- 1Department of Anesthesiology, School of Medicine, University of California, San Diego, CA, United States
- 2Department of Bioengineering, University of California, San Diego, CA, United States
- 3Instituto do Coração, Hospital das Clínicas, Faculdade de Medicina, Universidade de São Paulo (InCor-FMUSP), São Paulo, Brazil
- 4Skaggs School of Pharmacy and Pharmaceutical Sciences, University of California, San Diego, CA, United States
- 5Department of Pediatrics, School of Medicine, University of California, San Diego, CA, United States
- 6Department of Medicine, School of Medicine, University of California, San Diego, CA, United States
- 7Instituto de Ciência e Tecnologia, Universidade Federal de São Paulo, São Josê dos Campos, Brazil
- 8Department of Anesthesiology and Critical Care, VA San Diego Healthcare System, San Diego, CA, United States
Introduction: Infection by SARS-CoV-2 and subsequent COVID-19 can cause viral sepsis. We investigated plasma protease activity patterns in COVID-19-induced sepsis with bacterial superinfection, as well as plasma proteomics and peptidomics in order to assess the possible implications of enhanced proteolysis on major protein systems (e.g., coagulation).
Methods: Patients (=4) admitted to the intensive care units (ICUs) at the University of California, San Diego (UCSD) Medical Center with confirmed positive test for COVID-19 by real-time reverse transcription polymerase chain reaction (RT-PCR) were enrolled in a study approved by the UCSD Institutional Review Board (IRB# 190699, Protocol #20-0006). Informed consent was obtained for the collection of blood samples and de-identified use of the data. Blood samples were collected at multiple time points and analyzed to quantify a) the circulating proteome and peptidome by mass spectrometry; b) the aminopeptidase activity in plasma; and c) the endopeptidase activity in plasma using fluorogenic substrates that are cleaved by trypsin-like endopeptidases, specific clotting factors and plasmin. The one patient who died was diagnosed with bacterial superinfection on day 7 after beginning of the study.
Results: Spikes in protease activity (factor VII, trypsin-like activity), and corresponding increases in the intensity of peptides derived by hydrolysis of plasma proteins, especially of fibrinogen degradation products and downregulation of endogenous protease inhibitors were detected on day 7 for the patient who died. The activity of the analyzed proteases was stable in survivors.
Discussion: The combination of multiomics and enzymatic activity quantification enabled to i) hypothesize that elevated proteolysis occurs in COVID-19-induced septic shock with bacterial superinfection, and ii) provide additional insight into malfunctioning protease-mediated systems, such as hemostasis.
1 Introduction
The Coronavirus disease (COVID-19) pandemic is caused by viral infection with the coronavirus SARS-CoV-2. Affecting every organ of the body following initial infection of the respiratory system, this highly contagious virus has contributed to more than 6 million confirmed deaths to date. Despite the emphasis on COVID-19 research, our fundamental understanding of the disease remains incomplete and therapeutic options are limited (Osuchowski et al., 2020; Wiersing et al., 2020; Alhazzani et al., 2021; Chalmers et al., 2021; Lopes-Pacheco et al., 2021; Osuchowski et al., 2021; Welte et al., 2021). Frequent and fatal complications arising from SARS-CoV-2-induced sepsis include cytokine storm and coagulopathies (Iba et al., 2020; Tang et al., 2020). Less frequent (Garcia-Vidal et al., 2021), but not less dangerous, bacterial superinfections make the clinical treatment of COVID-19 patients in the intensive care unit (ICU) even more challenging, especially because of the unclear underlying pathology and interplay between viral and bacterial infection and their clinical implications (Lazzaroni et al., 2021).
We have previously shown, in both animal and human studies, that dysregulated proteolysis is a possible, additional pathological mechanism triggered by circulatory shock, including septic shock following bacterial infection (Aletti et al., 2016a; Aletti et al., 2016b; Bauzá-Martinez et al., 2018; Aletti et al., 2022). Several protease-mediated systems, such as the coagulation and complement systems, are known to be compromised in critical illness. To better understand the possible relationship between systemic proteolysis occurring in shock, including COVID-19-induced septic shock, and the malfunction of major protease-mediated systems, we have developed a complex, multimodal approach to assess protease activity and proteolysis in plasma, consisting of quantitative protease activity assays and mass spectrometry-based multi-omics analyses of the circulating proteome and peptidome. The combined use of these techniques has shown promise in the ability to i) estimate in vivo proteolysis in plasma as a marker of ongoing systemic proteolysis during shock (Aletti et al., 2016b; Bauzá-Martinez et al., 2018), and ii) detect circulating peptidase activity (Maffioli et al., 2020).
The broad hypothesis of this study was that a complex description of plasma proteins and peptides and of the activity of some of the most important circulating enzymes can be leveraged as a tool to shed light on the progression of COVID-19 and the risk for fatal complications, especially in the presence of bacterial superinfection and diagnosis of septic shock. In particular, we postulated that these measurements, which are not part of the clinical routine, would provide important pathophysiological information on the COVID-19-induced alterations of an all-important proteolytically controlled system such as hemostasis.
The specific goal was to propose a complex, multi-omics and multidata approach for the longitudinal description of the proteome, peptidome and the activity of several plasma peptidases able to assess: 1) the patterns of protease activity in the plasma of COVID-19 patients following admission to the ICU and their association with the development of bacterial superinfection by means of quantitative analysis of enzymatic activity, and 2) the implications of dysregulated proteolysis on important protein systems, such as hemostasis mediated both by coagulation factors and proteases involved in fibrinolysis, by analysis of plasma proteomics and peptidomics during the ICU stay of the participants.
2 Materials and methods
2.1 Clinical measurements
This was a prospective observational study. The University of California, San Diego Institutional Review Board (IRB) provided approval of the study conduct (IRB# 190699, Protocol #20-0006); informed consent was obtained for the collection of blood samples and de-identified use of the data. A convenience sample of patients (n = 4) admitted to the Intensive Care Units (ICUs) at the University of California, San Diego Medical Center with confirmed positive test for COVID-19 by real-time reverse transcription polymerase chain reaction (RT-PCR) was enrolled in the study. De-identified demographic and prior health history data were obtained. Three healthy subjects (male, age 35–56) were enrolled as a control group for protease activity assays. Standard laboratory measurements as necessary for optimal patient care were obtained by the UC San Diego Medical Center for Advanced Laboratory Medicine (CALM) laboratories. All non-standard laboratory assays were conducted off-site in academic research laboratories (protease activity measurements) and in the Biomolecular and Proteomics Mass Spectrometry Facility (mass spectrometry) at the University of California, San Diego, with investigators blinded to patient clinical course and outcomes. Patient blood samples (3–10 mL) were collected in sodium heparin (BD Vacutainer, REF: 366480) tubes. To prevent coagulation, the tubes were inverted 10 times prior to transport at room temperature. Blood was processed within 4 h of collection and kept at room temperature throughout the protocol. PolymorphprepTM was added to collected samples and centrifuged at 500 g × 30 min to separate plasma from white blood cells per manufacturer’s instructions (Progen) and transferred to new Eppendorf tubes and centrifuged at ×3,731 g for 5 min to remove any cellular debris. The supernatant was transferred to new tubes and flash frozen in dry ice and 95% ethanol. Plasma was aliquoted and stored at −80°C for further analysis. The protocol for the preprocessing, storage and subsequent analysis of the samples via mass spectrometry (see Section 2.3) ensured that all the samples would be analyzed under the same conditions and to avoid possible bias effects due to sample processing. The clinical data acquisition and blood sample collection started on the day following protocol study consent (usually day of ICU admission) and was repeated on the following day and every other day thereafter until patient discharge or death.
