- 1Department of Biostatistics and Bioinformatics, King Abdullah International Medical Research Center (KAIMRC), King Saud Bin Abdulaziz University for Health Sciences (KSAU-HS), Ministry of National Guard Health Affairs (MNGHA), Riyadh, Saudi Arabia
- 2Laboratory of Molecular Medicine, Division of Cancer Research, Regional Cancer Centre (RCC), Medical College, Thiruvanananthapuram, India
Matrix metalloproteinases (MMPs) are zinc-dependent endopeptidases that regulate the turnover of extracellular matrix (ECM) components. Gross and La Piere discovered MMPs in 1962 during an experiment on tissue samples from a tadpole’s tail. Several subtypes of MMPs have been identified, depending on their substrate specificity and localization. MMPs are involved as essential molecules in multiple and diverse physiological processes, such as reproduction, embryonic development, bone remodeling, tissue repair, and regulation of inflammatory processes. Its activity is controlled at various levels such as at transcription level, pro-peptide activation level and by the activity of a family of tissue inhibitors of metalloproteinase, endogenous inhibitors of MMPs. Cancer metastasis, which is the spread of a tumor to a distant site, is a complex process that is responsible for the majority of cancer-related death It is considered to be an indicator of cancer metastasis. During metastasis, the tumor cells have to invade the blood vessel and degrade the ECM to make a path to new loci in distant places. The degradation of blood vessels and ECM is mediated through the activity of MMPs. Hence, the MMP activity is critical to determining the metastatic potential of a cancer cell. Evasion of apoptosis is one of the hallmarks of cancer that are found to be correlated with the expression of MMPs. As a result, given the importance of MMPs in cancer, we describe the role of these multifunctional enzymes MMPs in various aspects of cancer formation and their rising possibilities as a novel therapeutic target in this review. There is also a brief discussion of various types of therapeutic components and drugs that function against MMPs.
Introduction
Matrix metalloproteinases (MMPs) are one of the most important families of proteases that act as a biological tool to cleave different components during the reconstruction of an extracellular matrix (ECM). ECM is a mechanical support to the cell and sets up to maintain the basic characteristic of the tissue. The interaction of cells with the ECM of their microenvironment determines the cell phenotype and its molecular functions. ECM is a complicated network composed of diverse biochemical components such as proteins, glycoproteins, proteoglycans, and polysaccharides (Yue, 2014). Apart from being a scaffold for cells/tissues to maintain their integrity and elasticity, ECM releases growth factors and other molecules that participate in various cellular pathways based on physiological demand (Csapo et al., 2020). Moreover, it mediates intercellular communication, signal transduction, and regulation of cellular events such as proliferation and cell death. ECM is a dynamic environment that constantly undergoes remodeling to maintain tissue homeostasis (Lu et al., 2011). ECM remodeling is an important and crucial event during normal and diseased physiological conditions. During ECM remodeling, cells undergo partial or complete degradation of different components of ECM. Degradation not only decreases the quantity of matrix proteins but also produces matrix protein degradation-derived bioactive fragments that are involved in various physiological and pathological processes (Cabral-Pacheco et al., 2020). This process of degradation is mediated by specific proteases such as MMPs that act spatially and temporally to bring about the remodeling (Kessenbrock et al., 2010).
The tight regulation of MMPs is responsible for maintaining the homeostasis of the body. Dysregulation in any regulatory mechanisms leads to aberrant expression of MMPs, which leads to different disease conditions like arthritis/osteoarthritis and fibrotic diseases as contributors to tissue destruction disease progression (Bonnans et al., 2014). Abnormal MMP expression has been observed in a variety of diseases, including neurological disorders such as Parkinson’s disease, Alzheimer’s disease, Japanese encephalitis, and glaucoma (Singh et al., 2015). MMPs also play role in diseases such as Crohn’s disease and hepatic ischemia (Ferrigno et al., 2020; Rautava et al., 2020). MMPs expression is generally very low in normal conditions, but elevated levels of MMPs are observed in different types of cancers and correlate with the enhanced proliferation and growth of tumors (Jiang et al., 2002). Inflammation is also now included in cancer hallmarks and has been found to be linked with the advancement of cancers and MMPs have been shown to influence inflammation in the tumor microenvironment in myriad ways (Coussens and Werb, 2002). Likewise, MMPs are also involved in various aspects of the development of cancer stem cells (Kessenbrock et al., 2015). In this review, we cover the involvement of the multifunctional enzymes MMPs in several facets of cancer formation, as well as the various therapeutic categories that can work against MMPs.
Matrix Metalloproteinase in Proliferation, Invasion, and Migration
Metastasis is the dissemination of the cancer cells from one organ to another into a local or distant site, constituting more than 90% of cell death. Metastasis has a crucial role in the prognosis of the disease (Eccles and Welch, 2007). It occurs in a cascade of events, involving seven different steps: 1) detachment of cells from the primary site, 2) intravasation of cells into vascular or lymphatic channels, 3) survival of cells in the circulation, 4) adhesion into blood vessels, 5) extravasation of cells into new loci, 6) establishment of colonies in a new site, and 7) formation of tumor-specific blood vessels and angiogenesis. It is estimated that only 0.01% of cells that enter the circulation will successfully colonize in distant organs, hence considered to be a highly inefficient process (Valastyan and Weinberg, 2011). Stephen Paget proposed the “seed and soil hypothesis,” which states that the spread of tumor cells is governed by the interaction between the cancer cells (seed) and the host organ (soil). Studies show that premetastatic niches prepare the target organ to accept and form a secondary tumor (Liu and Cao, 2016; Peinado et al., 2017). Premetastatic niches are a specialized environment that favors cancer cell seeding and tumor development by containing protumor immune cells and altered ECM components.
Degradation of ECM leads to the invasion of tumor cells to promote metastasis. MMPs not only degrade the ECM components but also expose some binding sites to other receptors and release biologically active molecules (Walker et al., 2018). Invasive cancer cells form specialized F-actin-based protrusions of the plasma membrane called invadopodia to clear the path by the ECM degradation (Paz et al., 2014). Invadopodia are found in cancer cells with high metastatic potential. Studies have documented that different types of growth factors and cytokines are found to stimulate invadopodia formation. In this regard, a trans-membrane-type 1 MMP (MT1-MMP), MMP-14, accumulates in the invadopodia and facilitates the localized degradation of ECM during the intra/extravasation process (Jacob and Prekeris, 2015). In 2020 Yan et al., demonstrate that MT4-MMP regulates invadopodia formation and cell movement and enhanced cell migration and invasion Yan et al. (2020). MT1-MMP, a multifunctional enzyme, is also involved in the activation of pro-MMP-2, leading to tumor growth (Deryugina et al., 2001).
Moreover, MT1-MMP degrades MMP-8, and MMP-13 MT1-MMP degrades multiple ECM components, including collagen types I, II, and III; fibronectin; laminin-1; vitronectin; aggrecan; gelatin; α2-macroglobulin; αl proteinase inhibitor (α1Pi); and proteoglycans (Shiomi et al., 2010). Apart from degrading various ECM components, MT1-MMP may additionally release bioactive matrix fragments named matrikines, which function as extracellular modulators (Adair-Kirk and Senior, 2008).
Role of Matrix Metalloproteinases in Epithelial–Mesenchymal Transition
Upregulated expression of MT1-MMP enhances metastasis by enhancing epithelial-to-mesenchymal transition (EMT) (Pang et al., 2016). In squamous cell carcinoma, EMT is associated with downregulation of E-cadherin (epithelial cadherin, E-cad) and upregulation of TWIST, ZEB, and zinc finger E-box-binding homeobox 1 (ZEB1) (Zhang et al., 2015). According to a study by Sato et al., MMP-2 was shown to be important in the invasive spread of ovarian cancer, while MT1-MMP was involved in both the activation and degradation of the extracellular matrix (ECM) as well as their cooperation with MMP-2 (Sato and Takino, 2010).
