- 1Guangxi University of Chinese Medicine, Nanning, Guangxi, China
- 2The First Affiliated Hospital of Guangxi University of Chinese Medicine, Nanning, Guangxi, Chinad
Heart failure is one of the major public health problems in the world. In recent years, more and more attention has been paid to the relationship between heart failure and mitochondrial function. In the past 2 decades, a growing number of research papers in this field have been published. This study conducted a bibliometric analysis of the published literature on the relationship between MF and HF in the past 20 years by utilizing Microsoft Excel 2019, Biblio metric analysis platform, WoSCC database, VosViewer and Citespace. The results show that the papers have increased year by year and China and the United States are the leading countries in this field, as well as the countries with the most cooperation and exchanges. University of california system is the research institution with the greatest impacts on research results, and Yip H.K. is the author with more papers. The American Journal of Physiology-heart and Circulatory Physiology is probably the most popular magazine. At present, most of the published articles on mitochondria and HF are cited from internationally influential journals. The research focus includes oxidative stress, metabolic dysfunction, mitochondrial Ca2+ homeostasis imbalance, mitochondrial quality control and mitochondrial dysfunction mediated by inflammation in the pathogenesis of HF. Targeted regulating of mitochondria will be the keynote of future research on prevention and treatment of HF.
1 Introduction
Heart failure (HF), a complex clinical syndrome and the end-stage manifestation of a variety of cardiovascular diseases caused by cardiac structural or functional abnormalities, is one of the major global public health issues characterized by impaired ventricular pumping or filling capacity (van der Meer et al., 2019). Epidemiological studies show that the 5-year survival rate of patients with HF is only 50%, and there are more than 37.7 million HF cases worldwide in 2016 (Ziaeian and Fonarow, 2016). At present, the morbidity, fatality, disability and readmission rate of HF remain high and the figure is expected to continue to rise in the next few decades, especially in the middle-aged and elderly population, placing a huge financial burden on patients (Yancy et al., 2013; Yancy et al., 2017). A report says that the 30-day all-cause readmission rate for HF in the United States is 19%, the all-cause mortality rate for acute HF in European countries is 17%, and 19.2% of HF patients in Asia died or were readmitted to hospital due to HF within 1 year (Rossignol et al., 2019). The understanding of the pathogenesis of HF has evolved from hemodynamic abnormalities to neuroendocrine abnormalities, and then to ventricular remodeling. However, these mechanisms do not fully explain the complexity of HF pathophysiology. Therefore, it is necessary to clarify the pathogenesis and explore new treatment options to delay or reverse the progress of HF. In recent years, the relationship between HF and mitochondrial function (MF) has been paid more and more attention. Researchers have noticed that mitochondrial dysfunction is widespread in the process of HF (Brown et al., 2017). The heart is a high energy-consuming organ and the most active organ in human metabolism which accounts for only 0.5% of human body weight. However, its ATP consumption accounts for 8% of the total, and about 95% of the ATP needed by the heart comes from the oxidative metabolism of mitochondria. Mitochondrion is a double-membrane organelle that exists in almost all eukaryotic cells. Its main function is to produce ATP by oxidative phosphorylation. The mitochondrial content of the heart is the highest in all human tissues, and myocardial mitochondria must operate efficiently to maintain the normal needs of the human body (Neubauer, 2007; Sabbah, 2020). Mitochondrial dysfunction is very common in the pathogenesis. Studies have found that changes in metabolic substrate utilization, increased oxidative stress, Ca2+ homeostasis imbalance, mitochondrial quality control imbalance and inflammatory response can directly or indirectly lead to mitochondrial dysfunction, and ultimately lead to irreversible damage of cardiac function (O'Rourke et al., 2021; Del et al., 2021; Dubois-Deruy et al., 2020; Keshavarz-Bahaghighat et al., 2020). Therefore, ensuring the normal function of myocardial mitochondria and maintaining myocardial energy metabolism have become new ideas for the treatment of HF. In the past 2 decades, the research on the relationship between HF and MF has been paid more attention, and the number of literature published has been increasing, but there is a lack of research in this field from the perspective of bibliometrics.
Introduced by Pritchard in 1969, bibliometrics is a discipline that applies mathematical and statistical methods to the study of books and other documents (Ma et al., 2021). This method can quickly extract useful information from a large number of literatures, help scholars grasp the research hotspots and development trends in a certain field instantly, and also evaluate the distribution of countries, institutions, authors and journals in the research field, so as to lay a foundation for future research. In addition, bibliometrics can also be used to evaluate the trend of literature research qualitatively and quantitatively. On the one hand, this method can quickly and accurately present the most influential research results in a certain field based on public databases, providing a theoretical basis for further research. On the other hand, the use of these information can also provide reference for decision makers. In this study, researchers conducted a bibliometric analysis of the literatures published from 2002 to 2022 on the relationship between MF and HF, summarized the development status and future research trends and hotspots in this field according to the analysis results, and provided reference and directions for future research.
2 Materials and methods
2.1 Data collection
The bibliometric analysis in the paper is based on the Web of Science Core Collection (WoSCC), the quality of the literature included in WoSCC is high and constantly updated, which can effectively ensure the quality of literature analysis (Zhang et al., 2020a; Zhang et al., 2020b). The search time is from 1 January 2002 to 31 December 2021.
In order to ensure the accuracy of the search, all searches will be completed on 10 March 2022. The search term is {[(TS = (Heart Failure)] OR TS = (Myocardial Failure) OR TS = (Cardiac Failure)} AND {[(TS = Mitochondrion) OR TS = (Mitochondria)] OR TS = (Mitochondrial)}. 6514 articles were retrieved, and the retrieved articles were further screened by two researchers according to the following criteria: 1) excluding non-English articles; 2) excluding the literature whose publication category is not “article”; 3) excluding non-SCI-E indexed literature; After screening, a total of 4236 documents were included, and further documents were exported in document format for analysis. The screening criteria are shown in Figure1.
2.2 Data analysis
In this study, the literature retrieved by WoSCC was imported into Microsoft Excel 2019, Bibliometric analysis platform, VosViewer and Citespace for visual analysis. Developed by Professor Chen Chaomei, CiteSpace is a visual analysis software based on JAVA environment and dedicated to the analysis of potential information in scientific literature. It can present the development rules and distribution of scientific knowledge in a certain field by visual means. Based on the existing data, the key points in the development of the field are identified, especially the turning points and key points in the field of knowledge (Chaomei, 2006). VOSviewer is a visualization software developed by the Science and Technology Research Center in Leiden of Netherlands, through which visual network maps can be constructed based on literature information, and ultimately a comprehensive understanding of the scientific structure and dynamic development trend of a field can be achieved (van Eck and Waltman, 2010). Bibliometric online analysis platform (https://bibliometric.com/) can make online bibliometric analysis of scientific citation data and provide valuable scientific information for researchers with simple operation methods and the most intuitive expression. It gives scholars a clear grasp of the discipline structure and relationship, research content and focus in a certain field, and it predicts the Frontier and development trend.
In this study, the countries/regions, institutions, authors, journals, references, keywords, citations, H index (the number of papers with citation number ≥ H) and impact factor (IF) in 2021were analyzed. Microsoft Excel 2019 was used to analyze the annual H index and publishing trends in this field, and knowledge maps were drawn through Citespace, VosViewer and online bibliometric.
3 Results
3.1 Global publications and trends
By analyzing the change of the number of publications in a certain field over time, we can effectively evaluate the historical process and current research status and predict the future development trend (Gao et al., 2020). It can be seen from (Figure2)that the number of literatures published on the relationship between MF and HF is increasing year by year from 2002 to 2021. The number of research literatures is small from 2002 to 2008, which indicates that the research on the relationship between HF and MF is still in its infancy during this period. After 2009, articles published increased rapidly until the output reached 404 in 2021. In addition, From the H index distribution, we can see that the year of 2010 is the highest, which shows that the papers published in this year have great influences and far-reaching significance. From the perspective of overall trend, more and more scholars are expected to participate in the future research. It has great potential to study HF from the aspect of mitochondrial dysfunction.