2.2 Plasma enzymatic activity
To test enzymatic activity, a variety of fluorogenic substrates was selected, with a specific focus on the activity of endopeptidases such as trypsin-like enzymes, clotting factors (thrombin, factors VII, IX and X), fibrinolytic enzymes (e.g., plasmin) and aminopeptidases. All plasma samples were processed and analyzed simultaneously for each substrate, including healthy control plasma. Briefly, a 384-well plate was used to combine 15 μL of plasma (1/50 final dilution in PBS, pH = 7.4) with 15 μL of each substrate (5 μM in PBS) in triplicate wells and immediately measured for activity with a spectrophotometer (FilterMax F5 Multi-Mode, Molecular Devices) with excitation of 360 nm and emission of 460 nm. Wells were read every 2 min and 45 s for 4 h and 50 min thereby generating fluorescent activity progress curves that each contain 100 data points. Fluorogenic activity was reported as relative fluorescence units per second using the slope from the progress curve where the r2 value was higher than .98. The activity was corrected by the dilution factor. Results shown are plotted as the percentage of enzymatic activity relative to the average of healthy control plasma samples on a log2 scale, so that the large intersubject variability is appropriately taken into consideration. The peptide sequences used in this assay were chosen as substrates for enzymes involved in the regulation of coagulation and hemostasis, tryptic-like endopeptidases and aminopeptidases. (Table 1).
2.3 Proteomics and peptidomics analysis of plasma via mass spectrometry
All plasma samples from the COVID-19 patients were subjected to a series of protein extraction and pretreatment steps prior to mass spectrometry. Briefly, for proteomics measurements, proteins were precipitated from the plasma samples, treated with denaturing, reducing, and alkylating reagents, and enzymatically digested with trypsin. To quantify peptides in plasma, high molecular weight proteins were removed following addition of methanol (without any tryptic digestion step in order to avoid ex vivo protein hydrolysis); the remaining peptides (exclusively generated by in vivo proteolysis) were quantified by tandem mass spectrometry (LC-MS/MS) using nano-spray ionization with a Orbitrap fusion Lumos hybrid mass spectrometer (Thermo Fisher Scientific). The sample preparation procedure both for proteomics and peptidomics and the setting of the mass spectrometry parameters followed established and previously described protocols (Courelli et al., 2021). PEAKS Studio 8.5 (Bioinformatics Solutions, Inc.) proteomics software was used for protein identification and quantification.
For the purposes of this study, the analysis of the circulating proteome and peptidome focused on three main groups of proteins, according to their function: 1) coagulation, e.g. clotting factors and fibrinogen; 2) complement system and proteins involved in the immune response and inflammation, e.g. complement factors, vascular endothelial growth factor receptor 2 (VEGF2) and C-reactive protein, and 3) endogenous protease inhibitors, e.g., serpins such as trypsin and chymotrypsin inhibitors, thrombin and plasmin inhibitors, C1 inhibitors and heparin cofactor II.
For proteome analysis, we used the log2 ratio of each individual intensity measurement to the average of all sample intensities for each protein. Heat maps for the three protein function groups described above were generated by Morpheus (https://software.broadinstitute.org/morpheus). Identified proteins were hierarchically clustered with the one minus Pearson’s correlation metric and average linkage method for each protein group. Columns were sorted by patient and ordered by day of sample collection. For peptidomic analysis, the degree of overall proteolysis was estimated by the sum of all fragment intensities in a sample. Similarly, the proteolysis of a single protein was estimated by the sum of the intensities of the peptides generated by that protein. An online web tool, Peptigram (@Bioware—© University College Dublin) (Manguy et al., 2017) was used to build cleavage maps representative of the number of peptides generated by a parent protein, abundance and length of each amino acid sequence, and cleavage site on the parent protein of origin.
3 Results
Patient characteristics are shown in Table 2.
The four patients were comprised of three males and one female and ranged in age from 29 to 88 years. Reflecting the overall demographics of the COVID-19 epidemic in San Diego, three of the subjects were Hispanic. One patient (88-year-old female), who will be referred to as “Patient 3” in the following, was diagnosed with bacterial superinfection and subsequent sepsis on day 7 and died on day 16 of her ICU stay. The remainder (Patients 1, 2 and 4) were discharged from the ICU with a mean ICU length-of-stay for all subjects of 11.25 ± 6.55 days and mean hospital stay of 15.25 ± 7.18 days. The only major co-morbidity reported was hypertension in three subjects and rheumatoid arthritis in Patient 4. Body mass index (BMI) was 28.84 ± 3.65 kg/m2 (range: 29.4–32.14 kg/m2) and self-described baseline health was either “fit” or “well.” All patients were initially treated with azithromycin. The three surviving subjects, who did not present with a bacterial superinfection, were also treated with ceftriaxone. Patient 3 was treated with piperacillin/tazobactam after positive culture identification (Pseudomonas aeruginosa in urine and Enterobacter aerogenes pneumonia), and later with meropenem and vancomycin, after clinical failure to respond to infection.
The three more critically ill patients of the group (Patients 1, 3 and 4) were intubated following diagnosis of Acute Respiratory Distress Syndrome (ARDS) and remained on mechanical ventilation for at least some duration of their ICU stay. These patients also required vasopressor agents to maintain hemodynamic stability. As regards other therapeutic interventions, none of these patients was systematically anti-coagulated, and at the time of their admission to the ICU and recruitment for this study (early in the COVID-19 pandemic), there was no recommendation yet resulting in widespread use of steroids and anti-viral therapies. Further details as to the ICU course and treatment of these patients are available in Supplementary File S1.
Protease activity data are reported in Figure 1 (hemostasis-mediating proteases) and in Figure 2 (trypsin-like enzymes and aminopeptidase). Figure 1 shows that plasmin activity remained stable over time and between patients, serving as internal control of enzymatic activity. Thrombin and Factor IX activity showed an increasing trend over the first 5 days of ICU admission followed by gradual decrease. Factor X activity decreased over time in Patients 1 and 2 compared to day 2 and increased in Patients 3 and 4. The main finding was the marked increase in Factor VII activity and tryptic-like activity, particularly at a terminal Val-Arg (Figure 2) in Patient 3 on day 7, when bacterial superinfection was diagnosed. Figure 2 also shows static methionine aminopeptidase (MetAP) activity (Vivitide®, Gardner, MA), serving as a de facto negative control for the assay. The intensity of the proteins of interest, derived from proteomic analysis, are reported in the heat maps in Figure 3. Proteins characterized by the most evident trends (over time and between patients) are highlighted with a diamond sign next to the protein name. For the complete proteome profile, see Supplementary File S2.
