- 1Department of Anesthesiology, The Second Affiliated Hospital of Nanchang University, Nanchang, China
- 2Key Laboratory of Anesthesiology of Jiangxi Province, Nanchang, China
- 3Department of Gastroenterology, The First Affiliated Hospital of Nanchang University, Nanchang, China
Aberrant Notch signaling profoundly affects cancer progression. Especially the Notch3 receptor was found to be dysregulated in cancer, where its expression is correlated with worse clinicopathological features and poor prognosis. The activation of Notch3 signaling is closely related to the activation of cancer stem cells (CSCs), a small subpopulation in cancer that is responsible for cancer progression. In addition, Notch3 signaling also contributes to tumor chemoresistance against several drugs, including doxorubicin, platinum, taxane, epidermal growth factor receptor (EGFR)–tyrosine kinase inhibitors (TKIs) and gemcitabine, through complex mechanisms. In this review, we mainly focus on discussing the molecular mechanisms by which Notch3 modulates cancer stemness and chemoresistance, as well as other cancer behaviors including metastasis and angiogenesis. What’s more, we propose potential treatment strategies to block Notch3 signaling, such as non-coding RNAs, antibodies and antibody-drug conjugates, providing a comprehensive reference for research on precise targeted cancer therapy.
Introduction
Notch signaling is a highly conserved among multicellular organisms, and it is involved in cell fate decision, cell proliferation/differentiation, as well as cell lineage specification (Bray, 2006; Bray, 2016). The activation of Notch signaling is mediated by cell-to-cell interactions with a Notch ligand. In mammals, there are four Notch receptors (Notch1-4) and five ligands [Jagged (JAG)1, 2 and Delta-like ligand (DLL)1, 3 and 4]. Before it is trafficked to the cell membrane, the full-length Notch receptor undergoes initial cleavage, also called S1 cleavage, in the Golgi apparatus. When a Notch ligand (JAG or DLL) in the cell membrane of an adjacent signal-sending cell interacts with a Notch receptor in the cell membrane of the signal-receiving cell, the Notch receptor is activated and undergoes another proteolytic cleavage. This so-called S2 and S3 cleavage steps are induced by A Disintegrin And Metalloprotease domain 10 (ADAM10) and the γ-secretase complex, respectively (Bray, 2006; Kopan and Ilagan, 2009; Bray, 2016). Subsequently, the Notch intracellular domain (NICD) is released and translocated into the nucleus, where it binds to the effector DNA-binding transcription factor CSL. The latter then recruits the transcription co-activator mastermind-like protein (MAML) to induce the transcription of downstream target genes. Finally, the Notch receptor or NICD undergoes proteasomal/lysosome degradation (Bray, 2006; Kopan and Ilagan, 2009; Bray, 2016; Xiu and Liu, 2019) (Figure 1, shown on the example of Notch3 signaling).
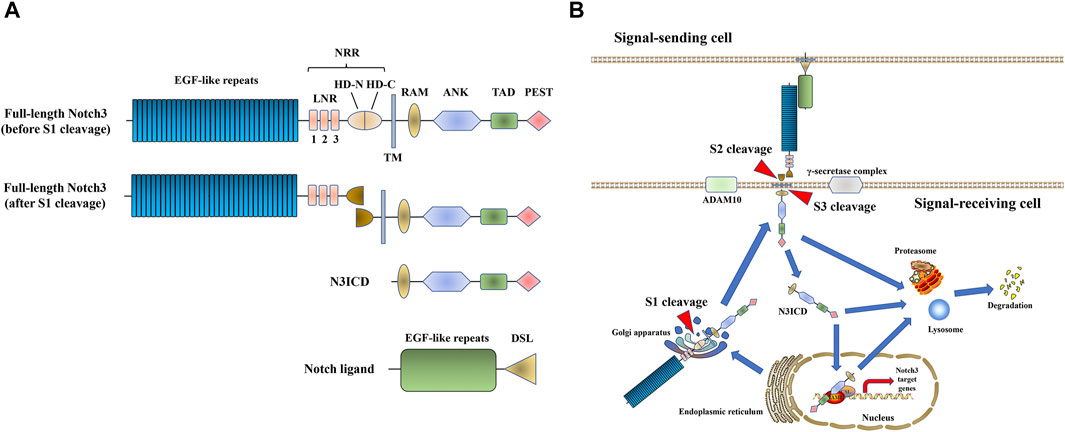
FIGURE 1. Notch3 signaling. (A) The structure of Notch3 and its ligand. The full-length Notch3 protein consists of 34 epidermal growth factor (EGF) repeats, a negative regulatory region (NRR) [composed of three Lin-Notch repeats (LNRs) and a heterodimerization domain (HD)], a transmembrane domain, an RBPJ-association module (RAM) domain, seven ankyrin (ANK) repeats, two nuclear localization signals (NLS), a transactivation domain (TAD) and a C-terminal domain rich in proline, glutamic acid, serine, and threonine (PEST domain) (Xiu and Liu, 2019). The Notch ligand consists of EGF repeats and a Delta/Serrate/LAG-2 (DSL) domain. (B) Notch3 signal transduction process.
The Notch3 receptor is encoded on chromosome 19p13.12 (19: 15159038-15200995), spanning 33 exons (https://www.ncbi.nlm.nih.gov/gene/4854). The aberrant high expression of Notch3 is common in human cancer tissues, as shown in several studies (Giovannini et al., 2009; Park et al., 2010; Zhang et al., 2011; Rahman et al., 2012; Hu et al., 2013; Ye et al., 2013; Ozawa et al., 2014; Liu et al., 2016b; Zhang et al., 2017; Tang et al., 2019; Xu et al., 2019), as well as the Cancer Genome Atlas (TCGA) and Oncomine database (Figure 2). High Notch3 expression in cancer tissues is correlated with a series of clinicopathological features, such as large tumor size, advanced TNM stage, high pathological grade and tumor metastasis, as well as a diminished prognosis of cancer patients, such as poor overall survival (OS), disease-free survival (DFS), relapse-free survival (RFS) and progression-free survival (PFS) (Table 1) (Park et al., 2010; Zhang et al., 2011; Mann et al., 2012; Rahman et al., 2012; Alqudah et al., 2013; Hu et al., 2013; Ye et al., 2013; Zhou et al., 2013; Ozawa et al., 2014; Yuan et al., 2015; Liu et al., 2016a; Liu et al., 2016b; Ma et al., 2016; Zhou et al., 2016; Kim et al., 2017a; Xue et al., 2017; Yu et al., 2017; Zhang et al., 2017; Lin et al., 2018; Tang et al., 2019; Xu et al., 2019; Zhang et al., 2019).
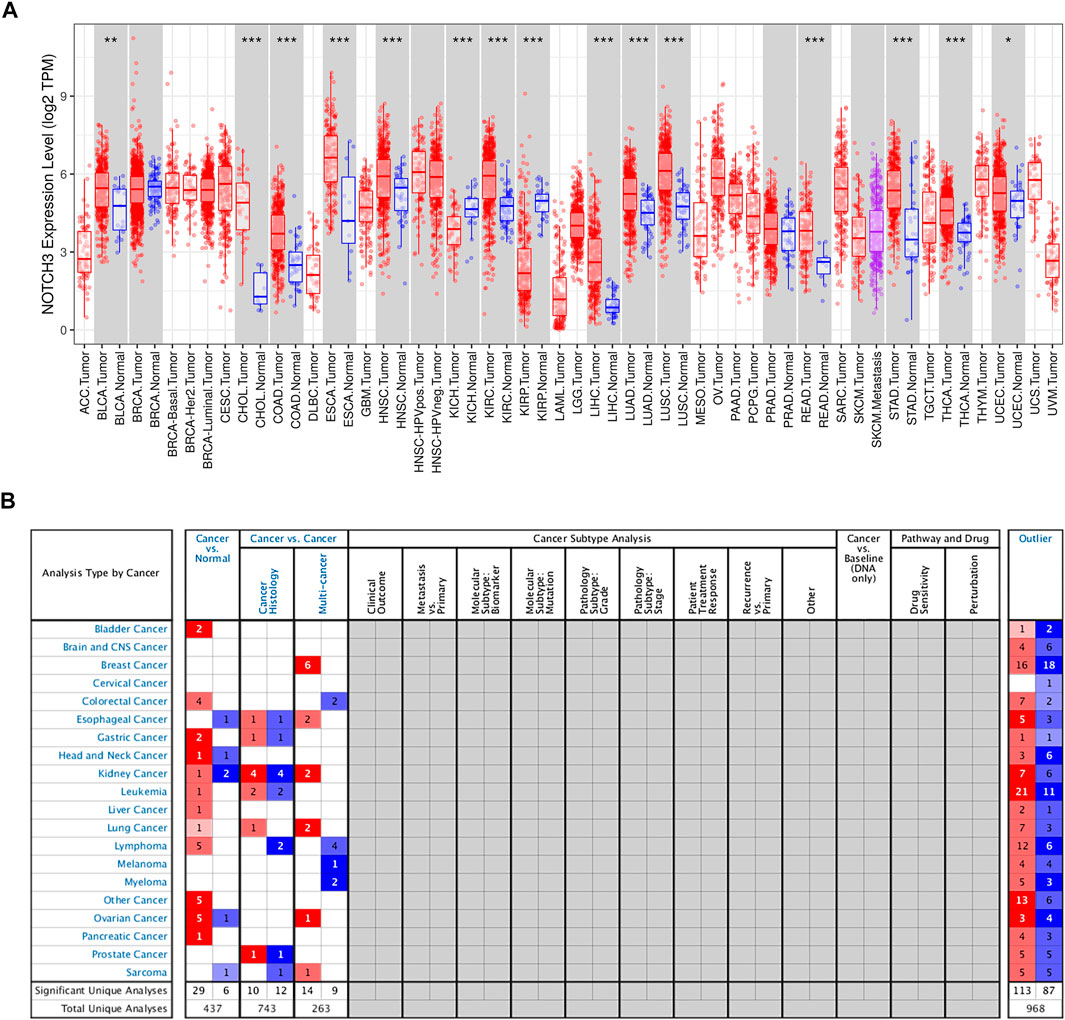
FIGURE 2. The expression of Notch3 in human cancers. (A) The Notch3 expression levels of different cancer types in TCGA were determined using TIMER (https://cistrome.shinyapps.io/timer/) (*p < 0.05, **p < 0.01, ***p < 0.001). (B). Notch3 expression levels in different cancer types from the Oncomine database (www.oncomine.org/).
Notch3 overexpression in cancer is mainly caused by alterations of the Notch3 gene. According to TCGA, the Notch3 gene was altered in 5% of cancer samples, mainly via amplification and mutation (Figure 3). Notch3 has been reported to be amplified in 10–25% of ovarian carcinoma (OC) (Park et al., 2006; Etemadmoghadam et al., 2009; Cancer Genome Atlas Research Network, 2011; Hu et al., 2014). Among all cancer types in the TCGA database, OC has the highest Notch3 amplification rate (11.64%, 68 of 584 cases) (Figure 3). In addition to amplification, mutations in the negative regulatory region (NRR) and proline (P), glutamic acid (E), serine (S), threonine (T)-rich (PEST) domains of Notch3 gene can cause Notch3 activation (gain-of-function/activating mutations), as seen in human T-cell acute lymphoblastic leukemia (T-ALL) (Bernasconi-Elias et al., 2016).
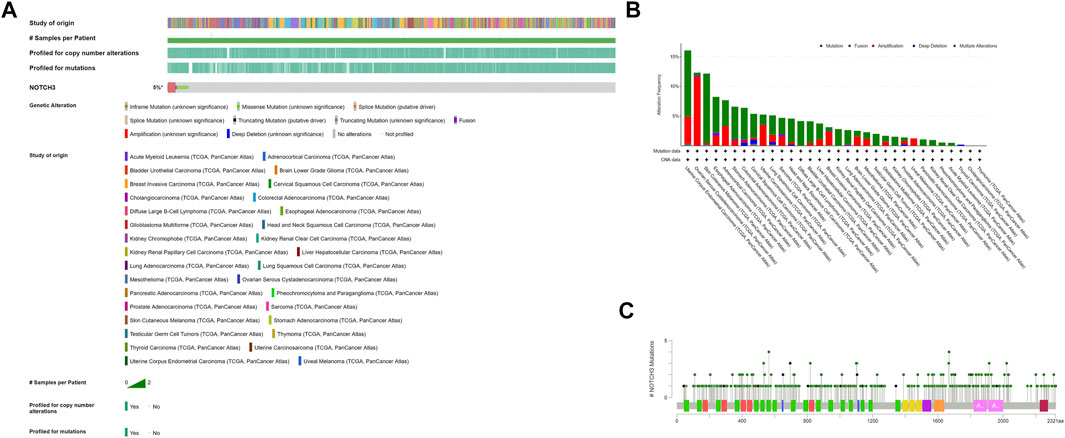
FIGURE 3. Alterations of Notch3 gene in human cancers from TCGA were determined using the cBio Cancer Genomics Portal (http://cbioportal.org). (A) Types of Notch3 gene alterations found in cancer. (B) Cancer-specific Notch3 gene alterations. (C) Cancer-related mutations of Notch3, including 354 missense mutations (green dots) and 55 truncation mutations (black dots).
