). lncRNA DGCR5 Up-Regulates TGF-Β1, Increases Cancer Cell Stemness and Predicts Survival of Prostate Cancer Patients. Cmar 11, 10657–10663. doi:10.2147/CMAR.S231112
Li, Z., Wang, F., and Zhang, S. (2019). Knockdown of Lnc RNA MNX 1‐ AS 1 Suppresses Cell Proliferation, Migration, and Invasion in Prostate Cancer. FEBS Open Bio 9 (5), 851–858. doi:10.1002/2211-5463.12611
Liao, T.-T., and Yang, M.-H. (2017). Revisiting Epithelial-Mesenchymal Transition in Cancer Metastasis: the Connection between Epithelial Plasticity and Stemness. Mol. Oncol. 11 (7), 792–804. doi:10.1002/1878-0261.12096
Liu, B., Qian, D., Zhou, W., Jiang, H., Xiang, Z., and Wu, D. (2020). A Novel Androgen-Induced lncRNA FAM83H-AS1 Promotes Prostate Cancer Progression via the miR-15a/CCNE2 Axis. Front. Oncol., 10, 620306doi:doi:10.3389/fonc.2020.620306
Liu, J., Li, Y., Zhang, Q., Lv, C., Wang, M., Jiao, Y., et al. (2021). PVT1 Expression Is a Predictor for Poor Survival of Prostate Cancer Patients. Technol. Cancer Res. Treat., 20, 153303382097161doi:doi:10.1177/1533033820971610
Liu, X., Choi, R. Y., Jawad, S. M., and Arnold, J. T. (2011). Androgen-induced PSA Expression Requires Not Only Activation of AR but Also Endogenous IGF-I or IGF-I/PI3K/Akt Signaling in Human Prostate Cancer Epithelial Cells. Prostate 71 (7), 766–777. doi:10.1002/pros.21293
Liu, X., Yun, F., Shi, L., Li, Z.-H., Luo, N.-R., and Jia, Y.-F. (2015). Roles of Signaling Pathways in the Epithelial-Mesenchymal Transition in Cancer. Asian Pac. J. Cancer Prev. 16 (15), 6201–6206. doi:10.7314/apjcp.2015.16.15.6201
Lo, U.-G., Lee, C.-F., Lee, M.-S., and Hsieh, J.-T. (2017). The Role and Mechanism of Epithelial-To-Mesenchymal Transition in Prostate Cancer Progression. Ijms 18 (10), 2079. doi:10.3390/ijms18102079
Lou, W., Ni, Z., Dyer, K., Tweardy, D. J., and Gao, A. C. (2000). Interleukin-6 Induces Prostate Cancer Cell Growth Accompanied by Activation of Stat3 Signaling Pathway. Prostate 42 (3), 239–242. doi:10.1002/(sici)1097-0045(20000215)42:3<239::aid-pros10>3.0.co;2-g
Lu, K., Yu, M., and Chen, Y. (2021). Non-coding RNAs Regulating Androgen Receptor Signaling Pathways in Prostate Cancer. Clin. Chim. Acta 513, 57–63. doi:10.1016/j.cca.2020.11.027
Lu, X., Chen, D., Yang, F., and Xing, N. (2020). Quercetin Inhibits Epithelial-To-Mesenchymal Transition (EMT) Process and Promotes Apoptosis in Prostate Cancer via Downregulating lncRNA MALAT1. Cancer Manag. Res. 12, 1741–1750. doi:10.2147/CMAR.S241093
Mao, J., Fan, S., Ma, W., Fan, P., Wang, B., Zhang, J., et al. (2014). Roles of Wnt/β-Catenin Signaling in the Gastric Cancer Stem Cells Proliferation and Salinomycin Treatment. Cell Death Dis. 5–e1039. doi:10.1038/cddis.2013.515
