- 1General Surgery Department, Wuhu Hospital of Traditional Chinese Medicine, Wuhu, China
- 2The First Affiliated Hospital of Wannan Medical College, Wuhu, China
- 3School of Basic Medicine, Wannan Medical College, Wuhu, China
Background: Ferroptosis induced by SLC7A11 has an important translational value in the treatment of cancers. However, the mechanism of SLC7A11 in the pathogenesis of colon adenocarcinoma (COAD) is rarely studied in detail.
Methods: SLC7A11 expression was explored with The Cancer Genome Atlas (TCGA), Gene Expression Omnibus (GEO) databases, and Western blot assay. The correlation of SLC7A11 expression with the abundance of infiltrating immune cells was evaluated via the TIMER database. The relation of SLC7A11 expression with immune cell markers was investigated via Gene Expression Profiling Interactive Analysis (GEPIA). The co-expression genes of SLC7A11 were screened by R packages, and the PPI was constructed via the STRING database. SLC7A11 and co-expressed gene modulators were selected by NetworkAnalyst and DSigDB database. The correlations between SLC7A11 and cancer immune characteristics were analyzed via the TIMER and TISIDB databases.
Results: SLC7A11 is overexpressed in most tumors, including COAD. The expression level of SLC7A11 has a significant correlation with the infiltration levels of CD8+ T cells, neutrophils, and dendritic cells in COAD. The infiltrated lymphocyte markers of Th1 cell such as TBX21, IL12RB2, IL27RA, STAT1, and IFN-γ were strongly correlated with SLC7A11 expression. Five hub genes co-expressed with SLC7A11 that induce ferroptosis were identified, and mir-335-5p, RELA, and securinine have regulatory effects on it. SLC7A11 was negatively correlated with the expression of chemokines and chemokine receptors, such as CCL17, CCL19, CCL22, CCL23, CXCL14, CCR10, CX3CR1, and CXCR3, in COAD.
Conclusion: SLC7A11 may play a role in induced ferroptosis and regulating tumor immunity, which can be considered as potential therapeutic targets in COAD.
Introduction
Colon adenocarcinoma (COAD) is one of the most common malignant tumors of the digestive system; the diagnosis rate and the mortality rate represent about 1/10 of the total cancer cases (El Kinany et al., 2020). In recent years, the incidence of COAD is increasing annually, with the highest incidence in China (Roslan et al., 2019). Traditional treatments for COAD include radiation, chemotherapy, and surgery. Currently, new therapeutic methods such as bio-targeted therapy, precision therapy, and immunotherapy have been gradually applied in the treatment of colon cancer (Sandhu et al., 2019; Bao et al., 2020). However, drug resistance and immune escape restrict the therapeutic effect of these therapies (Fidelle et al., 2020). Therefore, finding new therapeutic targets to halt or slow disease progression is an urgent priority.
Studies showed that ferroptosis as a novel-induced programmed cell death, which can effectively inhibit tumor growth by inducing ferroptosis of tumor cells (Mou et al., 2019), and it also plays a critical role in reversing cisplatin resistance of tumors (Guo et al., 2018). Therefore, modulating ferroptosis may have important transforming significance in various ferroptosis-associated diseases. Glutathione peroxidase 4 (GPX4) was shown to be a central regulator of ferroptosis; glutathione (GSH) is a necessary cofactor for the biological activity of GPX4; GSH synthesis depends on the Gys/Glu reverse transfer system (Xc-); System Xc- consists of two subunits, including solute carrier family 7, membrane 11 (SLC7A11), and heavy chain subunit SLC3A2; SLC7A11 is responsible for the primary activity of System Xc-, while SLC3A2 plays an auxiliary coordinating role (Xie et al., 2016; Koppula et al., 2018; Song et al., 2018). Latest studies have shown that 2-imino-6-methoxy-2H-chromene-3-carbothioamide (IMCA) downregulates SLC7A11 expression through the AMPK/mTOR pathway and induces ferroptosis (Zhang et al., 2020). However, whether SLC7A11 can be an effective target for COAD treatment needs more theoretical support.
In this study, bioinformatics methods were used to analyze SLC7A11 expression and clinical information in COAD patients. Then, we used the TIMER and GEPIA databases to study the relationship between SLC7A11 expression and infiltrating immune cells and their corresponding gene marker sets. In addition, the protein–protein interaction network of SLC7A11 was explored by STRING. The results showed that the overexpression of SLC7A11 inhibited the ferroptosis of tumor cells and promoted the development and metastasis of COAD. Targeted inhibition of SLC7A11 may be a promising therapeutic strategy for inducing ferroptosis or in combination with immunotherapy for COAD.
Materials and Methods
Clinical Samples and Ethics
Six patients undergoing radical resection of colon cancer were collected in the First Affiliated Hospital of Wannan Medical College and pathologically diagnosed with colon cancer. None of them received chemotherapy or immunotherapy before surgery. Tumor tissues and paracancerous tissues were collected from the six patients (the adjacent normal tissues were extracted >3 cm from the tumor margin). This study was approved by the Ethics Committee of Wannan Medical College (NO. 2021081).
Data Source
The Cancer Genome Atlas (TCGA) (https://genomecancer.ucsc.edu/), a free data portal of largescale cancer genome project. The data of COAD patients with the expression of RNA-Seq and matching clinical information (include pathologic stage, histologic grade, OS time, gender, and age) were obtained by the TCGA tools.
Retrieval of Datasets
In order to compare the expression level of SLC7A11 in cancer and normal tissues, two datasets were involved in this study, GSE21510 and GSE24514, where both were from the GEO database (https://www.ncbi.nlm.nih.gov/geo/). In GSE21510, the expression profiles of cancer cells in 104 patients with colorectal cancer were examined by laser microdissection and oligonucleotide microarray analysis (Tsukamoto et al., 2011). GSE24514 illustrated microsatellite instability in colorectal cancer patients (Alhopuro et al., 2012).
Western Blot (WB)
Total proteins were extracted from the cancer and paracancerous tissues, after protein concentration was detected, WB assay was performed. The primary antibodies used were as follows: SLC7A11 antibody (Abcam, ab175186) (diluted at 1:5,000) and GAPDH recombinant antibody (proteintech, 80570-1-RR) (diluted at 1:5,000) at room temperature (RT) for 1 h. After washing three times with TBST, 5 min/wash, the membrane was incubated with HRP-labeled goat antirabbit IgG (Beyotime, A0208) (diluted at 1:1,000) as the secondary antibody for 1 h at RT. Finally, the signal was detected by chemiluminescence, and the bands’ gray value was measured using ImageJ software.
Correlation Analysis Between SLC7A11 Expression and Immune Cell Infiltration
The correlation of SLC7A11 expression with the abundance of infiltrating immune cells, including tumor-associated macrophage (TAM), neutrophils, macrophages, dendritic cells, B cells, CD8+ T cells, CD4+ T cells, and their subtypes in COAD patients was evaluated via the TIMER database (http://cistrome.org/TIMER/). The relationship between the expression of the SLC7A11 gene and tumor purity was also displayed.