Notch signaling plays complex roles in regulating cellular behaviors during cancer progression, and each Notch receptor has its specific pattern (Majumder et al., 2021). A major role of Notch3 is maintaining the stemness of cancer stem cells (CSCs). As a population of self-renewing cells with high tumorigenic potency, CSCs are found to be activated by Notch3 signaling in several kinds of cancer and contribute to cancer progression through complex mechanisms (See Notch3 and Cancer Stem Cell Properties). Another main feature of Notch3 signaling is to induce tumor resistance against several kinds of chemotherapeutic drugs, including doxorubicin, platinum, taxane, epidermal growth factor receptor (EGFR)–tyrosine kinase inhibitors (TKIs) and gemcitabine (See in Notch3 and Drug Resistance). Of note, Notch3-supported CSC activity is also involved in the mechanisms of tumor chemoresistance, as well as tumor metastasis and angiogenesis, indicating the key role of Notch3 signaling in cancer (Sullivan et al., 2010; Xiao et al., 2011; McAuliffe et al., 2012; Cheung et al., 2016a; Sansone et al., 2016; Kim et al., 2017a; Kim et al., 2017b; Jeong et al., 2017; Wang et al., 2018a; Leontovich et al., 2018; Liu et al., 2018; Papadakos et al., 2019; Fan et al., 2020; Fang et al., 2020; Mansour et al., 2020).
There are numerous published review articles on the effects of Notch signaling in cancer treatment (Giovannini et al., 2016; Bellavia et al., 2018; Giuli et al., 2019; Katoh and Katoh, 2020). By contrast, this review mainly focuses on the underlying Notch3-related molecular mechanisms that regulate cancer stemness and chemoresistance. In addition, the relationships between Notch3 and other tumor biological characteristics, including metastasis and angiogenesis are also discussed. Finally, we summarize known Notch3-targeting strategies/methods for cancer therapy. Overall, this review provides comprehensive information on the role of Notch3 signaling in cancer and its value as a therapeutic target.
Notch3 and Cancer Stem Cell Properties
Tumor initiation and progression is driven by a small population of cancer cells with self-renewal and tumor-formation capacity, known as CSCs (Dawood et al., 2014). The activation of Notch3 signaling is widely found in CSCs, where it regulates their abundance and activity through several molecular mechanisms (Figure 4). The expression of aldehyde dehydrogenase (ALDH), a recognized CSC marker, is significantly positively correlated with Notch3 expression, as seen in OC, lung carcinoma (LC), hepatocellular carcinoma (HCC) and breast carcinoma (BC) (Sullivan et al., 2010; Xiao et al., 2011; Zhang et al., 2015; Kim et al., 2017a). Suppression of Notch3 signaling in LC cells by treatment with either a γ-secretase inhibitor (GSI) or short hairpin RNA (shRNA) against Notch3 resulted in a significant decrease of ALDH+ CSCs, indicating that Notch3 is critical for ALDH expression (Sullivan et al., 2010).
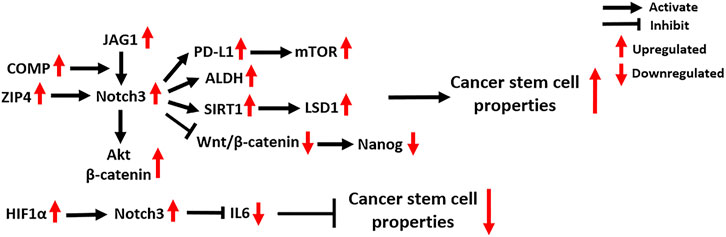
FIGURE 4. Notch3-related molecular mechanisms in cancer stem cells. COMP: Cartilage Oligomeric Matrix Protein; Akt: AKT Serine/Threonine Kinase; PD-L1: Programmed Death Ligand 1; ALDH: Aldehyde dehydrogenase; SIRT1: Sirtuin 1; LSD1: Lysine-specific demethylase 1; HIF1α: Hypoxia Inducible Factor 1 Subunit Alpha; IL6: Interleukin 6.
In OC, the zinc transporter ZIP4 was identified as a novel CSC marker that physically interacts with Notch3 and activates Notch3 signaling (Fan et al., 2020). Several studies also found that the activation of Notch3 signaling enhances CSC activity, especially in chemoresistant OC tumors (Kim et al., 2017b; Jeong et al., 2017; Fang et al., 2020), and the relevant mechanisms are discussed in Platinum and Taxane.
In HCC, the activation of Notch3 signaling was found to inhibit Wnt/β-catenin signaling and increase the expression of the stemness-related protein Nanog, which promotes the maintenance of the CSC population, thereby contributing to the pathogenesis of HCC (Zhang et al., 2015). In addition, Notch3 signaling in liver CSCs is supported by cancer-associated fibroblasts in the tumor microenvironment and maintains tumor cell self-renewal (Liu et al., 2018). Mechanistically, Notch3 signaling activates LSD1, a histone-modifying enzyme that promotes cancer stemness, by inducing its deacetylation by activating the class-III histone deacetylase (HDAC) SIRT1 (Liu et al., 2018).
In the tumor microenvironment of BC, a secreted protein named Cartilage Oligomeric Matrix Protein (COMP) physically bridges Notch3 and JAG1 on the cell membrane of CSCs, thus driving JAG1/Notch3 signaling and subsequently activating the β-catenin and Akt signaling pathways to maintain CSC status (Papadakos et al., 2019). A recent study showed that Notch3 signaling contributes to the overexpression of the T-cell inhibitory molecule PD-L1 in breast CSCs by activating mTOR signaling (Mansour et al., 2020). Specific knockdown of Notch3 can downregulate PD-L1 expression on CSCs and reduce CSC activity, providing a novel strategy for anti-PD-L1 combination therapies (Mansour et al., 2020). In addition to the mechanisms that promote CSC activity, Notch3 signaling is also found to reduce the population of breast CSCs by negatively regulating IL6 (Wang et al., 2018a). Furthermore, the activation of HIF1α in response to hypoxia is involved in Notch3-mediated IL6 inhibition in breast CSCs via direct binding to the Notch3 promoter. The combination of Notch and IL6 inhibitors significantly decreases the abundance of breast CSCs and inhibits BC growth, suggesting it might serve as a novel therapeutic strategy for treating Notch3-expressing BC (Wang et al., 2018a).
Notch3 and Drug Resistance
A large number of studies have shown that Notch3 signaling is closely related to the ability of tumors to chemotherapy. Here, we mainly introduce the roles of Notch3 in the resistance of tumors to five kinds of chemotherapeutic drugs (doxorubicin, platinum, taxane, EGFR-TKIs and gemcitabine), whose mechanisms are comparatively well-understood (Figure 5).
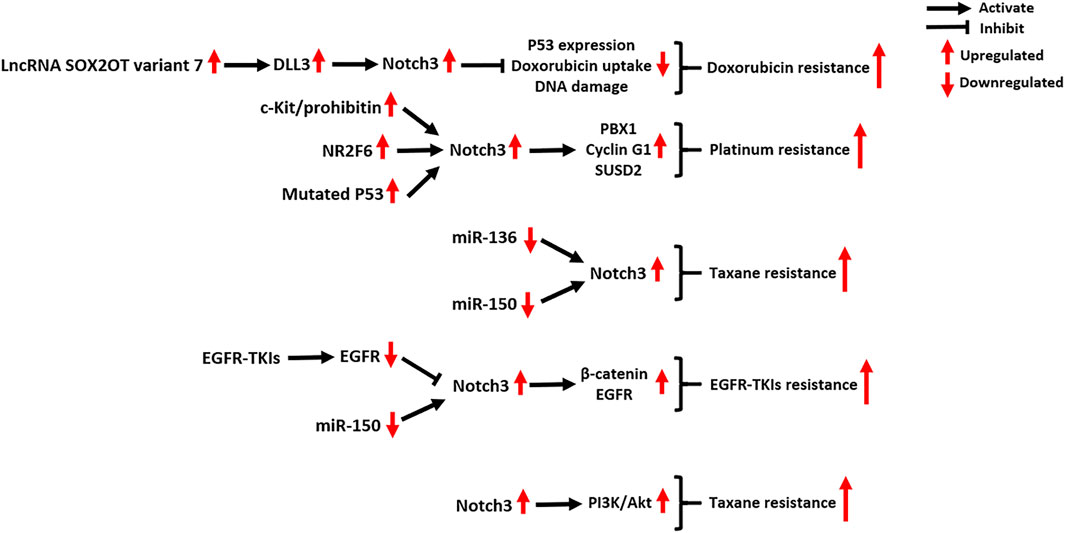
FIGURE 5. Notch3-related molecular mechanisms in cancer drug resistance. NR2F6: Nuclear Receptor Subfamily 2 Group F Member 6; PBX1: PBX Homeobox 1; SUSD2: Sushi Domain Containing two; EGFR-TKIs: epidermal growth factor receptor–tyrosine kinase inhibitors; PI3K: phosphatidylinositol 3-kinase; Akt: AKT Serine/Threonine Kinase.
Doxorubicin
The resistance of tumor cells to doxorubicin, a DNA topoisomerase II inhibitor, is associated with the activation of Notch3 signaling (Giovannini et al., 2009; Michishita et al., 2011; Wang et al., 2018b). In HCC cells, Notch3 signaling contributes to doxorubicin resistance by inhibiting p53 expression, doxorubicin uptake and DNA damage, which can be reversed by Notch3 depletion (Giovannini et al., 2009). In osteosarcoma, both in vivo and in vitro experiments indicate that the lncRNA SOX2OT variant seven can activate DLL3/Notch3 signaling, maintaining the stemness and doxorubicin-resistance of tumor cells (Wang et al., 2018b). Treatment of osteosarcoma cells with epigallocatechin gallate, a polyphenol from green tea, can counteract the SOX2OT-7/DLL3/Notch3 axis, thus inhibiting cancer progression (Wang et al., 2018b).
Platinum and Taxane
Notch3 signaling plays a critical role in tumor resistance to platinum, taxane, or their combination chemotherapy regimens, especially in OC. In a clinical study of 61 OC patients, the high expression of Notch3 was correlated with shorter PFS and OS in patients with stage III and IV disease treated with a standard platinum and taxane chemotherapy regimen (Rahman et al., 2012). In vitro experiments in OC cell lines also confirmed that Notch3 was significantly overexpressed in cisplatin-resistant A2780cis cells (2.5-fold) and paclitaxel (PTX)-resistant SKpac cells (25.5-fold) compared to chemo-sensitive A2780 cells (Kim et al., 2017a).
In cisplatin-resistant OC, Notch3 signaling was found to be induced by the activation of the c-Kit/prohibitin axis and the nuclear orphan receptor NR2F6, as well as P53 mutations (Li et al., 2019; Xu et al., 2019; Fang et al., 2020). The activation of Notch3 signaling activates the stem cell reprogramming factor PBX1, which supports the activity of CSCs contributing to platinum chemoresistance (Fang et al., 2020). In addition, Notch3 signaling also upregulates the expression of Cyclin G1 and SUSD2, which contributes to both tumor metastasis and cisplatin resistance of OC (Xu et al., 2018; Xu et al., 2019). As seen in OC, Epstein-Barr virus (EBV)-associated nasopharyngeal carcinoma and colorectal carcinoma (CRC), inhibition of Notch3 signaling can significantly enhance the cisplatin chemosensitivity of tumor cells, indicating the value of Notch3-targeted therapy (Man et al., 2012; McAuliffe et al., 2012; Tzeng et al., 2014; Xie et al., 2016).
In PTX-resistant OC, the decreased expression of the tumor-suppressive miRNAs miR-136 and miR-150 contributes to the overexpression of Notch3 (Kim et al., 2017b; Jeong et al., 2017). Ectopic expression of miR-136 and miR-150 was found to inhibit Notch3 expression, which suppressed the stemness and angiogenesis of SKpac cells (Kim et al., 2017b; Jeong et al., 2017). In addition, several pre-clinical studies indicated that concomitant treatment with PTX and Notch3-specific inhibitors, including GSI, Small interfering RNA (siRNA) or antibody drugs, can enhance the efficacy of PTX treatment in several tumors, including OC, pancreatic carcinoma (PC), and LC (Groeneweg et al., 2014; Yen et al., 2015; Kang et al., 2016; He et al., 2017; Morgan et al., 2017).