Marchese, F. P., Raimondi, I., and Huarte, M. (2017). The Multidimensional Mechanisms of Long Noncoding RNA Function. Genome Biol. 18 (1), 206. doi:10.1186/s13059-017-1348-2
Maruyama, I. (2014). Mechanisms of Activation of Receptor Tyrosine Kinases: Monomers or Dimers. Cells 3 (2), 304–330. doi:10.3390/cells3020304
Mather, R. L., Parolia, A., Carson, S. E., Venalainen, E., Roig‐Carles, D., Jaber, M., et al. (2021). The Evolutionarily Conserved Long Non‐coding RNA LINC00261 Drives Neuroendocrine Prostate Cancer Proliferation and Metastasis via Distinct Nuclear and Cytoplasmic Mechanisms. Mol. Oncol. 15 (7), 1921–1941. doi:10.1002/1878-0261.12954
Mills, I. G., Gaughan, L., Robson, C., Ross, T., McCracken, S., Kelly, J., et al. (2005). Huntingtin Interacting Protein 1 Modulates the Transcriptional Activity of Nuclear Hormone Receptors. J. Cell Biol. 170 (2), 191–200. doi:10.1083/jcb.200503106
Misawa, A., Takayama, K. I., and Inoue, S. (2017). Long Non‐coding RNAs and Prostate Cancer. Cancer Sci. 108 (11), 2107–2114. doi:10.1111/cas.13352
Montanari, M., Rossetti, S., Cavaliere, C., D’Aniello, C., Malzone, M. G., Vanacore, D., et al. (2017). Epithelial-mesenchymal Transition in Prostate Cancer: an Overview. Oncotarget 8 (21), 35376–35389. doi:10.18632/oncotarget.15686
Montano, M., and Bushman, W. (2017). Morphoregulatory Pathways in Prostate Ductal Development. Dev. Dyn. 246 (2), 89–99. doi:10.1002/dvdy.24478
Mora, L. B., Buettner, R., Seigne, J., Diaz, J., Ahmad, N., Garcia, R., et al. (2002). Constitutive Activation of Stat3 in Human Prostate Tumors and Cell Lines: Direct Inhibition of Stat3 Signaling Induces Apoptosis of Prostate Cancer Cells. Cancer Res. 62 (22), 6659–6666.
Morgan, R., da Silveira, W. A., Kelly, R. C., Overton, I., Allott, E. H., and Hardiman, G. (2021). Long Non-coding RNAs and Their Potential Impact on Diagnosis, Prognosis, and Therapy in Prostate Cancer: Racial, Ethnic, and Geographical Considerations. Expert Rev. Mol. Diagnostics 21 (12), 1257–1271. doi:10.1080/14737159.2021.1996227
Murata, M., and Kang, J.-H. (2018). Bisphenol A (BPA) and Cell Signaling Pathways. Biotechnol. Adv. 36 (1), 311–327. doi:10.1016/j.biotechadv.2017.12.002
Nakazawa, M., Paller, C., and Kyprianou, N. (2017). Mechanisms of Therapeutic Resistance in Prostate Cancer. Curr. Oncol. Rep. 19 (2), 13. doi:10.1007/s11912-017-0568-7
Ni, W., Yao, S., Zhou, Y., Liu, Y., Huang, P., Zhou, A., et al. (2019). Long Noncoding RNA GAS5 Inhibits Progression of Colorectal Cancer by Interacting with and Triggering YAP Phosphorylation and Degradation and Is Negatively Regulated by the m6A Reader YTHDF3. Mol. Cancer 18 (1), 143. doi:10.1186/s12943-019-1079-y
Nicola, N. A., and Babon, J. J. (2015). Leukemia Inhibitory Factor (LIF). Cytokine & Growth Factor Rev. 26 (5), 533–544. doi:10.1016/j.cytogfr.2015.07.001
