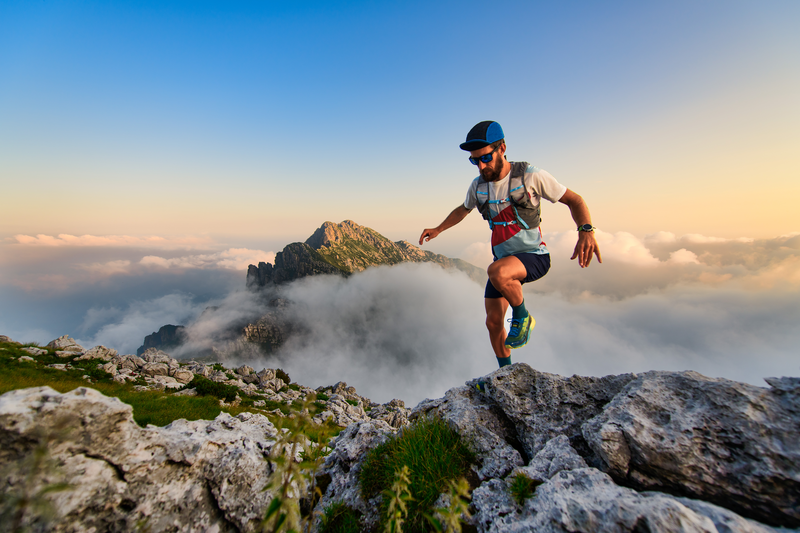
94% of researchers rate our articles as excellent or good
Learn more about the work of our research integrity team to safeguard the quality of each article we publish.
Find out more
ORIGINAL RESEARCH article
Front. Mol. Biosci. , 26 August 2021
Sec. Molecular Diagnostics and Therapeutics
Volume 8 - 2021 | https://doi.org/10.3389/fmolb.2021.741603
This article is part of the Research Topic Molecular Biomarkers for the Diagnosis, Prognosis, and Risk Prediction of Cancer View all 88 articles
RNA methylation plays a significant regulatory role in various of physiological activities and it has gradually become a hotspot of epigenetics in the past decade. 2′-O-methyladenosine (Am), 2′-O-methylguanosine (Gm), 2′-O-methylcytidine (Cm), 2′-O-methyluridine (Um), N6-methyladenosine (m6A), N1-methylguanosine (m1G), 5-methylcytidine (m5C), and 5-methyluridine (m5U) are representative 2′-O-methylation and base-methylation modified epigenetic marks of RNA. Abnormal levels of these ribonucleosides were found to be related to various diseases including cancer. Serum is an important source of biofluid for the discovery of biomarkers, and novel tumor biomarkers can be explored by measuring these ribonucleoside modifications in human serum. Herein, we developed and applied a hydrophilic interaction liquid chromatography tandem mass spectrometry (HILIC-MS/MS) method to determine the content of monomethylated ribonucleosides in human serum. The developed method enabled sensitive and accurate determination of these monomethylated ribonucleosides. By applying this robust method, we demonstrated the presence of Gm and Um in human serum for the first time, and we successfully quantified m6A, Gm, m1G, Cm, Um and m5U in serum samples collected from 61 patients with breast cancer and 69 healthy controls. We discovered that the levels of Gm, m1G, Cm, Um and m5U in serum were all significantly decreased in breast cancer patients whereas m6A was increased. We performed receiver operating characteristic (ROC) curve analysis, and obtained highest area under curve (AUC) value when combining these six monomethylated ribonucleosides together. These results suggest that m6A, Gm, m1G, Cm, Um and m5U might have great potential to be novel biomarkers for detection of breast cancer in the early stage. In addition, this study may stimulate future investigations about the regulatory roles of monomethylated ribonucleosides on the initiation and development of breast cancer.
Post-transcriptional modifications of RNA has great research prospects and RNA epigenetics/epitranscriptomics has been proposed (He, 2010). More than 170 different modifications of RNA have been identified in recent year and over half of them are RNA methylation modifications which are associated with the regulation of RNA functions (Jonkhout et al., 2017; Ontiveros et al., 2019). Due to the functional identification of important regulatory proteins such as methyltransferase METTL3/METTL14/WTAP complex (Liu et al., 2014) and demethylase FTO (Jia et al., 2011), RNA methylation modifications have attracted great attention and accumulating evidences have been obtained to confirm RNA methylation modifications as a novel layer of epigenetic alteration. Through their unique regulatory proteins, RNA methylation modifications play critical roles in various cellular functions, such as RNA splicing (Sun et al., 2020), stability (Zhang et al., 2019a; Chen et al., 2019; Shen et al., 2020), degradation (Ni et al., 2019; Yang et al., 2019; Chen et al., 2020b) and translation (Weng et al., 2018; Schumann et al., 2020; Song et al., 2020). Moreover, it has been revealed that RNA methylation is closely associated with the occurrence and development of human cancers.
