- Department of Pulmonary and Critical Care Medicine, Peking University Third Hospital, Beijing, China
Chronic obstructive pulmonary disease (COPD) is a common respiratory disease that brings about great social and economic burden, with oxidative stress and inflammation affecting the whole disease progress. Sulfur compounds such as hydrogen sulfide (H2S), thiols, and persulfides/polysulfides have intrinsic antioxidant and anti-inflammatory ability, which is engaged in the pathophysiological process of COPD. Hydrogen sulfide mainly exhibits its function by S-sulfidation of the cysteine residue of the targeted proteins. It also interacts with nitric oxide and acts as a potential biomarker for the COPD phenotype. Thiols’ redox buffer such as the glutathione redox couple is a major non-enzymatic redox buffer reflecting the oxidative stress in the organism. The disturbance of redox buffers was often detected in patients with COPD, and redressing the balance could delay COPD exacerbation. Sulfane sulfur refers to a divalent sulfur atom bonded with another sulfur atom. Among them, persulfides and polysulfides have an evolutionarily conserved modification with antiaging effects. Sulfur compounds and their relative signaling pathways are also associated with the development of comorbidities in COPD. Synthetic compounds which can release H2S and persulfides in the organism have gradually been developed. Naturally extracted sulfur compounds with pharmacological effects also aroused great interest. This study discussed the biological functions and mechanisms of sulfur compounds in regulating COPD and its comorbidities.
Introduction
Chronic obstructive pulmonary disease (COPD) was the third leading cause of death worldwide in 2019, causing the death of 3.23 million people, which was 6% of the total death (WHO, 2019). According to a large, nationally representative cross section of adults ≥ 40 years old, the estimated standardized prevalence of COPD among the Chinese population was 13.6% (95% CI 12.0–15.2) (Fang et al., 2018). For adults ≥20 years old, the overall prevalence was 8.6% (95% CI 7.5–9.9) (Wang et al., 2018). Meanwhile, COPD was the third leading cause of disability-adjusted life-years lost in China in 2017 (Zhou et al., 2019). Moreover, driven by the aging of the Chinese population, the morbidity of COPD is expected to increase accompanied with increasing economic and social burden. Patients with COPD suffer from irreversible bronchi obstruction; however, the current therapeutic strategies, including bronchodilators, antimuscarinic drugs, methylxanthines, and corticosteroids, were adopted to alleviate the symptoms but have little effect on delaying the disease progress. Innovative drugs that can delay the disease’s progress and ideally have the potential to cure the disease are extremely needed.
Sulfur compounds include hydrogen sulfide (H2S), sulfur dioxide (SO2), organic sulfur, sulfate, and elemental sulfur, which were widely distributed in the outer environment and in organisms. For centuries, scientists believed that these compounds were responsible for damage to our environment and for causing respiratory diseases. For example, H2S and SO2 are well-known air pollutants, the high concentrations of which can cause irritation of the respiratory system, resulting in coughing, throat irritation, and shortness of breath. Sulfur mustard is an alkylating compound used as a chemical warfare agent, whose exposure led to long-term respiratory effects with several features resembling those of COPD, including chronic bronchitis, bronchial hyper-responsiveness, dyspnea with respiratory failure in advanced stages, and predominance of neutrophils in bronchoalveolar lavage fluid (Sahebkar et al., 2015).
In current years, people gave more attention to the biochemical role of sulfur compounds in the physical and pathological processes beyond the toxic effects. Sulfur is integral to the origin of life. They created an essential redox gradient that allows life to survive and evolve (Olson, 2021). The sulfur atom, with six valence electrons, can change its oxidation states from -2 to +6, thus being able to react with various nucleophiles and electrophiles to form a variety of molecular arrangements and exhibit diverse biological functions (Szabo, 2018). For COPD, oxidative stress, chronic inflammation, and protease–antiprotease imbalance are three major pathogenetic factors, of which, oxidative stress can be an initiator and amplifier for respiratory inflammation (Barnes, 2016). Reduced sulfur compounds, such as H2S, thiols, and sulfate sulfur (e.g., per/polysulfides), which have intrinsic antioxidant capabilities, may contribute to alleviating COPD symptoms as well as preventing COPD exacerbation (Rahman, 2012; Rahman and MacNee, 2012). Complex sulfur redox regulators, in particular, glutathione (GSH) redox buffers, sulfur-regulating enzymes superoxide dismutase, catalase, electron-conducting sulfur, and seleneosulfur enzymes, including thioredoxin, glutaredoxin, and peroxiredoxin systems, were essential in maintaining normal bioactivity in living cells (Olson, 2021). Sulfur compounds also modify bioactivity through post-transcriptional modifications, for example, S-glycosylation, S-palmitoylation, S-nitrosylation, and S-sulfidation of the cysteine residues in proteins (Ramazi and Zahiri, 2021). In addition, many pharmaceuticals consist of sulfur subunits, including mucolytic drugs, antibacterial, anti-inflammatories, antihypertensive drugs, analgesics, and anticancer agents. The effect of these sulfur compounds on the development and treatment of COPD is discussed herein.
Hydrogen sulfide
H2S is the an endogenous gas transmitter, along with nitric oxide and carbon monoxide. Endogenous H2S can be synthesized via enzymatic and non-enzymatic pathways. The enzymatic pathway is mediated by cystathionine-γ-lyase (CSE), cystathionine-β-synthase (CBS), and mercaptopyruvate sulfurtransferase (MST) (Li et al., 2011) (Figure 1). The non-enzymatic pathway includes the reduction of elemental sulfur to H2S using reducing equivalents obtained from the oxidation of glucose (Wang, 2002). Intracellular sulfane sulfur also formed an H2S storage pool. CSE and CBS are located in the cytosol. When faced with stimuli, they can translocate to mitochondria to enhance H2S production (Fu et al., 2012; Teng et al., 2013). MST is combined with cysteine aminotransferase (CAT) to synthesis H2S in mitochondria. In the human lung tissue, they are expressed in the alveolar cells and endothelial cells based on the Protein Atlas Database (Olson et al., 2010; Pacitti et al., 2021). The deprivation of H2S is mediated by sulfide quinone reductase (SQR) in the mitochondria, where H2S was oxidized into sulfate and finally removed from the kidney (Libiad et al., 2019; Landry et al., 2021). H2S can also be directly exhaled via the airway.
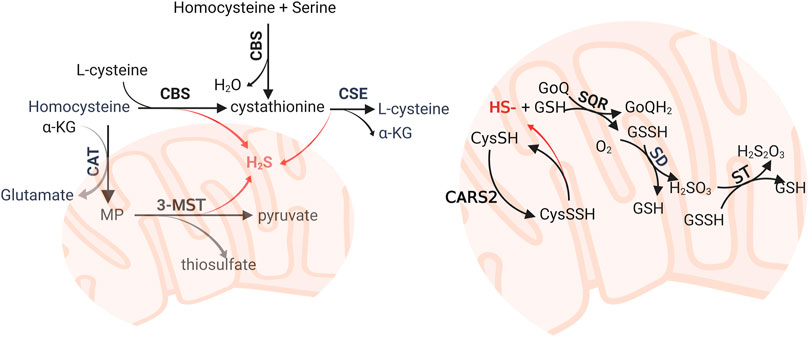
FIGURE 1. Enzymatic biosynthesis and oxidative pathway of hydrogen sulfide (H2S). The biosynthesis of H2S was mediated by CBS, CSE, and 3-MST. The major substrates are homocysteine and L-cysteine. Persulfide and polysulfides reduced in mitochondria also generate H2S. H2S can be oxidized by SQR, SD, and ST, which are mitochondrial inner membrane-anchored enzymes, to form sulfate and deprived from the organism. CBS: cystathionine β-synthase; CSE, cystathionine γ-lyase; CAT, cysteine aminotransferase; CoQ, coenzyme Q; CARS, cysteinyl-tRNA synthetases; 3-MST, 3-mercaptopyruvate; MST, 3-mercaptopyruvate sulfurtransferase; α-KG, α-ketoglutarate; SQR, sulfide quinone reductase; SD, sulfur dioxygenase; ST, sulfur transferase.
Impaired lung growth during gestation and childhood would reduce maximal attained lung function, which puts individuals at risk of developing COPD (Stern et al., 2007). Disturbing of CBS and CSE expression led to muscularization of small- and medium-sized lung vessels and incomplete lung alveolarization during fetal lung development, while exogenous introduced H2S improved alveolarization (Madurga et al., 2015). CSE was downregulated in smokers and COPD patients, but CBS mRNA transcript was increased in smokers and decreased in COPD patients when compared with healthy controls (Sun et al., 2015). H2S can be a valuable biomarker indicating the development of COPD and reflecting the disease states. Endogenous H2S can be detected in blood, sputum, and exhaled gas. Serum H2S was increased in patients with stable COPD when compared to healthy controls and acute exacerbation of COPD (AECOPD), and its concentration was positively correlated with the lung function of COPD patients (Chen et al., 2005). Low serum H2S was associated with respiratory tract infection, whose receiver operating characteristic curve for predicting the need for antibiotic treatment for COPD patients was 0.862 (Chen et al., 2009). H2S is involved in vascular remodeling in COPD, and its concentration was negatively correlated with indexes like main pulmonary artery diameter on HRCT, those indirectly reflecting the pulmonary artery tension (Liao et al., 2021). However, serum H2S was affected by systemic metabolism; for example, H2S is synthesized by bacteria in the gut or released from other organs, thus being less specific to identifying lung diseases. H2S in sputum was considered to more closely reflect lung diseases. An increase of the sputum-to-serum ratio of H2S was found among AECOPD subjects (Saito et al., 2014). Moreover, the ratio is positively associated with sputum neutrophils, both in COPD and in asthma (Saito et al., 2014; Suzuki et al., 2018). Exhaled H2S is a valuable clue to respiratory diseases since it changes dynamically with disease states and can be measured noninvasively. Current studies did not find the exhaled H2S differing significantly from stable COPD, AECOPD, and healthy groups. However, its concentration was negatively correlated with induced sputum eosinophils, thus being regarded as a biomarker that indicates a non-eosinophilic disease phenotype (Sun et al., 2013; Zhang et al., 2015). However, the result was not very conclusive due to the limited sample size. In addition, this study collected the exhaled H2S using reservoir bags to carry out off-line detection, which was not very competent in identifying the real-time changes. As a result, a large-scale, on-line survey of exhaled H2S among COPD patients and healthy controls is still needed to eliminate numerous confounding factors affecting H2S exhalation.
