- 1Department of Urology, National Clinical Research Center for Geriatrics, Institute of Urology, West China Hospital of Sichuan University, Chengdu, China
- 2Center of Biomedical Big Data, West China Hospital, Sichuan University, Chengdu, China
- 3Department of Endocrinology and Metabolism, West China Hospital of Sichuan University, Chengdu, China
The chemokine CXCL8 has been found to play an important role in tumor progression in recent years. CXCL8 activates multiple intracellular signaling pathways by binding to its receptors (CXCR1/2), and plays dual pro-tumorigenic roles in the tumor microenvironment (TME) including directly promoting tumor survival and affecting components of TME to indirectly facilitate tumor progression, which include facilitating tumor cell proliferation and epithelial-to-mesenchymal transition (EMT), pro-angiogenesis, and inhibit anti-tumor immunity. More recently, clinical trials indicate that CXCL8 can act as an independently predictive biomarker in patients receiving immune checkpoint inhibitions (ICIs) therapy. Preclinical studies also suggest that combined CXCL8 blockade and ICIs therapy can enhance the anti-tumor efficacy, and several clinical trials are being conducted to evaluate this therapy modality.
Introduction
The chemokine CXCL8, also known as interleukin-8 (IL-8), is initially known as a cytokine expressed by epithelial cells and macrophages for neutrophil recruitment to areas of inflammation, infection, or injury (Horn et al., 2020a). The biological effects of CXCL8 are mediated through its binding to two cell-surface G-protein-coupled receptors: CXCR1 and CXCR2, which are generally expressed on monocytes, granulocytes, and endothelial cells (Waugh and Wilson, 2008; Liu et al., 2016). Furthermore, CXCL8 monomer binds CXCR1 with high affinity, however, both monomer and dimer show similar affinities to CXCR2 (Helen et al., 2017).
Although CXCL8 has been originally described as a proinflammatory chemokine, in the context of cancer, CXCL8 is produced by multiple cell types in the tumor microenvironment (TME), including the infiltrating immune cells, stromal cells, and the tumour cells (Waugh and Wilson, 2008; Alfaro et al., 2017). Additionally, the mechanism of CXCL8-CXCR1/2 pathway in tumourigenesis, tumour progression and immune suppression in TME has been explored extensively. Recent investigations demonstrate several novel mechanisms of the crosstalk between CXCL8 and components in TME to facilitate tumor progression, even forming positive feedback loops. Immune checkpoint inhibitions (ICIs) have become the cornerstone of immunotherapy in many types of cancers. Emerging trials underline the crucial roles of CXCL8 in ICIs therapy.
In this review, we summarized the current understanding of CXCL8 signaling cascades and recently developed mechanisms of facilitating tumor survival, invasion, and immune suppression. Additionally, we discussed the CXCL8 as a biomarker of ICIs therapy and the role of anti-CXCL8 as a combination agent in immunotherapy.
Structure and Secretion of CXCL8
CXCL8 is initially translated as a protein with 99 amino acids, which is subsequently processed into two active isoforms: 1) 72 amino acids in monocytes and macrophages; 2) 77 amino acids in non-immune cells (Waugh and Wilson, 2008). According to the position of the first two cysteine residues on the N-terminus, chemokines can be divided into four highly conserved subtypes: CXC (the two cysteines nearest the N-termini are separated by a another single amino acid), CC (the first two cysteines nearest the N-termini are adjacent), C (only one cysteine near its N-terminus) and CX3C (with three amino acids between the first two cysteines at the N-terminal) (Rollins, 1997; Balkwill, 2004). Further, the family of CXC chemokines can be divided into to ELR- and ELR + groups based on the absence or presence of the tripeptide Glu-Leu-Arg (the ELR motif) which precedes the cysteine on the N-terminus (Baggiolini et al., 1997). CXCL8 is one of the ELR + CXC chemokines (Baldwin et al., 1991).
The gene encoding CXCL8 is located on 4q13-q21 (Modi et al., 1990). There are four common polymorphisms in the CXCL8 gene: rs4073(-251 A/T), rs2227532(-845T/C), rs2227307(+396 G/T) and rs2227306(+781 C/T) (Mukaida et al., 1989; Yao et al., 2019). Previously studies indicated that the single nucleotide polymorphism (SNPs) of CXCL8 gene were significantly associated with increased risk or progression of non small cell lung cancer, gastric cancer, differentiated thyroid cancer and ovarian cancer, especially CXCL8-251 A/T (Rafrafi et al., 2013; Koensgen et al., 2015; Kilic et al., 2016; Boonyanugomol et al., 2019). One of the most remarkable characteristics of CXCL8 is the variation of its expression levels. Normally, CXCL8 is undetectable in noninduced cells (Hoffmann et al., 2002). Mechanismly, in these unstimulated cells, the promoter of CXCL8 gene is repressed by three events: firstly, NF-κB-repressing factor (NRF) binds to the negative regulatory element (NRE) which overlaps the NF-κB binding site (Nourbakhsh et al., 2001); secondly, octamer-1 (OCT-1) binds to the complementary strand of the CXCL8 gene promoter in the opposite direction of the C/EBP site (Wu et al., 1997); and thirdly, deacetylation of the histone protein by histone deacetylase 1 (HDAC-1) (Ashburner et al., 2001). However, its expression is rapidly induced by various stimuli including cytokines such as IL-1 or TNFα, viral products or bacterials, other environmental stresses and transcription factors (including activator protein-1 (AP-1) and NF-κB) (Hoffmann et al., 2002; Helen et al., 2017). Remarkably, these stimuli cause a 5–100 folds increasing in CXCL8 expression(Hoffmann et al., 2002; Helen et al., 2017). Maximal CXCL8 expression and secretion is generated at least by a combination of three different mechanisms: 1) derepression of CXCL8 gene promoter; 2) transcriptional activation of CXCL8 gene by NF-κB and JNK pathways; 3) stabilization of CXCL8 mRNA by the p38 mitogen-activated protein kinase (MAPK) pathway (Hoffmann et al., 2002; Helen et al., 2017). The stability of the CXCL8 mRNA also plays an impact role on the secretion of CXCL8. In terms of mechanism, dual specificity mitogen-activated protein kinase kinase 6 (MKK6) by selectively activating p38 MAPK to activates MAP kinase-activated protein kinase 2 (MK2) which helps the stability of the CXCL8 mRNA (Helen et al., 2017; Hoffmann, et al., 2002). Additionally, in intestinal epithelial cells, carnosine could inhibit the translation of CXCL8 mRNA by phosphorylation of eIF4E (Son et al., 2008). Post-translational modification (PTM) of chemokines is an important mechanism of fine-tuning chemokine secretion, activation and selection of receptor (Vanheule et al., 2018). N-terminal shortening always associated with significantly increasing biological activity and receptor affinity of CXCL8. Numerous studies indicate that N-terminal truncation of CXCL8 (2 to 9–77) by CD13, CD26, MMP and so on (Van den Steen et al., 2003; Mortier et al., 2011; Vanheule et al., 2018). On the other side, citrullination of CXCL8 by peptidylarginine deiminase (PAD) could impair the effect of CXCL8 (Proost et al., 2008; Loos et al., 2009).
Receptors of CXCL8: CXCR1 and CXCR2
The receptors that bind to CXCL8 are two G protein coupled receptors (GPCR): CXCR1 and CXCR2 (Figure 1). The two receptors that are both the ELR + CXC receptors sharing 78% sequence homology between each other (Holmes et al., 1991; Murphy and Tiffany, 1991). CXCR1 and CXCR2 show different affinity to the different complexes of CXCL8. Both monomer and dimer forms of CXCL8 show similar affinity to CXCR2, however, only CXCL8 monomer functions as a potent CXCR1 agonist (Nasser et al., 2009; Das et al., 2010; Berkamp et al., 2017).
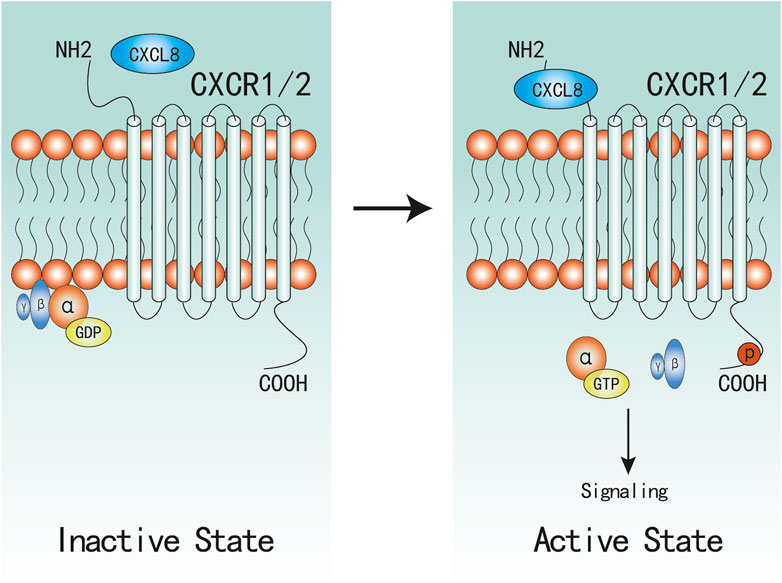
FIGURE 1. Active and Inactive Structures of CXCR1/2 Complex. CXCR1/2 is in complex with Gαβγ in inactive state. When CXCL8 binds to the N-terminal of CXCR1/2, α-GDP changes into α-GTP, and dissociates with βγ subunites, which would subsequently active associated signaling.
Upon CXCL8 binding, the following stable complexes will be formed: CXCL8(monomer)-CXCR1/2-G protein and CXCL8(dimer)-CXCR2-G protein (Park et al., 2012; Liu et al., 2020), which induce a conformational change of CXCR1/2 and facilitate the initiation of activation. Then, CXCR1/2 dissociate with the heterotrimeric G protein and then release the βγ subunites from the α subunite, which promotes the activation of several downstream signaling cascades (Waugh and Wilson, 2008; Liu et al., 2016). Similar to most GPCR, CXCR1/2 can also become phosphorylated, desensitized, and internalized upon binding to CXCL8. Despite evidenced that CXCR1/2 display similar downstream pathways, there remain marked differences between CXCR1 and CXCR2 in activation and signaling cascades. CXCR2 internalization occurs more rapidly and at lower ligand concentrations than CXCR1, and CXCR2 is also recycled back to the surface at a much slower rate than CXCR1 (Alfaro et al., 2017; Helen et al., 2017), which might be one possible mechanism that CXCR1 not CXCR2 can activate PLD (Stillie et al., 2009; Raghuwanshi et al., 2012; Cheng et al., 2019).