EMT is a highly coordinated event during which epithelial cells lose their epithelial characteristics and acquire a mesenchymal phenotype. In this process, epithelial cells undergo alterations in apical–basal polarity, disassemble their junctional structures, express mesenchyme cell proteins, acquire more spindle-shaped mesenchymal-like cells, and become migratory. This event is intrinsically linked to various processes such as embryonic development, wound healing, tissue fibrosis, and tumorogenesis. It has been considered to be an essential event in the invasion and migration of malignant cells during metastasis (Singh and Settleman, 2010). The loss of epithelial markers such as E-cad, claudins, and occludins and the rise of mesenchymal markers like vimentin, fibronectin, and N-cadherin are changes linked to EMT (neuronal cadherin, N-cad) (Lamouille et al., 2014; Nieto et al., 2016).
In cancer, EMT programs can be activated by various factors such as transforming growth factor-β (TGF-β), epidermal growth factor, and hepatocyte growth factor. Tumor hypoxia is a common feature of the microenvironment in solid tumors, which regulates the transcriptional factors such as ZEB1/2, TWIST, zinc finger protein SNAI1 (SNAIL), E2A proteins, and E2A immunoglobulin enhancer-binding factors E12/E47 (E12/E47) to downregulate the epithelial cell markers E-cad expression and induce mesenchymal gene expression.
Cadherins are transmembrane glycoproteins responsible for cell–cell adhesion and maintenance of normal tissue architecture (Oda and Takeichi, 2011). The role of different cadherins in the process of tumorogenesis has been studied extensively. “Cadherin-switch” is defined as the loss of E-cad and increased expression of N-cad during EMT, and this transition induces or enhances the metastatic potential of the tumor cells ( ). The adhesive activity of E-cadherin prevents cells in the tumor mass from dissociating from one another and therefore prevents spread into other tissues. The loss of E-cad can also result in the mislocalization of α-catenin and p120 catenin, which leads to the activation of mitogen-activated protein kinase (MAPK) pathways. E-cad thus acts as a tumor-suppressor protein (Na et al., 2020). Signaling pathways such as Wnt and TGF-β activate SNAIL and SLUG, and these molecules further regulate the “cadherin switch” by downregulating E-cad and inducing the expression of mesenchymal N-cad. N-cad stimulates cell proliferation through MAPK pathways. EMT also depends on the activity of MMPs through different mechanisms. Cells that undergo EMT can produce more MMPs and facilitate cell invasion and metastasis; the elevated levels of MMPs in turn enhance the EMT. In addition, stromal-like cells that are generated during EMT drive cancer progression via further MMP production (Radisky and Radisky, 2010). MMPs −1, −2, −3, −7, −9, −14, and −28 are the main MMPSs that participate in the EMT.
TWIST, a basic helix-loop-helix transcription factor have a major role in embryonic development. This gene was also found to be expressed in a number of malignancies, where it promotes the tumor initiation, its growth, and metastasis. Overexpression of TWIST induces EMT. Overexpression of twist increases the invasive and metastatic abilities of cancer cells by promoting the downregulation of E-cad and the induction of an EMT (Yang et al., 2004).
ZEB1 (also named TCF8 or DeltaEF1) is a zinc finger E-box binding homeobox 1, transcription factor that promotes tumor invasion and metastasis by inducing EMT. It induces EMT by downregulating the E-cad expression. Apart from this, ZEB1 regulates other target genes involved in tumor progression such as Lgl2, PATJ, HUGL2, and Crumbs3 (Zhang et al., 2015). ZEB1 can promote drug resistance and the survival of cancer cells (Zhang et al., 2015) According to a study, after focal ischemia, gelatinase A (MMP-2) and gelatinase B (MMP-9) activities in the human brain increase (Clark et al., 1997). It also indicates that increased levels in several forms of human malignancies are connected with a poor prognosis (Roomi et al., 2009; Kunz et al., 2016). Elevated MMP activity has been linked to a variety of pathologic diseases, and the therapeutic effect of MMP inhibitors is being investigated in a few animal models. MMP-7 mediated the conversion of E-cad into a soluble form, allowing cancer cells to dislodge from the primary tumor during the early stage of metastasis (Lee et al., 2007). In addition to the proteolytic functions, MT1-MMP controls the migration of tumor cells through non-proteolytic mechanisms (Gifford and Itoh, 2019). Overexpression of MMP-12 is positively correlated with metastasis of ovarian cancer (Zhang and Chen, 2017).
Matrix Metalloproteinases in Angiogenesis
Cancer research is now much better at understanding the functional mechanisms that focus on cell transformation, and tumor progression and also aid in the development of new indicators and medicines (Bremnes et al., 2011). MMPs have been implicated in angiogenesis regulation as well as angiogenesis, vasculogenesis, and lymphangiogenesis in cancer (Quintero-Fabián et al., 2019). Angiogenesis is the formation of new blood vessels or capillaries from existent vasculature. Collagenases (MMP-1, 8, and 13) are the most important proteins in angiogenesis. Although this is a healing process, it begins in illnesses such as cancer. As a result, angiogenesis provides cancer cells with nutrition, resulting in tumor growth (Lugano et al., 2020). There are assays available to detect angiogenesis and biological activity. During its Phase I clinical investigation, Lockhart et al. outlined an angiogenesis assay of an MMP inhibitor (MMPI), BMS-275291 Lockhart et al. (2003). This method is widely used to assess the activity of noncytotoxic chemotherapeutic medicines as a biomarker.
Angiogenesis is regulated by a fine balance of pro and antiangiogenic molecules (Bisht et al., 2010). Disturbance in this balance and dominancy of proangiogenic factors results in “Angiogenic Switch” leading to sprouting, and proliferation of endothelial cells results in angiogenesis (Miller et al., 2009). MMP knockout mice model studies revealed that MMPs act as a critical molecule in the “Angiogenic Switch” in the growth of malignant cells. MMP-9 expression is required for the angiogenic switch, whereas MMP-2 activates endothelial cell survival and proliferation and initiates integrin signaling to support the angiogenesis thereby contributing to tumor growth (Deryugina and Quigley, 2015). In addition to its ECM degrading activity, MMPs mediate the release of potent inducers of blood vessel sprouting including vascular endothelial growth factor, basic fibroblast growth factor, and tumor necrosis factor-α (Conway et al., 2001). Moreover, MMPs are involved in the generation of angiogenic molecules such as angiostatin and endostatin from their precursors (Ghajar et al., 2008). In short, MMPs contribute to multiple events during angiogenesis.
Matrix Metalloproteinases in Inflammation
MMPs play a crucial role in cancer progression and become therapeutic targets in cancer interventions (Shay et al., 2015). MMPs are multifunctional enzymes in inflammation. Both acute and chronic inflammation can be regulated by MMP activity. Inflammation is associated with most tumor tissues and is now considered to be a hallmark of cancer linked to genetic instability (Fanjul-Fernández et al., 2010). Numerous factors, including cytokines, growth factors, chemokines, and extracellular matrix-modifying enzymes like metalloproteinases, can contribute to inflammation's ability to increase the risk of cancer (Landskron et al., 2014). Some MMPs can play both beneficial and detrimental roles at different stages. MMPs control inflammation as soluble factors, at cell surfaces and even in nuclei. In almost every human tissue, the MMP family of enzymes plays a greater role in inflammation (Fingleton, 2017). MMPs act extensively in inflammation to modulate barrier function and also play a role in cytokine and chemokine activity, which leads to the formation of chemokine gradients, as demonstrated by mouse models of human disease with targeted deletions of individual MMPs (Löffek et al., 2011). Figure 1 shows an overview of the essential steps of metastasis. Because MMPs are involved in both host defense and pathological inflammatory disease, it is critical to understand the many molecular pathways by which specific MMPs participate in normal and abnormal inflammatory processes. Understanding the pathways through which MMPs work in distinct health and disease states may lead to the development of therapeutic approaches to combat MMP-mediated diseases. The cross-link between MMPs and inflammation in tumor progression is well addressed (Quintero-Fabián et al., 2019). Overexpression of MAPK phosphatases has been demonstrated to prevent MMP promoter activation (Westermarck et al., 2001). Some members of the MMP family behave as tumor-suppressor enzymes and should therefore be regarded as anti-targets in cancer therapy (Decock et al., 2011). Many investigations have found that MMPs play an important role in tumor invasion. MMPs in cancer have been extensively investigated; nevertheless, the specific involvement of different MMPs in cancer progression may be more complex than previously thought (Mittal et al., 2016). MMP-3, MMP-7, MMP-9, and MMP-12 have been identified as inhibitors of tumor development and invasion (Table 1).