Hydrogen sulfide is a novel gasotransmitter involved in a variety of bioactivities, such as vasodilation, antioxidant, anti-inflammation, mesenchymal transition, cell senescence, and apoptosis. H2S is a vasodilator, and its consumption may be O2-dependent as a mediator of the hypoxic pulmonary vessel constriction (Olson et al., 2010). Apart from being a vasodilator, the anti-inflammation and antioxidant effects of endogenous H2S have been well-documented, both in COPD and other diseases (Bhatia, 2012). (Figure 2) Exogenously introduced NaHS, a commonly adopted H2S donor, can protect against cigarette smoke-induced oxidative stress by promoting Akt phosphorylation. Consequently, the antioxidant transcription factor Nrf2 was upregulated, which otherwise would be inhibited by cigarette smoke. The pathological manifestation was also improved in the mouse model, with ameliorated bronchial remodeling, lung emphysema, and pulmonary hypertension (Han et al., 2011). The protective effect of H2S against particulate matter-induced emphysema and airway inflammation is exhibited via the Nrf2-dependent pathway through suppressing PYD domain-containing protein 3 (NLRP3) inflammasome formation and apoptosis (Jia et al., 2020). The H2S donor, GYY4137, promoted the production of GSH and superoxide dismutase and inhibited the release of inflammatory factors like TNF-α and IL-8 (Sun et al., 2015). H2S inhibited the transform growth factor beta-1 (TGF-β1)/Smads signaling pathway, which was associated with pulmonary fibrogenesis and airway remodeling of COPD (Jerzy et al., 2009; Liao et al., 2015; Wang et al., 2020). Airway epithelial–mesenchymal transition (EMT) is a highly plastic process through which epithelial cells change into a mesenchymal phenotype following epithelial damage. In human bronchial epithelial cells, NaHS treatment upregulated sirtuin 1 expression, which modified TGF-β1-mediated Smad3 transactivation, and then cigarette smoke extract-induced EMT, collagen deposition, and oxidative stress was reduced (Bhatia, 2015; Guan et al., 2020a). As a nicotinamide adenine dinucleotide (NAD+)-dependent histone deacetylase, sirtuin 1 plays a central role in cell senescence and aging (Xu et al., 2020). H2S upregulated sirtuin 1 expression, which improved mitochondrial function and reduced oxidative stress; thus, cell senescence induced by cigarette smoke was attenuated (Guan et al., 2019). Hydrogen sulfide also inhibits EMT by regulating endoplasm reticulum stress (ERS) (Lin et al., 2022). With ERS markers such as glucose-regulated protein-78 (GRP78), C/EBP homologous protein (CHOP), and caspase-12 were reduced in the COPD rat model that was established by passive smoke exposure and lipopolysaccharide irritation, pulmonary artery endothelial cell apoptosis was decreased (Ding et al., 2018). In a cigarette smoke-exposed rat model, H2S also inhibited ERS and apoptosis in bronchial epithelial cells (Lin et al., 2017). NaHS prevented emphysema via the suppression of the PHD2/HIF-1α/MAPK signaling pathway, and subsequently inhibition of inflammation, epithelial cell injury, and apoptosis (Guan et al., 2020b). Apart from cigarette smoke-induced COPD, H2S also alleviates lung emphysema induced by other etiologies. For example, NaHS administration prevented and partially reversed ozone-induced features of lung inflammation and emphysema via the regulation of the NLRP3-caspase-1, p38 MAPK, and Akt pathways (Li et al., 2016). A recent study found that H2S attenuates cigarette smoke-induced pyroptosis through inhibition of TLR4/NF-κB signaling (Wang L. et al., 2022). Ferroptosis was found to be elevated in COPD. Administration of NaHS can alleviate particulate matter-induced emphysema and airway inflammation by suppressing ferroptosis via PPAR-γ/Nrf2 signaling (Wang Y et al., 2022).
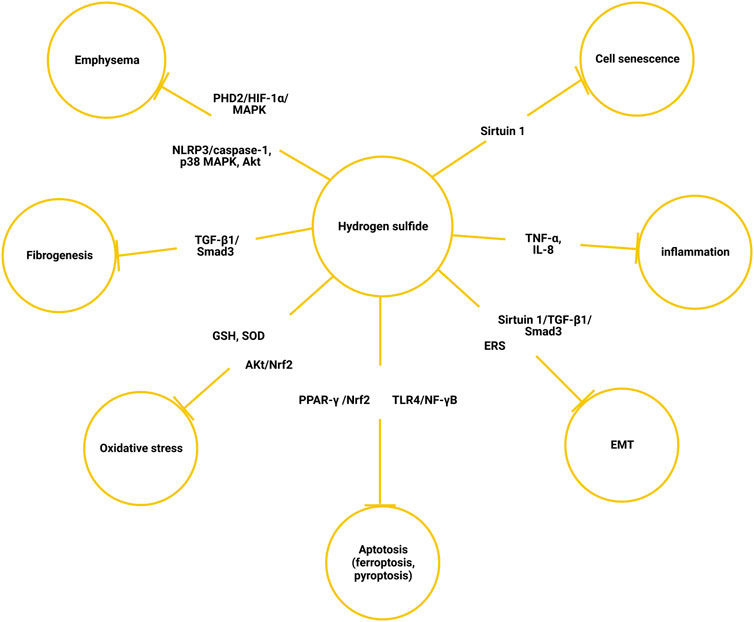
FIGURE 2. Mechanism of hydrogen sulfide alleviating chronic obstructive pulmonary disease. EMT, epithelial–mesenchymal transition; ERS, endoplasmic reticulum stress; SOD, superoxide dismutase.
Researchers usually introduced H2S to an animal model simultaneously or before harmful factors like cigarette smoking, lipopolysaccharide, and ozone exposure was introduced so that the preventive effect of H2S on the development of COPD was fully confirmed. However, its therapeutic potential to reverse bronchial remodeling or airway obstruction was still uncertain. One research showed that H2S inhibited phosphorylation of the MAPKs, extracellular signal-regulated kinase (ERK)-1/2, and p38 to regulate airway smooth muscle cell proliferation and cytokine release (IL-6 and CXCL8) among nonsmokers. However, in cell lines obtained from COPD patients, these protective processes were disturbed (Perry et al., 2018). As a result, whether exogenous administration of H2S can delay the disease progress after COPD was exhibited was under doubt. Moreover, most of the studies used exogenous H2S donors as an intervention in the biosystem, but the concentration of H2S generated from H2S donors is much higher than its physiological concentration (Pacitti et al., 2021). The huge gap between the level of exogenous introduced H2S and the endogenous circulated H2S raised a concern whether endogenous H2S has similar bioactivity with the exogenous introduced H2S.
Thiol-based family
Thiols are organosulfur compounds with the general formula R-SH, where R represents an alkyl or other organic substituent. They can be oxidized by reactive oxygen species (ROS) to form disulfide or sulfate. Thiols’ redox states reflect the oxidative stress in the organism, of which the GSH redox couple is the major non-enzymatic redox buffer that accounts for approximately 90% of intracellular small molecular thiols (Heffner and Repine, 1989; Sotgia et al., 2020). The disturbance of the redox state was observed in a variety of respiratory diseases, such as cystic fibrosis, acute respiratory distress syndrome, asthma, and COPD (Rahman and MacNee, 1999; Zinellu et al., 2016). In patients with COPD, increased oxidative stress and reduced GSH have been found in biofluids like exhaled breath condensate, sputum, and blood (van der Toorn et al., 2007). Epithelial lining fluid is an aqueous continuous layer with high GSH concentration. It covers the mucus of the airway and alveoli to defend against exogenous oxidants that are contained in tobacco and air pollution. The total GSH in the epithelial lining fluid is up to 140-fold higher than that in plasma such that 90% of them are in reduced form (Cantin et al., 1987). Smoke induced a rapid decline of GSH, but chronic exposure led to increasing GSH in the lung epithelial lining fluid to mount a protective response (Gould et al., 2011). Aging eliminated the adaptive response from GSH when compared to younger controls (Gould et al., 2010). In addition, the lowered plasma cysteine/cystine ratio of COPD is correlated with the extent of loss of lung function (Watson et al., 2019). It was reported that cigarette smoking can deplete the total GSH pool by oxidizing GSH to nonreducible GSH-aldehyde derivatives (van der Toorn et al., 2007). According to a meta-analysis that involved 18 studies with 974 COPD patients and 631 healthy groups, the pooled reduced GSH in blood was significantly lower in COPD than in controls, although the total GSH concentrations were increased (Sotgia et al., 2020). However, another meta-analysis including 14 articles of 902 COPD patients and 660 controls reported that the total GSH was not significantly different between patients and controls, and pooled reduced GSH concentrations showed either a significant or nonsignificant difference depending on whether the mean concentrations of reduced GSH in controls were correctly within the accepted normal range (0.5–5.0 umol/L) (Sotgia et al., 2021). Methodological factors vastly affect the measurement of GSH. For instance, reduced GSH can undergo autoxidation in vitro and present an artificial oxidative state. Also, some studies falsely measured reduced GSH by spectrophotometric methods using Ellman’s reagent because this reagent reacted with sulfhydryl compounds rather than specifically to reduced GSH (39). In fact, many small-molecular thiols like cysteine and homocysteine can react with oxidants and are functionally close to GSH; therefore, a comprehensive redox state analysis of thiols may be better in the evaluation of systemic oxidative stress in COPD.
N-acetylcysteine, carbocysteine, and erdosteine are thiol-based mucolytic drugs. N-acetylcysteine contains a free SH group. Carbocysteine and erdosteine are thiol derivatives that can produce sulfhydryl compounds via metabolization. They disturbed the disulfide bonds in proteins to decrease the viscosity and elasticity of the mucus (Cazzola et al., 2020). N-acetylcysteine can replenish reduced GSH shortage by supplementing cysteine via deacetylate in the gastrointestinal tract, and cysteine is the rate-limiting substrate for GSH synthesis (Atkuri et al., 2007; Rahman and MacNee, 2012). Apart from mucolytic activity, multiple pharmacological activities of these drugs have been documented (Cazzola et al., 2019; Cazzola et al., 2020). Thiol-based drugs can directly scavenge reactive oxidative species and reactive nitrogen species via reducing equivalent free SH group and indirectly suppress the oxidative stress via modulating neurokinin A levels. The anti-inflammatory effects were exhibited by their reducing the synthesis and release of cytokines, proteinases, and proinflammatory factors, as well as inhibiting neurogenic inflammation. They can also reduce bacterial adhesion to the airway epithelial cells and disturb biofilm formation, thus being auxiliary drugs to improve antibiotic activity. Moreover, thiol-based drugs can even regulate the tone of airway smooth muscles in human bronchi. Carbocysteine can restore steroid sensitivity by increasing histone deacetylase 2 expression in a thiol/GGSH-dependent manner (Song et al., 2015; Song et al., 2019). Thiols regulated autophagy augmentation, whose impairment led to emphysema of COPD (Vij et al., 2018; Bodas et al., 2019).
Traditionally, mucolytic drugs were used during COPD exacerbation at low dosage and for a short period of time. Recently, some evidence indicated that using these drugs in high dosage and in the long term can prevent COPD progression and exacerbation. Bridgeman et al. (1994) reported a dose-dependent effect of N-acetylcysteine administration on plasma GSH in COPD. N-acetylcysteine did not increase the plasma GSH at 600 mg daily until 600 mg, three times a day. Regular usage of N-acetylcysteine (1200 mg daily) can reduce COPD exacerbations, especially for patients with heavy smoking history and for those who did not receive ICS treatments (Papi et al., 2019). A meta-analysis included seven randomized clinical trials with 2,753 patients confirmed the safety and efficacy of regular using mucolytic drugs in COPD (Rogliani et al., 2019). Erdosteine (600 mg/day), carbocysteine (1500 mg/day), and N-acetylcysteine (1200 mg/day) can reduce the risk of exacerbation and hospitalization with few adverse events. The ranks of effectiveness were erdosteine > carbocysteine > N-acetylcysteine. Erdosteine even prevented mild exacerbation, irrespective of concurrent ICS treatment. However, the latest Global Initiative for Chronic Obstructive Lung Disease strategy’s approach (GOLD, 2022) did not suggest antioxidant mucolytic drugs being used constitutively in the management of stable COPD (GOLD, 2022). More evidences were needed to identify the selected subjects who would benefit from this strategy. The benefits and defects of long-term treatment of mucolytic drugs among patients with COPD should be further explored.