Signaling Pathways of CXCL8-CXCR1/2 Axis
Upon CXCL8 binding, CXCR1/2 can active multiple G-protein-mediated signalling cascades (Figure 2). Phosphatidylinositol-3 kinase (PI3K)/Akt is one of the principal downstream signal of CXCL8, which plays vital roles in modulating tumor motility, angiogenesis, and survival (Wilson et al., 2006; Waugh and Wilson, 2008). In androgen-independent prostate cancer, CXCL8 can also increase the expression of Akt (MacManus et al., 2007). PI3K can also act as an intermediate in coulping CXCR1/2 to MAPK and focal adhesion kinase (FAK)-Src signaling cascades (Knall et al., 1996; Waugh and Wilson, 2008). Phosphorylation of CXCR1/2 can also lead to two MyD88-dependent MAPK pathway: Erk-MAPK and p38 MAPK (Knall et al., 1997; Zhang et al., 2017; Cheng et al., 2019). Further, the Erk-MAPK cascades can be activated in indirect ways. Transactivation of epidermal growth factor receptor (EGFR) has been shown to occur in response to ligands of various GPCRs (Daub et al., 1996). The binding of CXCL8 to CXCR2 has been demonstrated to transactivate the EGFR resulting in Ras-GTPase activation, and subsequently actives the Erk-MAPK signaling cascades (Venkatakrishnan et al., 2000; Luppi et al., 2007). Activation of phospholipase C (PLC) by CXCL8 has been characterized in neutrophils and multiple cancer cells (Waugh and Wilson, 2008). The activated protein kinase C (PKC) can phosphorylate many cytoskeletal proteins that trigger dynamic alternations, facilitate cell adhesion and migration (Larsson, 2006; Quann et al., 2011). PKC can be classified into three categories (Larsson, 2006), and CXCL8 can active all the three categories of PKC mediated by PLC (Waugh and Wilson, 2008; Alassaf and Mueller, 2020). As a result of promoting these upstream signaling pathways, including PI3K/Akt, MAPK, and PLC/PKC, the activation of numerous transcription factors would be induced, one of which was nuclear factor-κB (NF-κB) (Waugh and Wilson, 2008; Gales, et al., 2013). Besides, activation of NF-κB is also one of the main mechanism to promote CXCL8 expression and secretion (Hoffmann, et al., 2002; Gales, et al., 2013; Helen, et al., 2017). Therefore, there exist a positive feedback between CXCL8 secretion and NF-κB activation, which has also been well described in a previous review (Gales, et al., 2013). Additionally, Numerous studies have confirmed that CXCL8 can induce the phosphorylation of protein tyrosine kinases, including FAK and Src kinases (Waugh and Wilson, 2008; Liu et al., 2016; Ju et al., 2017; Mohamed et al., 2020). Activation of FAK and Src kinases has been uncovered to promote cell proliferation, invasion, survival, and motility (Sulzmaier et al., 2014; Roskoski, 2015). In endothelial cells, it has revealed that CXCL8 can induce vascular endothelial growth factor receptor-2 (VEGFR2) phosphorylation mediated by the activation of Src kinases (Petreaca et al., 2007). CXCL8 can also promote dynamic and time-dependent induction of Rho-GTPases family in prostate cancer and endothelial cells (Schraufstatter et al., 2001; Waugh and Wilson, 2008; Yan et al., 2016). Recently, increased studies evidence that CXCL8 can induce the activation of Janus kinases and signal transducer and activator of transcription protein 3 (JAK/STAT3) signaling in both cancer and immune cells (Fu et al., 2015; Guo et al., 2017; Wu et al., 2019; Hu et al., 2020). Wu et al. demonstrated that CXCL8 could impair the function of NK cells by promoting STAT3 (Wu et al., 2019).
Roles of CXCL8 in Tumor Biology
Expression of CXCL8 is significantly higher in numerous types of cancers (Figure 3), and many studies have evidenced that serum level of CXCL8 in patients with cancer can act as a prognostic marker (Cheng et al., 2019; Fousek et al., 2021). CXCL8 can promote tumor proliferation, survival, invasion, angiogenesis, tumor stemness and suppress anti-tumor immunity in direct and indirect manner (Figure 4) (Table 1). Growing evidence indicates that CXCL8 can directly contribute to the development of resistance to chemotherapy, molecularly targeted therapy, and immune checkpoint inhibition (ICI) therapy (Alfaro et al., 2017; Fousek et al., 2021). Therefore, CXCL8 has already been described as a pro-tumorigenic chemokine by impacting cancer cells and modifying TME to promote tumor progression and metastasis.
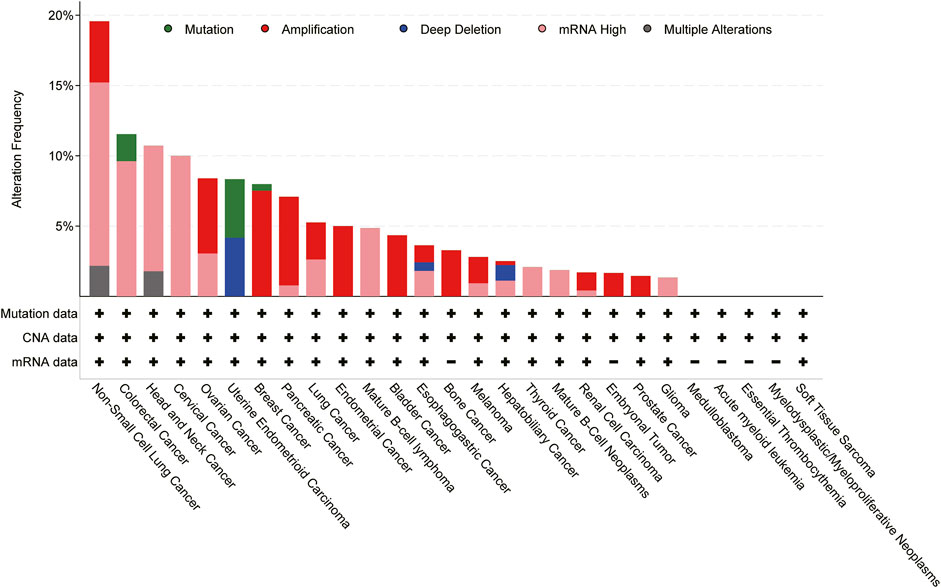
FIGURE 3. Genomic alterations of CXCL8 cross 27 cancer types; TCGA pan-cancer cohort from cBioPortal for Cancer Genomics were used for this analysis.
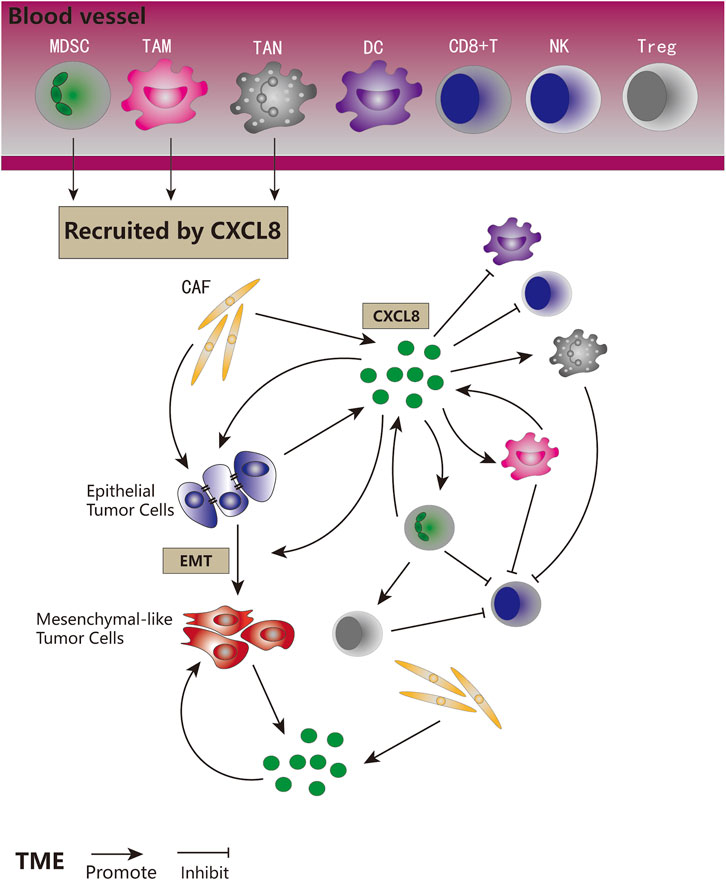
FIGURE 4. The role of CXCL8 signaling in tumor biology. CXCL8 recruited MDSCs, TAMs, and TANs to the TME. CXCL8 could be secreted by tumor cells, CAFs, MDSCs, and TAMs. CXCL8 could promote tumor cells proliferation and EMT, directly and indirectly. CXCL8 could facilitate the accumulation of pro-tumorigenic immune cells and tumor immune suppression, and inhibit anti-tumor immune cells in direct and indirect ways.
Promoting Tumor Cells Proliferation and Survival by Novel Mechanisms
Many studies have proved that CXCL8 can promote cell proliferation and inhibit apoptosis in multiple cancers, including breast cancer, prostate cancer, lung cancer, colon cancer and so on (Liu et al., 2016). CXCL8 can mediate cancer cell proliferation both in autocrine and paracrine manner. Previous review has demonstrated that CXCL8 could be secreted by tumor cells and subsequently promote themselves growth and/or inhibit apoptosis (Liu et al., 2016). Some recent researches again proved this mechanism (Guo et al., 2017; Cui et al., 2018; Jia et al., 2018; Kumar et al., 2019).
Recently, increased evidence highlighted several novel mechanisms. As metabolism reprogramming has already become a hallmark of cancer, Xu et al. illustrate that CXCL8 could mediate enhancement of aerobic glycolysis in colorectal cancer (CRC) cells and reduce intracellular reactive oxygen species (ROS) levels, which subsequently promote CRC cell proliferation and invasion (Xu et al., 2017). We have also shown that CXCL8 can reduce the level of intracellular ROS by inhibiting the function of GSK-3β to suppress prostate cancer cell apoptosis (Sun L. et al., 2019). Components of TME play a vital role in progression and metastasis of cancer and can induce an upregulated cytokines and chemokines, such as CXCL8. Two studies conducted in CRC and pancreatic ductal adenocarcinoma indicated that mesenchymal stem cells (MSCs) and cancer-associated fibroblasts (CAFs) could promote cancer cells secreting CXCL8, then enhancing the ability of proliferation and invasion (Wang et al., 2015; Awaji et al., 2019). Furthermore, Yang et al. have demonstrated that there exists a positive feedback between CRC and neutrophil extracellular traps (NETs) mediated by CXCL8 (Yang L. et al., 2020). In addition to malignant cells, cells in TME can also secret CXCL8 and promote cancer cell proliferation, which can be supported by a recent study that suggested that CAFs in TME can release CXCL8 to increase the proliferation ability of gallbladder cancer cells (Chen et al., 2020).