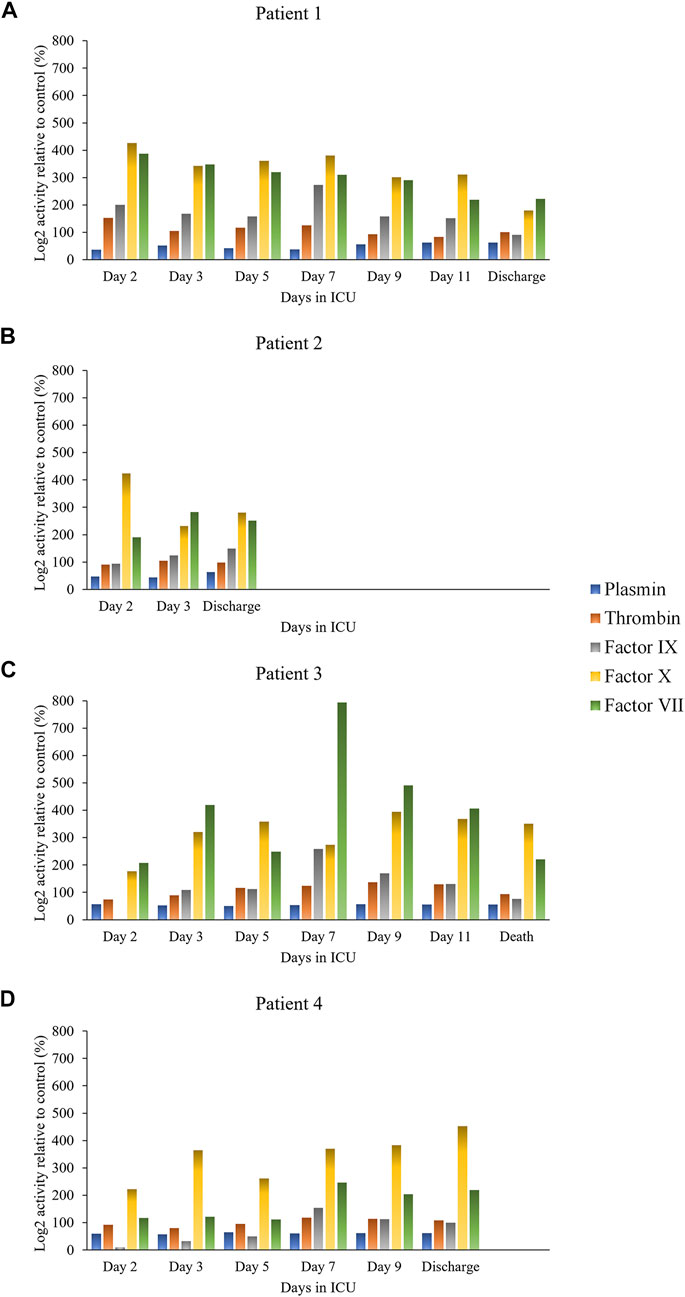
FIGURE 1. Individual activity of clotting factors and plasmin over time for each patient: Patient 1 (A), Patient 2 (B), Patient 3 (C), Patient 4 (D).
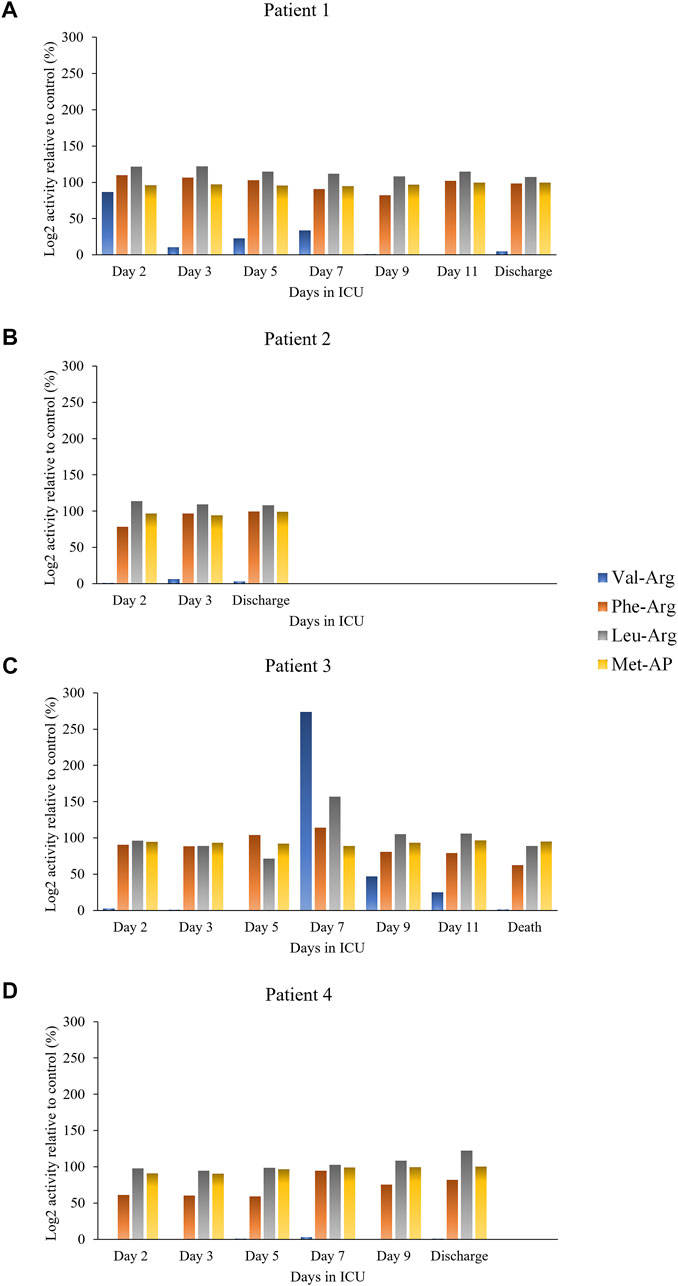
FIGURE 2. Individual trypsin-like and aminopeptidase activity over time for each patient: Patient 1 (A), Patient 2 (B), Patient 3 (C), Patient 4 (D).
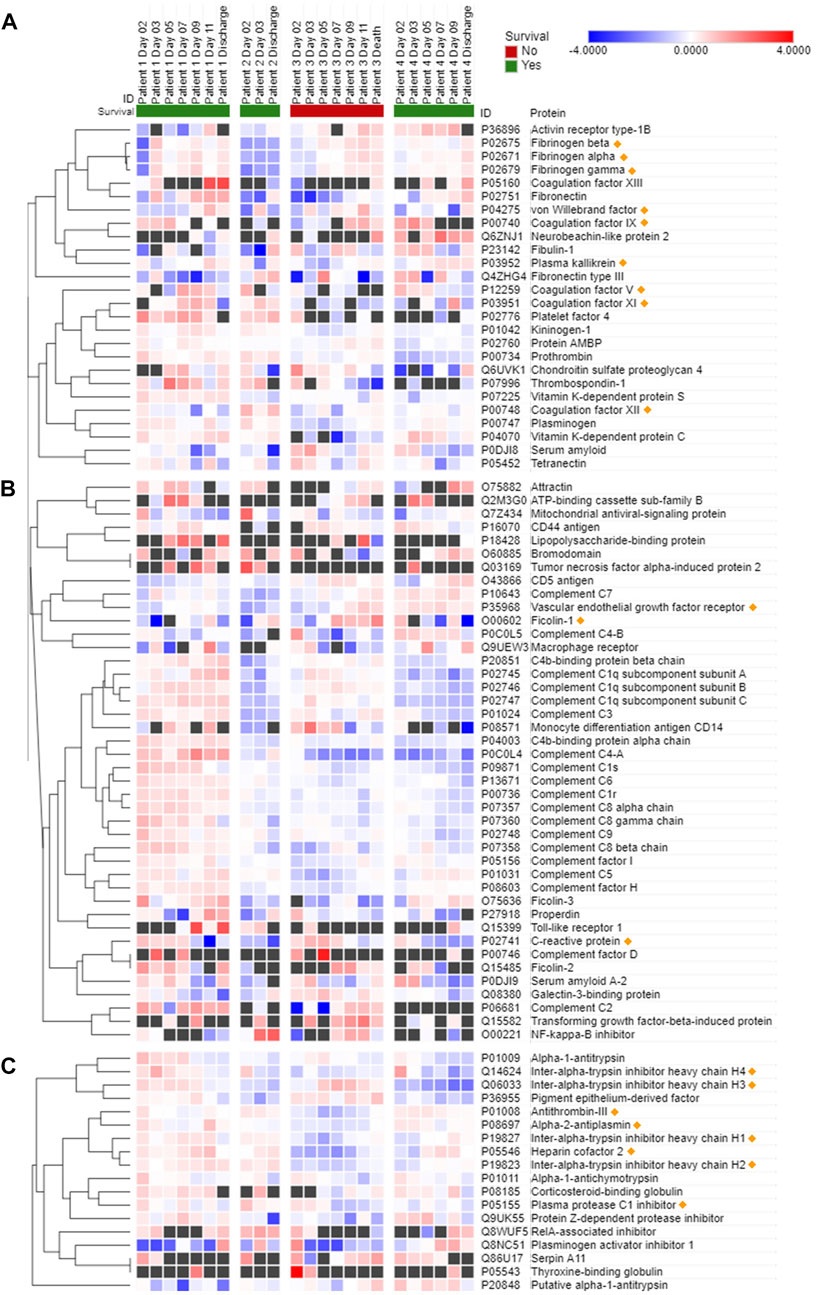
FIGURE 3. Most significant proteins involved in hemostasis (A), immune or inflammatory response (B), and endogenous protease inhibition (C) detected in COVID-19 patient plasma. Data are presented as Log2 ratio between the value at a specific time point and the average of the intensity values at all time points. Black squares indicate that a protein was not detected in the sample. Rows of identified proteins were hierarchically clustered with the one minus Pearson’s correlation metric and average linkage method for each protein group. Columns were sorted by patient and ordered by day of sample collection. Morpheus (https://software.broadinstitute.org/morpheus/) was used to generate the heatmaps.