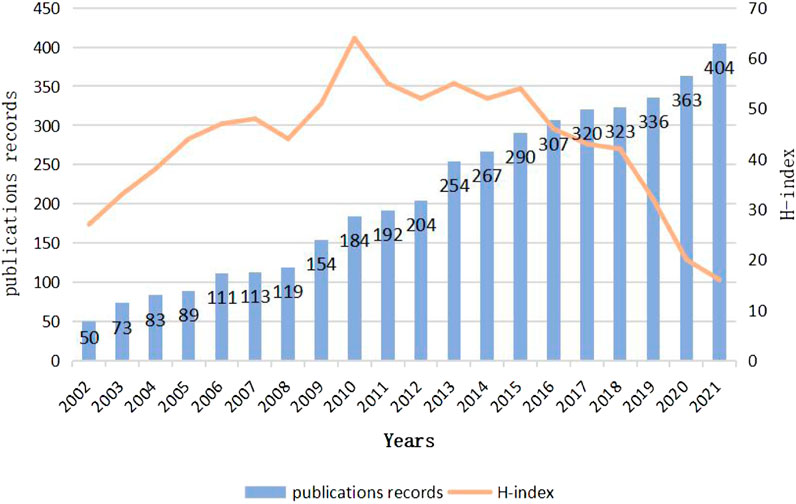
FIGURE 2. Global number of publications and H-index of publications in the field of relationship between Mitochondrial function and heart failure from 2002 to 2022.
3.2 Distribution of countries/regions
After visualized analysis of the publications, it was found that 79 countries/regions participated in the study (Figure 3), of which the United States (1744) and China (877) had the highest number of publications, almost equal to the sum of the other countries/regions. The next goes to Japan (336), Germany (335), Canada (255), United Kingdom (237), Italy (210), France (168), Taiwan (145) and Netherlands (129). In terms of H index, the United States (128) is the highest, followed by Germany (Wai et al., 2015), China (Gao and Hu, 2021), Canada (Lewis and Lewis, 1914) and Japan (Koentges et al., 2015) as more details can be seen in Table 1. In addition, from the perspective of cooperation among countries, the United States, which has the largest number of publications, has active cooperation with other countries, while China is the most cooperative country of the United States (Figure 4).
3.3 Distribution of institutions
VosViewer was used to visually analyze the publishing institutions. The results showed that a total of 3838 institutions were involved in and the top ten organizations of publications and H index were analyzed. UNIVERSITY OF CALIFORNIA SYSTEM 185) has the most papers, then goes to INSTITUT NATIONAL DE LA SANTE ET DE LA RECHERCHE MEDICAL E INSERM (125), UNIVERSITY OF TEXAS SYSTEM (119), and HARVARD UNIVERSITY (112). HARVARD UNIVERSITY 8374) was cited most frequently, followed by UNIVERSITY OF TEXAS SYSTEM 7811) and UNIVERSITY OF CALIFORNIA SYSTEM (7671). From the analysis of H index, the institutions with the highest H index are UNIVERSITY OF TEXAS SYSTEM (Odagiri et al., 2009) and UNIVERSITY OF CALIFORNIA SYSTEM (Odagiri et al., 2009). Therefore, it can be inferred that UNIVERSITY OF TEXAS SYSTEM and UNIVERSITY OF CALIFORNIA SYSTEM have greatest impacts in this field. In terms of cooperation among various agencies, Institutions in the United States have more cooperation with institutions in France, United Kingdom and China, while institutions in Taiwan mainly cooperate with Asia University, Chang Gung University and China Medical University (See Table 2Figure 5 for specific results). In a word, the above analysis results show that the research institutions in the United States have studied the relationship between MF and HF more deeply, which have more cooperation with other national institutions and greater influence in this field.
3.4 Distribution of authors
VOSviewer was used to visually analyze the authors’ posts and cooperation, and then publications of the top ten authors were exported to Table 3. The results show that there are 24,919 authors involved in the study. The table shows the number of publications, H index, cooperation of the top ten authors in this field. According to the analysis, Yip H. K., Huang C. Y. and O. ′Rourke B. have published more papers, while Huang C. Y., O.′ Rourke B. and Abel E. D. have higher H index, indicating that they have made some achievements in this field. In addition, according to the analysis of author cooperation (Figure 6), the authors worldwide are scattered and have not yet formed cooperation teams of a certain scale except Wang Wei’s. However, the Yip H. K. team and Huang C. Y. team from Taiwan do not have in-depth cooperation with other countries/regions.
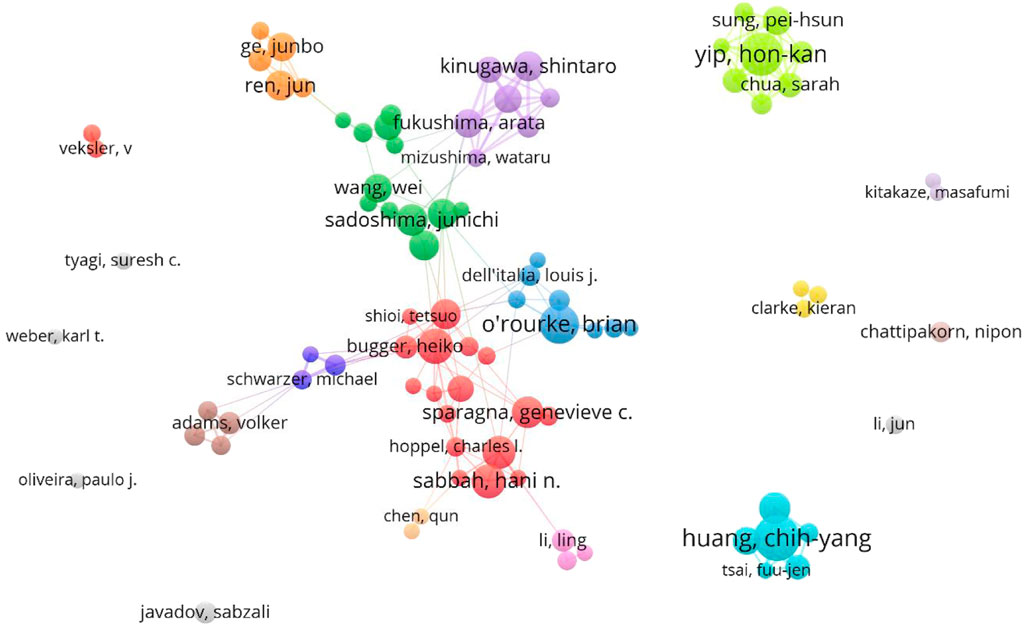
FIGURE 6. VOSviewer visualization map of authors involved in mitochondrial function and heart failure.
3.5 The analysis of Co-cited journals and journals
From 2002 to 2022, 832 journals reported the relationship between HF and MF. In this study, VosViewer was used to analyze the top 10 journals. American Journal of Physiology Heart and Circulatory Physiology (176), The Journal of Molecular and Cellular Cardiology (145) and PLoS One (140) reported the most. Circulation (9150) and Circulation Research (7884) were cited most frequently and had the highest H index. This shows that the literature published in the above two journals has great influence in this field. The impact factors of these journals range from 2.919 to 25.499, among which PLoS One has the lowest IF and Circulation has the highest, the JCR partition, Q1 (30%), Q2 (40%) and Q3 (30%) (Figure 7 and Table 4). Based on the above analysis, it can be inferred that American Journal of Physiology-heart and Circulatory Physiology is probably the most popular journal in this field.
3.6 The analysis of Co-cited references
Co-citation is a research method to measure the degree of relationship between articles. If two or more articles are cited by one or more papers at the same time, the two articles are considered to be co-cited. In this part of the study, the top ten cited articles in the 4236 articles were selected for analysis. According to the analysis results, the above articles were published between 1999 and 2010, and the IF was between 5.182 and 91.245, of which 90% were in the JCR Zone 1, and six articles have been cited more than 100 times (Table 5). Most of the cited literatures focused on exploring the pathogenesis of HF from the perspective of cardiac energy metabolism, mitochondrial quality control and oxidative stress.