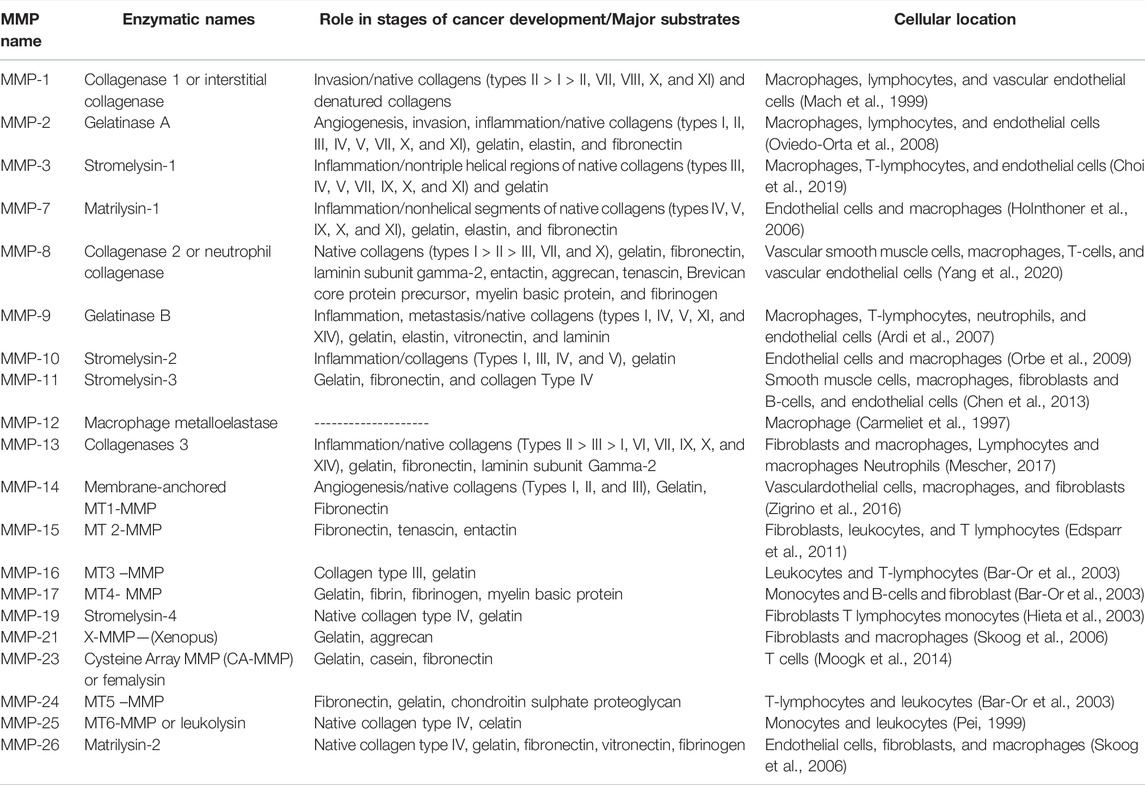
TABLE 1. List of matrix metalloproteinases (MMPs), their enzymatic names, major substrates, and cellular location.
MMP-2, MMP-7, MMP-9, TIMP-1, and TIMP-2 research has received considerable attention since they play a number of roles in cancer. One study found that serum antigen concentrations of MMP-7, MMP-9, TIMP-1, and TIMP-2 were considerably higher in colorectal cancer and adenomas patients compared to controls (Barabás et al., 2021). Their data suggest that MMPs, as well as their inhibitors TIMP-1 and TIMP-2, play a crucial role in colorectal cancer. MMP-2, MMP-7, MMP-9, and TIMP-2 were also investigated in the development of the recurrent depressive disorder (Table 2). A recent study sought to establish a link between MMP-2, MMP-7, and their inhibitor, TIMP-2, in adult and pediatric cancer (Kaczorowska et al., 2020).
C-c motif chemokine ligand 27 (CCL27), a chemokine primarily expressed by keratinocytes, and its enhanced expression activate the extracellular signal-regulated kinase 1/2 (ERK1/2) pathway and in turn overexpress MMP-7 leads to cell invasion and migration of breast cancers (Korbecki et al., 2020). Chemokines are a class of small proteins that play an important role in leukocyte migration and invasion (Martínez-Rodríguez and Monteagudo, 2021). They have the ability to participate in tumor cell cellular proliferation and migration. CCR10 expression was discovered to be elevated in breast cancer cells, and the CCL27/CCR10 axis eventually promoted breast cancer cell invasion and migration via elevating MMP-7 (Lin et al., 2017). CCL27 is the ligand of CCR10 (Monteagudo et al., 2012). Likewise, in gastric cancer activated CXC motif chemokine ligand 10 (CXCL10) during inflammation enhances the invasion and migration of cells through the upregulation of MMP-2 and MMP-9 (Ren et al., 2017).
MMP-8- and MMP-9-mediated collagen breakdown generates N-acetyl-proline-glycine-proline (ac-PGP) tripeptides that bind the CXC chemokine receptor 2 (CXCR2) and trigger chemotaxis of neutrophils and increases lung metastasis (Bekaert et al., 2017). In oral squamous cell carcinoma a positive correlation was observed between MMP-7 and cyclooxygenase-2. Thus, it is well documented that MMPs regulate the inflammatory status of tumor microenvironment and facilitate the advancement of tumors (Nasry et al., 2018).
Various Inhibitors of Matrix Metalloproteinases
MMP inhibition has been extensively investigated in cancer research. Tissue inhibitors of metalloproteinases (TIMPs) are naturally occurring proteins that inhibit MMPs specifically (Wojtowicz-Praga et al., 1997). According to studies, potent and selective MMPIs have been synthesized, and clinical trials of such synthetic MMPIs began in the 1990s and early 2000s (Brown, 1999). These studies failed because of ineffectiveness and significant. Batimastat (BB-94) and marimastat (BB-2516) are synthetic, low-molecular-weight MMPIs. They have a hydroxamate structure that mimics collagen. Batimastat was the first synthetic MMP inhibitor studied in patients with advanced cancer (Wojtowicz-Praga et al., 1997). Various investigations are now being conducted to identify potent MMP inhibitors (Hidalgo and Eckhardt, 2001; Vihinen and Kähäri, 2002; Devy and Dransfield, 2011). Selective MMP inhibition has been used with antibodies and small molecule components based on binding to protease secondary binding sites, blocking the protease active site, or preventing proMMP activation. Several of these inhibitors have just undergone clinical trials, whereas others are in advanced preclinical phases (Fields, 2019a). The humanized monoclonal antibody GS-5745, a potent and highly selective allosteric MMP9 inhibitor, has been developed for clinical trials in ulcerative colitis and colorectal cancer (Marshall et al., 2015; Fields, 2019b). However, many ongoing studies are attempting to comprehend the complexity of MMP function in many diseases (Das et al., 2021). In terms of inhibiting MMP expression through kinase pathways, it is feasible that selective pharmacologic inhibitors for specific signaling pathways (e.g., MAPK and PKC) may soon be accessible for preliminary clinical trials. This will improve outcomes in a range of illnesses, including cancer, heart disease, and neurodegenerative disease. With a greater understanding of MMP protein design, new techniques for designing MMP-targeted therapeutics have emerged.
Collagen peptidomimetics and nonpeptidomimetic MMP active site inhibitors, tetracycline derivatives, and bisphosphonates are the most commonly investigated MMP inhibitors (Hidalgo and Eckhardt, 2001). Batimastat, a hydroxamate peptidomimetic inhibitor, and marimastat were the first MMP inhibitors to be thoroughly explored. The analog of batimastat, marimastat, binds to the active site of MMPs (Chaudhary et al., 2010). Marimastat is the first orally accessible MMP inhibitor to be evaluated in humans, and it has been shown in animal models to limit the spread and progression of pancreatic cancer (Rosemurgy et al., 1999). Several nonpeptidic MMP inhibitors were also produced as part of the process of generating potential therapeutic options and determining the medicinal nature and bioavailability of peptidic medicines. Angiogenesis-promoting matrix-targeting metalloproteinases are also thought to be good therapeutic targets (Stetler-Stevenson, 1999). These mechanisms are useful in understanding how drugs work (Fields, 2019a).
