- 1Department of Rheumatology, The First Affiliated Hospital of Anhui Medical University, Hefei, China
- 2Department of Physiology, School of Basic Medicine of Anhui Medical University, Hefei, China
- 3Department of Endocrinology, The First Affiliated Hospital of Anhui Medical University, Hefei, China
Primary biliary cholangitis (PBC) is an autoimmune-mediated chronic cholestatic liver disease, fatigue, and skin itching are the most common clinical symptoms. Its main pathological feature is the progressive damage and destruction of bile duct epithelial cells. Non-coding RNA (NcRNA, mainly including microRNA, long non-coding RNA and circular RNA) plays a role in the pathological and biological processes of various diseases, especially autoimmune diseases. Many validated ncRNAs are expected to be biomarkers for the diagnosis or treatment of PBC. This review will elucidate the pathogenesis of PBC and help to identify potential ncRNA biomarkers for PBC.
Introduction
Primary biliary cholangitis (PBC) is a chronic cholestatic liver disease mediated by autoimmunity. Fatigue and pruritus are the most common clinical symptoms, usually preceding the appearance of jaundice by years (Erice et al., 2018; Beuers et al., 2015). The main histological features are chronic progressive damage and destruction of bile duct epithelial cells (non-suppurative cholangitis), portal inflammation (mainly lymphocytes, plasma cells, and eosinophils) and increased fibrosis (Poupon, 2010; Tsuneyama et al., 2017; Pandit and Samant, 2022). PBC is globally distributed and can occur in all races and ethnicities. A recent meta-analysis (Lv et al., 2021) showed that both the incidence and prevalence of PBC are on the rise globally, with an annual incidence rate of 0.23/100,000–5.31/100,000, and a prevalence of 1.91/100,000–40.2/100,000. The highest in North America and Nordic countries (Kim et al., 2000; Koulentaki et al., 2014; Metcalf et al., 1997; Marschall et al., 2019), and the lowest in Canada (Witt-Sullivan et al., 1990) and Australia (Watson et al., 1995). Population-based epidemiological data on PBC are still lacking in China. A recent meta-analysis (Zeng et al., 2019) estimated that the prevalence of PBC in China was 20.5/100,000, ranking second in the Asia-Pacific region after Japan. Figure 1. The cause of PBC is unknown, and may be caused by a highly complex interaction between genetic and environmental factors. There is also extensive evidence that inflammation and immune disorders have an important impact on PBC. The disease mostly affects middle-aged women (i.e., 85%–90% patients onset at 40–60 years old), with female-to-male ratios is about 1:10, while higher mortality was described in men (Gonzalez and Washington, 2018). Moreover, there are also recent literature of increasing in male PBC (Lleo et al., 2016). This may be related to additional environmental exposures, higher prevalence of viral hepatitis, greater awareness of the disease by physicians and patients and unknown gender factors that may modulate immunity (Lleo et al., 2016). The specific reasons need to be further explored.
PBC was also previously known as primary biliary cirrhosis. The name change reflects the fact that cirrhosis only occurs in the late stage, so it is difficult to correctly identify patients with early disease. According to the AASLD (American Association for the Study of Liver Diseases), two of the following three criteria are met to confirm the diagnosis of PBC: 1) biochemical evidence of cholestasis based on alkaline phosphatase (ALP) elevation; 2) anti-mitochondrial antibody (AMA) positivity; 3) histological evidence of intrahepatic destructive cholangitis (Carey et al., 2015). Among them, serum AMA, especially the positive AMA-M2 subtype, has high sensitivity and specificity for the diagnosis of PBC, and are recognized as distinct diagnostic biomarkers. There is also a PBC patient subpopulation (∼5%–10%) who have the same clinical and histological features as classic PBC, but AMAs are negative even with the most sensitive detection methods. For these patients, the diagnosis is mainly based on liver biopsy (Kouroumalis et al., 2018). However, problems can arise in the presence of ascites or infection and are not easily accepted by patients.
The first-line treatment of PBC is the daily administration of ursodeoxycholic acid (UDCA), which improves prognosis in ∼2/3 of patients in early stage of the disease. This means up to 40% of patients have an incomplete response to UDCA, and the long-term survival rate is lower than that of the general population (Parés et al., 2006). UDCA was approved by the United States FDA (Food and Drug Administration) in 2016 as a second-line treatment for patients with primary biliary cholangitis who are unresponsive to UDCA; however, approximately 50% of patients might need additional treatments to reach therapeutic goals. Otherwise, these patients may progress to liver transplantation or even die (Gulamhusein and Hirschfield, 2020). Therefore, identification of novel and promising biomarkers is crucial for PBC early diagnosis and (or) treatment.
Non-coding RNA (NcRNA) refers to a functional RNA molecule that cannot be translated into protein. They can perform their respective biological functions at the RNA level (Wang et al., 2019). Due to increasing development of microarray sequencing techniques, accumulating data have suggested ncRNAs play important roles in regulating autoimmunity and inflammation (Kempinska-Podhorodecka et al., 2017; Young et al., 2017; Wang et al., 2018a). In addition, different cells and tissues have different ncRNA expression profiles (Chen et al., 2016; Katsumi et al., 2016; Zheng et al., 2017a; Erice et al., 2018; Xiang et al., 2019). Erice studies have shown that miR-506 is overexpressed in cholangiocytes of PBC, induces PBC-like characteristics in cholangiocytes and promotes immune activation (Erice et al., 2018). Dai et al. compared miRNAs in renal biopsy samples from patients with class II lupus nephritis (LN) and nephrectomy samples from patients with renal tumors. The results showed that there were 66 differentially regulated miRNAs (36 upregulated and the remaining 30 downregulated) in lupus nephritis patients (Dai et al., 2009). Wu et al. showed that linc0949 and linc0597 were significantly decreased in patients with SLE compared with patients with RA and healthy control subjects. Moreover, linc0949 was positively correlated with SLEDAI-2K scores and negatively correlated with complement component C3 levels (Wu et al., 2015). However, the roles of these specifically expressed ncRNAs in the pathogenesis of PBC have not been fully elucidated.
In the present review, some functional ncRNAs are listed in Table 1, mainly including microRNAs (miRNAs), long non-coding RNAs (lncRNAs), and circular RNAs (circRNAs) (Katsushima et al., 2014; Nakagawa et al., 2017; Wang et al., 2017; Wasik et al., 2017; Afonso et al., 2018; Wasik et al., 2020). We aimed to elucidate the dysregulated ncRNAs in PBC that contribute to the understanding of the pathogenesis of PBC by reviewing all currently published studies. Most importantly, helping to identify those aberrantly expressed ncRNAs in PBC will facilitate the exploration of promising biomarkers for early diagnosis and treatment of PBC.
MicroRNAs and Primary Biliary Cholangitis
Broad Roles of MicroRNAs in Various Diseases
MiRNAs are evolutionarily conserved, non-coding small RNAs of 18–25 nucleotides in length. MiRNAs incompletely bind to complementary sequences in the 3′ untranslated region (3′UTR) of messenger RNA (mRNA) and regulate the expression of target genes at the post-transcriptional level by promoting the degradation of mRNA or repressing its translation (Tavasolian et al., 2018). MiRNAs can regulate about 90% of protein-coding genes and play important roles in various biological processes such as metabolism, cell differentiation, proliferation, apoptosis, and the maintenance of immune homeostasis (Alvarez-Garcia and Miska, 2005; Baltimore et al., 2008; Check Hayden, 2008). Disturbances in miRNAs expression profiles are associated with a variety of human diseases, including autoimmune diseases, such as systemic lupus erythematosus (SLE), PBC, and rheumatoid arthritis (RA) (Young et al., 2017; Wang et al., 2018a; Erice et al., 2018). Some of them have been proposed as non-invasive biomarkers of disease.
As shown in Table 1, various miRNAs were dysregulated in PBC. These miRNAs can regulate target genes of cytokines, oxidative stress, immunity and inflammation-related molecules, thereby participating in the pathogenesis or/and progression of PBC (Padgett et al., 2009; Qian et al., 2013; Liang et al., 2016; Erice et al., 2018; Song et al., 2018). Several published literatures have extensively explored the molecular mechanisms of differentially expressed miRNAs in PBC, particularly regarding their altering effects on inflammation and autoimmunity (Liang et al., 2016; Nakagawa et al., 2017; Erice et al., 2018).