As we known, the ribonucleoside compositions of RNA contain adenosine, guanosine, cytidine and uridine. The methylation usually occurs at nitrogen or carbon atom in the nucleobase part of the molecule to form m6A, m1G, m5C, m5U and so on. When the hydrogen on the 2′-hydroxyl (-OH) of the ribose moiety is replaced by a methyl group (-CH3), it will form 2′-O-methylation ribonucleosides (Nm) including Am, Gm, Cm and Um. M6A is the most predominant modification in mRNA, and the aberrant level of m6A modification has great connection with the tumorigenesis and development (Ma et al., 2019; Xu et al., 2020; Chen et al., 2021; Fang et al., 2021). In the recent study of our group, it was found that the significantly elevated m6A in human serum increased the risk of colorectal cancer and gastric cancer (Hu et al., 2021). It was reported that the dynamic level of m1G in human serum could help early detection of breast cancer (Rashed et al., 2020) and colorectal cancer (Zhu et al., 2015). M5C is another most abundant RNA modification which has been discovered to be a potential biomarker of various cancers (Guo et al., 2018a; Zhang et al., 2019b; Feng et al., 2020). In addition, a significant downregulation m5U in human serum can indicate the presence of prostate cancer (Buzatto et al., 2017). For Nm, they have also been revealed to participate in the pathogenesis of various cancers and play crucial roles (Hua et al., 2018; Zhu et al., 2019; Wu et al., 2020). Recently, Li et al. reported that the level of Cm in serum is related to the reduced risk of developing esophageal squamous cell carcinoma (Li et al., 2021). Therefore, these monomethylated ribonucleosides have significant potential to be used as indicators for early detection of cancers.
Breast cancer is the most prevalent tumor with the highest mortality among women worldwide (Sung et al., 2021). Generally, the prognosis and the survival rate of breast cancer are better in the early stages, but poorer in advanced stages even after receiving the surgery and adjuvant treatment (DeSantis et al., 2016). Therefore, it is important for early detection of breast cancer. Currently, ultrasonography and mammography, together with biopsy, are used for routine screening and staging. Limitations of these inspection methods including exposure to radiation, traumatic operation and often producing inaccurate results. Besides, breast tumors must be at least a few millimeters in size to be detected. Serum biomarkers, such as carbohydrate antigen-153 (CA153) and carcinoembryonic antigen (CEA), showed low sensitivity and/or low specificity and responded late to tumor formation and recurrence (Nagrath et al., 2011). From these points of view, it is necessary to hunt for robust biomarkers for early-stage of breast cancer to elongate the survival time and reduce the suffering of patients.
Serum is easy to obtain in the clinic and contains a large number of biomolecules, so it can be used as the body fluid of choice for the discovery of biomarkers. In the past decades, a variety of analytical methods have been utilized for analyzing modified ribonucleosides (Jiang and Ma, 2009; Wei et al., 2010; Beale et al., 2018; Pero-Gascon et al., 2018). Reversed-phase liquid chromatography (RPLC) or hydrophilic interaction liquid chromatography (HILIC) coupled with tandem mass spectrometry is more favored for biomarker discovery due to its great advantages in selectivity, sensitivity, accuracy and high throughput (Zhu et al., 2015; Guo et al., 2018b; Chen et al., 2020a; Guo et al., 2020; Su et al., 2021), compared with other analytical techniques. In our study, a fast, sensitive, simple and reliable HILIC-MS/MS method for qualitative and quantitative detection of monomethylated ribonucleosides in human serum was established. We revealed the presence of Gm and Um in human serum for the first time and quantified m6A, Gm, m1G, Cm, Um and m5U in serum from breast cancer patients and healthy controls. By analyzing these results, we demonstrated the differences of these modifications between breast cancer patients and healthy volunteers, and evaluate the potential of these monomethylated ribonucleosides as biomarkers for early detection of breast cancer.
Chromatographic grade acetonitrile was bought from Merck KGaA (Darmstadt, Germany). Methanol of HPLC grade was purchased from J.T.Baker (Radnor, PA, United States). Formic acid (HCOOH) was bought from Fluka (Muskegon, United States). Ammonium formate, malic acid and 5-methylcytidine (m5C) were bought from Sigma-Aldrich (St Louis, MO, United States). 2′-O-methyladenosine (Am), N6-methyladenosine (m6A), 2′-O-methylguanosine (Gm), 1-methylguanosine (m1G), 2′-O-methylcytidine (Cm), 2′-O-methyluridine (Um), 5-methyluridine (m5U) and isotopically labeled standards [D3]m6A, [D3]Um and [13C5]m5U were obtained from Toronto Research Chemicals (Toronto, Canada). [13C15N2]Gm, [13C15N2]m1G and [13C5]Cm were synthesized according to literature (Fu et al., 2015). Water was purified by a Milli-Q water purification device (Millipore, Milford, MA, United States).
Acquity UPLC system (Waters, Milford, MA, United States) achieved by Empower Pro 6.0 software was applied for analysis. A Waters Acquity BEH Amide column (100 mm × 2.1 mm, 1.7 μm) was applied for chromatographic separation. 4000 QTRAP mass spectrometer (AB SCIEX, Foster City, CA, United States) was applied for MS detection. The mass spectrometer was equipped with electrospray ionization (ESI) positive ion mode. Multiple-reaction monitoring (MRM) was chosen to acquire data. Data acquisition and processing were controlled by Analyst 1.6.3 software.