To target specific MMPs, researchers are currently working to identify new molecular components from nutraceuticals, such as betulinic acid, genistein, theaflavin, myricetin, curcumin, resveratrol, matlystatin B, nicotinamide, xanthorhizzol, oleanolic acid, glycyrrhetinic acid, and catechin derivatives (Mukherjee et al., 2013). Polyphenols, monophenols, and other secondary metabolites of food and nonedible plants are among these natural compounds (Pandey and Rizvi, 2009). Synthetic MMPIs were designed to prevent tumor cell-induced changes in ECM and thereby achieve antitumor activity (Mitsiades et al., 2001). Among marine-derived MMPIs that aid to suppress MMPs, marine saccharoid MMPIs are very popular (Zhang and Kim, 2009).
Nanodelivery System for Targeting Matrix Metalloproteinases in Cancer Treatment
Conventional cancer treatment options include radiation therapy, chemotherapy, and surgery, either alone or in combination (Baskar et al., 2012). Most of the time, these therapeutic approaches have multiple major adverse effects. Cells that rapidly proliferate may be destroyed because of a lack of specificity, resulting in immunosuppression, the development of multidrug resistance, and the growth of stem-like cells, all of which can lead to treatment failure and a low survival rate. Due to the lack of solubility, the drug will stay in circulation for a shorter amount of time, lowering the penetrance or availability of the cells and resulting in therapeutic failure. Inflammation of the digestive tract lining, alopecia (hair loss), and organ failure have all been linked to the drug. Nanotechnology’s application in cancer treatment allowed researchers to overcome many of the constraints of traditional treatments, resulting in significant advancements in cancer therapy (Jin et al., 2020). Nanotechnology makes use of nanoparticles with unique optical, magnetic, and electrical properties that are designed at the atomic or molecular level. Nanoparticles range in size from a few nanometers (nm) to several hundred nanometers (nm), depending on their intended function (Cheng et al., 2021). Nanomaterials of various sorts have been synthesized for a variety of cancer therapies. Nanoparticles may circulate more freely in the human body than larger particles (Patra et al., 2018); therefore, they could be used to deliver drugs to particular cells or tissues with a controlled release. The biophysical and biochemical properties of the targeted medications and loci being treated determine the use of an ideal nano-drug delivery technology (Rizvi and Saleh, 2018).
The targeted delivery is achieved by either passive targeting or active targeting. In active targeting, a drug is conjugated with a nanoparticle, whereas in passive targeting, it is based on enhanced permeability and retention effect (Ali et al., 2021). Thus, targeted delivery helps reduce toxicity in normal cells, protects drugs from degradation, increases half-life, etc., (Rosenblum et al., 2018).
MMPs are upregulated at all stages of expression in cancers. Different strategies have been developed to inhibit their expression and enzymatic activity. However, these inhibitors have produced serious side effects and nonspecific inhibition making other pathways or molecules involved in other pathways, which causes other pathological situations. For example, marimastat, a potent synthetic MMP inhibitor, chelates the zinc ion of the MMPs catalytic site but might also inhibit the activity of other zinc-dependent enzymes (Winer et al., 2018). In this context, nanotechnology-based approaches have been developed. The nanofiber system consisting of DOX linked to the KGFRWR peptide (an amyloid ß protein derivate) was found to reduce the tumor growth in hepatocellular carcinoma. In this system, the cytotoxicity of DOX kills the tumor and the KGFRWR peptide inhibits the MMP activity (Ji et al., 2018). Likewise, nanoparticles metallofullerenol Gd@C82(OH)22 can block MMP-2 and MMP-9 synthesis through allosteric inhibition (Kang et al., 2012). Nanocarriers such as lysolipid-containing thermosensitive liposomes deliver marimastat (Lyu et al., 2019). Likewise, peptide nanofibers conjugated with siRNA or shRNA MMPs could effectively effective target MMPs (Mazza et al., 2019). Another nanoplatform consisting of HPAA-MTX/shMMP-9 (cationic hyperbranched poly (amido amine) (HPAA) with MTX and shMMP-9 plasmid) could significantly reduce the tumor growth in MCF 7 tumor-bearing animal as well as the decrease in the invasiveness and apoptosis induction in nasopharyngeal carcinoma HNE-1 cells (Tang et al., 2018; Liu et al., 2019).
Conclusion
Remodeling and degradation of ECM are crucial events in metastasis and MMPs; a family of zinc-dependent proteases controls this process and promotes the progression of tumors into the distant site. MMPs play an important role in the inflammatory process and are known to influence the onset and progression of many cancer cases. Furthermore, MMPs play an important role in angiogenesis and cancer growth. MMPs play an important role in precision medicine because they can act as biomarkers. According to this review, investigating diverse classes of MMPs is critical in understanding their involvement in cancer progression and becoming therapeutic targets in cancer therapies. MMPs also are thought to promote the growth of the tumor cells once they have metastasized. In addition to ECM degradation, they are involved in the activation of cell surface proteins and the shedding of membrane-bound receptor molecules, regulating growth factors and chemokines. Moreover, inflammation, migration, and invasion of tumor cells and tumor-specific angiogenesis are also regulated by MMPs. MMPs are involved at various levels, during transcription, translation, and zymogen activation and by the activity of its endogenous inhibitor, TIMPs. TIMPs bind MMPs in a stoichiometric 1:1 ratio and thereby block access of substrates to the catalytic domain of the endopeptidases. An imbalance between active MMPs and TIMPs, favoring MMP activity can lead to ECM degradation, whereas favoring TIMPs leads to ECM deposition. Hence, MMPs is a novel target for cancer therapy, and several agents based on small molecule inhibitors, monoclonal antibody, and nanoparticles have been developed to improve patient survival.
Author Contributions
SM and SK designed the study. SK wrote the initial draft manuscript, LA performed literature data analysis, SM reviewed the manuscript, and SK and LA revised and edited the manuscript. All authors reviewed the manuscript.
Conflict of Interest
The authors declare that the research was conducted in the absence of any commercial or financial relationships that could be construed as potential conflicts of interest.
Publisher’s Note
All claims expressed in this article are solely those of the authors and do not necessarily represent those of their affiliated organizations, or those of the publisher, the editors, and the reviewers. Any product that may be evaluated in this article, or claim that may be made by its manufacturer, is not guaranteed or endorsed by the publisher.