3.7 The analysis of keywords
Key words are the core of a document. Through the summary of key words, we can intuitively understand the research hotspots and trends in a field. There are 5958 keywords in the 4236 articles of this study. VOSviewer and CiteSpace were used to visualize and analyze all the keywords to make a keyword co-occurrence map (Figure 8) and a keyword emergence map (Figure 9), and then the top 30 keywords were exported to Table 6. It can be seen from the keyword cluster map made by VOSviewer that it can be divided into four clusters according to different colors, namely red, green, purple and yellow. HF, oxidative stress and myocardial infarction were the main keywords in the red cluster, and mitochondrial, metabolism and myocardial infarction were the main keywords in the green cluster. The main keywords of yellow clustering of cardiomyopathy were activation, inhibition and autophagy, and the main keywords of purple clustering were apoptosis, cell death and calcium. Keyword emergence refers to the rapid increase in the citation frequency of the keyword in a certain period of time. Through this method, we can understand the development process and research hotspots of a research field. CiteSpace was used to analyze the emergence of keywords and further predicted the development trend in the past 20 years. From 2002 to 2010, there were many studies on the relationship between congestive HF and cytochrome C, TNFα and creatine kinae. From 2010 to 2017, scholars paid more attention to the relationship between HF and oxidative stress, mitochondrial dysfunction, mitochondrial permeability transition pore and oxidative phosphorylation. It was seen that key words such as doxorubicin, injury, cardiotoxicity and autophagy have emerged since 2017. It can be inferred that the cardiotoxicity of doxorubicin has been increasingly recognized and used in animal models of HF in recent years (Cappetta et al., 2017). At the same time, the study of mitophagy is also a hot spot in recent years. This study also excluded the high-frequency keywords related to the search topics such as heart-failure and mitochondria, and then interpreted the top keywords. The results showed that in the past 20 years, scholars focused mostly on the relationship between oxidative stress, apoptosis and mitochondrial dysfunction in the pathogenesis of HF and cardiometabolic effects.
4 Discussion
4.1 Research results
In this study, we analyzed the development process, research status and hot trends of the relationship between MF and HF in the past 20 years. From the overall trend of the number of papers published in this field, more and more scholars pay attention to the role of MF in the pathogenesis of HF, especially in the past 6 years, the number of papers published has increased greatly. Therefore, it can be inferred that in recent years, people’s understanding of MF has become more mature, and there are more related basic studies and the intervention of HF from MF may become a hot spot and trend in the prevention and treatment of HF in the future.
At present, there are many studies on the relationship between MF and HF in the United States, and extensive cooperation has been reached with other countries/regions, while China and the United States have the most cooperative studies. In addition, researchers in developed countries/regions such as Europe and the United States have paid more attention to the relationship between MF and HF. From the visualized analysis of the institutions, the institutions with more research and publications are universities and research institutes in the United States, and the United States has more cooperation with research institutions in China and other developed European countries, but there are still some countries/regions with less cooperation with foreign countries. Therefore, it is suggested that research institutions around the world should actively cooperate in the future to explore the role of MF in the prevention and treatment of HF.
From the author’s visualized analysis, Yip H. K., Huang C. Y. and O. 'Rourke B. have more studies on the relationship between MF and HF, and their outputs are higher. Meanwhile, Abel E .D., Huang C. Y. and O. ′Rourke B. have higher H index, which indicates that they have made some achievements in this field. In recent years, the Abel E. D. from the University of Utah of the United States, focused mainly on the relationship between cardiac energy metabolism and HF (Lopaschuk et al., 2021). The latest results of the team show that pyruvate enters the mitochondria through the mitochondrial pyruvate carrier (MPC) for citrate cycle metabolism, and plays an important role in the regulation of myocardial metabolic function (Zhang et al., 2020c). Huang C. Y. Team from China Med Univ’s has been working on the treatment of HF through mitophagy for a long time, and has found that IGF-IIR can induce mitophagy through Rab9-dependent selective autophagy, which provides a new idea for the treatment of HF (Huang et al., 2018a; Huang et al., 2018b). The O. 'Rourke B. team has long been engaged in the research on the improvement of myocardial energy metabolism by regulating MFs in multiple ways. The team found that the inhibition of miR-181c expression can reduce the generation of reactive oxygen species (ROS) and reduce the calcium level of myocardial mitochondria, thus achieving the effect of preventing and controlling HF (Roman et al., 2020). From the analysis of the author’s cooperation in the figure, it can be seen that the Wang Wei team has more cooperation with other countries/regions, forming a larger cluster, while the Yip H. K. team and the Huang C. Y. team in Taiwan have no in-depth cooperation with other countries/regions.
This study also conducted a visualized analysis of the published journals. According to the H index, it can be seen that the articles on mitochondria and HF published by Circulation and Circulation Research have great influence. From the analysis of co-citation journals, most of the cited journals in this field are high-quality journals in cardiovascular medicine, molecular biology, biochemistry, life sciences and other fields. Uch as circulation, Journal of Biological Chemistry, Journal of Molecular and Cellular Cardiology, Nature, etc. According to the analysis of the top ten journals, studies on the relationship between mitochondria and HF have been published not only in the cardiovascular field, but also in the cellular and molecular fields, and most of these papers have been published in high-quality journals. This shows that the related basic research and clinical research have high academic value in the eyes of global scholars. It is suggested that MF research should be actively carried out in the future to promote its greater role in the prevention and treatment of HF and even cardiovascular field.
From the co-citation analysis, it was found that most of the cited literatures had high impact factors, and most of the studies focused on exploring the pathogenesis of HF from the perspectives of cardiac metabolic abnormalities, mitochondrial quality control and oxidative stress. It is well known that the onset of HF is accompanied by a series of changes in energy metabolism. Including the conversion of energy substrates, mitochondrial dysfunction and the depletion of high-energy phosphates, myocardial substrate metabolism in HF is severely impaired, and the selection and utilization of substrates such as fatty acids and glucose are reconstructed, which can further lead to the progress and deterioration of HF (Bertero and Maack, 2018a). Mitochondrial quality control refers to the continuous biogenesis, fusion, fission and autophagy of mitochondria, which is essential for heart health (Tahrir et al., 2019).
4.2 Hot spots and trends
Keyword analysis reflects the core and research focus of a document, while the in-depth analysis of keyword co-occurrence can identify the research hotspots and trends more quickly. In this part, the visualized clustering analysis of keywords, combined with the color clustering map and the emergence map, was used to analyze the hot spots and frontiers of the current research on mitochondria and HF. According to the results, oxidative stress, metabolic disorders, mitochondrial Ca2+homeostasis imbalance, mitochondrial quality control and inflammation-mediated mitochondrial dysfunction in the pathogenesis of HF are the research hotspots, which are summarized as follows.
4.3 Activation mechanism of mitochondrial dysfunction in HF
4.3.1 Oxidative stress
Studies have confirmed that the damage caused by mitochondrial ROS (mROS) is the main pathogenic factor of HF. Scholars in early time generally believed that ROS is a by-product of mitochondrial respiration. With the deepening of research, people realized that when the generation rate of ROS exceeds the clearance rate or the endogenous antioxidant capacity is weakened, oxidative stress will occur, which will lead to heart damage (Wong et al., 2017; van der Pol et al., 2019). Excessive release of ROS in pathological conditions can induce nonspecific damage to cell membranes, proteins, and DNA, and these responses are particularly evident in mitochondria, where DNA and enzymes in the tricarboxylic acid (TCA) cycle are highly sensitive to oxidative damage. On the one hand, ROS-induced mitochondrial damage may in turn affect energy production. On the other hand, increasingly severe mitochondrial dysfunction can trigger sterile inflammation and cardiomyocyte death in the heart, which also contributes to the pathological progression of HF (Okonko and Shah, 2015). A research proposed that the elevated mROS is a major source of oxidative stress in HF and the reduced mROS in vivo may mitigate sudden cardiac death (SCD). It has been found that excess mROS may lead to HF and SCD by inhibiting or altering metabolic phosphorylation, antioxidants, and ion transporter networks. Reducing the level of mROS can prevent and reverse SCD and HF. At the same time, the results of this study also confirm the feasibility of mitochondria as potential therapeutic targets for HF and SCD (Dey et al., 2018). It has also been found that oxidative stress may interact with mitochondrial dysfunction in the development of HF. In the failing heart, ATP synthase activity is inhibited due to increased protein acetylation, which hinders ATP production, impairs electron flow and enhances ROS levels. The overproduced ROS interacts with cellular and mitochondrial components, such as proteins, DNA, lipids, and other molecules, which in turn exacerbate mitochondrial damage leading to more severe HF (Zorov et al., 2014). In addition, mROS has also been shown to be a molecular mediator of hypoxia signaling pathway, MAP kinase pathway, inflammatory pathway and retrograde communication between mitochondria and nucleus, but whether these mechanisms are related to the pathogenesis of HF still needs further research to prove (Hamanaka and Chandel, 2010; Ray et al., 2012; Javadov et al., 2014).