Promoting Tumor Cells Invasion and Migration by Novel Mechanisms
One of the main mechanisms used by tumor cells to obtain invasiveness and motility is the epithelial-to-mesenchymal transition (EMT). Present studies have proved that CXCL8 is essential for tumor cells to acquire and maintain this aggressive phenotype (Long et al., 2016; Fousek et al., 2021). As displayed above, components of TME can secret or promote cancer cells to secret CXCL8 which can also subsequently mediate the EMT of tumor cells. Further, there also exists an autocrine positive feedback loop between EMT and CXCL8 (David et al., 2016; Long et al., 2016). Many published researches indicate the important role of EMT in tumor resistance to chemotherapy, molecularly targeted therapy, and immune checkpoint inhibition (ICI) therapy (Horn et al., 2020b). Additionally, there are many excellent reviews about other mechanisms of CXCL8 in tumor therapy resistance (Alfaro et al., 2017; Cheng et al., 2019; Horn et al., 2020a; Fousek et al., 2021).
Emerging investigations have highlighted several novel mechanisms that are associated with the role of CXCL8 in cancer cell invasion and migration, including tumor heterogeneity, formation of feedback loop, and interacting with TME. Tumor heterogeneity is a vital feature of cancers, and cell sub-populations may interact with others to facilitate tumor progression (Meacham and Morrison, 2013). In the context of CRC, both hypoxic and fusobacterium nucleatum infected cancer cells can secret CXCL8 which subsequently contribute to the EMT of normoxic and noninfected cancer cells (Casasanta et al., 2020; Mi et al., 2020). Maynard et al. also demonstrate that only part of prostate cancer cells express CXCL8 in prostate cancer tissue microarrays, and high level of CXCL8 is associated with a more aggressive disease (Maynard et al., 2020). Pro-tumor feedback loops mediated by CXCL8 has been observed in multiple types of cancers. Xu et al. reported an intracellular feedback loop between CXCL8 and PTEN in HNSCC (Xu et al., 2020). Similarly, PTEN loss can also selectively upregulate the CXCL8 signaling in prostate cancer cells (Maxwell et al., 2013). Effects of CXCL8 on tumor cells could also influence the TME or be influenced by the components of TME. MSCs and NET can also form a positive pro-tumor feedback loop with osteosarcoma and glioma cells via CXCL8, respectively (Kawano et al., 2018; Zha et al., 2020). Previous reviews and many recent studies have illustrated that multiple cell types in TME can directly secret CXCL8, or regulate the expression of CXCL8 in cancer cells, or be regulated by CXCL8 derived from cancer cells to promote tumor invasion and migration (Zheng et al., 2018; Cheng et al., 2019; Nie et al., 2019; Fousek et al., 2021).
Promoting Tumorogenic Angiogenesis
Angiogenesis has been recognized as a hallmark of cancer, which is necessary for tumor survival and disseminating to a new location (Hanahan and Weinberg, 2011). Effect of CXCL8 on tumor angiogenesis has been widely investigated, and CXCL8 has already be defined as a pro-angiogenesis chemokine (Liu et al., 2016; Cheng et al., 2019; Fousek et al., 2021; Ueda et al., 2022). Human vascular endothelial cells constitutively express CXCR2 (Cheng et al., 2019). Upon cancer cells and some types of stroma cells secreting CXCL8 in TME, endothelial cells begin to express and secret matrix metalloproteinases (MMPs) to break down the extracellular matrix (ECM), then, resulting in angiogenesis (Li et al., 2003). In addition, CXCL8 can also induce recruitment of endothelial cells which participate directly in vascularization (Strieter et al., 1995). Intriguingly, there is also a crosstalk between CXCL8 and VEGFR2 in angiogenesis (Petreaca et al., 2007). In this context, a loop in endothelial cells have been discovered, which is that CXCL8 can increase the secretion of VEGF-A and induce the expression of VEGFR2 in endothelial cells (Martin et al., 2009; Alfaro et al., 2017).
Promoting Development of Cancer Stem Cells
A plethora of literature indicates that CXCL8 is involved in the maintenance of cancer stem cells (CSCs) which is always associated with tumor development and progression, treatment resistance and used to explain heterogeneity in solid tumors (Raza et al., 2022). Generally, the CXCL8-CXCR1/2 axis plays impact roles on formation, development or invasion of CSCs in colon cancer (Luo et al., 2018; Fisher et al., 2019; Kim et al., 2021), breast cancer (Choi et al., 2009), glioblastoma (Zhou et al., 2014; McCoy et al., 2019), clear cell renal cell carcinoma (ccRCC) (Corrò et al., 2019), pancreatic cancer (Chen et al., 2014), hepatocellular carcinoma (HCC) (Kahraman et al., 2019), lung cancer (Shimizu and Tanaka, 2019), bladder cancer (Zhou et al., 2021) and esophageal carcinoma (Huang et al., 2017). Furthermore, CXCL8 has also been found to promote the interaction between CSCs and mesenchymal stem cells (MSCs) to further enlarging the population of CSCs in colon cancer (Ma et al., 2020; Ma et al., 2021). Given the crucial role of CXCL8 in CSCs, targeting CXCL8-CXCR1/2 axis as a component of combination therapy has also been explored. Pre-clinical studies demonstrate that combining CXCR1/2 inhibitors with the human epidermal growth factor receptor 2 (HER2)-targeted therapies has potential as an effective treatment strategy to repress CSCs activity in breast cancer (Singh et al., 2013). In HCC, after inhibiting CXCR1/2 by Reparixin or knockdown CXCL8, CSCs features of HCC were reduced, and sensitivity to Sorafenib increased significantly (Kahraman et al., 2019).
Inhibiting Anti-Tumor Immunity
CXCL8 also has potent ability on modulating immune cell chemotaxis and functions. CXCL8 derived from tumor can act in a paracrine manner to change the composition of immune infiltration in TME, resulting the accumulation of pro-tumorigenic immune cells and tumor immune suppression (Alfaro et al., 2017; Horn et al., 2020b). Published reports collectively suggest that CXCL8 can recruit tumor-associated macrophages (TAMs), myeloid derived suppressor cells (MDSCs), and neutrophils to the TME, resulting in dampening the anti-tumor immune response of cytotoxic immune cells. Correspondingly, it can also attenuate the anti-tumor activity of dendritic cells (DCs) and NK cells (Alfaro et al., 2017; Fousek et al., 2021).
Neutrophils make up a sizeable part of immune cells in TME, so-called tumor-associated neutrophils (TANs), which can be divided into antitumor N1 and pro-tumor N2 phenotypes (Fridlender et al., 2009). Further, the N2 TANs promote tumor progression by activating tumor angiogenesis, suppressing the function of anti-tumor T cells, and recruiting T regulatory cells (Tregs) (Rodriguez et al., 2004; Nozawa et al., 2006; Mishalian et al., 2014; David et al., 2016). Unfortunately, there are no groups directly investigating the relationship between CXCL8 and N2 phenotype. Several recent reports preliminary demonstrate the effect of CXCL8 on TANs. In ovarian cancer, CXCL8 can recruit TANs in TME and induce the expression of Jagged2 (JAG2) in TANs which subsequently inhibit the activity of CD8(+) T cells (Yang M. et al., 2020). Additionally, TANs in the TME of diffuse large B-cell lymphoma (DLBCL) can also increase the secretion of april mediated by CXCL8, and promote DLBCL progression (Manfroi et al., 2017).
MDSCs are known as a heterogeneous population of immature immunosuppressive cells, which derive from myeloid progenitor cells, accumulate in the circulation and the TME of most cancer patients (Gabrilovich and Nagaraj, 2009; David et al., 2016). Generally, MDSCs can be divided into two subtypes: granulocytic (CD33 + CD11b + HLA-DR-/low CD15+, PMN-MDSC) or monocytic (CD33 + CD11b + HLA-DR-/low CD14+, M-MDSC) (Poschke and Kiessling, 2012). In TME, MDSCs have been shown to act as a driver of immune suppression by inactivating T cell receptors, starving T cell, inhibiting T cell proliferation, recruiting CAFs, and inducing Tregs (David et al., 2016; Horn et al., 2020a). Increasing investigations evidence the role of CXCL8 in attracting and enhancing the function of MDSCs which then attenuate the activation of CD8(+) T cell (Asfaha et al., 2013; Katoh et al., 2013; Dominguez et al., 2017; Najjar et al., 2017; Li et al., 2018). Interestingly, CXCL8 can recruit the both subtypes of MDSCs to TME, but they play different functions (Alfaro et al., 2016). The M-MDSCs can directly suppress the activity of T cells, however, the PMN-MDSC subset only induce the formation of NETs which might facilitate tumor cell migration. Further, a recent report indicates a novel subtype of MDSCs that attract by CXCL8 in human gastric cancer (Mao et al., 2018). After attracting CD45(+)CD33(low)CD11b(dim) MDSCs to TME, CXCL8 will promote this novel subset MDSCs expressing arginase I that contributes to CD8(+) T cell suppression via PI3K/Akt signaling. Supportively, in prostate cancer and melanoma, the increasing level of circulating MDSCs are evidenced to be associated poor clinical outcomes and high plasma CXCL8 concentration (Chi et al., 2014; Tobin et al., 2019).
Tumor-associated macrophages (TAMs) are present in all stages of tumors and exert a dual effectiveness on tumor progression (Noy and Pollard, 2014; Mantovani et al., 2017). Similar to TANs, TAMs have been divided into two subsets: antitumor M1-like and tumor-promoting M2-like phenotype (Noy and Pollard, 2014). Numerous studies have concentrated on the role of TAMs on CXCL8, which have indicated that TAMs can express or induce tumor cell secreting CXCL8 to contribute to tumor progression (Chen et al., 2003; Chen et al., 2018; Zheng et al., 2018). Emerging reports uncover that CXCL8 also have vital roles in recruiting and directing the polarization of TAMs. The findings of Zhang and colleagues suggest that the CXCL8-CXCR2 axis can promote trafficking of CXCR2(+) CD68(+) macrophages to pancreatic cancer TME, and the recruitment TAMs can inhibit the efficacy of PD1 blockade (Zhang et al., 2020). Further investigation performed in gastric cancer indicates that TAMs can induce themselves increasing the expression of PD-L1 and decrease CD8(+) T cells infiltration by secreting CXCL8 (Lin et al., 2019). Meanwhile, CXCL8 also plays a role in inducing a shift in TAMs toward the M2 phenotype (Krawczyk et al., 2017; Ning et al., 2018).
DCs and NK cells play important roles in adaptive and innate anti-tumor immune response, respectively. Previous studies suggest that CXCL8 derived from tumor cells can disorients DCs migration without impairing the stimulation of T-cell (Feijoó et al., 2005; Alfaro et al., 2011; Li et al., 2021). A recent investigations indicates that tumor cells can secret CXCL8 to impair the functions of NK cells via STAT3 signaling (Wu et al., 2019).