Sulfane sulfur
Sulfane sulfur refers to a divalent sulfur atom bonded with another sulfur, such as inorganic (H2Sn) or organic persulfides (RSnH or RSnR, n = 2) and polysulfides (n = 3–8). It also includes disulfides where the C–S bond is adjacent to an unsaturated bond, for example, C=C or C=O, because the unsaturated bond near the C-S can tautomerize to a thiosulfoxide that contains a sulfur atom with six electrons (Iciek et al., 2001). Unlike thiols that can only act as reductants, sulfane sulfur is both nucleophile and electrophile, which can react with a variety of compounds to exert its biological activities. The numerous intracellular sulfane sulfur compounds formed an H2S storage pool to maintain a reduced environment in the cytoplasm. Persulfide/polysulfide derives from H2S oxidation or reacts with nitric oxide (NO) (Figure 3) (Ida et al., 2014). CBS and CSE catalyze CysSSH biosynthesis using cystine (CysSSCys) as a substrate, and they play a role in the trans-sulfuration pathway along with rhodanese (Koj and Frendo, 1962; Iciek et al., 2019). However, some evidences showed that CSE and CBS do not contribute directly to persulfide production but may promote the biosynthesis of cysteine and its supply to cysteinyl-tRNA synthetases (CARS) (Akaike et al., 2017). CARS can incorporate CysSSH into the protein during translation. In addition to their canonical role in protein translation, CARS also act as the principal cysteine persulfide synthases in vivo, which catalyzed both low-molecular-weight polysulfides and polysulfidated proteins. Notably, CARS2, a mitochondrial isoform of CARS, is involved in mitochondrial biogenesis and bioenergetics via CysSSH production, and the cysteine persulfide and polysulfides that are generated by CARS2 were important resources for production of H2S.
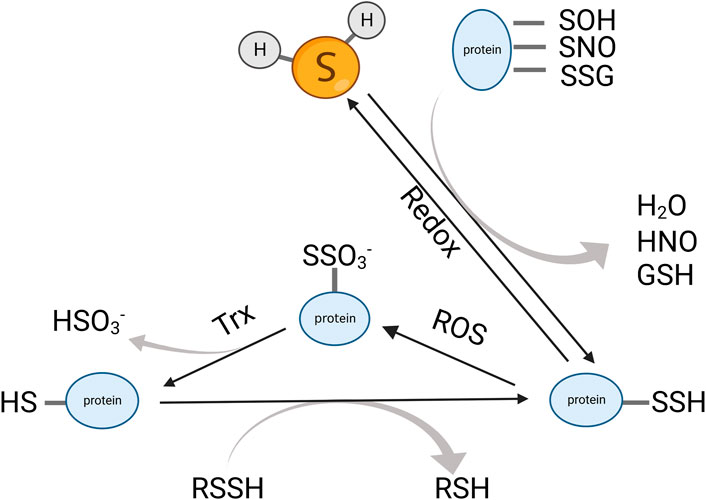
FIGURE 3. Dynamic interchange between protein persulfides, protein thiols, and H2S. H2S can react with an oxidized cysteine residue in protein (e.g., -SOH, -SNO, and -SSG) to generate protein persulfides. The protein persulfides can be oxidized by ROS and subsequently reduced by Trx to form thiols. The protein thiols can also transform into protein persulfides via trans-sulfidation. Trx, thioredoxin; ROS, reactive oxygen species.
Protein persulfidation is a recently recognized posttranslational modification. Sulfhydration can protect the proteins from oxidation and keep them in a more stable state. It usually enhances protein activity. Protein persulfidation was a major way that H2S exhibited its biological function. In 2009, Asif K. Mustafa reported that H2S can directly sulfhydrate the cysteine residue in GAPDH (Mustafa et al., 2009). After that, other target proteins of H2S with the cysteine residue have gradually been reported, such as Keap1, sirtuin 1, and ATP5A (Yang et al., 2013; Modis et al., 2016; Du et al., 2019). However, direct sulfhydration cannot be observed in a cell-free system. Some suppose that H2S cannot directly react with proteins that contain cysteine residues. This posttranslational modification is initiated by H2S reacting to oxidized protein thiols or protein thiols reacting to reactive sulfur species generated from oxidized H2S (Stubbert et al., 2014; Beltowski, 2015). Other studies found that H2S can break down disulfide bonds in proteins to exert biological function. For example, VEGFR2, insulin receptor, and EGFR underwent a disulfide bond molecular switch when reacted with H2S (Tao et al., 2013; Xue et al., 2013; Ge et al., 2014).
Protein persulfidation has been widely studied in the cardiovascular system, gastrointestinal system, neuron system, and immune system. However, few studies revealed the role of persulfidation relative to COPD. Persulfidation was confirmed to be an evolutionarily conserved modification with antiaging effects, and aging is a major risk factor for COPD. Zivanovic et al. (2019) revealed that the overall persulfidation increased in the early developmental stage, then hit a plateau, and finally declined in the late stage of growth, and this process was irrespective of species specificity. Increased persulfidation was associated with increased longevity and improved capacity to cope with stress stimuli. Furthermore, the level of persulfides and polysulfides as GSSH, CySSH, and GSSSH decreased in the epithelial lining fluid and primary lung cells of the patients with COPD when compared to healthy controls (Numakura et al., 2017). The reactive persulfides and polysulfide decreased significantly among patients with asthma-COPD overlap disease, when compared to the asthma group and healthy controls (Kyogoku et al., 2019). However, the persulfidation signaling pathway that is specifically related to COPD pathophysiology was not fully recognized, and more research is needed to identify the specific persulfidation signaling pathway involved in COPD.
Assessment of sulfur compounds
Accurate identification and assessment of sulfur compounds are the foundation for analyzing their biological functions. However, it is not simple as the sulfur compounds are under dynamic changes, so the measurement reflects a cross-section of the transient changes. Some of them have similar biochemical reactivities, which cause false-positive or false-negative results. Moreover, H2S would be auto-oxidized when it was exposed to oxidants in the air so that the precision of the result is affected by decay between the time of blood collection and sulfide measurement. There were many strategies for H2S detection, including spectroscopic, chromatographic, and electrochemical methods (Ibrahim et al., 2021). Each has advantages and limitations regarding the sample to be measured. For example, the methylene blue method was associated with strong acidic conditions, while H2S will be released from sulfane sulfur and acid-labile sulfur pool under the influence of reductants or strong acids, therefore resulting in overestimated H2S concentration (Li and Lancaster, 2013). The gas-sensing electrode is an electrochemical method that can detect sulfur ions with a sensitivity of 1–100 lM, but it is only sensitive to dissociated S2-, which is present under alkali and oxidation-free conditions (Cao et al., 2019). However, the alkaline conditions of the antioxidant buffer induce the lability of protein sulfur and produce artificially elevated sulfide values (Olson, 2009). An alternative method for electrochemical measurement of sulfide was the polarographic H2S sensor (Doeller et al., 2005; Whitfield et al., 2008). It permits real-time measurement of H2S gas in biological fluids without sample modification, while dissolved H2S (HS− and S2-) is estimated indirectly within the knowledge of pH and PKa (Whitfield et al., 2008). Fluorogenic probes can be adopted to visualize relative changes in H2S concentration in vivo, with a high spatiotemporal resolution of signals at the cellular and organelle level (Yu et al., 2013; Ibrahim et al., 2021). However, the results were diverse across imaging techniques and tissue autofluorescence and affected by different rates of uptake and retention of fluoroscopy dye across cell types, which makes it incomparable between different studies (Pacitti et al., 2021). Chromatography is a useful method for H2S detection with high sensitivity and specificity. It includes gas chromatography coupled to detectors as electrochemical, electron capture, flame photometry, mass spectrometry, ion mobility spectrometry, and liquid chromatography coupled to detectors as spectrophotometry, spectrofluorimetric, atomic fluorescence spectrometry, mass spectrometry, and electrochemical (Ibrahim et al., 2021). Chromatography can distinguish sulfide in different biochemical forms, including acid-labile sulfur and free and bound sulfane pools. With different strategies being applied, the physiological level of H2S in the tissue has not reached a consensus, with specifically some reported at the level of μM, and some confirmed to be at the level of nM (Furne et al., 2008; Whitfield et al., 2008).
The methods for detection of S-sulfhydration were continued to be modified. The Modified Biotin Switch Assay was derived from a nitrosylation assessment. The technology uses thiol-blocking agents, e.g., S-methyl methanethiosulfonate (MMTS) and S-4-bromobenzyl methanethiosulfonate (BBMTS), to block free thiols in the first step and then label the unreacted persulfide with N-[6-(biotinamido) hexyl]-3’-(2′-pyridyldithio) propionamide (biotin-HPDP). Finally, biotinylated protein was immunoprecipitated by Western blotting (Mustafa et al., 2009; Pan and Carroll, 2013). However, thiols have similar reactivity to MMTS with the persulfide group, which causes overestimation of persulfides. Another route is to block both thiols and persulfides with electrophiles (e.g., iodoacetic acid, IAP) and then use DDT to reduce persulfides and relabel them with biotinylated IAP (Krishnan et al., 2011). However, the specificity is under doubt because other oxidized cysteines such as disulfide bonds, sulfenic acids, and nitrosothiols can also be reduced by DDT (Krishnan et al., 2011; Pan and Carroll, 2013). The biotin thiol assay (BTA) labeled the reactive -SH and -SSH with a biotin-conjugated maleimide, and then they were bound on an avidin column. Finally, DTT was adopted to elute the retained proteins that contain a persulfide bridge (Gao et al., 2015). Protein persulfide being pulled down can be further analyzed by Western blot. The ProPerDP method has a similar mechanism as the BTA method (Doka et al., 2016). The tag switch assay first uses methylsulfonyl benzothiazole (MSBT) to block both -SH and -SSH, while the adducts resulting from persulfides are disulfides that can react with carbon-based nucleophiles (Park et al., 2015). Therefore, a cyanoacetate-based reagent CN-biotin can be introduced to be a “switch tag.” Recently, a dimedone-based probe switching method has been reported with high chemo-selectivity (Zivanovic et al., 2019). First, 4-chloro-7-nitrobenzofurazan (NBF-Cl) was applied to label persulfides, thiols, sulfenic acids, and amino groups and transform persulfides into mixed aromatic disulfides. Next, the NBF tag on persulfides is selectively switched by a dimedone-based probe. This method can enable both proteomic analysis and intracellular visualization of persulfides. Many fluorescent probes selectively reporting -SSH have been developed for detecting persulfides, poysulfides, and elemental sulfur (Chen et al., 2013; Takano et al., 2016; Bibli et al., 2018; Meng et al., 2018; Neill et al., 2019; Ran et al., 2019). Resonance synchronous spectroscopy (RS2) has been used to detect reactive sulfane sulfur, which displayed species-specific RS2 spectra (Li et al., 2019). The protonated form of persulfide (RSSH) was electrophilic and produced an RS2 signal, while RSS- was nucleophilic with no RS2 signal. Both the fluorescent probes and the resonance synchronous spectroscopy method enable quantitative measurement of reactive sulfane sulfur inside the cell or in the cellular subcompartment with high sensitivity and specificity. However, the major defect is that they do not allow qualitative detection of the sulfane sulfurs.