Correlation Analysis of SLC7A11 Expression and Immune Cell Markers
The relation of SLC7A11 expression with multiple markers for immune cells was investigated via The Gene Expression Profiling Interactive Analysis (GEPIA) (http://gepia.cancer-pku.cn/index.html). Moreover, we used TIMER data to validate the genes which were of significant correlation with SLC7A11 expression in the GEPIA web.
Co-Expression Network Establishment of SLC7A11 and GO–KEGG Analysis
The co-expression genes of SLC7A11 were screened by R packages (limma). The correlation between the SLC7A11 expression level and co-expression genes were examined by Pearson correlation coefficients and the Z-test from the TCGA databases (related parameter settings: |Pearson correlation coefficient| > 0.5 and p-value <0.001). The STRING database (v11.5, https://www.string-db.org/) was used to construct the protein–protein interactions (PPI). In addition, GO–KEGG enrichment analysis was performed by Bioconductor package ‘‘clusterProfiler.”
Construction of the TF-miRNA Co-Regulatory Network
The NetworkAnalyst database (https://www.networkanalyst.ca/) was used to identify TF-miRNA co-regulate with co-expression genes. The common network topology measures were also computed based on well-established igraph R package. TF and gene target data were derived from ENCODE ChIP-seq data. Only the peak intensity signal <500 and the predicted regulatory potential score <1 were used.
Screening of Potential Therapeutic Drugs
The Enrichr platform was used for drug screening of SLC7A11 and co-expression genes. The access of the DSigDB database was acquired using Enrichr (https://amp.pharm.mssm.edu/Enrichr/). Potential therapeutic agents were determined by the adj. p values and the abundance of acting on SLC7A11 and co-expression genes.
TISIDB Database Analysis
To study the association between SLC7A11 and chemokine/chemokine receptor expression, we evaluated the expression levels of chemokine/chemokine receptors of tumor-infiltrating immune cells through the “chemokine” module of the TISIDB database (http://cis.hku.hk/TISIDB/). To further clarify the immune correlation of SLC7A11 in cancer, the “immunomodulator” module was used to analyze and evaluate the correlation between SLC7A11 expression and the levels of immune checkpoint genes.
Enzyme-Linked Immunosorbent Assay (ELISA)
The levels of CCL17, CXCL14, and CXCR3 in cancer and paracancerous tissues were measured by ELISA kits from Yuanju, Inc. (Shanghai, China) (Yuanju, YJ779969/YJ714549/YJ719414) according to the manufacturer’s protocol.
Results
Patient Characteristics
In total, the RNA-sequencing data and detailed clinical prognostic information resources of 478 COAD samples were obtained from the TCGA database. The clinical information including tumor extent (T), lymph node invasion (N), detectable metastasis (M), histologic grade, age at diagnosis, overall survival (OS), and lymphatic invasion are summarized in Table 1.
Higher SLC7A11 Expression in Tumor Samples Than That in Normal Tissues
The mRNA expression level of SLC7A11 was analyzed in various cancer types (Figure 1A). The gene expression level of SLC7A11 was significantly higher in tumor samples than that in normal tissues of COAD in TCGA and GEO databases (p < 0.01, Figure 1B). The expression of SLC7A11 is downregulated in COAD tissue when lymphatic invasion occurs (p < 0.01, Figure 1C). However, SLC7A11 expression is relatively stable in all pathologic stages of COAD (p > 0.05, Figure 1D).
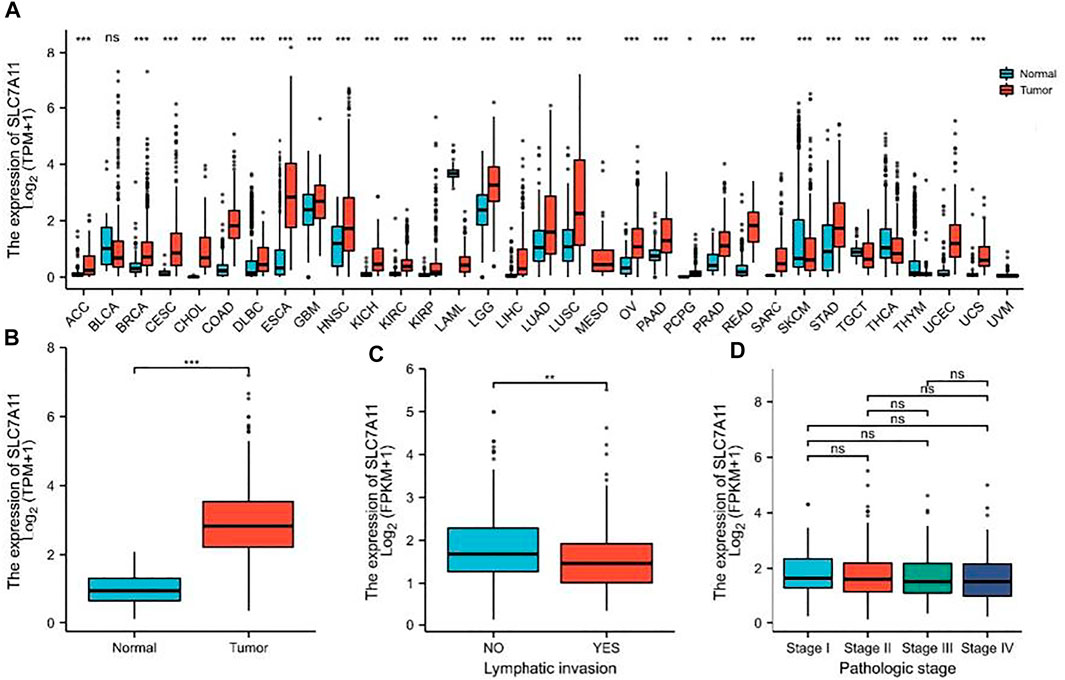
FIGURE 1. SLC7A11 expression status in pan-cancer. (A) Expression of human SLC7A11 in distinct cancers compared with normal tissues. (B) Expression of SLC7A11 in COAD compared with normal tissues, n (normal) = 41 and n (tumor) = 480. (C) Comparison of SLC7A11 expression levels in lymphatic invasion, n (NO) = 266 and n (YES) = 168. (D) There was no significant difference in the SLC7A11 mRNA level among different pathological stages, n (Stage I, II, III, IV) = 81, 187, 133, 66, respectively. *p < 0.05. **p < 0.01. ***p < 0.001. ns p > 0.05.
In addition, the high expression of SLC7A11 in COAD was also observed in two datasets of the GEO database (Figure 2A), and the aforementioned results were also verified by WB (Figure 2B).
