- 1Cancer Center, Department of Pathology, Zhejiang Provincial People’s Hospital (Affiliated People’s Hospital, Hangzhou Medical College), Hangzhou, China
- 2College of Life Sciences and Medicine, Xinyuan Institute of Medicine and Biotechnology, Zhejiang Sci-Tech University, Hangzhou, China
- 3Department of Laboratory Medicine, Tiantai People’s Hospital, Taizhou, China
Cancer is caused by the destruction or mutation of cellular genetic materials induced by environmental or genetic factors. It is defined by uncontrolled cell proliferation and abnormality of the apoptotic pathways. The majority of human malignancies are characterized by distant metastasis and dissemination. Currently, the most common means of cancer treatment include surgery, radiotherapy, and chemotherapy, which usually damage healthy cells and cause toxicity in patients. Targeted therapy is an effective tumor treatment method with few side effects. At present, some targeted therapeutic drugs have achieved encouraging results in clinical studies, but finding an effective solution to improve the targeting and delivery efficiency of these drugs remains a challenge. In recent years, oncolytic viruses (OVs) have been used to direct the tumor-targeted therapy or immunotherapy. Newcastle disease virus (NDV) is a solid oncolytic agent capable of directly killing tumor cells and increasing tumor antigen exposure. Simultaneously, NDV can trigger the proliferation of tumor-specific immune cells and thus improve the therapeutic efficacy of NDV in cancer. Based on NDV’s inherent oncolytic activity and the stimulation of antitumor immune responses, the combination of NDV and other tumor therapy approaches can improve the antitumor efficacy while reducing drug toxicity, indicating a broad application potential. We discussed the biological properties of NDV, the antitumor molecular mechanisms of oncolytic NDV, and its application in the field of tumor therapy in this review. Furthermore, we presented new insights into the challenges that NDV will confront and suggestions for increasing NDV’s therapeutic efficacy in cancer.
Introduction
Cancer seriously threatens human health due to its high incidence and mortality and is the second cause of death globally, exceeded only by cardiovascular diseases (Moliner et al., 2019; Xia et al., 2022). In 2020, there were an estimated 19.3 million new cancer cases worldwide and nearly 10 million cancer-related deaths (Sung et al., 2021). Cancer’s high mortality rate is mainly because patients with early cancer have no apparent symptoms and are already in the late stage or metastatic stage when diagnosed (Huang et al., 2017; Regel et al., 2020). Tumor cells evade immune system surveillance and inhibit the immune response due to high mutagenicity (Park et al., 2020). At the same time, uncontrolled cancer cells invade the tissue, eventually leading to organ failure and even death (Fares et al., 2020). Currently, surgery, radiotherapy, and chemotherapy are the main methods for cancer treatment (Moo et al., 2018; Yahya and Alqadhi, 2021). Although surgery, radio-/chemotherapy, and targeted therapy can help some patients with early tumors, most therapy methods for individuals are terminated because of severe side effects (Boshuizen and Peeper, 2020). Cancer prognosis is still not optimistic; therefore, an essential question in cancer therapy as to how to improve cancer patients’ survival rate effectively remains unexplored.
In recent years, several approaches have been developed for cancer therapy, such as immune checkpoint–based therapy (Chen et al., 2021), targeting circular RNAs (Chen et al., 2019), chimeric antigen receptor T (CAR-T) cell therapy (Adachi et al., 2018), and CRISPR/Cas9-based therapy (Zhen and Li, 2019). But all of these approaches have some limitations, which include off-target effects for targeted therapy, inefficiency of monotherapy, and unpredictable or predictable side effects (Zugazagoitia et al., 2016). An oncolytic virus (OV) is a promising cancer treatment strategy. OV is a useful therapeutic reagent that identifies and destroys malignant cells after a recurring viral infection (Martin and Bell, 2018; Raja et al., 2018; Leber et al., 2020). The lytic products after tumor dissolution can reverse the tumor microenvironment, promote the recruitment of immune cells, and further activate the antitumor immune response (Mahasa et al., 2017; Harrington et al., 2019). Several viruses, including the Newcastle disease virus (NDV), vaccinia virus, adenovirus, reovirus, herpes simplex virus, and measles virus, are being widely studied to treat various types of advanced cancer (Mondal et al., 2020; Goradel et al., 2021). Talimogene laherparepvec (T-VEC), a genetically engineered herpes simplex virus, is the first OV approved to treat advanced melanoma by the US FDA (Liu et al., 2003; Andtbacka et al., 2019). However, due to the heterogeneity of cancer tissue and the complexity of cancer cells, a single type of OV is not enough to destroy all cancer cells (Lawler et al., 2017; Martin and Bell, 2018). Some cancer cells and non-transformed supporting cells may be resistant to certain OVs (Alvarez-Breckenridge et al., 2013; Pol et al., 2016). Based on these, a single type of viral therapy may not be effective against all types of cancer (Kwan et al., 2021). Therefore, we believe that the combination of OV therapy and other cancer therapies will be significant for cancer patients (Dai et al., 2022).
NDV is a natural avian–derived virus (Sinkovics and Horvath, 2000), and its infection is a highly contagious disease that causes enormous economic losses to the poultry industry worldwide (Ganar et al., 2014; Susta et al., 2018). NDV has been developed as an oncolytic agent or a vaccination vector over the last 20 years due to its intrinsic oncolytic ability (Molouki and Peeters, 2017; Hu et al., 2020; Vannini et al., 2021). Compared with other OVs, oncolytic NDV has inherent antitumor advantages (Meng et al., 2021). Natural NDV strains exhibit an antitumor effect in human cancer cells and cause oncolysis without harming the normal cells (Yurchenko et al., 2019; Burman et al., 2020). In addition to causing direct damage to host cells through viral infection and replication, NDV activates multiple signaling pathways, triggering autophagy, inflammation, and apoptosis (Cuadrado-Castano et al., 2015; Li et al., 2019; Gong et al., 2021). It also activates antitumor immune responses, thus assisting viral replication (Wang et al., 2020; Zhan et al., 2020; Kan et al., 2021). With the constant maturity of the reverse genetic operating system of NDV (Peeters et al., 1999; Römer-Oberdörfer et al., 1999; Nettelbeck et al., 2021), an increasing number of transgenic NDVs are identified, which makes the application of NDV a new stage in cancer therapy. A genetically engineered NDV strain (NDV-F3aa) is effective in the experimental treatment of a gastric tumor peritoneal model without significant toxicity, and in some cases, it may completely cure gastric tumors (Song et al., 2010). Combining NDV therapy with other cancer therapies also provides new ideas for cancer treatment (Schirrmacher and Fournier, 2014; Xu et al., 2021). Hence, the NDV represents broad prospects for cancer treatment.
This review will concentrate on the biology, process of infection, and replication of NDV in cancer cells and the primary molecular mechanism of NDV oncolysis, and its preclinical and clinical applications in diverse cancers. In addition, we will highlight the limitations of NDV in clinical research and share our new insights into the use of NDV in cancer therapy.
Newcastle Disease Virus Biology
Twelve different serotypes of avian paramyxoviruses (APMVs) have been reported up to date (Gogoi et al., 2017). NDV is the most characterized member of the genus Avulavirus in the family of Paramyxoviridae (APMV-1) (Kapczynski et al., 2013). It is an RNA virus with diameters ranging from 100 to 500 nm, enclosed by a viral lipid membrane (Kapczynski et al., 2013; Nagai et al., 1989). NDV was first identified as a valuable virus for virulence studies in the 1970s (Gogoi et al., 2017; Cassel and Garrett, 1965). According to their pathogenicity and virulence in infected chickens, NDV strains are classified as lentogenic (avirulent), mesogenic, and velogenic (fully virulent) (Sinkovics and Horvath, 2000; Dimitrov et al., 2016). NDV contains a negative single-stranded RNA (ssRNA) genome of approximately 15.2 kb that consists of a leader (55 nucleotides) and trailer (114 nucleotides) terminal sequences (Nagai et al., 1989; Bello et al., 2020), which encode six different structural proteins: hemagglutinin–neuraminidase (HN), nucleocapsid (N) protein, fusion (F) protein, phosphoprotein (P) protein, matrix (M) protein, and RNA-dependent large polymerase (L) protein (Figure 1). V and W proteins are auxiliary and exist only in virus-infected cells. The V protein is an IFN antagonist and plays a vital role in the virulence of NDV (Alamares et al., 2010). Notably, in the NDV genome, each gene encodes a single protein and is characterized by a coding sequence flanked by highly conserved gene start (GS) and gene end (GE) transcriptional signals (Munir et al., 2012).
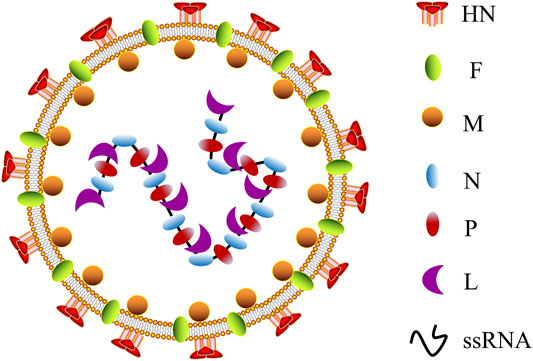
FIGURE 1. Schematic representation of NDV morphology. NDV, Newcastle disease virus; HN, hemagglutinin–neuraminidase; F, fusion protein; M, matrix protein; N, nucleocapsid protein; P, phosphoprotein; L, RNA-dependent large polymerase protein; ssRNA, single-stranded RNA.
The viral N protein, P protein, and L protein bind to the viral RNA genome to form a ribonucleoprotein complex (RNP), essential for virus replication (Yusoff and Tan, 2001). The M protein is located in the layer below the virus lipid membrane and participates in virus assembly and budding (Nagai et al., 1989). HN and F proteins are located on the virus membrane’s outer surface, where they join with the host cell’s lipid bilayer membrane to form a viral shell. In addition, HN and F proteins jointly mediate viral attachment and fusion on the cell surface (Fournier et al., 2004). The fusion of virus and host cell must be completed through the F protein and HN protein participation, and the cleavage site of virus F protein (Fcs) is the critical factor (Peeters et al., 1999; Seal et al., 2000). Simultaneously, antibodies F and HN are the significant components that resulted in vaccine-inducing body protection, following vaccination of avian or non-avian species (Xiao et al., 2012; Dey et al., 2014), revealing the potential of NDV as a vaccine vector resistant to the animal and human disease. Currently, the LaSota and Hicher B1 vaccine strains have been widely used as a live NDV vaccine throughout the world (Carrasco et al., 2016; Dey et al., 2017). The strains are naturally occurring lentogenic strains that are highly expressed in embryonated chicken eggs and elicit a significant immune response (Ginting et al., 2017). One of the advantages of NDV as an oncolytic agent is that both lytic and non-lytic strains of NDV can fast-replicate in all species of avian and multiple human cancer cells (Lam et al., 2011; Zamarin and Palese, 2012), resulting in effective cell lysis and offering substantial protection from disease.
Newcastle Disease Virus Dissolves Tumor and Activates an Antitumor Immune Response
The NDV oncolytic properties originate from its capacity to proliferate in cancer cells (Shobana et al., 2013). Further research showed that it might be related to the deficiency of the interferon (IFN) system in tumors (Stojdl et al., 2000). H. Song et al. discovered that NDV enters the cell through a pH-independent direct fusion of its envelope to the host membrane via receptor-mediated endocytosis (Sánchez-Felipe et al., 2014). The process of NDV infection and replication in tumor cells is described as follows (Moliner et al., 2019). NDV binds to the sialic acid receptor on the surface of tumor cells through the HN protein, and then, protein F initiates the fusion of the viral and host cell membranes (Song et al., 2019; Xia et al., 2022). Viral RNA polymerase transcribes the viral negative single-stranded RNA into positive single-stranded RNA as a template for mRNA and protein synthesis (Burman et al., 2020; Sung et al., 2021). The rough endoplasmic reticulum processes surface proteins F and HN, assembled on the host cell membrane and mature to produce new virions that start a new round of tumor cell infection (Cuadrado-Castano et al., 2015). Importantly, virus-mediated direct oncolysis causes the release of tumor-associated antigens (TAAs), pathogen-associated molecular patterns (PAMPs), and danger-associated molecular patterns (DAMPs). These can activate antigen-presenting cells (APCs), including antigen-cross-presenting dendritic cells (DCs). Activated APCs then activate the immune cells, resulting in the generation of CD4+ T cells, CD8+ T cells, and NK cells directed toward tumor and viral antigens (Burman et al., 2020; Schirrmacher and Fournier, 2014) (Figure 2). It is worth mentioning that NDV does not replicate in the normal cells of non-avian hosts (Fiola et al., 2006).