Cross-talk with nitric oxide
Airway inflammation is an important characterization for the COPD phenotype. Patients with COPD and airway eosinophilia were more sensitive to corticosteroid therapy than patients with non-eosinophilia inflammation. Elevated exhaled NO and high blood eosinophil count (≥300 cells/µL) are useful biomarkers that predict airway eosinophilia (Annangi et al., 2022; GOLD, 2022). NO is generated from guanidine nitrogen of L-arginine under the catalysis of the NO synthase (NOS) family, including endothelial (eNOS), inducible (iNOS), and neuronal (nNOS) (Gantner et al., 2020). The nNOS existed in the neurons and eNOS was most abundant in endothelial cells. iNOS was induced by inflammatory factors and expressed in inflammatory cells, while in the lung epithelium, there is a constitutive expression of iNOS (Bayarri et al., 2021). The biosynthesis of H2S was intertwined with NO production (Figure 4). A previous study indicated that exhaled H2S was positively correlated with exhaled NO in patients with COPD and healthy controls (Sun et al., 2013). H2S enhances the activity and expression of eNOS (Li et al., 2017). CSE deficiency in mice causes eNOS dysfunction, NO reduction, and aggravated myocardial ischemia/reperfusion injury (137). On the other hand, H2S restricted NO activity by forming a nitrosothiol compound, which inhibits eNOS in smooth muscle cells (Skovgaard et al., 2011). The regulation of H2S generation by NO is very complicated. NO was reported to enhance endogenous H2S production by promoting CSE expression and facilitating the uptake of L-cysteine as a substrate for H2S production in vascular endothelial cells (Li et al., 1999; Wang, 2012). In a pulmonary hypertensive rat model, L-arginine treatment elevated plasma H2S concentration, H2S production rate, and CSE mRNA expression in lung tissues. Meanwhile, there were contradictory findings stating that NO had no influence on H2S synthases nor inhibits CBS activation (Chen et al., 2014; Vicente et al., 2016). In lung cancer cells, NO can suppress CBS activity by oxidizing its ferric heme subunit (Wang and Yang, 2016). The crosstalk between H2S and NO was cell-specific and concentration-relevant. More research studies are needed to explore the relationship between H2S and NO in lung tissue during physiological and pathological states.
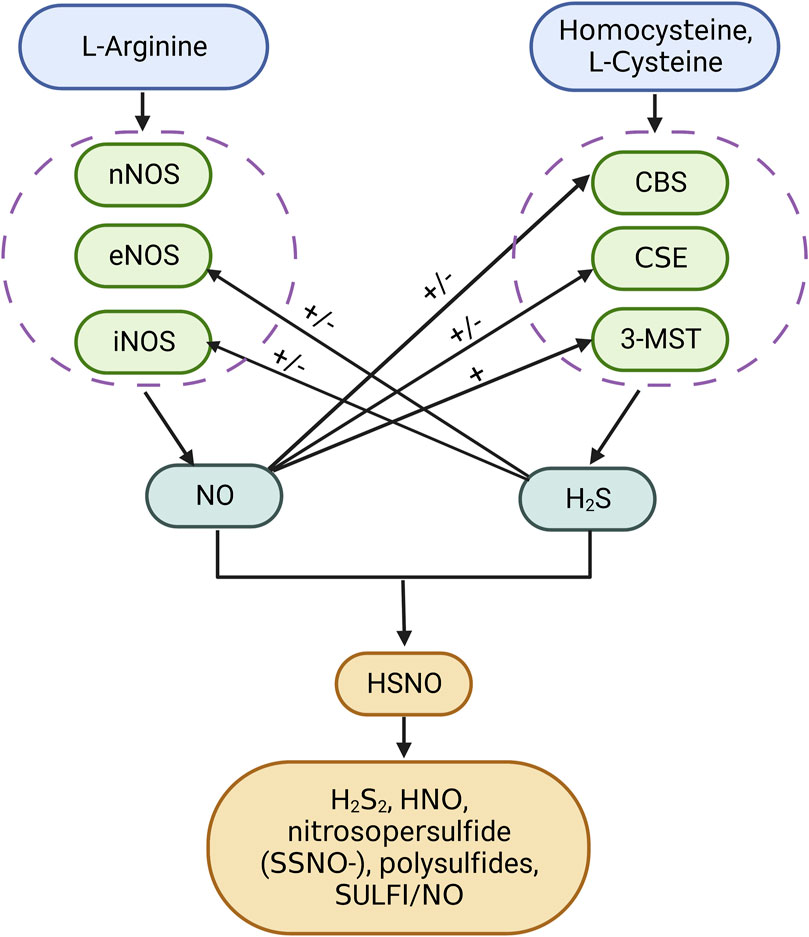
FIGURE 4. Interaction between hydrogen sulfide and nitric oxide. H2S can directly react with NO to form HSNO. HSNO is unstable, which can be reduced by H2S to form HNO and H2S2. Chemical interplay of the H2S donor Na2S with the NO donor (DEA/NO or RSNOs) produces nitrosopersulfide (SSNO-), polysulfides, and SULFI/NO. H2S has both activation and inhibition ability toward the synthesis of NO and vice versa. SULFI, N-nitrosohydroxylamine-N-sulfonate.
Sulfur molecules and comorbidities of COPD
The disturbance of the redox buffer makes the patients more vulnerable to oxidative stress, and this may be the reason for consistent systemic inflammation presented in patients with COPD. Systemic inflammation plays an important role in the development of multiple comorbidities. Cardiovascular diseases are very prevalent comorbidities in COPD. Elevated plasma homocysteine concentration has long been recognized as an independent risk factor for cardiovascular diseases (Ozkan et al., 2002). The trans-sulfuration pathway is the major way for homocysteine clearance, and during this process, a range of low-molecular thiols are produced, which act as the major antioxidant buffers in vivo. The concentrations of plasma homocysteine and cysteinylglycine were elevated in patients with COPD (Zinellu et al., 2020), which was significantly associated with abnormal lung function parameters as well as COPD severity, and the disturbance of small-molecule thiols may be involved in the increased cardiovascular risks among COPD patients. Patients with COPD and cardiovascular diseases have lower H2S and homocysteine levels than those in the COPD group (He et al., 2017). In addition, endogenous H2S plays a cardioprotective role in regulating heart rhythm, modifying cardiovascular remodeling, hypertension, and atherosclerosis (Ma et al., 2015; Meng et al., 2015; Xie et al., 2016; Watts et al., 2021). Disturbance of H2S and other sulfur-containing molecules could be initiator factors for the development of cardiovascular comorbidities among patients with COPD.
Obstructive sleep apnea (OSA) is a disorder characterized by repeated hypopnea and hypoxemia during sleep. Patients with OSA-COPD overlap syndrome experience more frequent hypoxemia and cardiac arrhythmias (Shepard et al., 1985; Chaouat et al., 1995). The oxygen homeostasis is sustained by a hypoxic ventilatory response, which is regulated by the carotid body, a peripheral arterial chemoreceptor for O2 sensor. Smoke can inhibit hypoxic ventilatory response and induce aggravated hypoxemia during sleep, especially in relevant clinical conditions such as COPD (Hildebrandt et al., 2016). The thiol/disulfide redox state in the plasma and in peripheral blood mononuclear cells could massively affect the hypoxic ventilatory response via affecting carotid body O2 chemosensitivity (Lipton et al., 2001; Hildebrandt et al., 2002). It is reasonable to infer that the thiol/disulphide redox state in COPD is one of the reasons for lower hypoxic ventilatory response, which leads to aggravated hypoxemia during sleep, especially in patients with OSA-COPD overlap syndrome.
Lung adenocarcinoma expressed high levels of CBS, CSE, and MST relative to adjacent lung tissue (Szczesny et al., 2016). H2S synthesized by these enzymes can promote mitochondrial DNA repair and bioenergetics. The enhanced H2S synthesis also protects the cancer cells from therapeutic drugs. Single-nucleotide polymorphisms in the CBS [CBS rs2850146 (-8283G > C)] were significantly associated with high methylation in males (Flores et al., 2012). The variant allele of rs2850146 was associated with increased plasma homocysteine concentrations and gene hypermethylation, a known biomarker for promoting lung cancer (Flores et al., 2012), while organosulfur compounds, for example, isothiocyanates, allyl compounds, and sulforaphane, can inhibit the reactivity of histone deacetylase inhibitors and induce histone hyperacetylation in cancer cells, resulting in elevated p21 protein expression and cell cycle arrest (Nian et al., 2009).
Innovative drugs derived from sulfur compounds
Although numerous research studies demonstrated the therapeutic potential of H2S, the pharmacological application of H2S was limited by its gaseous nature and toxicity at high concentrations. Selective and controllable release of H2S is essential for its therapeutic application. To solve this problem, people constructed H2S donors that were triggered by hydrolysis, thiols, which provide a more controllable release (Powell et al., 2018a; Zheng et al., 2018). Synthetic compounds that release H2S upon activation by external stimuli such as light, reactive oxygen species, and enzymes were also been developed (Zheng et al., 2016; Chauhan et al., 2017; Xiao et al., 2017). External stimuli can induce spatial and/or temporal control of H2S release. For example, using redox-activated metals, such as Cu, Pt, Co, Fe, Ru, Os, and Ir, can develop prodrugs that are specifically activated in hypoxic cells, and then the H2S can be selectively delivered into cancer cells or ischemic cells (Woods and Wilson, 2021). Some prefer the delivery of persulfides or polysulfides to direct protein S-sulfidation. Persulfides that are triggered by PH, esterase, reactive oxygen species, and photons were gradually been developed, for instance, a hydrogen peroxide-sensing motif was constructed to develop a reactive oxygen species-activated persulfide donor (Artaud and Galardon, 2014; Powell et al., 2018b; Yu et al., 2018; Yuan et al., 2018; Hankins et al., 2020).
Some naturally derived sulfur compounds were found to generate H2S in a controllable manner. Diallyl disulfide is a reactive sulfane sulfur with an unsaturated bond near the C-S. It is naturally present in garlic. Other sulfur compounds extracted from garlic extracts include cysteine alkyl disulfides, cysteine mercaptide, and diallyl trisulfide. Their antioxidant capabilities have garnered wide interests, although sometimes they displayed contradictory effects on health in clinical practice (Li et al., 2013). Recently, Cardoso et al. (2021) found that diallyl disulfide can effectively prevent emphysema induced by cigarette smoking, and this function may be exerted through modification of 4-hydroxynonenal, carbonyl reductase 1, and cytochrome P450 2E1 (CYP2E1). The diallyl disulfide can act as a histone deacetylase inhibitor and induce sustained histone hyperacetylation, thus modifying gene transcription (Nian et al., 2009). The pharmacologic potential of these reactive sulfane sulfurs in diseases accompanied by excessive oxidative stress deserves further exploration.
