- 1Department of Cell, Developmental and Cancer Biology, Oregon Health and Science University, Portland, OR, United States
- 2Center for Experimental Therapeutics, Knight Cancer Institute, Oregon Health and Science University, Portland, OR, United States
- 3High-Throughput Screening Services Laboratory, College of Pharmacy, Oregon State University, Corvallis, OR, United States
- 4Department of Radiation Medicine, Oregon Health and Science University, Portland, OR, United States
Enhancing the immune microenvironment in cancer by targeting the nucleic acid sensors is becoming a potent therapeutic strategy. Among the nucleic acid sensors, activation of the RNA sensor Retinoic Acid-inducible Gene (RIG-I) using small hairpin RNAs has been shown to elicit powerful innate and adaptive immune responses. Given the challenges inherent in pharmacokinetics and delivery of RNA based agonists, we set out to discover small molecule agonists of RIG-I using a cell-based assay. To this end, we established and validated a robust high throughput screening assay based on a commercially available HEK293 reporter cell line with a luciferase reporter downstream of tandem interferon stimulated gene 54 (ISG54) promoter elements. We first confirmed that the luminescence in this cell line is dependent on RIG-I and the interferon receptor using a hairpin RNA RIG-I agonist. We established a 96-well and a 384-well format HTS based on this cell line and performed a proof-of-concept screen using an FDA approved drug library of 1,200 compounds. Surprisingly, we found two HDAC inhibitors Entinostat, Mocetinostat and the PLK1 inhibitor Volasertib significantly enhanced ISG-luciferase activity. This luminescence was substantially diminished in the null reporter cell line indicating the increase in signaling was dependent on RIG-I expression. Combination treatment of tumor cell lines with Entinostat increased RIG-I induced cell death in a mammary carcinoma cell line that is resistant to either Entinostat or RIG-I agonist alone. Taken together, our data indicates an unexpected role for HDAC1,-3 inhibitors in enhancing RIG-I signaling and highlight potential opportunities for therapeutic combinations.
Introduction
Mammalian cells have mechanisms that activate the innate immune response to provide immediate defense upon infection. Infections are recognized by pattern recognition receptors (PRRs), which identify pathogen-associated molecular patterns (PAMPs) (Tan et al., 2018). PRRs that identify pathogen DNA or RNA in the cytoplasm are known as cytosolic nucleic sensors. One of the most important PRRs for viral infections is the retinoic acid-inducible gene I (RIG-I). RIG-I recognizes short double stranded RNA (dsRNA) with di or tri-phosphate group at the 5′end (Schlee et al., 2009). RIG-I, encoded by DDX58 (DEADbox helicase 58 gene) in humans, has two caspase activation and recruitment domains (CARDs) in the N-terminus, a central helicase domain (HD) and C-terminal domain (CTD) (Yoneyama et al., 2004). Under normal conditions, CARDs are bound to the HD in an auto-repress conformation. If viral RNA is detected, RIG-I dimerizes, and CARDs are released to bind with the CARDs from the mitochondrial activator of virus signaling (MAVS) protein. RIG-I and MAVS interaction triggers activation of the interferon regulatory factor (IRF) 3 and NF-κB. Activated IRF3 and NF-κB translocate to the nucleus to activate the expression of type 1 interferon (IFN-I) and subsequently, proinflammatory and anti-viral genes. IFN-I acts in an auto and paracrine manner activating a number of ISGs, whose products regulate cell growth, metabolism and immune response (Kell and Gale, 2015).
Several studies have identified synthetic RIG-I agonists which trigger IFN-I response for use as vaccine adjuvants, to treat viral infection or to enhance the immunogenicity in cold tumors (Elion and Cook, 2018; Yong and Luo, 2018). In fact, RIG-I agonists are showing promising results in pre-clinical and clinical trials. For instance, RNA loop SLR14 improved the effects of anti-PD1 in an MC-38 tumor model (Jiang et al., 2019); and MK-4621 is showing promising results in clinical trials in solid tumors (NCT03065023 and NCT03739138). However, delivery challenges as well as pharmacokinetics of synthetic RNA agonists argue for the development of small molecule agonists to target RIG-I. Thus, we decided to develop a cell-based high throughput screen (HTS) assay. Here we have demonstrated that it is feasible to identify small molecule agonists of RIG-I using this assay and discovered unexpected crosstalk between HDAC, PLK1 signaling and the RIG-I pathway.