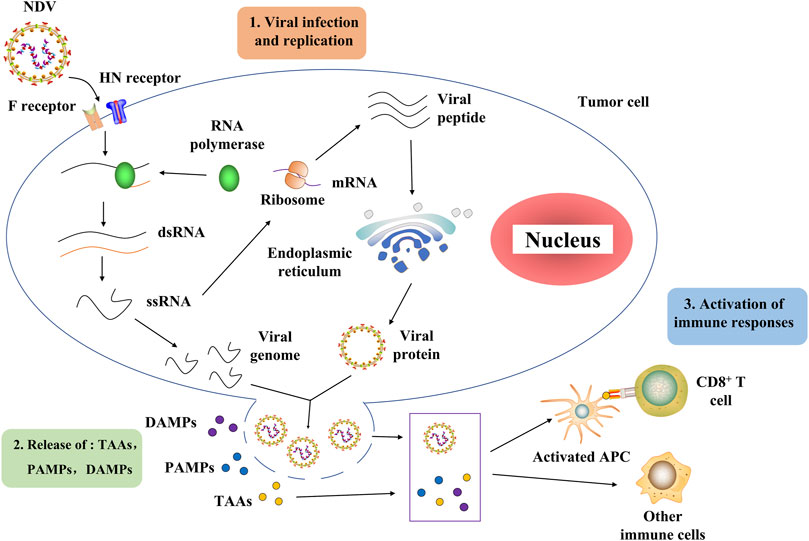
FIGURE 2. Process of NDV through which it infects tumor cells and activates the host immune system. NDV exerts its antitumor effect mainly in two stages. In the first stage, the NDV binds to the sialic acid receptor on the surface of tumor cells through the HN protein, and then protein F initiates the fusion of the viral and host cell membranes. Then, the viral RNA polymerase transcribes the viral negative single-stranded RNA into positive single-stranded RNA as a template for mRNA and protein synthesis. The rough endoplasmic reticulum processes surface proteins F and HN, assembled on the host cell membrane and germinated to produce new virions that begin a new round of tumor cell infection. In the second stage, the virus-mediated direct oncolysis leads to the release of TAAs, PAMPs, and DAMPs that activate APCs, including dendritic cells capable of antigen cross-presentation. Activated APCs activate immune cells, resulting in the generation of CD4+ T cells, CD8+ T cells, and NK cells directed toward tumor and viral antigens. NDV, Newcastle disease virus; HN, hemagglutinin–neuraminidase; F, fusion protein; dsRNA, double-stranded RNA; ssRNA, single-stranded RNA; TAAs, tumor-associated antigens, PAMPs, pathogen-associated molecular patterns, DAMPs, danger-associated molecular patterns; APCs, antigen-presenting cells.
NDV is an effective oncolytic agent. The oncolytic properties of NDVs are also correlated with the pathogenic classification of NDV strains (lytic or non-lytic) (Dey et al., 2014). It has been found that mesogenic and velogenic NDVs are lytic while lentogenic NDV is non-lytic (Fournier et al., 2012; Ganar et al., 2014). Velogenic NDV kills cancer cells rapidly because they destroy the cytoplasmic membrane of infected cells (Wu et al., 2014). Lytic NDV exhibits multi-loop replication, whereas the non-lytic virus exhibits only single-loop replication (Fournier et al., 2012). In addition, the replication process of NDV takes place in the cytoplasm. This replication mode prevents the virus from integrating with the host genome or recombining with the human virus itself (Ganar et al., 2014). Therefore, NDV is non-pathogenic to humans and thus relatively safe with no side effects, which is a significant advantage of NDV as an oncolytic agent.
Multiple Antitumor Molecular Mechanisms of Newcastle Disease Virus
The induction of apoptosis, autophagy, necroptosis, and immunogenic death (ICD), as well as the stimulation of the immune system, are among NDV’s oncolytic processes (Cuadrado-Castano et al., 2015; Zhang et al., 2018; Shao et al., 2019; Kan et al., 2021). The antitumor mechanism of NDV is briefly described in the following section.
Newcastle Disease Virus Activates the Immune Response
As mentioned earlier, NDV selectively infects tumor cells and rapidly replicates in tumor cells to directly dissolve tumors (Fiola et al., 2006). Significantly, NDV oncolysis reshapes the tumor microenvironment (TME), transforming cold tumors into hot tumors (Burman et al., 2020). This process is beneficial for immune cells to infiltrate tumors. On the one hand, NDV induces the release of the risk-related molecular model of strong antitumor immunity after oncolysis, such as TAAs, PAMPs, and DAMPs (Figure 2). These key risk–related molecular models can activate not only some innate immune cells (NK cells) but also tumor-specific T cells (CD4+ and CD8+ T cells) and recruit APCs into the tumor to initiate an immune response (Schild et al., 1989; Ricca et al., 2018). Remarkably, upregulation of many immune checkpoint molecules (CTLA-4 and PD-1) has been observed on CD4+ and CD8+ T cells in recent years (Zamarin et al., 2014; Nakao et al., 2020). This suggests the possibility of combining NDV and immune checkpoint inhibitors to break immune resistance. On the other hand, the activated non-specific immune cells kill and devour infected tumor cells that are not lysed or resistant to viral oncolysis (Fuertes et al., 2011); when the inflammatory response to NDV infection helps the immune system clear tumors, it also causes immune cells to clear NDV, limiting antitumor effects (Buijs et al., 2014). As a result, developing NDV-based cancer regimens necessitates striking a balance between appropriate viral replication, tumor lysis, and immune response activation.
Newcastle Disease Virus Mediates the Apoptosis Pathway
Apoptosis usually occurs as a defense mechanism, such as in the immune response or when cells are damaged by harmful substances (Norbury and Hickson, 2001); while NDV can induce apoptosis to dissolve tumors (Figure 3). The oncolytic selectivity of NDV on tumor cells depends on tumor cell resistance to apoptosis (Mansour et al., 2011). NDV infection induces the apoptosis of tumor cells mainly through the exogenous and the endogenous pathways (mitochondrial-related pathways) (Liao et al., 2017; Song et al., 2019). Tumor cells infected with NDV can cause the release of cytokines such as IFN-α, IFN-β, and TNF-α, which activates the NF-kB signaling pathway, which in turn stimulates the exogenous apoptotic pathway (Wilden et al., 2009; Elankumaran et al., 2010). Furthermore, compared with normal cells that can secrete both IFN-α and IFN-β, tumor cells infected with NDV strain AF2240 only release IFN-β (Ch’ng et al., 2013). A study by Ghrici et al. (2013)revealed that the mitochondrial-related pathway may be the central activator in NDV-induced apoptosis. They found that AF2240 infected cells activated the opening of mitochondrial transition pores, resulting in the activation of caspase-8 and then the viral NP gene expression. Therefore, the apoptosis-inducing effect of NDV may be independent of virus replication and protein synthesis. In 2015, the p38/MAPK pathway was fully elucidated in NDV-mediated apoptosis (Ch’ng et al., 2015). In NDV-infected tumor cells, phosphorylation of p38 mitogen–activated protein kinase (MAPK) was increased by proinflammatory cytokines during infection. This cytoplasmic stimulation degrades the inhibitor of NF-κB, thus releasing NF-κB (Lawrence, 2009). Furthermore, NDV stimulates the immune system to produce cytokines such as IFN-λ (Bu et al., 2016a), which targets phosphor-STAT1 degradation to block IFN-I signaling (Qiu et al., 2016) and exerts an antitumor effect. Thus, the IFN responsiveness may provide a detection indicator for virotherapy (Pease and Kratzke, 2017). ER stress contributes to the antiviral response to NDV by inducing and increasing apoptosis (Bu et al., 2016b; Shokeen et al., 2021). ER stress reduces viral replication due to eIF2α phosphorylation and induces an alternative caspase 12-dependent programmed cell death response (Bu et al., 2016b; Yan et al., 2018).
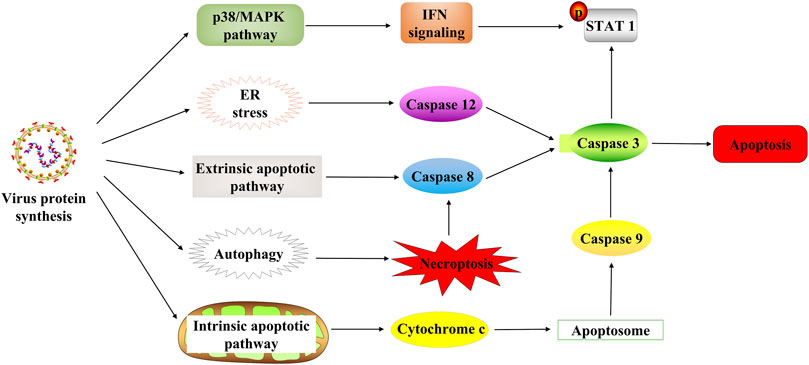
FIGURE 3. NDV-induced cell death in tumor cells. NDV regulates cell death through multiple mechanisms after infection with tumor cells, including the p38/MAPK pathway, ER stress, apoptosis pathway, and autophagy pathway. STAT1, signal transducer and activator of transcription 1; ER, endoplasmic reticulum.
Newcastle Disease Virus Regulates Autophagy
Autophagy is an evolutionarily conserved intracellular process that influences cellular immune responses (Su et al., 2015). At the same time, autophagy is associated with various diseases such as cancer (Smith and Macleod, 2019). The NDV infection also induces tumor-specific autophagy (Figure 3). Many recent studies have focused on the critical role of autophagy in the viral treatment of cancers (Huang et al., 2018; Mattoscio et al., 2018). In addition, NDV exploits the autophagic processes to facilitate their replication, enhancing oncolysis against tumor cells, often leading to tumor necroptosis (Cheng et al., 2016). Furthermore, NDV promotes viral replication via autophagy by inhibiting caspase-dependent apoptosis in cancer cells. Because NDV-induced apoptosis, host immune response, and autophagy affect NDV replication in cancers, it is reasonable to conclude that apoptosis and autophagy are mutually regulated (Pei et al., 2016; Ravegnini et al., 2017; Zhang et al., 2017). Previous research study has revealed that the oncolytic NDV strain NDV/FMW promotes apoptosis in lung cancer cells and facilitates oncolysis in resistant tumor cells suggesting a link between apoptosis and autophagy. Its effect is amplified by the pharmacological regulation of autophagy (Hu et al., 2015). Thus, NDV induces autophagy in apoptotic pathways through the regulation of autophagic activity (Figure 3). Then autophagy inhibits apoptosis and contributes to NDV infection in cancer cells, activating immunity responses in vivo and eventually killing the tumor cells.
Preclinical Application of Newcastle Disease Virus in Various Cancers
NDV has been widely used in preclinical research as a novel anticancer drug for numerous solid tumors and resistant cancers (Table 1). Combining NDV therapy with various cancer medications may fully activate the innate and adaptive antitumor immunity based on the inherent oncolytic capabilities of NDV and its interaction with the immune system. This part summarizes NDV’s use in the preclinical treatment of various cancers.
Gastric Cancer
Gastric cancer is one of the most common malignancies in the digestive system and the second leading cause of cancer-related deaths worldwide, with an approximately overall 5-year survival rate of 30% (Ma et al., 2019; Zhao et al., 2019). Although significant development has been achieved in the treatment of gastric cancer, the prognosis of most patients with gastric cancer remains poor (Liu et al., 2020). Fortunately, the established NDV strains can effectively target and kill gastric cancer cells and activate immune responses (Ma et al., 2019; Liu et al., 2020; Wang et al., 2020), improving tumor treatment efficacy. To detect the oncolytic effect of NDV in gastric cancer, NDV–GFP was constructed by Wong et al. (2010)by inserting the enhanced green fluorescent protein (EGFP) gene, which is a reporter gene. Their study revealed that the GFP-expressing cells counterstained positive for the carcinoembryonic antigen expression in peritoneal lavage samples from gastric adenocarcinoma patients undergoing staging laparoscopy.
Furthermore, NDV–GFP may provide a more sensitive method than conventional cytology for detecting gastric cancer. Bu et al., 2019a and Bu et al., 2019breported that the recombinant LaSota strain expressing rL-RVG (rabies virus glycoprotein) suppressed nAChRs (nicotinic acetylcholine receptors) to reduce cell migration and EMT (epithelial to mesenchymal transition) in gastric cells. Moreover, rL-RVG suppressed the growth of gastric cancer subcutaneous tumor cells in vivo (Bu et al., 2019a).