The Ethics Committee of Medical Research of the Second Affiliated Hospital, Zhejiang University School of Medicine (SAHZU) approved our study. A total of 69 healthy volunteers (mean age of 43.9 ± 11.1 years, range from 30 to 70 years) and 61 patients with breast cancer (mean age of 52.2 ± 11.9 years, range from 29 to 80 years) were recruited from SAHZU. All breast cancer patients had a diagnosis report with a pathological stage of stage I or stage II at SAHZU between June 2020 and December 2020. The exclusion criteria were as follows: 1) Co-suffering other malignant tumors. 2) Having received any type of treatment for tumors. 3) Suffering from metabolic diseases, kidney diseases or liver diseases. 4) Taking any drugs for a long time. All participating volunteers agreed the informed consent in advance. Then, the serum samples were collected in the early morning and reserved at −80°C.
At first, 10 μl of isotope-labeled internal standards (IS) mixed solution was added into 100 μl serum samples which were naturally thawed in ice. And then 330 μl pre-refrigerated acetonitrile/methanol of 2:1 (v/v) was added. After vortexed for 60 s, let it stand at −20°C for 2 h and centrifuged at 13,000 rpm, 4°C for 15 min orderly, 352 μl of the supernatant were taken out and then drain under vacuum. Then, 80 μl acetonitrile/water of 9:1 (v/v) was used to redissolve the dried samples. After vortexed for 10 s, ultrasonicated for 15 s and centrifuged at 13,000 rpm for 15 min at 4°C, 70 μl of the supernatant fraction were sucked into the sample bottle for subsequent HILIC-MS/MS detection.
The mobile phase was (A) H2O containing 10 mM ammonium formate, 0.2% formic acid and 0.06 mM malic acid, and (B) acetonitrile containing 2 mM ammonium formate, 0.2% formic acid and 0.06 mM malic acid. The eight analytes were perfectly separated at a flow rate of 0.4 ml/min by the optimized LC gradient program as follows: 0 min, 94% B; 4 min, 94% B; 6.1 min, 75% B; 6.5 min, 94% B; 8 min, 94% B. The BEH Amide column was set at 40°C and the samples temperature was maintained at 4°C. 5 μl of sample was injected each time and each sample was measured twice. To minimize the interference of the mass spectrometer, a switching valve was used and the eluents from the column were introduced into the ion source during 1.0–6.5 min.
The ion spray voltage was kept at 5.5 kV and the ion source temperature (TEM) was maintained at 550°C. Ion source gas 1 (GS1), ion source gas 2 (GS2) and curtain gas (CUR) were all set at 45 psi. The ion transitions of these eight ribonucleosides and corresponding isotope labeled internal standard (IS) were shown in Supplementary Table S1. The optimized MRM parameters of them including declustering potential (DP), entrance potential (EP), collision energy (CE) and collision cell exit potential (CXP) were also listed in Supplementary Table S1.
The standard working solutions of m6A, Gm, m1G, Cm, Um and m5U at different concentrations (1, 2.5, 5, 10, 25, 50, 100, 250, 500 nM), which were mixed with IS solution (final concentration: [D3]m6A (5 nM), [13C15N2]Gm (10 nM), [13C15N2]m1G (20 nM), [13C5]Cm (30 nM), [D3]Um (20 nM) and [13C5]m5U (100 nM)), were made and analyzed. The calibration curves could describe as y = ax + b, where y represents the peak area ratio of the analyte to the corresponding IS and x denotes the concentration of the analyte. The limit of detection (LOD) and limit of quantification (LOQ) of each ribonucleoside were obtained by analyzing standard solutions with a signal-to-noise ratio of three and ten, respectively.
For the purpose of evaluating intra-day and inter-day precision, the quality control (QC) samples at three different levels of m6A (2.5, 5, 50 nM), Gm (5, 10, 50 nM), m1G (5, 20, 100 nM), Cm (5, 30, 150 nM), Um (5, 20, 100 nM) and m5U (20, 100, 300 nM) were prepared in triplicate and were measured on the same day and three consecutive days, respectively. The accuracy was described as the ratio of measured value to the theoretical concentration.
For the purpose of evaluating the recovery of extraction, the serum samples were added with three different levels of m6A (2.5, 5, 30 nM), Gm (2.5, 10, 50 nM), m1G (5, 20, 60 nM), Cm (6, 30, 150 nM), Um (5, 20, 60 nM) and m5U (50, 150, 300 nM). After 10 μl of IS solution (same as described above) was added, the serum samples were treated and analyzed as mentioned above. The recovery (R) of each analyte was calculated by (concentration in added serum sample–concentration in original serum sample)/added concentration × 100%.
The matrix effect was estimated by using a slope comparison method. By adding different concentrations (1, 2.5, 5, 10, 25, 50, 100, 250, 500 nM) standard solution and IS to the serum extracts, the calibration curve was obtained. The ratio value of its slope to the slope of the standard solution calibration curve was matrix effect.