EGFR-TKIs
Studies have revealed novel mechanisms by which Notch3 induces EGFR-TKI resistance in EGFR-mutated tumors. Notch3 receptor was identified as a substrate for EGFR-mediated tyrosine phosphorylation, and EGFR kinase activity induces tyrosine phosphorylation of Notch3, thus inhibiting Notch3 signaling (Arasada et al., 2014). Unfortunately, EGFR-TKI therapy relieves this inhibition, resulting in Notch3 activation and subsequent CSC enrichment (Arasada et al., 2014). In response to EGFR-TKI therapy of non-small-cell lung carcinoma (NSCLC), Notch3 physically binds to β-catenin in the cytoplasm of tumor cells to activate β-catenin signaling (Arasada et al., 2018). The combination of EGFR-TKIs and a β-catenin inhibitor abrogates the Notch3-dependent activation of β-catenin, which strongly attenuates tumor onset, improving the OS and RFS of NSCLC xenograft mice (Arasada et al., 2018). Zhang et al. found that recovering the expression of miR-150 can directly downregulate Notch3 in TKI-resistant NSCLC cell lines, providing another method for reversing Notch3-mediated TKI resistance (Zhang et al., 2019).
In gliomas and triple-negative BC (TNBC), it was found that Notch3 signaling can promote EGFR expression (Alqudah et al., 2013; Diluvio et al., 2018). Notch3 silencing in TKI-resistant TNBC cells induces EGFR dephosphorylation and promotes its intracellular arrest, which increases tumor cell sensitivity to TKI–gefitinib treatment (Diluvio et al., 2018).
Gemcitabine
In a clinical study of 71 PC patients, Notch3 was identified as a novel biomarker for predicting the efficacy of gemcitabine (GEM), whereby low Notch3 expression was associated with better GEM treatment efficacy and longer OS of PC patients (Eto et al., 2013). Mechanistically, Notch3 increases the activity of PI3K/Akt signaling in PC cells in response to GEM treatment, and this effect can be reversed by Notch3-specific siRNAs (Yao and Qian, 2010). In addition, Notch3 signaling also contributes to GEM resistance in NSCLC cells. Treatment with GEM and GSI significantly enhances GEM sensitivity and leads to tumor cell apoptosis, but the underlying molecular mechanisms remain unclear (Hu et al., 2018).
Notch3 in Other Aspects of Cancer Biology
Notch3 in Cancer Epithelial-Mesenchymal Transition and Metastasis
Notch3 has a close relationship with tumor metastasis (Figure 6). In clinical studies, high expression of Notch3 was found to be associated with tumor metastasis in OC, NSCLC, prostate carcinoma (PCa), HCC, PC and gallbladder carcinoma (Ye et al., 2013; Zhou et al., 2013; Liu et al., 2016a; Liu et al., 2016b; Zhou et al., 2016; Kim et al., 2017a; Lin et al., 2018; Kim and Gu, 2019). Matrix metalloproteinases (MMPs) cascade with Notch3 signaling and promote tumor metastasis. The Notch3-MMP-3 axis contributes to bone metastasis of PC by promoting the formation of osteoblastic lesions and decreasing osteoblastogenesis (Ganguly et al., 2020). In HCC and pancreatic ductal adenocarcinoma (PDAC), Notch3 signaling activates the COX-2 and ERK1/2 pathways, which subsequently enhance the migration and invasion of tumor cells by upregulating the expression of MMP-2 and MMP-9 (Zhou et al., 2013; Zhou et al., 2016). In addition, MMP-14 and MMP-28 were found to promote tumor metastasis by inducing Notch3 signaling. The MMP-14-Notch3-β1-integrin axis can be activated by interactions between lymphatic endothelial cells and melanoma cells, leading to the transformation of non-metastatic melanoma cells into invasively sprouting melanoma cells (Pekkonen et al., 2018). The MMP-28-Notch3 axis promotes the Epithelial-Mesenchymal Transition (EMT), migration and invasion of HCC cells in vivo and in vitro (Zhou et al., 2019).
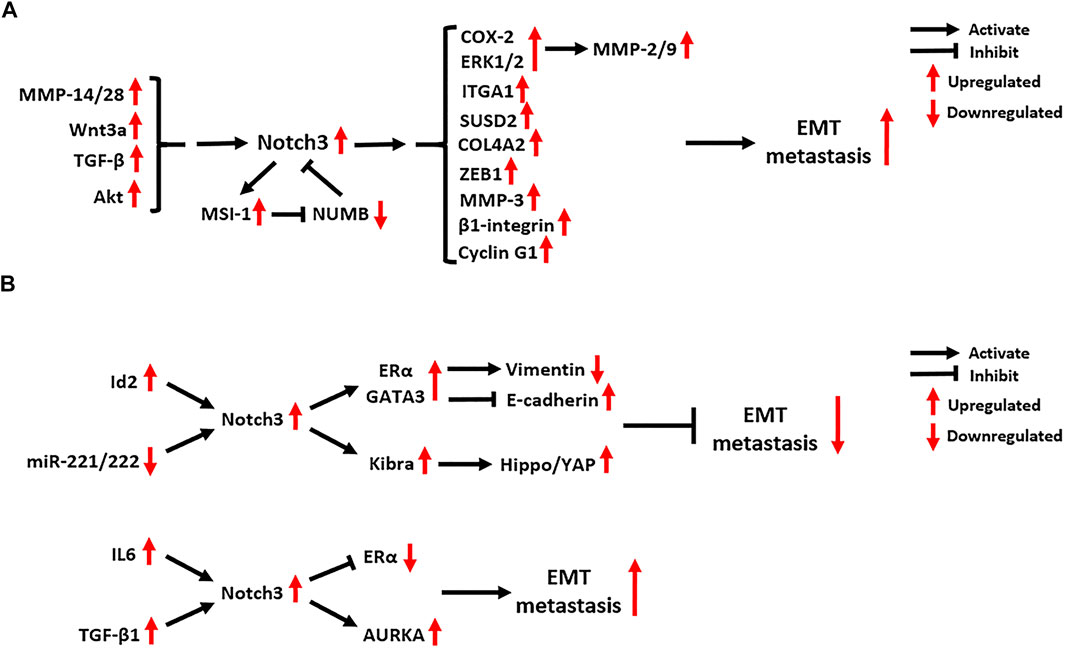
FIGURE 6. Notch3-related EMT molecular mechanisms in (A) tumors other than breast carcinoma and (B) breast carcinoma. EMT: Epithelial-Mesenchymal Transition; MMP: Matrix Metalloproteinase; Wnt3a: Wnt Family Member 3A; TGF-β: Transforming Growth Factor Beta; MSI-1: Musashi RNA Binding Protein 1; NUMB: NUMB Endocytic Adaptor Protein; COX-2: Cyclooxygenase 2; ERK1/2: Extracellular-regulated Kinase 1/2; ITGA1: Integrin Subunit Alpha 1; SUSD2: Sushi Domain Containing 2; COL4A2: Collagen Type IV Alpha 2 Chain; ZEB1: Zinc Finger E-Box Binding Homeobox 1; Id2: Inhibitor Of DNA Binding 2; ERα: Estrogen Receptor α; GATA3: GATA Binding Protein 3; IL6: Interleukin 6; KIBRA: KIdney and BRAin; AURKA: Aurora Kinase A.
In advanced CRC, Notch3 expression is positively correlated with lymph node as well as distant metastasis, and its expression is dependent on the activation of Akt signaling (Varga et al., 2020). Additionally, DLL4/Notch3 signaling was found to upregulate the expression of the RNA-binding protein MSI-1 in metastatic CRC tumors. Active MSI-1 can inhibit the expression of NUMB, a negative regulator of Notch signaling, which maintains the activation of oncogenic Notch1 and Notch3 signaling pathways (Pastò et al., 2014).
As a key component of Wnt signaling, Wnt3a can activate Notch3 signaling to promote the EMT and metastasis of NSCLC (Li et al., 2015). In bone metastasis of NSCLC, Notch3 signaling is also involved in TGF-β signaling-induced EMT by activating the EMT regulator ZEB1 (Liu et al., 2014a). In metastatic OC tumors, several downstream targets of Notch3 signaling were found to be activated, including type IV collagen (COL4A2), sushi domain containing 2 (SUSD2), Cyclin G1 and integrin subunit alpha 1 (ITGA1) (Brown et al., 2015; Xu et al., 2018; Xu et al., 2019; Price et al., 2020). Blocking Notch3 signaling in metastatic OC can inhibit the adhesion, migration and metastasis of tumor cells, while also enhancing their chemo-sensitivity (Brown et al., 2015; Xu et al., 2018; Xu et al., 2019; Price et al., 2020).
The role of Notch3 in BC metastasis is controversial. A study of 72 BC cases reported that Notch3 expression is correlated with a lower risk of lymph node metastasis, as well as the expression of estrogen receptor α (ERα), progesterone receptor (PR) and GATA3 (Lin et al., 2018). N3ICD in the nucleus of BC cells can bind to the promotors of ERα and GATA3 to promote their expression. ERα and GATA3 activated by Notch3 signaling upregulate vimentin expression and repress E-cadherin expression, which then suppresses the EMT and metastasis of BC by maintaining a luminal phenotype (Dou et al., 2017; Lin et al., 2018). Another Notch3-mediated EMT-suppression mechanism in BC relies on the activation of Hippo/YAP signaling by upregulating the transcription of KIBRA, an upstream factor of Hippo signaling (Zhang et al., 2016b). The inhibitor of DNA binding 2 (Id2), a transcription factor belonging to the bHLH family, can promote the transcription of Notch3, thus attenuating the EMT in BC (Wen et al., 2018). By contrast, microRNAs 221 and 222 were found to target the 3’ UTR of Notch3 and suppress its protein translation in BC cells, which reverses EMT inhibition by Notch3 signaling (Liang et al., 2018).
Although the inhibitory effect of Notch3 on BC metastasis has been confirmed in several studies, a pro-EMT function of Notch3 has been also identified. It was found that the activation of Notch3 signaling is linked to BC seeding and lung/brain metastasis, while abrogation of Notch3 reduces the self-renewal and invasion ability of BC cells, restoring a luminal CD44low/CD24high/ERαhigh phenotype (Leontovich et al., 2018). Mechanistically, aberrant Aurora A kinase activity activates Notch3 in breast CSCs and contributes to metastatic growth (Leontovich et al., 2018). In addition, IL6 was found to activate Notch3 signaling in CD133high/ERαlow/IL6high breast CSCs, where it promotes endocrine resistance and metastatic progression (Sansone et al., 2016). In bone-metastatic BC, the activation of JAG1/Notch3 signaling induced by osteoblasts and osteoblast-derived TGF-β1 contributes to aggressive osteolytic metastasis and bone destruction in vivo (Zhang et al., 2010). These findings indicate that the relationship between Notch3 and BC metastasis should be explored further.
Notch3 and Tumor Angiogenesis
The functions of Notch signaling in tumor vasculature are mainly determined by Notch ligands. JAG1-mediated Notch signaling induces neovascularization and sprouting angiogenesis, while DLL4-mediated Notch signaling inhibits tumor angiogenesis (Xiu et al., 2020b; Xiu et al., 2020a). Notch3 is also involved in the regulation of tumor angiogenesis (Figure 7). Immunohistochemistry for Notch3 expression in 105 TNBC tissues showed that its expression is positively correlated with tumor microvascular density (MVD), which suggests a potential pro-angiogenic role of Notch3 (Xue et al., 2017). MUC4, a large membrane-anchored glycoprotein, can facilitate tumor angiogenesis and increase tumor MVD in PC by activating Notch3 signaling and downstream pro-angiogenic genes, including VEGF-A and ANG-2 (Tang et al., 2016). In addition, Notch3 signaling can be activated by interactions between tumor cells and cells in the tumor microenvironment, which contribute to tumor angiogenesis in several cancers. The interactions between tumor cells and cancer-associated fibroblasts activate Notch3 signaling, thus promoting angiogenesis in oral squamous cell carcinoma (Kayamori et al., 2016). Similarly, the interactions between CSCs and endothelial cells (ECs) activate Notch3 signaling to promote angiogenesis in OC, which can be inhibited by Notch3-targeting miRNAs, including miR-136 and miR-150 (Kim et al., 2017b; Jeong et al., 2017). Additionally, the interactions between CSCs and ECs activate Notch3 signaling to promote angiogenesis and vasculogenic mimicry in melanoma (Hsu et al., 2017).