Odero-Marah, V., Hawsawi, O., Henderson, V., and Sweeney, J. (2018). Epithelial-Mesenchymal Transition (EMT) and Prostate Cancer. Adv. Exp. Med. Biol. 1095, 101–110. doi:10.1007/978-3-319-95693-0_6
Oh, H.-H., and Joo, Y.-E. (2020). Novel Biomarkers for the Diagnosis and Prognosis of Colorectal Cancer. Intest. Res. 18 (2), 168–183. doi:10.5217/ir.2019.00080
Pan, J., Xu, X., and Wang, G. (2020). lncRNA ZFAS1 Is Involved in the Proliferation, Invasion and Metastasis of Prostate Cancer Cells Through Competitively Binding to miR-135a-5p. Cmar 12, 1135–1149. doi:10.2147/CMAR.S237439
Park, S.-A., Kim, M.-J., Park, S.-Y., Kim, J.-S., Lee, S.-J., Woo, H. A., et al. (2015). EW-7197 Inhibits Hepatic, Renal, and Pulmonary Fibrosis by Blocking TGF-β/Smad and ROS Signaling. Cell. Mol. Life Sci. 72 (10), 2023–2039. doi:10.1007/s00018-014-1798-6
Peinado, H., Olmeda, D., and Cano, A. (2007). Snail, Zeb and bHLH Factors in Tumour Progression: an Alliance against the Epithelial Phenotype? Nat. Rev. Cancer 7 (6), 415–428. doi:10.1038/nrc2131
Prensner, J. R., and Chinnaiyan, A. M. (2011). The Emergence of lncRNAs in Cancer Biology. Cancer Discov. 1 (5), 391–407. doi:10.1158/2159-8290.CD-11-0209
Ramnarine, V. R., Kobelev, M., Gibb, E. A., Nouri, M., Lin, D., Wang, Y., et al. (2019). The Evolution of Long Noncoding RNA Acceptance in Prostate Cancer Initiation, Progression, and its Clinical Utility in Disease Management. Eur. Urol. 76 (5), 546–559. doi:10.1016/j.eururo.2019.07.040
Ring, A., Kim, Y.-M., and Kahn, M. (2014). Wnt/catenin Signaling in Adult Stem Cell Physiology and Disease. Stem Cell Rev Rep 10 (4), 512–525. doi:10.1007/s12015-014-9515-2
Roca, H., Jones, J. D., Purica, M. C., Weidner, S., Koh, A. J., Kuo, R., et al. (2017). Apoptosis-induced CXCL5 Accelerates Inflammation and Growth of Prostate Tumor Metastases in Bone. J. Clin. Investigation 128 (1), 248–266. doi:10.1172/JCI92466
Saha, S., Kiran, M., Kuscu, C., Chatrath, A., Wotton, D., Mayo, M. W., et al. (2020). Long Noncoding RNA DRAIC Inhibits Prostate Cancer Progression by Interacting with IKK to Inhibit NF-κB Activation. Cancer Res. 80 (5), 950–963. doi:10.1158/0008-5472.CAN-19-3460
Sar, M., Lubahn, D. B., French, F. S., and Wilson, E. M. (1990). Immunohistochemical Localization of the Androgen Receptor in Rat and Human Tissues*. Endocrinology 127 (6), 3180–3186. doi:10.1210/endo-127-6-3180
Schroeder, A., Herrmann, A., Cherryholmes, G., Kowolik, C., Buettner, R., Pal, S., et al. (2014). Loss of Androgen Receptor Expression Promotes a Stem-like Cell Phenotype in Prostate Cancer through STAT3 Signaling. Cancer Res. 74 (4), 1227–1237. doi:10.1158/0008-5472.CAN-13-0594