As previously stated, NDV infection results in the release of multiple cytokines, including type I interferon (IFN), interleukin 1 (IL-1), and tumor necrosis factor-alpha (TNF-α) in vitro and in vivo (Shobana et al., 2013). Meanwhile, it has been confirmed that NDV strains armed with IFN or IL gene result in higher oncolytic efficacy in tumor cells (Mohamed Amin et al., 2019). For gastric cancer, the presence of various polymorphisms for genes coding IL-2, which is associated with poor prognosis in gastric cancer patients, might provide a therapeutic target to inhibit gastric cancer progression (Bai et al., 2014; Andersen et al., 2017). Thus, NDV has an excellent application prospect in immunotherapy for gastric cancer. In addition, it is reported that immune cells with improved survival and prognosis induce immunological memory in the gastric cancer cells and enhance tumor regression (Wong et al., 2010; Lam et al., 2011). In humans, the progression of gastric cancer is associated with the immune function of specific lymphocytes, such as NK cells (Liu et al., 2015; Subhash et al., 2015; Zhao et al., 2018). Cytokines are secreted after the NDV infection of tumor cells, which causes NK cells to become activated. The activated NK cells promote the cytokine release and the activation of other immune cell functions (Bie et al., 2016). To summarize, NDV has a severe toxic effect on gastric cancer cells. NDV-activated cytokines and immune cells, on the other hand, increase antitumor cytotoxic activity against gastric cancer cells and are subsequently predicted to cure gastric cancer.
Liver Cancer
Liver cancer is the fifth most frequent cancer worldwide and the fourth contributor to cancer-related death globally (Mokrane et al., 2020; Ioannou, 2021). Curative resection or liver transplantation is the primary treatment for individuals with liver cancer, but therapeutic success is still poor (Huge et al., 2020). Therefore, novel treatment strategies are urgently needed to eliminate cancer cells effectively. Chen et al. (2016)demonstrated that a recombinant DNA vaccine containing the NDV HN gene inhibits hepatocellular carcinoma cell proliferation (HCC). Furthermore, it induces autophagy via the mitochondrial pathway in vitro and in vivo. This indicates that NDV-based cancer therapy is a promising candidate for liver cancer treatment.
In addition, modified NDV can significantly improve the therapeutic efficacy of NDV in the liver cancer model (Song et al., 2007; An et al., 2016). For some examples, NDV/Anh-TRAIL promotes the mouse liver cancer model to produce immune memory and protect mice from further malignant tumor challenges (Wu et al., 2017). IFN-stimulated gene (ISG)-12a mediates this process, but high basal ISG-12a may inhibit the replication and infection of NDV (Liu et al., 2014). An NDV vector with an L289A mutation inside the NDV F gene can improve NDV’s oncolytic action on HCC cells in vitro and in vivo, indicating promising potential (Altomonte et al., 2010). NDV expressing the chimeric antibody (cHAb18) against tumor-associated antigen CD147 inhibits HCC cell migration and invasion, induces tumor necrosis, and prolongs the survival time of mice (Wei et al., 2015).
Combination therapy, which offers more significant advantages than single-drug therapy, is becoming an increasingly essential aspect of anticancer therapies (Nastiuk and Krolewski, 2016; Martin and Bell, 2018). The combination of NDV therapy and traditional/non-traditional therapies may become a novel choice for HCC treatment. A recent study confirms that fludarabine as an adjuvant enhances the antitumor immunity of NDV-mediated HCC treatment (Meng et al., 2019). Also, the combination treatment of NDV with 5-FU has greater antitumor efficacy than treatment with NDV or 5-FU alone (Assayaghi et al., 2019). Although OVs can strongly trigger immune activation, a negative feedback is usually upregulated in TME (Reale et al., 2019). Meng et al. (2020)indicated that dichloroacetate improved NDV-mediated viral immunotherapy for HCC by reducing the negative immunological feedback and boosting viral replication. These data suggest that more research into the clinical transformation of NDV in immunotherapy for liver cancer is essential.
Lung Cancer
Non–small cell lung cancers (NSCLCs) account for 85% of lung cancer cases and are the leading cause of cancer death (Tan et al., 2021). Increasing evidence suggests that NDV, in addition to direct oncolysis, mediates lung cancer cell proliferation by controlling the cell immune response (Ye et al., 2018; Shao et al., 2019). NDV-D90, an NDV strain isolated from natural sources, exerts an antiproliferative effect in A549 cells (human lung cancer cell lines) (Fu et al., 2011). Another NDV strain, RL-RVG, decreased tumor growth, subcutaneous tumor necrosis, tumor apoptosis, and increased clusters of differentiation (CD)3-/CD49 + NK cells in the tumor-bearing mice group (Yan et al., 2014). These findings emphasize the significance of NDV eliciting an antitumor immune response in lung cancer treatment. Furthermore, multiple studies have found that the occurrence and progression of cancer are linked to the deregulation of a range of microRNAs (miRNAs) (Hao et al., 2011; Che et al., 2020). The overexpression or suppression of miR-204 was substantially associated with NDV-induced oncolysis in A549 cells (Liang et al., 2021). Therefore, targeting some key miRNAs may provide a new direction for cancer therapy.
Autophagy is a defensive reaction to the cellular stress, such as viral infection. NDV inhibits mitophagy to increase viral replication by inhibiting intrinsic apoptosis (Meng et al., 2014). The induction of ICD determinants by NDV was significantly reduced when autophagy-related genes were knocked out in lung cancer cells (Ye et al., 2018). Moreover, the treatment of lung cancer spheroids with the autophagy inhibitor chloroquine increases NDV/FMW-induced cytotoxicity (Hu et al., 2015), indicating NDV may be a potential strategy for targeting lung cancer stem cells. These findings imply that NDV combined with autophagy modulators helps improve NDV’s cancer therapeutic activity.
Breast Cancer
According to the most recent cancer statistics, breast cancer is currently the most frequent malignancy in women and one of the significant causes of death worldwide (Sung et al., 2021). Breast cancer can be classified based on immunohistochemical markers such as estrogen receptor (ER), progesterone receptor (PR), and human epidermal growth factor receptor 2 (HER2) (Xupeng et al., 2021). Despite considerable advancements in breast cancer treatment, patients with triple-negative breast cancer (TNBC) have restricted treatment options due to a lack of recognizable specific markers (Kalscheuer et al., 2019). NDV represents a great potential candidate in the treatment of breast cancer. According to the Kalantari et al. (2020)study, NDV killed breast cancer cells by triggering the intrinsic apoptotic pathway, characterized by elevated Bax, caspase-9, and caspase-3. NDV-D90 induced apoptosis by differentially modulating the expression of ERα and GPER in ER-positive/negative breast cancer cells exposed to estrogen, respectively (Shan et al., 2021).
Furthermore, NDV-AF2240, as an ideal inducer of apoptosis, induces the apoptosis of breast cancer cells and is more cytotoxic to breast cancer than other NDV strains (Raihan et al., 2019). These results suggest that NDV promotes breast tumor regression via apoptotic-dependent pathways. In addition, the breast cancer cells infected with NDV showed a significant decrease in glycolysis activity (Al-Ziaydi et al., 2020a). NDV also plays an essential role in the combined treatment of breast cancer. The 2-DG (2-deoxyglucose), a kind of glucose analog in combination with NDV, showed more significant tumor growth inhibition than in a single treatment (Al-Shammari et al., 2019). D-Mannoheptulose, a particular hexokinase inhibitor, was employed by Ahmed et al. to prevent glycolysis and increase the antitumor activity of NDV (Al-Ziaydi et al., 2020b). The hemagglutinin–neuraminidase (HN) protein of NDV enables NDV to target breast cancer cells (Al-Ziaydi et al., 2020b) effectively. Therefore, NDV has a promising future in the treatment of breast cancer.
Other Cancers
As an oncolytic agent, NDV has been reported in other types of cancers (Table 1), including cervical cancer (Keshavarz et al., 2020a), prostate cancer (Wang et al., 2020), colorectal cancer (Song et al., 2019), and glioblastoma (Abdullah et al., 2014). Cancer is a dynamic disease (Dagogo-Jack and Shaw, 2018), so there are significant differences in cancer cells from different tissue sources, even if there is phenotypic and functional heterogeneity among cancer cells in the same tumor (Meacham and Morrison, 2013). In addition, NDV has other killing mechanisms in different cell lines (Ginting et al., 2019; Li et al., 2019), suggesting that we should carry out the targeted treatment when developing NDV therapy. MSCs (mesenchymal stem cells) represent a potential delivery method (Uder et al., 2018). For instance, Mohsen K et al. found that an MSC-engineered system significantly reduced tumor growth, enhancing CD8+ T-cell cytolysis responses and splenic cytokine responses. This finding demonstrates that MSCs expressing oncolytic NDV may be a viable method for cancer immunotherapy (Keshavarz et al., 2020b).
Clinical Application of Newcastle Disease Virus
Increasing clinical evidence indicates that oncolytic NDV as a therapeutic agent (a type of immunotherapy) can eliminate glioma, metastatic cancer, and advanced solid tumor cells while stimulating patients’ immune systems as well (Table 2).
The major NDV strains evaluated for direct human injection are PV-701 (Pecora et al., 2002), 73-T (Cassel and Garrett, 1965), MTH-68/H (Csatary et al., 1999), and ATV-NDV (Schirrmacher and Fournier, 2009), which are lytic and HUJ (Yaacov et al., 2008), which is non-lytic. In 1964, Wheelock and Dingle (1964) first reported the use of NDV in the treatment of human cancer. After a patient with acute myeloid leukemia was continuously inoculated with the NDV Hickman strain, the number of leukemia cells decreased rapidly, and the symptoms improved, which lasted for nearly 2 weeks (Wheelock and Dingle, 1964). In the following year, a study by William Cassel and his colleagues showed that patients with stage II and III melanoma resected with NDV-73T strain oncolysis were vaccinated with improved overall survival (Cassel et al., 1977; Murray et al., 1977; Cassel et al., 1983). Long-term follow-up of these patients showed a 10-year survival rate of more than 60% and a 15-year survival rate of 55% compared with historical controls (Cassel and Murray, 1992; Batliwalla et al., 1998). This is the early use of NDV-based tumor vaccines for active tumor–specific immunity. Liang et al. later confirmed using an autologous NDV–modified tumor cell vaccination to treat gastrointestinal cancers. They compared 310 patients with stage I–IV colorectal cancer who received resection and immunotherapy with 257 patients who received chemotherapy with resection alone. The median overall survival of the vaccine group was more than 7 years, while that of the resection group was 4.46 years (Liang et al., 2003; Burman et al., 2020). In non-controlled experiments, adjuvant immunization with autologous NDV–modified cancer cells was safe and advantageous.
Immune checkpoint inhibitors are one of the most promising agents in tumor therapy in recent years (Nettelbeck et al., 2021). Durvalumab is a selective, high-affinity, human IgG1 monoclonal antibody that blocks programmed death-ligand 1 (PD-L1) binding to programmed death 1 (PD-1) (Stewart et al., 2015). Recombinant NDV (MEDI5395) expressing granulocyte-macrophage colony-stimulating factor (GMCSF), based on the strain NDV-73T, is being evaluated with intravenous administration (NCT03889275) in conjunction with durvalumab in patients with various advanced malignant tumors (Burke et al., 2020). Other recombinant NDVs are at different stages of development and are expected to enter clinical practice in the next few years. Meanwhile, NDV can be armed with foreign genes via the reverse genetic technology to achieve more effective and diverse antitumor effects. The combination of genetic engineering NDV with computational approaches may be beneficial to enhance the efficacy of clinical cancer treatment (Lathwal et al., 2020).
Conclusion
The tumor is a recalcitrant disease that poses a severe threat to human life and health. NDV acts as a potent oncolytic agent by causing apoptosis, autophagy and necrosis in tumor cells, limiting cell metabolism, and generating a series of immunological responses. At the same time, it has essentially no effect on human normal cells. NDV is also one of the few viruses that have been found to produce partial or even complete responses when treated with a single medication. The persistence of these responses suggests that the virus’s therapeutic effect may depend not only on direct oncolysis but also on the virus’s potential to promote long-term immunity. With the development of virotherapy, the activation of the immune responses through cancer virotherapy may eradicate tumors. NDV currently shows great promise in preclinical and clinical trials.
NDV replication occurs in the cytoplasm and does not integrate into the genome of the host, maintaining the safety of the parental virus. The oncolytic property of NDV is either lytic or non-lytic that only infect cells with a disturbed interferon system, which improves the safety of NDV as a vaccine. NDV does not need to be armed with foreign genes to have a strong antitumor effect and stable expression of foreign genes. The combination of NDV virus therapy and traditional/new tumor treatment techniques has been reported and has broad application prospects. However, many questions about NDV therapy, such as those about other OVs, remain unresolved, including the practical techniques of administration, the best genetic engineering strategies, the therapeutic sequence of immune checkpoint inhibitors, and the best combination partners. There is currently no conventional optimum method for how and when patients should use the virus. The tumor microenvironmental barrier and the cytoplasmic matrix of solid tumors may interfere with and inhibit virus invasion and replication, reducing its oncolytic action. Excessive foreign genes will affect the replication of NDV. Moreover, the preparation of NDV needs deep purification to obtain clinical-grade virus preparation.