Patient 3 exhibited a reduction in several plasma coagulation proteins such as coagulation factors V and XI on day 7 and thereafter, and a concomitant increase in the expression of fibrinogen, plasma kallikrein and von Willebrand factor (Figure 3A). Overall, there were mild changes over time in the abundance of proteins involved in the immune and inflammatory response of Patient 3, as shown by diverging trends of increase and decrease of the complement factors (Figure 3B). Interestingly, starting on day 7 ficolin-1 and VEGF 2 were increased in Patient 3, while the trend was less apparent and characterized by some variability in the other patients. C-reactive protein showed a progressively decreasing trend in expression over time in all four patients. A general trend towards a reduction in the expression of protease inhibitors, in particular of endogenous trypsin inhibitors, was observed in Patient 3 (Figure 3C). Thrombin inhibitors, such as antithrombin-III and heparin cofactor II, were also downregulated in Patient 3 compared to the survivors at all time points.
Additional clustering analyses focused specifically on Patient 3 (Figure 4) and revealed a trend of apparent correlation between the expression of several proteins (involved either in hemostasis regulation or in endogenous enzymatic inhibition) and the advancement of the disease after day 7 as opposed to the trends before day 7.
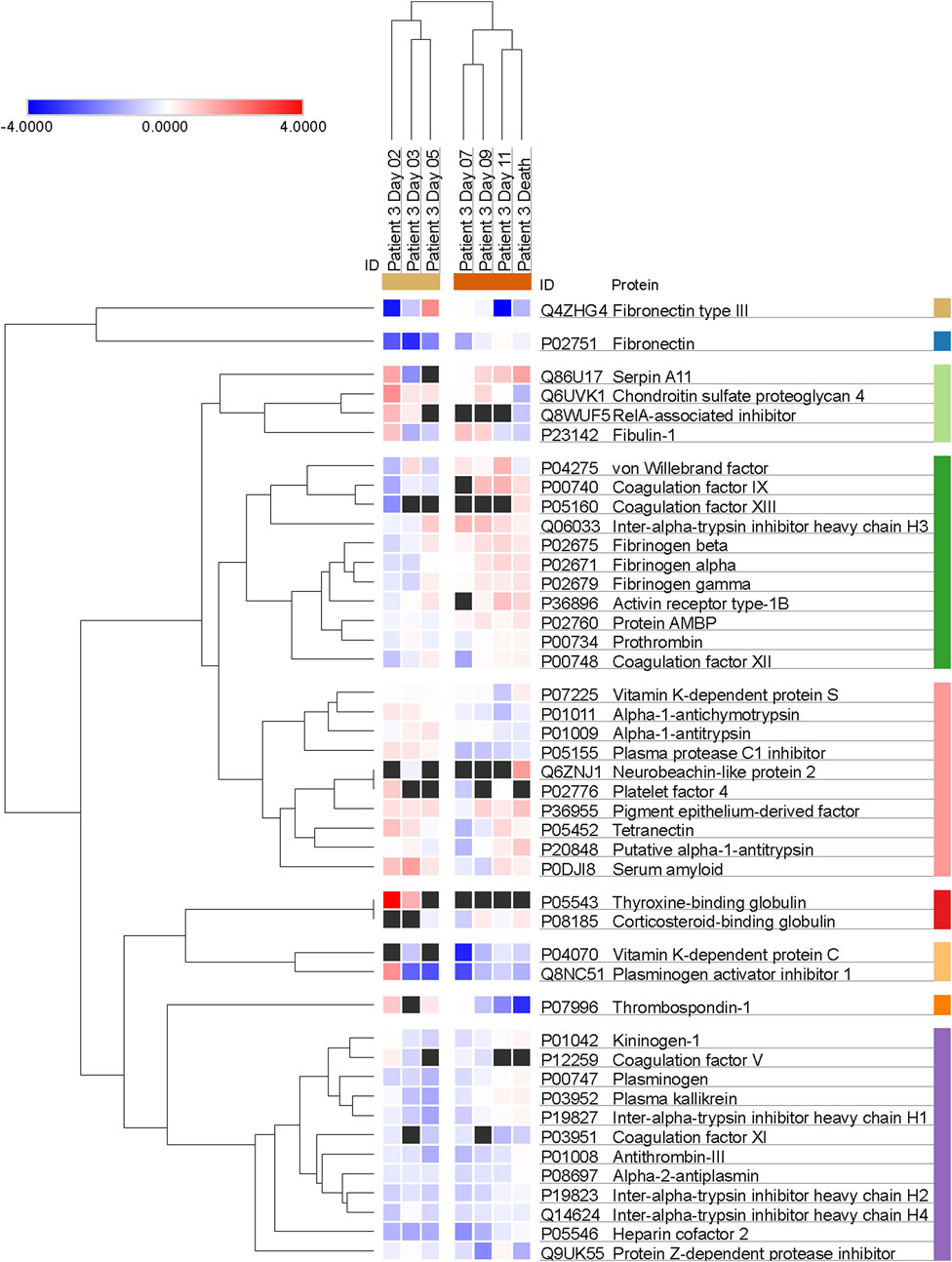
FIGURE 4. Trends of clustering between proteins involved in hemostasis regulation and endogenous protease inhibition across multiple days for Patient 3. Data are presented as Log2 ratio between the value at a specific time point and the average of the intensity values at all time points. Black squares indicate that a protein was not detected in the sample. Rows of identified proteins were hierarchically clustered with the one minus Pearson’s correlation metric and average linkage method for each protein group.