Results
An ISG-Luciferase Reporter Cell Line is a Sensitive Tool to Study RIG-I Activation In Vitro
HEK-Lucia™ RIG-I (Invivogen) cells have been engineered with the DDX58 (RIG-I human gene) and a secreted Lucia luciferase gene under the ISG54 promoter. We first established that the luciferase signal in the cell lysates were equivalent to the secreted luciferase signal in the supernatants and therefore decided to assay the cell lysates to minimize liquid handling (data not shown). We found that RIG-I activation by recognition of 3p-hpRNA (RIG-I agonist) triggers IFN-I response, which activates luciferase expression (Supplementary Figure S1A). In contrast HEK-Lucia™ Null cells which bear the same ISG54 responsive luciferase gene but lack DDX58 had significantly decreased luminescence upon RIG-I agonist treatment but retained equivalent responsiveness to IFN-β treatment (Supplementary Figure S1B). Importantly, addition of an IFNAR inhibitor decreased the luminescence induced by the RIG-I agonist suggesting the reporter activity is dependent on IFN signaling (Supplementary Figure S1C). To optimize the RIG-I activity assay, we performed dose-response experiments in 96 well plates following the manufacturer’s indications (approximately 5 × 105 cell/well) at 24 and 48 h. We observed a robust dose dependent increase in luciferase signal with the RIG-I agonist (Figure 1) Z′ values were 0.68 for 24 h (Figure 1A) and 0.88 for 48 h (Figure 1B) at 0.3 µg/ml of RIG-I or control agonist. We optimized cell number and kinetics and found that at 6h, luciferase expression was detected from 5,000 cells/well (Figure 1C); while at 48 h, only 500 cells/well were needed to detect luminescence (Figure 1B). Our data indicate that this 3p-hpRNA is a potent RIG-I activator, and the HEK-Lucia™ RIG-I as a sensitive tool to test RIG-I activation in vitro.
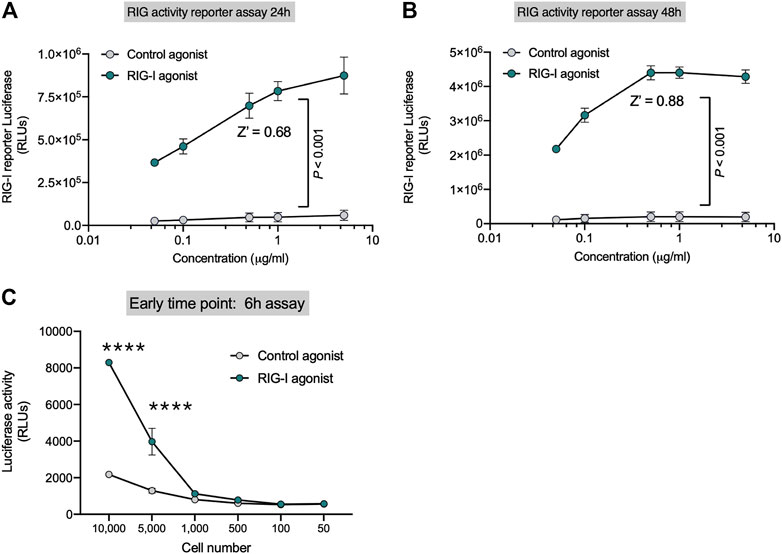
FIGURE 1. Sensitive Reporter Assay for measuring RIG-I activity. HEK293 RIG-I cells were seeded onto 96-well plates and treated with the indicated concentrations of a control agonist or RIG-I agonist. Luciferase activity was measured in supernatants as per manufacturer’s recommendations at (A) 24 h and (B) 48 h. (C) Sensitivity of the RIG-I activation was evaluated with different cell numbers as indicated at an early time point–6 h post treatment, with a control agonist or the RIG-I agonist (0.3 μg/ml). p value from a two-way ANOVA with post-hoc Sidak’s correction or unpaired two-tailed Student’s t-test.
High Throughput Screening Identifies RIG-I Activity in an FDA Approved Drug Library
To find new compounds that could activate RIG-I, we carried out a HTS using an FDA approved drug library. This library included 1,430 compounds in water or DMSO, with well characterized bioactivity, bioavailability, and safety profiles. We confirmed that the assay yielded robust signal in 384-well plates and tolerated DMSO with no decrease in luminescence till 5% DMSO (Figure 2A). We tested the FDA library compounds in 8-point 1/3 Log10 dose curves from 10 mM to 3 µM over several days of independent runs. Results indicated that our assay worked robustly with an average Z′ of 0.57 (Figure 2B), good signal to background noise difference (S/B) above 65 (Figure 2C). We defined a ‘hit’ as any compound with luminescence of at least 5 SDs above the average background of DMSO treated wells. We found that 12 compounds in the FDA library achieved this threshold in our HTS (Figure 2D).