The Role of MiR-506 in the Pathogenesis of Primary Biliary Cholangitis
Anion exchanger 2 (AE2) is essential in the maintenance of the protective bicarbonate- rich umbrella on the surface of BECs via regulation of biliary HCO3− secretion, which shields BECs from noxious luminal bile acids (Rodrigues et al., 2018). Banales et al. (2012) demonstrated that miR-506 is upregulated in cholangiocytes from PBC patients, binds the 3′UTR region of AE2 mRNA and prevents protein translation, resulting in diminished AE2 activity and impaired biliary secretory functions. Given the putative pathogenic role of decreased AE2 in PBC, miR-506 may constitute a potential therapeutic target for this disease. Furthermore, miR-506 also regulates other genes involved in maintaining the integrity of bicarbonate umbrellas, the type III inositol 1,4,5-triphosphate receptor, an important regulator of calcium release from cholangiocytes (Ananthanarayanan et al., 2015; Erice et al., 2018). Under physiological conditions, acetylcholine increases the level of inositol triphosphate (InsP3) in cholangiocytes, resulting in an increase in the level of cytoplasmic Ca2+. Apical Cl− secretion is further stimulated by the Ca2+ activated Cl− channel transmembrane protein 16F (TMEM16A), ultimately leading to bicarbonate secretion through AE2 (Minagawa et al., 2007). The downregulation of InsP3R3 expression in cholangiocytes from PBC patients leads to decreased intracellular Ca2+ signaling and bicarbonate secretion, thereby triggering cholestasis (Shibao et al., 2003). InsP3R3 mRNA contains two highly conserved miR-506 binding sites, both of which are functional. In miR-506-overexpressed cholangiocytes, InsP3R3 mRNA and protein levels were reduced, resulting in a marked reduction in Ca2+ release from the endoplasmic reticulum and failure of bile secretion (Ananthanarayanan et al., 2015). In vitro data demonstrate that upregulation of proinflammatory, profibrotic, and senescent markers in miR-506-overexpressing cholangiocytes results in increased cellular stress and increased sensitivity to toxic hydrophobic bile acids (Erice et al., 2018). These results suggest a mechanistic link between epigenetic regulation, cellular damage, and immune dysregulation in PBC. Banales’ team further expiored the role of inflammatory factors, such as interleukins (IL)-1β, IL-6, IL-8, IL-12, IL-17, IL-18, tumor necrosis factor alpha (TNF-α) and interferon gamma (IFN-γ), transforming growth factor beta 1 (TGF-β1), estrogens (17β-estradiol, 17β-E2), bile acids [cholic acid (CA), UDCA and tauroursodeoxycholic (TUCA)] and other factors in regulating miR-506 expression in cholangiocytes and the role of miR-506 in cholangiocyte pathophysiology and immunomodulation in PBC. The result show different inflammatory factors enhance the expression of miR-506 in biliary epithelial cells. MiR-506 induces PBC-like features in cholangiocytes and promotes immune activation (Erice et al., 2018). Figure 2. Intriguingly, miR-506 is an X- linked miRNA localized to Xq27.3, which helps explain the possibility that females predominate in PBC disease, although whether this hypothesis applies to human remains to be demonstrated (Arora et al., 2013).
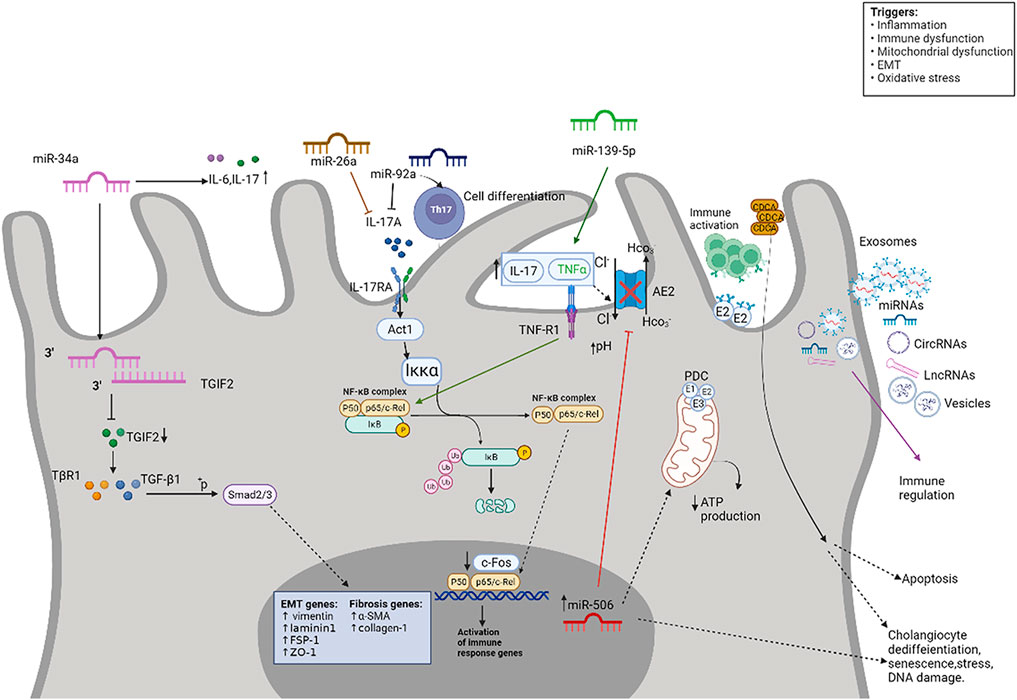
FIGURE 2. Signaling pathway of miRNAs in PBC. MiRNA are involved in regulating inflammation and autoimmunity. Some miRNAs are encapsulated in nanovesicles and exert critical effects on inflammatory and immune cells. miRNAs participate in PBC inflammation and autoimmune disorders primarily through NF-κB, TGF-β1, Th17, and so on.
Expression Profiles of MicroRNAs in Peripheral Blood and Liver Tissue of Primary Biliary Cholangitis Patients
Liang et al. (2016) used microarrays to identify 16 differentially expressed miRNAs (9 miRNAs upregulated and 7 miRNAs downregulated) in plasma from 3 PBC patients and 3 healthy controls. The most prominent finding was the downregulation of miR-92a, and the expression of miR-92a was negatively correlated with the Th17 cell population. Furthermore, the expression of miR-92a was colocalized with IL-17A in peripheral blood mononuclear cells (PBMCs) of patients, implying a direct regulation of IL-17A by miR-92a. Notably, studies have shown that Th17 cells and the IL-17 secreted by the cells may induce epithelial-mesenchymal transition (EMT) in intrahepatic bile ducts through IL-17A-IL-17RA-Act1 activation of the NF-κB pathway, and participate in the progression of PBC disease (Huang et al., 2016). Figure 2. Domestic studies have found that there are differences in the expression of miR-26a in initial CD4+ T cells, memory CD4+ T cells and effector CD4+ T cells of PBC patients, and no expression in Jurkat cells (human T cell line). The authors then cultured Th17 cells (derived from human CD4+ T cells) and transfected pre-miR-26a and anti-miR-26a, respectively. The results showed that compared with the normal control, the cells transfected with pre-miR-26a significantly decreased IL-17, significantly decreased the activities of NF-κB and AP-1, and induced increased apoptosis after activation; transfected with anti-miR-26a cells, IL-17 mRNA, NF-κB and AP-1 activities were significantly increased, cell proliferation activity increased, and apoptosis induced by activation decreased. The luciferase reporter further demonstrated that miRNA-26a could indeed regulate IL-17. Therefore, this study shows that miRNA-26a regulates the function of Thl7 cells by affecting apoptosis and cell proliferation activities induced by IL-17 activation (Tang, 2009). Figure 2. The above studies demonstrate that miRNAs may play a significant role in the fibrosis of bile duct epithelial cells in PBC patients through IL-17.
Pan et al. (2021) found that miR-34a was significantly overexpressed in the serum of PBC patients (30 PBC vs.30 controls). miR-34a upregulation inhibits proliferation of intrahepatic bile duct epithelium and increases mesenchymal markers zonula occluden-1 (ZO-1), laminin 1, vimentin and fibroblast-specific protein 1 (FSP-1) and expression of fibrotic markers α-SMA, and collagen I. The authors further demonstrated that miR-34a targets TGIF2 to induce EMT and fibrosis in intrahepatic bile duct epithelium through the TGF-β1/smad pathway (Pan et al., 2021). Figure 2. MiR-139-5p was significantly downregulated in clinically advanced PBC compared to control. In situ hybridization and RT-qPCR showed that the expression of miR-139-5p in lymphocytes of PBC patients was higher than that of lymphocytes of other liver diseases (chronic viral hepatitis and autoimmune hepatitis), while miR-139-5p in hepatocytes of PBC was lower than that in other liver disease, suggesting that lymphocytes are the main source of miR-139-5p. In vitro studies showed that with the upregulation of miR-139-5p, the level of TNF-α in the cell supernatant was significantly increased, the transcription of the c-FOS gene was inhibited, and the NF-κB signaling pathway was finally activated. Activation of NF-κB further induces the production of TNF-α, and increased levels of TNF-α may accelerate bile duct injury through positive feedback. The authors revealed a novel inflammation-regulatory mechanism between TNF-α and c-FOS transcription through miR-139-5p in the NF-κB pathway (Katsumi et al., 2016). Figure 2. Therefore, miR-139-5p could become not only a biomarker of disease progression, but also a novel therapeutic target for patients with progressive PBC. In addition, the expression of miR-155 was enhanced in PBMCs and liver tissues of PBC patients, accompanied by vitamin D receptor (VDR) mRNA and protein, cytokine signaling inhibitor 1 (SOCS1) protein expression decreased, indicating that the decreased VDR expression may lead to the dysregulation of the negative feedback loop through the VDR-miRNA155-SOCS1 pathway, thereby triggering a sustained inflammatory response (Kempinska-Podhorodecka et al., 2017). Padgett et al. (2009) analyzed liver tissue from 6 patients with end-stage PBC and 5 control subjects using a miRNA microarray platform. The results showed that 35 differentially expressed miRNAs (11 upregulated and 24 downregulated) were identified in the liver tissue of PBC patients compared with the liver tissue of normal controls. The targets they predicted were associated with the regulation of cell proliferation, apoptosis, inflammation, oxidative stress, and metabolism. According to the above studies, we can conclude that miRNAs may be derived from various immune cells and play a significant effect in the occurrence and development of PBC by inflammatory factors and immune-inflammatory pathways (such as TGF-β1/smad, TCR, NF-κB, and so on). Subsequent studies on miRNAs and PBC can start from immune cells and immune-inflammation-related pathways. Subsequent studies on miRNAs and PBC can start from immune cells (not only B cells, T cells, but also Th17 cells, Treg cells, macrophages, etc.), inflammatory factors and immune-inflammation-related pathways.