CXCL8 in Immune Checkpoint Therapy
Many excellent reviews demonstrate the role of CXCL8-CXCR1/2 axis in target therapy, chemotherapy and the prognostic role of plasma level of CXCL8 in cancer (Liu et al., 2016; Alfaro et al., 2017; Cheng et al., 2019; Fousek et al., 2021). Here, we highlight current preclinical and clinical studies correlating CXCL8 to immunotherapy. Immune checkpoint inhibitions (ICIs) has become the cornerstone of immunotherapy in many types of cancers (Ribas and Wolchok, 2018; Bakouny and Choueiri, 2020). However, not all cancer patients have a good response to ICIs, and early determining the clear group which is sensitive or resistant to ICIs can improve clinical outcomes. Recent evidences suggest that CXCL8 plays an important role in response quality on ICIs.
A small retrospective trial suggests that increasing level of serum CXCL8 can predict resistant to anti-PD-1 treatment in non-small-cell lung cancer (NSCLC) patients (Sanmamed et al., 2017). To further investigating the role of CXCL8 in predicting response of patients treated with ICIs, two recent trials with large sample size have been performed to evaluate the correlation between plasma CXCL8 and cancer progression (Schalper et al., 2020; Yuen et al., 2020). Schalper et al., using a large cohort with 1,344 patients, show that high baseline plasma CXCL8 level is associated with poor clinical outcomes in participants with advanced melanoma, NSCLC, and renal-cell carcinoma (RCC) treated with nivolumab or ipilimumab, everolimus or docetaxel, which indicate that serum CXCL8 level is an unfavorable factor in tumor immunobiology and can act as an independently predictive biomarker in patients receiving ICIs (Schalper et al., 2020). Another multiple randomized trials with 1,445 patients in metastatic urothelial carcinoma (mUC) and mRCC confirms this finding (Yuen et al., 2020). Both trials further suggest that greater CXCL8 expression in tumor is associated with higher plasma CXCL8 level, an immunosuppressive myeloid-enriched TME, and T cell suppression (Schalper et al., 2020; Yuen et al., 2020). Additionally, Yuen and colleagues also further stratify patients using plasma CXCL8 level and T cell effector signature score. Patients with high T cell effector signature score and low plasma CXCL8 level can derive best benefit from ICIs (Yuen et al., 2020).
Emerging studies suggest that combination of targeting CXCL8-CXCR1/2 axis and ICIs can provide further benefit in anti-tumor efficacy. In the context of breast and lung cancer, combined SX-682, an bioavailable small-molecule inhibitor of CXCR1 and CXCR2, and anti-PD-1/PD-L1 can achieve best tumor control in murine model (Sun Y. et al., 2019; Horn et al., 2020b). Interestingly, both studies indicate that inhibition of CXCR1/2 eventually results in reducing infiltration with PMN-MDSCs which play a vital role in T cell suppression. Zhang et al. discover that treated with interferon gamma (IFN-γ) can suppress a variety of pancreatic cancer derived CXCL8 and tumor-derived CXCL8 deficiency inhibit the trafficking of M2 TAM (Zhang et al., 2020). Further, combined IFN-γ and anti-PD-1 treatment enhance the anti-tumor efficacy. As for directly targeting CXCL8, in triple-negative breast cancer (TNBC), HuMax-IL8, a fully human monoclonal antibody that inhibits CXCL8, can significantly reduce the infiltration of PMN-MDSCs to TME and enhance the efficacy of immunotherapy (Dominguez et al., 2017).
Bilusic et al. conduct a phase I clinical trial including 15 patients with metastatic or unresectable solid tumors treated with HuMax-IL8 (Bilusic et al., 2019). The results of this trial indicate that HuMax-IL8 is safe and well-tolerated. This trial could be a basic evidence for further evaluating the combination of CXCL8 blockade and other immunotherapies. In addition, several ongoing studies have been designed to evaluate the safety and efficacy of combined HuMax-IL8 and immunotherapy in cancer patients (Table 2).

TABLE 2. Clinical Studies of combination of ICI agents and CXCL8 blockade. A = active; R = recruiting.
Future Directions
Expression of CXCL8 is significantly higher in numerous types of cancers, and high expression of CXCL8 is significantly associated with shorter median overall survival in many kinds of cancers based on TCGA database (Table 3). The findings of numerous researches involving preclinical in vitro and in vivo models illustrate that combination of targeting CXCL8-CXCR1/2 axis and ICIs can provide further benefit in anti-tumor efficacy. In addition, clinical trials demonstrate that CXCL8 plays an important role in response quality on ICIs. Furthermore, activation of CXCL8-CXCR1/2 axis and its downstream signaling pathways play important roles in tumor survival and invasion, and suppress antitumor immune responses in the TME. Therefore, therapies targeting this axis are likely to benefit patients with cancer by inhibiting tumor growth and stimulating antitumor immunity. Clinical trials are ongoing to prove this.
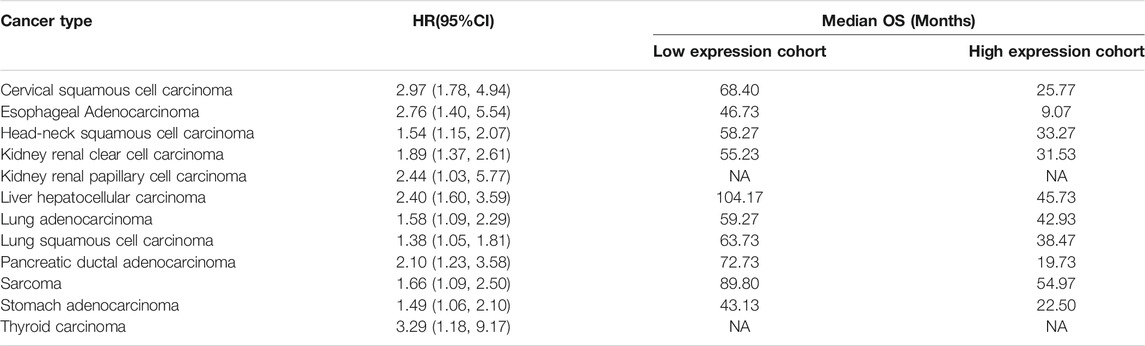
TABLE 3. The correlation between CXCL8 expression and cancer survival in TCGA cohorts. HR, hazard ratio; CI, confidence interval; OS, overall survival; NA, not analysis.
As metabolism reprogramming has already become a hallmark of cancer, the relationship between CXCL8 and tumor metabolism reprogramming is not widely explored. How CXCL8 influences tumor metabolism and subsequently facilitate cancer proliferation needing further investigation. In addition, present studies demonstrated that part of CRC cells could secreted CXCL8 which contributed to the EMT of the remaining cells not secreting CXCL8. However, the mechanism of this phenomenon is not clear. Further exploration is needed to determine if other types of cancer exist similar phenomenon. As mentioned, CXCL8 can recruit TANs in TME. Unfortunately, there are no groups directly investigating the relationship between CXCL8 and N2 phenotype. Meanwhile, CXCL8 also plays a role in inducing a shift in TAMs toward the M2 phenotype. However, the mechanism is unclear.
Conclusion
The Chemokine CXCL8 is well accepted to play a crucial role in tumor survival, invasion, and TME angiogenesis, immune suppression via several types of intracellular signaling pathway. To date, many novel mechanisms to mediate the above tumor biology by CXCL8 have been highlighted. More and more evidences indicate that CXCL8 should be considered as a pro-tumor factor with dual roles: directly promoting tumor survival and affecting components of TME to indirectly facilitate tumor progression. Further, CXCL8 can also act as an important predictor of the clinical outcomes for ICIs. As cancer therapy advances, some emerging clinical trials are ongoing to explore the efficacy and safety of combining anti-CXCL8 and ICIs therapy.
Author Contributions
LY and JA were responsible for the conception and design of the study. XX and SQ did the articles’ search. XX, XL, SZ, and SQ were responsible for the acquisition of relevant information. XX, HX, SZ, and SW wrote the first draft of the manuscript. SW, SZ, JA, and LY interpreted the relevant information and wrote the final version. All authors critically revised the Article for important intellectual content and approved the final version. LY and AJZ obtained public funding.
Funding
This work was supported by Sichuan International Science and technology innovation cooperation/Hong Kong, Macao and Taiwan Science and technology innovation cooperation project (2021YFH0172); the National key research and development program of China (Grant No. 2017YFC0908003); National Natural Science Foundation of China (Grant No.81974099, 81974098); Post-doctoral Science Research Foundation of Sichuan University (2020SCU12041); National Clinical Research Center for Geriatrics, West China Hospital, Sichuan University (Z2018C01); Technology Innovation Research and Development Project, Chengdu Science and Technology Bureau (2019-YF05-00296-SN). project (2021YFH0172).
Conflict of Interest
The authors declare that the research was conducted in the absence of any commercial or financial relationships that could be construed as a potential conflict of interest.
Publisher’s Note
All claims expressed in this article are solely those of the authors and do not necessarily represent those of their affiliated organizations, or those of the publisher, the editors and the reviewers. Any product that may be evaluated in this article, or claim that may be made by its manufacturer, is not guaranteed or endorsed by the publisher.