Future perspective
Sulfur compounds such as H2S, thiol-based families, and sulfane sulfurs were fully engaged in the initiating and progressing process of COPD. They have the potential to protect individuals from developing chronic inflammatory diseases due to their antioxidant and anti-inflammation capabilities. The disturbance of antioxidant buffers, particularly low-molecular thiols, contributes to excessive oxidative stress and systemic inflammation, which may result in a high prevalence of comorbidities like cardiovascular diseases. However, the correct measurement of these sulfur compounds in vivo is very difficult because some of the sulfur compounds have similar chemical reactivity and they were under dynamic interchange, which arouses a major limitation during studying their bioactivities. H2S exhibits its function by S-sulfidation of the target protein, and the specific signaling pathway in relation to COPD deserves further exploration. Furthermore, more research studies are needed to reveal the possible relationship between sulfur compounds and comorbidities of COPD.
Author contributions
YC and SJ contributed to the study design, data collection, analysis, interpretation, and editing of the manuscript.
Funding
This work received grants from the National Natural Science Foundation of China (No. 81970037 and No. 82090014) and Capital Health Development Scientific Research Foundation of China (No. 2020-2Z-40917).
Conflict of interest
The authors declare that the research was conducted in the absence of any commercial or financial relationships that could be construed as a potential conflict of interest.
Publisher’s note
All claims expressed in this article are solely those of the authors and do not necessarily represent those of their affiliated organizations, or those of the publisher, the editors, and the reviewers. Any product that may be evaluated in this article, or claim that may be made by its manufacturer, is not guaranteed or endorsed by the publisher.
References
Akaike, T., Ida, T., Wei, F. Y., Nishida, M., Kumagai, Y., Alam, M. M., et al. (2017). Cysteinyl-tRNA synthetase governs cysteine polysulfidation and mitochondrial bioenergetics. Nat. Commun. 8 (1), 1177. doi:10.1038/s41467-017-01311-y
Annangi, S., Nutalapati, S., Sturgill, J., Flenaugh, E., and Foreman, M. (2022). Eosinophilia and fractional exhaled nitric oxide levels in chronic obstructive lung disease. Thorax 77 (4), 351–356. doi:10.1136/thoraxjnl-2020-214644
Artaud, I., and Galardon, E. (2014). A persulfide analogue of the nitrosothiol SNAP: Formation, characterization and reactivity. Chembiochem 15 (16), 2361–2364. doi:10.1002/cbic.201402312
Atkuri, K. R., Mantovani, J. J., Herzenberg, L. A., and Herzenberg, L. A. (2007). N-Acetylcysteine-a safe antidote for cysteine/glutathione deficiency. Curr. Opin. Pharmacol. 7 (4), 355–359. doi:10.1016/j.coph.2007.04.005
Barnes, P. J. (2016). Inflammatory mechanisms in patients with chronic obstructive pulmonary disease. J. Allergy Clin. Immunol. 138 (1), 16–27. doi:10.1016/j.jaci.2016.05.011
Bayarri, M. A., Milara, J., Estornut, C., and Cortijo, J. (2021). Nitric oxide system and bronchial epithelium: More than a barrier. Front. Physiol. 12, 687381. doi:10.3389/fphys.2021.687381
Beltowski, J. (2015). Hydrogen sulfide in pharmacology and medicine-An update. Pharmacol. Rep. 67 (3), 647–658. doi:10.1016/j.pharep.2015.01.005
Bhatia, M. (2015). H2S and inflammation: An overview. Handb. Exp. Pharmacol. 230, 165–180. doi:10.1007/978-3-319-18144-8_8
Bhatia, M. (2012). Role of hydrogen sulfide in the pathology of inflammation. Sci. (Cairo) 2012, 159680. doi:10.6064/2012/159680
Bibli, S. I., Luck, B., Zukunft, S., Wittig, J., Chen, W., Xian, M., et al. (2018). A selective and sensitive method for quantification of endogenous polysulfide production in biological samples. Redox Biol. 18, 295–304. doi:10.1016/j.redox.2018.07.016
Bodas, M., Pehote, G., Silverberg, D., Gulbins, E., and Vij, N. (2019). Autophagy augmentation alleviates cigarette smoke-induced CFTR-dysfunction, ceramide-accumulation and COPD-emphysema pathogenesis. Free Radic. Biol. Med. 131, 81–97. doi:10.1016/j.freeradbiomed.2018.11.023
Bridgeman, M. M., Marsden, M., Selby, C., Morrison, D., and MacNee, W. (1994). Effect of N-acetyl cysteine on the concentrations of thiols in plasma, bronchoalveolar lavage fluid, and lung tissue. Thorax 49 (7), 670–675. doi:10.1136/thx.49.7.670
Cantin, A. M., North, S. L., Hubbard, R. C., and Crystal, R. G. (1987). Normal alveolar epithelial lining fluid contains high levels of glutathione. J. Appl. Physiol. 63 (1), 152–157. doi:10.1152/jappl.1987.63.1.152
Cao, X., Ding, L., Xie, Z. Z., Yang, Y., Whiteman, M., Moore, P. K., et al. (2019). A review of hydrogen sulfide synthesis, metabolism, and measurement: Is modulation of hydrogen sulfide a novel therapeutic for cancer? Antioxid. Redox Signal. 31 (1), 1–38. doi:10.1089/ars.2017.7058
Cardoso, A. O. P., Pecli, E. S. C., Dos Anjos, F. F., Quesnot, N., Valenca, H. D. M., Cattani-Cavalieri, I., et al. (2021). Diallyl disulfide prevents cigarette smoke-induced emphysema in mice. Pulm. Pharmacol. Ther. 69, 102053. doi:10.1016/j.pupt.2021.102053
Cazzola, M., Calzetta, L., Page, C., Rogliani, P., and Matera, M. G. (2019). Thiol-based drugs in pulmonary medicine: Much more than mucolytics. Trends Pharmacol. Sci. 40 (7), 452–463. doi:10.1016/j.tips.2019.04.015
Cazzola, M., Page, C., Rogliani, P., Calzetta, L., and Matera, M. G. (2020). Multifaceted beneficial effects of erdosteine: More than a mucolytic agent. Drugs 80 (17), 1799–1809. doi:10.1007/s40265-020-01412-x
Chaouat, A., Weitzenblum, E., Krieger, J., Ifoundza, T., Oswald, M., and Kessler, R. (1995). Association of chronic obstructive pulmonary disease and sleep apnea syndrome. Am. J. Respir. Crit. Care Med. 151 (1), 82–86. doi:10.1164/ajrccm.151.1.7812577
Chauhan, P., Bora, P., Ravikumar, G., Jos, S., and Chakrapani, H. (2017). Esterase activated carbonyl sulfide/hydrogen sulfide (H2S) donors. Org. Lett. 19 (1), 62–65. doi:10.1021/acs.orglett.6b03336
Chen, P. H., Fu, Y. S., Wang, Y. M., Yang, K. H., Wang, D. L., and Huang, B. (2014). Hydrogen sulfide increases nitric oxide production and subsequent S-nitrosylation in endothelial cells. ScientificWorldJournal. 2014, 480387. doi:10.1155/2014/480387
Chen, W., Liu, C., Peng, B., Zhao, Y., Pacheco, A., and Xian, M. (2013). New fluorescent probes for sulfane sulfurs and the application in bioimaging. Chem. Sci. 4 (7), 2892–2896. doi:10.1039/C3SC50754H
Chen, Y. H., Yao, W. Z., Gao, J. Z., Geng, B., Wang, P. P., and Tang, C. S. (2009). Serum hydrogen sulfide as a novel marker predicting bacterial involvement in patients with community-acquired lower respiratory tract infections. Respirology 14 (5), 746–752. doi:10.1111/j.1440-1843.2009.01550.x
Chen, Y. H., Yao, W. Z., Geng, B., Ding, Y. L., Lu, M., Zhao, M. W., et al. (2005). Endogenous hydrogen sulfide in patients with COPD. Chest 128 (5), 3205–3211. doi:10.1378/chest.128.5.3205
Ding, H. B., Liu, K. X., Huang, J. F., Wu, D. W., Chen, J. Y., and Chen, Q. S. (2018). Protective effect of exogenous hydrogen sulfide on pulmonary artery endothelial cells by suppressing endoplasmic reticulum stress in a rat model of chronic obstructive pulmonary disease. Biomed. Pharmacother. 105, 734–741. doi:10.1016/j.biopha.2018.05.131
Doeller, J. E., Isbell, T. S., Benavides, G., Koenitzer, J., Patel, H., Patel, R. P., et al. (2005). Polarographic measurement of hydrogen sulfide production and consumption by mammalian tissues. Anal. Biochem. 341 (1), 40–51. doi:10.1016/j.ab.2005.03.024
Doka, E., Pader, I., Biro, A., Johansson, K., Cheng, Q., Ballago, K., et al. (2016). A novel persulfide detection method reveals protein persulfide- and polysulfide-reducing functions of thioredoxin and glutathione systems. Sci. Adv. 2 (1), e1500968. doi:10.1126/sciadv.1500968
Du, C., Lin, X., Xu, W., Zheng, F., Cai, J., Yang, J., et al. (2019). Sulfhydrated sirtuin-1 increasing its deacetylation activity is an essential epigenetics mechanism of anti-atherogenesis by hydrogen sulfide. Antioxid. Redox Signal. 30 (2), 184–197. doi:10.1089/ars.2017.7195
Fang, L., Gao, P., Bao, H., Tang, X., Wang, B., Feng, Y., et al. (2018). Chronic obstructive pulmonary disease in China: A nationwide prevalence study. Lancet. Respir. Med. 6 (6), 421–430. doi:10.1016/s2213-2600(18)30103-6
Flores, K. G., Stidley, C. A., Mackey, A. J., Picchi, M. A., Stabler, S. P., Siegfried, J. M., et al. (2012). Sex-specific association of sequence variants in CBS and MTRR with risk for promoter hypermethylation in the lung epithelium of smokers. Carcinogenesis 33 (8), 1542–1547. doi:10.1093/carcin/bgs194
Fu, M., Zhang, W., Wu, L., Yang, G., Li, H., and Wang, R. (2012). Hydrogen sulfide (H2S) metabolism in mitochondria and its regulatory role in energy production. Proc. Natl. Acad. Sci. U. S. A. 109 (8), 2943–2948. doi:10.1073/pnas.1115634109
Furne, J., Saeed, A., and Levitt, M. D. (2008). Whole tissue hydrogen sulfide concentrations are orders of magnitude lower than presently accepted values. Am. J. Physiol. Regul. Integr. Comp. Physiol. 295 (5), R1479–R1485. doi:10.1152/ajpregu.90566.2008
Gantner, B. N., LaFond, K. M., and Bonini, M. G. (2020). Nitric oxide in cellular adaptation and disease. Redox Biol. 34, 101550. doi:10.1016/j.redox.2020.101550
Gao, X. H., Krokowski, D., Guan, B. J., Bederman, I., Majumder, M., Parisien, M., et al. (2015). Quantitative H2S-mediated protein sulfhydration reveals metabolic reprogramming during the integrated stress response. Elife 4, e10067. doi:10.7554/eLife.10067
Ge, S. N., Zhao, M. M., Wu, D. D., Chen, Y., Wang, Y., Zhu, J. H., et al. (2014). Hydrogen sulfide targets EGFR Cys797/Cys798 residues to induce Na(+)/K(+)-ATPase endocytosis and inhibition in renal tubular epithelial cells and increase sodium excretion in chronic salt-loaded rats. Antioxid. Redox Signal. 21 (15), 2061–2082. doi:10.1089/ars.2013.5304
GOLD (2022). Global strategy for the diagnosis, management, and prevention of chronic obstructive pulmonary disease. Available: https://goldcopd.org/wp-content/uploads/2021/12/GOLD-REPORT-2022-v1.1-22Nov2021_WMV.pdf (Accessed Sept 30th, 2022).