MicroRNAs and Therapeutic Targets for Primary Biliary Cholangitis
Nakagawa et al. (2017) examined total RNAs of CD4+ T cells from 6 PBC patients and 6 healthy controls using miRNA microarrays. The authors found that the expression levels of 16 miRNAs were significantly different in PBC patients compared with healthy controls (p < 0.05, fold change >1.2). Among them, five of these miRNAs were significantly downregulated in PBC patients by qRT-PCR. The integral analysis of miRNA and mRNA identified four significantly downregulated miRNAs (miR-181a, miR-181b, miR-374b, and miR-425) related to the T-cell receptor (TCR) signaling pathway in CD4+ T cells of PBC. N-Ras, a regulator of the TCR signaling pathway, was found to be targeted by all four identified miRNAs. In addition, in vitro assays confirmed that decreased miR-425 strongly induced inflammatory cytokines (IL-2 and IFN-γ) via N-Ras upregulation in the TCR signaling pathway. Therefore, the restoration of decreased miR-425 or the suppression of N-Ras may be promising for an immunotherapeutic strategy against PBC.
The authors believe that miR-122 is the most noteworthy therapeutic target for PBC. MiR-122 is a conserved liver-specific miRNA, accounting for 70% of total liver miRNAs (Chang et al., 2008). Multiple studies have shown that miR-122 plays a key role in lipid metabolism (Esau et al., 2006), cell differentiation (Kim et al., 2011), liver polyploidy (Hsu et al., 2016), hepatitis C virus replication (Luna et al., 2015), acetaminophen toxicity (Chowdhary et al., 2017; Yang et al., 2021), liver fibrosis in innate immunity of hepatocytes (Xu et al., 2019). Decreased miR-122 expression was found in HCV-negative liver cancer and was associated with metastasis in hepatocellular carcinoma (HCC) patients (Coulouarn et al., 2009; Tsai et al., 2009).
Tan et al. (2014) analyzed miRNA expression by Illumina sequencing of serum samples from 3 PBC patients and 3 controls and assessed the expression of selected miRNAs in a screened group (n = 40) by qRT-PCR. A logistic regression model was then constructed using the training cohort (n = 192) and validated with another cohort (n = 142). The results showed that serum miR-122-5p levels were elevated in PBC patients. Compared with ALP and ANA, the miRNA panel (hsa-miR-122-5p, hsa-miR-141-3p, and hsa-miR-26b-5p) was a more sensitive and specific biomarker for PBC (Tan et al., 2014). Circulating miR-122 levels have been reported to correlate with liver histological stage, inflammation grade, and ALT activity (Lewis and Jopling, 2010; Zhang et al., 2010; Hu et al., 2012; Wang et al., 2012; Iino et al., 2013). MiR-122 expression was significantly decreased in a carbon tetrachloride (CCl4)-induced mouse model of liver fibrosis and HCC (Li et al., 2013; Hayes and Chayama, 2016). Similarly, Padgett et al. (2009) used the miRNA array platform to analyze the liver tissue of 6 patients with end-stage PBC and 5 control subjects, and the results showed that miR-122 was also downregulated in the liver tissue of PBC patients. This is an interesting result. It means that the liver may overexpress miR-122 compensatory in the early stage of PBC. However, most of miR-122 reaches the peripheral circulation, which increases miR-122 in peripheral circulation in PBC patients; or in PBC patients, other tissues, organs or cells abnormally express miR-122 in addition to the liver. The above-mentioned known immune cells and inflammatory factors play an important role in the occurrence and development of PBC. Therefore, the authors speculate that in the peripheral blood of PBC patients, various immune cells are activated (such as T cells, B cells, Th cells, Treg cells, macrophages, etc.) abnormally expressing miR-122 increases the level of miR-122 in serum/plasma. MiR-122 is a negative regulator of liver fibrosis. Li et al. (2013) found that miR-122 inhibited the proliferation and activation of Lx2 in hepatic stellate cells (HSC) by targeting P4HA1, regulating collagen production, and inhibiting liver fibrosis.
Tsai et al. (2012) generated a mutant mouse strain with a germline deletion of Mir122a using homologous recombination (Mir122a−/−). Histological examination of the livers of Mir122a−/− mice revealed extensive lipid accumulation and reduced glycogen storage, as well as inflammation and fibrosis, compared with WT controls. A strong positive reaction to the anti-F4/80 antibody, which is specific for mouse macrophages and monocytes, was detected in the Mir122a−/− livers. Teng et al. (2020) also found that knockout of miR-122 in mouse liver developed spontaneous liver fibrosis. Therefore, the authors speculate that miR-122 may be a novel gene therapy strategy for patients with advanced PBC, especially targeting the liver. Whether the anti-fibrotic mechanism of miR-122 is related to its regulation of hepatic fatty acid and cholesterol synthesis still needs to be further explored (Esau et al., 2006; Naderi et al., 2017). In addition, due to the ability of miR-122 to enhance HCV replication, vigilance and monitoring of HCV infection should be exercised when using miR-122 (Luna et al., 2015). In the early stage of PBC, the main lesions are chronic inflammation and fibrosis of the intrahepatic bile ducts. Whether miR-122 can regulate the proliferation and apoptosis of intrahepatic bile duct cells has not been reported in the literature. This is also the direction our research group is working on.
Immunomodulatory Role of Exosomal MicroRNAs in Primary Biliary Cholangitis
Extracellular vesicles (EVs) are nano- or micro-lipid bilayer spheres produced by different cells. They are released into the extracellular space where they participate in intercellular communications. They are also found in bile and contain miRNAs (Li et al., 2014). Several nanovesicle-delivered miRNAs have been identified that are specifically expressed in PBC and play a modifying role in inflammation and autoimmunity (Olaizola et al., 2018). Exosomes are small vesicles formed by budding from endosomal membrane and released to extracellular by fusion with plasma membrane, which are important mediators of intercellular communication (Hirsova et al., 2016a; Martínez and Andriantsitohaina, 2017). Recent studies have reported that exosomes-mediated transfer of ncRNAs, proteins and lipids are associated with a variety of human diseases, including liver disease (Bala et al., 2012; Hirsova et al., 2016a; Debabrata and Mukhopadhyay, 2017; Pan et al., 2017; Szabo and Momen-Heravi, 2017). Hepatic epithelial cells, including cholangiocytes and hepatocytes, are exosomes-releasing cells (Masyuk et al., 2010; Masyuk et al., 2013; Hirsova et al., 2016b; Worst et al., 2017). Tomiyama et al. (2017) found plasma-derived exosomal miR-451a and miR-642a-3p were increased in PBC patients compared with healthy controls, and could regulate the expression of the co-stimulatory molecules such as CD86 and CD80 in peripheral antigen-presenting cells. Figure 2. In conclusion, accumulating evidence points to the critical role of miRNAs in regulating inflammation and autoimmunity, and many mature miRNAs are expected to be candidate biomarkers and therapeutic targets for PBC (Ninomiya et al., 2013; Qian et al., 2013; Yang, 2013; Katsushima et al., 2014; Tan et al., 2014; Sakamoto et al., 2016).
Long Non-Coding RNA and Primary Biliary Cholangitis
Expression Profiles of Long Non-Coding RNAs in Autoimmune Diseases
LncRNAs are highly conserved RNA sequences >200 nucleotides in length that can epigenetically regulate gene expression and broadly affect cellular biological processes (Mercer et al., 2009; Ponting et al., 2009). Published literature on lncRNA have been focused on cancer (Chan and Tay, 2018; Mitobe et al., 2018; Tian et al., 2018), and studies on lncRNA in innate immunity are relatively scarce, accounting for only about 4% of all lncRNA papers (Robinson et al., 2020). With increasing interest of lncRNA in autoimmune diseases, it has been found that different autoimmune diseases (including PBC) have specific lncRNA expression profiles in different cells and tissues (Zhang et al., 2013; Sigdel et al., 2015; Hur et al., 2019; Liang et al., 2019). Previous studies have shown that the lncRNA AK053349 is highly expressed in CD8+ T cells and is associated with autoimmunity and T lymphocyte activation (Pang et al., 2009). Zhang et al. (2013) wrote in his doctoral dissertation that the lncRNA AK053349 was increased in PBMC of PBC patients and positively correlated with the Mayo risk score, emphasizing its potential relevance to the pathogenesis of PBC and worthy of further study (Zhang et al., 2013). Another recent domestic study (She, 2020) found that the expression level of lncRNA XIST in NK cells and CD4+ T lymphocytes of PBC patients was significantly higher than that of healthy controls, and could be clearly located in the nucleus. The high expression of lncRNA XIST in Naïve CD4+ T cells of PBC patients can promote the proliferation of Naïve CD4+ T cells, stimulate the secretion of IFN-γ, IL-17, TGF-β and ROR-γ T cells, and increase the proportion of Th1 and Th7 cells, which led to the occurrence of PBC. This further supports the critical role of immune cells and inflammatory factors, especially IL-17 in PBC disease.