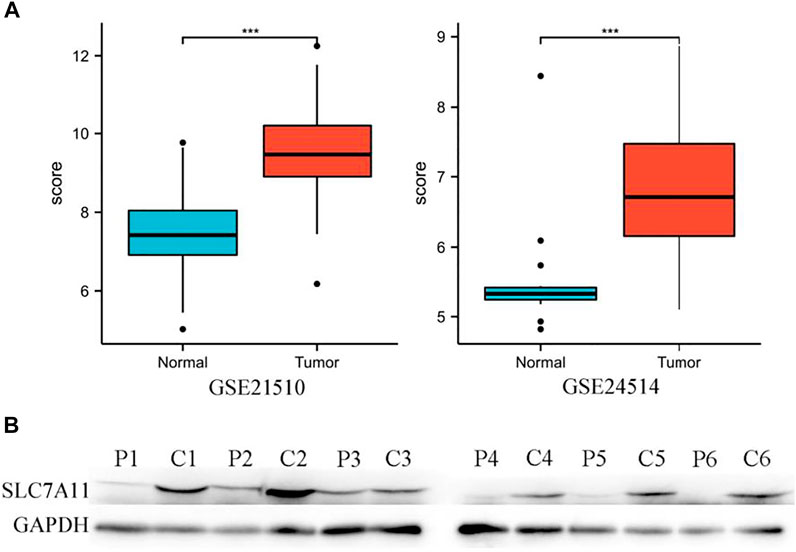
FIGURE 2. High expression of SLC7A11 in colon cancer. (A) Analysis of SLC7A11 gene expression in GEO datasets, n (normal) = 25 and n (tumor) = 123 in GSE21510, n (normal) = 15 and n (tumor) = 34 in GSE24514. ***p < 0.001. (B) Expression levels of SLC7A11 were detected by Western blot analysis, P1-6: paracancerous tissues of patients 1–6, C1-6: cancer tissues of patients 1–6, the bands gray value of C1-6 significantly higher than the P1-6 (p < 0.01). Correlation analysis between SLC7A11 expression and infiltrating immune cells.
The expression level of SLC7A11 had obviously positive correlation with infiltrating levels of CD8+ T cells (r = 0.358, p = 1.03e-13), neutrophils (r = 0.267, p = 5.72e-08), and dendritic cells (r = 0.171, p = 5.65e-04) in COAD, but it was negatively correlated with tumor purity (r = -0.104, p = 3.59e-02) (Figure 3).
To intensely explore the possible role of SLC7A11 in the infiltration of various immune cells in COAD, the GEPIA and TIMER databases were used to execute the relationships between SLC7A11 and several immune marker sets. Furthermore, various functional T cells including Th1, Th2, Th17, and Treg were also been examined in this study. Results showed that the levels of most immune sets marking different T cells, TAMs, macrophages, monocytes, and DCs were associated with the SLC7A11 expression in COAD (Table 2).
Network Establishment for SLC7A11-Correlated Genes in COAD
To further study the genes closely associated with SLC7A11 in COAD, the top 50 co-expressed genes were showed in a heatmap (Figure 4). Of note, 25 of 50 genes were negatively correlated with SLC7A11 (such as PRDX5, CLDN3, ROMO1, and VEGFB), and 25 genes were positively correlated (such as LARP1B,AHR,AP1S3,IBTK, and SPATA5) (Figure 4A). GO–KEGG enrichment analysis showed that the co-expression genes of SLC7A11 were mainly involved in the proteasomal protein catabolic process, Golgi vesicle transport, chromosome segregation, and other signaling pathways (Figure 4B).
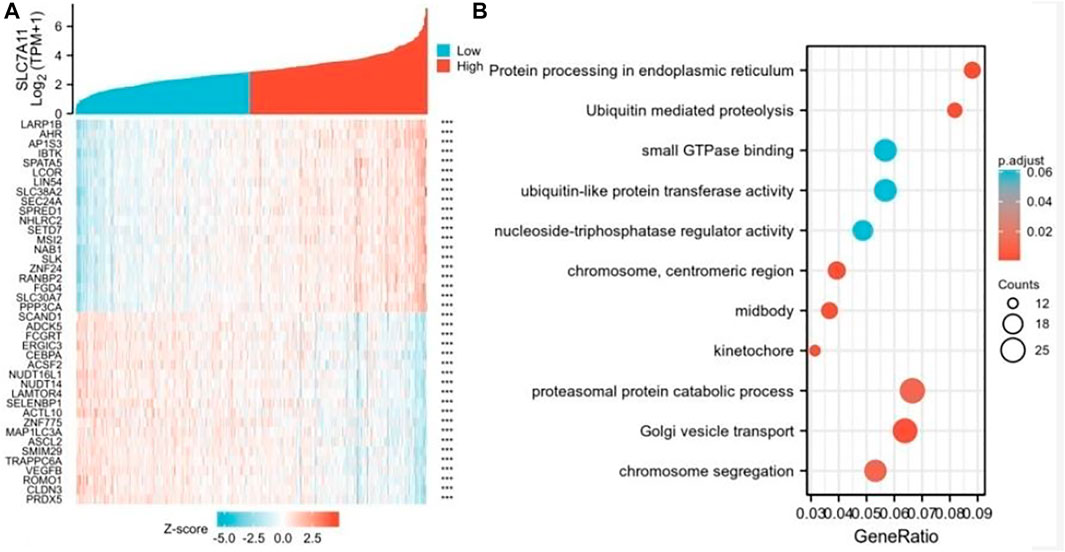
FIGURE 4. Genes analysis of closely associated with SLC7A11 in COAD. (A) The top 50 genes correlated with SLC7A11 in COAD are showed in the heatmap; ***p < 0.01. (B) GO–KEGG enrichment analysis of co-expression genes with SLC7A11 in COAD.
PPI and the TF-miRNA Co-regulatory Network
To determine the role of SLC7A11 protein interactions in COAD progression, protein–protein interaction (PPI) was constructed by the STRING tool. The proteins that interact most closely with SLC7A11 include GPX4, GCLC, GCLM, SLC3A2, SLC1A7, SLC1A5, SLC3A1, SLC1A2, CD44, and BECN1; among which SLC7A11, GPX4, GCLC, GCLM, and SLC3A2 are involved in the ferroptosis signaling pathway (Figure 5A). Furthermore, 193 miRNAs were identified that regulated the expression level of co-expression genes by NetworkAnalyst analysis, and the main co-regulated genes were SLC7A11, SLC1A2, SLC1A5, BECN1, GCLC, and CD44. The miRNA that hit more frequently were hsa-mir-335-5p, hsa-mir-155-5p, hsa-mir-34a-5p, hsa-mir-16-5p, hsa-mir-20a-5p, hsa-mir-93-5p, hsa-mir-98-5p, and hsa-mir-24-3p. The TF that hit more frequently were RELA, SP1, NFKB1, TP53, BRCA1, JUN, and ESR1 (Figure 5B).