Gould, N. S., Min, E., Gauthier, S., Chu, H. W., Martin, R., and Day, B. J. (2010). Aging adversely affects the cigarette smoke-induced glutathione adaptive response in the lung. Am. J. Respir. Crit. Care Med. 182 (9), 1114–1122. doi:10.1164/rccm.201003-0442OC
Gould, N. S., Min, E., Gauthier, S., Martin, R. J., and Day, B. J. (2011). Lung glutathione adaptive responses to cigarette smoke exposure. Respir. Res. 12 (1), 133. doi:10.1186/1465-9921-12-133
Guan, R., Cai, Z., Wang, J., Ding, M., Li, Z., Xu, J., et al. (2019). Hydrogen sulfide attenuates mitochondrial dysfunction-induced cellular senescence and apoptosis in alveolar epithelial cells by upregulating sirtuin 1. Aging (Albany NY) 11 (24), 11844–11864. doi:10.18632/aging.102454
Guan, R., Wang, J., Cai, Z., Li, Z., Wang, L., Li, Y., et al. (2020a). Hydrogen sulfide attenuates cigarette smoke-induced airway remodeling by upregulating SIRT1 signaling pathway. Redox Biol. 28, 101356. doi:10.1016/j.redox.2019.101356
Guan, R., Wang, J., Li, D., Li, Z., Liu, H., Ding, M., et al. (2020b). Hydrogen sulfide inhibits cigarette smoke-induced inflammation and injury in alveolar epithelial cells by suppressing PHD2/HIF-1α/MAPK signaling pathway. Int. Immunopharmacol. 81, 105979. doi:10.1016/j.intimp.2019.105979
Han, W., Dong, Z., Dimitropoulou, C., and Su, Y. (2011). Hydrogen sulfide ameliorates tobacco smoke-induced oxidative stress and emphysema in mice. Antioxid. Redox Signal. 15 (8), 2121–2134. doi:10.1089/ars.2010.3821
Hankins, R. A., Suarez, S. I., Kalk, M. A., Green, N. M., Harty, M. N., and Lukesh, J. C. (2020). An innovative hydrogen peroxide-sensing scaffold and insight towards its potential as an ROS-activated persulfide donor. Angew. Chem. Int. Ed. Engl. 59 (49), 22238–22245. doi:10.1002/anie.202010530
He, Y., Liu, S., Zhang, Z., Liao, C., Lin, F., Yao, W., et al. (2017). Imbalance of endogenous hydrogen sulfide and homocysteine in chronic obstructive pulmonary disease combined with cardiovascular disease. Front. Pharmacol. 8, 624. doi:10.3389/fphar.2017.00624
Heffner, J. E., and Repine, J. E. (1989). Pulmonary strategies of antioxidant defense. Am. Rev. Respir. Dis. 140 (2), 531–554. doi:10.1164/ajrccm/140.2.531
Hildebrandt, W., Alexander, S., Bartsch, P., and Droge, W. (2002). Effect of N-acetyl-cysteine on the hypoxic ventilatory response and erythropoietin production: Linkage between plasma thiol redox state and O(2) chemosensitivity. Blood 99 (5), 1552–1555. doi:10.1182/blood.v99.5.1552
Hildebrandt, W., Sauer, R., Koehler, U., Bartsch, P., and Kinscherf, R. (2016). Lower hypoxic ventilatory response in smokers compared to non-smokers during abstinence from cigarettes. BMC Pulm. Med. 16 (1), 159. doi:10.1186/s12890-016-0323-0
Ibrahim, H., Serag, A., and Farag, M. A. (2021). Emerging analytical tools for the detection of the third gasotransmitter H2S, a comprehensive review. J. Adv. Res. 27, 137–153. doi:10.1016/j.jare.2020.05.018
Iciek, M., Bilska-Wilkosz, A., and Gorny, M. (2019). Sulfane sulfur - new findings on an old topic. Acta Biochim. Pol. 66 (4), 533–544. doi:10.18388/abp.2019_2909
Iciek, M. B., Rokita, H. B., and Wlodek, L. B. (2001). Effects of diallyl disulfide and other donors of sulfane sulfur on the proliferation of human hepatoma cell line (HepG2). Neoplasma 48 (4), 307–312.
Ida, T., Sawa, T., Ihara, H., Tsuchiya, Y., Watanabe, Y., Kumagai, Y., et al. (2014). Reactive cysteine persulfides and S-polythiolation regulate oxidative stress and redox signaling. Proc. Natl. Acad. Sci. U. S. A. 111 (21), 7606–7611. doi:10.1073/pnas.1321232111
Jia, G., Yu, S., Sun, W., Yang, J., Wang, Y., Qi, Y., et al. (2020). Hydrogen sulfide attenuates particulate matter-induced emphysema and airway inflammation through nrf2-dependent manner. Front. Pharmacol. 11, 29. doi:10.3389/fphar.2020.00029
Koj, A., and Frendo, J. (1962). The activity of cysteine desulphhydrase and rhodanase in animal tissues. Acta Biochim. Pol. 9, 373–379.
Krishnan, N., Fu, C., Pappin, D. J., and Tonks, N. K. (2011). H2S-Induced sulfhydration of the phosphatase PTP1B and its role in the endoplasmic reticulum stress response. Sci. Signal. 4 (203), ra86. doi:10.1126/scisignal.2002329
Kyogoku, Y., Sugiura, H., Ichikawa, T., Numakura, T., Koarai, A., Yamada, M., et al. (2019). Nitrosative stress in patients with asthma-chronic obstructive pulmonary disease overlap. J. Allergy Clin. Immunol. 144 (4), 972–983. doi:10.1016/j.jaci.2019.04.023
Landry, A. P., Ballou, D. P., and Banerjee, R. (2021). Hydrogen sulfide oxidation by sulfide quinone oxidoreductase. Chembiochem. 22 (6), 949–960. doi:10.1002/cbic.202000661
Li, F., Zhang, P., Zhang, M., Liang, L., Sun, X., Li, M., et al. (2016). Hydrogen sulfide prevents and partially reverses ozone-induced features of lung inflammation and emphysema in mice. Am. J. Respir. Cell Mol. Biol. 55 (1), 72–81. doi:10.1165/rcmb.2015-0014OC
Li, H., Liu, H., Chen, Z., Zhao, R., Wang, Q., Ran, M., et al. (2019). Using resonance synchronous spectroscopy to characterize the reactivity and electrophilicity of biologically relevant sulfane sulfur. Redox Biol. 24, 101179. doi:10.1016/j.redox.2019.101179
Li, H., Marshall, Z. M., and Whorton, A. R. (1999). Stimulation of cystine uptake by nitric oxide: Regulation of endothelial cell glutathione levels. Am. J. Physiol. 276 (4), C803–C811. doi:10.1152/ajpcell.1999.276.4.C803
Li, L., Rose, P., and Moore, P. K. (2011). Hydrogen sulfide and cell signaling. Annu. Rev. Pharmacol. Toxicol. 51, 169–187. doi:10.1146/annurev-pharmtox-010510-100505
Li, L., Sun, T., Tian, J., Yang, K., Yi, K., and Zhang, P. (2013). Garlic in clinical practice: An evidence-based overview. Crit. Rev. Food Sci. Nutr. 53 (7), 670–681. doi:10.1080/10408398.2010.537000
Li, Q., and Lancaster, J. R. (2013). Chemical foundations of hydrogen sulfide biology. Nitric Oxide 35, 21–34. doi:10.1016/j.niox.2013.07.001
Li, X. H., Xue, W. L., Wang, M. J., Zhou, Y., Zhang, C. C., Sun, C., et al. (2017). H2S regulates endothelial nitric oxide synthase protein stability by promoting microRNA-455-3p expression. Sci. Rep. 7, 44807. doi:10.1038/srep44807
Liao, C. C., Chen, Y. H., Lin, F., and Qi, Y. F. (2015). Hydrogen sulfide inhibits transforming growth factor beta-1 induced bronchial epithelial-mesenchymal transition. Chin. Med. J. 128 (23), 3247–3250. doi:10.4103/0366-6999.170266
Liao, Y. X., Wang, X. H., Bai, Y., Lin, F., Li, M. X., Mi, W. J., et al. (2021). Relationship between endogenous hydrogen sulfide and pulmonary vascular indexes on high-resolution computed tomography in patients with chronic obstructive pulmonary disease. Int. J. Chron. Obstruct. Pulmon. Dis. 16, 2279–2289. doi:10.2147/COPD.S314349
Libiad, M., Vitvitsky, V., Bostelaar, T., Bak, D. W., Lee, H. J., Sakamoto, N., et al. (2019). Hydrogen sulfide perturbs mitochondrial bioenergetics and triggers metabolic reprogramming in colon cells. J. Biol. Chem. 294 (32), 12077–12090. doi:10.1074/jbc.RA119.009442
Liebhart, J., and Dobek, R. (2009). Transforming growth factor-beta in the pathogenesis of chronic obstructive pulmonary disease. J. Organ Dysfunct. 5 (3), 161–170. doi:10.1080/17471060701721985
Lin, F., Liao, C., Sun, Y., Zhang, J., Lu, W., Bai, Y., et al. (2017). Hydrogen sulfide inhibits cigarette smoke-induced endoplasmic reticulum stress and apoptosis in bronchial epithelial cells. Front. Pharmacol. 8, 675. doi:10.3389/fphar.2017.00675
Lin, F., Liao, C., Zhang, J., Sun, Y., Lu, W., Bai, Y., et al. (2022). Hydrogen sulfide inhibits bronchial epithelial cell epithelial mesenchymal transition through regulating endoplasm reticulum stress. Front. Mol. Biosci. 9, 828766. doi:10.3389/fmolb.2022.828766
Lipton, A. J., Johnson, M. A., Macdonald, T., Lieberman, M. W., Gozal, D., and Gaston, B. (2001). S-nitrosothiols signal the ventilatory response to hypoxia. Nature 413 (6852), 171–174. doi:10.1038/35093117
Ma, S. F., Luo, Y., Ding, Y. J., Chen, Y., Pu, S. X., Wu, H. J., et al. (2015). Hydrogen sulfide targets the cys320/cys529 motif in Kv4.2 to inhibit the ito potassium channels in cardiomyocytes and regularizes fatal arrhythmia in myocardial infarction. Antioxid. Redox Signal. 23 (2), 129–147. doi:10.1089/ars.2014.6094
Madurga, A., Golec, A., Pozarska, A., Ishii, I., Mizikova, I., Nardiello, C., et al. (2015). The H2S-generating enzymes cystathionine beta-synthase and cystathionine gamma-lyase play a role in vascular development during normal lung alveolarization. Am. J. Physiol. Lung Cell. Mol. Physiol. 309 (7), L710–L724. doi:10.1152/ajplung.00134.2015
Meng, G., Ma, Y., Xie, L., Ferro, A., and Ji, Y. (2015). Emerging role of hydrogen sulfide in hypertension and related cardiovascular diseases. Br. J. Pharmacol. 172 (23), 5501–5511. doi:10.1111/bph.12900
Meng, W., Chen, Y., Feng, Y., Zhang, H., Xu, Q., Sun, M., et al. (2018). An off-on fluorescent probe for the detection of mitochondria-specific protein persulfidation. Org. Biomol. Chem. 16 (34), 6350–6357. doi:10.1039/c8ob01608a