Exosomal Long Non-Coding RNA H19 Promotes Bile Duct Proliferation and Liver Fibrosis
Huiping Zhou et al. (Li et al., 2017) used Mdr2−/− mice as an animal model of cholestatic biliary disease. Their study showed that H19 expression was significantly increased in the liver and bile duct cells of female Mdr2−/− mice compared with male Mdr2−/− mice, and that abnormal H19 expression was associated with the severity of biliary fibrosis in female Mdr2−/− mice. Knockdown of H19 alleviate cholestatic liver injury in female Mdr2−/− mice. Furthermore, both Taurocholic acid (TCA) and Estradiol 2 (E2) upregulated the expression of H19, but there was no superposition or synergy. The role of H19 in cholestatic injury in female Mdr2−/− mice may be related to extracellular regulated protein kinase 1/2 (ERK1/2) signaling pathway. In a follow up study, Huiping Zhou’s team further demonstrated that exosomal H19 is derived from bile duct cells and transferred to hepatocytes, inhibit the expression of small heterodimeric partner (SHP) in hepatocytes and promote cholestatic injury (Li et al., 2018a). Figure 3. To further determine the effect of H19 expression in the progression of liver fibrosis, the authors examined the expression levels of hepatic H19, Ck19, and fibrosis marker genes (Acta2, Loxl2, and Collagen 1) in both male and female 2-week bile duct ligation (BDL) mice and in 100 day old female Mdr2−/− mice and perform a linear analysis. The results showed that the expression level of hepatic H19 was significantly positively correlated with Ck19 and fibrosis marker genes. Similarly, in primary sclerosing cholangitis (PSC) and PBC patients, the hepatic mRNA levels of H19, CK19, and fibrosis marker genes were all increased. In BDL mice with H19 knockout (H19KO-BDL), collagen deposition and α-SMA-positive fibroblast distribution near the bile ducts of mice were significantly reduced. H19 deficiency also significantly alleviated serum AST, ALT, ALP, and total bile acid (TBA) levels induced by BDL. H19 deficiency in DKO (Mdr2 and H19 double knockout) mice significantly reduced bile duct proliferation, immune cell infiltration and fibrosis in the periportal area. Next, the authors isolated exosomes from H19-enriched and H19-free (control) cholangiocytel culture medium and injected mice via tail vein. The results showed that bile duct proliferation, hepatic inflammation, collagen deposition, and fibroblast activation were more severe in mice treated with H19-enriched exosomes when compared to those treated with control exosomes. This effect is related to the promotion of hepatic stellate cell (HSC) activation and proliferation by exosomal H19 (Liu et al., 2019). Furthermore, cholangiocyte-derived exosomal H19 promotes macrophage activation, differentiation, chemotaxis and liver inflammation through the CCL-2/CCR-2 signaling pathway. Figure 3. H19-deficiency ameliorates the liver cholestasis and macrophage activation in both BDL and Mdr2−/− Mice (Li et al., 2020a). Thus, exosomal H19 represents a noninvasive biomarker and potential therapeutic target for cholestatic disease. Notably, Mdr2−/− mice are actually a suitable model for PSC and not an animal model for PBC disease. What role lncRNA H19 plays in PBC disease and whether the molecular mechanism is the same remains to be discussed.
Circular RNAs and Primary Biliary Cholangitis
CircRNA is an endogenous non-coding RNA, the most representative characteristic of which is the covalently closed RNA circle (Salzman, 2016; Ouyang et al., 2017). Circularization results in resistance and high stability of RNA to exonuclease-mediated degradation. In addition, circRNAs are abundant and evolutionarily conserved in the cytoplasm. These properties make circRNAs potentially more suitable as clinical biomarkers than other types of RNAs (Jeck and Sharpless, 2014; Lasda and Parker, 2014; Li et al., 2015). Most importantly, the expression profiles of circRNAs are specific in different cell types and can generally be found in peripheral blood, exosomes, and tissues. Due to their various forms of epigenetic modifications, circRNAs play important roles in various diseases, such as cancer, neurologic disorders and cardiovascular diseases (Zhao et al., 2017; Yang et al., 2018a; Ojha et al., 2018; Sheng et al., 2018). Ma et al. (2019) showed that circARSP91 promotes cancer immune surveillance by regulating NK cells in liver cancer, suggesting a key role of circRNAs in tumor immunity. Furthermore, circRNA Malat-1 is thought to act as a key regulator of alloimmune rejection by promoting dendritic cell-induced T cell exhaustion and regulatory T cell generation, suggesting a critical role for circRNAs in adaptive immunity (Zhang et al., 2018). In conclusion, circRNAs play key roles not only in innate immunity but also in adaptive immunity.
In recent years, accumulated studies have shown that circRNAs are closely related to the occurrence and development of autoimmune diseases (Yang et al., 2018b), including SLE (Li et al., 2018b; Wang et al., 2018b), RA (Zheng et al., 2017b), PBC (Zheng et al., 2017a), etc. Zheng et al. (2017a) used microarray to identify 22 aberrantly expressed circRNAs (18 upregulated, 4 downregulated) in the plasma of PBC patients. Notably, PBC patients who did not receive UDCA had higher levels of hsa-circ-402458 than those who received UDCA. Hsa-circ-402458 may target hsa-miR-943 and hsa-miR-522-3p. For miR-522-3p, it may be an effective target for regulating chronic inflammatory diseases. Therefore, the authors speculate that hsa-circ-402458 may act as a miRNA sponge to regulate inflammation-related pathways, thereby promoting the development of PBC. Unfortunately, there are few studies on the relationship between circRNAs and PBC, and the molecular mechanism behind the regulation of circRNAs in PBC disease is still unclear. Whether circRNAs, like miRNAs and/or lncRNAs, play a role in PBC through signaling pathways such as TGF-β, NF-κB, TLR, TCR and oxidative stress, remains to be further explored.
Conclusion and Future Directions
Studies on ncRNAs in human biology have gained much interest in the scientific world in recent years (Kapoor et al., 2021; Makkos et al., 2021; Wang et al., 2021). The role of ncRNAs in immune regulation, inflammation and autoimmunity can be of significant translational implication in medicine. Although specific expression profiles of miRNAs, lnRNAs, and cirRNAs have been well-documented in the literature (Padgett et al., 2009; Sigdel et al., 2015; Zheng et al., 2017a), the underlying mechanisms of ncRNAs in the development of PBC is unclear and may involve autoimmune regulatory pathways such as TGF-B1, NF-kB, Th17, and TCR. Furthermore, the effects of ncRNAs in oxidative stress, apoptosis, immune cells homing, and others in PBC are also confounding factors. Further studies including a combination of wet-bench studies and the use of bioinformatics tools (Li et al., 2020b; Zhu and Leung, 2021) to discover the target gene networks of non-coding RNAs are necessary to decipher the mechanistic role of ncRNAs in the pathogenesis of PBC and their potential application as diagnostic markers and/or therapeutic checkpoints.
Author Contributions
YZ wrote the manuscript, and ZJ assisted in the drawing. MC, BS, and ZS revised the manuscript.
Funding
This work was supported by the Natural Foundation of Anhui Province (NO.1808085MH279).
Conflict of Interest
The authors declare that the research was conducted in the absence of any commercial or financial relationships that could be construed as a potential conflict of interest.
Publisher’s Note
All claims expressed in this article are solely those of the authors and do not necessarily represent those of their affiliated organizations, or those of the publisher, the editors and the reviewers. Any product that may be evaluated in this article, or claim that may be made by its manufacturer, is not guaranteed or endorsed by the publisher.