4.3.2 Mitochondrial metabolic pathway
Normally, the energy metabolism of the heart is closely coordinated with the utilization of substrates to meet the energy needs of the human body. The oxidation of fatty acids, glucose, ketone bodies and other amino acids in myocardial mitochondria provides a large amount of ATP for the heart (Bedi et al., 2016). However, myocardial substrate metabolism is severely impaired in HF, and the selective utilization of substrates such as FA, glucose and ketone bodies is reconstructed, resulting in myocardial energy metabolism disorders, which further leads to the deterioration of HF (Bertero and Maack, 2018a). Down-regulation of fatty acid oxidation and increased glucose utilization in cardiac hypertrophy have been identified as markers of metabolic remodeling (Zhou and Tian, 2018). FA has always been regarded as the main “fuel” for heart operation, which can provide 70% of ATP for the heart (Bedi et al., 2016). It has been found that FA oxidation is significantly reduced in different HF models, so various FA oxidation stimulation schemes have been widely involved in the prevention and treatment of HF and have achieved some results (Bedi et al., 2016; Legchenko et al., 2018). However, it has also been found that increased FA uptake and oxidation rate may lead to the accumulation of lipotoxic metabolites and cardiac damage (Goldberg et al., 2012). Therefore, it still needs further exploration to find a reasonable treatment plan. Additionally, appropriate regulation of glucose metabolism may also be a potential treatment for HF. The dependence of the heart on glucose in pathological conditions leads to increased glycolysis. The decoupling of glycolysis from oxidation leads to increased cytoplasmic lactate production in mitochondria, which further reduces the efficiency of ATP synthesis. And exacerbates cardiac pathological remodeling (Bertero and Maack, 2018b). For example, sodium-glucose cotransporter 2 (SGLT2) inhibitors are effective hypoglycemic drugs, and some studies have found that SGLT2 inhibitors significantly reduce the risk of death and rehospitalization in patients with HF, but the specific mechanism still needs to be studied (Zannad et al., 2020). In recent years, more and more attention has been paid to the study of ketone body metabolism and HF. Some studies have found that the level of ketone body oxidation increases during HF, which is related to the increase of circulating ketone levels in patients with HF (Takahara et al., 2022). Another hypothesis is that ketones are more likely to function as signaling molecules in disease (Newman and Verdin, 2014). Although new research suggests that the increase in ketone body oxidation is cardioprotective (Monzo et al., 2021). However, it is still controversial whether increasing ketone body content or its oxidation can promote ATP production to further improve cardiac function.
4.3.3 Dysregulated mitochondrial Ca2+homeostasis
Ca2+homeostasis imbalance has long been considered as a marker of HF (Santulli et al., 2015). It is well known that Ca2+ plays a central role in the regulation of excitation-contraction coupling (ECC) in cardiomyocytes. Under normal conditions, the transient increase of Ca2+ level in cardiomyocytes stimulates myocardial contraction. Mitochondria accumulate Ca2+ during contraction and participate in mitochondrial oxidative phosphorylation and ATP synthesis in the electron transport chain (ETC). In the early stages of HF, the increased energy demand of the heart triggers a complementary regulation of mitochondrial oxidative phosphorylation via Ca2+ and ADP, which ultimately leads to a disruption of Ca2+homeostasis in myocardial mitochondria. As a ubiquitous second messenger, Ca2+ is involved in the regulation of a variety of biological processes, including oxidative phosphorylation, ATP synthesis, ROS generation, mPTP opening and mitochondrial autophagy. These effects make Ca2+ an important regulator of MF (Xu et al., 2020). In addition, in the presence of mitochondrial Ca2+homeostasis imbalance, Ca2+ reuptake by the sarcoplasmic reticulum (SR) is impaired and Ca (TS4) leakage through ryanodine receptors (RYR) is increased, which results in a transient decrease in cytosolic Ca (TS4) during excitation but an increase in cytosolic Ca2+ at baseline, causing Ca2+ overload (Odagiri et al., 2009; Santulli et al., 2015). Ca2+ overload can lead to the opening of mitochondrial permeability transition pore (mPTP), the increase of mitochondrial oxidative stress, the collapse of mitochondrial membrane potential, the disorder of ATP production and the necrosis of myocardial cells, and finally induce HF (Cao et al., 2019; Smith and Eisner, 2019; Xu et al., 2020). The mechanism of Ca2+ overload is still unclear. The mitochondrial calcium uniporter (MCU) complex is an ion channel located in the mitochondrial inner membrane (IMM), which can transport Ca2+ into the mitochondrial matrix. It is essential to prevent mitochondrial Ca2+ overload and maintain mitochondrial Ca2+homeostasis (Yu et al., 2018). It has been found that the level of mitochondrial MCU protein in the heart of mice with pressure-induced cardiac hypertrophy is significantly increased, which implies that the level of MCU will increase compensatively when the myocardium is short of energy, resulting in increased mitochondrial Ca2+ uptake to promote ATP synthesis (Zaglia et al., 2017). However, it has also been found that MCU may mediate acute mitochondrial Ca2+ overload during acute ischemic injury, which ultimately induces cardiomyocyte death. Taken together, these finding provide insight into that potential of MCU complexes for target therapy of HF (Garbincius et al., 2020). On the other hand, the mitochondrial (Na+/Ca2+)exchanger (NCLX) is believed to be the main pathway for Ca2+ efflux to the cytoplasm, which is essential for maintaining Ca2+ homeostasis (Takeuchi and Matsuoka, 2021). Conditional knockout of NCLX has been found to lead to rapid fatal HF (Kostic and Sekler, 2019). Overexpression of NCLX has also been shown to increase mitochondrial calcium efflux and prevent the onset of HF by reducing the production of reactive oxygen species and limiting myocardial fibrosis in animal experiments. NCLX has strong research potential as a target for HF intervention (Zaglia et al., 2017).
4.3.4 Mitochondrial quality control
Mitochondrial quality control refers to the coordinated biogenesis, mitochondrial dynamics and autophagy of myocardial mitochondria that maintain their normal morphology, quantity and quality, so as to ensure the normal function of mitochondria. Failure of mitochondrial quality control leads to cardiomyocyte dysfunction and ultimately to HF (Qiu et al., 2019). Defects in mitochondrial biogenesis have been found to reduce myocardial ATP supply and trigger mitochondrial dysfunction, peroxisome proliferator-activated receptor-γ coactivator-1α (PGC-1α) and SIRT1 and SIRT3 among type III histone deacetylases (sirtuins, SIRTs) have important effects on mitochondrial biogenesis. SIRT1 is the upstream regulator of PGC-1α, and overexpression of SIRT1 can enhance the deacetylation of PGC-1α and promote mitochondrial biogenesis. PGC-1α promotes the binding of the transcription factor estrogen related receptor α (ERRα) to the ERRα response element located in the SIRT3 promoter, which in turn activates SIRT3 expression (Koentges et al., 2015; Wang et al., 2020). Recent studies have also found that melatonin promotes mitochondrial biogenesis by increasing the expression of SIRT1 and PGC-1α. (Niu et al., 2020); The concept of mitochondrial dynamics was first proposed by Lewis et al., In 1914. The team believed that mitochondria changed their shape too frequently by fusion and fission to maintain the stability of their network structure (Lewis and Lewis, 1914). Mitochondrial fusion and fission occur mainly in the inner and outer mitochondrial membranes and are controlled by a group of dynamic-related regulatory proteins containing conserved GTPase domains. Mitochondrial fission occurs only in the outer mitochondrial membrane, and its regulatory proteins include dynamic-related protein 1 (Drp1), mitochondrial fission protein 1 (Fis1), and mitochondrial fission factor (MFF) (Westermann, 2010; Schmitt et al., 2018; Qiu et al., 2019). It has been found that activation of Drp1 can induce mitochondrial damage and cardiac dysfunction, while inhibition of Drp1 signaling can alleviate mitochondrial dysfunction during HF (Xia et al., 2017). Mitochondrial fusion is a beneficial process in which healthy mitochondria can replace damaged mitochondria and restore their activity to maintain normal metabolism. Mitochondrial fusion plays an important role in mitotic protein one and 2 (Mfn1, Mfn2), fuzzy onions 1 (Fzo1) and optic atrophy associated protein 1 (OPA1) (Gao and Hu, 2021). It has been found that disruption of mitochondrial fusion induced by Mfn1 and Mfn2 knockout can cause fatal HF (Chen et al., 2011). It has also been shown that OPA1-mediated mitochondrial fragmentation triggers dilated cardiomyopathy and HF in mice (Wai et al., 2015); Mitochondrial autophagy is an important mediator of mitochondrial quality control in cardiomyocytes. Under pathological conditions, cells achieve self-renewal and normal metabolism by activating autophagy and other pathways. In the process of mitophagy, defective mitochondria are labeled by ubiquitin chains, and then phagocytized into double-layer vesicles and autophagosomes, which are finally transmitted to lysosomes for degradation and clearance (Shirakabe et al., 2016). At present, mitochondrial autophagy in cardiomyocytes has attracted much attention, and PTEN-induced putative kinase-1 (PINK1) and E3 ubiquitin ligase Parkin-mediated autophagy are the most studied (Rub et al., 2017). The role of BAG3 in the regulation of mitochondrial dynamics and mitochondrial quality control in cardiomyocytes has also been reported, but the exact underlying mechanism remains unclear (Tahrir et al., 2017).