References
Alassaf, E., and Mueller, A. (2020). The Role of PKC in CXCL8 and CXCL10 Directed Prostate, Breast and Leukemic Cancer Cell Migration. Eur. J. Pharmacol. 886, 173453. doi:10.1016/j.ejphar.2020.173453
Alfaro, C., Sanmamed, M. F., Rodríguez-Ruiz, M. E., Teijeira, Á., Oñate, C., González, Á., et al. (2017). Interleukin-8 in Cancer Pathogenesis, Treatment and Follow-Up. Cancer Treat. Rev. 60, 24–31. doi:10.1016/j.ctrv.2017.08.004
Alfaro, C., Suárez, N., Martínez-Forero, I., Palazón, A., Rouzaut, A., Solano, S., et al. (2011). Carcinoma-derived Interleukin-8 Disorients Dendritic Cell Migration without Impairing T-Cell Stimulation. PLoS One 6, e17922. doi:10.1371/journal.pone.0017922
Alfaro, C., Teijeira, A., Oñate, C., Pérez, G., Sanmamed, M. F., Andueza, M. P., et al. (2016). Tumor-Produced Interleukin-8 Attracts Human Myeloid-Derived Suppressor Cells and Elicits Extrusion of Neutrophil Extracellular Traps (NETs). Clin. Cancer Res. 22, 3924–3936. doi:10.1158/1078-0432.CCR-15-2463
Asfaha, S., Dubeykovskiy, A. N., Tomita, H., Yang, X., Stokes, S., Shibata, W., et al. (2013). Mice that Express Human Interleukin-8 Have Increased Mobilization of Immature Myeloid Cells, Which Exacerbates Inflammation and Accelerates colon Carcinogenesis. Gastroenterology 144, 155–166. doi:10.1053/j.gastro.2012.09.057
Ashburner, B. P., Westerheide, S. D., and Baldwin, A. S. (2001). The P65 (RelA) Subunit of NF-Κb Interacts with the Histone Deacetylase (HDAC) Corepressors HDAC1 and HDAC2 to Negatively Regulate Gene Expression. Mol. Cel Biol 21, 7065–7077. doi:10.1128/MCB.21.20.7065-7077.2001
Awaji, M., Futakuchi, M., Heavican, T., Iqbal, J., and Singh, R. K. (2019). Cancer-Associated Fibroblasts Enhance Survival and Progression of the Aggressive Pancreatic Tumor via FGF-2 and CXCL8. Cancer Microenvironment 12, 37–46. doi:10.1007/s12307-019-00223-3
Baggiolini, M., Dewald, B., and Moser, B. (1997). Human Chemokines: an Update. Annu. Rev. Immunol. 15, 675–705. doi:10.1146/annurev.immunol.15.1.675
Bakouny, Z., and Choueiri, T. K. (2020). IL-8 and Cancer Prognosis on Immunotherapy. Nat. Med. 26, 650–651. doi:10.1038/s41591-020-0873-9
Baldwin, E. T., Weber, I. T., St Charles, R., Xuan, J. C., Appella, E., Yamada, M., et al. (1991). Crystal Structure of Interleukin 8: Symbiosis of NMR and Crystallography. Proc. Natl. Acad. Sci. 88, 502–506. doi:10.1073/pnas.88.2.502
Balkwill, F. (2004). Cancer and the Chemokine Network. Nat. Rev. Cancer 4, 540–550. doi:10.1038/nrc1388
Berkamp, S., Park, S. H., De Angelis, A. A., Marassi, F. M., and Opella, S. J. (2017). Structure of Monomeric Interleukin-8 and its Interactions with the N-Terminal Binding Site-I of CXCR1 by Solution NMR Spectroscopy. J. Biomol. NMR 69, 111–121. doi:10.1007/s10858-017-0128-3
Bilusic, M., Heery, C. R., Collins, J. M., Donahue, R. N., Palena, C., Madan, R. A., et al. (2019). Phase I Trial of HuMax-IL8 (BMS-986253), an anti-IL-8 Monoclonal Antibody, in Patients with Metastatic or Unresectable Solid Tumors. J. Immunotherapy Cancer 7, 240. doi:10.1186/s40425-019-0706-x
Boonyanugomol, W., Rukseree, K., Kongkasame, W., Palittapongarnpim, P., Baik, S.-C., and Manwong, M. (2019). Genetic Polymorphisms of CXCL8 (−251) Are Associated with the Susceptibility of Helicobacter pylori Infection Increased the Risk of Inflammation and Gastric Cancer in Thai Gastroduodenal Patients. Ijaai 18, 393–401. doi:10.18502/ijaai.v18i4.1417
Casasanta, M. A., Yoo, C. C., Udayasuryan, B., Sanders, B. E., Umaña, A., Zhang, Y., et al. (2020). Fusobacterium Nucleatum Host-Cell Binding and Invasion Induces IL-8 and CXCL1 Secretion that Drives Colorectal Cancer Cell Migration. Sci. Signal. 13. doi:10.1126/scisignal.aba9157
Chen, C., Zhang, R., Ma, L., Li, Q., Zhao, Y. L., Zhang, G. J., et al. (2020). Neuropilin‐1 Is Up‐regulated by Cancer‐associated Fibroblast‐secreted IL‐8 and Associated with Cell Proliferation of Gallbladder Cancer. J. Cel. Mol. Med. 24, 12608–12618. doi:10.1111/jcmm.15825
Chen, J. J., Yao, P. L., Yuan, A., Hong, T. M., Shun, C. T., Kuo, M. L., et al. (2003). Up-regulation of Tumor Interleukin-8 Expression by Infiltrating Macrophages: its Correlation with Tumor Angiogenesis and Patient Survival in Non-small Cell Lung Cancer. Clin. Cancer Res. 9, 729–737.
Chen, L., Fan, J., Chen, H., Meng, Z., Chen, Z., Wang, P., et al. (2014). The IL-8/CXCR1 axis Is Associated with Cancer Stem Cell-like Properties and Correlates with Clinical Prognosis in Human Pancreatic Cancer Cases. Sci. Rep. 4, 5911. doi:10.1038/srep05911
Chen, S.-j., Lian, G.-d., Li, J.-j., Zhang, Q.-b., Zeng, L.-j., Yang, K.-g., et al. (2018). Tumor-driven like Macrophages Induced by Conditioned media from Pancreatic Ductal Adenocarcinoma Promote Tumor Metastasis via Secreting IL-8. Cancer Med. 7, 5679–5690. doi:10.1002/cam4.1824
Cheng, Y., Ma, X.-l., Wei, Y.-q., and Wei, X.-W. (2019). Potential Roles and Targeted Therapy of the CXCLs/CXCR2 axis in Cancer and Inflammatory Diseases. Biochim. Biophys. Acta (Bba) - Rev. Cancer 1871, 289–312. doi:10.1016/j.bbcan.2019.01.005
Chi, N., Tan, Z., Ma, K., Bao, L., and Yun, Z. (2014). Increased Circulating Myeloid-Derived Suppressor Cells Correlate with Cancer Stages, Interleukin-8 and -6 in Prostate Cancer. Int. J. Clin. Exp. Med. 7, 3181–3192.
Choi, H. S., Kim, J.-H., Kim, S.-L., and Lee, D.-S. (2009). Disruption of the NF-κB/IL-8 Signaling Axis by Sulconazole Inhibits Human Breast Cancer Stem Cell Formation. Cells 8, 1007. doi:10.3390/cells8091007
Corrò, C., Healy, M. E., Engler, S., Bodenmiller, B., Li, Z., Schraml, P., et al. (2019). IL‐8 and CXCR1 Expression Is Associated with Cancer Stem Cell‐like Properties of clear Cell Renal Cancer. J. Pathol. 248, 377–389. doi:10.1002/path.5267
Cui, Z. Y., Jo, E., Jang, H. J., Hwang, I.-H., Lee, K.-B., Yoo, H.-S., et al. (2018). Modified Ginseng Extract Induces Apoptosis in HepG2 Cancer Cells by Blocking the CXCL8-Mediated Akt/Nuclear Factor-Κb Signaling Pathway. Am. J. Chin. Med. 46, 1645–1662. doi:10.1142/S0192415X18500842
Das, S. T., Rajagopalan, L., Guerrero-Plata, A., Sai, J., Richmond, A., Garofalo, R. P., et al. (2010). Monomeric and Dimeric CXCL8 Are Both Essential for In Vivo Neutrophil Recruitment. PLoS One 5, e11754. doi:10.1371/journal.pone.0011754
Daub, H., Ulrich Weiss, F., Wallasch, C., and Ullrich, A. (1996). Role of Transactivation of the EGF Receptor in Signalling by G-Protein-Coupled Receptors. Nature 379, 557–560. doi:10.1038/379557a0
David, J., Dominguez, C., Hamilton, D., and Palena, C. (2016). The IL-8/IL-8R Axis: A Double Agent in Tumor Immune Resistance. Vaccines 4, 22. doi:10.3390/vaccines4030022
Dominguez, C., McCampbell, K. K., David, J. M., and Palena, C. (2017). Neutralization of IL-8 Decreases Tumor PMN-MDSCs and Reduces Mesenchymalization of Claudin-Low Triple-Negative Breast Cancer. JCI Insight 2. doi:10.1172/jci.insight.94296
Feijoó, E., Alfaro, C., Mazzolini, G., Serra, P., Peñuelas, I., Arina, A., et al. (2005). Dendritic Cells Delivered inside Human Carcinomas Are Sequestered by Interleukin-8. Int. J. Cancer 116, 275–281. doi:10.1002/ijc.21046
Fisher, R. C., Bellamkonda, K., Alex Molina, L., Xiang, S., Liska, D., Sarvestani, S. K., et al. (2019). Disrupting Inflammation-Associated CXCL8-CXCR1 Signaling Inhibits Tumorigenicity Initiated by Sporadic- and Colitis-Colon Cancer Stem Cells. Neoplasia 21, 269–281. doi:10.1016/j.neo.2018.12.007
Fousek, K., Horn, L. A., and Palena, C. (2021). Interleukin-8: A Chemokine at the Intersection of Cancer Plasticity, Angiogenesis, and Immune Suppression. Pharmacol. Ther. 219, 107692. doi:10.1016/j.pharmthera.2020.107692
Fridlender, Z. G., Sun, J., Kim, S., Kapoor, V., Cheng, G., Ling, L., et al. (2009). Polarization of Tumor-Associated Neutrophil Phenotype by TGF-β: "N1" versus "N2" TAN. Cancer Cell 16, 183–194. doi:10.1016/j.ccr.2009.06.017
Fu, X.-T., Dai, Z., Song, K., Zhang, Z.-J., Zhou, Z.-J., Zhou, S.-L., et al. (2015). Macrophage-secreted IL-8 Induces Epithelial-Mesenchymal Transition in Hepatocellular Carcinoma Cells by Activating the JAK2/STAT3/Snail Pathway. Int. J. Oncol. 46, 587–596. doi:10.3892/ijo.2014.2761
Gabrilovich, D. I., and Nagaraj, S. (2009). Myeloid-derived Suppressor Cells as Regulators of the Immune System. Nat. Rev. Immunol. 9, 162–174. doi:10.1038/nri2506
Guo, Y., Zang, Y., Lv, L., Cai, F., Qian, T., Zhang, G., et al. (2017). IL-8 Promotes Proliferation and Inhibition of Apoptosis via STAT3/AKT/NF-κB Pathway in Prostate Cancer. Mol. Med. Rep. 16, 9035–9042. doi:10.3892/mmr.2017.7747