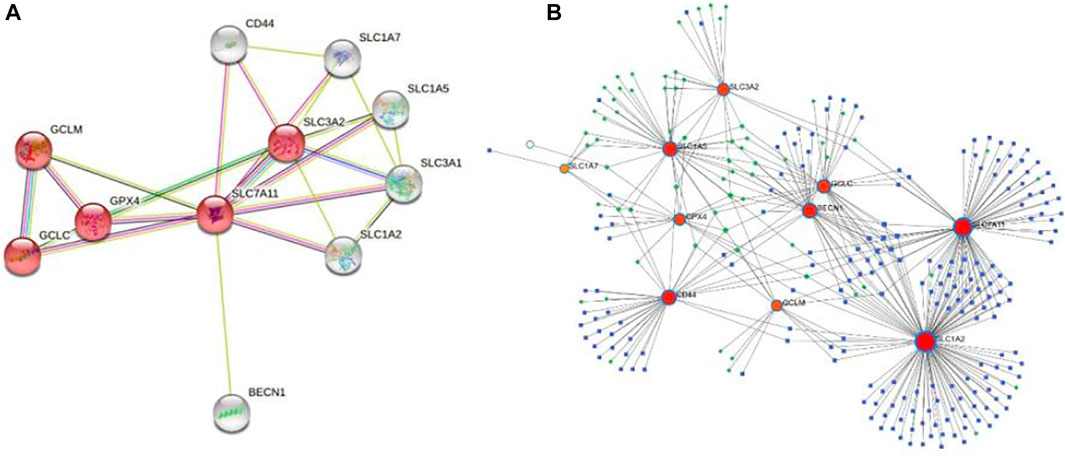
FIGURE 5. PPI and the co-regulatory network of SLC7A11. (A) SLC7A11-interaction proteins in COAD tissue. The network consists of 11 nodes and 23 edges, the red nodes are involved in the ferroptosis signaling pathway. (B) TF-miRNA co-regulatory network. The network consists of 270 nodes and 397 edges. The nodes in red color are the co-expression genes, the blue nodes represent miRNA, the green nodes represent TFs, and the yellow nodes represent protein.
Screening of Potential Therapeutic Drugs
Enrichr platform analysis found that F0447-0125 PC3 UP, semustine PC3 UP, securinine PC3 UP, alpha-tocopherol CTD 00007387, ampicillin CTD 00005394 etc. have been identified as drugs that regulate action for both SLC7A11 and co-expression genes (Table 3).
Correlation of SLC7A11 Expression With Immune Characteristics
The correlation between the expression level of SLC7A11 and immune cell chemokines (or receptors) in COAD were analyzed via the TISIDB database. The results showed that several chemokines and chemokine receptors were significantly correlated with the expression of SLC7A11 in COAD. Concretely, SLC7A11 expression was negatively correlated with CCL17 (r = -0.24 and p = 2.07e-07), CCL19 (r = -0.201 and p = 1.44e-05), CCL23 (r = -0.27 and p = 4.96e-09), CXCL14 (r = -0.289 and p = 3.25e-10), CCR10 (r = -0.33 and p = 5.11e-13), CX3CR1 (r = -0.203 and p = 1.2e-05), CXCR3 (r = -0.332 and p = 4.17e-13) etc (Figures 6A,B). Subsequently, the correlation between SLC7A11 and the expressions of immunoinhibitors (or immunostimulators) in different cancers were analyzed. The results showed that SLC7A11 was negatively correlated with the expression of several immunoinhibitors and immunostimulators, such as ADORA2A (r = −0.224, p = 1.36e-06), CSF1R (r = −0.176, p = 1.54e-04), TGFB1 (r = −0.162, p = 4.95e-04), CD27 (r = −0.247, p = 9.01e-08), LTA (r = −0.181, p = 1.01e-04), TMIGD2 (r = −0.141, p = 2.47e-03), TNFRSF13B (r = −0.248, p = 7.64e-08) et al. Interestingly, SLC7A11 was positively correlated with the expression of several immunostimulators, such as TNFSF9 (r = 0.209, p = 6.41e-06), ULBP1 (r = 0.303, p = 4.09e-11), and NT5E (r = 0.337, p = 1.59e-13) (Figures 6C,D). Therefore, these results suggest that SLC7A11 may play a role in regulating tumor immunity.
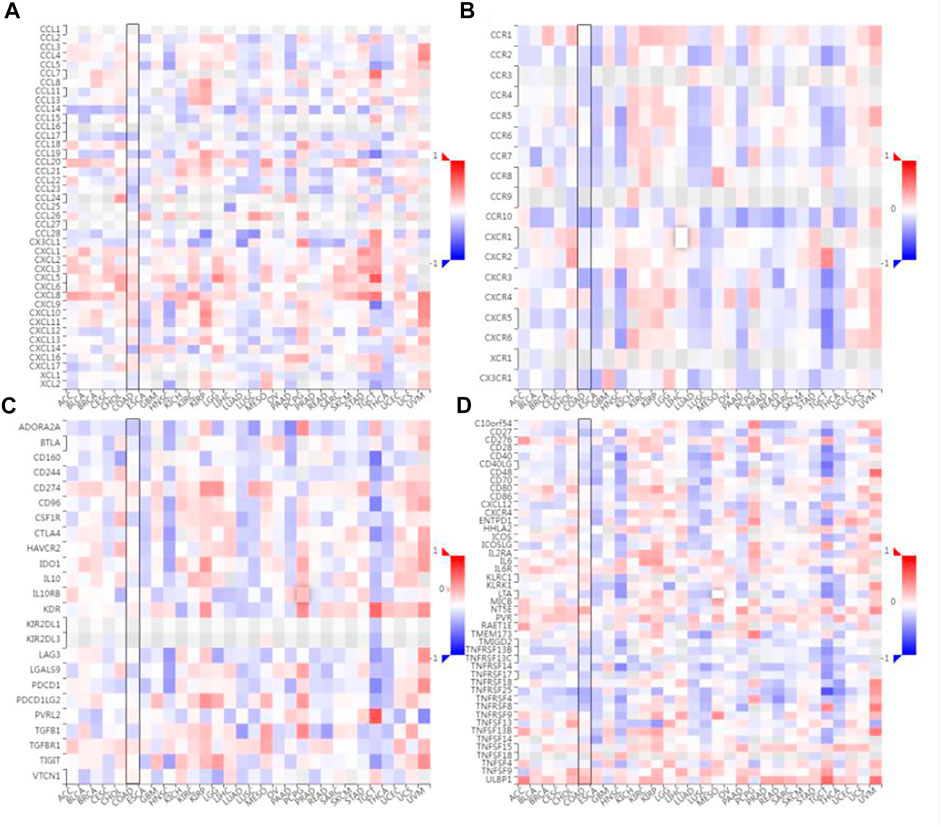
FIGURE 6. Correlation analysis between SLC7A11 expression and immunomodulators or chemokine receptors. (A) Heatmap analysis of the correlation between SLC7A11 and chemokines in tumors. (B) Heatmap analysis of the correlation between SLC7A11 and chemokine receptors in tumors. (C) Correlation between SLC7A11 and immunoinhibitors in tumors via heatmap analysis. (D) Correlation between SLC7A11 and immunostimulators in tumors by heatmap analysis.