References
Afonso, M. B., Rodrigues, P. M., Simão, A. L., Gaspar, M. M., Carvalho, T., Borralho, P., et al. (2018). miRNA-21 Ablation Protects against Liver Injury and Necroptosis in Cholestasis. Cell Death Differ. 25 (5), 857–872. doi:10.1038/s41418-017-0019-x
Alvarez-Garcia, I., and Miska, E. A. (2005). MicroRNA Functions in Animal Development and Human Disease. Development 132 (21), 4653–4662. doi:10.1242/dev.02073
Ananthanarayanan, M., Banales, J. M., Guerra, M. T., Spirli, C., Munoz-Garrido, P., Mitchell-Richards, K., et al. (2015). Post-Translational Regulation of the Type III Inositol 1,4,5-trisphosphate Receptor by miRNA-506. J. Biol. Chem. 290 (1), 184–196. doi:10.1074/jbc.M114.587030
Arora, H., Qureshi, R., and Park, W.-Y. (2013). miR-506 Regulates Epithelial Mesenchymal Transition in Breast Cancer Cell Lines. PLoS One 8 (5), e64273. doi:10.1371/journal.pone.0064273
Bala, S., Petrasek, J., Mundkur, S., Catalano, D., Levin, I., Ward, J., et al. (2012). Circulating microRNAs in Exosomes Indicate Hepatocyte Injury and Inflammation in Alcoholic, Drug-Induced, and Inflammatory Liver Diseases. Hepatology 56 (5), 1946–1957. doi:10.1002/hep.25873
Baltimore, D., Boldin, M. P., Rao, R. M. R. M., O'ConnellRao, D. S., and Taganov, K. D. (2008). MicroRNAs: New Regulators of Immune Cell Development and Function. Nat. Immunol. 9 (8), 839–845. doi:10.1038/ni.f.209
Banales, J. M., Sáez, E., Úriz, M., Sarvide, S., Urribarri, A. D., Splinter, P., et al. (2012). Upregulation of microRNA 506 Leads to Decreased Cl−/HCO3− Anion Exchanger 2 Expression in Biliary Epithelium of Patients with Primary Biliary Cirrhosis. Hepatology 56 (2), 687–697. doi:10.1002/hep.25691
Beuers, U., Gershwin, M. E., Gish, R. G., Invernizzi, P., Jones, D. E. J., Lindor, K., et al. (2015). Changing Nomenclature for PBC: From 'cirrhosis' to 'cholangitis'. J. Hepatology 63 (5), 1285–1287. doi:10.1016/j.jhep.2015.06.031
Carey, E. J., Ali, A. H., and Lindor, K. D. (2015). Primary Biliary Cirrhosis. Lancet 386 (10003), 1565–1575. doi:10.1016/s0140-6736(15)00154-3
Chan, J., and Tay, Y. (2018). Noncoding RNA:RNA Regulatory Networks in Cancer. Ijms 19 (5), 1310. doi:10.3390/ijms19051310
Chang, J., Guo, J.-T., Jiang, D., Guo, H., Taylor, J. M., and Block, T. M. (2008). Liver-specific microRNA miR-122 Enhances the Replication of Hepatitis C Virus in Nonhepatic Cells. J. Virol. 82 (16), 8215–8223. doi:10.1128/jvi.02575-07
Check Hayden, E. (2008). Thousands of Proteins Affected by miRNAs. Nature 454 (7204), 562. doi:10.1038/454562b
Chen, J.-Q., Papp, G., Szodoray, P., and Zeher, M. (2016). The Role of microRNAs in the Pathogenesis of Autoimmune Diseases. Autoimmun. Rev. 15 (12), 1171–1180. doi:10.1016/j.autrev.2016.09.003
Chowdhary, V., Teng, K.-y., Thakral, S., Zhang, B., Lin, C.-h., Wani, N., et al. (2017). miRNA-122 Protects Mice and Human Hepatocytes from Acetaminophen Toxicity by Regulating Cytochrome P450 Family 1 Subfamily A Member 2 and Family 2 Subfamily E Member 1 Expression. Am. J. Pathology 187 (12), 2758–2774. doi:10.1016/j.ajpath.2017.08.026
Coulouarn, C., Factor, V. M., Andersen, J. B., Durkin, M. E., and Thorgeirsson, S. S. (2009). Loss of miR-122 Expression in Liver Cancer Correlates with Suppression of the Hepatic Phenotype and Gain of Metastatic Properties. Oncogene 28 (40), 3526–3536. doi:10.1038/onc.2009.211
Dai, Y., Sui, W., Lan, H., Yan, Q., Huang, H., and Huang, Y. (2009). Comprehensive Analysis of microRNA Expression Patterns in Renal Biopsies of Lupus Nephritis Patients. Rheumatol. Int. 29 (7), 749–754. doi:10.1007/s00296-008-0758-6
Debabrata, J. M., and Mukhopadhyay, D. (2017). Exosomes and Their Role in the Micro-/macro-environment: a Comprehensive Review. J. Biomed. Res. 31 (5), 386–394. doi:10.7555/jbr.30.20150162
Erice, O., Munoz-Garrido, P., Vaquero, J., Perugorria, M. J., Fernandez-Barrena, M. G., Saez, E., et al. (2018). MicroRNA-506 Promotes Primary Biliary Cholangitis-like Features in Cholangiocytes and Immune Activation. Hepatology 67 (4), 1420–1440. doi:10.1002/hep.29533
Esau, C., Davis, S., Murray, S. F., Yu, X. X., Pandey, S. K., Pear, M., et al. (2006). miR-122 Regulation of Lipid Metabolism Revealed by In Vivo Antisense Targeting. Cell Metab. 3 (2), 87–98. doi:10.1016/j.cmet.2006.01.005
Gonzalez, R. S., and Washington, K. (2018). Primary Biliary Cholangitis and Autoimmune Hepatitis. Surg. Pathol. Clin. 11 (2), 329–349. doi:10.1016/j.path.2018.02.010
Gulamhusein, A. F., and Hirschfield, G. M. (2020). Primary Biliary Cholangitis: Pathogenesis and Therapeutic Opportunities. Nat. Rev. Gastroenterol. Hepatol. 17 (2), 93–110. doi:10.1038/s41575-019-0226-7
Hayes, C., and Chayama, K. (2016). MicroRNAs as Biomarkers for Liver Disease and Hepatocellular Carcinoma. Ijms 17 (3), 280. doi:10.3390/ijms17030280
Hirsova, P., Ibrahim, S. H., Krishnan, A., Verma, V. K., Bronk, S. F., Werneburg, N. W., et al. (2016). Lipid-Induced Signaling Causes Release of Inflammatory Extracellular Vesicles from Hepatocytes. Gastroenterology 150 (4), 956–967. doi:10.1053/j.gastro.2015.12.037
Hirsova, P., Ibrahim, S. H., Verma, V. K., Morton, L. A., Shah, V. H., LaRusso, N. F., et al. (2016). Extracellular Vesicles in Liver Pathobiology: Small Particles with Big Impact. Hepatology 64 (6), 2219–2233. doi:10.1002/hep.28814
Hsu, S. H., Delgado, E. R., Otero, P. A., Teng, K. Y., Kutay, H., Meehan, K. M., et al. (2016). MicroRNA‐122 Regulates Polyploidization in the Murine Liver. Hepatology 64 (2), 599–615. doi:10.1002/hep.28573
Hu, J., Xu, Y., Hao, J., Wang, S., Li, C., and Meng, S. (2012). MiR-122 in Hepatic Function and Liver Diseases. Protein Cell 3 (5), 364–371. doi:10.1007/s13238-012-2036-3
Huang, Q., Chu, S., Yin, X., Yu, X., Kang, C., Li, X., et al. (2016). Interleukin-17A-Induced Epithelial-Mesenchymal Transition of Human Intrahepatic Biliary Epithelial Cells: Implications for Primary Biliary Cirrhosis. Tohoku J. Exp. Med. 240 (4), 269–275. doi:10.1620/tjem.240.269
Hur, K., Kim, S.-H., and Kim, J.-M. (2019). Potential Implications of Long Noncoding RNAs in Autoimmune Diseases. Immune Netw. 19 (1), e4. doi:10.4110/in.2019.19.e4
Iino, I., Kikuchi, H., Miyazaki, S., Hiramatsu, Y., Ohta, M., Kamiya, K., et al. (2013). Effect of miR-122 and its Target Gene Cationic Amino Acid Transporter 1 on Colorectal Liver Metastasis. Cancer Sci. 104 (5), 624–630. doi:10.1111/cas.12122
Jeck, W. R., and Sharpless, N. E. (2014). Detecting and Characterizing Circular RNAs. Nat. Biotechnol. 32 (5), 453–461. doi:10.1038/nbt.2890
Kapoor, P., Chowdhry, A., Bagga, D. K., Bhargava, D., and Aishwarya, S. (2021). MicroRNAs in Oral Fluids (Saliva and Gingival Crevicular Fluid) as Biomarkers in Orthodontics: Systematic Review and Integrated Bioinformatic Analysis. Prog. Orthod. 22 (1), 31. doi:10.1186/s40510-021-00377-1
Katsumi, T., Ninomiya, M., Nishina, T., Mizuno, K., Tomita, K., Haga, H., et al. (2016). MiR-139-5p Is Associated with Inflammatory Regulation through C-FOS Suppression, and Contributes to the Progression of Primary Biliary Cholangitis. Lab. Invest. 96 (11), 1165–1177. doi:10.1038/labinvest.2016.95
Katsushima, F., Takahashi, A., Sakamoto, N., Kanno, Y., Abe, K., and Ohira, H. (2014). Expression of Micro-RNAs in Peripheral Blood Mononuclear Cells from Primary Biliary Cirrhosis Patients. Hepatol. Res. 44 (10), E189–E197. doi:10.1111/hepr.12198