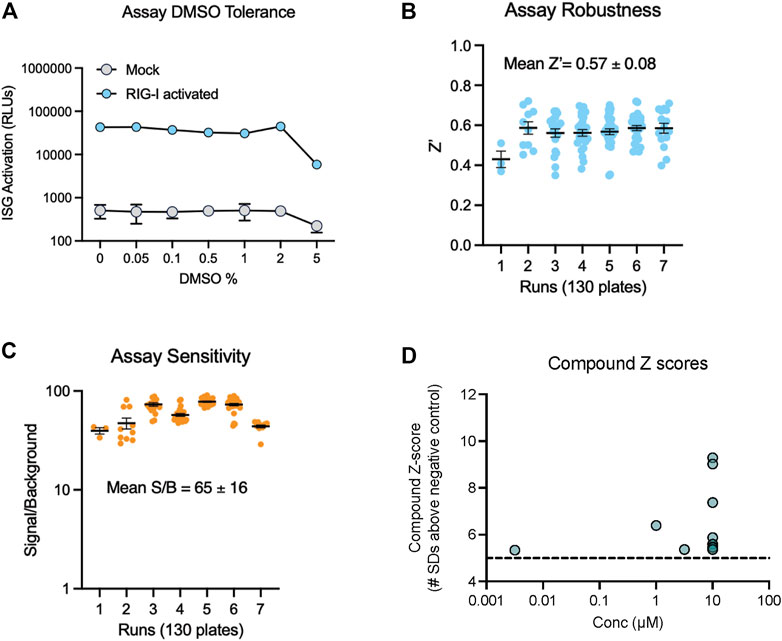
FIGURE 2. Miniaturization and validation of RIG activity HTS assay in 384 well plate. (A) DMSO tolerance of HEK293 RIG-I cell line as measured by luciferase activation with positive control RIG-I agonist (B,C) Luciferase activity was measured in cell lysates as per manufacturer’s recommendations at 24 h post treatment with three compound libraries across 8 doses- the FDA approved drug collection, a LOPAC collection and a collection of natural product derived compounds from Microsource. Screening data from 7 independent runs totaling 130 plates show (B) high Z′ values, (C) good signal to noise ratios. (D) Compound Z-scores of hits (at any concentration) in the FDA library defined as number of standard deviations (SDs) above the mean of negative control wells.
Interestingly, we found three compounds-2 HDAC inhibitors- Entinostat, Mocetinostat and the PLK1 inhibitor Volasertib increased the ISG activation signal at more than one dose (Figures 3A–C). IFN-I response can be activated by different pathways in the cells. In fact, HEK-Lucia™ RIG-I and HEK-Lucia™ Null cells endogenously express NOD1, TLR3 and TLR5. However, our data showed that HEK-Lucia™ RIG-I cells do not respond to LPS or Poly I:C across a range of concentrations (Supplementary Figure S2). To study the RIG-I specificity of these compounds, we assayed them in HEK-Lucia™ Null cells for responses across 4-log doses. We found that for the HDAC inhibitors and PLKi, and to a lesser extent the JAK inhibitor Fediratinib, the luminescence was dependent on expression of RIG-I in the cells (Figure 3D). Independent dose response experiments confirmed that the activity of Entinostat, Mocetinostat and Volasertib was specific to the RIG-I expressing but not null cells (Figures 3E–G). Notably, higher concentrations of Volasertib and to a lesser extent Mocetinostat, enhanced cell death leading to a loss of signal (Figures 3F,G).
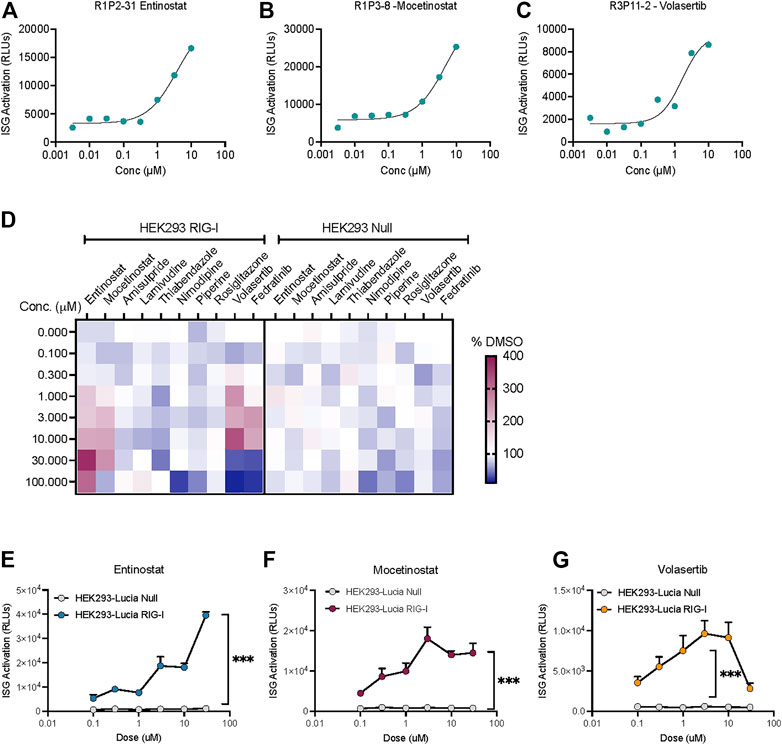
FIGURE 3. HDAC inhibitors and a PLK inhibitor increase ISG activation in a RIG-I dependent manner. Dose response curves showing low μM activation of ISG-luciferase by (A,B) HDAC inhibitors Entinostat and Mocetinostat and (C) PLK1 inhibitor volasertib. (D) Heatmap depicts % change vs DMSO treatment for top 10 hits in the same cell line. (E–G) Comparison of dose dependent ISG-Luciferase activation by the drugs in Null vs RIG-I expressing reporter cell lines. ***p < 0.001 by 2-way ANOVA with Sidak’s post-hoc correction.