References
Adair-kirk, T., and Senior, R. (2008). Fragments of Extracellular Matrix as Mediators of Inflammation. Int. J. Biochem. Cell. Biol. 40, 1101–1110. doi:10.1016/j.biocel.2007.12.005
Ali, E. S., Sharker, S. M., Islam, M. T., Khan, I. N., Shaw, S., Rahman, M. A., et al. (2021). Targeting Cancer Cells with Nanotherapeutics and Nanodiagnostics: Current Status and Future Perspectives. Seminars Cancer Biol. 69, 52–68. doi:10.1016/j.semcancer.2020.01.011
Ardi, V. C., Kupriyanova, T. A., Deryugina, E. I., and Quigley, J. P. (2007). Human Neutrophils Uniquely Release TIMP-free MMP-9 to Provide a Potent Catalytic Stimulator of Angiogenesis. Proc. Natl. Acad. Sci. U.S.A. 104, 20262–20267. doi:10.1073/pnas.0706438104
Bar-Or, A., Nuttall, R. K., Duddy, M., Alter, A., Kim, H. J., Ifergan, I., et al. (2003). Analyses of All Matrix Metalloproteinase Members in Leukocytes Emphasize Monocytes as Major Inflammatory Mediators in Multiple Sclerosis. Brain 126, 2738–2749. doi:10.1093/brain/awg285
Barabás, L., Hritz, I., István, G., Tulassay, Z., and Herszényi, L. (2021). The Behavior of MMP-2, MMP-7, MMP-9, and Their Inhibitors TIMP-1 and TIMP-2 in Adenoma-Colorectal Cancer Sequence. Dig. Dis. 39, 217–224. doi:10.1159/000511765
Baskar, R., Lee, K. A., Yeo, R., and Yeoh, K.-W. (2012). Cancer and Radiation Therapy: Current Advances and Future Directions. Int. J. Med. Sci. 9, 193–199. doi:10.7150/ijms.3635
Bekaert, S., Fillet, M., Detry, B., Pichavant, M., Marée, R., Noel, A., et al. (2017). Inflammation-Generated Extracellular Matrix Fragments Drive Lung Metastasis. Cancer Growth Metastasis. 10, 117906441774553–1179064417745539. doi:10.1177/1179064417745539
Bisht, M., Dhasmana, D., and Bist, S. (2010). Angiogenesis: Future of Pharmacological Modulation. Indian J. Pharmacol. 42, 2–8. doi:10.4103/0253-7613.62395
Bonnans, C., Chou, J., and Werb, Z. (2014). Remodelling the Extracellular Matrix in Development and Disease. Nat. Rev. Mol. Cell. Biol. 15, 786–801. doi:10.1038/nrm3904
Bremnes, R. M., Dønnem, T., Al-Saad, S., Al-Shibli, K., Andersen, S., Sirera, R., et al. (2011). The Role of Tumor Stroma in Cancer Progression and Prognosis: Emphasis on Carcinoma-Associated Fibroblasts and Non-small Cell Lung Cancer. J. Thorac. Oncol. 6, 209–217. doi:10.1097/JTO.0b013e3181f8a1bd
Brown, P. D. (1999). Clinical studies with matrix metalloproteinase inhibitors. APMIS. 107, 174–180. doi:10.1111/j.1699-0463.1999.tb01541.x
Cabral-Pacheco, G. A., Garza-Veloz, I., Castruita-De la Rosa, C., Ramirez-Acuña, J. M., Perez-Romero, B. A., Guerrero-Rodriguez, J. F., et al. (2020). The Roles of Matrix Metalloproteinases and Their Inhibitors in Human Diseases. Ijms 21, 9739. doi:10.3390/ijms21249739
Carmeliet, P., Moons, L., Lijnen, R., Baes, M., Lemaître, V., Tipping, P., et al. (1997). Urokinase-generated Plasmin Activates Matrix Metalloproteinases during Aneurysm Formation. Nat. Genet. 17, 439–444. doi:10.1038/ng1297-439
Chaudhary, A. K., Singh, M., Bharti, A. C., Asotra, K., Sundaram, S., and Mehrotra, R. (2010). Genetic Polymorphisms of Matrix Metalloproteinases and Their Inhibitors in Potentially Malignant and Malignant Lesions of the Head and Neck. J. Biomed. Sci. 17, 10. doi:10.1186/1423-0127-17-10
Chen, Q., Jin, M., Yang, F., Zhu, J., Xiao, Q., and Zhang, L. (2013). Matrix Metalloproteinases: Inflammatory Regulators of Cell Behaviors in Vascular Formation and Remodeling. Mediat. Inflamm. 2013, 1–14. doi:10.1155/2013/928315
Cheng, Z., Li, M., Dey, R., and Chen, Y. (2021). Nanomaterials for Cancer Therapy: Current Progress and Perspectives. J. Hematol. Oncol. 14, 85. doi:10.1186/s13045-021-01096-0
Choi, B., Shin, M.-K., Kim, E.-Y., Park, J.-E., Lee, H., Kim, S. W., et al. (2019). Elevated Neuropeptide Y in Endothelial Dysfunction Promotes Macrophage Infiltration and Smooth Muscle Foam Cell Formation. Front. Immunol. 10. doi:10.3389/fimmu.2019.01701
Clark, A. W., Krekoski, C. A., Bou, S.-S., Chapman, K. R., and Edwards, D. R. (1997). Increased Gelatinase A (MMP-2) and Gelatinase B (MMP-9) Activities in Human Brain after Focal Ischemia. Neurosci. Lett. 238, 53–56. doi:10.1016/s0304-3940(97)00859-8
Conway, E., Collen, D., and Carmeliet, P. (2001). Molecular Mechanisms of Blood Vessel Growth. Cardiovasc. Res. 49, 507–521. doi:10.1016/S0008-6363(00)00281-9
Coussens, L. M., and Werb, Z. (2002). Inflammation and Cancer. Nature 420, 860–867. doi:10.1038/nature01322
Csapo, R., Gumpenberger, M., and Wessner, B. (2020). Skeletal Muscle Extracellular Matrix - what Do We Know about its Composition, Regulation, and Physiological Roles? A Narrative Review. Front. Physiol. 11. doi:10.3389/fphys.2020.00253
Das, N., Benko, C., Gill, S. E., and Dufour, A. (2021). The Pharmacological TAILS of Matrix Metalloproteinases and Their Inhibitors. Pharmaceuticals 14, 31. doi:10.3390/ph14010031
Decock, J., Thirkettle, S., Wagstaff, L., and Edwards, D. R. (2011). Matrix Metalloproteinases: Protective Roles in Cancer. J. Cell. Mol. Med. 15, 1254–1265. doi:10.1111/j.1582-4934.2011.01302.x
Deryugina, E. I., and Quigley, J. P. (2015). Tumor Angiogenesis: MMP-Mediated Induction of Intravasation- and Metastasis-Sustaining Neovasculature. Matrix Biol. 44-46 (46), 94–112. doi:10.1016/j.matbio.2015.04.004
Deryugina, E. I., Ratnikov, B., Monosov, E., Postnova, T. I., DiScipio, R., Smith, J. W., et al. (2001). MT1-MMP Initiates Activation of Pro-MMP-2 and Integrin αvβ3 Promotes Maturation of MMP-2 in Breast Carcinoma Cells. Exp. Cell. Res. 263, 209–223. doi:10.1006/excr.2000.5118
Devy, L., and Dransfield, D. T. (2011). New Strategies for the Next Generation of Matrix-Metalloproteinase Inhibitors: Selectively Targeting Membrane-Anchored MMPs with Therapeutic Antibodies. Biochem. Res. Int. 2011, 1–11. doi:10.1155/2011/191670
Eccles, S. A., and Welch, D. R. (2007). Metastasis: Recent Discoveries and Novel Treatment Strategies. Lancet 369, 1742–1757. doi:10.1016/S0140-6736(07)60781-8
Edsparr, K., Basse, P. H., Goldfarb, R. H., and Albertsson, P. (2011). Matrix Metalloproteinases in Cytotoxic Lymphocytes Impact on Tumour Infiltration and Immunomodulation. Cancer Microenviron. 4, 351–360. doi:10.1007/s12307-010-0057-0
Fanjul-Fernández, M., Folgueras, A. R., Cabrera, S., and López-Otín, C. (2010). Matrix Metalloproteinases: Evolution, Gene Regulation and Functional Analysis in Mouse Models. Biochimica Biophysica Acta (BBA) - Mol. Cell. Res. 1803, 3–19. doi:10.1016/j.bbamcr.2009.07.004
Farhadi, S., and Mohamadi, M. (2017). Significance of MMPs Expression on OSCC Clinical Outcome. SL Dent. Oral Disord. Ther. 1 (1), 111.