Ha, H., Debnath, B., and Neamati, N. (2017). Role of the CXCL8-Cxcr1/2 Axis in Cancer and Inflammatory Diseases. Theranostics 7, 1543–1588. doi:10.7150/thno.15625
Hanahan, D., and Weinberg, R. A. (2011). Hallmarks of Cancer: the Next Generation. Cell 144, 646–674. doi:10.1016/j.cell.2011.02.013
Hoffmann, E., Dittrich-Breiholz, O., Holtmann, H., and Kracht, M. (2002). Multiple Control of Interleukin-8 Gene Expression. J. Leukoc. Biol. 72, 847–855. doi:10.1189/jlb.72.5.847
Holmes, W. E., Lee, J., Kuang, W.-J., Rice, G. C., and Wood, W. I. (1991). Structure and Functional Expression of a Human Interleukin-8 Receptor. Science 253, 1278–1280. doi:10.1126/science.1840701
Horn, L. A., Fousek, K., and Palena, C. (2020a). Tumor Plasticity and Resistance to Immunotherapy. Trends Cancer 6, 432–441. doi:10.1016/j.trecan.2020.02.001
Horn, L. A., Riskin, J., Hempel, H. A., Fousek, K., Lind, H., Hamilton, D. H., et al. (2020b). Simultaneous Inhibition of CXCR1/2, TGF-β, and PD-L1 Remodels the Tumor and its Microenvironment to Drive Antitumor Immunity. J. Immunother. Cancer 8, e000326. doi:10.1136/jitc-2019-000326
Hu, X., Yuan, L., and Ma, T. (2020). Mechanisms of JAK-STAT S-ignaling P-athway M-ediated by CXCL8 G-ene S-ilencing on E-pithelial-mesenchymal T-ransition of H-uman C-utaneous M-elanoma C-ells. Oncol. Lett. 20, 1973–1981. doi:10.3892/ol.2020.11706
Huang, L., Lian, J., Chen, X., Qin, G., Zheng, Y., and Zhang, Y. (2017). WASH Overexpression Enhances Cancer Stem Cell Properties and Correlates with Poor Prognosis of Esophageal Carcinoma. Cancer Sci. 108 (12), 2358–2365. doi:10.1111/cas.13400
Jia, L., Li, F., Shao, M., Zhang, W., Zhang, C., Zhao, X., et al. (2018). IL-8 Is Upregulated in Cervical Cancer Tissues and Is Associated with the Proliferation and Migration of HeLa Cervical Cancer Cells. Oncol. Lett. 15, 1350–1356. doi:10.3892/ol.2020.11706
Ju, L., Zhou, Z., Jiang, B., Lou, Y., and Guo, X. (2017). Autocrine VEGF and IL-8 Promote Migration via Src/Vav2/Rac1/PAK1 Signaling in Human Umbilical Vein Endothelial Cells. Cell Physiol Biochem 41, 1346–1359. doi:10.1159/000465389
Kahraman, D. C., Kahraman, T., and Cetin Atalay, R. (2019). Targeting PI3K/Akt/mTOR Pathway Identifies Differential Expression and Functional Role of IL-8 in Liver Cancer Stem Cell Enrichment. Mol. Cancer Ther. 18, 0004–2157. doi:10.1158/1535-7163.MCT-19-0004
Katoh, H., Wang, D., Daikoku, T., Sun, H., Dey, S. K., and Dubois, R. N. (2013). CXCR2-expressing Myeloid-Derived Suppressor Cells Are Essential to Promote Colitis-Associated Tumorigenesis. Cancer Cell 24, 631–644. doi:10.1016/j.ccr.2013.10.009
Kawano, M., Tanaka, K., Itonaga, I., Iwasaki, T., and Tsumura, H. (2018). Interaction between Human Osteosarcoma and Mesenchymal Stem Cells via an Interleukin-8 Signaling Loop in the Tumor Microenvironment. Cell Commun Signal 16, 13. doi:10.1186/s12964-018-0225-2
Kilic, I., Guldiken, S., Sipahi, T., Palabiyik, O., Akker, M., Celik, O., et al. (2016). Investigation of VEGF and IL-8 Gene Polymorphisms in Patients with Differentiated Thyroid Cancer. Clin. Lab. 62, 2319–2325. doi:10.7754/Clin.Lab.2016.160403
Kim, B., Seo, Y., Kwon, J. H., Shin, Y., Kim, S., Park, S. J., et al. (2021). IL‐6 and IL‐8, Secreted by Myofibroblasts in the Tumor Microenvironment, Activate HES1 to Expand the Cancer Stem Cell Population in Early Colorectal Tumor. Mol. Carcinogenesis 60, 188–200. doi:10.1002/mc.23283
Knall, C., Worthen, G. S., and Johnson, G. L. (1997). Interleukin 8-stimulated Phosphatidylinositol-3-Kinase Activity Regulates the Migration of Human Neutrophils Independent of Extracellular Signal-Regulated Kinase and P38 Mitogen-Activated Protein Kinases. Proc. Natl. Acad. Sci. 94, 3052–3057. doi:10.1073/pnas.94.7.3052
Knall, C., Young, S., Nick, J. A., Buhl, A. M., Worthen, G. S., and Johnson, G. L. (1996). Interleukin-8 Regulation of the Ras/Raf/mitogen-Activated Protein Kinase Pathway in Human Neutrophils. J. Biol. Chem. 271, 2832–2838. doi:10.1074/jbc.271.5.2832
Koensgen, D., Bruennert, D., Ungureanu, S., Sofroni, D., Braicu, E. I., Sehouli, J., et al. (2015). Polymorphism of the IL-8 Gene and the Risk of Ovarian Cancer. Cytokine 71, 334–338. doi:10.1016/j.cyto.2014.07.254
Krawczyk, K. M., Nilsson, H., Allaoui, R., Lindgren, D., Arvidsson, M., Leandersson, K., et al. (2017). Papillary Renal Cell Carcinoma-Derived Chemerin, IL-8, and CXCL16 Promote Monocyte Recruitment and Differentiation into Foam-Cell Macrophages. Lab. Invest. 97, 1296–1305. doi:10.1038/labinvest.2017.78
Kumar, S., O’Malley, J., Chaudhary, A. K., Inigo, J. R., Yadav, N., Kumar, R., et al. (2019). Hsp60 and IL-8 axis Promotes Apoptosis Resistance in Cancer. Br. J. Cancer 121, 934–943. doi:10.1038/s41416-019-0617-0
Larsson, C. (2006). Protein Kinase C and the Regulation of the Actin Cytoskeleton. Cell Signal. 18, 276–284. doi:10.1016/j.cellsig.2005.07.010
Li, A., Dubey, S., Varney, M. L., Dave, B. J., and Singh, R. K. (2003). IL-8 Directly Enhanced Endothelial Cell Survival, Proliferation, and Matrix Metalloproteinases Production and Regulated Angiogenesis. J. Immunol. 170, 3369–3376. doi:10.4049/jimmunol.170.6.3369
Li, E., Yang, X., Du, Y., Wang, G., Chan, D. W., Wu, D., et al. (2021). CXCL8 Associated Dendritic Cell Activation Marker Expression and Recruitment as Indicators of Favorable Outcomes in Colorectal Cancer. Front. Immunol. 12, 667177. doi:10.3389/fimmu.2021.667177
Li, P., Chen, X., Qin, G., Yue, D., Zhang, Z., Ping, Y., et al. (2018). Maelstrom Directs Myeloid-Derived Suppressor Cells to Promote Esophageal Squamous Cell Carcinoma Progression via Activation of the Akt1/RelA/IL8 Signaling Pathway. Cancer Immunol. Res. 6, 1246–1259. doi:10.1158/2326-6066.CIR-17-0415
Lin, C., He, H., Liu, H., Li, R., Chen, Y., Qi, Y., et al. (2019). Tumour-associated Macrophages-Derived CXCL8 Determines Immune Evasion through Autonomous PD-L1 Expression in Gastric Cancer. Gut 68, 1764–1773. doi:10.1136/gutjnl-2018-316324
Liu, K., Wu, L., Yuan, S., Wu, M., Xu, Y., Sun, Q., et al. (2020). Structural Basis of CXC Chemokine Receptor 2 Activation and Signalling. Nature 585, 135–140. doi:10.1038/s41586-020-2492-5
Liu, Q., Li, A., Tian, Y., Wu, J. D., Liu, Y., Li, T., et al. (2016). The CXCL8-Cxcr1/2 Pathways in Cancer. Cytokine Growth Factor. Rev. 31, 61–71. doi:10.1016/j.cytogfr.2016.08.002
Long, X., Ye, Y., Zhang, L., Liu, P., Yu, W., Wei, F., et al. (2016). IL-8, a Novel Messenger to Cross-Link Inflammation and Tumor EMT via Autocrine and Paracrine Pathways (Review). Int. J. Oncol. 48, 5–12. doi:10.3892/ijo.2015.3234
Loos, T., Opdenakker, G., Van Damme, J., and Proost, P. (2009). Citrullination of CXCL8 Increases This Chemokine's Ability to Mobilize Neutrophils into the Blood Circulation. Haematologica 94, 1346–1353. doi:10.3324/haematol.2009.006973
Luo, C.-W., Hsiao, I.-L., Wang, J.-Y., Wu, C.-C., Hung, W.-C., Lin, Y.-H., et al. (2018). Cell Motility Facilitated by Mono(2-Ethylhexyl) Phthalate via Activation of the AKT−β-Catenin-IL-8 Axis in Colorectal Cancer. J. Agric. Food Chem. 66, 9635–9644. doi:10.1021/acs.jafc.8b03558
Luppi, F., Longo, A. M., de Boer, W. I., Rabe, K. F., and Hiemstra, P. S. (2007). Interleukin-8 Stimulates Cell Proliferation in Non-small Cell Lung Cancer through Epidermal Growth Factor Receptor Transactivation. Lung Cancer 56, 25–33. doi:10.1016/j.lungcan.2006.11.014
Ma, X., Chen, J., Liu, J., Xu, B., Liang, X., Yang, X., et al. (2021). IL‐8/CXCR2 Mediates Tropism of Human Bone Marrow‐derived Mesenchymal Stem Cells toward CD133 +/CD44 + Colon Cancer Stem Cells. J. Cel Physiol 236, 3114–3128. doi:10.1002/jcp.30080
Ma, X., Liu, J., Yang, X., Fang, K., Zheng, P., Liang, X., et al. (2020). Mesenchymal Stem Cells Maintain the Stemness of colon Cancer Stem Cells via Interleukin-8/mitogen-Activated Protein Kinase Signaling Pathway. Exp. Biol. Med. (Maywood) 245, 562–575. doi:10.1177/1535370220910690
MacManus, C. F., Pettigrew, J., Seaton, A., Wilson, C., Maxwell, P. J., Berlingeri, S., et al. (2007). Interleukin-8 Signaling Promotes Translational Regulation of Cyclin D in Androgen-independent Prostate Cancer Cells. Mol. Cancer Res. 5, 737–748. doi:10.1158/1541-7786.MCR-07-0032
Manfroi, B., McKee, T., Mayol, J. F., Tabruyn, S., Moret, S., Villiers, C., et al. (2017). CXCL-8/IL8 Produced by Diffuse Large B-Cell Lymphomas Recruits Neutrophils Expressing a Proliferation-Inducing Ligand APRIL. Cancer Res. 77, 1097–1107. doi:10.1158/0008-5472.CAN-16-0786
Mantovani, A., Marchesi, F., Malesci, A., Laghi, L., and Allavena, P. (2017). Tumour-associated Macrophages as Treatment Targets in Oncology. Nat. Rev. Clin. Oncol. 14, 399–416. doi:10.1038/nrclinonc.2016.217