4.3.5 Inflammation correlation with mitochondrial dysfunction
HF with reduced ejection fraction is associated with increased levels of proinflammatory cytokines, which reveals the potential role of the immune system in the pathogenesis of HF. However, the results of all treatments for HF inflammation in clinical trials so far are not satisfactory, and the pathogenic mechanism of inflammation in disease progression is still unclear (Adamo et al., 2021). Most HFs are aseptic inflammation, a chronic inflammatory state characterized by activation of the innate immune system. The innate immune system is activated by the recognition of specific receptors, namely, pattern recognition receptors (PRRs), by pathogen-associated molecular patterns (PAMPs) or damage-associated molecular patterns (DAMPs), which are derived from host cells and released in response to cellular stress or tissue injury. The NLRP3 inflammasome is an important part of the PPRs signaling pathway (Katsuumi et al., 2016). Recently, more scholars have found that NLRP3 inflammasome is closely related to the activation of mitochondrial DAMPs, and the activation of NLRP3 inflammasome is one of the important mechanisms to ensure the normal function of mitochondria (Zhang et al., 2010; Dela and Kang, 2018). Currently, it has been found that overexpression of NOX1 and NOX4 in cardiomyocytes can activate dynamic-related protein 1 (Drp1) and induce mitochondrial fission, which further activates NLRP3-mediated cardiomyocyte apoptosis in a caspase-1-dependent manner, resulting in myocardial dysfunction (Zeng et al., 2020).
4.4 Research progress of mitochondrial targeting therapy for HF
At present, the treatment of HF has entered a stage that exploring the pathogenesis of HF and finding the corresponding treatment are urgent. In the past, the drugs for the treatment of HF mainly included beta blockers, angiotensin-converting enzyme inhibitors, angiotensin receptor blockers (ARB), vasodilators and diuretics. It works mainly by reducing the load on the failing heart (Brown et al., 2017). Based on the above analysis, the recovery of MF in the treatment of HF is the best way to fundamentally solve the problem of energy imbalance, and mitochondria can be used as an important target for the development of therapeutic intervention drugs for HF. Several drugs targeting treating HF have been listed, mainly through the prevention of oxidative damage, regulating myocardial substrate metabolism, improving mitochondrial quality control, maintaining mitochondrial Ca2+homeostasis, and anti-inflammatory.
Coenzyme Q10 (CoQ10) is a fat-soluble quinone present in all cells. It is one of the substances involved in the electron transport chain and aerobic respiration in the mitochondria of eukaryotic cells. As a natural antioxidant, CoQ10 has been shown to be effective in a variety of metabolic diseases (Martelli et al., 2020). At present, the role of CoQ10 in the treatment of HF has been generally recognized. In 1994, an Italian study of 2664 patients with HF for 3 months found that CoQ10 could significantly improve the clinical sign and symptom of patients, improve the quality of life of patients, and has a high safety (Baggio et al., 1994). MitoQ is a mitochondria-targeted antioxidant with strong antioxidant activity (Botting et al., 2020). MitoQ has been shown to improve mitochondrial dysfunction in rat HF by reducing hydrogen peroxide formation, improving mitochondrial respiration, and modulating mPTP opening (Ribeiro et al., 2018). Randomized controlled studies have also shown that oral administration of MitoQ for 6 weeks can improve vascular endothelial function and reduce aortic stiffness in the elderly, but there are few clinical trials on the specific treatment of HF (Rossman et al., 2018); Studies have shown that SGLT2 inhibitor can benefit patients with cardiovascular disease by improve substrate availability and myocardial energy metabolism. Commonly used SGLT2 inhibitors are canagliflozin, dapagliflozin, empagliflozin and ertugliflozin. They decrease the maximal ability of the renal tubules to reabsorb glucose, thereby increasing the excretion of glucose in the urine and decreasing the concentration of plasma glucose, thereby improving glucose metabolism (Cowie and Fisher, 2020). It has also been found that trimetazidine can improve isoproterenol-induced myocardial metabolic remodeling in HF rats by activating AMPK and PPARα to regulate myocardial substrate utilization, especially ketone body metabolism (Li et al., 2020); It has been found that mitochondrial quality control regulators are also potential new drug targets in mitochondrial therapy. Mdivi-1 is an inhibitor of mitochondrial fission protein Drp1, and early studies have shown that it may improve the therapeutic effect of myocardial infarction by altering mitochondrial dynamics. However, the use of mdivi-1 treatment in clinically relevant large animal models of myocardial infarction did not reduce the size of myocardial infarction or improve cardiac function, so the relevant studies still need to be validated in large samples (Ong et al., 2019); CDGSH iron-sulfur domain protein 2 (Cisd2) is critical for mitochondrial integrity and intracellular Ca2+homeostasis. Cisd2 deficiency disrupts Ca2+homeostasis by inducing dysregulation of muscle/endoplasmic reticulum Ca2+-ATPase (Serca2a) activity and leads to mitochondrial Ca2+overload in cardiomyocytes. It has been found that Cisd2 activators can up-regulate Cisd2 expression, enhance mitochondrial and Serca2a activity, and maintain intracellular Ca2+homeostasis. Thereby reducing age-related cardiac dysfunction and slowing the progression of HF. In addition, the study highlights Cisd2 as a novel drug target for the development of therapies to delay cardiac dysfunction (Yeh et al., 2019); The relationship between inflammation and the onset of HF has long been recognized, but current therapies aimed at reducing inflammation in patients with HF have not shown appreciable results (Adamo et al., 2020). The hypothesis that the interleukin-1β inhibitor canakinumab reduces the hospitalization rate and mortality rate in patients with HF has been proposed, and the final results show that the efficacy of canakinumab increases in a dose-dependent manner, which is convincing (Everett et al., 2019). In conclusion, the development of new treatments for mitochondrial dysfunction has great research potential, which is of great practical significance to reduce the economic burden of patients with HF and improve their symptoms.
5 Limitations
This study is based on the WoSCC database for visualized analysis, and some limitations are: (van der Meer et al., 2019): The retrieval time of this study is limited to the literature published in the past 20 years from January 2002 to December 2021, but with the update of the WoSCC database, the actual number of literature will continue to change; (Ziaeian and Fonarow, 2016); Because the bibliometrics software can not distinguish the author’s initials at present, there may be wrong conclusions in the author’s analysis part of this paper; (Yancy et al., 2017); Due to the limited number of keywords included in the literature, some core words may be omitted in the analysis process, so the analysis results may be affected; (Yancy et al., 2013); Because the retrieval of this study was limited to the SCI-E index database in WOS, the literature not included in the SCI-E index database was not included in the analysis. However, the results of this study reveal the research status, hot spots and trends of the relationship between MF and the pathogenesis of HF, which can provide some reference for researchers to further explore in this field.
6 Conclusion
Based on the WoSCC database, this study conducted a bibliometric analysis of 4236 studies published from 2002 to 2021 on the relationship between MF and HF. The results show that the number of papers on the relationship between mitochondria and HF has increased year by year in the past 20 years, and China and the United States are the leading countries in this field. At the same time, UNIVERSITY OF CALIFORNIA SYSTEM is the research institution that has the greatest impacts on the research results, and Yip H K is the most fruitful author in this field. American Journal of Physiology-heart and Circulatory Physiology is probably the most popular journal, and most of the articles published on mitochondria and HF are cited from internationally influential journals. In addition, oxidative stress, metabolic dysfunction, mitochondrial Ca2+homeostasis imbalance, mitochondrial quality control and mitochondrial dysfunction mediated by inflammation in the pathogenesis of HF are the hot spots. To sum up, the study of the relationship between mitochondria and HF has broad prospects for development, and targeted regulating of mitochondria will also be the focus of future research on the prevention and treatment of HF.
Data availability statement
The raw data supporting the conclusion of this article will be made available by the authors, without undue reservation.