Kempinska-Podhorodecka, A., Milkiewicz, M., Wasik, U., Ligocka, J., Zawadzki, M., Krawczyk, M., et al. (2017). Decreased Expression of Vitamin D Receptor Affects an Immune Response in Primary Biliary Cholangitis via the VDR-miRNA155-SOCS1 Pathway. Ijms 18 (2), 289. doi:10.3390/ijms18020289
Kim, N., Kim, H., Jung, I., Kim, Y., Kim, D., and Han, Y.-M. (2011). Expression Profiles of miRNAs in Human Embryonic Stem Cells during Hepatocyte Differentiation. Hepatol. Res. 41 (2), 170–183. doi:10.1111/j.1872-034X.2010.00752.x
Kim, W. R., Lindor, K. D., Locke, G. R., Therneau, T. M., Homburger, H. A., Batts, K. P., et al. (2000). Epidemiology and Natural History of Primary Biliary Cirrhosis in a U.S. Community. Gastroenterology 119 (6), 1631–1636. doi:10.1053/gast.2000.20197
Koulentaki, M., Mantaka, A., Sifaki-Pistolla, D., Thalassinos, E., Tzanakis, N., and Kouroumalis, E. (2014). Geoepidemiology and Space-Time Analysis of Primary Biliary Cirrhosis in Crete, Greece. Liver Int. 34 (7), e200–e207. doi:10.1111/liv.12479
Kouroumalis, E., Samonakis, D., and Voumvouraki, A. (2018). Biomarkers for Primary Biliary Cholangitis: Current Perspectives. Hmer 10, 43–53. doi:10.2147/hmer.s135337
Lasda, E., and Parker, R. (2014). Circular RNAs: Diversity of Form and Function. Rna 20 (12), 1829–1842. doi:10.1261/rna.047126.114
Lewis, A. P., and Jopling, C. L. (2010). Regulation and Biological Function of the Liver-specific miR-122. Biochem. Soc. Trans. 38 (6), 1553–1557. doi:10.1042/bst0381553
Li, B., Dong, J., Yu, J., Fan, Y., Shang, L., Zhou, X., et al. (2020). Pinpointing miRNA and Genes Enrichment over Trait-Relevant Tissue Network in Genome-wide Association Studies. BMC Med. Genomics 13 (Suppl. 11), 191. doi:10.1186/s12920-020-00830-w
Li, J., Ghazwani, M., Zhang, Y., Lu, J., Li, J., Fan, J., et al. (2013). miR-122 Regulates Collagen Production via Targeting Hepatic Stellate Cells and Suppressing P4HA1 Expression. J. Hepatology 58 (3), 522–528. doi:10.1016/j.jhep.2012.11.011
Li, L.-J., Zhu, Z.-W., Zhao, W., Tao, S.-S., Li, B.-Z., Xu, S.-Z., et al. (2018). Circular RNA Expression Profile and Potential Function of Hsa_circ_0045272 in Systemic Lupus Erythematosus. Immunology 155 (1), 137–149. doi:10.1111/imm.12940
Li, L., Masica, D., Ishida, M., Tomuleasa, C., Umegaki, S., Kalloo, A. N., et al. (2014). Human Bile Contains microRNA-Laden Extracellular Vesicles that Can Be Used for Cholangiocarcinoma Diagnosis. Hepatology 60 (3), 896–907. doi:10.1002/hep.27050
Li, P., Chen, S., Chen, H., Mo, X., Li, T., Shao, Y., et al. (2015). Using Circular RNA as a Novel Type of Biomarker in the Screening of Gastric Cancer. Clin. Chim. Acta 444, 132–136. doi:10.1016/j.cca.2015.02.018
Li, X., Liu, R., Huang, Z., Gurley, E. C., Wang, X., Wang, J., et al. (2018). Cholangiocyte-derived Exosomal Long Noncoding RNA H19 Promotes Cholestatic Liver Injury in Mouse and Humans. Hepatology 68 (2), 599–615. doi:10.1002/hep.29838
Li, X., Liu, R., Wang, Y., Zhu, W., Zhao, D., Wang, X., et al. (2020). Cholangiocyte-Derived Exosomal lncRNA H19 Promotes Macrophage Activation and Hepatic Inflammation under Cholestatic Conditions. Cells 9 (1), 190. doi:10.3390/cells9010190
Li, X., Liu, R., Yang, J., Sun, L., Zhang, L., Jiang, Z., et al. (2017). The Role of Long Noncoding RNA H19 in Gender Disparity of Cholestatic Liver Injury in Multidrug Resistance 2 Gene Knockout Mice. Hepatology 66 (3), 869–884. doi:10.1002/hep.29145
Liang, D.-Y., Hou, Y.-Q., Luo, L.-J., and Ao, L. (2016). Altered Expression of miR-92a Correlates with Th17 Cell Frequency in Patients with Primary Biliary Cirrhosis. Int. J. Mol. Med. 38 (1), 131–138. doi:10.3892/ijmm.2016.2610
Liang, J., Chen, W., and Lin, J. (2019). LncRNA: An All-Rounder in Rheumatoid Arthritis. J. Transl. Int. Med. 7 (1), 3–9. doi:10.2478/jtim-2019-0002
Liu, R., Li, X., Zhu, W., Wang, Y., Zhao, D., Wang, X., et al. (2019). Cholangiocyte‐Derived Exosomal Long Noncoding RNA H19 Promotes Hepatic Stellate Cell Activation and Cholestatic Liver Fibrosis. Hepatology 70 (4), 1317–1335. doi:10.1002/hep.30662
Lleo, A., Jepsen, P., Morenghi, E., Carbone, M., Moroni, L., Battezzati, P. M., et al. (2016). Evolving Trends in Female to Male Incidence and Male Mortality of Primary Biliary Cholangitis. Sci. Rep. 6, 25906. doi:10.1038/srep25906
Luna, J. M., Scheel, T. K. H., Danino, T., Shaw, K. S., Mele, A., Fak, J. J., et al. (2015). Hepatitis C Virus RNA Functionally Sequesters miR-122. Cell 160 (6), 1099–1110. doi:10.1016/j.cell.2015.02.025
Lv, T., Chen, S., Li, M., Zhang, D., Kong, Y., and Jia, J. (2021). Regional Variation and Temporal Trend of Primary Biliary Cholangitis Epidemiology: A Systematic Review and Meta‐analysis. J. Gastroenterology Hepatology 36 (6), 1423–1434. doi:10.1111/jgh.15329
Ma, Y., Zhang, C., Zhang, B., Yu, H., and Yu, Q. (2019). circRNA of AR-suppressed PABPC1 91 B-p E-nhances the C-ytotoxicity of N-atural K-iller C-ells against H-epatocellular C-arcinoma via U-pregulating UL16 B-inding P-rotein 1. Oncol. Lett. 17 (1), 388–397. doi:10.3892/ol.2018.9606
Makkos, A., Ágg, B., Petrovich, B., Varga, Z. V., Görbe, A., and Ferdinandy, P. (2021). Systematic Review and Network Analysis of microRNAs Involved in Cardioprotection against Myocardial Ischemia/reperfusion Injury and Infarction: Involvement of Redox Signalling. Free Radic. Biol. Med. 172, 237–251. doi:10.1016/j.freeradbiomed.2021.04.034
Marschall, H.-U., Henriksson, I., Lindberg, S., Söderdahl, F., Thuresson, M., Wahlin, S., et al. (2019). Incidence, Prevalence, and Outcome of Primary Biliary Cholangitis in a Nationwide Swedish Population-Based Cohort. Sci. Rep. 9 (1), 11525. doi:10.1038/s41598-019-47890-2
Martínez, M. C., and Andriantsitohaina, R. (2017). Extracellular Vesicles in Metabolic Syndrome. Circ. Res. 120 (10), 1674–1686. doi:10.1161/circresaha.117.309419
Masyuk, A. I., Huang, B. Q., Ward, C. J., Gradilone, S. A., Banales, J. M., Masyuk, T. V., et al. (2010). Biliary Exosomes Influence Cholangiocyte Regulatory Mechanisms and Proliferation through Interaction with Primary Cilia. Am. J. Physiology-Gastrointestinal Liver Physiology 299 (4), G990–G999. doi:10.1152/ajpgi.00093.2010
Masyuk, A. I., Masyuk, T. V., and Larusso, N. F. (2013). Exosomes in the Pathogenesis, Diagnostics and Therapeutics of Liver Diseases. J. Hepatology 59 (3), 621–625. doi:10.1016/j.jhep.2013.03.028
Mercer, T. R., Dinger, M. E., and Mattick, J. S. (2009). Long Non-coding RNAs: Insights into Functions. Nat. Rev. Genet. 10 (3), 155–159. doi:10.1038/nrg2521
Metcalf, J., Bhopal, R., Gray, J., Howel, D., and James, O. (1997). Incidence and Prevalence of Primary Biliary Cirrhosis in the City of Newcastle upon Tyne, England. Int. J. Epidemiol. 26 (4), 830–836. doi:10.1093/ije/26.4.830
Minagawa, N., Nagata, J., Shibao, K., Masyuk, A. I., Gomes, D. A., Rodrigues, M. A., et al. (2007). Cyclic AMP Regulates Bicarbonate Secretion in Cholangiocytes through Release of ATP into Bile. Gastroenterology 133 (5), 1592–1602. doi:10.1053/j.gastro.2007.08.020
Mitobe, Y., Takayama, K.-i., Horie-Inoue, K., and Inoue, S. (2018). Prostate Cancer-Associated lncRNAs. Cancer Lett. 418, 159–166. doi:10.1016/j.canlet.2018.01.012
Naderi, M., Pazouki, A., Arefian, E., Hashemi, S. M., Jamshidi-Adegani, F., Gholamalamdari, O., et al. (2017). Two Triacylglycerol Pathway Genes, CTDNEP1 and LPIN1, Are Down-Regulated by Hsa-miR-122-5p in Hepatocytes. Arch. Iran. Med. 20 (3), 165–171.