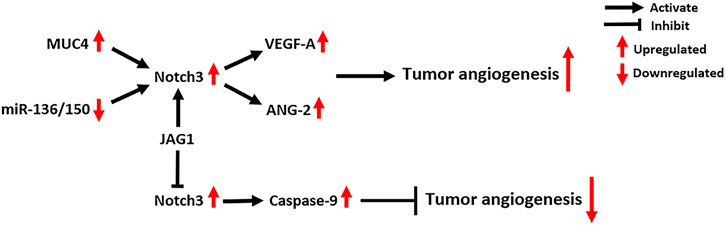
FIGURE 7. Notch3-related molecular mechanisms in tumor angiogenesis. MUC4: Mucin 4, Cell Surface Associated; VEGF-A: Vascular Endothelial Growth Factor A; ANG-2: Angiogenin 2.
Notch3 signaling promotes tumor angiogenesis in a canonical CSL-dependent manner, which requires cell-cell interactions and is driven by Notch ligands. However, Notch3 was also found to act as a dependent receptor in tumor ECs to negatively regulate tumor angiogenesis, circumventing CSL (Lin et al., 2017). Mechanistically, Notch3 receptors on the surface of tumor ECs can directly activate caspase-9, which induces the caspase-dependent cell death of ECs (Lin et al., 2017). Interestingly, overexpression of JAG1 ligand can abrogate this effect and promote tumor neovascularization. This indicates that the effects of Notch3 on tumor angiogenesis depend on the cellular context, such as the availability and amounts of Notch ligands in tumors (Lin et al., 2017).
Notch3-Targeting Strategies for Cancer Therapy
GSIs that prevent the S3 cleavage of Notch receptor are the most commonly used therapeutic option for blocking Notch signaling in cancer (Shih Ie and Wang, 2007). However, they lack specificity and may interfere with the processing of other transmembrane proteins. What’s more, pre-clinical studies have shown that the use of GSIs is associated with severe side effects, such as gastrointestinal tract toxicity (Milano et al., 2004; van Es et al., 2005). Therefore, it is essential to propose other potential methods/strategies that target Notch3 signaling.
Small Interfering RNAs and Short Hairpin RNAs
siRNAs and shRNAs are powerful molecules that can directly knock down the expression of target genes (Rao et al., 2009). In pre-clinical experiments, Notch3-specific siRNAs or shRNAs were able to prevent Notch3 activation and potently inhibit tumor cell growth in vivo and in vitro (Yao and Qian, 2010; Serafin et al., 2011; Hassan et al., 2016; Kang et al., 2016; Diluvio et al., 2018). However, the low efficiency of traditional siRNA/shRNA delivery vehicles remains a significant obstacle for their clinical application. To overcome this, researchers developed a novel aptamer-siRNA chimera-based delivery system to inhibit Notch3 expression (Cheng et al., 2017). The chimera consisting of an aptamer and Notch3-specific siRNA was bonded with cationic Au-Fe3O4 nanoparticles (NPs). This chimera exhibited a high Notch3 silencing efficiency in OC cell lines, as well as potent anti-tumor effects (Cheng et al., 2017). However, there are still no reports on the in vivo use of this Au-Fe3O4 NP-chimera, and its potential value also needs evaluation in clinical studies.
Non-Coding RNAs
Many non-coding RNAs (ncRNAs), including microRNAs (miRNAs), long noncoding RNAs (lncRNAs) and circular RNAs (circRNAs), play oncogenic or tumor-suppressor roles by regulating Notch3 expression (Table 2) (Song et al., 2009; Furukawa et al., 2013; Liu et al., 2014b; Wang et al., 2015; Cheung et al., 2016a; Zhang et al., 2016a; Kim et al., 2017b; Kim et al., 2017b; Cai et al., 2017; Jeong et al., 2017; Liang et al., 2018; Zhang et al., 2018; Yao et al., 2019; Zhang et al., 2019; Zhu et al., 2019; Cai et al., 2020; Chen et al., 2020; Pei et al., 2020; Zhang et al., 2020; Zong et al., 2020; Kang et al., 2021). MiRNAs are small noncoding nucleotides that directly interact with the 3′-untranslated region (3′-UTR) to degrade a targeted mRNA or inhibit its translation (Vishnoi and Rani, 2017). Most Notch3-targeting miRNAs are tumor-suppressive, and are downregulated in tumor tissues compared to normal tissues. Several lncRNAs and circRNAs were found to “sponge” Notch3-targeting miRNAs, thus resulting in Notch3 overexpression and forming competing endogenous RNA (ceRNA) networks in cancer, including the RUSC1-AS1-miR-7-Notch3 axis (Chen et al., 2020), Circ_PUM1-miR-136-Notch3 axis (Zong et al., 2020), LINC00707-miR-206-Notch3 axis (Zhu et al., 2019), Circ_0058124-miR-218-Notch3 axis (Yao et al., 2019), LINC00210-miR-328-Notch3 axis (Zhang et al., 2018), FAM225A/HOTAIR-miR-613-Notch3 axis (Cai et al., 2017; Zhang et al., 2020), and TUG1-miR-1299-Notch3 axis (Pei et al., 2020). Treatment strategies based on inhibiting the expression of these lncRNAs and circRNAs or recovering the expression of miRNAs have been confirmed to suppress Notch3 expression and block Notch3-controlled oncogenic mechanisms in vivo and/or in vitro (Song et al., 2009; Furukawa et al., 2013; Liu et al., 2014b; Wang et al., 2015; Cheung et al., 2016a; Zhang et al., 2016a; Cheung et al., 2016b; Kim et al., 2017b; Cai et al., 2017; Jeong et al., 2017; Liang et al., 2018; Zhang et al., 2018; Yao et al., 2019; Zhang et al., 2019; Zhu et al., 2019; Cai et al., 2020; Chen et al., 2020; Pei et al., 2020; Zhang et al., 2020; Zong et al., 2020; Kang et al., 2021).
Antibodies
Antibodies that target Notch receptors/ligands have been confirmed to effectively modulate Notch signaling activity (Xiu et al., 2020b; Gharaibeh et al., 2020). The monoclonal antibodies (mAbs) named A4, A8, MOR20350 and MOR20358, were designed to bind the NRR domain (Lin-Notch repeat (LNR) and heterodimerization domain (HD) domain) of Notch3 protein, which prevents the exposure of the S2 cleavage site and blocks Notch3 activation (Li et al., 2008; Tiyanont et al., 2013; Bernasconi-Elias et al., 2016). In T-ALL harboring Notch3 gain-of-function mutations, anti-Notch3 NRR mAbs show potent anti-leukemic activity in T-ALL cell lines and tumor xenografts (Bernasconi-Elias et al., 2016).
In addition to anti-Notch3 NRR mAbs, another mAb against epidermal growth factor (EGF) repeats of Notch2/3 named tarextumab (also called OMP-59R5) has been used to block Notch2/3 signaling in pre- and clinical studies (O'Reilly et al., 2015; Yen et al., 2015; Hu et al., 2019; Smith et al., 2019). Tarextumab was found to significantly inhibit the growth of PC, BC, OC and small-cell lung carcinoma (SCLC) xenograft tumors, partly by reducing the abundance of CSCs. Additionally, the combination of tarextumab with GEM plus nab-paclitaxel exhibited more potent anti-tumor effects (Yen et al., 2015). In the phase 1b clinical study NCT01647828, tarextumab in combination with gemcitabine plus nab-PTX was evaluated in 38 untreated metastatic PDAC patients, and the overall response rate (ORR) was 29% (O'Reilly et al., 2015). The recommended phase 2 dose was 15 mg/kg with standard doses of the cytotoxic agents. The frequent tarextumab-related emergent adverse events (TEAEs) were diarrhea (60%) and fatigue (43%), which were mostly grade 1 or 2 (O'Reilly et al., 2015). In another phase 1 study (NCT01277146) dose escalation and expansion of tarextumab was evaluated in 42 patients with solid tumors (Smith et al., 2019). Tarextumab was well tolerated at doses of 2.5 mg/kg weekly, as well as 7.5 mg/kg every 14 or 21 days. Diarrhea (81%) was the most common TEAE, followed by fatigue (48%), nausea (45%) and decreased appetite (38%) (Smith et al., 2019). Unfortunately, the results of a phase 2 study (NCT01859741) indicated that tarextumab treatment in combination with platinum-based therapy in 145 untreated SCLC patients did not improve PFS, OS, or ORR of patients (Daniel et al., 2017). In another phase 2 study (NCT01647828) of 177 untreated metastatic PDAC patients, tarextumab treatment in combination with GEM plus nab-paclitaxel also did not improve the OS, PFS, or ORR, while PFS was even statistically worse in tarextumab-treated patients (Hu et al., 2019). Due to the adverse effects of tarextumab shown in phase 2 clinical trials, its clinical development was discontinued.
As mentioned in EGFR-TKIs, co-blockage of EGFR and Notch receptors is necessary in some cases. In recent studies, bispecific mAbs targeting both Notch2/3 (tarextumab) and EGFR/HER3 (panitumumab/RG7116/MEHD7945A) have been established using the “Knobs into holes” and “CrossMAb” technologies (Hu et al., 2017; Fu et al., 2019). In vivo and in vitro experiments on NSCLC and TNBC showed that EGFR/Notch-bispecific mAbs exhibit potent anti-tumor effects, especially decreasing the abundance of CSCs, which limits tumor resistance to EGFR-TKIs and has potential value for clinical applications (Hu et al., 2017; Fu et al., 2019).
Antibody-Drug Conjugates
Antibody-drug conjugates (ADCs) are mAbs conjugated to small-molecule chemotherapeutic agents via a chemical linker. ADCs can selectively bind to specific targets on the surface of cancer cells and directly deliver the ultra-toxic payload, thus killing cancer cells (Chau et al., 2019). PF06650808, a novel Notch3-targeting ADC, contains a humanized anti-Notch3 IgG1 antibody, a cleavable maleimidocapronic-valinecitruline-p-aminobenzylooxycarbonyl peptide linker, and an auristatin-based cytotoxic payload (Geles et al., 2015). Pre-clinical experiments revealed that PF06650808 can effectively inhibit the growth of TNBC, OC and NSCLC xenograft tumors (Geles et al., 2015). In a recent phase 1, dose-escalation study with 40 solid tumor patients, PF-06650808 was well tolerated at doses ≤2.0 mg/kg, and the maximum tolerated dose was 2.4 mg/kg (Rosen et al., 2020). The most common TEAEs were fatigue (40.0%), decreased appetite (37.5%), nausea (35.0%) and alopecia (32.5%). The ORR and clinical benefit response in the 31 response-evaluable patients was 9.7 and 35.5%, respectively (Rosen et al., 2020). However, the study has been terminated due to a change in sponsor prioritization.
Histone Deacetylase Inhibitors
Histone deacetylases (HDACs) regulate gene transcription by removing active histone marks such as acetyl groups from ε-N-acetyl lysine on a histone, allowing the histones to wrap the DNA more tightly (Jenke et al., 2021). Several reports indicate that impairing the acetylation/deacetylation balance of Notch3 using HDAC inhibitors (HDACi) that favor hyperacetylation can negatively affect the stability and function of Notch3 in cancer cells and tumor xenograft mouse models (Palermo et al., 2012; Jaskula-Sztul et al., 2015; Zhang et al., 2017; Pinazza et al., 2018). Mechanistically, HDACi such as trichostatin A (TSA), suberoylanilide hydroxamic acid (SAHA) and AB3 increase the ubiquitination and proteasomal/lysosomal degradation of Notch3, which reduces its abundance at the cell surface and impairs Notch3 signaling (Palermo et al., 2012; Jaskula-Sztul et al., 2015; Zhang et al., 2017; Pinazza et al., 2018).
Other Drugs/Compounds
Temozolomide (TMZ) is an alkylating chemotherapeutic agent that can penetrate the blood-brain barrier and is clinically used in the treatment of glioblastoma (GBM). One study demonstrated that the inhibition of Notch3 expression contributes to TMZ-induced GBM cytotoxicity (Chen et al., 2017). Mechanistically, TMZ enhances the expression of the ER stress protein CHAC1 by activating JNK1/c-JUN signaling. Subsequently, CHAC1 binds to Notch3 protein, which reduces the generation of N3ICD, thus preventing Notch3 signaling (Chen et al., 2017). However, a recent study found that TMZ can also activate DLL4/Notch3 signaling to maintain CSC properties in GBM by upregulating MMP14 expression (Ulasov et al., 2020). Thus, the effect of TMZ on Notch3 signaling needs further exploration.