Ferrigno, A., Di Pasqua, L. G., Palladini, G., Berardo, C., Verta, R., Richelmi, P., et al. (2020). Transient Expression of Reck under Hepatic Ischemia/Reperfusion Conditions Is Associated with Mapk Signaling Pathways. Biomolecules 10, 747. doi:10.3390/biom10050747
Fields, G. B. (2019a). Mechanisms of Action of Novel Drugs Targeting Angiogenesis-Promoting Matrix Metalloproteinases. Front. Immunol. 10. doi:10.3389/fimmu.2019.01278
Fields, G. B. (2019b). The Rebirth of Matrix Metalloproteinase Inhibitors: Moving beyond the Dogma. Cells 8, 984. doi:10.3390/cells8090984
Fingleton, B. (2017). Matrix Metalloproteinases as Regulators of Inflammatory Processes. Biochimica Biophysica Acta (BBA) - Mol. Cell. Res. 1864, 2036–2042. doi:10.1016/j.bbamcr.2017.05.010
Ghajar, C. M., George, S. C., and Putnam, A. (2008). Matrix Metalloproteinase Control of Capillary Morphogenesis. Crit. Rev. Eukar Gene Expr. 18, 251–278. doi:10.1615/critreveukargeneexpr.v18.i3.30
Gifford, V., and Itoh, Y. (2019). MT1-MMP-dependent Cell Migration: Proteolytic and Non-proteolytic Mechanisms. Biochem. Soc. Trans. 47, 811–826. doi:10.1042/BST20180363
Hidalgo, M., and Eckhardt, S. G. (2001). Development of Matrix Metalloproteinase Inhibitors in Cancer Therapy. JNCI J. Natl. Cancer Inst. 93, 178–193. doi:10.1093/jnci/93.3.178
Hieta, N., Impola, U., López-Otín, C., Saarialho-Kere, U., and Kähäri, V.-M. (2003). Matrix Metalloproteinase-19 Expression in Dermal Wounds and by Fibroblasts in Culture. J. Investigative Dermatology 121, 997–1004. doi:10.1046/j.1523-1747.2003.12533.x
Holnthoner, W., Kerenyi, M., Gröger, M., Kratochvill, F., and Petzelbauer, P. (2006). Regulation of Matrilysin Expression in Endothelium by Fibroblast Growth Factor-2. Biochem. Biophysical Res. Commun. 342, 725–733. doi:10.1016/j.bbrc.2006.02.011
Jacob, A., and Prekeris, R. (2015). The Regulation of MMP Targeting to Invadopodia during Cancer Metastasis. Front. Cell. Dev. Biol. 3, 4. doi:10.3389/fcell.2015.00004
Ji, Y., Xiao, Y., Xu, L., He, J., Qian, C., Li, W., et al. (2018). Drug-Bearing Supramolecular MMP Inhibitor Nanofibers for Inhibition of Metastasis and Growth of Liver Cancer. Adv. Sci. 5, 1700867. doi:10.1002/advs.201700867
Jiang, Y., Goldberg, I. D., and Shi, Y. E. (2002). Complex Roles of Tissue Inhibitors of Metalloproteinases in Cancer. Oncogene 21, 2245–2252. doi:10.1038/sj.onc.1205291
Jin, C., Wang, K., Oppong-Gyebi, A., and Hu, J. (2020). Application of Nanotechnology in Cancer Diagnosis and Therapy - A Mini-Review. Int. J. Med. Sci. 17, 2964–2973. doi:10.7150/ijms.49801
Kaczorowska, A., Miękus, N., Stefanowicz, J., and Adamkiewicz-Drożyńska, E. (2020). Selected Matrix Metalloproteinases (MMP-2, MMP-7) and Their Inhibitor (TIMP-2) in Adult and Pediatric Cancer. Diagnostics 10, 547. doi:10.3390/diagnostics10080547
Kang, S.-g., Zhou, G., Yang, P., Liu, Y., Sun, B., Huynh, T., et al. (2012). Molecular Mechanism of Pancreatic Tumor Metastasis Inhibition by Gd@C 82 (OH) 22 and its Implication for De Novo Design of Nanomedicine. Proc. Natl. Acad. Sci. U.S.A. 109, 15431–15436. doi:10.1073/pnas.1204600109
Kessenbrock, K., Plaks, V., and Werb, Z. (2010). Matrix Metalloproteinases: Regulators of the Tumor Microenvironment. Cell. 141, 52–67. doi:10.1016/j.cell.2010.03.015
Kessenbrock, K., Wang, C.-Y., and Werb, Z. (2015). Matrix Metalloproteinases in Stem Cell Regulation and Cancer. Matrix Biol. 44-46, 184–190. doi:10.1016/j.matbio.2015.01.022
Köhrmann, A., Kammerer, U., Kapp, M., Dietl, J., and Anacker, J. (2009). Expression of Matrix Metalloproteinases (MMPs) in Primary Human Breast Cancer and Breast Cancer Cell Lines: New Findings and Review of the Literature. BMC Cancer 9, 188. doi:10.1186/1471-2407-9-188
Korbecki, J., Grochans, S., Gutowska, I., Barczak, K., and Baranowska-Bosiacka, I. (2020). CC Chemokines in a Tumor: A Review of Pro-cancer and Anti-cancer Properties of Receptors CCR5, CCR6, CCR7, CCR8, CCR9, and CCR10 Ligands. Ijms 21, 7619. doi:10.3390/ijms21207619
Kunz, P., Sähr, H., Lehner, B., Fischer, C., Seebach, E., and Fellenberg, J. (2016). Elevated Ratio of MMP2/MMP9 Activity Is Associated with Poor Response to Chemotherapy in Osteosarcoma. BMC Cancer 16, 223. doi:10.1186/s12885-016-2266-5
Lamouille, S., Xu, J., and Derynck, R. (2014). Molecular Mechanisms of Epithelial-Mesenchymal Transition. Nat. Rev. Mol. Cell. Biol. 15, 178–196. doi:10.1038/nrm3758
Landskron, G., De la Fuente, M., Thuwajit, P., Thuwajit, C., and Hermoso, M. A. (20142014). Chronic Inflammation and Cytokines in the Tumor Microenvironment. J. Immunol. Res. 2014, 1–19. doi:10.1155/2014/149185
Lee, K. H., Choi, E. Y., Hyun, M. S., Jang, B. I., Kim, T. N., Kim, S. W., et al. (2007). Association of Extracellular Cleavage of E-Cadherin Mediated by MMP-7 with HGF-Induced In Vitro Invasion in Human Stomach Cancer Cells. Eur. Surg. Res. 39, 208–215. doi:10.1159/000101452
Lin, H.-Y., Sun, S.-M., Lu, X.-F., Chen, P.-Y., Chen, C.-F., Liang, W.-Q., et al. (2017). CCR10 Activation Stimulates the Invasion and Migration of Breast Cancer Cells through the ERK1/2/MMP-7 Signaling Pathway. Int. Immunopharmacol. 51, 124–130. doi:10.1016/j.intimp.2017.07.018
Liu, T., Wu, X., Chen, S., Wu, P., Han, H., Zhang, H., et al. (2019). A Cationic Polymeric Prodrug with Chemotherapeutic Self-Sensibilization Co-delivering MMP-9 shRNA Plasmid for a Combined Therapy to Nasopharyngeal Carcinoma. Drug Deliv. 26, 1280–1291. doi:10.1080/10717544.2019.1698674
Liu, Y., and Cao, X. (2016). Characteristics and Significance of the Pre-metastatic Niche. Cancer Cell. 30, 668–681. doi:10.1016/j.ccell.2016.09.011
Lockhart, A. C., Braun, R. D., Yu, D., Ross, J. R., Dewhirst, M. W., Humphrey, J. S., et al. (2003). Reduction of Wound Angiogenesis in Patients Treated with BMS-275291, a Broad Spectrum Matrix Metalloproteinase Inhibitor. Clin. Cancer Res. 9, 586–593.