Shang, Z., Yu, J., Sun, L., Tian, J., Zhu, S., Zhang, B., et al. (2019). LncRNA PCAT1 Activates AKT and NF-κB Signaling in Castration-Resistant Prostate Cancer by Regulating the PHLPP/FKBP51/IKKα Complex. Nucleic Acids Res. 47 (8), 4211–4225. doi:10.1093/nar/gkz108
Shangguan, L., Ti, X., Krause, U., Hai, B., Zhao, Y., Yang, Z., et al. (2012). Inhibition of TGF‐β/Smad Signaling by BAMBI Blocks Differentiation of Human Mesenchymal Stem Cells to Carcinoma‐Associated Fibroblasts and Abolishes Their Protumor Effects. Stem Cells 30 (12), 2810–2819. doi:10.1002/stem.1251
Shi, Z., Chen, J., Wumaner, A., Li, M., Liang, C., and Li, M. (2021). A Novel Long Non-coding RNA PCLN16 Facilitates Androgen Receptor Signaling in Prostate Cancer. Biochem. biophysical Res. Commun. 537, 78–84. doi:10.1016/j.bbrc.2020.12.043
Shore, N. D., Saad, F., Cookson, M. S., George, D. J., Saltzstein, D. R., Tutrone, R., et al. (2020). Oral Relugolix for Androgen-Deprivation Therapy in Advanced Prostate Cancer. N. Engl. J. Med. 382 (23), 2187–2196. doi:10.1056/NEJMoa2004325
Shorning, B. Y., Dass, M. S., Smalley, M. J., and Pearson, H. B. (2020). The PI3K-AKT-mTOR Pathway and Prostate Cancer: At the Crossroads of AR, MAPK, and WNT Signaling. Ijms 21 (12), 4507. doi:10.3390/ijms21124507
Siegel, D. A., O’Neil, M. E., Richards, T. B., Dowling, N. F., and Weir, H. K. (2020). Prostate Cancer Incidence and Survival, by Stage and Race/Ethnicity - United States, 2001-2017. MMWR Morb. Mortal. Wkly. Rep. 69 (41), 1473–1480. doi:10.15585/mmwr.mm6941a1
Siegel, R. L., Miller, K. D., Fuchs, H. E., and Jemal, A. (2021). Cancer Statistics, 2021. CA A Cancer J. Clin. 71 (1), 7–33. doi:10.3322/caac.21654
Siegel, R. L., Miller, K. D., Fuchs, H. E., and Jemal, A. (2022). Cancer Statistics, 2022. CA A Cancer J. Clin. 72 (1), 7–33. doi:10.3322/caac.21708
Subudhi, S. K., Vence, L., Zhao, H., Blando, J., Yadav, S. S., Xiong, Q., et al. (2020). Neoantigen Responses, Immune Correlates, and Favorable Outcomes after Ipilimumab Treatment of Patients with Prostate Cancer. Sci. Transl. Med. 12 (537), eaaz3577. doi:10.1126/scitranslmed.aaz3577
Sun, D., Li, H., Cao, M., He, S., Lei, L., Peng, J., et al. (2020). Cancer Burden in China: Trends, Risk Factors and Prevention. Cancer Biol. Med. 17 (4), 879–895. doi:10.20892/j.issn.2095-3941.2020.0387
Sun, W., Zu, S., Shao, G., Wang, W., and Gong, F. (2021). Long Non‐coding DANCR Targets miR‐185‐5p to Upregulate LIM and SH3 Protein 1 Promoting Prostate Cancer via the FAK/PI3K/AKT/GSK3β/snail Pathway. J. Gene Med. 23 (7), e3344. doi:10.1002/jgm.3344
Sung, H., Ferlay, J., Siegel, R. L., Laversanne, M., Soerjomataram, I., Jemal, A., et al. (2021). Global Cancer Statistics 2020: GLOBOCAN Estimates of Incidence and Mortality Worldwide for 36 Cancers in 185 Countries. CA A Cancer J. Clin. 71 (3), 209–249. doi:10.3322/caac.21660