Statistical analyses were achieved through SPSS 24.0 software (IBM, Armonk, NY, United States). The concentration differences of serum monomethylated modifications between healthy volunteers and breast cancer patients were accessed by Two-tailed Student’s t-test, where p value less than 0.05 was considered meaningful. The area under the curve (AUC) was acquired by receiver operating characteristic (ROC) curve analysis, and the optimal cut-off value of the methylation modification in serum for the diagnosis of breast cancer was determined by the Youden index (Youden index = sensitivity + specificity −1). Receiver operating characteristic (ROC) analysis was performed to evaluate the ability of monomethylated modifications to distinguish cancer patients from healthy controls.
The optimization of chromatographic conditions is mainly achieved by optimizing the type of chromatographic column and the composition of the mobile phase. In order to acquire symmetrical peak shape and great separation effect in a short time, it is very important to choose a column with high separation efficiency. The chemical structures of these monomethylated ribonucleosides were illustrated in Figure 1. In our previous study, we found malic acid could enhance the detection of methylated nucleosides in HILIC-MS/MS (Guo et al., 2018a). Therefore, a hydrophilic interaction column of BEH Amide (100 mm × 2.1 mm, 1.7 μm, Waters) was selected for analysis, and malic acid was added into the mobile phase. As showed in Figure 2, it could acquire satisfactory separation for these eight ribonucleoside modifications. Besides, the analysis could be accomplished less than 6.5 min. It meant that this analytical method was quick, high throughput and fit for large clinical practice.
FIGURE 2. The MRM chromatograms of Am, m6A, Gm, m1G, Cm, m5C, Um, m5U standards. The concentrations of Um and m5U were 1,000 nM, and the concentrations of other nucleosides standards were 100 nM. The injection volume was 5.0 μl.
For the purpose of optimizing the MRM parameters, the mass spectrometer analyzed the standard solution injected by the peristaltic pump. Abundant [M + H]+ ions were observed in full scan ESI-MS. Then, the collision induced dissociation (CID) experiment was used to calculate the ion transitions of all analytes. In MS/MS, the ribose group can be easily eliminated due to the cleavage of the C-N bond. Taking Am and [13C5]Am as examples, abundant [M + H]+ ions at m/z 282.1 and 287.1 were observed for Am and [13C5]Am, respectively. The [M + H]+ ion of Am lost 146 and 151 Da was lost from [M + H]+ ion of [13C5]Am. Therefore, ion transition m/z 282.1→136.0 and m/z 287.1→136.0 was used for the quantification of Am and [13C5]Am, respectively. The ion transitions of other ribonucleosides and corresponding IS were shown in Supplementary Table S1. In addition, the optimized parameter values of DP, EP, CE and CXP were also listed. Under these optimized conditions, The LODs and LOQs of these monomethylated ribonucleosides can reach sub femtomole level (Supplementary Table S2).
According to the aforementioned method, the prepared calibration curve showed excellent linearities (R2 < 0.999) in appropriate analytical ranges, and equations were showed in Table 1. The slope ratio values for these eight monomethylated ribonucleosides ranged from 94.7 to 104.7% (Table 1), which indicated the interference of matrix in this study was minimal.
TABLE 1. Linear equations and matrix effect values of m6A, Gm, m1G, Cm, Um and m5U in HILIC-MS/MS analysis.
As showed in Supplementary Table S3, the intra- and inter-day accuracy assays were in the range of 92.20–112.96% and 92.29–112.76%, respectively, and the precision of intra- and inter-day were both within 8.6%. These data indicated that sufficient reproducibility and accuracy were obtained. As showed in Supplementary Table S4, the recoveries ranged from 98.04 to 114.01% (RSD <10%), indicating an excellent recovery rate.
In a word, all these results mentioned above revealed that the established HILIC-MS/MS method could meet quantitative requirement of m6A, Gm, m1G, Cm, Um and m5U in human serum samples, and it was quick, accurate, sensitive, reproducible and reliable.
By using this HILIC-MS/MS method, we detected these modified ribonucleosides in serum samples from 61 patients with breast cancer and 69 healthy volunteers. The results showed that m6A, Gm, m1G, Cm, Um and m5U were detected in all the serum samples, whereas Am and m5C could not be monitored due to their extremely low levels. As demonstrated in Figure 3, the retention time of Um, m6A, m5U, Cm, m1G and Gm were 1.44, 2.10, 2.42, 3.38, 4.34 and 4.91 min, respectively. Of note, the retention time of these compounds were consistent with their corresponding isotope-labeled internal standard. The same tandem mass spectrometry behaviors and chromatographic retention time of each modified ribonucleoside in serum as those of the isotope-labeled IS confirmed the existence of m6A, Gm, m1G, Cm, Um and m5U in human serum indubitably. It is worth nothing that the presence of Gm and Um in human serum was revealed for the first time, as far as we known.
FIGURE 3. Representative MRM chromatograms of (A) Um, (B) m6A, (C) m5U, (D) Cm, (E) m1G, (F) Gm and spiked isotope-labeled internal standards in a serum sample.