Cancer patients are usually immunocompromised, while immunocompromised patients may benefit more from OV therapy. For example, cancer patients infected with COVID-19 have low levels of antibodies against the spike protein. An oncolytic vaccine based on the spike protein not only has a strong antitumor effect but also may be beneficial to the prevention of COVID-19. Further understanding of the immunological system may help develop more effective oncolytic NDV and the elimination of the NDV treatment barrier in solid tumors. The combination of NDV therapy and traditional/non-traditional therapies may become a novel choice for cancer treatment. Combining NDV viral therapy with existing immunotherapy, which uses NDV’s effect on the immune response, may result in a higher antitumor effect. As a result, NDV is likely to be an ideal tumor therapeutic agent in the future.
Author Contributions
Conceptualization, FH, YW, and GR; writing—original draft preparation, FH, CD, YZ, and YZ; writing—review and editing, FH, YW, and GR; supervision, YW and GR; project administration and funding acquisition, FH and YW.
Funding
This study was supported by the Public Welfare Technology Project of Zhejiang Province (LGF21H160033), the Zhejiang Medical Technology Plan Project (2021KY047), the National Natural Science Foundation of China (No. 81803069), and the Grant for 521 talent project of ZSTU.
Conflict of Interest
The authors declare that the research was conducted in the absence of any commercial or financial relationships that could be construed as a potential conflict of interest.
Publisher’s Note
All claims expressed in this article are solely those of the authors and do not necessarily represent those of their affiliated organizations, or those of the publisher, the editors, and the reviewers. Any product that may be evaluated in this article, or claim that may be made by its manufacturer, is not guaranteed or endorsed by the publisher.
References
Abdullah, J. M., Mustafa, Z., and Ideris, A. (2014). Newcastle Disease Virus Interaction in Targeted Therapy against Proliferation and Invasion Pathways of Glioblastoma Multiforme. BioMed Res. Int. 2014, 1–11. doi:10.1155/2014/386470
Adachi, K., Kano, Y., Nagai, T., Okuyama, N., Sakoda, Y., and Tamada, K. (2018). IL-7 and CCL19 Expression in CAR-T Cells Improves Immune Cell Infiltration and CAR-T Cell Survival in the Tumor. Nat. Biotechnol. 36 (4), 346–351. doi:10.1038/nbt.4086
Al-Shammari, A. M., Abdullah, A. H., Allami, Z. M., and Yaseen, N. Y. (2019). 2-Deoxyglucose and Newcastle Disease Virus Synergize to Kill Breast Cancer Cells by Inhibition of Glycolysis Pathway through Glyceraldehyde3-Phosphate Downregulation. Front. Mol. Biosci. 6, 90. doi:10.3389/fmolb.2019.00090
Al-Ziaydi, A. G., Al-Shammari, A. M., Hamzah, M. I., Kadhim, H. S., and Jabir, M. S. (2020). Hexokinase Inhibition Using D-Mannoheptulose Enhances Oncolytic Newcastle Disease Virus-Mediated Killing of Breast Cancer Cells. Cancer Cell Int. 20, 420. doi:10.1186/s12935-020-01514-2
Al-Ziaydi, A. G., Al-Shammari, A. M., Hamzah, M. I., Kadhim, H. S., and Jabir, M. S. (2020). Newcastle Disease Virus Suppress Glycolysis Pathway and Induce Breast Cancer Cells Death. VirusDis. 31 (3), 341–348. doi:10.1007/s13337-020-00612-z
Alamares, J. G., Elankumaran, S., Samal, S. K., and Iorio, R. M. (2010). The Interferon Antagonistic Activities of the V Proteins from Two Strains of Newcastle Disease Virus Correlate with Their Known Virulence Properties. Virus Res. 147 (1), 153–157. doi:10.1016/j.virusres.2009.10.020
Altomonte, J., Marozin, S., Schmid, R. M., and Ebert, O. (2010). Engineered Newcastle Disease Virus as an Improved Oncolytic Agent against Hepatocellular Carcinoma. Mol. Ther. 18 (2), 275–284. doi:10.1038/mt.2009.231
Alvarez-Breckenridge, C. A., Yu, J., Caligiuri, M. A., and Chiocca, E. A. (2013). Uncovering a Novel Mechanism Whereby NK Cells Interfere with Glioblastoma Virotherapy. Oncoimmunology 2 (4), e23658. doi:10.4161/onci.23658
An, Y., Liu, T., He, J., Wu, H., Chen, R., Liu, Y., et al. (2016). Recombinant Newcastle Disease Virus Expressing P53 Demonstrates Promising Antitumor Efficiency in Hepatoma Model. J. Biomed. Sci. 23 (1), 55. doi:10.1186/s12929-016-0273-0
Andersen, S. H., Vervelde, L., Sutton, K., Norup, L. R., Wattrang, E., Juul-Madsen, H. R., et al. (2017). Quantification and Phenotypic Characterisation of Peripheral IFN-γ Producing Leucocytes in Chickens Vaccinated against Newcastle Disease. Veterinary Immunol. Immunopathol. 193-194, 18–28. doi:10.1016/j.vetimm.2017.10.001
Andtbacka, R. H. I., Collichio, F., Harrington, K. J., Middleton, M. R., Downey, G., Ӧhrling, K., et al. (2019). Final Analyses of OPTiM: a Randomized Phase III Trial of Talimogene Laherparepvec versus Granulocyte-Macrophage Colony-Stimulating Factor in Unresectable Stage III-IV Melanoma. J. Immunother. Cancer 7 (1), 145. doi:10.1186/s40425-019-0623-z
Assayaghi, R. M., Alabsi, A. M., Swethadri, G., and Ali, A. M. (2019). Liver Pathology in Rats Treated with Newcastle Disease Virus Strains AF2240 and V4-UPM. Asian Pac J. Cancer Prev. 20 (10), 3071–3075. doi:10.31557/APJCP.2019.20.10.3071
Bai, F.-L., Yu, Y.-H., Tian, H., Ren, G.-P., Wang, H., Zhou, B., et al. (2014). Genetically Engineered Newcastle Disease Virus Expressing Interleukin-2 and TNF-Related Apoptosis-Inducing Ligand for Cancer Therapy. Cancer Biol. Ther. 15 (9), 1226–1238. doi:10.4161/cbt.29686
Bai, Y., Chen, Y., Hong, X., Liu, X., Su, X., Li, S., et al. (2018). Newcastle Disease Virus Enhances the Growth-Inhibiting and Proapoptotic Effects of Temozolomide on Glioblastoma Cells In Vitro and In Vivo. Sci. Rep. 8 (1), 11470. doi:10.1038/s41598-018-29929-y
Batliwalla, F. M., Bateman, B. A., Serrano, D., Murray, D., Macphail, S., Maino, V. C., et al. (1998). A 15-year Follow-Up of AJCC Stage III Malignant Melanoma Patients Treated Postsurgically with Newcastle Disease Virus (NDV) Oncolysate and Determination of Alterations in the CD8 T Cell Repertoire. Mol. Med. 4 (12), 783–794. doi:10.1007/bf03401771
Bello, M. B., Yusoff, K., Ideris, A., Hair-Bejo, M., Jibril, A. H., Peeters, B. P. H., et al. (2020). Exploring the Prospects of Engineered Newcastle Disease Virus in Modern Vaccinology. Viruses 12 (4), 451. doi:10.3390/v12040451
Bie, Q., Sun, C., Gong, A., Li, C., Su, Z., Zheng, D., et al. (2016). Non-tumor Tissue Derived interleukin-17B Activates IL-17RB/AKT/β-catenin Pathway to Enhance the Stemness of Gastric Cancer. Sci. Rep. 6, 25447. doi:10.1038/srep25447
Boshuizen, J., and Peeper, D. S. (2020). Rational Cancer Treatment Combinations: An Urgent Clinical Need. Mol. Cell 78 (6), 1002–1018. doi:10.1016/j.molcel.2020.05.031
Bu, X., Zhao, Y., Zhang, Z., Wang, M., Li, M., and Yan, Y. (2016). Recombinant Newcastle Disease Virus (rL-RVG) Triggers Autophagy and Apoptosis in Gastric Carcinoma Cells by Inducing ER Stress. Am. J. Cancer Res. 6 (5), 924–936.
Bu, X., Li, M., Zhao, Y., Liu, S., Wang, M., Ge, J., et al. (2016). Genetically Engineered Newcastle Disease Virus Expressing Human Interferon-Λ1 Induces Apoptosis in Gastric Adenocarcinoma Cells and Modulates the Th1/Th2 Immune Response. Oncol. Rep. 36 (3), 1393–1402. doi:10.3892/or.2016.4925
Bu, X., Yin, C., Zhang, X., Zhang, A., Shao, X., Zhang, Y., et al. (2019). LaSota Strain Expressing the Rabies Virus Glycoprotein (rL-RVG) Suppresses Gastric Cancer by Inhibiting the Alpha 7 Nicotinic Acetylcholine Receptor (α7 nAChR)/Phosphoinositide 3-Kinase (PI3K)/AKT Pathway. Med. Sci. Monit. 25, 5482–5492. doi:10.12659/MSM.915251
Bu, X., Zhang, A., Chen, Z., Zhang, X., Zhang, R., Yin, C., et al. (2019). Migration of Gastric Cancer Is Suppressed by Recombinant Newcastle Disease Virus (rL-RVG) via Regulating α7-nicotinic Acetylcholine Receptors/ERK- EMT. BMC Cancer 19 (1), 976. doi:10.1186/s12885-019-6225-9
Buijs, P. R. A., van Eijck, C. H. J., Hofland, L. J., Fouchier, R. A. M., and van den Hoogen, B. G. (2014). Different Responses of Human Pancreatic Adenocarcinoma Cell Lines to Oncolytic Newcastle Disease Virus Infection. Cancer Gene Ther. 21 (1), 24–30. doi:10.1038/cgt.2013.78
Burke, S., Shergold, A., Elder, M. J., Whitworth, J., Cheng, X., Jin, H., et al. (2020). Oncolytic Newcastle Disease Virus Activation of the Innate Immune Response and Priming of Antitumor Adaptive Responses In Vitro. Cancer Immunol. Immunother. 69 (6), 1015–1027. doi:10.1007/s00262-020-02495-x
Burman, B., Pesci, G., and Zamarin, D. (2020). Newcastle Disease Virus at the Forefront of Cancer Immunotherapy. Cancers 12 (12), 3552. doi:10.3390/cancers12123552
Carrasco, A. d. O. T., Seki, M. C., Benevenute, J. L., Ikeda, P., and Pinto, A. A. (2016). Experimental Infection with Brazilian Newcastle Disease Virus Strain in Pigeons and Chickens. Braz. J. Microbiol. 47 (1), 231–242. doi:10.1016/j.bjm.2015.07.001
Cassel, W. A., and Garrett, R. E. (1965). Newcastle Disease Virus as an Antineoplastic Agent. Cancer 18, 863–868. doi:10.1002/1097-0142(196507)18:7<863:aid-cncr2820180714>3.0.co;2-v
Cassel, W. A., and Murray, D. R. (1992). A Ten-Year Follow-Up on Stage II Malignant Melanoma Patients Treated Postsurgically with Newcastle Disease Virus Oncolysate. Med Oncol. Tumor Pharmacother. 9 (4), 169–171. doi:10.1007/bf02987752
Cassel, W. A., Murray, D. R., and Phillips, H. S. (1983). A Phase II Study on the Postsurgical Management of Stage II Malignant Melanoma with a Newcastle Disease Virus Oncolysate. Cancer 52 (5), 856–860. doi:10.1002/1097-0142(19830901)52:5<856:aid-cncr2820520519>3.0.co;2-4
Cassel, W. A., Murray, D. R., Torbin, A. H., Olkowski, Z. L., and Moore, M. E. (1977). Viral Oncolysate in the Management of Malignant melanoma.I. Preparation of the Oncolysate and Measurement of Immunologic Responses. Cancer 40 (2), 672–679. doi:10.1002/1097-0142(197708)40:2<672:aid-cncr2820400213>3.0.co;2-y
Ch'ng, W.-C., Stanbridge, E. J., Yusoff, K., and Shafee, N. (2013). The Oncolytic Activity of Newcastle Disease Virus in Clear Cell Renal Carcinoma Cells in Normoxic and Hypoxic Conditions: The Interplay Between von Hippel-Lindau and Interferon-β Signaling. J. Interferon. Cytokine Res. 33 (7), 346–354. doi:10.1089/jir.2012.0095
Che, X., Jian, F., Chen, C., Liu, C., Liu, G., and Feng, W. (2020). PCOS Serum-Derived Exosomal miR-27a-5p Stimulates Endometrial Cancer Cells Migration and Invasion. J. Mol. Endocrinol. 64 (1), 1–12. doi:10.1530/JME-19-0159
Chen, H., Jiang, T., Lin, F., Guan, H., Zheng, J., Liu, Q., et al. (2021). PD-1 Inhibitor Combined with Apatinib Modulate the Tumor Microenvironment and Potentiate Anti-tumor Effect in Mice Bearing Gastric Cancer. Int. Immunopharmacol. 99, 107929. doi:10.1016/j.intimp.2021.107929
Chen, L.-G., Liu, Y.-S., Zheng, T.-H., Chen, X., Li, P., Xiao, C.-X., et al. (2016). Therapeutic Targeting of Liver Cancer with a Recombinant DNA Vaccine Containing the Hemagglutinin-Neuraminidase Gene of Newcastle Disease Virus via Apoptotic-dependent Pathways. Oncol. Lett. 12 (5), 3344–3350. doi:10.3892/ol.2016.5114
Chen, R. X., Liu, H. L., Yang, L. L., Kang, F. H., Xin, L. P., Huang, L. R., et al. (2019). Circular RNA circRNA_0000285 Promotes Cervical Cancer Development by Regulating FUS. Eur. Rev. Med. Pharmacol. Sci. 23 (20), 8771–8778. doi:10.26355/eurrev_201910_19271
Cheng, J.-H., Sun, Y.-J., Zhang, F.-Q., Zhang, X.-R., Qiu, X.-S., Yu, L.-P., et al. (2016). Newcastle Disease Virus NP and P Proteins Induce Autophagy via the Endoplasmic Reticulum Stress-Related Unfolded Protein Response. Sci. Rep. 6, 24721. doi:10.1038/srep24721
Ch’ng, W.-C., Abd-Aziz, N., Ong, M.-H., Stanbridge, E. J., and Shafee, N. (2015). Human Renal Carcinoma Cells Respond to Newcastle Disease Virus Infection through Activation of the P38 MAPK/NF-κB/IκBα Pathway. Cell Oncol. 38 (4), 279–288. doi:10.1007/s13402-015-0229-5
Csatary, L. K., Gosztonyi, G., Szeberenyi, J., Fabian, Z., Liszka, V., Bodey, B., et al. (2004). MTH-68/H Oncolytic Viral Treatment in Human High-Grade Gliomas. J. Neurooncol. 67 (1-2), 83–93. doi:10.1023/b:neon.0000021735.85511.05
Csatary, L. K., Moss, R. W., Beuth, J., Töröcsik, B., Szeberenyi, J., and Bakacs, T. (1999). Beneficial Treatment of Patients with Advanced Cancer Using a Newcastle Disease Virus Vaccine (MTH-68/H). Anticancer Res. 19 (1B), 635–638.