Entinostat Enhances RIG-I Signaling in a Resistant Tumor Cell Line In Vitro
Since we established that these compounds activate RIG-I signaling in HEK293 cells, we next evaluated their ability to drive cell death in tumor cell lines. Both HDAC inhibitors as well as the PLK inhibitor are approved drugs with potent anti-tumor activity in a range of cancers. To evaluate whether these inhibitors can confer additive or even synergistic effects with RIG-I agonists, we chose the 4T1 mammary carcinoma cells which showed resistance to both RIG-I agonist induced cell death (Figure 4A) and Entinostat induced cell death (Figure 4B). We found that combination of RIG-I agonist with Entinostat increased apoptosis compared more significantly to either RIG-I alone or the drug alone (Figure 4C). We chose the dose of Entinostat that activated RIG-I maximally (Figure 3D) but did not induce significant cell death (Figure 4B). While the mechanisms by which Entinostat increases RIG-I cell death remains to be determined, we observed two bona fide type I interferon response genes, Mx1 and Usp18 were significantly induced by Entinostat treatment (Figure 4D).
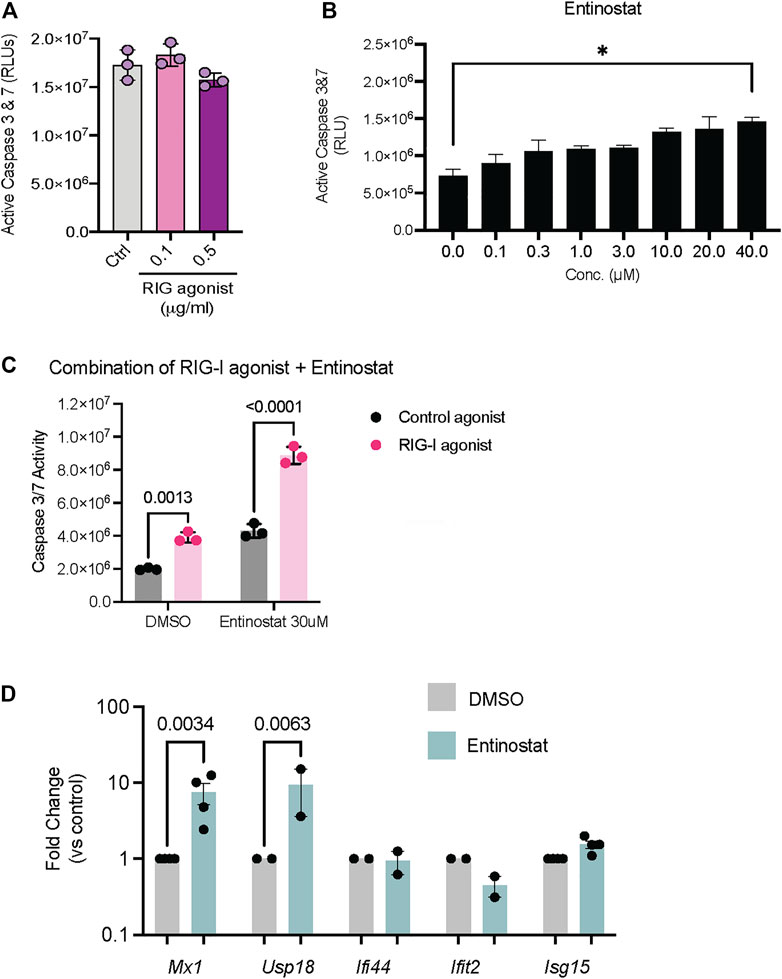
FIGURE 4. Activation of RIG-I signaling in tumor cell lines in response to HDAC and PLK inhibitors. (A) 4T1 mammary carcinoma cells were treated with RIG-I agonist alone or (B) Entinostat alone or (C) RIG-I agonist at 0.5 ug/ml followed by Entinostat at the indicated concentration. 24 h later cell death was measured by a CaspaseGlo assay. 4T1 mammary carcinoma cells were treated with DMSO, Entinostat (30 uM). qRT-PCR for indicated genes is shown. p values from a two-way ANOVA with a post-hoc Sidak’s test (A–C) or an uncorrected Fisher’s LSD test (D).