The detailed concentrations of m6A, Gm, m1G, Cm, Um and m5U in all the serum samples were presented in Supplementary Table S5. The measured concentrations (nM) in serum samples of m6A, Gm, m1G, Cm, Um and m5U from healthy volunteers were in the range of 0.93–6.01, 7.91–20.85, 20.29–39.74, 29.97–66.58, 14.47–36.83 and 101.97–260.34 nM respectively, and the average concentrations were 2.97, 13.47, 27.97, 22.60 and 186.43 nM, respectively (n = 69). In the serum of breast cancer patients, the concentration of m6A, Gm, m1G, Cm, Um and m5U were in the range of 1.45–7.01, 7.84–21.39, 16.59–34.41, 24.61–57.14, 13.83–39.07 and 108.20–226.84 nM respectively, and the average concentrations were 4.48, 11.55, 25.15, 37.31, 20.27 and 159.40 nM, respectively (n = 61). As illustrated in Figure 4, it was obvious that the levels of Gm, m1G, Cm, Um and m5U in serum were intensely decreased in patients with breast cancer compared to healthy controls (p < 0.0001 for Gm, p < 0.0001 for m1G, p < 0.0001 for Cm, p < 0.01 for Um, p < 0.0001 for m5U), but the concentration of m6A in breast cancer patients was much higher than that in healthy volunteers (p < 0.0001).
FIGURE 4. The measured concentrations of (A) m6A, (B) Gm, (C) m1G, (D) Cm, (E) Um, (F) m5U in serum samples and statistical analysis.
To verify the significance of m6A, Gm, m1G, Cm, Um and m5U as potential breast cancer biomarkers, receiver operating characteristic (ROC) curve was plotted. Based on the Youden index, the optimal cut-off values of m6A, Gm, m1G, Cm, Um and m5U were 3.76, 12.99, 25.92, 37.31,21.62 and 169.58 nM, which indicates that if the detection values break through these thresholds, it increases the risk of breast cancer. Trapezoidal rule was used to calculate area under the curve (AUC). As demonstrated in Figure 5, the AUC values were 0.78, 0.70, 0.70, 0.78, 0.65, 0.78 and 0.68 for m6A, Gm, m1G, Cm, Um, m5U and CA153, respectively. Most of the AUC values of ribonucleosides were higher than that of CA153, implying there might be better correlation between the levels of m6A, Gm, m1G, Cm and m5U in serum and the incidence of breast cancer, compared with CA153. Moreover, when all these serum monomethylated ribonucleosides were combined, the AUC could reach 0.93, which suggested the sensitivity and specificity for breast cancer diagnosis were dramatically increased. These results indicated that lower levels of Gm, m1G, Cm, Um and m5U and higher levels of m6A could be regarded as potential diagnostic indicators for the screening of breast cancer.
FIGURE 5. ROC analysis for m6A, Gm, m1G, Cm, Um, m5U, SMRS and CA153 in serum samples of breast cancer patients. *SMRS: serum monomethylated ribonucleosides score.
In our study, a fast, robust, sensitive and trustable HILIC-MS/MS method was established for analysis of monomethylated ribonucleosides. A total of 130 serum samples from two groups containing breast cancer patients and healthy control were analyzed. Six modified ribonucleosides including m6A, Gm, m1G, Cm, Um and m5U were identified and quantified. Of note, to the best of our knowledge, this is the first time that Gm and Um were detected in human serum. Besides, we elucidated the differences in the contents of these monomethylated ribonucleosides between these two groups and our data, to some extent, indicated that these six modified ribonucleosides might play as potential indicators in the early detection of breast cancer.
The original contributions presented in the study are included in the article/Supplementary Material, further inquiries can be directed to the corresponding author.
The studies involving human participants were reviewed and approved by the Institutional Review Board of Medical Research, The Second Affiliated Hospital, Zhejiang University School of Medicine (SAHZU). The patients/participants provided their written informed consent to participate in this study.
CG designed the study; ZF and YH performed the experiments; ZF, JC and KW collected the serum samples; CG and ZF analyzed and interpreted the data; CG and ZF wrote the manuscript; KX and SZ edited the manuscript. All authors commented and approved the final manuscript.
This study was supported by the Natural Science Foundation of Zhejiang Province (LY19B050007), Key R&D Program of Zhejiang Province (2021C03125), National Key R&D Program of China (2016YFC1302803), and National Natural Science Foundation of China (21402172).
The authors declare that the research was conducted in the absence of any commercial or financial relationships that could be construed as a potential conflict of interest.
All claims expressed in this article are solely those of the authors and do not necessarily represent those of their affiliated organizations, or those of the publisher, the editors and the reviewers. Any product that may be evaluated in this article, or claim that may be made by its manufacturer, is not guaranteed or endorsed by the publisher.