Author contributions
XQ, ZZ, and JL contributed to conception and design of the study. ZJ, LZ, and YP collected the data. XQ wrote the first draft of the manuscript. ZW and JL reviewed and revised the manuscript. All authors contributed to the article and approved the submitted version.
Funding
This work is supported by the National Natural Science Foundation of China (No. 82160887), Guangxi Natural Science Fundation (No. 2021GXNSFBA196018),Guangxi Natural Science Fundation (No. 2021GXNSFAA220111).
Acknowledgments
The authors thank the First Affiliated Hospital of Guangxi University of Chinese Medicine, for their support of this workand the reviewers for allowing us to make improvements to the manuscript.
Conflict of interest
The authors declare that the research was conducted in the absence of any commercial or financial relationships that could be construed as a potential conflict of interest.
Publisher’s note
All claims expressed in this article are solely those of the authors and do not necessarily represent those of their affiliated organizations, or those of the publisher, the editors and the reviewers. Any product that may be evaluated in this article, or claim that may be made by its manufacturer, is not guaranteed or endorsed by the publisher.
References
Adamo, L., Rocha-Resende, C., Prabhu, S. D., and Mann, D. L. (2021). Publisher correction: Reappraising the role of inflammation in heart failure. Nat. Rev. Cardiol. 18, 735. doi:10.1038/s41569-021-00534-3
Adamo, L., Rocha-Resende, C., Prabhu, S. D., and Mann, D. L. (2020). Reappraising the role of inflammation in heart failure. Nat. Rev. Cardiol. 17, 269–285. doi:10.1038/s41569-019-0315-x
Baggio, E., Gandini, R., Plancher, A. C., Passeri, M., and Carmosino, G. (1994). Italian multicenter study on the safety and efficacy of coenzyme q10 as adjunctive therapy in heart failure. Coq10 drug surveillance investigators. Mol. Asp. Med. 15 (15), s287–s294. doi:10.1016/0098-2997(94)90040-x
Bedi, K. J., Snyder, N. W., Brandimarto, J., Aziz, M., Mesaros, C., Worth, A. J., et al. (2016). Evidence for intramyocardial disruption of lipid metabolism and increased myocardial ketone utilization in advanced human heart failure. Circulation 133, 706–716. doi:10.1161/CIRCULATIONAHA.115.017545
Bertero, E., and Maack, C. (2018). Metabolic remodelling in heart failure. Nat. Rev. Cardiol. 15, 457–470. doi:10.1038/s41569-018-0044-6
Bertero, E., and Maack, C. (2018). Reply to 'metabolic remodelling in heart failure revisited. Nat. Rev. Cardiol. 15, 780–781. doi:10.1038/s41569-018-0116-7
Botting, K. J., Skeffington, K. L., Niu, Y., Allison, B. J., Brain, K. L., Itani, N., et al. (2020). Translatable mitochondria-targeted protection against programmed cardiovascular dysfunction. Sci. Adv. 6, b1929. doi:10.1126/sciadv.abb1929
Brown, D. A., Perry, J. B., Allen, M. E., Sabbah, H. N., Stauffer, B. L., Shaikh, S. R., et al. (2017). Expert consensus document: Mitochondrial function as a therapeutic target in heart failure. Nat. Rev. Cardiol. 14, 238–250. doi:10.1038/nrcardio.2016.203
Cao, J. L., Adaniya, S. M., Cypress, M. W., Suzuki, Y., Kusakari, Y., Jhun, B. S., et al. (2019). Role of mitochondrial ca(2+) homeostasis in cardiac muscles. Arch. Biochem. Biophys. 663, 276–287. doi:10.1016/j.abb.2019.01.027
Cappetta, D., Esposito, G., Coppini, R., Piegari, E., Russo, R., Ciuffreda, L. P., et al. (2017). Effects of ranolazine in a model of doxorubicin-induced left ventricle diastolic dysfunction. Br. J. Pharmacol. 174, 3696–3712. doi:10.1111/bph.13791
Chaomei, C. (2006). Citespace ii: Detecting and visualizing emerging trends and transient patterns in scientific literature. J. Am. Soc. Inf. Sci. Technol. 57, 359–377. doi:10.1002/asi.20317
Chen, Y., Liu, Y., and Dorn, G. N. (2011). Mitochondrial fusion is essential for organelle function and cardiac homeostasis. Circ. Res. 109, 1327–1331. doi:10.1161/CIRCRESAHA.111.258723
Cowie, M. R., and Fisher, M. (2020). Sglt2 inhibitors: Mechanisms of cardiovascular benefit beyond glycaemic control. Nat. Rev. Cardiol. 17, 761–772. doi:10.1038/s41569-020-0406-8
Del, C. A., Perez, G., Castro, P. F., Parra, V., and Verdejo, H. E. (2021). Mitochondrial function, dynamics and quality control in the pathophysiology of hfpef. Biochim. Biophys. Acta. Mol. Basis Dis. 1867, 166208. doi:10.1016/j.bbadis.2021.166208
Dela, C. C., and Kang, M. J. (2018). Mitochondrial dysfunction and damage associated molecular patterns (damps) in chronic inflammatory diseases. Mitochondrion 41, 37–44. doi:10.1016/j.mito.2017.12.001
Dey, S., DeMazumder, D., Sidor, A., Foster, D. B., and O'Rourke, B. (2018). Mitochondrial ros drive sudden cardiac death and chronic proteome remodeling in heart failure. Circ. Res. 123, 356–371. doi:10.1161/CIRCRESAHA.118.312708
Dubois-Deruy, E., Peugnet, V., Turkieh, A., and Pinet, F. (2020). Oxidative stress in cardiovascular diseases. Antioxidants (Basel) 9, E864. doi:10.3390/antiox9090864
Everett, B. M., Cornel, J. H., Lainscak, M., Anker, S. D., Abbate, A., Thuren, T., et al. (2019). Anti-inflammatory therapy with canakinumab for the prevention of hospitalization for heart failure. Circulation 139, 1289–1299. doi:10.1161/CIRCULATIONAHA.118.038010
Gao, S., and Hu, J. (2021). Mitochondrial fusion: The machineries in and out. Trends Cell Biol. 31, 62–74. doi:10.1016/j.tcb.2020.09.008
Gao, Y., Wang, F., Song, Y., and Liu, H. (2020). The status of and trends in the pharmacology of berberine: A bibliometric review [1985-2018. Chin. Med. 15, 7. doi:10.1186/s13020-020-0288-z
Garbincius, J. F., Luongo, T. S., and Elrod, J. W. (2020). The debate continues - what is the role of mcu and mitochondrial calcium uptake in the heart? J. Mol. Cell. Cardiol. 143, 163–174. doi:10.1016/j.yjmcc.2020.04.029
Goldberg, I. J., Trent, C. M., and Schulze, P. C. (2012). Lipid metabolism and toxicity in the heart. Cell Metab. 15, 805–812. doi:10.1016/j.cmet.2012.04.006
Hamanaka, R. B., and Chandel, N. S. (2010). Mitochondrial reactive oxygen species regulate cellular signaling and dictate biological outcomes. Trends biochem. Sci. 35, 505–513. doi:10.1016/j.tibs.2010.04.002
Huang, C. Y., Kuo, W. W., Ho, T. J., Chiang, S. F., Pai, P. Y., Lin, J. Y., et al. (2018). Rab9-dependent autophagy is required for the igf-iir triggering mitophagy to eliminate damaged mitochondria. J. Cell. Physiol. 233, 7080–7091. doi:10.1002/jcp.26346
Huang, C. Y., Lai, C. H., Kuo, C. H., Chiang, S. F., Pai, P. Y., Lin, J. Y., et al. (2018). Inhibition of erk-drp1 signaling and mitochondria fragmentation alleviates igf-iir-induced mitochondria dysfunction during heart failure. J. Mol. Cell. Cardiol. 122, 58–68. doi:10.1016/j.yjmcc.2018.08.006
Javadov, S., Jang, S., and Agostini, B. (2014). Crosstalk between mitogen-activated protein kinases and mitochondria in cardiac diseases: Therapeutic perspectives. Pharmacol. Ther. 144, 202–225. doi:10.1016/j.pharmthera.2014.05.013
Katsuumi, G., Shimizu, I., Yoshida, Y., and Minamino, T. (2016). The pathological role of vascular aging in cardio-metabolic disorder. Inflamm. Regen. 36, 16. doi:10.1186/s41232-016-0021-6