Tan, X., Chen, W.-b., Lv, D.-j., Yang, T.-w., Wu, K.-h., Zou, L.-b., et al. (2021). LncRNA SNHG1 and RNA Binding Protein hnRNPL Form a Complex and Coregulate CDH1 to Boost the Growth and Metastasis of Prostate Cancer. Cell Death Dis. 12 (2), 138. doi:10.1038/s41419-021-03413-4
Tong, D., Liu, Q., Liu, G., Xu, J., Lan, W., Jiang, Y., et al. (2017). Metformin Inhibits Castration-Induced EMT in Prostate Cancer by Repressing COX2/PGE2/STAT3 axis. Cancer Lett. 389, 23–32. doi:10.1016/j.canlet.2016.12.031
Videira, A., Beckedorff, F. C., daSilva, L. F., and Verjovski-Almeida, S. (2021). PVT1 Signals an Androgen-dependent Transcriptional Repression Program in Prostate Cancer Cells and a Set of the Repressed Genes Predicts High-Risk Tumors. Cell Commun. Signal 19 (1), 5. doi:10.1186/s12964-020-00691-x
Wang, C.-Y., Mayo, M. W., and Baldwin, A. S. (1996). TNF- and Cancer Therapy-Induced Apoptosis: Potentiation by Inhibition of NF-κB. Science 274 (5288), 784–787. doi:10.1126/science.274.5288.784
Wang, T., Fahrmann, J. F., Lee, H., Li, Y.-J., Tripathi, S. C., Yue, C., et al. (2018). JAK/STAT3-Regulated Fatty Acid β-Oxidation Is Critical for Breast Cancer Stem Cell Self-Renewal and Chemoresistance. Cell Metab. 27 (1), 136–150. doi:10.1016/j.cmet.2017.11.001
Wang, Y., Wang, S., Ren, Y., and Zhou, X. (2020). The Role of lncRNA Crosstalk in Leading Cancer Metastasis of Head and Neck Squamous Cell Carcinoma. Front. Oncol., 10, 561833doi:doi:10.3389/fonc.2020.561833
Wang, Y. C., He, W. Y., Dong, C. H., Pei, L., and Ma, Y. L. (2019). lncRNA HCG11 Regulates Cell Progression by Targeting miR‐543 and Regulating AKT/mTOR Pathway in Prostate Cancer. Cell Biol. Int. 43, 1453–1462. doi:10.1002/cbin.11194
Watanabe, O., Imamura, H., Shimizu, T., Kinoshita, J., Okabe, T., Hirano, A., et al. (2004). Expression of Twist and Wnt in Human Breast Cancer. Anticancer Res. 24 (6), 3851–3856.
Wei, X., Hou, Y., Zhang, Y., Zhang, H., Sun, Z., Meng, X., et al. (2022). Long Non-coding RNA MAGI2-AS3 Inactivates STAT3 Pathway to Inhibit Prostate Cancer Cell Proliferation via Acting as a microRNA-424-5p Sponge. J. Cancer 13 (1), 343–353. doi:10.7150/jca.60749
Wen, S., Wei, Y., Zen, C., Xiong, W., Niu, Y., and Zhao, Y. (2020). Long Non-coding RNA NEAT1 Promotes Bone Metastasis of Prostate Cancer through N6-Methyladenosine. Mol. Cancer 19 (1), 171. doi:10.1186/s12943-020-01293-4
Wilusz, J. E., Sunwoo, H., and Spector, D. L. (2009). Long Noncoding RNAs: Functional Surprises from the RNA World. Genes Dev. 23 (13), 1494–1504. doi:10.1101/gad.1800909
Wu, G., Hao, C., Qi, X., Nie, J., Zhou, W., Huang, J., et al. (2020). LncRNA SNHG17 Aggravated Prostate Cancer Progression through Regulating its Homolog SNORA71B via a Positive Feedback Loop. Cell Death Dis. 11 (5), 393. doi:10.1038/s41419-020-2569-y