Mao, F.-y., Zhao, Y.-l., Lv, Y.-p., Teng, Y.-s., Kong, H., Liu, Y.-g., et al. (2018). CD45+CD33lowCD11bdim Myeloid-Derived Suppressor Cells Suppress CD8+ T Cell Activity via the IL-6/IL-8-arginase I axis in Human Gastric Cancer. Cell Death Dis 9, 763. doi:10.1038/s41419-018-0803-7
Martin, D., Galisteo, R., and Gutkind, J. S. (2009). CXCL8/IL8 Stimulates Vascular Endothelial Growth Factor (VEGF) Expression and the Autocrine Activation of VEGFR2 in Endothelial Cells by Activating NFκB through the CBM (Carma3/Bcl10/Malt1) Complex. J. Biol. Chem. 284, 6038–6042. doi:10.1074/jbc.C800207200
Maxwell, P. J., Coulter, J., Walker, S. M., McKechnie, M., Neisen, J., McCabe, N., et al. (2013). Potentiation of Inflammatory CXCL8 Signalling Sustains Cell Survival in PTEN-Deficient Prostate Carcinoma. Eur. Urol. 64, 177–188. doi:10.1016/j.eururo.2012.08.032
Maynard, J. P., Ertunc, O., Kulac, I., Baena-Del Valle, J. A., De Marzo, A. M., and Sfanos, K. S. (2020). IL8 Expression Is Associated with Prostate Cancer Aggressiveness and Androgen Receptor Loss in Primary and Metastatic Prostate Cancer. Mol. Cancer Res. 18, 153–165. doi:10.1158/1541-7786.MCR-19-0595
McCoy, M. G., Nyanyo, D., Hung, C. K., Goerger, J. P., R. Zipfel, W., Williams, R. M., et al. (2019). Endothelial Cells Promote 3D Invasion of GBM by IL-8-dependent Induction of Cancer Stem Cell Properties. Sci. Rep. 9, 9069. doi:10.1038/s41598-019-45535-y
Meacham, C. E., and Morrison, S. J. (2013). Tumour Heterogeneity and Cancer Cell Plasticity. Nature 501, 328–337. doi:10.1038/nature12624
Mi, Y., Mu, L., Huang, K., Hu, Y., Yan, C., Zhao, H., et al. (2020). Hypoxic Colorectal Cancer Cells Promote Metastasis of Normoxic Cancer Cells Depending on IL-8/p65 Signaling Pathway. Cel Death Dis 11, 610. doi:10.1038/s41419-020-02797-z
Mishalian, I., Bayuh, R., Eruslanov, E., Michaeli, J., Levy, L., Zolotarov, L., et al. (2014). Neutrophils Recruit Regulatory T-Cells into Tumorsviasecretion of CCL17-A New Mechanism of Impaired Antitumor Immunity. Int. J. Cancer 135, 1178–1186. doi:10.1002/ijc.28770
Modi, W., Dean, M., Seuanez, H., Mukaida, N., Matsushima, K., and O'Brien, S. (1990). Monocyte-derived Neutrophil Chemotactic Factor (MDNCF/IL-8) Resides in a Gene Cluster along with Several Other Members of the Platelet Factor 4 Gene Superfamily. Hum. Genet. 84, 185–187. doi:10.1007/BF00208938
Mohamed, H. T., El-Ghonaimy, E. A., El-Shinawi, M., Hosney, M., Götte, M., Woodward, W. A., et al. (2020). IL-8 and MCP-1/CCL2 Regulate Proteolytic Activity in Triple Negative Inflammatory Breast Cancer a Mechanism that Might Be Modulated by Src and Erk1/2. Toxicol. Appl. Pharmacol. 401, 115092. doi:10.1016/j.taap.2020.115092
Mortier, A., Berghmans, N., Ronsse, I., Grauwen, K., Stegen, S., Van Damme, J., et al. (2011). Biological Activity of CXCL8 Forms Generated by Alternative Cleavage of the Signal Peptide or by Aminopeptidase-Mediated Truncation. PLoS One 6, e23913. doi:10.1371/journal.pone.0023913
Mukaida, N., Shiroo, M., and Matsushima, K. (1989). Genomic Structure of the Human Monocyte-Derived Neutrophil Chemotactic Factor IL-8. J. Immunol. 143, 1366–1371.
Murphy, P. M., and Tiffany, H. L. (1991). Cloning of Complementary DNA Encoding a Functional Human Interleukin-8 Receptor. Science 253, 1280–1283. doi:10.1126/science.1891716
Najjar, Y. G., Rayman, P., Jia, X., Pavicic, P. G., Rini, B. I., Tannenbaum, C., et al. (2017). Myeloid-Derived Suppressor Cell Subset Accumulation in Renal Cell Carcinoma Parenchyma Is Associated with Intratumoral Expression of IL1β, IL8, CXCL5, and Mip-1α. Clin. Cancer Res. 23, 2346–2355. doi:10.1158/1078-0432.CCR-15-1823
Nasser, M. W., Raghuwanshi, S. K., Grant, D. J., Jala, V. R., Rajarathnam, K., and Richardson, R. M. (2009). Differential Activation and Regulation of CXCR1 and CXCR2 by CXCL8 Monomer and Dimer. J. Immunol. 183, 3425–3432. doi:10.4049/jimmunol.0900305
Nie, M., Yang, L., Bi, X., Wang, Y., Sun, P., Yang, H., et al. (2019). Neutrophil Extracellular Traps Induced by IL8 Promote Diffuse Large B-Cell Lymphoma Progression via the TLR9 Signaling. Clin. Cancer Res. 25, 1867–1879. doi:10.1158/1078-0432.CCR-18-1226
Ning, Y., Cui, Y., Li, X., Cao, X., Chen, A., Xu, C., et al. (2018). Co-culture of Ovarian Cancer Stem-like Cells with Macrophages Induced SKOV3 Cells Stemness via IL-8/STAT3 Signaling. Biomed. Pharmacother. 103, 262–271. doi:10.1016/j.biopha.2018.04.022
Nourbakhsh, M., Kälble, S., Dörrie, A., Hauser, H., Resch, K., and Kracht, M. (2001). The NF-Κb Repressing Factor Is Involved in Basal Repression and Interleukin (IL)-1-induced Activation of IL-8 Transcription by Binding to a Conserved NF-Κb-Flanking Sequence Element. J. Biol. Chem. 276, 4501–4508. doi:10.1074/jbc.M007532200
Noy, R., and Pollard, J. W. (2014). Tumor-associated Macrophages: from Mechanisms to Therapy. Immunity 41, 49–61. doi:10.1016/j.immuni.2014.06.010
Nozawa, H., Chiu, C., and Hanahan, D. (2006). Infiltrating Neutrophils Mediate the Initial Angiogenic Switch in a Mouse Model of Multistage Carcinogenesis. Proc. Natl. Acad. Sci. 103, 12493–12498. doi:10.1073/pnas.0601807103
Park, S. H., Das, B. B., Casagrande, F., Tian, Y., Nothnagel, H. J., Chu, M., et al. (2012). Structure of the Chemokine Receptor CXCR1 in Phospholipid Bilayers. Nature 491, 779–783. doi:10.1038/nature11580
Petreaca, M. L., Yao, M., Liu, Y., Defea, K., and Martins-Green, M. (2007). Transactivation of Vascular Endothelial Growth Factor Receptor-2 by Interleukin-8 (IL-8/CXCL8) Is Required for IL-8/CXCL8-induced Endothelial Permeability. MBoC 18, 5014–5023. doi:10.1091/mbc.e07-01-0004
Poschke, I., and Kiessling, R. (2012). On the Armament and Appearances of Human Myeloid-Derived Suppressor Cells. Clin. Immunol. 144, 250–268. doi:10.1016/j.clim.2012.06.003
Proost, P., Loos, T., Mortier, A., Schutyser, E., Gouwy, M., Noppen, S., et al. (2008). Citrullination of CXCL8 by Peptidylarginine Deiminase Alters Receptor Usage, Prevents Proteolysis, and Dampens Tissue Inflammation. J. Exp. Med. 205, 2085–2097. doi:10.1084/jem.20080305
Quann, E. J., Liu, X., Altan-Bonnet, G., and Huse, M. (2011). A cascade of Protein Kinase C Isozymes Promotes Cytoskeletal Polarization in T Cells. Nat. Immunol. 12, 647–654. doi:10.1038/ni.2033
Rafrafi, A., Chahed, B., Kaabachi, S., Kaabachi, W., Maalmi, H., Hamzaoui, K., et al. (2013). Association of IL-8 Gene Polymorphisms with Non Small Cell Lung Cancer in Tunisia: A Case Control Study. Hum. Immunol. 74, 1368–1374. doi:10.1016/j.humimm.2013.06.033
Raghuwanshi, S. K., Su, Y., Singh, V., Haynes, K., Richmond, A., and Richardson, R. M. (2012). The Chemokine Receptors CXCR1 and CXCR2 Couple to Distinct G Protein-Coupled Receptor Kinases to Mediate and Regulate Leukocyte Functions. J.I. 189, 2824–2832. doi:10.4049/jimmunol.1201114
Raza, S., Rajak, S., Tewari, A., Gupta, P., Chattopadhyay, N., Sinha, R. A., et al. (2022). Multifaceted Role of Chemokines in Solid Tumors: From Biology to Therapy. Semin. Cancer Biol. S1044-579X, 00304–00307. doi:10.1016/j.semcancer.2021.12.011
Ribas, A., and Wolchok, J. D. (2018). Cancer Immunotherapy Using Checkpoint Blockade. Science 359, 1350–1355. doi:10.1126/science.aar4060
Rodriguez, P. C., Quiceno, D. G., Zabaleta, J., Ortiz, B., Zea, A. H., Piazuelo, M. B., et al. (2004). Arginase I Production in the Tumor Microenvironment by Mature Myeloid Cells Inhibits T-Cell Receptor Expression and Antigen-specific T-Cell Responses. Cancer Res. 64, 5839–5849. doi:10.1158/0008-5472.CAN-04-0465
Roskoski, R. (2015). Src Protein-Tyrosine Kinase Structure, Mechanism, and Small Molecule Inhibitors. Pharmacol. Res. 94, 9–25. doi:10.1016/j.phrs.2015.01.003
Sanmamed, M. F., Perez-Gracia, J. L., Schalper, K. A., Fusco, J. P., Gonzalez, A., Rodriguez-Ruiz, M. E., et al. (2017). Changes in Serum Interleukin-8 (IL-8) Levels Reflect and Predict Response to Anti-PD-1 Treatment in Melanoma and Non-small-cell Lung Cancer Patients. Ann. Oncol. 28, 1988–1995. doi:10.1093/annonc/mdx190
Schalper, K. A., Carleton, M., Zhou, M., Chen, T., Feng, Y., Huang, S.-P., et al. (2020). Elevated Serum Interleukin-8 Is Associated with Enhanced Intratumor Neutrophils and Reduced Clinical Benefit of Immune-Checkpoint Inhibitors. Nat. Med. 26, 688–692. doi:10.1038/s41591-020-0856-x
Schraufstatter, I. U., Chung, J., and Burger, M. (2001). IL-8 Activates Endothelial Cell CXCR1 and CXCR2 through Rho and Rac Signaling Pathways. Am. J. Physiology-Lung Cell Mol. Physiol. 280, L1094–L1103. doi:10.1152/ajplung.2001.280.6.L1094