Overexpressed SLC7A11-Inhibited Chemokine and Receptors Expression
For validation, we examined the expression of CCL17, CXCL14, and CXCR3 in cancer and adjacent tissues of six patients with SLC7A11 overexpression by ELISA. The results showed that the levels of CCL17, CXCL14, and CXCR3 in cancer were 1.558 ± 0.104 ng/ml, 2.169 ± 0.214 ng/ml, and 2.843 ± 0.423 ng/ml, respectively, significantly lower than the corresponding adjacent tissues 1.795 ± 0.207 ng/ml, 2.625 ± 0.093 ng/ml, and 6.868 ± 0.455 ng/ml (p < 0.05 or 0.01, Figure 7).
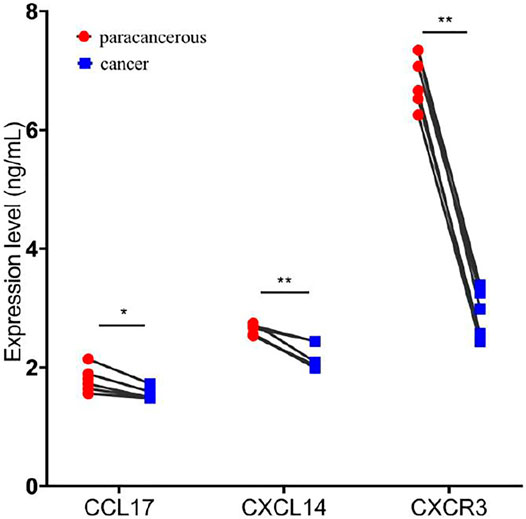
FIGURE 7. Overexpression of SLC7A11 inhibits the expression of chemokines and chemokine receptors. n = 6, *p < 0.05, and **p < 0.01.
Discussion
Despite adjuvant chemotherapy, immunotherapy, and other anticancer therapies in early cancer treatment have shown encouraging efficacy, the middle and later stages of treatment in most cases are challenging. Therefore, it is necessary and urgent to find a more effective strategy to treat cancer. The ideal therapeutic target to be inhibited by an anticancer drug should have a selective effect on tumor growth, allowing the corresponding drug to produce the desired toxic effect in cancer cells without side effects on normal cells. Ferroptosis is a newly discovered mechanism of iron-dependent cell death, characterized by increased reactive oxygen species (ROS) and lipid peroxidation due to metabolic dysfunction, which is considered as a powerful weapon in the elimination of cancer cells (Tang et al., 2020). SLC7A11 is a core target-regulating ferroptosis, and its overexpression leads to downregulation of the sensitivity of cancer cells to ferroptosis. Recent research suggests that cancer therapies, such as immunotherapy and radiotherapy, can induce ferroptosis partly through modulating SLC7A11 expression (Lang et al., 2019; Lei et al., 2020); knockout or inhibition of SLC7A11 has a significant inhibitory effect on lung cancer and pancreatic cancer cells (Ji et al., 2018; Sharbeen et al., 2021). SLC7A11 has become a central hub linking ferroptosis to its suppressive function on tumor.
The study showed that SLC7A11 is essential to elicit tumor formation and maintain tumorigenicity by relieving oxidative stress in COAD, pancreatic ductal adenocarcinoma (PDAC), and lung adenocarcinoma (LUAD) (Lim et al., 2019). This study found that SLC7A11 is highly expressed in most cancers, such as COAD, LUAD, and esophageal cancer (ESCA). This notion is further supported by the GEO database. The results indicated that many cases may shrink ferroptosis by upregulating SLC7A11 expression. Interestingly, the expression of SLC7A11 was relatively stable in all pathological stages of COAD, but decreased after lymphatic invasion. These results suggest that the overexpression of SLC7A11 promotes lymphatic metastasis of tumor cells, which was also confirmed in pancreatic carcinoma (Zhu et al., 2020).
Our study demonstrated that the expression level of SLC7A11 has a significant correlation with the infiltration levels of CD8+ T cells, neutrophils, and dendritic cells in COAD. Studies show that immunotherapy-activated CD8+ T cells enhance ferroptosis-specific lipid peroxidation in tumor cells, and that increased ferroptosis contributes to the antitumor efficacy of immunotherapy (Wang et al., 2019). Further analysis of infiltrated lymphocyte markers showed that the markers of Th1 cell such as TBX21, IL12RB2, IL27RA, STAT1, and IFN-γ were strongly correlated with SLC7A11 expression, as well as the CD8+ T cell marker CD8A and the NK cell marker KIR3DL1. Intriguingly, IFN-γ upregulates the level of intracellular Fe2+ and decreases the level of GPX4, which lead the cells to be more sensitive to ferroptosis; STAT1 inhibitors could reverse the reduction of SLC7A11 expression induced by IFN-γ and induces ferroptosis via activation of the JAK1-2/STAT1/SLC7A11 signaling pathway (Wei et al., 2021). Meanwhile, SLC7A11 also affects the infiltration of immune cells in rectal cancer, such as CD8+ T cells (Supplementary Figure S1). These results further implied that SLC7A11 was the key molecule for bridging the ferroptosis process and immunotherapy. Moreover, we noticed that most of the immune cells or their markers were weak in the prediction of correlations via the TIMER and GEPIA databases. A similar situation has occurred in some recent studies (Zhao et al., 2021). This weak correlation does not mean that the detected target molecule can be ignored. If the correlations of target molecules, which are considered to be highly reliable, are calculated with consistent results in different databases (positive or negative), and p values indicate significant differences.
SLC7A11 is a key gene regulating ferroptosis and is overexpressed in COAD. Further study found that the genes closely associated with SLC7A11, such as PRDX5, CLDN3, ROMO1, and VEGFB genes were also overexpressed; LARP1B,AP1S3, IBTK, and SPATA5 genes were under-expressed. PRX5 can promote epithelial-to-mesenchymal transition (EMT) properties by inducing the expression of EMT-inducing transcription factors in colorectal cancer (Ahn et al., 2017). Li, et al. study found that CLDN3 is overexpressed in colorectal cancer tissues, and its high expression may promote the occurrence and progression of colorectal cancer (Li et al., 2017). VEGFB overexpression is thought to be highly associated with poor prognosis (Lautenschlaeger et al., 2013). In contrast, among the genes that are under-expressed, IBTK inhibits the survival and proliferation of tumor cells by influencing the tumor microenvironment (Pal Singh et al., 2018). These results suggest that SLC7A11 and its co-expressed gene may be an important gene promoting the development of COAD.