Wu, J., Cheng, G., Zhang, C., Zheng, Y., Xu, H., Yang, H., et al. (2017). Long Noncoding RNA LINC01296 Is Associated with Poor Prognosis in Prostate Cancer and Promotes Cancer-Cell Proliferation and Metastasis. Onco Targets Ther. 10, 1843–1852. doi:10.2147/OTT.S129928
Wu, J., Lv, Y., Li, Y., Jiang, Y., Wang, L., Zhang, X., et al. (2020). MCM3AP-AS1/miR-876-5p/WNT5A axis Regulates the Proliferation of Prostate Cancer Cells. Cancer Cell Int. 20, 307. doi:10.1186/s12935-020-01365-x
Wu, X., He, Y., Zhang, G., Wu, J., Hou, Y., Gu, Y., et al. (2018). Royleanone Diterpenoid Exhibits Potent Anticancer Effects in LNCaP Human Prostate Carcinoma Cells by Inducing Mitochondrial Mediated Apoptosis, Cell Cycle Arrest, Suppression of Cell Migration and Downregulation of mTOR/PI3K/AKT Signalling Pathway. J. BUON 23 (4), 1055–1060.
Xiao, S., and Song, B. (2020). LncRNA HOXA-AS2 Promotes the Progression of Prostate Cancer via Targeting miR-509-3p/PBX3 axis. Biosci. Rep. 40 (8). doi:10.1042/BSR20193287
Xie, W., Regan, M. M., Buyse, M., Halabi, S., Kantoff, P. W., Sartor, O., et al. (2020). Event-Free Survival, a Prostate-Specific Antigen-Based Composite End Point, Is Not a Surrogate for Overall Survival in Men with Localized Prostate Cancer Treated with Radiation. J. Clin. Oncol. 38 (26), 3032–3041. doi:10.1200/JCO.19.03114
Xu, S., Yi, X.-M., Tang, C.-P., Ge, J.-P., Zhang, Z.-Y., and Zhou, W.-Q. (2016). Long Non-coding RNA ATB Promotes Growth and Epithelial-Mesenchymal Transition and Predicts Poor Prognosis in Human Prostate Carcinoma. Oncol. Rep. 36 (1), 10–22. doi:10.3892/or.2016.4791
Xu, Y.-H., Deng, J.-L., Wang, G., and Zhu, Y.-S. (2019). Long Non-coding RNAs in Prostate Cancer: Functional Roles and Clinical Implications. Cancer Lett. 464, 37–55. doi:10.1016/j.canlet.2019.08.010
Xue, J., Lin, X., Chiu, W.-T., Chen, Y.-H., Yu, G., Liu, M., et al. (2014). Sustained Activation of SMAD3/SMAD4 by FOXM1 Promotes TGF-β-dependent Cancer Metastasis. J. Clin. Invest. 124 (2), 564–579. doi:10.1172/JCI71104
Yamamoto, Y., and Gaynor, R. (2001). Role of the NF-kB Pathway in the Pathogenesis of Human Disease States. Curr. Mol. Med. 1 (3), 287–296. doi:10.2174/1566524013363816
Yan, Y., and Huang, H. (2019). Interplay Among PI3K/AKT, PTEN/FOXO and AR Signaling in Prostate Cancer. Adv. Exp. Med. Biol. 1210, 319–331. doi:10.1007/978-3-030-32656-2_14
Yang, J., Li, C., Mudd, A., and Gu, X. (2017). LncRNA PVT1 Predicts Prognosis and Regulates Tumor Growth in Prostate Cancer. Biosci. Biotechnol. Biochem. 81 (12), 2301–2306. doi:10.1080/09168451.2017.1387048