Modis, K., Ju, Y., Ahmad, A., Untereiner, A. A., Altaany, Z., Wu, L., et al. (2016). S-Sulfhydration of ATP synthase by hydrogen sulfide stimulates mitochondrial bioenergetics. Pharmacol. Res. 113, 116–124. doi:10.1016/j.phrs.2016.08.023
Mustafa, A. K., Gadalla, M. M., Sen, N., Kim, S., Mu, W., Gazi, S. K., et al. (2009). H2S signals through protein S-sulfhydration. Sci. Signal. 2 (96), ra72. doi:10.1126/scisignal.2000464
Neill, D. L., Chang, Y. C., Chen, W., Li, L., and Xian, M. (2019). A smartphone based device for the detection of sulfane sulfurs in biological systems. Sens. Actuators. B Chem. 292, 263–269. doi:10.1016/j.snb.2019.04.099
Nian, H., Delage, B., Ho, E., and Dashwood, R. H. (2009). Modulation of histone deacetylase activity by dietary isothiocyanates and allyl sulfides: Studies with sulforaphane and garlic organosulfur compounds. Environ. Mol. Mutagen. 50 (3), 213–221. doi:10.1002/em.20454
Numakura, T., Sugiura, H., Akaike, T., Ida, T., Fujii, S., Koarai, A., et al. (2017). Production of reactive persulfide species in chronic obstructive pulmonary disease. Thorax 72 (12), 1074–1083. doi:10.1136/thoraxjnl-2016-209359
Olson, K. R. (2009). Is hydrogen sulfide a circulating "gasotransmitter" in vertebrate blood? Biochim. Biophys. Acta 1787 (7), 856–863. doi:10.1016/j.bbabio.2009.03.019
Olson, K. R. (2021). The biological legacy of sulfur: A roadmap to the future. Comp. Biochem. Physiol. A Mol. Integr. Physiol. 252, 110824. doi:10.1016/j.cbpa.2020.110824
Olson, K. R., Whitfield, N. L., Bearden, S. E., St Leger, J., Nilson, E., Gao, Y., et al. (2010). Hypoxic pulmonary vasodilation: A paradigm shift with a hydrogen sulfide mechanism. Am. J. Physiol. Regul. Integr. Comp. Physiol. 298 (1), R51–R60. doi:10.1152/ajpregu.00576.2009
Ozkan, Y., Ozkan, E., and Simsek, B. (2002). Plasma total homocysteine and cysteine levels as cardiovascular risk factors in coronary heart disease. Int. J. Cardiol. 82 (3), 269–277. doi:10.1016/s0167-5273(02)00010-4
Pacitti, D., Scotton, C. J., Kumar, V., Khan, H., Wark, P. A. B., Torregrossa, R., et al. (2021). Gasping for sulfide: A critical appraisal of hydrogen sulfide in lung disease and accelerated aging. Antioxid. Redox Signal. 35 (7), 551–579. doi:10.1089/ars.2021.0039
Pan, J., and Carroll, K. S. (2013). Persulfide reactivity in the detection of protein s-sulfhydration. ACS Chem. Biol. 8 (6), 1110–1116. doi:10.1021/cb4001052
Papi, A., Zheng, J., Criner, G. J., Fabbri, L. M., and Calverley, P. M. A. (2019). Impact of smoking status and concomitant medications on the effect of high-dose N-acetylcysteine on chronic obstructive pulmonary disease exacerbations: A post-hoc analysis of the pantheon study. Respir. Med. 147, 37–43. doi:10.1016/j.rmed.2018.12.014
Park, C. M., Macinkovic, I., Filipovic, M. R., and Xian, M. (2015). Use of the "tag-switch" method for the detection of protein S-sulfhydration. Methods Enzymol. 555, 39–56. doi:10.1016/bs.mie.2014.11.033
Perry, M. M., Tildy, B., Papi, A., Casolari, P., Caramori, G., Rempel, K. L., et al. (2018). The anti-proliferative and anti-inflammatory response of COPD airway smooth muscle cells to hydrogen sulfide. Respir. Res. 19 (1), 85. doi:10.1186/s12931-018-0788-x
Powell, C. R., Dillon, K. M., and Matson, J. B. (2018a). A review of hydrogen sulfide (H2S) donors: Chemistry and potential therapeutic applications. Biochem. Pharmacol. 149, 110–123. doi:10.1016/j.bcp.2017.11.014
Powell, C. R., Dillon, K. M., Wang, Y., Carrazzone, R. J., and Matson, J. B. (2018b). A persulfide donor responsive to reactive oxygen species: Insights into reactivity and therapeutic potential. Angew. Chem. Int. Ed. Engl. 57 (21), 6324–6328. doi:10.1002/anie.201803087
Rahman, I., and MacNee, W. (2012). Antioxidant pharmacological therapies for COPD. Curr. Opin. Pharmacol. 12 (3), 256–265. doi:10.1016/j.coph.2012.01.015
Rahman, I., and MacNee, W. (1999). Lung glutathione and oxidative stress: Implications in cigarette smoke-induced airway disease. Am. J. Physiol. 277 (6), L1067–L1088. doi:10.1152/ajplung.1999.277.6.L1067
Rahman, I. (2012). Pharmacological antioxidant strategies as therapeutic interventions for COPD. Biochim. Biophys. Acta 1822 (5), 714–728. doi:10.1016/j.bbadis.2011.11.004
Ramazi, S., and Zahiri, J. (2021). Posttranslational modifications in proteins: Resources, tools and prediction methods. Database (Oxford) 2021, baab012. doi:10.1093/database/baab012
Ran, M., Wang, T., Shao, M., Chen, Z., Liu, H., Xia, Y., et al. (2019). Sensitive method for reliable quantification of sulfane sulfur in biological samples. Anal. Chem. 91 (18), 11981–11986. doi:10.1021/acs.analchem.9b02875
Rogliani, P., Matera, M. G., Page, C., Puxeddu, E., Cazzola, M., and Calzetta, L. (2019). Efficacy and safety profile of mucolytic/antioxidant agents in chronic obstructive pulmonary disease: A comparative analysis across erdosteine, carbocysteine, and N-acetylcysteine. Respir. Res. 20 (1), 104. doi:10.1186/s12931-019-1078-y
Sahebkar, A., Antonelli-Incalzi, R., Panahi, Y., Ghanei, M., and Pedone, C. (2015). Mustard lung and COPD: Common features and treatment? Lancet. Respir. Med. 3 (10), 747–748. doi:10.1016/S2213-2600(15)00363-X
Saito, J., Mackay, A. J., Rossios, C., Gibeon, D., Macedo, P., Sinharay, R., et al. (2014). Sputum-to-serum hydrogen sulfide ratio in COPD. Thorax 69 (10), 903–909. doi:10.1136/thoraxjnl-2013-204868
Shepard, J. W., Garrison, M. W., Grither, D. A., Evans, R., and Schweitzer, P. K. (1985). Relationship of ventricular ectopy to nocturnal oxygen desaturation in patients with chronic obstructive pulmonary disease. Am. J. Med. 78 (1), 28–34. doi:10.1016/0002-9343(85)90457-7
Skovgaard, N., Gouliaev, A., Aalling, M., and Simonsen, U. (2011). The role of endogenous H2S in cardiovascular physiology. Curr. Pharm. Biotechnol. 12 (9), 1385–1393. doi:10.2174/138920111798280956
Song, Y., Chi, D. Y., Yu, P., Lu, J. J., Xu, J. R., Tan, P. P., et al. (2019). Carbocisteine improves histone deacetylase 2 deacetylation activity via regulating sumoylation of histone deacetylase 2 in human tracheobronchial epithelial cells. Front. Pharmacol. 10, 166. doi:10.3389/fphar.2019.00166
Song, Y., Lu, H. Z., Xu, J. R., Wang, X. L., Zhou, W., Hou, L. N., et al. (2015). Carbocysteine restores steroid sensitivity by targeting histone deacetylase 2 in a thiol/GSH-dependent manner. Pharmacol. Res. 91, 88–98. doi:10.1016/j.phrs.2014.12.002
Sotgia, S., Fois, A. G., Paliogiannis, P., Carru, C., Mangoni, A. A., and Zinellu, A. (2021). Methodological fallacies in the determination of serum/plasma glutathione limit its translational potential in chronic obstructive pulmonary disease. Molecules 26 (6), 1572. doi:10.3390/molecules26061572
Sotgia, S., Paliogiannis, P., Sotgiu, E., Mellino, S., Zinellu, E., Fois, A. G., et al. (2020). Systematic review and meta-analysis of the blood glutathione redox state in chronic obstructive pulmonary disease. Antioxidants (Basel) 9 (11), E1146. doi:10.3390/antiox9111146
Stern, D. A., Morgan, W. J., Wright, A. L., Guerra, S., and Martinez, F. D. (2007). Poor airway function in early infancy and lung function by age 22 years: A non-selective longitudinal cohort study. Lancet 370 (9589), 758–764. doi:10.1016/S0140-6736(07)61379-8
Stubbert, D., Prysyazhna, O., Rudyk, O., Scotcher, J., Burgoyne, J. R., and Eaton, P. (2014). Protein kinase G Iα oxidation paradoxically underlies blood pressure lowering by the reductant hydrogen sulfide. Hypertension 64 (6), 1344–1351. doi:10.1161/HYPERTENSIONAHA.114.04281
Sun, Y., Wang, K., Li, M. X., He, W., Chang, J. R., Liao, C. C., et al. (2015). Metabolic changes of H2S in smokers and patients of COPD which might involve in inflammation, oxidative stress and steroid sensitivity. Sci. Rep. 5, 14971. doi:10.1038/srep14971
Sun, Y., Wang, X. M., Chen, Y. H., Zhu, R. X., and Liao, C. C. (2013). Exhaled hydrogen sulfide in patients with chronic obstructive pulmonary disease and its correlation with exhaled nitric oxide. Chin. Med. J. 126 (17), 3240–3244. doi:10.3760/cma.j.issn.0366-6999.20123064
Suzuki, Y., Saito, J., Kikuchi, M., Uematsu, M., Fukuhara, A., Sato, S., et al. (2018). Sputum-to-serum hydrogen sulphide ratio as a novel biomarker of predicting future risks of asthma exacerbation. Clin. Exp. Allergy 48 (9), 1155–1163. doi:10.1111/cea.13173
Szabo, C. (2018). A timeline of hydrogen sulfide (H(2)S) research: From environmental toxin to biological mediator. Biochem. Pharmacol. 149, 5–19. doi:10.1016/j.bcp.2017.09.010
Szczesny, B., Marcatti, M., Zatarain, J. R., Druzhyna, N., Wiktorowicz, J. E., Nagy, P., et al. (2016). Inhibition of hydrogen sulfide biosynthesis sensitizes lung adenocarcinoma to chemotherapeutic drugs by inhibiting mitochondrial DNA repair and suppressing cellular bioenergetics. Sci. Rep. 6, 36125. doi:10.1038/srep36125
Takano, Y., Shimamoto, K., and Hanaoka, K. (2016). Chemical tools for the study of hydrogen sulfide (H2S) and sulfane sulfur and their applications to biological studies. J. Clin. Biochem. Nutr. 58 (1), 7–15. doi:10.3164/jcbn.15-91