The Supplementary Material for this article can be found online at: https://www.frontiersin.org/articles/10.3389/fmolb.2021.741603/full#supplementary-material
Beale, D. J., Pinu, F. R., Kouremenos, K. A., Poojary, M. M., Narayana, V. K., Boughton, B. A., et al. (2018). Review of Recent Developments in GC-MS Approaches to Metabolomics-Based Research. Metabolomics 14 (11), 152. doi:10.1007/s11306-018-1449-2
Buzatto, A. Z., de Oliveira Silva, M., Poppi, R. J., and Simionato, A. V. C. (2017). Assessment of Nucleosides as Putative Tumor Biomarkers in Prostate Cancer Screening by CE-UV. Anal. Bioanal. Chem. 409 (13), 3289–3297. doi:10.1007/s00216-017-0297-7
Chen, H., Gao, S., Liu, W., Wong, C.-C., Wu, J., Wu, J., et al. (2021). RNA N6-Methyladenosine Methyltransferase METTL3 Facilitates Colorectal Cancer by Activating the m6A-GLUT1-mTORC1 Axis and Is a Therapeutic Target. Gastroenterology 160 (4), 1284–1300. e1216. doi:10.1053/j.gastro.2020.11.013
Chen, Q., Hu, Y., Fang, Z., Ye, M., Li, J., Zhang, S., et al. (2020a). Elevated Levels of Oxidative Nucleic Acid Modification Markers in Urine from Gastric Cancer Patients: Quantitative Analysis by Ultra Performance Liquid Chromatography-Tandem Mass Spectrometry. Front. Chem. 8, 606495. doi:10.3389/fchem.2020.606495
Chen, X., Li, A., Sun, B.-F., Yang, Y., Han, Y.-N., Yuan, X., et al. (2019). 5-methylcytosine Promotes Pathogenesis of Bladder Cancer through Stabilizing mRNAs. Nat. Cel Biol. 21 (8), 978–990. doi:10.1038/s41556-019-0361-y
Chen, X., Xu, M., Xu, X., Zeng, K., Liu, X., Pan, B., et al. (2020b). METTL14-mediated N6-Methyladenosine Modification of SOX4 mRNA Inhibits Tumor Metastasis in Colorectal Cancer. Mol. Cancer 19 (1), 106. doi:10.1186/s12943-020-01220-7
DeSantis, C. E., Fedewa, S. A., Goding Sauer, A., Kramer, J. L., Smith, R. A., and Jemal, A. (2016). Breast Cancer Statistics, 2015: Convergence of Incidence Rates between Black and white Women. CA: A Cancer J. Clinicians 66 (1), 31–42. doi:10.3322/caac.21320
Fang, Z., Hu, Y., Hu, J., Huang, Y., Zheng, S., and Guo, C. (2021). The Crucial Roles of N6-Methyladenosine (m6A) Modification in the Carcinogenesis and Progression of Colorectal Cancer. Cell Biosci. 11 (1), 72. doi:10.1186/s13578-021-00583-8
Feng, Y., Ma, C.-J., Ding, J.-H., Qi, C.-B., Xu, X.-J., Yuan, B.-F., et al. (2020). Chemical Labeling - Assisted Mass Spectrometry Analysis for Sensitive Detection of Cytidine Dual Modifications in RNA of Mammals. Analytica Chim. Acta 1098, 56–65. doi:10.1016/j.aca.2019.11.016
Fu, L., Amato, N. J., Wang, P., McGowan, S. J., Niedernhofer, L. J., and Wang, Y. (2015). Simultaneous Quantification of Methylated Cytidine and Adenosine in Cellular and Tissue RNA by Nano-Flow Liquid Chromatography-Tandem Mass Spectrometry Coupled with the Stable Isotope-Dilution Method. Anal. Chem. 87 (15), 7653–7659. doi:10.1021/acs.analchem.5b00951
Guo, C., Chen, Q., Chen, J., Yu, J., Hu, Y., Zhang, S., et al. (2020). 8-Hydroxyguanosine as a Possible RNA Oxidative Modification Marker in Urine from Colorectal Cancer Patients: Evaluation by Ultra Performance Liquid Chromatography-Tandem Mass Spectrometry. J. Chromatogr. B 1136, 121931. doi:10.1016/j.jchromb.2019.121931
Guo, C., Xie, C., Chen, Q., Cao, X., Guo, M., Zheng, S., et al. (2018a). A Novel Malic Acid-Enhanced Method for the Analysis of 5-Methyl-2′-Deoxycytidine, 5-Hydroxymethyl-2′-Deoxycytidine, 5-methylcytidine and 5-hydroxymethylcytidine in Human Urine Using Hydrophilic Interaction Liquid Chromatography-Tandem Mass Spectrometry. Analytica Chim. Acta 1034, 110–118. doi:10.1016/j.aca.2018.06.081
Guo, C., Xie, C., Ding, P., Qin, G., Mo, W., Cao, X., et al. (2018b). Quantification of Glycocholic Acid in Human Serum by Stable Isotope Dilution Ultra Performance Liquid Chromatography Electrospray Ionization Tandem Mass Spectrometry. J. Chromatogr. B 1072, 315–319. doi:10.1016/j.jchromb.2017.11.037
He, C. (2010). Grand challenge Commentary: RNA Epigenetics? Nat. Chem. Biol. 6 (12), 863–865. doi:10.1038/nchembio.482