Cuadrado-Castano, S., Sanchez-Aparicio, M. T., García-Sastre, A., and Villar, E. (2015). The Therapeutic Effect of Death: Newcastle Disease Virus and its Antitumor Potential. Virus Res. 209, 56–66. doi:10.1016/j.virusres.2015.07.001
Dagogo-Jack, I., and Shaw, A. T. (2018). Tumour Heterogeneity and Resistance to Cancer Therapies. Nat. Rev. Clin. Oncol. 15 (2), 81–94. doi:10.1038/nrclinonc.2017.166
Dai, C.-J., Cao, Y.-T., Huang, F., and Wang, Y.-G. (2022). Multiple Roles of Mothers against Decapentaplegic Homolog 4 in Tumorigenesis, Stem Cells, Drug Resistance, and Cancer Therapy. World J. Stem Cells 14 (1), 41–53. doi:10.4252/wjsc.v14.i1.41
Dey, S., Chellappa, M. M., Gaikwad, S., Kataria, J. M., and Vakharia, V. N. (2014). Genotype Characterization of Commonly Used Newcastle Disease Virus Vaccine Strains of India. PloS One 9 (6), e98869. doi:10.1371/journal.pone.0098869
Dey, S., Chellappa, M., Pathak, D., Gaikwad, S., Yadav, K., Ramakrishnan, S., et al. (2017). Newcastle Disease Virus Vectored Bivalent Vaccine against Virulent Infectious Bursal Disease and Newcastle Disease of Chickens. Vaccines 5 (4), 31. doi:10.3390/vaccines5040031
Dimitrov, K. M., Ramey, A. M., Qiu, X., Bahl, J., and Afonso, C. L. (2016). Temporal, Geographic, and Host Distribution of Avian Paramyxovirus 1 (Newcastle Disease Virus). Infect. Genet. Evol. 39, 22–34. doi:10.1016/j.meegid.2016.01.008
Elankumaran, S., Chavan, V., Qiao, D., Shobana, R., Moorkanat, G., Biswas, M., et al. (2010). Type I Interferon-Sensitive Recombinant Newcastle Disease Virus for Oncolytic Virotherapy. J. Virol. 84 (8), 3835–3844. doi:10.1128/JVI.01553-09
Fares, J., Fares, M. Y., Khachfe, H. H., Salhab, H. A., and Fares, Y. (2020). Molecular Principles of Metastasis: a Hallmark of Cancer Revisited. Sig Transduct. Target Ther. 5 (1), 28. doi:10.1038/s41392-020-0134-x
Fiola, C., Peeters, B., Fournier, P., Arnold, A., Bucur, M., and Schirrmacher, V. (2006). Tumor Selective Replication of Newcastle Disease Virus: Association with Defects of Tumor Cells in Antiviral Defence. Int. J. Cancer 119 (2), 328–338. doi:10.1002/ijc.21821
Fournier, P., Bian, H., Szeberényi, J., and Schirrmacher, V. (2012). Analysis of Three Properties of Newcastle Disease Virus for Fighting Cancer: Tumor-Selective Replication, Antitumor Cytotoxicity, and Immunostimulation. Methods Mol. Biol. 797, 177–204. doi:10.1007/978-1-61779-340-0_13
Fournier, P., Zeng, J., Von Der Lieth, C.-W., Washburn, B., Ahlert, T., and Schirrmacher, V. (2004). Importance of Serine 200 for Functional Activities of the Hemagglutinin-Neuraminidase Protein of Newcastle Disease Virus. Int. J. Oncol. 24 (3), 623–634. doi:10.3892/ijo.24.3.623
Freeman, A. I., Zakay-Rones, Z., Gomori, J. M., Linetsky, E., Rasooly, L., Greenbaum, E., et al. (2006). Phase I/II Trial of Intravenous NDV-HUJ Oncolytic Virus in Recurrent Glioblastoma Multiforme. Mol. Ther. 13 (1), 221–228. doi:10.1016/j.ymthe.2005.08.016
Fu, F., Zhao, M., Yang, Y.-J., Tong, G.-Z., Yang, B.-F., Song, C., et al. (2011). Antiproliferative Effect of Newcastle Disease Virus Strain D90 on Human Lung Cancer Cell Line A549. Oncol. Res. 19 (7), 323–333. doi:10.3727/096504011x13079697132880
Fuertes, M. B., Kacha, A. K., Kline, J., Woo, S.-R., Kranz, D. M., Murphy, K. M., et al. (2011). Host Type I IFN Signals Are Required for Antitumor CD8+ T Cell Responses through CD8α+ Dendritic Cells. J. Exp. Med. 208 (10), 2005–2016. doi:10.1084/jem.20101159
Ganar, K., Das, M., Sinha, S., and Kumar, S. (2014). Newcastle Disease Virus: Current Status and Our Understanding. Virus Res. 184, 71–81. doi:10.1016/j.virusres.2014.02.016
Ghrici, M., El Zowalaty, M., Omar, A. R., and Ideris, A. (2013). Newcastle Disease Virus Malaysian Strain AF2240 Induces Apoptosis in MCF-7 Human Breast Carcinoma Cells at an Early Stage of the Virus Life Cycle. Int. J. Mol. Med. 31 (3), 525–532. doi:10.3892/ijmm.2013.1244
Ginting, T. E., Christian, S., Larasati, Y. O., Suryatenggara, J., Suriapranata, I. M., and Mathew, G. (2019). Antiviral Interferons Induced by Newcastle Disease Virus (NDV) Drive a Tumor-Selective Apoptosis. Sci. Rep. 9 (1), 15160. doi:10.1038/s41598-019-51465-6
Ginting, T., Suryatenggara, J., Christian, S., and Mathew, G. (2017). Proinflammatory Response Induced by Newcastle Disease Virus in Tumor and Normal Cells. Oncolytic Virother 6, 21–30. doi:10.2147/OV.S123292
Gogoi, P., Ganar, K., and Kumar, S. (2017). Avian Paramyxovirus: A Brief Review. Transbound. Emerg. Dis. 64 (1), 53–67. doi:10.1111/tbed.12355
Gong, Y., Tang, N., Liu, P., Sun, Y., Lu, S., Liu, W., et al. (2021). Newcastle Disease Virus Degrades SIRT3 via PINK1-PRKN-dependent Mitophagy to Reprogram Energy Metabolism in Infected Cells. Autophagy 2021, 1–19. doi:10.1080/15548627.2021.1990515
Goradel, N. H., Baker, A. T., Arashkia, A., Ebrahimi, N., Ghorghanlu, S., and Negahdari, B. (2021). Oncolytic Virotherapy: Challenges and Solutions. Curr. Probl. Cancer 45 (1), 100639. doi:10.1016/j.currproblcancer.2020.100639
Hao, J., Zhang, S., Zhou, Y., Liu, C., Hu, X., and Shao, C. (2011). MicroRNA 421 Suppresses DPC4/Smad4 in Pancreatic Cancer. Biochem. Biophys. Res. Commun. 406 (4), 552–557. doi:10.1016/j.bbrc.2011.02.086
Harrington, K., Freeman, D. J., Kelly, B., Harper, J., and Soria, J.-C. (2019). Optimizing Oncolytic Virotherapy in Cancer Treatment. Nat. Rev. Drug Discov. 18 (9), 689–706. doi:10.1038/s41573-019-0029-0
Hu, L., Sun, S., Wang, T., Li, Y., Jiang, K., Lin, G., et al. (2015). Oncolytic Newcastle Disease Virus Triggers Cell Death of Lung Cancer Spheroids and Is Enhanced by Pharmacological Inhibition of Autophagy. Am. J. Cancer Res. 5 (12), 3612–3623.