Tao, B. B., Liu, S. Y., Zhang, C. C., Fu, W., Cai, W. J., Wang, Y., et al. (2013). VEGFR2 functions as an H2S-targeting receptor protein kinase with its novel Cys1045-Cys1024 disulfide bond serving as a specific molecular switch for hydrogen sulfide actions in vascular endothelial cells. Antioxid. Redox Signal. 19 (5), 448–464. doi:10.1089/ars.2012.4565
Teng, H., Wu, B., Zhao, K., Yang, G., Wu, L., and Wang, R. (2013). Oxygen-sensitive mitochondrial accumulation of cystathionine beta-synthase mediated by Lon protease. Proc. Natl. Acad. Sci. U. S. A. 110 (31), 12679–12684. doi:10.1073/pnas.1308487110
van der Toorn, M., Smit-de Vries, M. P., Slebos, D. J., de Bruin, H. G., Abello, N., van Oosterhout, A. J., et al. (2007). Cigarette smoke irreversibly modifies glutathione in airway epithelial cells. Am. J. Physiol. Lung Cell. Mol. Physiol. 293 (5), L1156–L1162. doi:10.1152/ajplung.00081.2007
Vicente, J. B., Malagrino, F., Arese, M., Forte, E., Sarti, P., and Giuffre, A. (2016). Bioenergetic relevance of hydrogen sulfide and the interplay between gasotransmitters at human cystathionine beta-synthase. Biochim. Biophys. Acta 1857 (8), 1127–1138. doi:10.1016/j.bbabio.2016.03.030
Vij, N., Chandramani-Shivalingappa, P., Van Westphal, C., Hole, R., and Bodas, M. (2018). Cigarette smoke-induced autophagy impairment accelerates lung aging, COPD-emphysema exacerbations and pathogenesis. Am. J. Physiol. Cell Physiol. 314 (1), C73–C87. doi:10.1152/ajpcell.00110.2016
Wang, C., Xu, J., Yang, L., Xu, Y., Zhang, X., Bai, C., et al. (2018). Prevalence and risk factors of chronic obstructive pulmonary disease in China (the China pulmonary health [CPH] study): A national cross-sectional study. Lancet 391 (10131), 1706–1717. doi:10.1016/s0140-6736(18)30841-9
Wang, L., Meng, J., Wang, C., Wang, Y., Yang, C., and Li, Y. (2022). Hydrogen sulfide attenuates cigarette smoke-induced pyroptosis through the TLR4/NF-κB signaling pathway. Int. J. Mol. Med. 49 (5), 56. doi:10.3892/ijmm.2022.5112
Wang, L., Meng, J., Wang, C., Yang, C., Wang, Y., Li, Y., et al. (2020). Hydrogen sulfide alleviates cigarette smoke-induced COPD through inhibition of the TGF-β1/smad pathway. Exp. Biol. Med. 245 (3), 190–200. doi:10.1177/1535370220904342
Wang, R. (2012). Shared signaling pathways among gasotransmitters. Proc. Natl. Acad. Sci. U. S. A. 109 (23), 8801–8802. doi:10.1073/pnas.1206646109
Wang, R. (2002). Two's company, three's a crowd: Can H2S be the third endogenous gaseous transmitter? FASEB J. 16 (13), 1792–1798. doi:10.1096/fj.02-0211hyp
Wang, X. Y., and Yang, H. W. (2016). Upregulation of CBS/H2S system contributes to asymmetric dimethylarginine-triggered protection against the neurotoxicity of glutamate to PC12 cells by inhibiting NOS/NO pathway. Exp. Cell Res. 346 (1), 111–118. doi:10.1016/j.yexcr.2016.06.010
Wang, Y., Liao, S., Pan, Z., Jiang, S., Fan, J., Yu, S., et al. (2022). Hydrogen sulfide alleviates particulate matter-induced emphysema and airway inflammation by suppressing ferroptosis. Free Radic. Biol. Med. 186, 1–16. doi:10.1016/j.freeradbiomed.2022.04.014
Watson, W. H., Ritzenthaler, J. D., Peyrani, P., Wiemken, T. L., Furmanek, S., Reyes Vega, A. M., et al. (2019). Plasma cysteine/cystine and glutathione/glutathione disulfide redox potentials in HIV and COPD patients. Free Radic. Biol. Med. 143, 55–61. doi:10.1016/j.freeradbiomed.2019.07.031
Watts, M., Kolluru, G. K., Dherange, P., Pardue, S., Si, M., Shen, X., et al. (2021). Decreased bioavailability of hydrogen sulfide links vascular endothelium and atrial remodeling in atrial fibrillation. Redox Biol. 38, 101817. doi:10.1016/j.redox.2020.101817
Whitfield, N. L., Kreimier, E. L., Verdial, F. C., Skovgaard, N., and Olson, K. R. (2008). Reappraisal of H2S/sulfide concentration in vertebrate blood and its potential significance in ischemic preconditioning and vascular signaling. Am. J. Physiol. Regul. Integr. Comp. Physiol. 294 (6), R1930–R1937. doi:10.1152/ajpregu.00025.2008
WHO (2019). Global health estimates: Leading causes of death. Cause-Specific mortality 2000–2019. Available: https://www.who.int/data/gho/data/themes/mortality-and-global-health-estimates/ghe-leading-causes-of-death (Accessed Sept 30th, 2022).
Woods, J. J., and Wilson, J. J. (2021). A dinuclear persulfide-bridged ruthenium compound is a hypoxia-selective hydrogen sulfide (H2 S) donor. Angew. Chem. Int. Ed. Engl. 60 (3), 1588–1592. doi:10.1002/anie.202012620
Xiao, Z., Bonnard, T., Shakouri-Motlagh, A., Wylie, R. A. L., Collins, J., White, J., et al. (2017). Triggered and tunable hydrogen sulfide release from photogenerated thiobenzaldehydes. Chemistry 23 (47), 11294–11300. doi:10.1002/chem.201701206
Xie, L., Gu, Y., Wen, M., Zhao, S., Wang, W., Ma, Y., et al. (2016). Hydrogen sulfide induces Keap1 S-sulfhydration and suppresses diabetes-accelerated atherosclerosis via Nrf2 activation. Diabetes 65 (10), 3171–3184. doi:10.2337/db16-0020
Xu, C., Wang, L., Fozouni, P., Evjen, G., Chandra, V., Jiang, J., et al. (2020). SIRT1 is downregulated by autophagy in senescence and ageing. Nat. Cell Biol. 22 (10), 1170–1179. doi:10.1038/s41556-020-00579-5
Xue, R., Hao, D. D., Sun, J. P., Li, W. W., Zhao, M. M., Li, X. H., et al. (2013). Hydrogen sulfide treatment promotes glucose uptake by increasing insulin receptor sensitivity and ameliorates kidney lesions in type 2 diabetes. Antioxid. Redox Signal. 19 (1), 5–23. doi:10.1089/ars.2012.5024
Yang, G., Zhao, K., Ju, Y., Mani, S., Cao, Q., Puukila, S., et al. (2013). Hydrogen sulfide protects against cellular senescence via S-sulfhydration of Keap1 and activation of Nrf2. Antioxid. Redox Signal. 18 (15), 1906–1919. doi:10.1089/ars.2012.4645
Yu, B., Zheng, Y., Yuan, Z., Li, S., Zhu, H., De La Cruz, L. K., et al. (2018). Toward direct protein S-persulfidation: A prodrug approach that directly delivers hydrogen persulfide. J. Am. Chem. Soc. 140 (1), 30–33. doi:10.1021/jacs.7b09795
Yu, C., Li, X., Zeng, F., Zheng, F., and Wu, S. (2013). Carbon-dot-based ratiometric fluorescent sensor for detecting hydrogen sulfide in aqueous media and inside live cells. Chem. Commun. 49 (4), 403–405. doi:10.1039/c2cc37329g
Yuan, Z., Zheng, Y., Yu, B., Wang, S., Yang, X., and Wang, B. (2018). Esterase-sensitive glutathione persulfide donor. Org. Lett. 20 (20), 6364–6367. doi:10.1021/acs.orglett.8b02611
Zhang, J., Wang, X., Chen, Y., and Yao, W. (2015). Exhaled hydrogen sulfide predicts airway inflammation phenotype in COPD. Respir. Care 60 (2), 251–258. doi:10.4187/respcare.03519
Zheng, Y., Yu, B., De La Cruz, L. K., Roy Choudhury, M., Anifowose, A., and Wang, B. (2018). Toward hydrogen sulfide based therapeutics: Critical drug delivery and developability issues. Med. Res. Rev. 38 (1), 57–100. doi:10.1002/med.21433
Zheng, Y., Yu, B., Ji, K., Pan, Z., Chittavong, V., and Wang, B. (2016). Esterase-sensitive prodrugs with tunable release rates and direct generation of hydrogen sulfide. Angew. Chem. Int. Ed. Engl. 55 (14), 4514–4518. doi:10.1002/anie.201511244
Zhou, M., Wang, H., Zeng, X., Yin, P., Zhu, J., Chen, W., et al. (2019). Mortality, morbidity, and risk factors in China and its provinces, 1990-2017: A systematic analysis for the global burden of disease study 2017. Lancet 394 (10204), 1145–1158. doi:10.1016/s0140-6736(19)30427-1
Zinellu, A., Fois, A. G., Sotgia, S., Zinellu, E., Bifulco, F., Pintus, G., et al. (2016). Plasma protein thiols: An early marker of oxidative stress in asthma and chronic obstructive pulmonary disease. Eur. J. Clin. Invest. 46 (2), 181–188. doi:10.1111/eci.12582
Zinellu, A., Zinellu, E., Sotgiu, E., Fois, A. G., Paliogiannis, P., Scano, V., et al. (2020). Systemic transsulfuration pathway thiol concentrations in chronic obstructive pulmonary disease patients. Eur. J. Clin. Invest. 50, e13267. doi:10.1111/eci.13267
Keywords: chronic obstructive pulmonary disease, hydrogen sulfide, sulfur compound, sulfane sulfur, thiols
Citation: Jiang S and Chen Y (2022) The role of sulfur compounds in chronic obstructive pulmonary disease. Front. Mol. Biosci. 9:928287. doi: 10.3389/fmolb.2022.928287
Received: 25 April 2022; Accepted: 03 October 2022;
Published: 19 October 2022.
Edited by:
Chunbin Zou, University of Pittsburgh, United StatesReviewed by:
Alexandru Corlateanu, Nicolae Testemitanu State University of Medicine and Pharmacy, MoldovaFabiao Yu, Hainan Medical University, China
Copyright © 2022 Jiang and Chen. This is an open-access article distributed under the terms of the Creative Commons Attribution License (CC BY). The use, distribution or reproduction in other forums is permitted, provided the original author(s) and the copyright owner(s) are credited and that the original publication in this journal is cited, in accordance with accepted academic practice. No use, distribution or reproduction is permitted which does not comply with these terms.
*Correspondence: Yahong Chen, chenyahong@vip.sina.com