Yao, M., Shi, X., Li, Y., Xiao, Y., Butler, W., Huang, Y., et al. (2020). LINC00675 Activates Androgen Receptor axis Signaling Pathway to Promote Castration-Resistant Prostate Cancer Progression. Cell Death Dis. 11 (8), 638. doi:10.1038/s41419-020-02856-5
Yeh, Y., Guo, Q., Connelly, Z., Cheng, S., Yang, S., Prieto-Dominguez, N., et al. (2019). Wnt/Beta-Catenin Signaling and Prostate Cancer Therapy Resistance. Adv. Exp. Med. Biol. 1210, 351–378. doi:10.1007/978-3-030-32656-2_16
Yoshida, G. J., and Saya, H. (2014). Inversed Relationship between CD44 Variant and C-Myc Due to Oxidative Stress-Induced Canonical Wnt Activation. Biochem. biophysical Res. Commun. 443 (2), 622–627. doi:10.1016/j.bbrc.2013.12.016
Yoshimoto, M., Ludkovski, O., DeGrace, D., Williams, J. L., Evans, A., Sircar, K., et al. (2012). PTEN Genomic Deletions that Characterize Aggressive Prostate Cancer Originate Close to Segmental Duplications. Genes Chromosom. Cancer 51 (2), 149–160. doi:10.1002/gcc.20939
Yu, B., and Shan, G. (2016). Functions of Long Noncoding RNAs in the Nucleus. Nucleus 7 (2), 155–166. doi:10.1080/19491034.2016.1179408
Zhang, A., Zhao, J. C., Kim, J., Fong, K.-w., Yang, Y. A., Chakravarti, D., et al. (2015). LncRNA HOTAIR Enhances the Androgen-Receptor-Mediated Transcriptional Program and Drives Castration-Resistant Prostate Cancer. Cell Rep. 13 (1), 209–221. doi:10.1016/j.celrep.2015.08.069
Zhang, B., Zhang, M., Shen, C., Liu, G., Zhang, F., Hou, J., et al. (2021). LncRNA PCBP1-AS1-mediated AR/AR-V7 Deubiquitination Enhances Prostate Cancer Enzalutamide Resistance. Cell Death Dis. 12 (10), 856. doi:10.1038/s41419-021-04144-2
Zhang, H., Meng, H., Huang, X., Tong, W., Liang, X., Li, J., et al. (2019). lncRNA MIR4435-2HG P-romotes C-ancer C-ell M-igration and I-nvasion in P-rostate C-arcinoma by U-pregulating TGF-β1. Oncol. Lett. 18 (4), 4016–4021. doi:10.3892/ol.2019.10757
Zhang, J., Li, X.-Y., Hu, P., and Ding, Y.-S. (2018). lncRNA NORAD Contributes to Colorectal Cancer Progression by Inhibition of miR-202-5p. Oncol. Res. 26 (9), 1411–1418. doi:10.3727/096504018X15190844870055
Zhang, M., Sun, Y., Huang, C.-P., Luo, J., Zhang, L., Meng, J., et al. (2021). Targeting the Lnc-OPHN1-5/androgen receptor/hnRNPA1 Complex Increases Enzalutamide Sensitivity to Better Suppress Prostate Cancer Progression. Cell Death Dis. 12 (10), 855. doi:10.1038/s41419-021-03966-4
Zhang, W., Shi, X., Chen, R., Zhu, Y., Peng, S., Chang, Y., et al. (2020). Novel Long Non-coding RNA lncAMPC Promotes Metastasis and Immunosuppression in Prostate Cancer by Stimulating LIF/LIFR Expression. Mol. Ther. 28 (11), 2473–2487. doi:10.1016/j.ymthe.2020.06.013
Zhang, Y., Zhang, J., Liang, S., Lang, G., Liu, G., Liu, P., et al. (2019). Long Non-coding RNA VIM-AS1 Promotes Prostate Cancer Growth and Invasion by Regulating Epithelial-Mesenchymal Transition. J. BUON 24 (5), 2090–2098.