Taken together, our data indicates that a cell-based screening assay can function robustly to perform high throughput screening for RIG-I agonists. We surprisingly observed that RIG-I signaling can be activated by HDAC inhibitors and a PLK1 inhibitor and showcase a potential utility in driving cell death in tumors that maybe resistant to RIG-I or HDAC inhibition monotherapies.
Discussion
RIG-I is one of the most important defenses against viral infection in cells functioning as a PRR, but emerging data suggests broader roles in cancer. Previous studies showed RIG-I as tumor suppressor in different cancer types such as hepatocellular carcinoma and glioblastoma multiforme (Nguyen et al., 2016; Bufalieri et al., 2020). Several studies have shown that RIG-I activation is a potent driver of immune responses in cancer (Jacobson et al., 2018; Lurescia et al., 2018; Helms et al., 2019; Jiang et al., 2019). While RNA agonists have shown remarkable activity in preclinical models and a phase I clinical trial, there are still challenges in delivering RNA to solid tumors in humans over longer durations of treatment (Iurescia et al., 2020). Therefore, we set out to establish a screening assay for the discovery of small molecules that can activate RIG-I in a selective manner.
Our data indicates that this cell-based assay is robust and highly sensitive to identify compounds that activate RIG-I dependent ISG signaling (Figures 1, 2). We also serendipitously discovered that HDAC inhibition and PLK inhibition can also activate RIG-I signaling (Figure 3). Observations from independent experiments using our RIG-I null cell lines (Figure 3D), the IFNAR inhibitors (Supplementary Figure S1C) and the TLR ligand treatment experiments (Supplementary Figure S2) suggest that the luminescence in our assay is 1) directly dependent on the ectopic expression of RIG-I 2) is type I interferon dependent and 3) is not driven by other typical RNA sensors in cells. We also show that the ability of HDAC inhibitors and PLK inhibitor to drive RIG-I signaling holds true in tumor cell lines in vitro. Interestingly the HDAC inhibitor Entinostat confers an additive benefit in driving cell death in combination with RIG-I activation (Figure 4C) in a mouse mammary carcinoma cell line that is typically insensitive to RIG-I induced cell death.
While further in-depth studies are needed to validate the cross-talk between the RIG-I and HDAC/PLK pathways in tumor cells, we propose a few hypotheses on how these pathways interact. There is evidence that HDAC3 inhibition can increase transcription of type I interferons (Angiolilli et al., 2017). Interestingly, it is thought that HDAC inhibition can also enhance the transcription of endogenous retroviral elements and other non-coding RNAs both in the human and mouse genome (Daskalakis et al., 2018). Given the role of RIG-I in sensing RNA, it is possible that these endogenous RNAs can activate RIG-I. RIG-I is also regulated by acetylation at multiple sites. There is evidence that HDAC6 interacts with and acetylates RIG-I (Choi et al., 2016; Liu et al., 2016). Similarly, it has been reported that PLK1 can directly bind and phosphorylate MAVS leading to an increase in type I interferon signaling (Vitour et al., 2009). Based on our data, we suspect similar mechanisms are contributing to how HDAC inhibition or PLK inhibition can enhance RIG-I signaling.
Beyond the biological basis for how these important pathways engage and interact, there is also a potential clinical utility for our findings. Although HDAC inhibitors and PLK1 inhibitors have been extensively studied, their proposed mechanism(s) of action have been tumor centric (Gutteridge et al., 2016; Eckschlager et al., 2017). However, recent evidence suggests that HDAC inhibition may have roles in the tumor microenvironment (Li et al., 2021). For instance, it has been shown that HDAC inhibition potentiates immunotherapy responses in CT26 and MC38 tumor models (Bissonnette et al., 2021). Our observations introduce the possibility that HDAC inhibition also contribute type I interferons to activate the immune microenvironment.
In summary, we have described a new, highly sensitive cell-based assay to discover small molecule agonists of RIG-I signaling. Using this assay we have discovered that HDAC inhibitors can activate RIG-I signaling in tumor cells and can enhance tumor cell death in combination with RIG-I agonists. We anticipate further work will elucidate both the biological mechanisms and the functional significance of HDACs - RIG-I interaction.