Hu, Z., Ni, J., Cao, Y., and Liu, X. (2020). Newcastle Disease Virus as a Vaccine Vector for 20 years: A Focus on Maternally Derived Antibody Interference. Vaccines 8 (2), 222. doi:10.3390/vaccines8020222
Huang, F., Wang, B.-R., and Wang, Y.-G. (2018). Role of Autophagy in Tumorigenesis, Metastasis, Targeted Therapy and Drug Resistance of Hepatocellular Carcinoma. World J. Gastroenterol. 24 (41), 4643–4651. doi:10.3748/wjg.v24.i41.4643
Huang, M., He, Y.-R., Liang, L.-C., Huang, Q., and Zhu, Z.-Q. (2017). Circular RNA Hsa_circ_0000745 May Serve as a Diagnostic Marker for Gastric Cancer. World J. Gastroenterol. 23 (34), 6330–6338. doi:10.3748/wjg.v23.i34.6330
Huge, N., Sandbothe, M., Schröder, A. K., Stalke, A., Eilers, M., Schäffer, V., et al. (2020). Wnt Status-dependent Oncogenic Role of BCL9 and BCL9L in Hepatocellular Carcinoma. Hepatol. Int. 14 (3), 373–384. doi:10.1007/s12072-019-09977-w
Ioannou, G. N. (2021). HCC Surveillance after SVR in Patients with F3/F4 Fibrosis. J. hepatology 74 (2), 458–465. doi:10.1016/j.jhep.2020.10.016
Kalantari, A., Farashi Bonab, S., Keyvanfar, H., and Mortazavi, P. (2020). Evaluation of Apoptosis Induction by Newcastle Disease Virus LaSota Strain in Human Breast Carcinoma Cells. Arch. Razi Inst. 75 (3), 367–376. doi:10.22092/ari.2019.125824.1322
Kalscheuer, S., Khanna, V., Kim, H., Li, S., Sachdev, D., DeCarlo, A., et al. (2019). Discovery of HSPG2 (Perlecan) as a Therapeutic Target in Triple Negative Breast Cancer. Sci. Rep. 9 (1), 12492. doi:10.1038/s41598-019-48993-6
Kan, X., Yin, Y., Song, C., Tan, L., Qiu, X., Liao, Y., et al. (2021). Newcastle-disease-virus-induced Ferroptosis through Nutrient Deprivation and Ferritinophagy in Tumor Cells. iScience 24 (8), 102837. doi:10.1016/j.isci.2021.102837
Kapczynski, D. R., Afonso, C. L., and Miller, P. J. (2013). Immune Responses of Poultry to Newcastle Disease Virus. Dev. Comp. Immunol. 41 (3), 447–453. doi:10.1016/j.dci.2013.04.012
Karcher, J., Dyckhoff, G., Beckhove, P., Reisser, C., Brysch, M., Ziouta, Y., et al. (2004). Antitumor Vaccination in Patients with Head and Neck Squamous Cell Carcinomas with Autologous Virus-Modified Tumor Cells. Cancer Res. 64 (21), 8057–8061. doi:10.1158/0008-5472.can-04-1545
Kazimirsky, G., Jiang, W., Slavin, S., Ziv-Av, A., and Brodie, C. (2016). Mesenchymal Stem Cells Enhance the Oncolytic Effect of Newcastle Disease Virus in Glioma Cells and Glioma Stem Cells via the Secretion of TRAIL. Stem Cell Res. Ther. 7 (1), 149. doi:10.1186/s13287-016-0414-0
Keshavarz, M., Ebrahimzadeh, M. S., Miri, S. M., Dianat-Moghadam, H., Ghorbanhosseini, S. S., Mohebbi, S. R., et al. (2020). Oncolytic Newcastle Disease Virus Delivered by Mesenchymal Stem Cells-Engineered System Enhances the Therapeutic Effects Altering Tumor Microenvironment. Virol. J. 17 (1), 64. doi:10.1186/s12985-020-01326-w
Keshavarz, M., Nejad, A. S. M., Esghaei, M., Bokharaei-Salim, F., Dianat-Moghadam, H., Keyvani, H., et al. (2020). Oncolytic Newcastle Disease Virus Reduces Growth of Cervical Cancer Cell by Inducing Apoptosis. Saudi J. Biol. Sci. 27 (1), 47–52. doi:10.1016/j.sjbs.2019.04.015
Koks, C. A., Garg, A. D., Ehrhardt, M., Riva, M., Vandenberk, L., Boon, L., et al. (2015). Newcastle Disease Virotherapy Induces Long‐term Survival and Tumor‐specific Immune Memory in Orthotopic Glioma through the Induction of Immunogenic Cell Death. Int. J. Cancer 136 (5), E313–E325. doi:10.1002/ijc.29202
Kwan, A., Winder, N., and Muthana, M. (2021). Oncolytic Virotherapy Treatment of Breast Cancer: Barriers and Recent Advances. Viruses 13 (6), 1128. doi:10.3390/v13061128
Lam, H. Y., Yeap, S. K., Rasoli, M., Omar, A. R., Yusoff, K., Suraini, A. A., et al. (2011). Safety and Clinical Usage of Newcastle Disease Virus in Cancer Therapy. J. Biomed. Biotechnol. 2011, 1–13. doi:10.1155/2011/718710
Lathwal, A., Kumar, R., and Raghava, G. P. S. (2020). Computer-aided Designing of Oncolytic Viruses for Overcoming Translational Challenges of Cancer Immunotherapy. Drug Discov. Today 25 (7), 1198–1205. doi:10.1016/j.drudis.2020.04.008
Lawler, S. E., Speranza, M.-C., Cho, C.-F., and Chiocca, E. A. (2017). Oncolytic Viruses in Cancer Treatment. JAMA Oncol. 3 (6), 841–849. doi:10.1001/jamaoncol.2016.2064
Lawrence, T. (2009). The Nuclear Factor NF- B Pathway in Inflammation. Cold Spring Harb. Perspect. Biol. 1 (6), a001651. doi:10.1101/cshperspect.a001651
Leber, M. F., Neault, S., Jirovec, E., Barkley, R., Said, A., Bell, J. C., et al. (2020). Engineering and Combining Oncolytic Measles Virus for Cancer Therapy. Cytokine Growth Factor Rev. 56, 39–48. doi:10.1016/j.cytogfr.2020.07.005
Li, Y., Jiang, W., Niu, Q., Sun, Y., Meng, C., Tan, L., et al. (2019). eIF2α-CHOP-BCl-2/JNK and IRE1α-XBP1/JNK Signaling Promote Apoptosis and Inflammation and Support the Proliferation of Newcastle Disease Virus. Cell Death Dis. 10 (12), 891. doi:10.1038/s41419-019-2128-6
Liang, W., Wang, H., Sun, T.-M., Yao, W.-Q., Chen, L.-L., Jin, Y., et al. (2003). Application of Autologous Tumor Cell Vaccine and NDV Vaccine in Treatment of Tumors of Digestive Traet. World J. Gastroenterol. 9 (3), 495–498. doi:10.3748/wjg.v9.i3.495
Liang, Y., Tian, W.-Y., Huang, J.-J., Gao, L.-X., and Fan, X.-H. (2021). MicroRNA-204 P-lays a R-ole as a T-umor S-uppressor in Newcastle D-isease V-irus-induced O-ncolysis in L-ung C-ancer A549 C-ells. Oncol. Lett. 21 (6), 482. doi:10.3892/ol.2021.12743
Liao, Y., Wang, H.-X., Mao, X., Fang, H., Wang, H., Li, Y., et al. (2017). RIP1 Is a Central Signaling Protein in Regulation of TNF-α/TRAIL Mediated Apoptosis and Necroptosis during Newcastle Disease Virus Infection. Oncotarget 8 (26), 43201–43217. doi:10.18632/oncotarget.17970
Liu, B. L., Robinson, M., Han, Z.-Q., Branston, R. H., English, C., Reay, P., et al. (2003). ICP34.5 Deleted Herpes Simplex Virus with Enhanced Oncolytic, Immune Stimulating, and Anti-tumour Properties. Gene Ther. 10 (4), 292–303. doi:10.1038/sj.gt.3301885
Liu, J., Dai, X., Guo, X., Cheng, A., Mac, S. M., and Wang, Z. (2020). Circ-OXCT1 Suppresses Gastric Cancer EMT and Metastasis by Attenuating TGF-β Pathway through the Circ-OXCT1/miR-136/smad4 Axis. Onco. Targets Ther. 13, 3987–3998. doi:10.2147/OTT.S239789
Liu, K., Yang, K., Wu, B., Chen, H., Chen, X., Chen, X., et al. (2015). Tumor-Infiltrating Immune Cells Are Associated with Prognosis of Gastric Cancer. Medicine 94 (39), e1631. doi:10.1097/MD.0000000000001631
Liu, N., Long, Y., Liu, B., Yang, D., Li, C., Chen, T., et al. (2014). ISG12a Mediates Cell Response to Newcastle Disease Viral Infection. Virology 462-463, 283–294. doi:10.1016/j.virol.2014.06.014
Ma, Y., Yan, F., Wei, W., Deng, J., Li, L., Liu, L., et al. (2019). MicroRNA-598 Inhibits the Growth and Maintenance of Gastric Cancer Stem-like Cells by Down-Regulating RRS1. Cell Cycle 18 (20), 2757–2769. doi:10.1080/15384101.2019.1657338
Mahasa, K. J., Eladdadi, A., de Pillis, L., and Ouifki, R. (2017). Oncolytic Potency and Reduced Virus Tumor-Specificity in Oncolytic Virotherapy. A Mathematical Modelling Approach. PloS one 12 (9), e0184347. doi:10.1371/journal.pone.0184347
Mansour, M., Palese, P., and Zamarin, D. (2011). Oncolytic Specificity of Newcastle Disease Virus Is Mediated by Selectivity for Apoptosis-Resistant Cells. J. Virol. 85 (12), 6015–6023. doi:10.1128/JVI.01537-10
Martin, N. T., and Bell, J. C. (2018). Oncolytic Virus Combination Therapy: Killing One Bird with Two Stones. Mol. Ther. 26 (6), 1414–1422. doi:10.1016/j.ymthe.2018.04.001
Mattoscio, D., Medda, A., and Chiocca, S. (2018). Human Papilloma Virus and Autophagy. Int. J. Mol. Sci. 19 (6), 1775. doi:10.3390/ijms19061775
McAusland, T. M., van Vloten, J. P., Santry, L. A., Guilleman, M. M., Rghei, A. D., Ferreira, E. M., et al. (2021). Combining Vanadyl Sulfate with Newcastle Disease Virus Potentiates Rapid Innate Immune-Mediated Regression with Curative Potential in Murine Cancer Models. Mol. Ther. - Oncolytics 20, 306–324. doi:10.1016/j.omto.2021.01.009
Meacham, C. E., and Morrison, S. J. (2013). Tumour Heterogeneity and Cancer Cell Plasticity. Nature 501 (7467), 328–337. doi:10.1038/nature12624
Meng, G., Fei, Z., Fang, M., Li, B., Chen, A., Xu, C., et al. (2019). Fludarabine as an Adjuvant Improves Newcastle Disease Virus-Mediated Antitumor Immunity in Hepatocellular Carcinoma. Mol. Ther. - Oncolytics 13, 22–34. doi:10.1016/j.omto.2019.03.004
Meng, G., Li, B., Chen, A., Zheng, M., Xu, T., Zhang, H., et al. (2020). Targeting Aerobic Glycolysis by Dichloroacetate Improves Newcastle Disease Virus-Mediated Viro-Immunotherapy in Hepatocellular Carcinoma. Br. J. Cancer 122 (1), 111–120. doi:10.1038/s41416-019-0639-7
Meng, G., Xia, M., Wang, D., Chen, A., Wang, Y., Wang, H., et al. (2014). Mitophagy Promotes Replication of Oncolytic Newcastle Disease Virus by Blocking Intrinsic Apoptosis in Lung Cancer Cells. Oncotarget 5 (15), 6365–6374. doi:10.18632/oncotarget.2219
Meng, Q., He, J., Zhong, L., and Zhao, Y. (2021). Advances in the Study of Antitumour Immunotherapy for Newcastle Disease Virus. Int. J. Med. Sci. 18 (11), 2294–2302. doi:10.7150/ijms.59185
Mohamed Amin, Z., Che Ani, M. A., Tan, S. W., Yeap, S. K., Alitheen, N. B., Syed Najmuddin, S. U. F., et al. (2019). Evaluation of a Recombinant Newcastle Disease Virus Expressing Human IL12 against Human Breast Cancer. Sci. Rep. 9 (1), 13999. doi:10.1038/s41598-019-50222-z
Mokrane, F.-Z., Lu, L., Vavasseur, A., Otal, P., Peron, J.-M., Luk, L., et al. (2020). Radiomics Machine-Learning Signature for Diagnosis of Hepatocellular Carcinoma in Cirrhotic Patients with Indeterminate Liver Nodules. Eur. Radiol. 30 (1), 558–570. doi:10.1007/s00330-019-06347-w
Moliner, P., Lupón, J., Antonio, M., Domingo, M., Santiago‐Vacas, E., Zamora, E., et al. (2019). Trends in Modes of Death in Heart Failure over the Last Two Decades: Less Sudden Death but Cancer Deaths on the Rise. Eur. J. Heart Fail 21 (10), 1259–1266. doi:10.1002/ejhf.1569
Molouki, A., and Peeters, B. (2017). Rescue of Recombinant Newcastle Disease Virus: a Short History of How it All Started. Arch. Virol. 162 (7), 1845–1854. doi:10.1007/s00705-017-3308-2
Mondal, M., Guo, J., He, P., and Zhou, D. (2020). Recent Advances of Oncolytic Virus in Cancer Therapy. Hum. Vaccines Immunother. 16 (10), 2389–2402. doi:10.1080/21645515.2020.1723363
Moo, T.-A., Sanford, R., Dang, C., and Morrow, M. (2018). Overview of Breast Cancer Therapy. Pet. Clin. 13 (3), 339–354. doi:10.1016/j.cpet.2018.02.006
Mozaffari Nejad, A. S., Fotouhi, F., Mehrbod, P., and Alikhani, M. Y. (2021). Antitumor Immunity Enhancement through Newcastle Viral Oncolysate in Mice Model: A Promising Method to Treat Tumors. Saudi J. Biol. Sci. 28 (10), 5833–5840. doi:10.1016/j.sjbs.2021.06.043
Munir, M., Zohari, S., Abbas, M., and Berg, M. (2012). Sequencing and Analysis of the Complete Genome of Newcastle Disease Virus Isolated from a Commercial Poultry Farm in 2010. Arch. Virol. 157 (4), 765–768. doi:10.1007/s00705-011-1220-8
Murray, D. R., Cassel, W. A., Torbin, A. H., Olkowski, Z. L., and Moore, M. E. (1977). Viral Oncolysate in the Management of Malignant Melanoma. II. Clinical Studies. Cancer 40 (2), 680–686. doi:10.1002/1097-0142(197708)40:2<680:aid-cncr2820400214>3.0.co;2-#
Nagai, Y., Hamaguchi, M., and Toyoda, T. (1989). Molecular Biology of Newcastle Disease Virus. Prog. Vet. Microbiol. Immunol. 5, 16–64.