Shimizu, M., and Tanaka, N. (2019). IL-8-induced O-GlcNAc Modification via GLUT3 and GFAT Regulates Cancer Stem Cell-like Properties in colon and Lung Cancer Cells. Oncogene 38, 1520–1533. doi:10.1038/s41388-018-0533-4
Singh, J. K., Farnie, G., Bundred, N. J., Simões, B. M., Shergill, A., Landberg, G., et al. (2013). Targeting CXCR1/2 Significantly Reduces Breast Cancer Stem Cell Activity and Increases the Efficacy of Inhibiting HER2 via HER2-dependent and -independent Mechanisms. Clin. Cancer Res. 19, 643–656. doi:10.1158/1078-0432.CCR-12-1063
Son, D. O., Satsu, H., Kiso, Y., Totsuka, M., and Shimizu, M. (2008). Inhibitory Effect of Carnosine on Interleukin-8 Production in Intestinal Epithelial Cells through Translational Regulation. Cytokine 42, 265–276. doi:10.1016/j.cyto.2008.02.011
Stillie, R., Farooq, S. M., Gordon, J. R., and Stadnyk, A. W. (2009). The Functional Significance behind Expressing Two IL-8 Receptor Types on PMN. J. Leukoc. Biol. 86, 529–543. doi:10.1189/jlb.0208125
Strieter, R. M., Polverini, P. J., Kunkel, S. L., Arenberg, D. A., Burdick, M. D., Kasper, J., et al. (1995). The Functional Role of the ELR Motif in CXC Chemokine-Mediated Angiogenesis. J. Biol. Chem. 270, 27348–27357. doi:10.1074/jbc.270.45.27348
Sulzmaier, F. J., Jean, C., and Schlaepfer, D. D. (2014). FAK in Cancer: Mechanistic Findings and Clinical Applications. Nat. Rev. Cancer 14, 598–610. doi:10.1038/nrc3792
Sun, L., Clavijo, P. E., Robbins, Y., Patel, P., Friedman, J., Greene, S., et al. (2019a). Inhibiting Myeloid-Derived Suppressor Cell Trafficking Enhances T Cell Immunotherapy. JCI Insight 4. doi:10.1172/jci.insight.126853
Sun, Y., Ai, J. Z., Jin, X., Liu, L. R., Lin, T. H., Xu, H., et al. (2019b). IL‐8 Protects Prostate Cancer Cells from GSK‐3β‐induced Oxidative Stress by Activating the mTOR Signaling Pathway. Prostate 79, 1180–1190. doi:10.1002/pros.23836
Tobin, R. P., Jordan, K. R., Kapoor, P., Spongberg, E., Davis, D., Vorwald, V. M., et al. (2019). IL-6 and IL-8 Are Linked with Myeloid-Derived Suppressor Cell Accumulation and Correlate with Poor Clinical Outcomes in Melanoma Patients. Front. Oncol. 9, 1223. doi:10.3389/fonc.2019.01223
Ueda, G., Matsuo, Y., Murase, H., Aoyama, Y., Kato, T., Omi, K., et al. (2022). 10Z-Hymenialdisine I-nhibits A-ngiogenesis by S-uppressing NF-κB A-ctivation in P-ancreatic C-ancer C-ell L-ines. Oncol. Rep. 47, 48. doi:10.3892/or.2022.8259
Van den Steen, P. E., Wuyts, A., Husson, S. J., Proost, P., Van Damme, J., and Opdenakker, G. (2003). Gelatinase B/MMP-9 and Neutrophil collagenase/MMP-8 Process the Chemokines Human GCP-2/CXCL6, ENA-78/CXCL5 and Mouse GCP-2/LIX and Modulate Their Physiological Activities. Eur. J. Biochem. 270, 3739–3749. doi:10.1046/j.1432-1033.2003.03760.x
Vanheule, V., Metzemaekers, M., Janssens, R., Struyf, S., and Proost, P. (2018). How post-translational Modifications Influence the Biological Activity of Chemokines. Cytokine 109, 29–51. doi:10.1016/j.cyto.2018.02.026
Venkatakrishnan, G., Salgia, R., and Groopman, J. E. (2000). Chemokine Receptors CXCR-1/2 Activate Mitogen-Activated Protein Kinase via the Epidermal Growth Factor Receptor in Ovarian Cancer Cells. J. Biol. Chem. 275, 6868–6875. doi:10.1074/jbc.275.10.6868
Wang, J., Wang, Y., Wang, S., Cai, J., Shi, J., Sui, X., et al. (2015). Bone Marrow-Derived Mesenchymal Stem Cell-Secreted IL-8 Promotes the Angiogenesis and Growth of Colorectal Cancer. Oncotarget 6, 42825–42837. doi:10.18632/oncotarget.5739
Waugh, D. J. J., and Wilson, C. (2008). The Interleukin-8 Pathway in Cancer. Clin. Cancer Res. 14, 6735–6741. doi:10.1158/1078-0432.CCR-07-4843
Wilson, C., Seaton, A., and Maxwell, P. J. (2006). CXCR2/NF-kB/BCL-2-survivin Signalling Pathway Promotes Resistance to Oxaliplatin in Metastatic Prostate Cancer Cells. Proceedings of 99th AACR Annual Meeting. Washington (DC).
Wu, G. D., Lai, E. J., Huang, N., and Wen, X. (1997). Oct-1 and CCAAT/Enhancer-binding Protein (C/EBP) Bind to Overlapping Elements within the Interleukin-8 Promoter. J. Biol. Chem. 272, 2396–2403. doi:10.1074/jbc.272.4.2396
Wu, J., Gao, F.-x., Wang, C., Qin, M., Han, F., Xu, T., et al. (2019). IL-6 and IL-8 Secreted by Tumour Cells Impair the Function of NK Cells via the STAT3 Pathway in Oesophageal Squamous Cell Carcinoma. J. Exp. Clin. Cancer Res. 38, 321. doi:10.1186/s13046-019-1310-0
Xu, H., Zeng, Y., Liu, L., Gao, Q., Jin, S., Lan, Q., et al. (2017). PRL-3 Improves Colorectal Cancer Cell Proliferation and Invasion through IL-8 Mediated Glycolysis Metabolism. Int. J. Oncol. 51, 1271–1279. doi:10.3892/ijo.2017.4090
Xu, Q., Ma, H., Chang, H., Feng, Z., Zhang, C., and Yang, X. (2020). The Interaction of Interleukin-8 and PTEN Inactivation Promotes the Malignant Progression of Head and Neck Squamous Cell Carcinoma via the STAT3 Pathway. Cel Death Dis 11, 405. doi:10.1038/s41419-020-2627-5
Yan, Z., Liu, J., Xie, L., Liu, X., Zeng, Y., and Yang, X. (2016). Role of Heparan Sulfate in Mediating CXCL8-Induced Endothelial Cell Migration. PeerJ 4, e1669. doi:10.1038/s41419-020-2627-510.7717/peerj.1669
Yang, L., Liu, L., Zhang, R., Hong, J., Wang, Y., Wang, J., et al. (2020a). IL-8 Mediates a Positive Loop Connecting Increased Neutrophil Extracellular Traps (NETs) and Colorectal Cancer Liver Metastasis. J. Cancer 11, 4384–4396. doi:10.7150/jca.44215
Yang, M., Zhang, G., Wang, Y., He, M., Xu, Q., Lu, J., et al. (2020b). Tumour-associated Neutrophils Orchestrate Intratumoural IL-8-driven Immune Evasion through Jagged2 Activation in Ovarian Cancer. Br. J. Cancer 123, 1404–1416. doi:10.1038/s41416-020-1026-0
Yao, W., Sun, Y., Sun, Y., Chen, P., Meng, Z., Xiao, M., et al. (2019). A Preliminary Report of the Relationship between Gene Polymorphism of IL-8 and its Receptors and Systemic Inflammatory Response Syndrome Caused by Wasp Stings. DNA Cel Biol. 38, 1512–1518. doi:10.1089/dna.2019.4855
Yuen, K. C., Liu, L.-F., Gupta, V., Madireddi, S., Keerthivasan, S., Li, C., et al. (2020). High Systemic and Tumor-Associated IL-8 Correlates with Reduced Clinical Benefit of PD-L1 Blockade. Nat. Med. 26, 693–698. doi:10.1038/s41591-020-0860-1
Zha, C., Meng, X., Li, L., Mi, S., Qian, D., Li, Z., et al. (2020). Neutrophil Extracellular Traps Mediate the Crosstalk between Glioma Progression and the Tumor Microenvironment via the HMGB1/RAGE/IL-8 axis. Cancer Biol. Med. 17, 154–168. doi:10.20892/j.issn.2095-3941.2019.0353
Zhang, H., Ye, Y.-L., Li, M.-X., Ye, S.-B., Huang, W.-R., Cai, T.-T., et al. (2017). CXCL2/MIF-CXCR2 Signaling Promotes the Recruitment of Myeloid-Derived Suppressor Cells and Is Correlated with Prognosis in Bladder Cancer. Oncogene 36, 2095–2104. doi:10.1038/onc.2016.367
Zhang, M., Huang, L., Ding, G., Huang, H., Cao, G., Sun, X., et al. (2020). Interferon Gamma Inhibits CXCL8-CXCR2 axis Mediated Tumor-Associated Macrophages Tumor Trafficking and Enhances Anti-PD1 Efficacy in Pancreatic Cancer. J. Immunother. Cancer 8, e000308. doi:10.1136/jitc-2019-000308
Zheng, T., Ma, G., Tang, M., Li, Z., and Xu, R. (2018). IL-8 Secreted from M2 Macrophages Promoted Prostate Tumorigenesis via STAT3/MALAT1 Pathway. Ijms 20, 98. doi:10.3390/ijms20010098
Zhou, J., Yi, L., Ouyang, Q., Xu, L., Cui, H., and Xu, M. (2014). Neurotensin Signaling Regulates Stem-like Traits of Glioblastoma Stem Cells through Activation of IL-8/CXCR1/STAT3 Pathway. Cell Signal. 26, 2896–2902. doi:10.1016/j.cellsig.2014.08.027
Keywords: CXCL8, tumor microenvironment, tumor progression, tumor immune suppression, immunotherapy
Citation: Xiong X, Liao X, Qiu S, Xu H, Zhang S, Wang S, Ai J and Yang L (2022) CXCL8 in Tumor Biology and Its Implications for Clinical Translation. Front. Mol. Biosci. 9:723846. doi: 10.3389/fmolb.2022.723846
Received: 11 June 2021; Accepted: 21 February 2022;
Published: 15 March 2022.
Edited by:
José Alexandre Ferreira, Portuguese Oncology Institute, PortugalCopyright © 2022 Xiong, Liao, Qiu, Xu, Zhang, Wang, Ai and Yang. This is an open-access article distributed under the terms of the Creative Commons Attribution License (CC BY). The use, distribution or reproduction in other forums is permitted, provided the original author(s) and the copyright owner(s) are credited and that the original publication in this journal is cited, in accordance with accepted academic practice. No use, distribution or reproduction is permitted which does not comply with these terms.
*Correspondence: Jianzhong Ai, YWp6NjM2M0AxMjYuY29t; Lu Yang, d3ljbGVmbHVlQDE2My5jb20=
†These authors have contributed equally to this work