Hu, Y., Fang, Z., Mu, J., Huang, Y., Zheng, S., Yuan, Y., et al. (2021). Quantitative Analysis of Methylated Adenosine Modifications Revealed Increased Levels of N6-Methyladenosine (m6A) and N6,2′-O-Dimethyladenosine (m6Am) in Serum from Colorectal Cancer and Gastric Cancer Patients. Front. Cel Dev. Biol. 9, 694673. doi:10.3389/fcell.2021.694673
Hua, W., Zhao, Y., Jin, X., Yu, D., He, J., Xie, D., et al. (2018). METTL3 Promotes Ovarian Carcinoma Growth and Invasion through the Regulation of AXL Translation and Epithelial to Mesenchymal Transition. Gynecol. Oncol. 151 (2), 356–365. doi:10.1016/j.ygyno.2018.09.015
Jia, G., Fu, Y., Zhao, X., Dai, Q., Zheng, G., Yang, Y., et al. (2011). N6-methyladenosine in Nuclear RNA Is a Major Substrate of the Obesity-Associated FTO. Nat. Chem. Biol. 7 (12), 885–887. doi:10.1038/nchembio.687
Jiang, Y., and Ma, Y. (2009). A Fast Capillary Electrophoresis Method for Separation and Quantification of Modified Nucleosides in Urinary Samples. Anal. Chem. 81 (15), 6474–6480. doi:10.1021/ac901216n
Jonkhout, N., Tran, J., Smith, M. A., Schonrock, N., Mattick, J. S., and Novoa, E. M. (2017). The RNA Modification Landscape in Human Disease. Rna 23 (12), 1754–1769. doi:10.1261/rna.063503.117
Li, X., Zhao, L., Wei, M., Lv, J., Sun, Y., Shen, X., et al. (2021). Serum Metabolomics Analysis for the Progression of Esophageal Squamous Cell Carcinoma. J. Cancer 12 (11), 3190–3197. doi:10.7150/jca.54429
Liu, J., Yue, Y., Han, D., Wang, X., Fu, Y., Zhang, L., et al. (2014). A METTL3-METTL14 Complex Mediates Mammalian Nuclear RNA N6-Adenosine Methylation. Nat. Chem. Biol. 10 (2), 93–95. doi:10.1038/nchembio.1432
Ma, F., Liu, X., Zhou, S., Li, W., Liu, C., Chadwick, M., et al. (2019). Long Non-coding RNA FGF13-AS1 Inhibits Glycolysis and Stemness Properties of Breast Cancer Cells through FGF13-AS1/IGF2BPs/Myc Feedback Loop. Cancer Lett. 450, 63–75. doi:10.1016/j.canlet.2019.02.008
Nagrath, D., Caneba, C., Karedath, T., and Bellance, N. (2011). Metabolomics for Mitochondrial and Cancer Studies. Biochim. Biophys. Acta (Bba) - Bioenerg. 1807 (6), 650–663. doi:10.1016/j.bbabio.2011.03.006
Ni, W., Yao, S., Zhou, Y., Liu, Y., Huang, P., Zhou, A., et al. (2019). Long Noncoding RNA GAS5 Inhibits Progression of Colorectal Cancer by Interacting with and Triggering YAP Phosphorylation and Degradation and Is Negatively Regulated by the m6A Reader YTHDF3. Mol. Cancer 18 (1), 143. doi:10.1186/s12943-019-1079-y
Ontiveros, R. J., Stoute, J., and Liu, K. F. (2019). The Chemical Diversity of RNA Modifications. Biochem. J. 476 (8), 1227–1245. doi:10.1042/bcj20180445
Pero-Gascon, R., Sanz-Nebot, V., Berezovski, M. V., and Benavente, F. (2018). Analysis of Circulating microRNAs and Their Post-Transcriptional Modifications in Cancer Serum by On-Line Solid-phase Extraction-Capillary Electrophoresis-Mass Spectrometry. Anal. Chem. 90 (11), 6618–6625. doi:10.1021/acs.analchem.8b00405
Rashed, R., Darwish, H., Omran, M., Belal, A., and Zahran, F. (2020). A Novel Serum Metabolome Score for Breast Cancer Diagnosis. Br. J. Biomed. Sci. 77 (4), 196–201. doi:10.1080/09674845.2020.1784568
Schumann, U., Zhang, H.-N., Sibbritt, T., Pan, A., Horvath, A., Gross, S., et al. (2020). Multiple Links between 5-methylcytosine Content of mRNA and Translation. BMC Biol. 18 (1), 40. doi:10.1186/s12915-020-00769-5
Shen, C., Xuan, B., Yan, T., Ma, Y., Xu, P., Tian, X., et al. (2020). m6A-dependent Glycolysis Enhances Colorectal Cancer Progression. Mol. Cancer 19 (1), 72. doi:10.1186/s12943-020-01190-w
Song, P., Feng, L., Li, J., Dai, D., Zhu, L., Wang, C., et al. (2020). β-Catenin Represses miR455-3p to Stimulate m6A Modification of HSF1 mRNA and Promote its Translation in Colorectal Cancer. Mol. Cancer 19 (1), 129. doi:10.1186/s12943-020-01244-z
Su, X., Li, X., Wang, H., and Cai, Z. (2021). Simultaneous Determination of Methionine Cycle Metabolites, Urea Cycle Intermediates and Polyamines in Serum, Urine and Intestinal Tissue by Using UHPLC-MS/MS. Talanta 224, 121868. doi:10.1016/j.talanta.2020.121868