Materials and Methods
Cell Culture
RIG-I reporter cell line HEK-Lucia™ RIG-I (Invivogen, #hkl-hrigi) was cultured in Dulbecco’s Modified Eagles Medium (DMEM), High Glucose (HyClone™) supplemented with 10% Fetal Bovine Serum (FBS) (Bio-Techne R&D Systems #S11550H), 30 µg/ml blasticidin, 100 µg/ml.
Normocin™ and 100 µg/ml of Zeocin™. HEK-Lucia™ Null cells (Invivogen, #hkl-null) were used as control and cultured in the same media with neither blasticidin nor Zeocin™. MC-38 (Kerafast #ENH204-FP) and CT-26 (ATCC #CRL-2638) 4T1 cells were cultured in DMEM or RPMI 1640 with L-Glutamine (Lonza™ BioWhittaker™ # BW12115F12) supplemented with 10% of FBS. Cell lines were routinely tested and found to be Mycoplasma free. Cell line identities were genetically validated through Cell Line Authentication Service at OHSU Genomics Core Facility.
RIG-I Activity Assay
HEK-Lucia™ RIG-I or Null cells were transferred into antibiotic free media (DMEM supplemented with 10% FBS) and seeded in 96 or 384 well plate at 70–85% confluence. RIG-I agonist (Invivogen #tlrl-hprna) or control agonist (Invivogen #tlrl-3prnac) were transfected with LyoVec™ (Invivogen #lyec-12) following the manufacturer´s instructions. Cells were incubated for 6–48 h at 37°C and 5% of CO2. QUANTI-Luc™ Gold (Invivogen #rep-qlcg-500) was dissolved in sterile water and added directly into the wells. Luminescence was immediately measure in Spark® Multimode Microplate Reader (Tecan) set at 0.1 s of reading time.
RNA Extraction, RT-PCR and Gene Expression
Genomic RNA from cells and homogenized tumors was extracted using the GeneMATRIX Universal RNA Purification Kit (EuRx #E3598) following the manufacturer’s instruction. Samples were treated with DNAse I (Invitrogen™ #18068015) to avoid DNA contamination. Reverse transcription was performed with 500 ng of RNA using the High-Capacity cDNA Reverse Transcription Kit (Cat: 4368814, Applied Biosystems). Gene expression was assayed by quantitative PCR using TaqManTM Master Mix II no UNG (Cat 4440049, Thermofisher Scientific) with the following probes: Ddx58 (Mm01216853_m1), Mx1 (Mm00487796_m1), Isg15 (Mm01705338_s1), Usp18 (Mm01188805_m1), Ifit2 (Mm00492606_m1), Ifi44 (Mm00505670_m1), Gapdh (Mm99999915_g1). Data was normalized to internal control Gapdh and gene expression quantified using the using the 2–ΔΔCt method with the control treatment as reference.
Cell Titer-Glo/Caspase Glo
Cells were seeded in white 96 well plates (Greiner Bio-One™ CellStar™) and treated for 24 h. Cell Titer-Glo (Promega, #G7572) and Caspase 3/7 Glo (Promega #G8091) were added and assayed according to the manufacturer´s instructions. Luminescence was measure using either a Promega GloMax, BioTex Synergy 4 or Tecan Spark reader.
High-Throughput Screening
Reporter cells were seeded in cell culture 384 well plates (Greiner, #781080) at 1.5 × 10 (Kell and Gale, 2015) cells/well in 50 µl of DMEM supplemented with 10% FBS using BioTek™ MultiFlo™ FX Microplate Dispensers (BioTek Instruments). Cells were treated with an FDA approved library (Selleckchem) using a Sciclone ALH3000 Liquid Handler (PerkinElmer). Specific inhibitors were purchased from Selleckchem, Microsource or LOPAC collection as indicated by the catalog numbers - Entinostat (S1053), Mocetinostat (S1122), Lamivudine (S1706), Amisulpride (M01502285), Nimodipine (N149), Piperine (M01500873), Rosiglitazone (M01504263), Volasertib (S2235) and Fedratinib (S2736). Each compound was assayed across 8 doses and incubated for 24 h. Using the Matrix WellMate dispenser (Thermo Scientific), 30 µl of QUANTI-Luc™ Gold was added. Immediately, luminescence was measured at 0.1 s of reading time. Results were analyzed using Dotmatics software.
For each plate, the Z’ factor was calculated as 1- (3 x SD positive control + 3 x SD of negative control)/|Mean of positive control - Mean of negative control). The Signal to Background ratios were calculated for each plate based on the mean signal from positive control wells over the mean signal of all the negative control wells.