Keshavarz-Bahaghighat, H., Darwesh, A. M., Sosnowski, D. K., and Seubert, J. M. (2020). Mitochondrial dysfunction and inflammaging in heart failure: Novel roles of cyp-derived epoxylipids, Cells 9, E1565. doi:10.3390/cells9071565
Koentges, C., Pfeil, K., Schnick, T., Wiese, S., Dahlbock, R., Cimolai, M. C., et al. (2015). Sirt3 deficiency impairs mitochondrial and contractile function in the heart. Basic Res. Cardiol. 110, 36. doi:10.1007/s00395-015-0493-6
Kostic, M., and Sekler, I. (2019). Functional properties and mode of regulation of the mitochondrial na(+)/ca(2+) exchanger, nclx. Semin. Cell Dev. Biol. 94, 59–65. doi:10.1016/j.semcdb.2019.01.009
Legchenko, E., Chouvarine, P., Borchert, P., Fernandez-Gonzalez, A., Snay, E., Meier, M., et al. (2018). PPARγ agonist pioglitazone reverses pulmonary hypertension and prevents right heart failure via fatty acid oxidation. Sci. Transl. Med. 10, eaao0303. doi:10.1126/scitranslmed.aao0303
Lewis, M. R., and Lewis, W. H. (1914). Mitochondria in tissue culture. Science 39, 330–333. doi:10.1126/science.39.1000.330
Li, H., Ma, Z., Zhai, Y., Lv, C., Yuan, P., Zhu, F., et al. (2020). Trimetazidine ameliorates myocardial metabolic remodeling in isoproterenol-induced rats through regulating ketone body metabolism via activating AMPK and PPAR α. Front. Pharmacol. 11, 1255. doi:10.3389/fphar.2020.01255
Lopaschuk, G. D., Karwi, Q. G., Tian, R., Wende, A. R., and Abel, E. D. (2021). Cardiac energy metabolism in heart failure. Circ. Res. 128, 1487–1513. doi:10.1161/CIRCRESAHA.121.318241
Ma, D., Yang, B., Guan, B., Song, L., Liu, Q., Fan, Y., et al. (2021). A bibliometric analysis of pyroptosis from 2001 to 2021. Front. Immunol. 12, 731933. doi:10.3389/fimmu.2021.731933
Martelli, A., Testai, L., Colletti, A., and Cicero, A. (2020). Coenzyme q10: Clinical applications in cardiovascular diseases. Antioxidants (Basel) 9, E341. doi:10.3390/antiox9040341
Monzo, L., Sedlacek, K., Hromanikova, K., Tomanova, L., Borlaug, B. A., Jabor, A., et al. (2021). Myocardial ketone body utilization in patients with heart failure: The impact of oral ketone ester. Metabolism. 115, 154452. doi:10.1016/j.metabol.2020.154452
Neubauer, S. (2007). The failing heart-an engine out of fuel. N. Engl. J. Med. 356, 1140–1151. doi:10.1056/NEJMra063052
Newman, J. C., and Verdin, E. (2014). Ketone bodies as signaling metabolites. Trends Endocrinol. Metab. 25, 42–52. doi:10.1016/j.tem.2013.09.002
Niu, Y. J., Zhou, W., Nie, Z. W., Shin, K. T., and Cui, X. S. (2020). Melatonin enhances mitochondrial biogenesis and protects against rotenone-induced mitochondrial deficiency in early porcine embryos. J. Pineal Res. 68, e12627. doi:10.1111/jpi.12627
O'Rourke, B., Ashok, D., and Liu, T. (2021). Mitochondrial ca(2+) in heart failure: Not enough or too much? J. Mol. Cell. Cardiol. 151, 126–134. doi:10.1016/j.yjmcc.2020.11.014
Odagiri, K., Katoh, H., Kawashima, H., Tanaka, T., Ohtani, H., Saotome, M., et al. (2009). Local control of mitochondrial membrane potential, permeability transition pore and reactive oxygen species by calcium and calmodulin in rat ventricular myocytes. J. Mol. Cell. Cardiol. 46, 989–997. doi:10.1016/j.yjmcc.2008.12.022
Okonko, D. O., and Shah, A. M. (2015). Heart failure: Mitochondrial dysfunction and oxidative stress in chf. Nat. Rev. Cardiol. 12, 6–8. doi:10.1038/nrcardio.2014.189
Ong, S. B., Kwek, X. Y., Katwadi, K., Hernandez-Resendiz, S., Crespo-Avilan, G. E., Ismail, N. I., et al. (2019). Targeting mitochondrial fission using mdivi-1 in a clinically relevant large animal model of acute myocardial infarction: A pilot study. Int. J. Mol. Sci. 20, E3972. doi:10.3390/ijms20163972
Qiu, Z., Wei, Y., Song, Q., Du, B., Wang, H., Chu, Y., et al. (2019). The role of myocardial mitochondrial quality control in heart failure. Front. Pharmacol. 10, 1404. doi:10.3389/fphar.2019.01404
Ray, P. D., Huang, B. W., and Tsuji, Y. (2012). Reactive oxygen species (ros) homeostasis and redox regulation in cellular signaling. Cell. Signal. 24, 981–990. doi:10.1016/j.cellsig.2012.01.008
Ribeiro, J. R., Dabkowski, E. R., Shekar, K. C., K, O. C., Hecker, P. A., and Murphy, M. P. (2018). Mitoq improves mitochondrial dysfunction in heart failure induced by pressure overload. Free Radic. Biol. Med. 117, 18–29. doi:10.1016/j.freeradbiomed.2018.01.012
Roman, B., Kaur, P., Ashok, D., Kohr, M., Biswas, R., O'Rourke, B., et al. (2020). Nuclear-mitochondrial communication involving mir-181c plays an important role in cardiac dysfunction during obesity. J. Mol. Cell. Cardiol. 144, 87–96. doi:10.1016/j.yjmcc.2020.05.009
Rossignol, P., Hernandez, A. F., Solomon, S. D., and Zannad, F. (2019). Heart failure drug treatment. Lancet 393, 1034–1044. doi:10.1016/S0140-6736(18)31808-7
Rossman, M. J., Santos-Parker, J. R., Steward, C., Bispham, N. Z., Cuevas, L. M., Rosenberg, H. L., et al. (2018). Chronic supplementation with a mitochondrial antioxidant (mitoq) improves vascular function in healthy older adults. Hypertension 71, 1056–1063. doi:10.1161/HYPERTENSIONAHA.117.10787
Rub, C., Wilkening, A., and Voos, W. (2017). Mitochondrial quality control by the pink1/parkin system. Cell Tissue Res. 367, 111–123. doi:10.1007/s00441-016-2485-8
Sabbah, H. N. (2020). Targeting the mitochondria in heart failure: A translational perspective. JACC. Basic Transl. Sci. 5, 88–106. doi:10.1016/j.jacbts.2019.07.009
Santulli, G., Xie, W., Reiken, S. R., and Marks, A. R. (2015). Mitochondrial calcium overload is a key determinant in heart failure. Proc. Natl. Acad. Sci. U. S. A. 112, 11389–11394. doi:10.1073/pnas.1513047112
Schmitt, K., Grimm, A., Dallmann, R., Oettinghaus, B., Restelli, L. M., Witzig, M., et al. (2018). Circadian control of drp1 activity regulates mitochondrial dynamics and bioenergetics. Cell Metab. 27, 657–666. doi:10.1016/j.cmet.2018.01.011
Shirakabe, A., Zhai, P., Ikeda, Y., Saito, T., Maejima, Y., Hsu, C. P., et al. (2016). Drp1-dependent mitochondrial autophagy plays a protective role against pressure overload-induced mitochondrial dysfunction and heart failure. Circulation 133, 1249–1263. doi:10.1161/CIRCULATIONAHA.115.020502
Smith, G. L., and Eisner, D. A. (2019). Calcium buffering in the heart in health and disease. Circulation 139, 2358–2371. doi:10.1161/CIRCULATIONAHA.118.039329
Tahrir, F. G., Knezevic, T., Gupta, M. K., Gordon, J., Cheung, J. Y., Feldman, A. M., et al. (2017). Evidence for the role of bag3 in mitochondrial quality control in cardiomyocytes. J. Cell. Physiol. 232, 797–805. doi:10.1002/jcp.25476
Tahrir, F. G., Langford, D., Amini, S., Mohseni, A. T., and Khalili, K. (2019). Mitochondrial quality control in cardiac cells: Mechanisms and role in cardiac cell injury and disease. J. Cell. Physiol. 234, 8122–8133. doi:10.1002/jcp.27597
Takahara, S., Soni, S., Maayah, Z. H., Ferdaoussi, M., and Dyck, J. (2022). Ketone therapy for heart failure: Current evidence for clinical use. Cardiovasc. Res. 118, 977–987. doi:10.1093/cvr/cvab068