In HCC chemotherapy, a well-tolerated combination of sorafenib and valproic acid was found to synergistically inhibit tumor growth by downregulating Notch3 and p-Akt (Zhu et al., 2017). Mangiferin, a C-glucosyl xanthone (1,3,6,7-tetrahydroxy-xanthone-C2-β-D-glucoside), can specifically repress Notch3 signaling, which increases apoptosis and inhibits OC tumor growth both in vitro and in vivo (Zou et al., 2017). In spite of these success stories, more drugs/compounds that potentially target Notch3 should be screened in the future.
Discussion and Conclusion
Notch3 signaling plays critical roles in cancer progression, and the related molecular mechanisms have been studied in some detail. Stem cell-like properties are a primary feature of Notch3-positive cancer cells, and the overexpression of Notch3 may act as a biomarker for CSCs (See Notch3 and Cancer Stem Cell Properties). Notch3 signaling can regulate tumor resistance to chemotherapeutic drugs including doxorubicin, platinum, taxane, EGFR-TKIs, and gemcitabine, which is also dependent on CSCs (See Notch3 and Drug Resistance).
To maintain the stemness and proliferation of tumor cells, Notch3 signaling can activate the expression of downstream genes, such as cell cycle-related genes (CCND1, C-MYC and NF-kB1), antiapoptotic genes (SURVIVIN and BCL2), as well as stemness-related genes (OCT-4, ALDH1, NANOG, PBX1, CD44 and CD133) (Park et al., 2010; Man et al., 2012; McAuliffe et al., 2012; Alqudah et al., 2013; Jeong et al., 2017). In addition, there are several associations and cross-talk interactions between Notch3 signaling and other signaling pathways, mainly including the Wnt/β-catenin, Akt, IL6, EGFR, TGF-β and NF-κB signaling pathways, which affect several aspects of cancer cell behavior (Table 3). In tumor metastasis, Notch3 signaling was found to cascade the MMP, Wnt, Akt, IL6 and TGF-β signaling pathways, thereby promoting the invasion and EMT of tumor cells (See Notch3 in Cancer EMT and Metastasis). However, anti-EMT properties and mechanisms of Notch3 signaling were also found in BC, suggesting a controversial role of Notch3 in BC metastasis (Zhang et al., 2016b; Dou et al., 2017; Liang et al., 2018; Lin et al., 2018; Wen et al., 2018). Moreover, Notch3 signaling activated by cell-cell interactions between tumor cells and tumor ECs can promote tumor angiogenesis and vasculogenic mimicry (See Notch3 and Tumor Angiogenesis). These findings suggest that Notch3 has diverse, complex and wide-ranging roles in tumor cells.
To prevent the abnormal activation of Notch3 signaling in cancer, key Notch3-targeting strategies have been proposed and confirmed effective in pre-clinical studies, including the application of siRNAs/shRNAs, ncRNAs, antibodies, ADCs, and HDACi (See Notch3-Targeting Strategies for Cancer Therapy). Different mAbs against Notch3 can specially block Notch3 signaling. However, phase 2 clinical trials of the anti-Notch2/3 mAb drug tarextumab have showed poor efficacy, and the relevant clinical trials have been terminated (Daniel et al., 2017; Hu et al., 2019). Recently, bispecific mAbs targeting both Notch2/3 and EGFR/HER3 have been developed. The main advantage of these bispecific mAbs is that they can target/block both Notch3 and EGFR signaling, which reverses the activation of Notch3 signaling in response to EGFR-TKIs (Hu et al., 2017; Fu et al., 2019). The efficacy of bispecific mAbs may be worth testing in future clinical trials.
ADCs are novel drugs that can kill tumor cells which express specific target molecules. The first Notch3-targeting ADC drug PF06650808 has been evaluated in pre-clinical experiments and a phase 1 clinical trial. Although preliminary, the results demonstrate a manageable safety profile and early signs of anti-tumor activity in cancer patients (Geles et al., 2015; Rosen et al., 2020). Other Notch3-targeting strategies, such as siRNAs/shRNAs, non-coding RNAs and HDACi, have not been tested in clinical trials, so that their efficacy and safety in the treatment of cancer patients need to be evaluated in the future.
In order to develop Notch3-targeting methods/drugs for cancer treatment, the following potential strategies should be considered: 1) Inhibiting Notch3 gene expression using siRNAs, shRNAs, or ncRNAs. 2) Preventing the cleavage of Notch3 protein using small molecules such as using ADAM10 inhibitors (prevent S2 cleavage) and GSIs (prevent S3 cleavage). 3) Antibodies targeting Notch3 protein. 4) Killing Notch3-positive tumor cells by ADCs. 5) Promoting Notch3 degradation by HDACi. Notably, Notch3-specific inhibitors such as antibodies and ADCs are more specific than pan-Notch inhibitors such as GSIs, which may merit further pre- and clinical evaluation.
In summary, Notch3 signaling affects cancer progression through complex molecular mechanisms. Future studies should investigate the relevant mechanisms and exact roles of Notch3 signaling in regulating different cancer behaviors (such as CSC properties, Epithelial-Mesenchymal Transition (EMT), metastasis, drug resistance and angiogenesis) in different tumor types. Furthermore, it is necessary to propose, establish and evaluate more potential Notch3-targeting methods/strategies for cancer treatment.
Author Contributions
MX wrote the manuscript. YW, BL, XW, FX, SC, LZ, BZ, and FH directed the project. All authors read and approved the manuscript.
Funding
This work was supported by grants from the National Natural Science Foundation of China (81860259); Provincal Science foudation of Jiangxi (20202BABL206016); Youth Team Project of the Second Affiliated Hospital of Nanchang University (2019YNTD12003).
Conflict of Interest
The authors declare that the research was conducted in the absence of any commercial or financial relationships that could be construed as a potential conflict of interest.
Abbreviations
CSL, CBF-1 (RBPJ)/suppressor of hairless/Lag1; TNM, tumor node metastasis; ZIP4, zinc transporter 4; LSD1, lysine-specific demethylase 1; SIRT1, Sirtuin1; akt, AKT serine/threonine kinase; PBX1, PBX homeobox 1; SUSD2, sushi domain containing 2; PI3K, phosphatidylinositol 3-kinase; PD-L1, programmed death ligand 1; mTOR, mammalian target of rapamycin; IL6, interleukin 6; HIF1α, hypoxia inducible factor 1 subunit alpha; COX-2, cyclooxygenase 2; ERK1/2, extracellular-regulated kinase 1/2; MSI-1, musashi RNA binding protein 1; NUMB, NUMB endocytic adaptor protein; TGF-β, transforming growth factor beta; ZEB1, zinc finger E-box binding homeobox 1; GATA3, GATA binding protein 3; KIBRA, kidney and BRAin; NR2F6, nuclear receptor subfamily 2 group F member 6; MUC4, mucin 4, cell surface associated; VEGF-A, vascular endothelial growth factor a; ANG-2, angiogenin 2; CHAC1, cation transport regulator-like protein 1; JNK1, c-jun N-terminal kinase 1; CCND1, cyclin D1; NF-κB, nuclear factor kappa B; OCT-4, organic cation/carnitine transporter 4.
References
Alqudah, M. A. Y., Agarwal, S., Al-Keilani, M. S., Sibenaller, Z. A., Ryken, T. C., and Assem, M. (2013). NOTCH3 Is a Prognostic Factor that Promotes Glioma Cell Proliferation, Migration and Invasion via Activation of CCND1 and EGFR. PLoS One 8 (10), e77299, doi:10.1371/journal.pone.0077299
Arasada, R. R., Amann, J. M., Rahman, M. A., Huppert, S. S., and Carbone, D. P. (2014). EGFR Blockade Enriches for Lung Cancer Stem-like Cells through Notch3-dependent Signaling. Cancer Res. 74 (19), 5572–5584. doi:10.1158/0008-5472.Can-13-3724
Arasada, R. R., Shilo, K., Yamada, T., Zhang, J., Yano, S., Ghanem, R., et al. (2018). Notch3-dependent β-catenin Signaling Mediates EGFR TKI Drug Persistence in EGFR Mutant NSCLC. Nat. Commun. 9 (1), 3198. doi:10.1038/s41467-018-05626-2
Bellavia, D., Campese, A. F., Alesse, E., Vacca, A., Felli, M. P., Balestri, A., et al. (2000). Constitutive Activation of NF-kappaB and T-Cell Leukemia/lymphoma in Notch3 Transgenic Mice. Embo j 19 (13), 3337–3348. doi:10.1093/emboj/19.13.3337
Bellavia, D., Checquolo, S., Palermo, R., and Screpanti, I. (2018). The Notch3 Receptor and its Intracellular Signaling-dependent Oncogenic Mechanisms. Adv. Exp. Med. Biol. 1066, 205–222. doi:10.1007/978-3-319-89512-3_10
Bernasconi-Elias, P., Hu, T., Jenkins, D., Firestone, B., Gans, S., Kurth, E., et al. (2016). Characterization of Activating Mutations of NOTCH3 in T-Cell Acute Lymphoblastic Leukemia and Anti-leukemic Activity of NOTCH3 Inhibitory Antibodies. Oncogene 35 (47), 6077–6086. doi:10.1038/onc.2016.133
Bray, S. J. (2016). Notch Signalling in Context. Nat. Rev. Mol. Cel Biol 17 (11), 722–735. doi:10.1038/nrm.2016.94
Bray, S. J. (2006). Notch Signalling: a Simple Pathway Becomes Complex. Nat. Rev. Mol. Cel Biol 7 (9), 678–689. doi:10.1038/nrm2009
Brown, C. W., Brodsky, A. S., and Freiman, R. N. (2015). Notch3 Overexpression Promotes Anoikis Resistance in Epithelial Ovarian Cancer via Upregulation of COL4A2. Mol. Cancer Res. 13 (1), 78–85. doi:10.1158/1541-7786.Mcr-14-0334
Cai, H., Yao, J., An, Y., Chen, X., Chen, W., Wu, D., et al. (2017). LncRNA HOTAIR Acts as Competing Endogenous RNA to Control the Expression of Notch3 via Sponging miR-613 in Pancreatic Cancer. Oncotarget 8 (20), 32905–32917. doi:10.18632/oncotarget.16462
Cai, W. T., Guan, P., Lin, M. X., Fu, B., Wu, B., and Wu, J. (2020). MiRNA-206 Suppresses the Metastasis of Osteosarcoma via Targeting Notch3. J. Biol. Regul. Homeost Agents 34 (3), 775–783. doi:10.23812/20-72-a-26
Cancer Genome Atlas Research Network (2011). Integrated Genomic Analyses of Ovarian Carcinoma. Nature 474 (7353), 609–615. doi:10.1038/nature10166