Zhang, Y., Pitchiaya, S., Cieślik, M., Niknafs, Y. S., Tien, J. C.-Y., Hosono, Y., et al. (2018). Analysis of the Androgen Receptor-Regulated lncRNA Landscape Identifies a Role for ARLNC1 in Prostate Cancer Progression. Nat. Genet. 50 (6), 814–824. doi:10.1038/s41588-018-0120-1
Zhao, B., Lu, Y.-L., Yang, Y., Hu, L.-B., Bai, Y., Li, R.-Q., et al. (2018). Overexpression of lncRNA ANRIL Promoted the Proliferation and Migration of Prostate Cancer Cells via Regulating Let-7a/TGF-Β1/Smad Signaling Pathway. Cbm 21 (3), 613–620. doi:10.3233/CBM-170683
Zhao, J., Li, G., Wei, J., Dang, S., Yu, X., Ding, L., et al. (2020). Ellagic Acid Induces Cell Cycle Arrest and Apoptosis via the TGF-β1/Smad3 S-ignaling P-athway in H-uman C-olon C-ancer HCT-116 C-ells. Oncol. Rep. 44 (2), 768–776. doi:10.3892/or.2020.7617
Zhao, X., Liu, Y., Luo, C., and Zuo, Y. (2021). AGAP2-AS1/miR-628-5p/FOXP2 Feedback Loop Facilitates the Growth of Prostate Cancer via Activating WNT Pathway. Carcinogenesis 42, 1270–1280. doi:10.1093/carcin/bgab062
Zheng, J., Zhao, S., He, X., Zheng, Z., Bai, W., Duan, Y., et al. (2016). The Up-Regulation of Long Non-coding RNA CCAT2 Indicates a Poor Prognosis for Prostate Cancer and Promotes Metastasis by Affecting Epithelial-Mesenchymal Transition. Biochem. Biophysical Res. Commun. 480 (4), 508–514. doi:10.1016/j.bbrc.2016.08.120
Zheng, P., Li, H., Xu, P., Wang, X., Shi, Z., Han, Q., et al. (2018). High lncRNA HULC Expression Is Associated with Poor Prognosis and Promotes Tumor Progression by Regulating Epithelial-Mesenchymal Transition in Prostate Cancer. Arch. Med. Sci. 14 (3), 679–686. doi:10.5114/aoms.2017.69147
Zheng, T., Ma, G., Tang, M., Li, Z., and Xu, R. (2018). IL-8 Secreted from M2 Macrophages Promoted Prostate Tumorigenesis via STAT3/MALAT1 Pathway. Ijms 20 (1), 98. doi:10.3390/ijms20010098
Keywords: prostate cancer (PCa), long non-coding RNA (lncRNA), epithelial-mesenchymal transition (EMT), androgen receptor (AR), wnt/β-catenin
Citation: Shen D, Peng H, Xia C, Deng Z, Tong X, Wang G and Qian K (2022) The Role of Long Non-Coding RNAs in Epithelial-Mesenchymal Transition-Related Signaling Pathways in Prostate Cancer. Front. Mol. Biosci. 9:939070. doi: 10.3389/fmolb.2022.939070
Received: 08 May 2022; Accepted: 10 June 2022;
Published: 18 July 2022.
Edited by:
Rui Cao, Capital Medical University, ChinaCopyright © 2022 Shen, Peng, Xia, Deng, Tong, Wang and Qian. This is an open-access article distributed under the terms of the Creative Commons Attribution License (CC BY). The use, distribution or reproduction in other forums is permitted, provided the original author(s) and the copyright owner(s) are credited and that the original publication in this journal is cited, in accordance with accepted academic practice. No use, distribution or reproduction is permitted which does not comply with these terms.
*Correspondence: Gang Wang, gangwang.uro@whu.edu.cn; Kaiyu Qian, qky1009@whu.edu.cn
†These authors have contributed equally to this work