Takeuchi, A., and Matsuoka, S. (2021). Physiological and pathophysiological roles of mitochondrial na(+)-ca(2+) exchanger, nclx, in hearts. Biomolecules 11, 1876. doi:10.3390/biom11121876
van der Meer, P., Gaggin, H. K., and Dec, G. W. (2019). Acc/aha versus esc guidelines on heart failure: Jacc guideline comparison. J. Am. Coll. Cardiol. 73, 2756–2768. doi:10.1016/j.jacc.2019.03.478
van der Pol, A., van Gilst, W. H., Voors, A. A., and van der Meer, P. (2019). Treating oxidative stress in heart failure: Past, present and future. Eur. J. Heart Fail. 21, 425–435. doi:10.1002/ejhf.1320
van Eck, N. J., and Waltman, L. (2010). Software survey: Vosviewer, a computer program for bibliometric mapping. Scientometrics 84, 523–538. doi:10.1007/s11192-009-0146-3
Wai, T., Garcia-Prieto, J., Baker, M. J., Merkwirth, C., Benit, P., Rustin, P., et al. (2015). Imbalanced opa1 processing and mitochondrial fragmentation cause heart failure in mice. Science 350, aad0116. doi:10.1126/science.aad0116
Wang, X., Shen, K., Wang, J., Liu, K., Wu, G., Li, Y., et al. (2020). Hypoxic preconditioning combined with curcumin promotes cell survival and mitochondrial quality of bone marrow mesenchymal stem cells, and accelerates cutaneous wound healing via PGC-1α/SIRT3/HIF-1α signaling. Free Radic. Biol. Med. 159, 164–176. doi:10.1016/j.freeradbiomed.2020.07.023
Westermann, B. (2010). Mitochondrial fusion and fission in cell life and death. Nat. Rev. Mol. Cell Biol. 11, 872–884. doi:10.1038/nrm3013
Wong, H. S., Dighe, P. A., Mezera, V., Monternier, P. A., and Brand, M. D. (2017). Production of superoxide and hydrogen peroxide from specific mitochondrial sites under different bioenergetic conditions. J. Biol. Chem. 292, 16804–16809. doi:10.1074/jbc.R117.789271
Xia, Y., Chen, Z., Chen, A., Fu, M., Dong, Z., Hu, K., et al. (2017). Lcz696 improves cardiac function via alleviating drp1-mediated mitochondrial dysfunction in mice with doxorubicin-induced dilated cardiomyopathy. J. Mol. Cell. Cardiol. 108, 138–148. doi:10.1016/j.yjmcc.2017.06.003
Xu, H. X., Cui, S. M., Zhang, Y. M., and Ren, J. (2020). Mitochondrial ca(2+) regulation in the etiology of heart failure: Physiological and pathophysiological implications. Acta Pharmacol. Sin. 41, 1301–1309. doi:10.1038/s41401-020-0476-5
Yancy, C. W., Jessup, M., Bozkurt, B., Butler, J., Casey, D. J., Colvin, M. M., et al. (2017). 2017 ACC/AHA/HFSA focused update of the 2013 ACCF/AHA guideline for the management of heart failure: A report of the American college of Cardiology/American heart association task force on clinical practice guidelines and the heart failure society of America. Circulation 136, e137–e161. doi:10.1161/CIR.0000000000000509
Yancy, C. W., Jessup, M., Bozkurt, B., Butler, J., Casey, D. J., Drazner, M. H., et al. (2013). 2013 ACCF/AHA guideline for the management of heart failure: A report of the American college of Cardiology foundation/American heart association task force on practice guidelines. J. Am. Coll. Cardiol. 62, e147–e239. doi:10.1016/j.jacc.2013.05.019
Yeh, C. H., Shen, Z. Q., Hsiung, S. Y., Wu, P. C., Teng, Y. C., Chou, Y. J., et al. (2019). Cisd2 is essential to delaying cardiac aging and to maintaining heart functions. PLoS Biol. 17, e3000508. doi:10.1371/journal.pbio.3000508
Yu, Z., Chen, R., Li, M., Yu, Y., Liang, Y., Han, F., et al. (2018). Mitochondrial calcium uniporter inhibition provides cardioprotection in pressure overload-induced heart failure through autophagy enhancement. Int. J. Cardiol. 271, 161–168. doi:10.1016/j.ijcard.2018.05.054
Zaglia, T., Ceriotti, P., Campo, A., Borile, G., Armani, A., Carullo, P., et al. (2017). Content of mitochondrial calcium uniporter (mcu) in cardiomyocytes is regulated by microrna-1 in physiologic and pathologic hypertrophy. Proc. Natl. Acad. Sci. U. S. A. 114, E9006–E9015. doi:10.1073/pnas.1708772114
Zannad, F., Ferreira, J. P., Pocock, S. J., Anker, S. D., Butler, J., Filippatos, G., et al. (2020). Sglt2 inhibitors in patients with heart failure with reduced ejection fraction: A meta-analysis of the emperor-reduced and dapa-hf trials. Lancet 396, 819–829. doi:10.1016/S0140-6736(20)31824-9
Zeng, C., Duan, F., Hu, J., Luo, B., Huang, B., Lou, X., et al. (2020). Nlrp3 inflammasome-mediated pyroptosis contributes to the pathogenesis of non-ischemic dilated cardiomyopathy. Redox Biol. 34, 101523. doi:10.1016/j.redox.2020.101523
Zhang, Q., Raoof, M., Chen, Y., Sumi, Y., Sursal, T., Junger, W., et al. (2010). Circulating mitochondrial damps cause inflammatory responses to injury. Nature 464, 104–107. doi:10.1038/nature08780
Zhang, T., Yin, X., Yang, X., Man, J., He, Q., Wu, Q., et al. (2020). Research trends on the relationship between microbiota and gastric cancer: A bibliometric analysis from 2000 to 2019. J. Cancer 11, 4823–4831. doi:10.7150/jca.44126
Zhang, X. L., Zheng, Y., Xia, M. L., Wu, Y. N., Liu, X. J., Xie, S. K., et al. (2020). Knowledge domain and emerging trends in vinegar research: A bibliometric review of the literature from woscc. Foods 9, E166. doi:10.3390/foods9020166
Zhang, Y., Taufalele, P. V., Cochran, J. D., Robillard-Frayne, I., Marx, J. M., Soto, J., et al. (2020). Mitochondrial pyruvate carriers are required for myocardial stress adaptation. Nat. Metab. 2, 1248–1264. doi:10.1038/s42255-020-00288-1
Zhou, B., and Tian, R. (2018). Mitochondrial dysfunction in pathophysiology of heart failure. J. Clin. Invest. 128, 3716–3726. doi:10.1172/JCI120849
Ziaeian, B., and Fonarow, G. C. (2016). Epidemiology and aetiology of heart failure. Nat. Rev. Cardiol. 13, 368–378. doi:10.1038/nrcardio.2016.25
Keywords: mitochondrial dysfunction, heart failure, bibliometric analysis, visualization, research trend
Citation: Qi X, Zhu Z, Wang Y, Wen Z, Jiang Z, Zhang L, Pang Y and Lu J (2022) Research progress on the relationship between mitochondrial function and heart failure: A bibliometric study from 2002 to 2021. Front. Mol. Biosci. 9:1036364. doi: 10.3389/fmolb.2022.1036364
Received: 04 September 2022; Accepted: 07 October 2022;
Published: 18 October 2022.
Edited by:
Elena G. Govorunova, University of Texas Health Science Center at Houston, United StatesReviewed by:
Eberval Figueiredo, University of São Paulo, BrazilSangram Keshari Samal, Regional Medical Research Center (ICMR), India
Copyright © 2022 Qi, Zhu, Wang, Wen, Jiang, Zhang, Pang and Lu. This is an open-access article distributed under the terms of the Creative Commons Attribution License (CC BY). The use, distribution or reproduction in other forums is permitted, provided the original author(s) and the copyright owner(s) are credited and that the original publication in this journal is cited, in accordance with accepted academic practice. No use, distribution or reproduction is permitted which does not comply with these terms.
*Correspondence: Yan Pang, MjcxNTI4NDk1QHFxLmNvbQ==; Jianqi Lu, bHVqaWFucWk2NjZAMTYzLmNvbQ==
†These authors have contributed equally to this work and share first authorship