Nakao, S., Arai, Y., Tasaki, M., Yamashita, M., Murakami, R., Kawase, T., et al. (2020). Intratumoral Expression of IL-7 and IL-12 Using an Oncolytic Virus Increases Systemic Sensitivity to Immune Checkpoint Blockade. Sci. Transl. Med. 12 (526). doi:10.1126/scitranslmed.aax7992
Nastiuk, K. L., and Krolewski, J. J. (2016). Opportunities and Challenges in Combination Gene Cancer Therapy. Adv. drug Deliv. Rev. 98, 35–40. doi:10.1016/j.addr.2015.12.005
Nettelbeck, D. M., Leber, M. F., Altomonte, J., Angelova, A., Beil, J., Berchtold, S., et al. (2021). Virotherapy in Germany-Recent Activities in Virus Engineering, Preclinical Development, and Clinical Studies. Viruses 13 (8), 1420. doi:10.3390/v13081420
Norbury, C. J., and Hickson, I. D. (2001). Cellular Responses to DNA Damage. Annu. Rev. Pharmacol. Toxicol. 41, 367–401. doi:10.1146/annurev.pharmtox.41.1.367
Park, J. H., Pyun, W. Y., and Park, H. W. (2020). Cancer Metabolism: Phenotype, Signaling and Therapeutic Targets. Cells 9 (10), 2308. doi:10.3390/cells9102308
Pease, D. F., and Kratzke, R. A. (2017). Oncolytic Viral Therapy for Mesothelioma. Front. Oncol. 7, 179. doi:10.3389/fonc.2017.00179
Pecora, A. L., Rizvi, N., Cohen, G. I., Meropol, N. J., Sterman, D., Marshall, J. L., et al. (2002). Phase I Trial of Intravenous Administration of PV701, an Oncolytic Virus, in Patients with Advanced Solid Cancers. J. Clin. Oncol. 20 (9), 2251–2266. doi:10.1200/jco.2002.08.042
Peeters, B. P. H., de Leeuw, O. S., Koch, G., and Gielkens, A. L. J. (1999). Rescue of Newcastle Disease Virus from Cloned cDNA: Evidence that Cleavability of the Fusion Protein Is a Major Determinant for Virulence. J. Virol. 73 (6), 5001–5009. doi:10.1128/jvi.73.6.5001-5009.1999
Pei, J., Deng, J., Ye, Z., Wang, J., Gou, H., Liu, W., et al. (2016). Absence of Autophagy Promotes Apoptosis by Modulating the ROS-dependent RLR Signaling Pathway in Classical Swine Fever Virus-Infected Cells. Autophagy 12 (10), 1738–1758. doi:10.1080/15548627.2016.1196318
Pol, J., Buqué, A., Aranda, F., Bloy, N., Cremer, I., Eggermont, A., et al. (2016). Trial Watch-Oncolytic Viruses and Cancer Therapy. Oncoimmunology 5 (2), e1117740. doi:10.1080/2162402x.2015.1117740
Qiu, X., Fu, Q., Meng, C., Yu, S., Zhan, Y., Dong, L., et al. (2016). Newcastle Disease Virus V Protein Targets Phosphorylated STAT1 to Block IFN-I Signaling. PloS one 11 (2), e0148560. doi:10.1371/journal.pone.0148560
Raihan, J., Ahmad, U., Yong, Y. K., Eshak, Z., Othman, F., and Ideris, A. (2019). Regression of Solid Breast Tumours in Mice by Newcastle Disease Virus Is Associated with Production of Apoptosis Related-Cytokines. BMC cancer 19 (1), 315. doi:10.1186/s12885-019-5516-5
Raja, J., Ludwig, J. M., Gettinger, S. N., Schalper, K. A., and Kim, H. S. (2018). Oncolytic Virus Immunotherapy: Future Prospects for Oncology. J. Immunother. Cancer 6 (1), 140. doi:10.1186/s40425-018-0458-z
Ravegnini, G., Sammarini, G., Nannini, M., Pantaleo, M. A., Biasco, G., Hrelia, P., et al. (2017). Gastrointestinal Stromal Tumors (GIST): Facing Cell Death between Autophagy and Apoptosis. Autophagy 13 (3), 452–463. doi:10.1080/15548627.2016.1256522
Reale, A., Vitiello, A., Conciatori, V., Parolin, C., Calistri, A., and Palù, G. (2019). Perspectives on Immunotherapy via Oncolytic Viruses. Infect. Agents Cancer 14, 5. doi:10.1186/s13027-018-0218-1
Regel, I., Mayerle, J., and Ujjwal Mukund, M. (2020). Current Strategies and Future Perspectives for Precision Medicine in Pancreatic Cancer. Cancers 12 (4), 1024. doi:10.3390/cancers12041024
Ricca, J. M., Oseledchyk, A., Walther, T., Liu, C., Mangarin, L., Merghoub, T., et al. (2018). Pre-existing Immunity to Oncolytic Virus Potentiates its Immunotherapeutic Efficacy. Mol. Ther. 26 (4), 1008–1019. doi:10.1016/j.ymthe.2018.01.019
Römer-Oberdörfer, A., Mundt, E., Mebatsion, T., Buchholz, U. J., and Mettenleiter, T. C. (1999). Generation of Recombinant Lentogenic Newcastle Disease Virus from cDNA. J. General Virology 80 (Pt 11), 2987–2995. doi:10.1099/0022-1317-80-11-2987
Sánchez-Felipe, L., Villar, E., and Muñoz-Barroso, I. (2014). Entry of Newcastle Disease Virus into the Host Cell: Role of Acidic pH and Endocytosis. Biochimica Biophysica Acta (BBA) - Biomembr. 1838 (1 Pt B), 300–309. doi:10.1016/j.bbamem.2013.08.008
Schild, H., von Hoegen, P., and Schirrmacher, V. (1989). Modification of Tumor Cells by a Low Dose of Newcastle Disease Virus. Cancer Immunol. Immunother 28 (1), 22–28. doi:10.1007/bf00205796
Schirrmacher, V., and Fournier, P. (2014). Multimodal Cancer Therapy Involving Oncolytic Newcastle Disease Virus, Autologous Immune Cells, and Bi-specific Antibodies. Front. Oncol. 4, 224. doi:10.3389/fonc.2014.00224
Schirrmacher, V., and Fournier, P. (2009). Newcastle Disease Virus: a Promising Vector for Viral Therapy, Immune Therapy, and Gene Therapy of Cancer. Methods Mol. Biol. 542, 565–605. doi:10.1007/978-1-59745-561-9_30
Schirrmacher, V., Schlude, C., Weitz, J., and Beckhove, P. (2015). Strong T-Cell Costimulation Can Reactivate Tumor Antigen-specific T Cells in Late-Stage Metastasized Colorectal Carcinoma Patients: Results from a Phase I Clinical Study. Int. J. Oncol. 46 (1), 71–77. doi:10.3892/ijo.2014.2692
Seal, B. S., King, D. J., and Meinersmann, R. J. (2000). Molecular Evolution of the Newcastle Disease Virus Matrix Protein Gene and Phylogenetic Relationships Among the Paramyxoviridae. Virus Res. 66 (1), 1–11. doi:10.1016/s0168-1702(99)00119-7
Shan, P., Tang, B., Xie, S., Zhang, Z., Fan, J., Wei, Z., et al. (2021). NDV‐D90 Inhibits 17β‐estradiol‐mediated Resistance to Apoptosis by Differentially Modulating Classic and Nonclassic Estrogen Receptors in Breast Cancer Cells. J. Cell Biochem. 122 (1), 3–15. doi:10.1002/jcb.28118
Shao, X., Wang, X., Guo, X., Jiang, K., Ye, T., Chen, J., et al. (2019). STAT3 Contributes to Oncolytic Newcastle Disease Virus-Induced Immunogenic Cell Death in Melanoma Cells. Front. Oncol. 9, 436. doi:10.3389/fonc.2019.00436
Sharma, K. K., Kalyani, I. H., Mohapatra, J., Patel, S. D., Patel, D. R., Vihol, P. D., et al. (2017). Evaluation of the Oncolytic Potential of R2B Mukteshwar Vaccine Strain of Newcastle Disease Virus (NDV) in a Colon Cancer Cell Line (SW-620). Arch. Virol. 162 (9), 2705–2713. doi:10.1007/s00705-017-3411-4
Shobana, R., Samal, S. K., and Elankumaran, S. (2013). Prostate-specific Antigen-Retargeted Recombinant Newcastle Disease Virus for Prostate Cancer Virotherapy. J. Virol. 87 (7), 3792–3800. doi:10.1128/JVI.02394-12
Shokeen, K., Srivathsan, A., and Kumar, S. (2021). Lithium Chloride Functions as Newcastle Disease Virus-Induced ER-Stress Modulator and Confers Anti-viral Effect. Virus Res. 292, 198223. doi:10.1016/j.virusres.2020.198223
Sinkovics, J. G., and Horvath, J. C. (2000). Newcastle Disease Virus (NDV): Brief History of its Oncolytic Strains. J. Clin. Virol. 16 (1), 1–15. doi:10.1016/s1386-6532(99)00072-4
Smith, A. G., and Macleod, K. F. (2019). Autophagy, Cancer Stem Cells and Drug Resistance. J. Pathol. 247 (5), 708–718. doi:10.1002/path.5222
Song, D.-Z., Liang, Y., Xiao, Q., Yin, J., Gong, J.-L., Lai, Z.-P., et al. (2007). TRAIL Is Involved in the Tumoricidal Activity of Mouse Natural Killer Cells Stimulated by Newcastle Disease Virusin Vitro. Anat. Rec. 296 (10), 1552–1560. doi:10.1002/ar.22768
Song, H., Zhong, L.-P., He, J., Huang, Y., and Zhao, Y.-X. (2019). Application of Newcastle Disease Virus in the Treatment of Colorectal Cancer. Wjcc 7 (16), 2143–2154. doi:10.12998/wjcc.v7.i16.2143
Song, K. Y., Wong, J., Gonzalez, L., Sheng, G., Zamarin, D., and Fong, Y. (2010). Antitumor Efficacy of Viral Therapy Using Genetically Engineered Newcastle Disease Virus [NDV(F3aa)-GFP] for Peritoneally Disseminated Gastric Cancer. J. Mol. Med. 88 (6), 589–596. doi:10.1007/s00109-010-0605-6
Steiner, H. H., Bonsanto, M. M., Beckhove, P., Brysch, M., Geletneky, K., Ahmadi, R., et al. (2004). Antitumor Vaccination of Patients with Glioblastoma Multiforme: a Pilot Study to Assess Feasibility, Safety, and Clinical Benefit. J. Clin. Oncol. 22 (21), 4272–4281. doi:10.1200/jco.2004.09.038
Stewart, R., Morrow, M., Hammond, S. A., Mulgrew, K., Marcus, D., Poon, E., et al. (2015). Identification and Characterization of MEDI4736, an Antagonistic Anti-PD-L1 Monoclonal Antibody. Cancer Immunol. Res. 3 (9), 1052–1062. doi:10.1158/2326-6066.CIR-14-0191
Stojdl, D. F., Lichty, B., Knowles, S., Marius, R., Atkins, H., Sonenberg, N., et al. (2000). Exploiting Tumor-specific Defects in the Interferon Pathway with a Previously Unknown Oncolytic Virus. Nat. Med. 6 (7), 821–825. doi:10.1038/77558
Su, Z., Yang, Z., Xu, Y., Chen, Y., and Yu, Q. (2015). Apoptosis, Autophagy, Necroptosis, and Cancer Metastasis. Mol. Cancer 14, 48. doi:10.1186/s12943-015-0321-5
Subhash, V. V., Yeo, M. S., Tan, W. L., and Yong, W. P. (2015). Strategies and Advancements in Harnessing the Immune System for Gastric Cancer Immunotherapy. J. Immunol. Res. 2015, 1–14. doi:10.1155/2015/308574
Sui, H., Wang, K., Xie, R., Li, X., Li, K., Bai, Y., et al. (2017). NDV-D90 Suppresses Growth of Gastric Cancer and Cancer-Related Vascularization. Oncotarget 8 (21), 34516–34524. doi:10.18632/oncotarget.16563
Sung, H., Ferlay, J., Siegel, R. L., Laversanne, M., Soerjomataram, I., Jemal, A., et al. (2021). Global Cancer Statistics 2020: GLOBOCAN Estimates of Incidence and Mortality Worldwide for 36 Cancers in 185 Countries. CA A Cancer J. Clin. 71 (3), 209–249. doi:10.3322/caac.21660