Chau, C. H., Steeg, P. S., and Figg, W. D. (2019). Antibody-drug Conjugates for Cancer. The Lancet 394 (10200), 793–804. doi:10.1016/s0140-6736(19)31774-x
Chen, P.-H., Shen, W.-L., Shih, C.-M., Ho, K.-H., Cheng, C.-H., Lin, C.-W., et al. (2017). The CHAC1-Inhibited Notch3 Pathway Is Involved in Temozolomide-Induced Glioma Cytotoxicity. Neuropharmacology 116, 300–314. doi:10.1016/j.neuropharm.2016.12.011
Chen, X., Stoeck, A., Lee, S. J., Shih, I.-M., Wang, M. M., and Wang, T.-L. (2010). Jagged1 Expression Regulated by Notch3 and Wnt/β-Catenin Signaling Pathways in Ovarian Cancer. Oncotarget 1 (3), 210–218. doi:10.18632/oncotarget.127
Chen, Y. A., Cheng, L., Zhang, Y., Peng, L., and Yang, H. G. (2021). LncRNA RUSC1-AS1 Promotes the Proliferation of Hepatocellular Carcinoma Cells through Modulating NOTCH Signaling. neo 67 (6), 1204–1213. doi:10.4149/neo_2020_191010N1024
Cheng, R., Cai, X.-r., Ke, K., and Chen, Y.-l. (2017). Notch4 Inhibition Suppresses Invasion and Vasculogenic Mimicry Formation of Hepatocellular Carcinoma Cells. Curr. Med. Sci. 37 (5), 719–725. doi:10.1007/s11596-017-1794-9
Cheung, C. C.-M., Lun, S. W.-M., Chung, G. T.-Y., Chow, C., Lo, C., Choy, K.-W., et al. (2016a). MicroRNA-183 Suppresses Cancer Stem-like Cell Properties in EBV-Associated Nasopharyngeal Carcinoma. BMC Cancer 16, 495. doi:10.1186/s12885-016-2525-5
Cheung, C. M., Lun, W. M., Chung, T. Y., Chow, C., Lo, C., Choy, K. W., et al. (2016b). MicroRNA-183 Suppresses Cancer Stem-like Cell Properties in EBV-Associated Nasopharyngeal Carcinoma. Bmc Cancer 16 (1), 495. doi:10.1186/s12885-016-2525-5
Daniel, D. B., Rudin, C. M., Hart, L., Spigel, D. R., Edelman, M. J., Goldschmidt, J., et al. (2017). Results of a Randomized, Placebo-Controlled, Phase 2 Study of Tarextumab (TRXT, Anti-notch2/3) in Combination with Etoposide and Platinum (EP) in Patients (Pts) with Untreated Extensive-Stage Small-Cell Lung Cancer (ED-SCLC). Ann. Oncol. 28, v540–v542. doi:10.1093/annonc/mdx386.004
Dawood, S., Austin, L., and Cristofanilli, M. (2014). Cancer Stem Cells: Implications for Cancer Therapy. Oncology (Williston Park) 28 (12), 1101–1110. 25510809
Diluvio, G., Del Gaudio, F., Giuli, M. V., Franciosa, G., Giuliani, E., Palermo, R., et al. (2018). NOTCH3 Inactivation Increases Triple Negative Breast Cancer Sensitivity to Gefitinib by Promoting EGFR Tyrosine Dephosphorylation and its Intracellular Arrest. Oncogenesis 7 (5), 42. doi:10.1038/s41389-018-0051-9
Dou, X.-W., Liang, Y.-K., Lin, H.-Y., Wei, X.-L., Zhang, Y.-Q., Bai, J.-W., et al. (2017). Notch3 Maintains Luminal Phenotype and Suppresses Tumorigenesis and Metastasis of Breast Cancer via Trans-activating Estrogen Receptor-α. Theranostics 7 (16), 4041–4056. doi:10.7150/thno.19989
Etemadmoghadam, D., deFazio, A., Beroukhim, R., Mermel, C., George, J., Getz, G., et al. (2009). Integrated Genome-wide DNA Copy Number and Expression Analysis Identifies Distinct Mechanisms of Primary Chemoresistance in Ovarian Carcinomas. Clin. Cancer Res. 15 (4), 1417–1427. doi:10.1158/1078-0432.Ccr-08-1564
Eto, K., Kawakami, H., Kuwatani, M., Kudo, T., Abe, Y., Kawahata, S., et al. (2013). Human Equilibrative Nucleoside Transporter 1 and Notch3 Can Predict Gemcitabine Effects in Patients with Unresectable Pancreatic Cancer. Br. J. Cancer 108 (7), 1488–1494. doi:10.1038/bjc.2013.108
Fan, Q., Zhang, W., Emerson, R. E., and Xu, Y. (2020). ZIP4 Is a Novel Cancer Stem Cell Marker in High-Grade Serous Ovarian Cancer. Cancers 12 (12), 3692. doi:10.3390/cancers12123692
Fang, C.-H., Lin, Y.-T., Liang, C.-M., and Liang, S.-M. (2020). A Novel C-Kit/phospho-Prohibitin axis Enhances Ovarian Cancer Stemness and Chemoresistance via Notch3-PBX1 and β-catenin-ABCG2 Signaling. J. Biomed. Sci. 27 (1), 42. doi:10.1186/s12929-020-00638-x
Fu, W., Lei, C., Yu, Y., Liu, S., Li, T., Lin, F., et al. (2019). EGFR/Notch Antagonists Enhance the Response to Inhibitors of the PI3K-Akt Pathway by Decreasing Tumor-Initiating Cell Frequency. Clin. Cancer Res. 25 (9), 2835–2847. doi:10.1158/1078-0432.Ccr-18-2732
Furukawa, S., Kawasaki, Y., Miyamoto, M., Hiyoshi, M., Kitayama, J., and Akiyama, T. (2013). The miR-1-NOTCH3-Asef Pathway Is Important for Colorectal Tumor Cell Migration. PLoS One 8 (11), e80609. doi:10.1371/journal.pone.0080609
Ganguly, S. S., Hostetter, G., Tang, L., Frank, S. B., Saboda, K., Mehra, R., et al. (2020). Notch3 Promotes Prostate Cancer-Induced Bone Lesion Development via MMP-3. Oncogene 39 (1), 204–218. doi:10.1038/s41388-019-0977-1
Geles, K. G., Gao, Y., Sridharan, L., Giannakou, A., Yamin, T.-T., Golas, J., et al. (2015). Abstract 1697: Therapeutic Targeting the NOTCH3 Receptor with Antibody Drug Conjugates. Cancer Res. 75, 1697. doi:10.1158/1538-7445.AM2015-1697
Gharaibeh, L., Elmadany, N., Alwosaibai, K., and Alshaer, W. (2020). Notch1 in Cancer Therapy: Possible Clinical Implications and Challenges. Mol. Pharmacol. 98 (5), 559–576. doi:10.1124/molpharm.120.000006
Giovannini, C., Bolondi, L., and Gramantieri, L. (2016). Targeting Notch3 in Hepatocellular Carcinoma: Molecular Mechanisms and Therapeutic Perspectives. Ijms 18 (1), 56. doi:10.3390/ijms18010056
Giovannini, C., Gramantieri, L., Chieco, P., Minguzzi, M., Lago, F., Pianetti, S., et al. (2009). Selective Ablation of Notch3 in HCC Enhances Doxorubicin's Death Promoting Effect by a P53 Dependent Mechanism. J. Hepatol. 50 (5), 969–979. doi:10.1016/j.jhep.2008.12.032
Giuli, M. V., Giuliani, E., Screpanti, I., Bellavia, D., and Checquolo, S. (2019). Notch Signaling Activation as a Hallmark for Triple-Negative Breast Cancer Subtype. J. Oncol. 2019, 1–15. doi:10.1155/2019/8707053
Groeneweg, J. W., DiGloria, C. M., Yuan, J., Richardson, W. S., Growdon, W. B., Sathyanarayanan, S., et al. (2014). Inhibition of Notch Signaling in Combination with Paclitaxel Reduces Platinum-Resistant Ovarian Tumor Growth. Front. Oncol. 4, 171. doi:10.3389/fonc.2014.00171
Guest, R. V., Boulter, L., Dwyer, B. J., Kendall, T. J., Man, T.-Y., Minnis-Lyons, S. E., et al. (2016). Notch3 Drives Development and Progression of Cholangiocarcinoma. Proc. Natl. Acad. Sci. USA 113 (43), 12250–12255. doi:10.1073/pnas.1600067113
Hassan, W. A., Yoshida, R., Kudoh, S., Motooka, Y., and Ito, T. (2016). Evaluation of Role of Notch3 Signaling Pathway in Human Lung Cancer Cells. J. Cancer Res. Clin. Oncol. 142 (5), 981–993. doi:10.1007/s00432-016-2117-4
He, F., Du, T., Jiang, Q., and Zhang, Y. (2017). Synergistic Effect of Notch-3-specific Inhibition and Paclitaxel in Non-small Cell Lung Cancer (NSCLC) Cells via Activation of the Intrinsic Apoptosis Pathway. Med. Sci. Monit. 23, 3760–3769. doi:10.12659/msm.902641
Hsu, M.-Y., Yang, M. H., Schnegg, C. I., Hwang, S., Ryu, B., and Alani, R. M. (2017). Notch3 Signaling-Mediated Melanoma-Endothelial Crosstalk Regulates Melanoma Stem-like Cell Homeostasis and Niche Morphogenesis. Lab. Invest. 97 (6), 725–736. doi:10.1038/labinvest.2017.1
Hu, B. D., Guo, J., Ye, Y. Z., Du, T., Cheng, C. S., Jiang, Q., et al. (2018). Specific Inhibitor of Notch-3 Enhances the Sensitivity of NSCLC Cells to Gemcitabine. Oncol. Rep. 40 (1), 155–164. doi:10.3892/or.2018.6448
Hu, L., Xue, F., Shao, M., Deng, A., and Wei, G. (2013). Aberrant Expression of Notch3 Predicts Poor Survival for Hepatocellular Carcinomas. Biosci. Trends 7 (3), 152–156.
Hu, S., Fu, W., Li, T., Yuan, Q., Wang, F., Lv, G., et al. (2017). Antagonism of EGFR and Notch Limits Resistance to EGFR Inhibitors and Radiation by Decreasing Tumor-Initiating Cell Frequency. Sci. Transl. Med. 9 (380), eaag0339. doi:10.1126/scitranslmed.aag0339
Hu, W., Liu, T., Ivan, C., Sun, Y., Huang, J., Mangala, L. S., et al. (2014). Notch3 Pathway Alterations in Ovarian Cancer. Cancer Res. 74 (12), 3282–3293. doi:10.1158/0008-5472.Can-13-2066
Hu, Z. I., Bendell, J. C., Bullock, A., LoConte, N. K., Hatoum, H., Ritch, P., et al. (2019). A Randomized Phase II Trial of Nab‐paclitaxel and Gemcitabine with Tarextumab or Placebo in Patients with Untreated Metastatic Pancreatic Cancer. Cancer Med. 8 (11), 5148–5157. doi:10.1002/cam4.2425
Jaskula-Sztul, R., Eide, J., Tesfazghi, S., Dammalapati, A., Harrison, A. D., Yu, X.-M., et al. (2015). Tumor-suppressor Role of Notch3 in Medullary Thyroid Carcinoma Revealed by Genetic and Pharmacological Induction. Mol. Cancer Ther. 14 (2), 499–512. doi:10.1158/1535-7163.Mct-14-0073
Jenke, R., Reßing, N., Hansen, F. K., Aigner, A., and Büch, T. (2021). Anticancer Therapy with HDAC Inhibitors: Mechanism-Based Combination Strategies and Future Perspectives. Cancers 13 (4), 634. doi:10.3390/cancers13040634
Jeong, J.-Y., Kang, H., Kim, T. H., Kim, G., Heo, J.-H., Kwon, A.-Y., et al. (2017). MicroRNA-136 Inhibits Cancer Stem Cell Activity and Enhances the Anti-tumor Effect of Paclitaxel against Chemoresistant Ovarian Cancer Cells by Targeting Notch3. Cancer Lett. 386, 168–178. doi:10.1016/j.canlet.2016.11.017
Kang, H., Jeong, J.-Y., Song, J.-Y., Kim, T. H., Kim, G., Huh, J. H., et al. (2016). Notch3-specific Inhibition Using siRNA Knockdown or GSI Sensitizes Paclitaxel-Resistant Ovarian Cancer Cells. Mol. Carcinog. 55 (7), 1196–1209. doi:10.1002/mc.22363
Kang, W., Zhang, J., Huang, T., Zhou, Y., Wong, C. C., Chan, R. C. K., et al. (2021). NOTCH3, a Crucial Target of miR-491-5p/miR-875-5p, Promotes Gastric Carcinogenesis by Upregulating PHLDB2 Expression and Activating Akt Pathway. Oncogene 40, 1578–1594. doi:10.1038/s41388-020-01579-3
Katoh, M., and Katoh, M. (2020). Precision Medicine for Human Cancers with Notch Signaling Dysregulation (Review). Int. J. Mol. Med. 45 (2), 279–297. doi:10.3892/ijmm.2019.4418