Figure 5 summarizes the main results of the peptidomic analysis, considering the specific aims of our study. The sum of the intensities of all the peptide fragments detected at each time point from a total of 233 source proteins suggests marked proteolytic activity in Patient 3 on day 7 (Figure 5A). This ongoing protein degradation was particularly evident in fibrinogen-α and fibrinogen-β (Figures 5B, C). Of note, peptides derived from α2-antiplasmin (Figure 5D) had a characteristic trend in Patients 1, 3 and 4, with a progressive increase until day 5, followed by an abrupt reduction and a progressive increase thereafter. Figure 5E shows the unique patterns of protein cleavage for fibrinogen-α obtained by Peptigram analysis and the size and location of the fragments that suggest that the enhanced proteolytic activity seen on day 7 in Patient 3 increases the amount of circulating fibrinopeptide A (FPA).
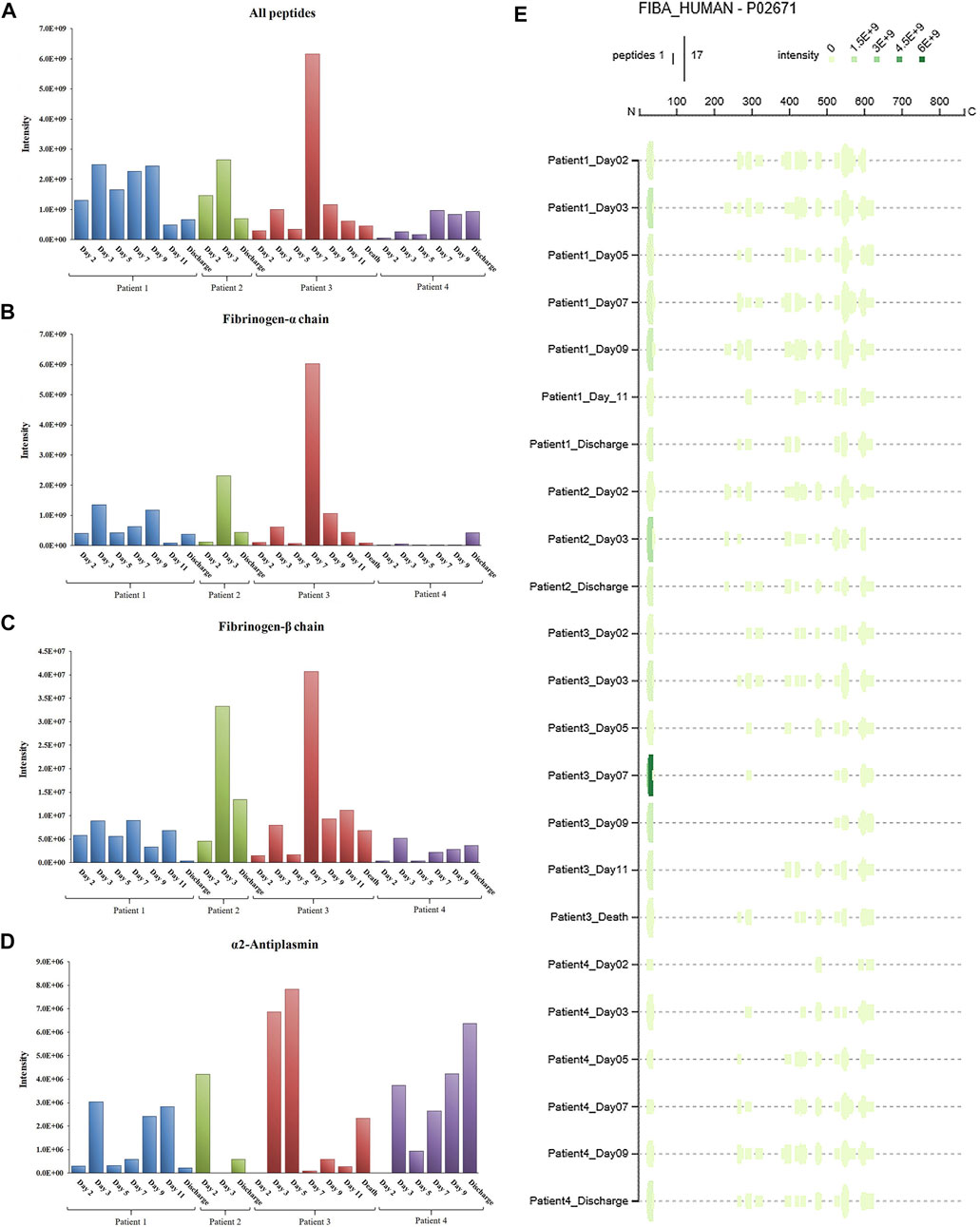
FIGURE 5. Total intensity as the sum of the intensities of the peptides detected in COVID-19 patient plasma: a) from the whole proteome (A); b) from fibrinogen-α (B); c) from fibrinogen-β (C); d) from alpha-2-antiplasmin (D). Peptigram representation of the cleavage pattern of fibrinogen-α in the four patients at each time point (E).
The link to the complete peptidome profile as analyzed via Peptigram can be found at: http://bioware.ucd.ie/peptigram/job/7jbnkglrh290.
4 Discussion
Omics approaches (metabolomics, proteomics, peptidomics, transcriptomics, genomics) to study disease, including acute illness, have become increasingly popular due to the unique multiscale insights they provide that can result in better targeted treatments (Aletti et al., 2016a; Cambiaghi et al., 2017; Sharma et al., 2017; Bauzá-Martinez et al., 2018; Cambiaghi et al., 2018; Braga et al., 2019; Mangioni et al., 2020; Schuurman et al., 2021). Several contributions have already been made to the field of COVID-19 by proteomics (Messner et al., 2020; Demichev et al., 2021; Haas et al., 2021; McArdle et al., 2021).
We have previously leveraged -omics analyses to test and validate the hypothesis that dysregulated systemic proteolysis occurs in circulatory shock and degrades several proteins with an important role in the maintenance of physiologic homeostasis. To this end, we introduced a peptidomic-based assay, which estimates the magnitude of in vivo proteolysis by assessing the abundance and count of peptides in a plasma sample (Aletti et al., 2016b; Bauzá-Martinez et al., 2018). Analysis of the circulating peptidome by this method showed an association between enhanced proteolysis and mortality (Bauzá-Martinez et al., 2018) in patients with septic shock caused by bacterial infection. Therefore, we investigated whether a similar association may also be found in viral sepsis caused by COVID-19 infection with bacterial superinfection developing over the course of the ICU stay. In addition to our previously developed mass spectrometry-based peptidomic assay, we also quantified the proteolytic activity of proteases that are known to be activated in acute illness, such as enzymes responsible for hemostasis regulation (clotting factors and plasmin), and other serine proteases characterized by tryptic-like activity. The combination of peptidomic (and proteomic) data and enzymatic activity measurements during the course of patients’ disease process aimed to shed light on systemic intravascular proteolysis, which may play a pivotal role in the pathogenesis of potentially lethal coagulopathies seen in acute illness, given the crucial role of proteases in the regulation of the balance between coagulation and fibrinolysis.