Statistics
All statistical analysis was performed using Prism software (GraphPad Software, San Diego, CA). Differences between pairs of groups were analyzed by Student’s t-test. Comparison among multiple groups was performed by one-way ANOVA followed by a post hoc test (Tukey’s or Holm-Sidak). In the absence of multiple comparisons, Fisher’s LSD test was used. Values of n refer to the number of experiments used to obtain each value. For experiments where the data was not normally distributed, we used the Kruskal-Wallis test. Values of p ≤ 0.05 were considered significant.
Data Availability Statement
The original contributions presented in the study are included in the article/Supplementary Material, further inquiries can be directed to the corresponding author.
Author Contributions
EF-B, MF, DN, SM, and SA designed experiments, EF-B and MF performed experiments, EF-B, MF, DN, SM, and SA analyzed the data, EF-B and SA wrote the manuscript. All authors reviewed and edited the manuscript.
Funding
This work was supported by funding from NHLBI to SA (R01 HL137779 and R01 HL143803). Funder had no role in the execution of these studies or data analysis/interpretation or writing of the manuscript.
Conflict of Interest
EF-B, MF, SM, and SA are named inventors on a patent application based on some of the findings described in this manuscript.
Publisher’s Note
All claims expressed in this article are solely those of the authors and do not necessarily represent those of their affiliated organizations, or those of the publisher, the editors and the reviewers. Any product that may be evaluated in this article, orclaim that may be made by its manufacturer, is not guaranteed or endorsed by the publisher.
Acknowledgments
We thank Sokchea Khou, Rebecca Ruhl, Adrian Baris for technical help and members of the Anand lab and Malhotra lab for useful discussions. Schematic in Supplementary Figure S1 was created with BioRender.com.
Supplementary Material
The Supplementary Material for this article can be found online at: https://www.frontiersin.org/articles/10.3389/fmolb.2022.837610/full#supplementary-material
References
Angiolilli, C., Kabala, P. A., Grabiec, A. M., Van Baarsen, I. M., Ferguson, B. S., García, S., et al. (2017). Histone Deacetylase 3 Regulates the Inflammatory Gene Expression Programme of Rheumatoid Arthritis Fibroblast-like Synoviocytes. Ann. Rheum. Dis. 76, 277–285. doi:10.1136/annrheumdis-2015-209064
Bissonnette, R. P., Cesario, R. M., Goodenow, B., Shojaei, F., and Gillings, M. (2021). The Epigenetic Immunomodulator, HBI-8000, Enhances the Response and Reverses Resistance to Checkpoint Inhibitors. BMC Cancer 21, 969. doi:10.1186/s12885-021-08702-x
Bufalieri, F., Caimano, M., Lospinoso Severini, L., Basili, I., Paglia, F., Sampirisi, L., et al. (2020). The RNA-Binding Ubiquitin Ligase MEX3A Affects Glioblastoma Tumorigenesis by Inducing Ubiquitylation and Degradation of RIG-I. Cancers 12, 321. doi:10.3390/cancers12020321
Choi, S. J., Lee, H. C., Kim, J. H., Park, S. Y., Kim, T. H., Lee, W. K., et al. (2016). HDAC 6 Regulates Cellular Viral RNA Sensing by Deacetylation of RIG ‐I. EMBO J. 35, 429–442. doi:10.15252/embj.201592586
Daskalakis, M., Brocks, D., Sheng, Y.-H., Islam, M. S., Ressnerova, A., Assenov, Y., et al. (2018). Reactivation of Endogenous Retroviral Elements via Treatment with DNMT- and HDAC-Inhibitors. Cell Cycle 17, 811–822. doi:10.1080/15384101.2018.1442623
Eckschlager, T., Plch, J., Stiborova, M., and Hrabeta, J. (2017). Histone Deacetylase Inhibitors as Anticancer Drugs. Int. J. Mol. Sci. 18. doi:10.3390/ijms18071414
Elion, D. L., and Cook, R. S. (2018). Harnessing RIG-I and Intrinsic Immunity in the Tumor Microenvironment for Therapeutic Cancer Treatment. Oncotarget 9, 29007–29017. doi:10.18632/oncotarget.25626
Gutteridge, R. E. A., Ndiaye, M. A., Liu, X., and Ahmad, N. (2016). Plk1 Inhibitors in Cancer Therapy: From Laboratory to Clinics. Mol. Cancer Ther. 15, 1427–1435. doi:10.1158/1535-7163.mct-15-0897