Kayamori, K., Katsube, K.-i., Sakamoto, K., Ohyama, Y., Hirai, H., Yukimori, A., et al. (2016). NOTCH3 Is Induced in Cancer-Associated Fibroblasts and Promotes Angiogenesis in Oral Squamous Cell Carcinoma. PLoS One 11 (4), e0154112. doi:10.1371/journal.pone.0154112
Kim, A. R., and Gu, M. J. (2019). The Clinicopathologic Significance of Notch3 Expression in Prostate Cancer. Int. J. Clin. Exp. Pathol. 12 (9), 3535–3541. 31934201
Kim, M. J., Kim, A.-R., Jeong, J.-Y., Kim, K.-i., Kim, T.-H., Lee, C., et al. (2017a). Correlation of ALDH1 and Notch3 Expression: Clinical Implication in Ovarian Carcinomas. J. Cancer 8 (16), 3331–3342. doi:10.7150/jca.18955
Kim, T. H., Jeong, J.-Y., Park, J.-Y., Kim, S.-W., Heo, J. H., Kang, H., et al. (2017b). miR-150 Enhances Apoptotic and Anti-tumor Effects of Paclitaxel in Paclitaxel-Resistant Ovarian Cancer Cells by Targeting Notch3. Oncotarget 8 (42), 72788–72800. doi:10.18632/oncotarget.20348
Kopan, R., and Ilagan, M. X. G. (2009). The Canonical Notch Signaling Pathway: Unfolding the Activation Mechanism. Cell 137 (2), 216–233. doi:10.1016/j.cell.2009.03.045
Leontovich, A. A., Jalalirad, M., Salisbury, J. L., Mills, L., Haddox, C., Schroeder, M., et al. (2018). NOTCH3 Expression Is Linked to Breast Cancer Seeding and Distant Metastasis. Breast Cancer Res. 20 (1), 105. doi:10.1186/s13058-018-1020-0
Li, C., Song, G., Zhang, S., Wang, E., and Cui, Z. (2015). Wnt3a Increases the Metastatic Potential of Non-small Cell Lung Cancer Cells In Vitro in Part via its Upregulation of Notch3. Oncol. Rep. 33 (3), 1207–1214. doi:10.3892/or.2014.3700
Li, C., Zhang, S., Lu, Y., Zhang, Y., Wang, E., and Cui, Z. (2013). The Roles of Notch3 on the Cell Proliferation and Apoptosis Induced by CHIR99021 in NSCLC Cell Lines: a Functional Link between Wnt and Notch Signaling Pathways. PLoS One 8 (12), e84659. doi:10.1371/journal.pone.0084659
Li, C., Zhang, Y., Lu, Y., Cui, Z., Yu, M., Zhang, S., et al. (2011). Evidence of the Cross Talk between Wnt and Notch Signaling Pathways in Non-small-cell Lung Cancer (NSCLC): Notch3-siRNA Weakens the Effect of LiCl on the Cell Cycle of NSCLC Cell Lines. J. Cancer Res. Clin. Oncol. 137 (5), 771–778. doi:10.1007/s00432-010-0934-4
Li, H., Zhang, W., Niu, C., Lin, C., Wu, X., Jian, Y., et al. (2019). Nuclear Orphan Receptor NR2F6 Confers Cisplatin Resistance in Epithelial Ovarian Cancer Cells by Activating the Notch3 Signaling Pathway. Int. J. Cancer 145 (7), 1921–1934. doi:10.1002/ijc.32293
Li, K., Li, Y., Wu, W., Gordon, W. R., Chang, D. W., Lu, M., et al. (2008). Modulation of Notch Signaling by Antibodies Specific for the Extracellular Negative Regulatory Region of NOTCH3. J. Biol. Chem. 283 (12), 8046–8054. doi:10.1074/jbc.M800170200
Liang, Y.-K., Lin, H.-Y., Dou, X.-W., Chen, M., Wei, X.-L., Zhang, Y.-Q., et al. (2018). MiR-221/222 Promote Epithelial-Mesenchymal Transition by Targeting Notch3 in Breast Cancer Cell Lines. NPJ Breast Cancer 4, 20. doi:10.1038/s41523-018-0073-7
Lin, H.-Y., Liang, Y.-K., Dou, X.-W., Chen, C.-F., Wei, X.-L., Zeng, D., et al. (2018). Notch3 Inhibits Epithelial-Mesenchymal Transition in Breast Cancer via a Novel Mechanism, Upregulation of GATA-3 Expression. Oncogenesis 7 (8), 59. doi:10.1038/s41389-018-0069-z
Lin, S., Negulescu, A., Bulusu, S., Gibert, B., Delcros, J.-G., Ducarouge, B., et al. (2017). Non-canonical NOTCH3 Signalling Limits Tumour Angiogenesis. Nat. Commun. 8, 16074. doi:10.1038/ncomms16074
Liu, C., Liu, L., Chen, X., Cheng, J., Zhang, H., Zhang, C., et al. (2018). LSD1 Stimulates Cancer-Associated Fibroblasts to Drive Notch3-dependent Self-Renewal of Liver Cancer Stem-like Cells. Cancer Res. 78 (4), 938–949. doi:10.1158/0008-5472.Can-17-1236
Liu, L., Chen, X., Wang, Y., Qu, Z., Lu, Q., Zhao, J., et al. (2014a). Notch3 Is Important for TGF-β-Induced Epithelial-Mesenchymal Transition in Non-small Cell Lung Cancer Bone Metastasis by Regulating ZEB-1. Cancer Gene Ther. 21 (9), 364–372. doi:10.1038/cgt.2014.39
Liu, L., Yang, Z.-l., Wang, C., Miao, X., Liu, Z., Li, D., et al. (2016a). The Expression of Notch 1 and Notch 3 in Gallbladder Cancer and Their Clinicopathological Significance. Pathol. Oncol. Res. 22 (3), 483–492. doi:10.1007/s12253-015-0019-4
Liu, W., Xu, C., Wan, H., Liu, C., Wen, C., Lu, H., et al. (2014b). MicroRNA-206 Overexpression Promotes Apoptosis, Induces Cell Cycle Arrest and Inhibits the Migration of Human Hepatocellular Carcinoma HepG2 Cells. Int. J. Mol. Med. 34 (2), 420–428. doi:10.3892/ijmm.2014.1800
Liu, Z., Yun, R., Yu, X., Hu, H., Huang, G., Tan, B., et al. (2016b). Overexpression of Notch3 and pS6 Is Associated with Poor Prognosis in Human Ovarian Epithelial Cancer. Mediators Inflamm. 2016, 1–6. doi:10.1155/2016/5953498
Ma, Y., Li, M., Si, J., Xiong, Y., Lu, F., Zhang, J., et al. (2016). Blockade of Notch3 Inhibits the Stem-like Property and Is Associated with ALDH1A1 and CD44 via Autophagy in Non-small Lung Cancer. Int. J. Oncol. 48 (6), 2349–2358. doi:10.3892/ijo.2016.3464
Majumder, S., Crabtree, J. S., Golde, T. E., Minter, L. M., Osborne, B. A., and Miele, L. (2021). Targeting Notch in Oncology: the Path Forward. Nat. Rev. Drug Discov. 20 (2), 125–144. doi:10.1038/s41573-020-00091-3
Man, C.-H., Wei-Man Lun, S., Wai-Ying Hui, J., To, K.-F., Choy, K.-W., Wing-Hung Chan, A., et al. (2012). Inhibition of NOTCH3 Signalling Significantly Enhances Sensitivity to Cisplatin in EBV-Associated Nasopharyngeal Carcinoma. J. Pathol. 226 (3), 471–481. doi:10.1002/path.2997
Mann, C. D., Bastianpillai, C., Neal, C. P., Masood, M. M., Jones, D. J. L., Teichert, F., et al. (2012). Notch3 and HEY-1 as Prognostic Biomarkers in Pancreatic Adenocarcinoma. PLoS One 7 (12), e51119. doi:10.1371/journal.pone.0051119
Mansour, F. A., Al-Mazrou, A., Al-Mohanna, F., Al-Alwan, M., and Ghebeh, H. (2020). PD-L1 Is Overexpressed on Breast Cancer Stem Cells through notch3/mTOR axis. Oncoimmunology 9 (1), 1729299. doi:10.1080/2162402x.2020.1729299
McAuliffe, S. M., Morgan, S. L., Wyant, G. A., Tran, L. T., Muto, K. W., Chen, Y. S., et al. (2012). Targeting Notch, a Key Pathway for Ovarian Cancer Stem Cells, Sensitizes Tumors to Platinum Therapy. Proc. Natl. Acad. Sci. 109 (43), E2939–E2948. doi:10.1073/pnas.1206400109
Michishita, M., Akiyoshi, R., Yoshimura, H., Katsumoto, T., Ichikawa, H., Ohkusu-Tsukada, K., et al. (2011). Characterization of Spheres Derived from Canine Mammary Gland Adenocarcinoma Cell Lines. Res. Vet. Sci. 91 (2), 254–260. doi:10.1016/j.rvsc.2010.11.016
Milano, J., McKay, J., Dagenais, C., Foster-Brown, L., Pognan, F., Gadient, R., et al. (2004). Modulation of Notch Processing by γ-Secretase Inhibitors Causes Intestinal Goblet Cell Metaplasia and Induction of Genes Known to Specify Gut Secretory Lineage Differentiation. Toxicol. Sci. 82 (1), 341–358. doi:10.1093/toxsci/kfh254
Morgan, K. M., Fischer, B. S., Lee, F. Y., Shah, J. J., Bertino, J. R., Rosenfeld, J., et al. (2017). Gamma Secretase Inhibition by BMS-906024 Enhances Efficacy of Paclitaxel in Lung Adenocarcinoma. Mol. Cancer Ther. 16 (12), 2759–2769. doi:10.1158/1535-7163.Mct-17-0439
O'Reilly, E. M., Smith, L. S., Bendell, J. C., Strickler, J. H., Zalupski, M., Gluck, W., et al. (2015). Final Results of Phase Ib of Anticancer Stem Cell Antibody Tarextumab (OMP-59R5, TRXT, Anti-notch 2/3) in Combination with Nab-Paclitaxel and Gemcitabine (Nab-P+Gem) in Patients (Pts) with Untreated Metastatic Pancreatic Cancer (mPC). Jco 33 (3_Suppl. l), 278. doi:10.1200/jco.2015.33.3_suppl.278
Ozawa, T., Kazama, S., Akiyoshi, T., Murono, K., Yoneyama, S., Tanaka, T., et al. (2014). Nuclear Notch3 Expression Is Associated with Tumor Recurrence in Patients with Stage II and III Colorectal Cancer. Ann. Surg. Oncol. 21 (8), 2650–2658. doi:10.1245/s10434-014-3659-9
Palermo, R., Checquolo, S., Giovenco, A., Grazioli, P., Kumar, V., Campese, A. F., et al. (2012). Acetylation Controls Notch3 Stability and Function in T-Cell Leukemia. Oncogene 31 (33), 3807–3817. doi:10.1038/onc.2011.533
Papadakos, K. S., Bartoschek, M., Rodriguez, C., Gialeli, C., Jin, S.-B., Lendahl, U., et al. (2019). Cartilage Oligomeric Matrix Protein Initiates Cancer Stem Cells through Activation of Jagged1-Notch3 Signaling. Matrix Biol. 81, 107–121. doi:10.1016/j.matbio.2018.11.007
Park, J. T., Chen, X., Tropè, C. G., Davidson, B., Shih, I.-M., and Wang, T.-L. (2010). Notch3 Overexpression Is Related to the Recurrence of Ovarian Cancer and Confers Resistance to Carboplatin. Am. J. Pathol. 177 (3), 1087–1094. doi:10.2353/ajpath.2010.100316
Park, J. T., Li, M., Nakayama, K., Mao, T.-L., Davidson, B., Zhang, Z., et al. (2006). Notch3 Gene Amplification in Ovarian Cancer. Cancer Res. 66 (12), 6312–6318. doi:10.1158/0008-5472.Can-05-3610
Pastò, A., Serafin, V., Pilotto, G., Lago, C., Bellio, C., Trusolino, L., et al. (2014). NOTCH3 Signaling Regulates MUSASHI-1 Expression in Metastatic Colorectal Cancer Cells. Cancer Res. 74 (7), 2106–2118. doi:10.1158/0008-5472.Can-13-2022
Pei, Y., Li, K., Lou, X., Wu, Y., Dong, X., Wang, W., et al. (2020). miR-1299/NOTCH3/TUG1 Feedback Loop Contributes to the Malignant Proliferation of Ovarian Cancer. Oncol. Rep. 44 (2), 438–448. doi:10.3892/or.2020.7623
Pekkonen, P., Alve, S., Balistreri, G., Gramolelli, S., Tatti-Bugaeva, O., Paatero, I., et al. (2018). Lymphatic Endothelium Stimulates Melanoma Metastasis and Invasion via MMP14-dependent Notch3 and β1-integrin Activation. Elife 7, e32490. doi:10.7554/eLife.32490
Pinazza, M., Ghisi, M., Minuzzo, S., Agnusdei, V., Fossati, G., Ciminale, V., et al. (2018). Histone Deacetylase 6 Controls Notch3 Trafficking and Degradation in T-Cell Acute Lymphoblastic Leukemia Cells. Oncogene 37 (28), 3839–3851. doi:10.1038/s41388-018-0234-z