Protease activity patterns showed a marked increase of Factor VII and Factor IX activity on day 7 in the patient who died of superinfection. In particular, the spike in Factor VII activity was unique amongst our sample cohort and represents an 80-fold increase relative to that found in healthy control plasma. Further, the same trend in activity was also found in two substrates identified by the cleavage sites Val-Arg and Leu-Arg, representative of tryptic-like proteolytic activity. Although the activity of all four substrates (Factor VII, Factor IX, Val-Arg, Leu-Arg) tended to decrease after the initial increase, the peak on day 7 in Patient 3 coincided with the diagnosis of bacterial superinfection and with an increase in peptide abundance, particularly from fibrinogen (as detailed in Figure 5). Most fibrinogen-derived peptides in Patient 3 on day 7 originated from the Fibrinopeptide A (FPA) domain, located at the N-terminal region of the fibrinogen Aα chain (Aα 20–35), which is normally generated by thrombin cleavage of fibrinogen upon activation of the clotting cascade. FPA is a known biomarker of the activation of the coagulation system and was shown to be related to elevated inflammation in sepsis, including COVID-19-induced sepsis (Ranucci et al., 2020). Although thrombin was not found to be overactivated in our patient sample, other serine proteases may contribute to fibrinogen cleavage at typical thrombin cleaving sites.
Further, our proteomic assay detected reduced levels of antithrombin-III, heparin cofactor II and, perhaps most importantly, Protein C in Patient 3 compared to the three surviving patients. These findings, especially the minimum of Protein C concentration measured on day 7, also hint at an impairment of hemostasis in this patient. The limited availability of one of the major circulating anticoagulant factors, such as Protein C, and of inhibitors responsible for thrombin regulation, such as antithrombin-III, regardless of thrombin expression and activity levels, and of etiology (pathological proteolysis, increased protein use, decreased expression, etc.) is suggestive of a possible imbalance between procoagulant and anticoagulant factors, with a potential impact on the risk for either thrombosis or bleeding. Future studies on the impairment induced by shock on hemostasis should address the dynamic changes in this balance, in order to further explore the possible associations between infection, enhanced proteolysis, and dysregulation of the clotting cascade and hemostasis in general. Non-etheless, while we cannot draw any conclusion regarding the possible trends of correlation between the expression of proteins or groups of proteins that could be affected by the progression of the disease due to the limitation of the clustering analysis that was applied to the single patient who didn't survive (as shown in Figure 4 for Patient 3), it is interesting to observe that there may be patterns of concomitant dysregulation in specific clotting factors and enzyme inhibitors with possible pathophysiological and clinical implications.
Consistent with our prior findings of enhanced proteolysis over time in patients who died of septic shock (Bauzá-Martinez et al., 2018), these results may indicate a possible relationship between a surge in proteolytic activity in concert with impairment of the coagulation cascade and fibrinolysis and worsening overall clinical course after development of bacterial infection following the initial progression of COVID-19 infection. This was clinically apparent in Patient 3 who had been on either phenylephrine or norepinephrine (but not both) for hypotension from admission to the ICU, and on day 7 necessitated the addition of two simultaneous pressor agents (norepinephrine and vasopressin) to maintain adequate hemodynamics. This pressor requirement subsequently increased the following day to three simultaneous pressor agents (norepinephrine, phenylephrine, and vasopressin) before later subsiding.
As COVID-19 infection has a strong association with coagulopathy (Asakura and Ogawa, 2020; Iba et al., 2020), we focused on the activity of enzymes involved in the clotting cascade and fibrinolysis regulation and other trypsin-like proteases, which could be characterized by a similar array of target proteins, including but not limited to coagulation enzymes and fibrinogen. Thromboembolism and reports of microvascular thrombi akin to disseminated intravascular coagulation (DIC) have been a common reported cause of death in patients admitted to the ICU with severe COVID-19. Although the cause of death in Patient 3 was classified as septic shock, the possible association between enhanced proteolytic activity, bacterial superinfection and disease severity is non-etheless of interest and could point to i) the presence of additional pathological mechanisms (i.e., proteolysis) that contribute to poor outcomes, and ii) the relationship between enzymatic activity patterns and bacterial superinfection that causes further illness, in addition to the original viral infection.
Our approach could also help disentangle the role of specific enzymes in the overall enhancement of systemwide proteolysis. In particular, we generated data potentially useful to better understand the pathophysiological alterations of biological systems regulated by proteases with competing functions. The observation that the activity of specific clotting factors was enhanced, while plasmin activity levels remained unaltered, suggests that the dynamic equilibrium between coagulation and fibrinolysis could be disrupted in acute illness. In turn, this could lead to hemostasis impairment and coagulopathies. In the absence of accurate measurements to predict hemostasis disorders, the combined use of quantitative enzymatic activity assay and mass spectrometry analysis of the circulating proteome and peptidome warrants further research to advance the understanding of the possible imbalance between enzymes with competing functions, as well as between proteases and their endogenous inhibitors.
The association between disease severity and -omics patterns is also supported by the measurements of other proteins that play a role in immunity and inflammation. For instance, the trends in the expression of ficolin-1 and VEGFR2, and to some extent C-reactive protein (which showed a decrease over time in all four patients but was much milder in Patient 3) correlate with the exacerbation of the inflammatory state of Patient 3 following diagnosis of bacterial superinfection. Ficolin-1 is a known activator of the complement system and innate immunity through recognition of pathogen-associated molecular patterns (PAMPs) (Bidula et al., 2019; Polycarpou et al., 2020), while VEGFR2 has been related to COVID-19 progression (Kong et al., 2020; Birnhuber et al., 2021). In fact, the patient with the least severe course of disease (Patient 2) had the lowest VEGFR2 concentration and, importantly, was the only patient who wasn't diagnosed with ARDS. Patient 4, on the other hand, had rheumatoid arthritis and subsequently greater VEGFR2 intensity. These observations highlight that the complex interplay between baseline comorbidities, disease severity and variability of immune and inflammatory responses prevents from interpreting conclusively the expression trends of complement factors and warrants further research in the future.
There are some limitations of this study that need to be acknowledged. First, the low sample size of the studied patient population and the non-uniform time distribution of the collected samples and relevant data hinders the ability to make stronger inferences than are made here; the robustness of the presented results would doubtlessly have been strengthened with a larger sample size and a more homogeneous database, especially considering the impact on the available -omics data. Therefore, no firm generalizations can be made about multiomics and proteolytic changes in COVID-19 patients based on the reported results. Second, the four participants in our study were characterized by significant inter-subject variability and heterogeneity, as shown for example by age distribution, duration of ICU stay and hospitalization and of days on/off mechanical ventilation, combination of antibiotic and vasopressor therapy, severity, incomplete coagulation panel measures, etc. While it is possible that age was a strong determinant of Patient 3’s outcome, the presence of bacterial superinfection is strongly suspected to have been responsible for the unique results that our approach generated. Third, the inclusion of a patient subgroup with acute illness other than COVID-19, for instance bacterial sepsis and septic shock, would have added an important control sample. Unfortunately, the emergency logistics of the ICUs during the first waves of the pandemic made it impossible to have access to other types of patients, and convenience sampling was the only realistic option for patient recruitment. Fourth, regarding data drop-out, this was a real-world observational study, and the clinical laboratory measurements and values reflect patient management of severely ill COVID-19 patients in the ICU. Fifth, the mass spectrometry approach was necessarily untargeted; if specific protein systems and products of their degradation were targeted, it is possible that potential alterations in these systems could be highlighted in greater detail. Finally, when comparing and integrating proteomics and peptidomics data, the availability of transcriptomics data, which weren't part of the experimental design of this observational study, could add information on protein regulation, and help understand to a greater depth the impact of abnormal proteolysis and protein synthesis, breakdown, and turnover.