Helms, M. W., Jahn-Hofmann, K., Gnerlich, F., Metz-Weidmann, C., Braun, M., Dietert, G., et al. (2019). Utility of the RIG-I Agonist Triphosphate RNA for Melanoma Therapy. Mol. Cancer Ther. 18, 2343–2356. doi:10.1158/1535-7163.mct-18-1262
Iurescia, S., Fioretti, D., and Rinaldi, M. (2020). The Innate Immune Signalling Pathways: Turning RIG-I Sensor Activation against Cancer. Cancers (Basel) 12, 1–26. doi:10.3390/cancers12113158
Jacobson, M. E., Wang-Bishop, L., Becker, K. W., and Wilson, J. T. (2018). Delivery of 5'-triphosphate RNA with Endosomolytic Nanoparticles Potently Activates RIG-I to Improve Cancer Immunotherapy. Biomater. Sci. 7, 547–559. doi:10.1039/c8bm01064a
Jiang, X., Muthusamy, V., Fedorova, O., Kong, Y., Kim, D. J., Bosenberg, M., et al. (2019). Intratumoral Delivery of RIG-I Agonist SLR14 Induces Robust Antitumor Responses. J. Exp. Med. 216, 2854–2868. doi:10.1084/jem.20190801
Kell, A. M., and Gale, M. (2015). RIG-I in RNA Virus Recognition. Virology 479-480, 110–121. doi:10.1016/j.virol.2015.02.017
Li, X., Dai, H., and Wang, H. (2021). Low-dose HDACi Potentiates Anti-tumor Activity of Macrophages in Immunotherapy. Oncoimmunology 10, 1935668. doi:10.1080/2162402x.2021.1935668
Liu, H. M., Jiang, F., Loo, Y. M., Hsu, S., Hsiang, T.-Y., Marcotrigiano, J., et al. (2016). Regulation of Retinoic Acid Inducible Gene-I (RIG-I) Activation by the Histone Deacetylase 6. EBioMedicine 9, 195–206. doi:10.1016/j.ebiom.2016.06.015
Lurescia, S., Fioretti, D., and Rinaldi, M. (2018). Targeting Cytosolic Nucleic Acid-Sensing Pathways for Cancer Immunotherapies. Front. Immunol. 9, 711.
Nguyen, N. T. H., Now, H., Kim, W.-J., Kim, N., and Yoo, J.-Y. (2016). Ubiquitin-like Modifier FAT10 Attenuates RIG-I Mediated Antiviral Signaling by Segregating Activated RIG-I from its Signaling Platform. Sci. Rep. 6, 23377. doi:10.1038/srep23377
Schlee, M., Roth, A., Hornung, V., Hagmann, C. A., Wimmenauer, V., Barchet, W., et al. (2009). Recognition of 5′ Triphosphate by RIG-I Helicase Requires Short Blunt Double-Stranded RNA as Contained in Panhandle of Negative-Strand Virus. Immunity 31, 25–34. doi:10.1016/j.immuni.2009.05.008
Tan, X., Sun, L., Chen, J., and Chen, Z. J. (2018). Detection of Microbial Infections through Innate Immune Sensing of Nucleic Acids. Annu. Rev. Microbiol. 72, 447–478. doi:10.1146/annurev-micro-102215-095605
Vitour, D., Dabo, S., Ahmadi Pour, M., Vilasco, M., Vidalain, P.-O., Jacob, Y., et al. (2009). Polo-like Kinase 1 (PLK1) Regulates Interferon (IFN) Induction by MAVS. J. Biol. Chem. 284, 21797–21809. doi:10.1074/jbc.m109.018275
Yoneyama, M., Kikuchi, M., Natsukawa, T., Shinobu, N., Imaizumi, T., Miyagishi, M., et al. (2004). The RNA Helicase RIG-I Has an Essential Function in Double-Stranded RNA-Induced Innate Antiviral Responses. Nat. Immunol. 5, 730–737. doi:10.1038/ni1087
Keywords: RIG-1, HDAC, cancer biology, high throughput screen, cell-based screening assay
Citation: Fraile-Bethencourt E, Foss MH, Nelson D, Malhotra SV and Anand S (2022) A Cell-Based Screen Identifies HDAC Inhibitors as Activators of RIG-I Signaling. Front. Mol. Biosci. 9:837610. doi: 10.3389/fmolb.2022.837610
Received: 16 December 2021; Accepted: 27 January 2022;
Published: 14 February 2022.
Edited by:
Prasun K. Datta, Tulane University, United StatesReviewed by:
Valentyn Oksenych, University of Oslo, NorwaySubodh Samrat, University of Arizona, United States
Copyright © 2022 Fraile-Bethencourt, Foss, Nelson, Malhotra and Anand. This is an open-access article distributed under the terms of the Creative Commons Attribution License (CC BY). The use, distribution or reproduction in other forums is permitted, provided the original author(s) and the copyright owner(s) are credited and that the original publication in this journal is cited, in accordance with accepted academic practice. No use, distribution or reproduction is permitted which does not comply with these terms.
*Correspondence: Sudarshan Anand, YW5hbmRzQG9oc3UuZWR1