Loffek, S., Schilling, O., and Franzke, C.-W. (2011). Biological Role of Matrix Metalloproteinases: a Critical Balance. Eur. Respir. J. 38, 191–208. LP – 208. doi:10.1183/09031936.00146510
Lu, P., Takai, K., Weaver, V. M., and Werb, Z. (2011). Extracellular Matrix Degradation and Remodeling in Development and Disease. Cold Spring Harb. Perspect. Biol. 3, a005058. doi:10.1101/cshperspect.a005058
Lugano, R., Ramachandran, M., and Dimberg, A. (2020). Tumor Angiogenesis: Causes, Consequences, Challenges and Opportunities. Cell. Mol. Life Sci. 77, 1745–1770. doi:10.1007/s00018-019-03351-7
Lyu, Y., Xiao, Q., Yin, L., Yang, L., and He, W. (2019). Potent Delivery of an MMP Inhibitor to the Tumor Microenvironment with Thermosensitive Liposomes for the Suppression of Metastasis and Angiogenesis. Sig Transduct. Target Ther. 4, 26. doi:10.1038/s41392-019-0054-9
Mach, F., Schönbeck, U., Fabunmi, R. P., Murphy, C., Atkinson, E., Bonnefoy, J.-Y., et al. (1999). T Lymphocytes Induce Endothelial Cell Matrix Metalloproteinase Expression by a CD40L-dependent Mechanism. Am. J. Pathology 154, 229–238. doi:10.1016/S0002-9440(10)65269-8
Marshall, D. C., Lyman, S. K., McCauley, S., Kovalenko, M., Spangler, R., Liu, C., et al. (2015). Selective Allosteric Inhibition of MMP9 Is Efficacious in Preclinical Models of Ulcerative Colitis and Colorectal Cancer. PLoS One 10, e0127063. doi:10.1371/journal.pone.0127063
Martínez-Rodríguez, M., and Monteagudo, C. (2021). CCL27 Signaling in the Tumor Microenvironment. Adv. Exp. Med. Biol. 1302, 113–132. doi:10.1007/978-3-030-62658-7_9
Mazza, M., Ahmad, H., Hadjidemetriou, M., Agliardi, G., Pathmanaban, O. N., King, A. T., et al. (2019). Hampering Brain Tumor Proliferation and Migration Using Peptide nanofiber:siPLK1/MMP2 Complexes. Nanomedicine 14, 3127–3142. doi:10.2217/nnm-2019-0298
Merchant, N., Nagaraju, G. P., Rajitha, B., Lammata, S., Jella, K. K., Buchwald, Z. S., et al. (2017). Matrix Metalloproteinases: Their Functional Role in Lung Cancer. Carcinogenesis 38, 766–780. doi:10.1093/carcin/bgx063
Mescher, A. L. (2017). Macrophages and Fibroblasts during Inflammation and Tissue Repair in Models of Organ Regeneration. Regeneration 4, 39–53. doi:10.1002/reg2.77
Miller, T. W., Isenberg, J. S., and Roberts, D. D. (2009). Molecular Regulation of Tumor Angiogenesis and Perfusion via Redox Signaling. Chem. Rev. 109, 3099–3124. doi:10.1021/cr8005125
Mitsiades, N., Anderson, K. C., Poulaki, V., and Mitsiades, C. S. (2001). Induction of Tumour Cell Apoptosis by Matrix Metalloproteinase Inhibitors: New Tricks from a (Not So) Old Drug. Expert Opin. Investigational Drugs 10, 1075–1084. doi:10.1517/13543784.10.6.1075
Mittal, R., Patel, A. P., Debs, L. H., Nguyen, D., Patel, K., Grati, M. h., et al. (2016). Intricate Functions of Matrix Metalloproteinases in Physiological and Pathological Conditions. J. Cell. Physiol. 231, 2599–2621. doi:10.1002/jcp.25430
Monteagudo, C., Ramos, D., Pellín-Carcelén, A., Gil, R., Callaghan, R. C., Martín, J. M., et al. (2012). CCL27-CCR10 and CXCL12-CXCR4 Chemokine Ligand-Receptor mRNA Expression Ratio: New Predictive Factors of Tumor Progression in Cutaneous Malignant Melanoma. Clin. Exp. Metastasis 29, 625–637. doi:10.1007/s10585-012-9476-2
Moogk, D., da Silva, I. P., Ma, M. W., Friedman, E. B., de Miera, E. V.-S., Darvishian, F., et al. (2014). Melanoma Expression of Matrix Metalloproteinase-23 Is Associated with Blunted Tumor Immunity and Poor Responses to Immunotherapy. J. Transl. Med. 12, 342. doi:10.1186/s12967-014-0342-7
Mukherjee, P. K., Maity, N., Nema, N. K., and Sarkar, B. K. (2013). “Natural Matrix Metalloproteinase Inhibitors,” in Studies in Natural Products Chemistry. Editor F. R. S. Atta-ur-Rahman (Karachi, Pakistan: Elsevier), 91–113. doi:10.1016/B978-0-444-62615-8.00003-5
Na, T.-Y., Schecterson, L., Mendonsa, A. M., and Gumbiner, B. M. (2020). The Functional Activity of E-Cadherin Controls Tumor Cell Metastasis at Multiple Steps. Proc. Natl. Acad. Sci. U.S.A. 117, 5931–5937. doi:10.1073/pnas.1918167117
Naim, A., Pan, Q., and Baig, M. S. (2017). Matrix Metalloproteinases (MMPs) in Liver Diseases. J. Clin. Exp. Hepatology 7, 367–372. doi:10.1016/j.jceh.2017.09.004
Nasry, W., Rodriguez-Lecompte, J., and Martin, C. (2018). Role of COX-2/PGE2 Mediated Inflammation in Oral Squamous Cell Carcinoma. Cancers 10, 348. doi:10.3390/cancers10100348
Niedzwiecki, M. W., Monterrey, J. C., Kalinovsky, T., Rath, M., and Niedzwiecki, A. (2009). Patterns of MMP-2 and MMP-9 Expression in Human Cancer Cell Lines. Oncol. Rep. 21, 1323–1333. doi:10.3892/or_00000358
Nieto, M. A., Huang, R. Y.-J., Jackson, R. A., and Thiery, J. P. (2016). EMT: 2016. Cell. 166, 21–45. doi:10.1016/j.cell.2016.06.028
Oda, H., and Takeichi, M. (2011). Structural and Functional Diversity of Cadherin at the Adherens Junction. J. Cell. Biol. 193, 1137–1146. doi:10.1083/jcb.201008173
Orbe, J., Rodríguez, J. A., Calvayrac, O., Rodríguez-Calvo, R., Rodríguez, C., Roncal, C., et al. (2009). Matrix Metalloproteinase-10 Is Upregulated by Thrombin in Endothelial Cells and Increased in Patients with Enhanced Thrombin Generation. Atvb 29, 2109–2116. doi:10.1161/ATVBAHA.109.194589
Oviedo-Orta, E., Bermudez-Fajardo, A., Karanam, S., Benbow, U., and Newby, A. C. (2008). Comparison of MMP-2 and MMP-9 Secretion from T Helper 0, 1 and 2 Lymphocytes Alone and in Coculture with Macrophages. Immunology 124, 42–50. doi:10.1111/j.1365-2567.2007.02728.x
Pandey, K. B., and Rizvi, S. I. (2009). Plant Polyphenols as Dietary Antioxidants in Human Health and Disease. Oxidative Med. Cell. Longev. 2, 270–278. doi:10.4161/oxim.2.5.9498
Pang, L., Li, Q., Li, S., He, J., Cao, W., Lan, J., et al. (2016). Membrane Type 1-matrix Metalloproteinase Induces Epithelial-To-Mesenchymal Transition in Esophageal Squamous Cell Carcinoma: Observations from Clinical and In Vitro Analyses. Sci. Rep. 6, 22179. doi:10.1038/srep22179
Patra, J. K., Das, G., Fraceto, L. F., Campos, E. V. R., Rodriguez-Torres, M. d. P., Acosta-Torres, L. S., et al. (2018). Nano Based Drug Delivery Systems: Recent Developments and Future Prospects. J. Nanobiotechnol 16, 71. doi:10.1186/s12951-018-0392-8
Paz, H., Pathak, N., and Yang, J. (2014). Invading One Step at a Time: the Role of Invadopodia in Tumor Metastasis. Oncogene 33, 4193–4202. doi:10.1038/onc.2013.393
Pei, D. (1999). Leukolysin/MMP25/MT6-MMP: a Novel Matrix Metalloproteinase Specifically Expressed in the Leukocyte Lineage. Cell. Res. 9, 291–303. doi:10.1038/sj.cr.7290028
Peinado, H., Zhang, H., Matei, I. R., Costa-Silva, B., Hoshino, A., Rodrigues, G., et al. (2017). Pre-metastatic Niches: Organ-specific Homes for Metastases. Nat. Rev. Cancer 17, 302–317. doi:10.1038/nrc.2017.6
Quintero-Fabián, S., Arreola, R., Becerril-Villanueva, E., Torres-Romero, J. C., Arana-Argáez, V., Lara-Riegos, J., et al. (2019). Role of Matrix Metalloproteinases in Angiogenesis and Cancer. Front. Oncol. 9. doi:10.3389/fonc.2019.01370
Radisky, E. S., and Radisky, D. C. (2010). Matrix Metalloproteinase-Induced Epithelial-Mesenchymal Transition in Breast Cancer. J. Mammary Gland. Biol. Neoplasia 15, 201–212. doi:10.1007/s10911-010-9177-x
Rautava, J., Gürsoy, U. K., Kullström, A., Könönen, E., Sorsa, T., Tervahartiala, T., et al. (2020). An Oral Rinse Active Matrix Metalloproteinase-8 Point-of-Care Immunotest May Be Less Accurate in Patients with Crohn's Disease. Biomolecules 10, 395. doi:10.3390/biom10030395
Ren, T., Zhu, L., and Cheng, M. (2017). CXCL10 Accelerates EMT and Metastasis by MMP-2 in Hepatocellular Carcinoma. Am. J. Transl. Res. 9, 2824–2837. Available at: https://pubmed.ncbi.nlm.nih.gov/28670372.