TFs-miRNAs can jointly regulate target gene expression in the forms of feed-forward loops or feedback loops; these regulatory loops play an important role in gene regulatory networks and disease processes (Zhang et al., 2015). In this study, miRNA (such as hsa-mir-335-5p, hsa-mir-155-5p, and hsa-mir-34a-5p) and TFs (such as RELA, SP1, and NFKB1) with high frequency of action were identified. Studies have shown that mir-335-5P mediates the proliferation, apoptosis, and invasion of osteosarcoma and breast cancer cells (Chen et al., 2018; Zhang et al., 2021). RELA of NF-κB-driven cytokine by myeloid cells is required for colitis-associated cancer growth (Greten et al., 2004). Therefore, it is assumed that targeting TFs and miRNAs to regulate the expression of SLC7A11 may have a potential role in the treatment of COAD.
The construction of the PPI network is of great significance in analyzing protein signal transduction, gene expression regulation, and functional relationship among proteins. According to the PPIs network, SLC7A11, GPX4, GCLC, GCLM, and SLC3A2 were declared as hub genes involved in ferroptosis. Further examination of the hub genes of the PPI network revealed that these genes were also highly expressed in COAD (Supplementary Figure S2). As can be seen from the ferroptosis signaling pathway Supplementary Figure S3), SLC7A11 is the upstream gene, and its expression level is positively correlated with hub genes. These results further suggest that SLC7A11 was a central regulator of ferroptosis in COAD. Furthermore, securinine PC3 UP, alpha-tocopherol CTD 00007387, and N-acetyl-l-cysteine CTD 00005305 are the peak drug candidates for regulating hub genes. Growing evidence suggest that securinine and alpha-tocopherol have anticancer and antimetastasis properties (Middha et al., 2019; Ashraf et al., 2021).
Chemokines and chemokine receptors are essential for the infiltration of immune cells into tumors. In this study, it is proved that the expression level of SLC7A11 was negatively correlated with the expressions of CCL17, CCL19, CCL22, CCL23, CXCL14, CCR10, CX3CR1, and CXCR3, suggesting that the high expression of SLC7A11 may inhibit the migration of immune cells. In contrast, the SLC7A11 expression level was positively correlated with the expressions of CXCL1-6/8-11. Studies have shown that CXCL9/10/11 are the main chemokines of CD8+ T cells (Shigeta et al., 2020; Marcovecchio et al., 2021); CXCL2/8 are the main chemokines of neutrophil (Xu et al., 2021). This further confirmed the results of immune cell infiltration. However, a high proportion of neutrophils can inhibit the function of immune cells such as CD8+ T cells, and promote tumor growth (Zhou et al., 2018). This may explain the poor immunotherapy response in patients with SLC7A11 high expression. CCL17 and CCL22 are the ligands for CCR4, which effect on the recruitment of Treg, Th2, and Th17 into the tumor (Korbecki et al., 2020). In addition, they exert an anticancer effect by causing the infiltration of tumor-infiltrating lymphocytes (TIL) into the tumor (Kanagawa et al., 2007). Chemokine CXCL14 is a key regulatory factor in cancer and represents a potential target for future cancer immunotherapies (Westrich et al., 2020). The CXCR3 and CX3CR1 are mainly responsible for the tumor-suppressive lymphocytic infiltration into the tumor micromilieu (Bronger et al., 2019). Interestingly, the high expression of SLC7A11 also affects the expression of immunoinhibitors and immunostimulators. Therefore, these results suggest that SLC7A11 may play a role in regulating tumor immunity.
Conclusion
In summary, our study found that the expression of SLC7A11 and genes interact with it are significantly upregulated in COAD, and results in more GSH synthesis. GPX4 uses GSH to improve the antioxidant activity of tumor cells, thereby suppressing ferroptosis. Meanwhile, the overexpression of SLC7A11 can affect the expression of chemokines, leading to the infiltration of immune cells such as CD8+ T cells/neutrophils, and deficiency of other immune cells, and causes immunosuppression of tumor cells. Regulation of SLC7A11 expression would be a potential therapeutic approach in inducing ferroptosis and/or immunotherapy for tumors.
Data Availability Statement
The original contributions presented in the study are included in the article/Supplementary Material; further inquiries can be directed to the corresponding author.
Ethics Statement
The studies involving human participants were reviewed and approved by the ethics committee of the First Affiliated Hospital of Wannan Medical College, Wuhu, Anhui, China. The patients provided their written informed consent to participate in this study.
Author Contributions
XC and JX participated in study design and drafted the manuscript. YW and LL performed the statistical analysis. CL (4th author) and CL (5th author) collected the samples and clinical data. JX contributed for overall editing and supervision. All authors approved the final manuscript.
Funding
This work was supported by the Key Project in Natural Science Research in Higher Education Institutions of Anhui Province (KJ 2019A0403) and the Natural Science Foundation of Wannan Medical College (WK202123).
Conflict of Interest
The authors declare that the research was conducted in the absence of any commercial or financial relationships that could be construed as a potential conflict of interest.
Publisher’s Note
All claims expressed in this article are solely those of the authors and do not necessarily represent those of their affiliated organizations, or those of the publisher, the editors, and the reviewers. Any product that may be evaluated in this article, or claim that may be made by its manufacturer, is not guaranteed or endorsed by the publisher.