Susta, L., Segovia, D., Olivier, T. L., Dimitrov, K. M., Shittu, I., Marcano, V., et al. (2018). Newcastle Disease Virus Infection in Quail. Vet. Pathol. 55 (5), 682–692. doi:10.1177/0300985818767996
Syed Najmuddin, S. U. F., Amin, Z. M., Tan, S. W., Yeap, S. K., Kalyanasundram, J., Veerakumarasivam, A., et al. (2020). Oncolytic Effects of the Recombinant Newcastle Disease Virus, rAF-IL12, against Colon Cancer Cells In Vitro and in Tumor-Challenged NCr-Foxn1nu Nude Mice. PeerJ 8, e9761. doi:10.7717/peerj.9761
Tan, X., Tong, L., Li, L., Xu, J., Xie, S., Ji, L., et al. (2021). Loss of Smad4 Promotes Aggressive Lung Cancer Metastasis by De-repression of PAK3 via miRNA Regulation. Nat. Commun. 12 (1), 4853. doi:10.1038/s41467-021-24898-9
Uder, C., Brückner, S., Winkler, S., Tautenhahn, H.-M., and Christ, B. (2018). Mammalian MSC from Selected Species: Features and Applications. Cytometry 93 (1), 32–49. doi:10.1002/cyto.a.23239
Vannini, A., Leoni, V., and Campadelli-Fiume, G. (2021). Targeted Delivery of IL-12 Adjuvants Immunotherapy by Oncolytic Viruses. Adv. Exp. Med. Biol. 1290, 67–80. doi:10.1007/978-3-030-55617-4_4
Wang, X., Shao, X., Gu, L., Jiang, K., Wang, S., Chen, J., et al. (2020). Targeting STAT3 Enhances NDV‐induced Immunogenic Cell Death in Prostate Cancer Cells. J. Cell Mol. Med. 24 (7), 4286–4297. doi:10.1111/jcmm.15089
Wei, D., Li, Q., Wang, X.-L., Wang, Y., Xu, J., Feng, F., et al. (2015). Oncolytic Newcastle Disease Virus Expressing Chimeric Antibody Enhanced Anti-tumor Efficacy in Orthotopic Hepatoma-Bearing Mice. J. Exp. Clin. Cancer Res. 34, 153. doi:10.1186/s13046-015-0271-1
Wheelock, E. F., and Dingle, J. H. (1964). Observations on the Repeated Administration of Viruses to a Patient with Acute Leukemia. N. Engl. J. Med. 271, 645–651. doi:10.1056/nejm196409242711302
Wilden, H., Fournier, P., Zawatzky, R., and Schirrmacher, V. (2009). Expression of RIG-I, IRF3, IFN-Beta and IRF7 Determines Resistance or Susceptibility of Cells to Infection by Newcastle Disease Virus. Int. J. Oncol. 34 (4), 971–982. doi:10.3892/ijo_00000223
Wong, J., Schulman, A., Kelly, K., Zamarin, D., Palese, P., and Fong, Y. (2010). Detection of Free Peritoneal Cancer Cells in Gastric Cancer Using Cancer-specific Newcastle Disease Virus. J. Gastrointest. Surg. 14 (1), 7–14. doi:10.1007/s11605-009-1071-8
Wu, Y., He, J., An, Y., Wang, X., Liu, Y., Yan, S., et al. (2016). Recombinant Newcastle Disease Virus (NDV/Anh-IL-2) Expressing Human IL-2 as a Potential Candidate for Suppresses Growth of Hepatoma Therapy. J. Pharmacol. Sci. 132 (1), 24–30. doi:10.1016/j.jphs.2016.03.012
Wu, Y., He, J., Geng, J., An, Y., Ye, X., Yan, S., et al. (2017). Recombinant Newcastle Disease Virus Expressing Human TRAIL as a Potential Candidate for Hepatoma Therapy. Eur. J. Pharmacol. 802, 85–92. doi:10.1016/j.ejphar.2017.02.042
Wu, Y., Yan, S., Lv, Z., Chen, L., Geng, J., He, J., et al. (2014). Recombinant Newcastle Disease Virus Anhinga Strain (NDV/Anh-EGFP) for Hepatoma Therapy. Technol. Cancer Res. Treat. 13 (2), 169–175. doi:10.7785/tcrt.2012.500356
Xia, C., Dong, X., Li, H., Cao, M., Sun, D., He, S., et al. (2022). Cancer Statistics in China and United States, 2022: Profiles, Trends, and Determinants. Chin. Med. J. 135 (5), 584–590. doi:10.1097/CM9.0000000000002108
Xiao, S., Nayak, B., Samuel, A., Paldurai, A., Kanabagattebasavarajappa, M., Prajitno, T. Y., et al. (2012). Generation by Reverse Genetics of an Effective, Stable, Live-Attenuated Newcastle Disease Virus Vaccine Based on a Currently Circulating, Highly Virulent Indonesian Strain. PloS one 7 (12), e52751. doi:10.1371/journal.pone.0052751
Xu, X., Zhang, D., Ding, W., Wang, W., Jin, N., and Ding, Z. (2021). NDV Related Exosomes Enhance NDV Replication through Exporting NLRX1 mRNA. Veterinary Microbiol. 260, 109167. doi:10.1016/j.vetmic.2021.109167
Xupeng, B., Jie, N., Julia, B., Wasinger, V. C., Shanping, W., Ying, Z., et al. (2021). Activation of the eIF2α/ATF4 axis Drives Triple-Negative Breast Cancer Radioresistance by Promoting Glutathione Biosynthesis. Redox Biol. 43, 101993. doi:10.1016/j.redox.2021.101993
Yaacov, B., Elihaoo, E., lazar, I., Ben-Shlomo, M., Greenbaum, I., Panet, A., et al. (2008). Selective Oncolytic Effect of an Attenuated Newcastle Disease Virus (NDV-HUJ) in Lung Tumors. Cancer Gene Ther. 15 (12), 795–807. doi:10.1038/cgt.2008.31
Yahya, E. B., and Alqadhi, A. M. (2021). Recent Trends in Cancer Therapy: A Review on the Current State of Gene Delivery. Life Sci. 269, 119087. doi:10.1016/j.lfs.2021.119087
Yan, Y., Jia, L., Zhang, J., Liu, Y., and Bu, X. (2014). Effect of Recombinant Newcastle Disease Virus Transfection on Lung Adenocarcinoma A549 Cells In Vivo. Oncol. Lett. 8 (6), 2569–2576. doi:10.3892/ol.2014.2562
Yan, Y., Liang, B., Zhang, J., Liu, Y., and Bu, X. (2015). Apoptotic Induction of Lung Adenocarcinoma A549 Cells Infected by Recombinant RVG Newcastle Disease Virus (rL-RVG) In Vitro. Mol. Med. Rep. 11 (1), 317–326. doi:10.3892/mmr.2014.2657
Yan, Y., Liu, S., Li, M., Zhao, Y., Shao, X., Hang, M., et al. (2018). Recombinant Newcastle Disease Virus Expressing Human IFN-Λ1 (rL-hIFN-Λ1)-Induced Apoptosis of A549 Cells Is Connected to Endoplasmic Reticulum Stress Pathways. Thorac. Cancer 9 (11), 1437–1452. doi:10.1111/1759-7714.12857
Ye, T., Jiang, K., Wei, L., Barr, M. P., Xu, Q., Zhang, G., et al. (2018). Oncolytic Newcastle Disease Virus Induces Autophagy-dependent Immunogenic Cell Death in Lung Cancer Cells. Am. J. Cancer Res. 8 (8), 1514–1527.
Yurchenko, K. S., Jing, Y., and Shestopalov, A. M. (2019). Adaptation of the Newcastle Disease Virus to Cell Cultures for Enhancing its Oncolytic Properties. Acta Naturae 11 (1), 66–73. doi:10.32607/20758251-2019-11-1-66-73
Yusoff, K., and Tan, W. S. (2001). Newcastle Disease Virus: Macromolecules and Opportunities. Avian Pathol. 30 (5), 439–455. doi:10.1080/03079450120078626
Zamarin, D., Holmgaard, R. B., Subudhi, S. K., Park, J. S., Mansour, M., Palese, P., et al. (2014). Localized Oncolytic Virotherapy Overcomes Systemic Tumor Resistance to Immune Checkpoint Blockade Immunotherapy. Sci. Transl. Med. 6 (226), 226ra32. doi:10.1126/scitranslmed.3008095
Zamarin, D., and Palese, P. (2012). Oncolytic Newcastle Disease Virus for Cancer Therapy: Old Challenges and New Directions. Future Microbiol. 7 (3), 347–367. doi:10.2217/fmb.12.4
Zhan, Y., Yu, S., Yang, S., Qiu, X., Meng, C., Tan, L., et al. (2020). Newcastle Disease Virus Infection Activates PI3K/Akt/mTOR and P38 MAPK/Mnk1 Pathways to Benefit Viral mRNA Translation via Interaction of the Viral NP Protein and Host eIF4E. PLoS Pathog. 16 (6), e1008610. doi:10.1371/journal.ppat.1008610
Zhang, L., Qin, Y., and Chen, M. (2018). Viral Strategies for Triggering and Manipulating Mitophagy. Autophagy 14 (10), 1665–1673. doi:10.1080/15548627.2018.1466014
Zhang, P., Zheng, Z., Ling, L., Yang, X., Zhang, N., Wang, X., et al. (2017). w09, a Novel Autophagy Enhancer, Induces Autophagy-dependent Cell Apoptosis via Activation of the EGFR-Mediated RAS-RAF1-Map2k-Mapk1/3 Pathway. Autophagy 13 (7), 1093–1112. doi:10.1080/15548627.2017.1319039
Zhao, H., Janke, M., Fournier, P., and Schirrmacher, V. (2008). Recombinant Newcastle Disease Virus Expressing Human Interleukin-2 Serves as a Potential Candidate for Tumor Therapy. Virus Res. 136 (1-2), 75–80. doi:10.1016/j.virusres.2008.04.020
Zhao, L., Niu, C., Shi, X., Xu, D., Li, M., Cui, J., et al. (2018). Dendritic Cells Loaded with the Lysate of Tumor Cells Infected with Newcastle Disease Virus Trigger Potent Anti-tumor I-mmunity by P-romoting the S-ecretion of IFN-γ and IL-2 from T C-ells. Oncol. Lett. 16 (1), 1180–1188. doi:10.3892/ol.2018.8785
Zhao, Q., Cao, L., Guan, L., Bie, L., Wang, S., Xie, B., et al. (2019). Immunotherapy for Gastric Cancer: Dilemmas and Prospect. Briefings Funct. genomics 18 (2), 107–112. doi:10.1093/bfgp/ely019
Zhen, S., and Li, X. (2019). Application of CRISPR-Cas9 for Long Noncoding RNA Genes in Cancer Research. Hum. Gene Ther. 30 (1), 3–9. doi:10.1089/hum.2018.063
Keywords: Newcastle disease virus, oncolytic virotherapy, tumor, apoptosis, antitumor immunity
Citation: Huang F, Dai C, Zhang Y, Zhao Y, Wang Y and Ru G (2022) Development of Molecular Mechanisms and Their Application on Oncolytic Newcastle Disease Virus in Cancer Therapy. Front. Mol. Biosci. 9:889403. doi: 10.3389/fmolb.2022.889403
Received: 04 March 2022; Accepted: 10 May 2022;
Published: 04 July 2022.
Edited by:
Ahmed Majeed Al-Shammari, Mustansiriyah University, IraqReviewed by:
Mohsen Keshavarz, Bushehr University of Medical Sciences, IranDi Yu, Uppsala University, Sweden
Rajesh Kumar, National Institutes of Health (NIH), United States
Copyright © 2022 Huang, Dai, Zhang, Zhao, Wang and Ru. This is an open-access article distributed under the terms of the Creative Commons Attribution License (CC BY). The use, distribution or reproduction in other forums is permitted, provided the original author(s) and the copyright owner(s) are credited and that the original publication in this journal is cited, in accordance with accepted academic practice. No use, distribution or reproduction is permitted which does not comply with these terms.
*Correspondence: Yigang Wang, d2FuZ3lpZ2FuZzQzQDE2My5jb20=; Guoqing Ru, cmdxcTExMUAxNjMuY29t
†These authors have contributed equally to this work