Rizvi, S. A. A., and Saleh, A. M. (2018). Applications of Nanoparticle Systems in Drug Delivery Technology. Saudi Pharm. J. 26, 64–70. doi:10.1016/j.jsps.2017.10.012
Rosemurgy, A., Harris, J., Langleben, A., Casper, E., Goode, S., and Rasmussen, H. (1999). Marimastat in Patients with Advanced Pancreatic Cancer. Am. J. Clin. Oncol. 22, 247–252. doi:10.1097/00000421-199906000-00007
Rosenblum, D., Joshi, N., Tao, W., Karp, J. M., and Peer, D. (2018). Progress and Challenges towards Targeted Delivery of Cancer Therapeutics. Nat. Commun. 9, 1410. doi:10.1038/s41467-018-03705-y
Rosenthal, E. L., and Matrisian, L. M. (2006). Matrix Metalloproteases in Head and Neck Cancer. Head. Neck 28, 639–648. doi:10.1002/hed.20365
Said, A., Raufman, J.-P., and Xie, G. (2014). The Role of Matrix Metalloproteinases in Colorectal Cancer. Cancers 6, 366–375. doi:10.3390/cancers6010366
Sato, H., and Takino, T. (2010). Coordinate Action of Membrane-type Matrix Metalloproteinase-1 (MT1-MMP) and MMP-2 Enhances Pericellular Proteolysis and Invasion. Cancer Sci. 101, 843–847. doi:10.1111/j.1349-7006.2010.01498.x
Shay, G., Lynch, C. C., and Fingleton, B. (2015). Moving Targets: Emerging Roles for MMPs in Cancer Progression and Metastasis. Matrix Biol. 44-46, 200–206. doi:10.1016/j.matbio.2015.01.019
Shiomi, T., Lemaître, V., D'Armiento, J., and Okada, Y. (2010). Matrix Metalloproteinases, a Disintegrin and Metalloproteinases, and a Disintegrin and Metalloproteinases with Thrombospondin Motifs in Non-neoplastic Diseases. Pathol. Int. 60, 477–496. doi:10.1111/j.1440-1827.2010.02547.x
Singh, A., and Settleman, J. (2010). EMT, Cancer Stem Cells and Drug Resistance: an Emerging axis of Evil in the War on Cancer. Oncogene 29, 4741–4751. doi:10.1038/onc.2010.215
Singh, D., Srivastava, S. K., Chaudhuri, T. K., and Upadhyay, G. (2015). Multifaceted Role of Matrix Metalloproteinases (MMPs). Front. Mol. Biosci. 2. doi:10.3389/fmolb.2015.00019
Skoog, T., Ahokas, K., Orsmark, C., Jeskanen, L., Isaka, K., and Ulpu, S.-K. (2006). MMP-21 Is Expressed by Macrophages and Fibroblasts In Vivo and in Culture. Exp. Dermatol. 15 (10), 775–783. doi:10.1111/j.1600-0625.2006.00460.x
Stetler-Stevenson, W. G. (1999). Matrix Metalloproteinases in Angiogenesis: a Moving Target for Therapeutic Intervention. J. Clin. Invest. 103, 1237–1241. doi:10.1172/JCI6870
Tang, Q., Ma, X., Zhang, Y., Cai, X., Xue, W., and Ma, D. (2018). Self-sensibilized Polymeric Prodrug Co-delivering MMP-9 shRNA Plasmid for Combined Treatment of Tumors. Acta Biomater. 69, 277–289. doi:10.1016/j.actbio.2018.01.014
Valastyan, S., and Weinberg, R. A. (2011). Tumor Metastasis: Molecular Insights and Evolving Paradigms. Cell. 147, 275–292. doi:10.1016/j.cell.2011.09.024
Vihinen, P., and Kähäri, V.-M. (2002). Matrix Metalloproteinases in Cancer: Prognostic Markers and Therapeutic Targets. Int. J. Cancer 99, 157–166. doi:10.1002/ijc.10329
Walker, C., Mojares, E., and del Río Hernández, A. (2018). Role of Extracellular Matrix in Development and Cancer Progression. Ijms 19, 3028. doi:10.3390/ijms19103028
Westermarck, J., Li, S.-P., Kallunki, T., Han, J., and Kähäri, V.-M. (2001). p38 Mitogen-Activated Protein Kinase-dependent Activation of Protein Phosphatases 1 and 2A Inhibits MEK1 and MEK2 Activity and Collagenase 1 (MMP-1) Gene Expression. Mol. Cell. Biol. 21, 2373–2383. doi:10.1128/MCB.21.7.2373-2383.2001
Wilson, S. R., Gallagher, S., Warpeha, K., and Hawthorne, S. J. (2004). Amplification of MMP-2 and MMP-9 Production by Prostate Cancer Cell Lines via Activation of Protease-Activated Receptors. Prostate 60, 168–174. doi:10.1002/pros.20047
Winer, A., Adams, S., and Mignatti, P. (2018). Matrix Metalloproteinase Inhibitors in Cancer Therapy: Turning Past Failures into Future Successes. Mol. Cancer Ther. 17, 1147–1155. doi:10.1158/1535-7163.MCT-17-0646
Wojtowicz-Praga, S. M., Dickson, R. B., and Hawkins, M. J. (1997). Matrix Metalloproteinase Inhibitors. Invest. New Drugs 15, 61–75. doi:10.1023/a:1005722729132
Yan, X., Cao, N., Chen, Y., Lan, H.-Y., Cha, J.-H., Yang, W.-H., et al. (2020). MT4-MMP Promotes Invadopodia Formation and Cell Motility in FaDu Head and Neck Cancer Cells. Biochem. Biophysical Res. Commun. 522, 1009–1014. doi:10.1016/j.bbrc.2019.12.009
Yang, F., Chen, Q., Yang, M., Maguire, E. M., Yu, X., He, S., et al. (2020). Macrophage-derived MMP-8 Determines Smooth Muscle Cell Differentiation from Adventitia Stem/progenitor Cells and Promotes Neointima Hyperplasia. Cardiovasc. Res. 116, 211–225. doi:10.1093/cvr/cvz044
Yang, J., Mani, S. A., Donaher, J. L., Ramaswamy, S., Itzykson, R. A., Come, C., et al. (2004). Twist, a Master Regulator of Morphogenesis, Plays an Essential Role in Tumor Metastasis. Cell. 117, 927–939. doi:10.1016/j.cell.2004.06.006
Yue, B. (2014). Biology of the Extracellular Matrix. J. Glaucoma 23, S20–S23. doi:10.1097/IJG.0000000000000108
Zhang, C., and Kim, S.-K. (2009). Matrix Metalloproteinase Inhibitors (MMPIs) from Marine Natural Products: the Current Situation and Future Prospects. Mar. Drugs 7, 71–84. doi:10.3390/md7020071
Zhang, P., Sun, Y., and Ma, L. (2015). ZEB1: at the Crossroads of Epithelial-Mesenchymal Transition, Metastasis and Therapy Resistance. Cell. Cycle 14, 481–487. doi:10.1080/15384101.2015.1006048
Zhang, Y., and Chen, Q. (2017). Relationship between Matrix Metalloproteinases and the Occurrence and Development of Ovarian Cancer. Braz J. Med. Biol. Res. 50, e6104. doi:10.1590/1414-431X20176104
Keywords: MMPs (metalloproteinases), cancer, angiogenesis, stem cells, ECM
Citation: Mustafa S, Koran S and AlOmair L (2022) Insights Into the Role of Matrix Metalloproteinases in Cancer and its Various Therapeutic Aspects: A Review. Front. Mol. Biosci. 9:896099. doi: 10.3389/fmolb.2022.896099
Received: 14 March 2022; Accepted: 16 June 2022;
Published: 29 September 2022.
Edited by:
Shiv Bharadwaj, Yeungnam University, South KoreaReviewed by:
Sanjay Kumar, Jawaharlal Nehru University, IndiaRamachandran Vinayagam, Yeungnam University, South Korea
Copyright © 2022 Mustafa, Koran and AlOmair. This is an open-access article distributed under the terms of the Creative Commons Attribution License (CC BY). The use, distribution or reproduction in other forums is permitted, provided the original author(s) and the copyright owner(s) are credited and that the original publication in this journal is cited, in accordance with accepted academic practice. No use, distribution or reproduction is permitted which does not comply with these terms.
*Correspondence: Sabeena Mustafa, msabeena@gmail.com