In summary, we show here that the analysis of the circulating proteome and peptidome, coupled with quantitation of proteolytic activity in plasma of COVID-19 patients in time, is a powerful methodological tool that can provide pathophysiologically and clinically meaningful data. Our approach may prove particularly useful to more thoroughly describe how the activation and regulation of hemostasis, a complex protease-mediated system which plays a critical role in the management of acutely ill patients in the ICU, is impaired in septic shock. The association between proteolytic activity and protease expression patterns achieved by mass spectrometry investigation of plasma and bacterial superinfection in COVID-19 may also contribute to anticipate the signs of possible coagulation disorders and aid in the early diagnosis and delivery of a timely therapy. However, considering the limitations related to the low sample size of our study, its main contribution is represented by the proof-of-concept of the usefulness of the proposed experimental and analytical workflow. Despite the small number of subjects enrolled in the study, we tested as a rigorous approach as possible to quantitatively measure several important, but clinically neglected parameters of protease-mediated processes that may be fatally dysregulated in septic shock. Future research will have to thoroughly address the limitations of this report, with the goal of validating our preliminary observations about the proteolytic activity and degradation patterns of proteins involved in hemostasis regulation. This will be made possible by the application of the complex, multidata methodological approach utilized in this study to larger and more homogenous patient groups, characterized by comparable clinical representations and including different degrees of severity and specific manifestations of hemostasis disorders.
Data availability statement
The datasets presented in this study can be found in online repositories. The names of the repository and accession number can be found below: ProteomeXchange (http://proteomecentral.proteomexchange.org/cgi/GetDataset), PXD029181.
Ethics statement
The studies involving human participants were reviewed and approved by the University of California San Diego Medical Center Institutional Review Board (IRB# 190699, Protocol #20-0006). The patients/participants provided their written informed consent to participate in this study.
Author contributions
FDS designed and carried out the experiments with blood samples, analyzed the data, interpreted the results, drafted the methods and results description, and revised the manuscript. JL analyzed the data, interpreted the results, drafted the methods and results description, and revised the manuscript. NJ analyzed the data, interpreted the results, and revised the manuscript. RM interpreted the results and revised the manuscript. LB designed the experiments with blood samples, interpreted the results and revised the manuscript. NGC designed the study, collected the clinical data, interpreted the results, and revised the manuscript. ML collected the clinical data and revised the manuscript. MO collected the clinical data and revised the manuscript. MI interpreted the results and revised the manuscript. AO’D designed the enzymatic activity assays, interpreted the results and revised the manuscript. FA designed and interpreted the multiomics analyses, interpreted the results, reviewed the literature, and drafted the manuscript. EK designed the study, collected the clinical data, interpreted the results, and drafted the manuscript.
Funding
California Breast Cancer Research Program of the University of California, RGPO Grant R01RG3766 (R00RG2541) (to EK); US Army Medical Research Acquisition Activity (USAMRAA) Award #W81XWH-17-2-0047 (to EK).
Conflict of interest
The authors declare that the research was conducted in the absence of any commercial or financial relationships that could be construed as a potential conflict of interest.
Publisher’s note
All claims expressed in this article are solely those of the authors and do not necessarily represent those of their affiliated organizations, or those of the publisher, the editors and the reviewers. Any product that may be evaluated in this article, or claim that may be made by its manufacturer, is not guaranteed or endorsed by the publisher.
Supplementary material
The Supplementary Material for this article can be found online at: https://www.frontiersin.org/articles/10.3389/fmolb.2022.1051471/full#supplementary-material
References
Aletti, F., Conti, C., Ferrario, M., Ribas, V., Bollen Pinto, B., Herpain, A., et al. (2016). ShockOmics: Multiscale approach to the identification of molecular biomarkers in acute heart failure induced by shock. Scand. J. Trauma Resusc. Emerg. Med. 24, 9. doi:10.1186/s13049-016-0197-4
Aletti, F., DeLano, F. A., Maffioli, E., Mu, H., Schmid-Schönbein, G. W., Tedeschi, G., et al. (2022). Continuous enteral protease inhibition as a novel treatment for experimental trauma/hemorrhagic shock. Eur. J. Trauma Emerg. Surg. 48 (3), 1579–1588. doi:10.1007/s00068-020-01591-y
Aletti, F., Maffioli, E., Negri, A., Santamaria, M. H., DeLano, F. A., Kistler, E. B., et al. (2016). Peptidomic analysis of rat plasma: Proteolysis in hemorrhagic shock. Shock 45 (5), 540–554. doi:10.1097/SHK.0000000000000532
Alhazzani, W., Evans, L., Alshamsi, F., Møller, M. H., Ostermann, M., Prescott, H. C., et al. (2021). Surviving sepsis campaign guidelines on the management of adults with coronavirus disease 2019 (COVID-19) in the ICU: First update. Crit. Care Med. 49 (3), e219–e234. doi:10.1097/CCM.0000000000004899
Asakura, H., and Ogawa, H. (2020). COVID-19-associated coagulopathy and disseminated intravascular coagulation. Int. J. Hematol. 7, 45–57. doi:10.1007/s12185-020-03029-y
Bauzá-Martinez, J., Aletti, F., Pinto, B. B., Ribas, V., Odena, M. A., Díaz, R., et al. (2018). Proteolysis in septic shock patients: Plasma peptidomic patterns are associated with mortality. Br. J. Anaesth. 121 (5), 1065–1074. doi:10.1016/j.bja.2018.05.072
Bidula, S., Sexton, D. W., and Schelenz, S. (2019). Ficolins and the recognition of pathogenic microorganisms: An overview of the innate immune response and contribution of single nucleotide polymorphisms. J. Immunol. Res. 2019, 3205072. doi:10.1155/2019/3205072
Birnhuber, A., Fließer, E., Gorkiewicz, G., Zacharias, M., Seeliger, B., David, S., et al. (2021). Between inflammation and thrombosis: Endothelial cells in COVID-19. Eur. Respir. J. 58 (3), 2100377. doi:10.1183/13993003.00377-2021
Braga, D., Barcella, M., Herpain, A., Aletti, F., Kistler, E. B., Bollen Pinto, B., et al. (2019). A longitudinal study highlights shared aspects of the transcriptomic response to cardiogenic and septic shock. Crit. Care 23 (1), 414. doi:10.1186/s13054-019-2670-8
Cambiaghi, A., Díaz, R., Martinez, J. B., Odena, A., Brunelli, L., Caironi, P., et al. (2018). An innovative approach for the integration of proteomics and metabolomics data in severe septic shock patients stratified for mortality. Sci. Rep. 8 (1), 6681. doi:10.1038/s41598-018-25035-1