Sun, L., Wan, A., Zhou, Z., Chen, D., Liang, H., Liu, C., et al. (2020). RNA-binding Protein RALY Reprogrammes Mitochondrial Metabolism via Mediating miRNA Processing in Colorectal Cancer. Gut 70, 1698–1712. doi:10.1136/gutjnl-2020-320652
Sung, H., Ferlay, J., Siegel, R. L., Laversanne, M., Soerjomataram, I., Jemal, A., et al. (2021). Global Cancer Statistics 2020: GLOBOCAN Estimates of Incidence and Mortality Worldwide for 36 Cancers in 185 Countries. CA A. Cancer J. Clin. 71, 209–249. doi:10.3322/caac.21660
Wei, R., Li, G., and Seymour, A. B. (2010). High-throughput and Multiplexed LC/MS/MRM Method for Targeted Metabolomics. Anal. Chem. 82 (13), 5527–5533. doi:10.1021/ac100331b
Weng, H., Huang, H., Wu, H., Qin, X., Zhao, B. S., Dong, L., et al. (2018). METTL14 Inhibits Hematopoietic Stem/Progenitor Differentiation and Promotes Leukemogenesis via mRNA m6A Modification. Cell Stem Cell 22 (2), 191–205. e199. doi:10.1016/j.stem.2017.11.016
Wu, H., Qin, W., Lu, S., Wang, X., Zhang, J., Sun, T., et al. (2020). Long Noncoding RNA ZFAS1 Promoting Small Nucleolar RNA-Mediated 2′-O-Methylation via NOP58 Recruitment in Colorectal Cancer. Mol. Cancer 19 (1), 95. doi:10.1186/s12943-020-01201-w
Xu, Y., Ye, S., Zhang, N., Zheng, S., Liu, H., Zhou, K., et al. (2020). The FTO/miR‐181b‐3p/ARL5B Signaling Pathway Regulates Cell Migration and Invasion in Breast Cancer. Cancer Commun. 40 (10), 484–500. doi:10.1002/cac2.12075
Yang, S., Wei, J., Cui, Y.-H., Park, G., Shah, P., Deng, Y., et al. (2019). m6A mRNA Demethylase FTO Regulates Melanoma Tumorigenicity and Response to Anti-PD-1 Blockade. Nat. Commun. 10 (1), 2782. doi:10.1038/s41467-019-10669-0
Zhang, Y.-F., Qi, C.-B., Yuan, B.-F., and Feng, Y.-Q. (2019b). Determination of Cytidine Modifications in Human Urine by Liquid Chromatography - Mass Spectrometry Analysis. Analytica Chim. Acta 1081, 103–111. doi:10.1016/j.aca.2019.07.002
Zhang, Y., Kang, M., Zhang, B., Meng, F., Song, J., Kaneko, H., et al. (2019a). m6A Modification-Mediated CBX8 Induction Regulates Stemness and Chemosensitivity of colon Cancer via Upregulation of LGR5. Mol. Cancer 18 (1), 185. doi:10.1186/s12943-019-1116-x
Zhu, H., Gan, X., Jiang, X., Diao, S., Wu, H., and Hu, J. (2019). ALKBH5 Inhibited Autophagy of Epithelial Ovarian Cancer through miR-7 and BCL-2. J. Exp. Clin. Cancer Res. 38 (1), 163. doi:10.1186/s13046-019-1159-2
Keywords: HILIC-MS/MS, RNA modification, monomethylated ribonucleosides, serum, breast cancer
Citation: Fang Z, Hu Y, Chen J, Xu K, Wang K, Zheng S and Guo C (2021) Mass Spectrometry-Based Targeted Serum Monomethylated Ribonucleosides Profiling for Early Detection of Breast Cancer. Front. Mol. Biosci. 8:741603. doi: 10.3389/fmolb.2021.741603
Received: 15 July 2021; Accepted: 16 August 2021;
Published: 26 August 2021.
Edited by:
William C. Cho, QEH, Hong Kong, SAR ChinaReviewed by:
Mengzhe Guo, Xuzhou Medical University, ChinaCopyright © 2021 Fang, Hu, Chen, Xu, Wang, Zheng and Guo. This is an open-access article distributed under the terms of the Creative Commons Attribution License (CC BY). The use, distribution or reproduction in other forums is permitted, provided the original author(s) and the copyright owner(s) are credited and that the original publication in this journal is cited, in accordance with accepted academic practice. No use, distribution or reproduction is permitted which does not comply with these terms.
*Correspondence: Cheng Guo, Y2hlbmdfZ3VvQHpqdS5lZHUuY24=
Disclaimer: All claims expressed in this article are solely those of the authors and do not necessarily represent those of their affiliated organizations, or those of the publisher, the editors and the reviewers. Any product that may be evaluated in this article or claim that may be made by its manufacturer is not guaranteed or endorsed by the publisher.
Research integrity at Frontiers
Learn more about the work of our research integrity team to safeguard the quality of each article we publish.