Supplementary Material
The Supplementary Material for this article can be found online at: https://www.frontiersin.org/articles/10.3389/fmolb.2022.889688/full#supplementary-material
References
Ahn, H.-M., Yoo, J.-W., Lee, S., Lee, H. J., Lee, H.-S., and Lee, D.-S. (2017). Peroxiredoxin 5 Promotes the Epithelial-Mesenchymal Transition in colon Cancer. Biochem. Biophysical Res. Commun. 487 (3), 580–586. doi:10.1016/j.bbrc.2017.04.094
Alhopuro, P., Sammalkorpi, H., Niittymäki, I., Biström, M., Raitila, A., Saharinen, J., et al. (2012). Candidate Driver Genes in Microsatellite-Unstable Colorectal Cancer. Int. J. Cancer 130 (7), 1558–1566. doi:10.1002/ijc.26167
Ashraf, S. M., Mahanty, S., and Rathinasamy, K. (2021). Securinine Induces Mitotic Block in Cancer Cells by Binding to Tubulin and Inhibiting Microtubule Assembly: A Possible Mechanistic Basis for its Anticancer Activity. Life Sci. 287, 120105. doi:10.1016/j.lfs.2021.120105
Bao, X., Zhang, H., Wu, W., Cheng, S., Dai, X., Zhu, X., et al. (2020). Analysis of the Molecular Nature Associated with Microsatellite Status in colon Cancer Identifies Clinical Implications for Immunotherapy. J. Immunother. Cancer 8 (2), e001437. doi:10.1136/jitc-2020-001437
Bronger, H., Magdolen, V., Goettig, P., and Dreyer, T. (2019). Proteolytic Chemokine Cleavage as a Regulator of Lymphocytic Infiltration in Solid Tumors. Cancer Metastasis Rev. 38 (3), 417–430. doi:10.1007/s10555-019-09807-3
Chen, Y., Chen, Q., Zou, J., Zhang, Y., and Bi, Z. (2018). Construction and Analysis of a ceRNA-ceRNA Network Reveals Two Potential Prognostic Modules Regulated by hsa-miR-335-5p in Osteosarcoma. Int. J. Mol. Med. 42 (3), 1237–1246. doi:10.3892/ijmm.2018.3709
El Kinany, K., Mint Sidi Deoula, M., Hatime, Z., Boudouaya, H. A., Huybrechts, I., El Asri, A., et al. (2020). Consumption of Modern and Traditional Moroccan Dairy Products and Colorectal Cancer Risk: a Large Case Control Study. Eur. J. Nutr. 59 (3), 953–963. doi:10.1007/s00394-019-01954-1
Fidelle, M., Yonekura, S., Picard, M., Cogdill, A., Hollebecque, A., Roberti, M. P., et al. (2020). Resolving the Paradox of Colon Cancer through the Integration of Genetics, Immunology, and the Microbiota. Front. Immunol. 11, 600886. doi:10.3389/fimmu.2020.600886
Greten, F. R., Eckmann, L., Greten, T. F., Park, J. M., Li, Z.-W., Egan, L. J., et al. (2004). IKKβ Links Inflammation and Tumorigenesis in a Mouse Model of Colitis-Associated Cancer. Cell 118 (3), 285–296. doi:10.1016/j.cell.2004.07.013
Guo, J., Xu, B., Han, Q., Zhou, H., Xia, Y., Gong, C., et al. (2018). Ferroptosis: A Novel Anti-tumor Action for Cisplatin. Cancer Res. Treat. 50 (2), 445–460. doi:10.4143/crt.2016.572
Ji, X., Qian, J., Rahman, S. M. J., Siska, P. J., Zou, Y., Harris, B. K., et al. (2018). xCT (SLC7A11)-Mediated Metabolic Reprogramming Promotes Non-small Cell Lung Cancer Progression. Oncogene 37 (36), 5007–5019. doi:10.1038/s41388-018-0307-z
Kanagawa, N., Niwa, M., Hatanaka, Y., Tani, Y., Nakagawa, S., Fujita, T., et al. (2007). CC-chemokine Ligand 17 Gene Therapy Induces Tumor Regression through Augmentation of Tumor-Infiltrating Immune Cells in a Murine Model of Preexisting CT26 colon Carcinoma. Int. J. Cancer 121 (9), 2013–2022. doi:10.1002/ijc.22908
Koppula, P., Zhang, Y., Zhuang, L., and Gan, B. (2018). Amino Acid Transporter SLC7A11/xCT at the Crossroads of Regulating Redox Homeostasis and Nutrient Dependency of Cancer. Cancer Commun. 38 (1), 12. doi:10.1186/s40880-018-0288-x
Korbecki, J., Kojder, K., Simińska, D., Bohatyrewicz, R., Gutowska, I., Chlubek, D., et al. (2020). CC Chemokines in a Tumor: A Review of Pro-cancer and Anti-cancer Properties of the Ligands of Receptors CCR1, CCR2, CCR3, and CCR4. Ijms 21 (21), 8412. doi:10.3390/ijms21218412
Lang, X., Green, M. D., Wang, W., Yu, J., Choi, J. E., Jiang, L., et al. (2019). Radiotherapy and Immunotherapy Promote Tumoral Lipid Oxidation and Ferroptosis via Synergistic Repression of SLC7A11. Cancer Discov. 9 (12), 1673–1685. doi:10.1158/2159-8290.CD-19-0338
Lautenschlaeger, T., George, A., Klimowicz, A. C., Efstathiou, J. A., Wu, C.-L., Sandler, H., et al. (2013). Bladder Preservation Therapy for Muscle-Invading Bladder Cancers on Radiation Therapy Oncology Group Trials 8802, 8903, 9506, and 9706: Vascular Endothelial Growth Factor B Overexpression Predicts for Increased Distant Metastasis and Shorter Survival. Oncologist 18 (6), 685–686. doi:10.1634/theoncologist.2012-0461
Lei, G., Zhang, Y., Koppula, P., Liu, X., Zhang, J., Lin, S. H., et al. (2020). The Role of Ferroptosis in Ionizing Radiation-Induced Cell Death and Tumor Suppression. Cell Res 30 (2), 146–162. doi:10.1038/s41422-019-0263-3
Li, J. Y., Xie, F., Xu, X. P., Ma, J. J., Zhou, D. C., Liao, Y., et al. (2017). Claudin-3 Expression in Colorectal Carcinoma and its Significance. Nan Fang Yi Ke Da Xue Xue Bao 37 (1), 63–67. doi:10.3969/j.issn.1673-4254.2017.01.11
Lim, J. K. M., Delaidelli, A., Minaker, S. W., Zhang, H.-F., Colovic, M., Yang, H., et al. (2019). Cystine/glutamate Antiporter xCT (SLC7A11) Facilitates Oncogenic RAS Transformation by Preserving Intracellular Redox Balance. Proc. Natl. Acad. Sci. U.S.A. 116 (19), 9433–9442. doi:10.1073/pnas.1821323116
Marcovecchio, P. M., Thomas, G., and Salek-Ardakani, S. (2021). CXCL9-expressing Tumor-Associated Macrophages: New Players in the Fight against Cancer. J. Immunother. Cancer 9 (2), e002045. doi:10.1136/jitc-2020-002045
Middha, P., Weinstein, S. J., Männistö, S., Albanes, D., and Mondul, A. M. (2019). β-Carotene Supplementation and Lung Cancer Incidence in the Alpha-Tocopherol, Beta-Carotene Cancer Prevention Study: The Role of Tar and Nicotine. Nicotine Tob. Res. 21 (8), 1045–1050. doi:10.1093/ntr/nty115