Nakagawa, R., Muroyama, R., Saeki, C., Goto, K., Kaise, Y., Koike, K., et al. (2017). miR-425 Regulates Inflammatory Cytokine Production in CD4+ T Cells via N-Ras Upregulation in Primary Biliary Cholangitis. J. Hepatology 66 (6), 1223–1230. doi:10.1016/j.jhep.2017.02.002
Ninomiya, M., Kondo, Y., Funayama, R., Nagashima, T., Kogure, T., Kakazu, E., et al. (2013). Distinct microRNAs Expression Profile in Primary Biliary Cirrhosis and Evaluation of miR 505-3p and miR197-3p as Novel Biomarkers. PLoS One 8 (6), e66086. doi:10.1371/journal.pone.0066086
Ojha, R., Nandani, R., Chatterjee, N., and Prajapati, V. K. (2018). Emerging Role of Circular RNAs as Potential Biomarkers for the Diagnosis of Human Diseases. Adv. Exp. Med. Biol. 1087, 141–157. doi:10.1007/978-981-13-1426-1_12
Olaizola, P., Lee-Law, P. Y., Arbelaiz, A., Lapitz, A., Perugorria, M. J., Bujanda, L., et al. (2018). MicroRNAs and Extracellular Vesicles in Cholangiopathies. Biochimica Biophysica Acta (BBA) - Mol. Basis Dis. 1864 (4 Pt B), 1293–1307. doi:10.1016/j.bbadis.2017.06.026
Ouyang, Q., Wu, J., Jiang, Z., Zhao, J., Wang, R., Lou, A., et al. (2017). Microarray Expression Profile of Circular RNAs in Peripheral Blood Mononuclear Cells from Rheumatoid Arthritis Patients. Cell Physiol. Biochem. 42 (2), 651–659. doi:10.1159/000477883
Padgett, K. A., Lan, R. Y., Leung, P. C., Lleo, A., Dawson, K., Pfeiff, J., et al. (2009). Primary Biliary Cirrhosis Is Associated with Altered Hepatic microRNA Expression. J. Autoimmun. 32 (3-4), 246–253. doi:10.1016/j.jaut.2009.02.022
Pan, L., Liang, W., Fu, M., Huang, Z.-h., Li, X., Zhang, W., et al. (2017). Exosomes-mediated Transfer of Long Noncoding RNA ZFAS1 Promotes Gastric Cancer Progression. J. Cancer Res. Clin. Oncol. 143 (6), 991–1004. doi:10.1007/s00432-017-2361-2
Pan, Y., Wang, J., He, L., and Zhang, F. (2021). MicroRNA-34a Promotes EMT and Liver Fibrosis in Primary Biliary Cholangitis by Regulating TGF-β1/smad Pathway. J. Immunol. Res. 2021, 6890423. doi:10.1155/2021/6890423
Pandit, S., and Samant, H. (2022). “Primary Biliary Cholangitis,” in StatPearls (Treasure Island FL: StatPearls Publishing LLC.). Copyright © 2022.
Pang, K. C., Dinger, M. E., Mercer, T. R., Malquori, L., Grimmond, S. M., Chen, W., et al. (2009). Genome-Wide Identification of Long Noncoding RNAs in CD8+T Cells. J. Immunol. 182 (12), 7738–7748. doi:10.4049/jimmunol.0900603
Parés, A., Caballería, L., and Rodés, J. (2006). Excellent Long-Term Survival in Patients with Primary Biliary Cirrhosis and Biochemical Response to Ursodeoxycholic Acid. Gastroenterology 130 (3), 715–720. doi:10.1053/j.gastro.2005.12.029
Ponting, C. P., Oliver, P. L., and Reik, W. (2009). Evolution and Functions of Long Noncoding RNAs. Cell 136 (4), 629–641. doi:10.1016/j.cell.2009.02.006
Poupon, R. (2010). Primary Biliary Cirrhosis: a 2010 Update. J. Hepatology 52 (5), 745–758. doi:10.1016/j.jhep.2009.11.027
Qian, C., Chen, S. X., Ren, C. L., Zhong, R. Q., Deng, A. M., and Qin, Q. (2013). [Abnormal Expression of miR-Let-7b in Primary Biliary Cirrhosis and its Clinical Significance]. Zhonghua Gan Zang Bing Za Zhi 21 (7), 533–536. doi:10.3760/cma.j.issn.1007-3418.2013.07.014
Robinson, E. K., Covarrubias, S., and Carpenter, S. (2020). The How and Why of lncRNA Function: An Innate Immune Perspective. Biochimica Biophysica Acta (BBA) - Gene Regul. Mech. 1863 (4), 194419. doi:10.1016/j.bbagrm.2019.194419
Rodrigues, P. M., Perugorria, M. J., Santos-Laso, A., Bujanda, L., Beuers, U., and Banales, J. M. (2018). Primary Biliary Cholangitis: A Tale of Epigenetically-Induced Secretory Failure? J. Hepatology 69 (6), 1371–1383. doi:10.1016/j.jhep.2018.08.020
Sakamoto, T., Morishita, A., Nomura, T., Tani, J., Miyoshi, H., Yoneyama, H., et al. (2016). Identification of microRNA Profiles Associated with Refractory Primary Biliary Cirrhosis. Mol. Med. Rep. 14 (4), 3350–3356. doi:10.3892/mmr.2016.5606
Salzman, J. (2016). Circular RNA Expression: Its Potential Regulation and Function. Trends Genet. 32 (5), 309–316. doi:10.1016/j.tig.2016.03.002
She, C. (2020). Study on the Role of LncRNA XIST in Immune Cells of Primary Biliary Cholangitis. Shandong, China: Qingdao University. doi:10.27262/d.cnki.gqdau.2020.000390
Sheng, J. Q., Liu, L., Wang, M. R., and Li, P. Y. (2018). Circular RNAs in Digestive System Cancer: Potential Biomarkers and Therapeutic Targets. Am. J. Cancer Res. 8 (7), 1142–1156.
Shibao, K., Hirata, K., Robert, M. E., and Nathanson, M. H. (2003). Loss of Inositol 1,4,5-trisphosphate Receptors from Bile Duct Epithelia Is a Common Event in Cholestasis. Gastroenterology 125 (4), 1175–1187. doi:10.1016/s0016-5085(03)01201-0
Sigdel, K. R., Cheng, A., Wang, Y., Duan, L., and Zhang, Y. (2015). The Emerging Functions of Long Noncoding RNA in Immune Cells: Autoimmune Diseases. J. Immunol. Res. 2015, 848790. doi:10.1155/2015/848790
Song, Y., Yang, H., Jiang, K., Wang, B.-M., and Lin, R. (2018). miR-181a Regulates Th17 Cells Distribution via Up-Regulated BCL-2 in Primary Biliary Cholangitis. Int. Immunopharmacol. 64, 386–393. doi:10.1016/j.intimp.2018.09.027
Szabo, G., and Momen-Heravi, F. (2017). Extracellular Vesicles in Liver Disease and Potential as Biomarkers and Therapeutic Targets. Nat. Rev. Gastroenterol. Hepatol. 14 (8), 455–466. doi:10.1038/nrgastro.2017.71
Tan, Y., Pan, T., Ye, Y., Ge, G., Chen, L., Wen, D., et al. (2014). Serum microRNAs as Potential Biomarkers of Primary Biliary Cirrhosis. PLoS One 9 (10), e111424. doi:10.1371/journal.pone.0111424
Tang, Y. (2009). The Regulation of Micro RNA on Thl7 Cells in Patients with Primary Biliary Cirrhosis. China: Second Military Medical University.