Cambiaghi, A., Pinto, B. B., Brunelli, L., Falcetta, F., Aletti, F., Bendjelid, K., et al. (2017). Characterization of a metabolomic profile associated with responsiveness to therapy in the acute phase of septic shock. Sci. Rep. 7 (1), 9748. doi:10.1038/s41598-017-09619-x
Chalmers, J. D., Crichton, M. L., Goeminne, P. C., Cao, B., Humbert, M., Shteinberg, M., et al. (2021). Update june 2022: Management of hospitalised adults with coronavirus disease 2019 (COVID-19): A European respiratory society living guideline. Eur. Respir. J. 57 (4), 2200803. doi:10.1183/13993003.00803-2022
Courelli, V., Ahmad, A., Ghassemian, M., Pruitt, C., Mills, J. P., and Schmid-Schönbein, G. W. (2021). Digestive enzyme activity and protein degradation in plasma of heart failure patients. Cell Mol. Bioeng. 14 (6), 583–596. doi:10.1007/s12195-021-00693-w
Demichev, V., Tober-Lau, P., Lemke, O., Nazarenko, T., Thibeault, C., Whitwell, H., et al. (2021). A time-resolved proteomic and prognostic map of COVID-19. Cell Syst. 12 (8), 780–794.e7. doi:10.1016/j.cels.2021.05.005
Garcia-Vidal, C., Sanjuan, G., Moreno-García, E., Puerta-Alcalde, P., Garcia-Pouton, N., Chumbita, M., et al. COVID-19 Researchers Group (2021). Incidence of co-infections and superinfections in hospitalized patients with COVID-19: A retrospective cohort study. Clin. Microbiol. Infect. 27 (1), 83–88. doi:10.1016/j.cmi.2020.07.041
Haas, P., Muralidharan, M., Krogan, N. J., Kaake, R. M., and Hüttenhain, R. (2021). Proteomic approaches to study SARS-CoV-2 Biology and COVID-19 pathology. J. Proteome Res. 20 (2), 1133–1152. doi:10.1021/acs.jproteome.0c00764
Iba, T., Levy, J. H., Levi, M., and Thachil, J. (2020). Coagulopathy in COVID-19. J. Thromb. Haemost. 18 (9), 2103–2109. doi:10.1111/jth.14975
Kong, Y., Han, J., Wu, X., Zeng, H., Liu, J., and Zhang, H. (2020). VEGF-D: A novel biomarker for detection of COVID-19 progression. Crit. Care 24 (1), 373. doi:10.1186/s13054-020-03079-y
Lazzaroni, M. G., Piantoni, S., Masneri, S., Garrafa, E., Martini, G., Tincani, A., et al. (2021). Coagulation dysfunction in COVID-19: The interplay between inflammation, viral infection and the coagulation system. Blood Rev. 46, 100745. doi:10.1016/j.blre.2020.100745
Lopes-Pacheco, M., Silva, P. L., Cruz, F. F., Battaglini, D., Robba, C., Pelosi, P., et al. (2021). Pathogenesis of multiple organ injury in COVID-19 and potential therapeutic strategies. Front. Physiol. 12, 593223. doi:10.3389/fphys.2021.593223
Maffioli, E., Jiang, Z., Nonnis, S., Negri, A., Romeo, V., Lietz, C. B., et al. (2020). High-resolution mass spectrometry-based approaches for the detection and quantification of peptidase activity in plasma. Molecules 25 (18), 4071. doi:10.3390/molecules25184071
Mangioni, D., Peri, A. M., Rossolini, G. M., Viaggi, B., Perno, C. F., Gori, A., et al. (2020). Toward rapid sepsis diagnosis and patient stratification: What's new from microbiology and omics Science. J. Infect. Dis. 221 (7), 1039–1047. doi:10.1093/infdis/jiz585
Manguy, J., Jehl, P., Dillon, E. T., Davey, N. E., Shields, D. C., and Holton, T. A. (2017). Peptigram: A web-based application for peptidomics data visualization. J. Proteome Res. 16 (2), 712–719. doi:10.1021/acs.jproteome.6b00751
McArdle, A., Washington, K. E., Chazarin Orgel, B., Binek, A., Manalo, D. M., Rivas, A., et al. (2021). Discovery proteomics for COVID-19: Where we are now. J. Proteome Res. 20 (10), 4627–4639. doi:10.1021/acs.jproteome.1c00475
Messner, C. B., Demichev, V., Wendisch, D., Michalick, L., White, M., Freiwald, A., et al. (2020). Ultra-high-throughput clinical proteomics reveals classifiers of COVID-19 infection. Cell Syst. 11 (1), 11–24. doi:10.1016/j.cels.2020.05.012
Osuchowski, M. F., Aletti, F., Cavaillon, J. M., Flohé, S. B., Giamarellos-Bourboulis, E. J., Huber-Lang, M., et al. (2020). SARS-CoV-2/COVID-19: Evolving reality, global response, knowledge gaps, and opportunities. Shock 54 (4), 416–437. doi:10.1097/SHK.0000000000001565
Osuchowski, M. F., Winkler, M. S., Skirecki, T., Cajander, S., Shankar-Hari, M., Lachmann, G., et al. (2021). The COVID-19 puzzle: Deciphering pathophysiology and phenotypes of a new disease entity. Lancet Respir. Med. 9 (6), 622–642. doi:10.1016/S2213-2600(21)00218-6
Polycarpou, A., Howard, M., Farrar, C. A., Greenlaw, R., Fanelli, G., Wallis, R., et al. (2020). Rationale for targeting complement in COVID-19. EMBO Mol. Med. 12 (8), e12642. doi:10.15252/emmm.202012642
Ranucci, M., Sitzia, C., Baryshnikova, E., Di Dedda, U., Cardani, R., Martelli, F., et al. (2020). Covid-19-Associated coagulopathy: Biomarkers of thrombin generation and fibrinolysis leading the outcome. J. Clin. Med. 9 (11), 3487. doi:10.3390/jcm9113487
Schuurman, A. R., Reijnders, T. D. Y., Kullberg, R. F. J., Butler, J. M., van der Poll, T., and Wiersinga, W. J. (2021). Sepsis: Deriving biological meaning and clinical applications from high-dimensional data. Intensive Care Med. Exp. 9 (1), 27. doi:10.1186/s40635-021-00383-x
Sharma, N. K., Tashima, A. K., Brunialti, M. K. C., Ferreira, E. R., Torquato, R. J. S., Mortara, R. A., et al. (2017). Proteomic study revealed cellular assembly and lipid metabolism dysregulation in sepsis secondary to community-acquired pneumonia. Sci. Rep. 7, 15606. doi:10.1038/s41598-017-15755-1
Tang, Y., Liu, J., Zhang, D., Xu, Z., Ji, J., and Wen, C. (2020). Cytokine storm in COVID-19: The current evidence and treatment strategies. Front. Immunol. 11, 1708. doi:10.3389/fimmu.2020.01708
Welte, T., Ambrose, L. J., Sibbring, G. C., Sheikh, S., Müllerová, H., and Sabir, I. (2021). Current evidence for COVID-19 therapies: A systematic literature review. Eur. Respir. Rev. 30 (159), 200384. doi:10.1183/16000617.0384-2020
Keywords: COVID-19, sepsis, proteolysis, enzymatic activity, peptidomics, proteomics
Citation: Dos Santos F, Li JB, Juocys N, Mazor R, Beretta L, Coufal NG, Lam MTY, Odish MF, Irigoyen MC, O’Donoghue AJ, Aletti F and Kistler EB (2023) Plasma enzymatic activity, proteomics and peptidomics in COVID-19-induced sepsis: A novel approach for the analysis of hemostasis. Front. Mol. Biosci. 9:1051471. doi: 10.3389/fmolb.2022.1051471
Received: 22 September 2022; Accepted: 20 December 2022;
Published: 11 January 2023.
Edited by:
Francesco Balestri, University of Pisa, ItalyReviewed by:
Marcin Poreba, Wrocław University of Science and Technology, PolandGiovanni Signore, Fondazione Pisana per la Scienza Onlus, Italy
Copyright © 2023 Dos Santos, Li, Juocys, Mazor, Beretta, Coufal, Lam, Odish, Irigoyen, O’Donoghue, Aletti and Kistler. This is an open-access article distributed under the terms of the Creative Commons Attribution License (CC BY). The use, distribution or reproduction in other forums is permitted, provided the original author(s) and the copyright owner(s) are credited and that the original publication in this journal is cited, in accordance with accepted academic practice. No use, distribution or reproduction is permitted which does not comply with these terms.
*Correspondence: Federico Aletti, ZmFsZXR0aUB1bmlmZXNwLmJy