Mou, Y., Wang, J., Wu, J., He, D., Zhang, C., Duan, C., et al. (2019). Ferroptosis, a New Form of Cell Death: Opportunities and Challenges in Cancer. J. Hematol. Oncol. 12 (1), 34. doi:10.1186/s13045-019-0720-y
Pal Singh, S., Dammeijer, F., and Hendriks, R. W. (2018). Role of Bruton's Tyrosine Kinase in B Cells and Malignancies. Mol. Cancer 17 (1), 57. doi:10.1186/s12943-018-0779-z
Roslan, N. H., Makpol, S., and Mohd Yusof, Y. A. (2019). A Review on Dietary Intervention in Obesity Associated Colon Cancer. Asian Pac. J. Cancer Prev. 20 (5), 1309–1319. doi:10.31557/APJCP.2019.20.5.1309
Sandhu, J., Lavingia, V., and Fakih, M. (2019). Systemic Treatment for Metastatic Colorectal Cancer in the Era of Precision Medicine. J. Surg. Oncol. 119 (5), 564–582. doi:10.1002/jso.25421
Sharbeen, G., McCarroll, J. A., Akerman, A., Kopecky, C., Youkhana, J., Kokkinos, J., et al. (2021). Cancer-Associated Fibroblasts in Pancreatic Ductal Adenocarcinoma Determine Response to SLC7A11 Inhibition. Cancer Res. 81 (13), 3461–3479. doi:10.1158/0008-5472.CAN-20-2496
Shigeta, K., Matsui, A., Kikuchi, H., Klein, S., Mamessier, E., Chen, I. X., et al. (2020). Regorafenib Combined with PD1 Blockade Increases CD8 T-Cell Infiltration by Inducing CXCL10 Expression in Hepatocellular Carcinoma. J. Immunother. Cancer 8 (2), e001435. doi:10.1136/jitc-2020-001435
Song, X., Zhu, S., Chen, P., Hou, W., Wen, Q., Liu, J., et al. (2018). AMPK-mediated BECN1 Phosphorylation Promotes Ferroptosis by Directly Blocking System Xc- Activity. Curr. Biol. 28 (15), 2388–2399. doi:10.1016/j.cub.2018.05.094
Tang, R., Xu, J., Zhang, B., Liu, J., Liang, C., Hua, J., et al. (2020). Ferroptosis, Necroptosis, and Pyroptosis in Anticancer Immunity. J. Hematol. Oncol. 13 (1), 110. doi:10.1186/s13045-020-00946-7
Tsukamoto, S., Ishikawa, T., Iida, S., Ishiguro, M., Mogushi, K., Mizushima, H., et al. (2011). Clinical Significance of Osteoprotegerin Expression in Human Colorectal Cancer. Clin. Cancer Res. 17 (8), 2444–2450. doi:10.1158/1078-0432.CCR-10-2884
Wang, W., Green, M., Choi, J. E., Gijón, M., Kennedy, P. D., Johnson, J. K., et al. (2019). CD8+ T Cells Regulate Tumour Ferroptosis during Cancer Immunotherapy. Nature 569 (7755), 270–274. doi:10.1038/s41586-019-1170-y
Wei, T. T., Zhang, M. Y., Zheng, X. H., Xie, T. H., Wang, W., Zou, J., et al. (2021). Interferon‐γ Induces Retinal Pigment Epithelial Cell Ferroptosis by a JAK1‐2/STAT1/SLC7A11 Signaling Pathway in Age‐related Macular Degeneration. FEBS J. 289 (7), 1968–1983. doi:10.1111/febs.16272
Westrich, J. A., Vermeer, D. W., Colbert, P. L., Spanos, W. C., and Pyeon, D. (2020). The Multifarious Roles of the Chemokine CXCL14 in Cancer Progression and Immune Responses. Mol. Carcinogenesis 59 (7), 794–806. doi:10.1002/mc.23188
Xie, Y., Hou, W., Song, X., Yu, Y., Huang, J., Sun, X., et al. (2016). Ferroptosis: Process and Function. Cell Death Differ 23 (3), 369–379. doi:10.1038/cdd.2015.158
Xu, X., Ye, L., Zhang, Q., Shen, H., Li, S., Zhang, X., et al. (2021). Group‐2 Innate Lymphoid Cells Promote HCC Progression through CXCL2 ‐Neutrophil‐Induced Immunosuppression. Hepatology 74 (5), 2526–2543. doi:10.1002/hep.31855
Zhang, D., An, X., Yu, H., and Li, Z. (2021). The Regulatory Effect of 6-TG on lncRNA-miRNA-mRNA ceRNA Network in Triple-Negative Breast Cancer Cell Line. Biosci. Rep. 41 (2), BSR20203890. doi:10.1042/BSR20203890
Zhang, H.-M., Kuang, S., Xiong, X., Gao, T., Liu, C., and Guo, A.-Y. (2015). Transcription Factor and microRNA Co-regulatory Loops: Important Regulatory Motifs in Biological Processes and Diseases. Brief. Bioinform. 16 (1), 45–58. doi:10.1093/bib/bbt085
Zhang, L., Liu, W., Liu, F., Wang, Q., Song, M., Yu, Q., et al. (2020). IMCA Induces Ferroptosis Mediated by SLC7A11 through the AMPK/mTOR Pathway in Colorectal Cancer. Oxidative Med. Cell Longevity 2020, 1–14. doi:10.1155/2020/1675613
Zhao, H., Xu, Y., Xie, Y., Zhang, L., Gao, M., Li, S., et al. (2021). m6A Regulators Is Differently Expressed and Correlated with Immune Response of Esophageal Cancer. Front. Cel Dev. Biol. 9, 650023. doi:10.3389/fcell.2021.650023
Zhou, J., Nefedova, Y., Lei, A., and Gabrilovich, D. (2018). Neutrophils and PMN-MDSC: Their Biological Role and Interaction with Stromal Cells. Semin. Immunol. 35, 19–28. doi:10.1016/j.smim.2017.12.004
Zhu, J.-H., De Mello, R. A., Yan, Q.-L., Wang, J.-W., Chen, Y., Ye, Q.-H., et al. (2020). MiR-139-5p/SLC7A11 Inhibits the Proliferation, Invasion and Metastasis of Pancreatic Carcinoma via PI3K/Akt Signaling Pathway. Biochim. Biophys. Acta (Bba) - Mol. Basis Dis. 1866 (6), 165747. doi:10.1016/j.bbadis.2020.165747
Keywords: SLC7A11, ferroptosis, immune infiltrate, immune microenvironment, COAD
Citation: Cheng X, Wang Y, Liu L, Lv C, Liu C and Xu J (2022) SLC7A11, a Potential Therapeutic Target Through Induced Ferroptosis in Colon Adenocarcinoma. Front. Mol. Biosci. 9:889688. doi: 10.3389/fmolb.2022.889688
Received: 04 March 2022; Accepted: 23 March 2022;
Published: 20 April 2022.
Edited by:
Xin Wang, National Institutes of Health (NIH), United StatesReviewed by:
Wenjuan Wang, National Institutes of Health (NIH), United StatesZhenyi Su, Columbia University, United States
Copyright © 2022 Cheng, Wang, Liu, Lv, Liu and Xu. This is an open-access article distributed under the terms of the Creative Commons Attribution License (CC BY). The use, distribution or reproduction in other forums is permitted, provided the original author(s) and the copyright owner(s) are credited and that the original publication in this journal is cited, in accordance with accepted academic practice. No use, distribution or reproduction is permitted which does not comply with these terms.
*Correspondence: Jingyun Xu, MjAyMDAwMTFAd25tYy5lZHUuY24=