Tavasolian, F., Abdollahi, E., Rezaei, R., Momtazi‐borojeni, A. A., Henrotin, Y., and Sahebkar, A. (2018). Altered Expression of MicroRNAs in Rheumatoid Arthritis. J. Cell. Biochem. 119 (1), 478–487. doi:10.1002/jcb.26205
Teng, K.-Y., Barajas, J. M., Hu, P., Jacob, S. T., and Ghoshal, K. (2020). Role of B Cell Lymphoma 2 in the Regulation of Liver Fibrosis in miR-122 Knockout Mice. Biology 9 (7), 157. doi:10.3390/biology9070157
Tian, T., Wang, M., Lin, S., Guo, Y., Dai, Z., Liu, K., et al. (2018). The Impact of lncRNA Dysregulation on Clinicopathology and Survival of Breast Cancer: A Systematic Review and Meta-Analysis. Mol. Ther. - Nucleic Acids 12, 359–369. doi:10.1016/j.omtn.2018.05.018
Tomiyama, T., Yang, G.-X., Zhao, M., Zhang, W., Tanaka, H., Wang, J., et al. (2017). The Modulation of Co-stimulatory Molecules by Circulating Exosomes in Primary Biliary Cirrhosis. Cell Mol. Immunol. 14 (3), 276–284. doi:10.1038/cmi.2015.86
Tsai, W.-C., Hsu, P. W.-C., Lai, T.-C., Chau, G.-Y., Lin, C.-W., Chen, C.-M., et al. (2009). MicroRNA-122, a Tumor Suppressor microRNA that Regulates Intrahepatic Metastasis of Hepatocellular Carcinoma. Hepatology 49 (5), 1571–1582. doi:10.1002/hep.22806
Tsai, W.-C., Hsu, S.-D., Hsu, C.-S., Lai, T.-C., Chen, S.-J., Shen, R., et al. (2012). MicroRNA-122 Plays a Critical Role in Liver Homeostasis and Hepatocarcinogenesis. J. Clin. Invest. 122 (8), 2884–2897. doi:10.1172/jci63455
Tsuneyama, K., Baba, H., Morimoto, Y., Tsunematsu, T., and Ogawa, H. (2017). Primary Biliary Cholangitis: Its Pathological Characteristics and Immunopathological Mechanisms. J. Med. Invest. 64 (1.2), 7–13. doi:10.2152/jmi.64.7
Wang, J., Yan, S., Yang, J., Lu, H., Xu, D., and Wang, Z. (2019). Non-coding RNAs in Rheumatoid Arthritis: From Bench to Bedside. Front. Immunol. 10, 3129. doi:10.3389/fimmu.2019.03129
Wang, X., Wen, X., Zhou, J., Qi, Y., Wu, R., Wang, Y., et al. (2017). MicroRNA-223 and microRNA-21 in Peripheral Blood B Cells Associated with Progression of Primary Biliary Cholangitis Patients. PLoS One 12 (9), e0184292. doi:10.1371/journal.pone.0184292
Wang, X. W., Heegaard, N. H. H., and Ørum, H. (2012). MicroRNAs in Liver Disease. Gastroenterology 142 (7), 1431–1443. doi:10.1053/j.gastro.2012.04.007
Wang, X., Zhang, C., Wu, Z., Chen, Y., and Shi, W. (2018). CircIBTK Inhibits DNA Demethylation and Activation of AKT Signaling Pathway via miR-29b in Peripheral Blood Mononuclear Cells in Systemic Lupus Erythematosus. Arthritis Res. Ther. 20 (1), 118. doi:10.1186/s13075-018-1618-8
Wang, Y., Fu, L., Lu, T., Zhang, G., Zhang, J., Zhao, Y., et al. (2021). Clinicopathological and Prognostic Significance of Long Non-coding RNA MIAT in Human Cancers: A Review and Meta-Analysis. Front. Genet. 12, 729768. doi:10.3389/fgene.2021.729768
Wang, Y., Zheng, F., Gao, G., Yan, S., Zhang, L., Wang, L., et al. (2018). MiR‐548a‐3p Regulates Inflammatory Response via TLR4/NF‐κB Signaling Pathway in Rheumatoid Arthritis. J. Cell Biochem. 120, 1133–1140. doi:10.1002/jcb.26659
Wasik, U., Kempinska-Podhorodecka, A., Bogdanos, D. P., Milkiewicz, P., and Milkiewicz, M. (2020). Enhanced Expression of miR-21 and miR-150 Is a Feature of Anti-mitochondrial Antibody-Negative Primary Biliary Cholangitis. Mol. Med. 26 (1), 8. doi:10.1186/s10020-019-0130-1
Wasik, U., Milkiewicz, M., Kempinska-Podhorodecka, A., and Milkiewicz, P. (2017). Protection against Oxidative Stress Mediated by the Nrf2/Keap1 axis Is Impaired in Primary Biliary Cholangitis. Sci. Rep. 7, 44769. doi:10.1038/srep44769
Watson, R. G., Angus, P. W., Dewar, M., Goss, B., Sewell, R. B., and Smallwood, R. A. (1995). Low Prevalence of Primary Biliary Cirrhosis in Victoria, Australia. Melbourne Liver Group. Gut 36 (6), 927–930. doi:10.1136/gut.36.6.927
Witt-Sullivan, H., Heathcote, J., Cauch, K., Blendis, L., Ghent, C., Katz, A., et al. (1990). The Demography of Primary Biliary Cirrhosis in Ontario, Canada. Hepatology 12 (1), 98–105. doi:10.1002/hep.1840120116
Worst, T. S., von Hardenberg, J., Gross, J. C., Erben, P., Schnölzer, M., Hausser, I., et al. (2017). Database-augmented Mass Spectrometry Analysis of Exosomes Identifies Claudin 3 as a Putative Prostate Cancer Biomarker. Mol. Cell. Proteomics 16 (6), 998–1008. doi:10.1074/mcp.M117.068577
Wu, Y., Zhang, F., Ma, J., Zhang, X., Wu, L., Qu, B., et al. (2015). Association of Large Intergenic Noncoding RNA Expression with Disease Activity and Organ Damage in Systemic Lupus Erythematosus. Arthritis Res. Ther. 17 (1), 131. doi:10.1186/s13075-015-0632-3
Xiang, G., Fan, J., Ye, Z., Fu, X., Li, H., Zhang, H., et al. (2019). Differential Expression Analysis of Long-Chain Non-coding RNA in Peripheral Blood of Patients with Primary Biliary Cirrhosis. Chongqing Med. 48, 1149–1154.
Xu, H., Xu, S.-J., Xie, S.-J., Zhang, Y., Yang, J.-H., Zhang, W.-Q., et al. (2019). MicroRNA-122 Supports Robust Innate Immunity in Hepatocytes by Targeting the RTKs/STAT3 Signaling Pathway. Elife 8, e41159. doi:10.7554/eLife.41159
Yang, J., Chen, M., Cao, R. Y., Li, Q., and Zhu, F. (2018). The Role of Circular RNAs in Cerebral Ischemic Diseases: Ischemic Stroke and Cerebral Ischemia/Reperfusion Injury. Adv. Exp. Med. Biol. 1087, 309–325. doi:10.1007/978-981-13-1426-1_25
Yang, L., Fu, J., and Zhou, Y. (2018). Circular RNAs and Their Emerging Roles in Immune Regulation. Front. Immunol. 9, 2977. doi:10.3389/fimmu.2018.02977
Yang, Y. (2013). Preliminary Study of microRNA as a New Disease Marker and its Function in Primary Biliary Cirrhosis. China, Beijing: Peking Union Medical College.
Yang, Z., Wu, W., Ou, P., Wu, M., Zeng, F., Zhou, B., et al. (2021). MiR-122-5p Knockdown Protects against APAP-Mediated Liver Injury through Up-Regulating NDRG3. Mol. Cell Biochem. 476 (2), 1257–1267. doi:10.1007/s11010-020-03988-0
Young, N. A., Valiente, G. R., Hampton, J. M., Wu, L.-C., Burd, C. J., Willis, W. L., et al. (2017). Estrogen-regulated STAT1 Activation Promotes TLR8 Expression to Facilitate Signaling via microRNA-21 in Systemic Lupus Erythematosus. Clin. Immunol. 176, 12–22. doi:10.1016/j.clim.2016.12.005
Zeng, N., Duan, W., Chen, S., Wu, S., Ma, H., Ou, X., et al. (2019). Epidemiology and Clinical Course of Primary Biliary Cholangitis in the Asia-Pacific Region: a Systematic Review and Meta-Analysis. Hepatol. Int. 13 (6), 788–799. doi:10.1007/s12072-019-09984-x
Zhang, L., Huang, Y., Wang, H., Kong, W., Ye, X., Chen, Y., et al. (2013). Increased Expression of lncRNA AK053349 in Peripheral Blood Mononuclear Cells from Patients with Primary Biliary Cirrhosis and its Clinical Significance. Int. J. Lab. Med. 34 (20), 2656–2657+2659.
Zhang, Y., Jia, Y., Zheng, R., Guo, Y., Wang, Y., Guo, H., et al. (2010). Plasma microRNA-122 as a Biomarker for Viral-, Alcohol-, and Chemical-Related Hepatic Diseases. Clin. Chem. 56 (12), 1830–1838. doi:10.1373/clinchem.2010.147850
Zhang, Y., Zhang, G., Liu, Y., Chen, R., Zhao, D., McAlister, V., et al. (2018). GDF15 Regulates Malat-1 Circular RNA and Inactivates NFκB Signaling Leading to Immune Tolerogenic DCs for Preventing Alloimmune Rejection in Heart Transplantation. Front. Immunol. 9, 2407. doi:10.3389/fimmu.2018.02407
Zhao, Z., Li, X., Gao, C., Jian, D., Hao, P., Rao, L., et al. (2017). Peripheral Blood Circular RNA Hsa_circ_0124644 Can Be Used as a Diagnostic Biomarker of Coronary Artery Disease. Sci. Rep. 7, 39918. doi:10.1038/srep39918
Zheng, F., Yu, X., Huang, J., and Dai, Y. (2017). Circular RNA Expression Profiles of Peripheral Blood Mononuclear Cells in Rheumatoid Arthritis Patients, Based on Microarray Chip Technology. Mol. Med. Rep. 16 (6), 8029–8036. doi:10.3892/mmr.2017.7638
Zheng, J., Li, Z., Wang, T., Zhao, Y., and Wang, Y. (2017). Microarray Expression Profile of Circular RNAs in Plasma from Primary Biliary Cholangitis Patients. Cell Physiol. Biochem. 44 (4), 1271–1281. doi:10.1159/000485487
Keywords: primary biliary cholangitis, non-coding RNA, autoimmunity, ursodeoxycholic acid, biomarker
Citation: Zhang Y, Jiao Z, Chen M, Shen B and Shuai Z (2022) Roles of Non-Coding RNAs in Primary Biliary Cholangitis. Front. Mol. Biosci. 9:915993. doi: 10.3389/fmolb.2022.915993
Received: 08 April 2022; Accepted: 20 June 2022;
Published: 08 July 2022.
Edited by:
Inhan Lee, miRcore, United StatesCopyright © 2022 Zhang, Jiao, Chen, Shen and Shuai. This is an open-access article distributed under the terms of the Creative Commons Attribution License (CC BY). The use, distribution or reproduction in other forums is permitted, provided the original author(s) and the copyright owner(s) are credited and that the original publication in this journal is cited, in accordance with accepted academic practice. No use, distribution or reproduction is permitted which does not comply with these terms.
*Correspondence: Zongwen Shuai, c2h1YWl6b25nd2VuQGFobXUuZWR1LmNu
†These authors have contributed equally to this work