Price, J. C., Azizi, E., Naiche, L. A., Parvani, J. G., Shukla, P., Kim, S., et al. (2020). Notch3 Signaling Promotes Tumor Cell Adhesion and Progression in a Murine Epithelial Ovarian Cancer Model. PLoS One 15 (6), e0233962. doi:10.1371/journal.pone.0233962
Qiao, J., Liu, J., Jia, K., Li, N., Liu, B., Zhang, Q., et al. (2016). Diosmetin Triggers Cell Apoptosis by Activation of the p53/Bcl-2 Pathway and Inactivation of the Notch3/NF-Κb Pathway in HepG2 Cells. Oncol. Lett. 12 (6), 5122–5128. doi:10.3892/ol.2016.5347
Rahman, M. T., Nakayama, K., Rahman, M., Katagiri, H., Katagiri, A., Ishibashi, T., et al. (2012). Notch3 Overexpression as Potential Therapeutic Target in Advanced Stage Chemoresistant Ovarian Cancer. Am. J. Clin. Pathol. 138 (4), 535–544. doi:10.1309/ajcpkdlrq8f3ewns
Rao, D. D., Vorhies, J. S., Senzer, N., and Nemunaitis, J. (2009). siRNA vs. shRNA: Similarities and Differences. Adv. Drug Deliv. Rev. 61 (9), 746–759. doi:10.1016/j.addr.2009.04.004
Rosen, L. S., Wesolowski, R., Baffa, R., Liao, K.-H., Hua, S. Y., Gibson, B. L., et al. (2020). A Phase I, Dose-Escalation Study of PF-06650808, an Anti-notch3 Antibody-Drug Conjugate, in Patients with Breast Cancer and Other Advanced Solid Tumors. Invest. New Drugs 38 (1), 120–130. doi:10.1007/s10637-019-00754-y
Sansone, P., Ceccarelli, C., Berishaj, M., Chang, Q., Rajasekhar, V. K., Perna, F., et al. (2016). Self-renewal of CD133hi Cells by IL6/Notch3 Signalling Regulates Endocrine Resistance in Metastatic Breast Cancer. Nat. Commun. 7, 10442. doi:10.1038/ncomms10442
Serafin, V., Persano, L., Moserle, L., Esposito, G., Ghisi, M., Curtarello, M., et al. (2011). Notch3 Signalling Promotes Tumour Growth in Colorectal Cancer. J. Pathol. 224 (4), 448–460. doi:10.1002/path.2895
Shih, I.-M., and Wang, T.-L. (2007). Notch Signaling, γ-Secretase Inhibitors, and Cancer Therapy: Figure 1. Cancer Res. 67 (5), 1879–1882. doi:10.1158/0008-5472.Can-06-3958
Smith, D. C., Chugh, R., Patnaik, A., Papadopoulos, K. P., Wang, M., Kapoun, A. M., et al. (2019). A Phase 1 Dose Escalation and Expansion Study of Tarextumab (OMP-59R5) in Patients with Solid Tumors. Invest. New Drugs 37 (4), 722–730. doi:10.1007/s10637-018-0714-6
Song, G., Zhang, Y., and Wang, L. (2009). MicroRNA-206 Targets Notch3, Activates Apoptosis, and Inhibits Tumor Cell Migration and Focus Formation. J. Biol. Chem. 284 (46), 31921–31927. doi:10.1074/jbc.M109.046862
Sullivan, J. P., Spinola, M., Dodge, M., Raso, M. G., Behrens, C., Gao, B., et al. (2010). Aldehyde Dehydrogenase Activity Selects for Lung Adenocarcinoma Stem Cells Dependent on Notch Signaling. Cancer Res. 70 (23), 9937–9948. doi:10.1158/0008-5472.Can-10-0881
Tang, J., Zhu, Y., Xie, K., Zhang, X., Zhi, X., Wang, W., et al. (2016). The Role of the AMOP Domain in MUC4/Y-Promoted Tumour Angiogenesis and Metastasis in Pancreatic Cancer. J. Exp. Clin. Cancer Res. 35 (1), 91. doi:10.1186/s13046-016-0369-0
Tang, X., Cao, Y., Peng, D., Zhao, G., Zeng, Y., Gao, Z., et al. (2019). Overexpression of Notch3 Is Associated with Metastasis and Poor Prognosis in Osteosarcoma Patients. Cmar 11, 547–559. doi:10.2147/cmar.S185495
Tiyanont, K., Wales, T. E., Siebel, C. W., Engen, J. R., and Blacklow, S. C. (2013). Insights into Notch3 Activation and Inhibition Mediated by Antibodies Directed against its Negative Regulatory Region. J. Mol. Biol. 425 (17), 3192–3204. doi:10.1016/j.jmb.2013.05.025
Tzeng, T. J., Cao, L., Fu, Y., Zeng, H., and Cheng, W.-H. (2014). Methylseleninic Acid Sensitizes Notch3-Activated OVCA429 Ovarian Cancer Cells to Carboplatin. PLoS One 9 (7), e101664. doi:10.1371/journal.pone.0101664
Ulasov, I. V., Mijanovic, O., Savchuk, S., Gonzalez‐Buendia, E., Sonabend, A., Xiao, T., et al. (2020). TMZ Regulates GBM stemnessviaMMP14‐DLL4‐Notch3 Pathway. Int. J. Cancer 146 (8), 2218–2228. doi:10.1002/ijc.32636
Vacca, A., Felli, M. P., Palermo, R., Di Mario, G., Calce, A., Di Giovine, M., et al. (2006). Notch3 and Pre-TCR Interaction Unveils Distinct NF-Κb Pathways in T-Cell Development and Leukemia. Embo j 25 (5), 1000–1008. doi:10.1038/sj.emboj.7600996
van Es, J. H., van Gijn, M. E., Riccio, O., van den Born, M., Vooijs, M., Begthel, H., et al. (2005). Notch/γ-secretase Inhibition Turns Proliferative Cells in Intestinal Crypts and Adenomas into Goblet Cells. Nature 435 (7044), 959–963. doi:10.1038/nature03659
Varga, J., Nicolas, A., Petrocelli, V., Pesic, M., Mahmoud, A., Michels, B. E., et al. (2020). AKT-dependent NOTCH3 Activation Drives Tumor Progression in a Model of Mesenchymal Colorectal Cancer. J. Exp. Med. 217 (10). e20191515. doi:10.1084/jem.20191515
Vishnoi, A., and Rani, S. (2017). MiRNA Biogenesis and Regulation of Diseases: An Overview. Methods Mol. Biol. 1509, 1–10. doi:10.1007/978-1-4939-6524-3_1
Wang, D., Xu, J., Liu, B., He, X., Zhou, L., Hu, X., et al. (2018a). IL6 Blockade Potentiates the Anti-tumor Effects of γ-secretase Inhibitors in Notch3-Expressing Breast Cancer. Cell Death Differ 25 (2), 330–339. doi:10.1038/cdd.2017.162
Wang, W., Chen, D., and Zhu, K. (2018b). SOX2OT Variant 7 Contributes to the Synergistic Interaction between EGCG and Doxorubicin to Kill Osteosarcoma via Autophagy and Stemness Inhibition. J. Exp. Clin. Cancer Res. 37 (1), 37. doi:10.1186/s13046-018-0689-3
Wang, X.-W., Xi, X.-Q., Wu, J., Wan, Y.-Y., Hui, H.-X., and Cao, X.-F. (2015). MicroRNA-206 Attenuates Tumor Proliferation and Migration Involving the Downregulation of NOTCH3 in Colorectal Cancer. Oncol. Rep. 33 (3), 1402–1410. doi:10.3892/or.2015.3731
Wen, X.-F., Chen, M., Wu, Y., Chen, M.-N., Glogowska, A., Klonisch, T., et al. (2018). Inhibitor of DNA Binding 2 Inhibits Epithelial-Mesenchymal Transition via Up-Regulation of Notch3 in Breast Cancer. Translational Oncol. 11 (5), 1259–1270. doi:10.1016/j.tranon.2018.07.015
Xiao, Y., Ye, Y., Zou, X., Jones, S., Yearsley, K., Shetuni, B., et al. (2011). The Lymphovascular Embolus of Inflammatory Breast Cancer Exhibits a Notch 3 Addiction. Oncogene 30 (3), 287–300. doi:10.1038/onc.2010.405
Xie, T., Li, Y., Li, S.-L., and Luo, H.-F. (2016). Astragaloside IV Enhances Cisplatin Chemosensitivity in Human Colorectal Cancer via Regulating NOTCH3. Oncol. Res. 24 (6), 447–453. doi:10.3727/096504016x14685034103590
Xiu, M.-x., Liu, Y.-m., and Kuang, B.-h. (2020a). The Oncogenic Role of Jagged1/Notch Signaling in Cancer. Biomed. Pharmacother. 129, 110416. doi:10.1016/j.biopha.2020.110416
Xiu, M.-X., Liu, Y.-M., and Kuang, B.-h. (2020b). The Role of DLLs in Cancer: A Novel Therapeutic Target. Ott 13, 3881–3901. doi:10.2147/ott.S244860
Xiu, M. X., and Liu, Y. M. (2019). The Role of Oncogenic Notch2 Signaling in Cancer: a Novel Therapeutic Target. Am. J. Cancer Res. 9 (5), 837–854.
Xu, Y., Miao, C., Jin, C., Qiu, C., Li, Y., Sun, X., et al. (2018). SUSD2 Promotes Cancer Metastasis and Confers Cisplatin Resistance in High Grade Serous Ovarian Cancer. Exp. Cel Res. 363 (2), 160–170. doi:10.1016/j.yexcr.2017.12.029
Xu, Y., Zhang, Q., Miao, C., Dongol, S., Li, Y., Jin, C., et al. (2019). CCNG1 (Cyclin G1) Regulation by Mutant-P53 via Induction of Notch3 Expression Promotes High-Grade Serous Ovarian Cancer (HGSOC) Tumorigenesis and Progression. Cancer Med. 8 (1), 351–362. doi:10.1002/cam4.1812
Xue, S., He, L., Zhang, X., Zhou, J., Li, F., and Wang, X. (2017). Expression of Jagged1/Notch3 Signaling Pathway and Their Relationship with the Tumor Angiogenesis in TNBC. Arch. Med. Res. 48 (2), 169–179. doi:10.1016/j.arcmed.2017.03.014
Yao, J., and Qian, C. (2010). Inhibition of Notch3 Enhances Sensitivity to Gemcitabine in Pancreatic Cancer through an Inactivation of PI3K/Akt-dependent Pathway. Med. Oncol. 27 (3), 1017–1022. doi:10.1007/s12032-009-9326-5
Yao, Y., Chen, X., Yang, H., Chen, W., Qian, Y., Yan, Z., et al. (2019). Hsa_circ_0058124 Promotes Papillary Thyroid Cancer Tumorigenesis and Invasiveness through the NOTCH3/GATAD2A axis. J. Exp. Clin. Cancer Res. 38 (1), 318. doi:10.1186/s13046-019-1321-x
Ye, Y.-z., Zhang, Z.-h., Fan, X.-y., Xu, X.-l., Chen, M.-l., Chang, B.-w., et al. (2013). Notch3 Overexpression Associates with Poor Prognosis in Human Non-small-cell Lung Cancer. Med. Oncol. 30 (2), 595. doi:10.1007/s12032-013-0595-7
Yen, W.-C., Fischer, M. M., Axelrod, F., Bond, C., Cain, J., Cancilla, B., et al. (2015). Targeting Notch Signaling with a Notch2/Notch3 Antagonist (Tarextumab) Inhibits Tumor Growth and Decreases Tumor-Initiating Cell Frequency. Clin. Cancer Res. 21 (9), 2084–2095. doi:10.1158/1078-0432.Ccr-14-2808
Yu, T., Han, C., Zhu, G., Liao, X., Qin, W., Yang, C., et al. (2017). Prognostic Value of Notch Receptors in Postsurgical Patients with Hepatitis B Virus-Related Hepatocellular Carcinoma. Cancer Med. 6 (7), 1587–1600. doi:10.1002/cam4.1077
Yuan, X., Wu, H., Xu, H., Han, N., Chu, Q., Yu, S., et al. (2015). Meta-analysis Reveals the Correlation of Notch Signaling with Non-small Cell Lung Cancer Progression and Prognosis. Sci. Rep. 5, 10338. doi:10.1038/srep10338
Zhang, H., Liu, L., Liu, C., Pan, J., Lu, G., Zhou, Z., et al. (2017). Notch3 Overexpression Enhances Progression and Chemoresistance of Urothelial Carcinoma. Oncotarget 8 (21), 34362–34373. doi:10.18632/oncotarget.16156
Zhang, Q., Li, Q., Xu, T., Jiang, H., and Xu, L.-G. (2016a). miR-491-5p Suppresses Cell Growth and Invasion by Targeting Notch3 in Nasopharyngeal Carcinoma. Oncol. Rep. 35 (6), 3541–3547. doi:10.3892/or.2016.4713
Zhang, Q., Lu, C., Fang, T., Wang, Y., Hu, W., Qiao, J., et al. (2015). Notch3 Functions as a Regulator of Cell Self-Renewal by Interacting with the β-catenin Pathway in Hepatocellular Carcinoma. Oncotarget 6 (6), 3669–3679. doi:10.18632/oncotarget.2898
Zhang, S., Li, P., Zhao, L., and Xu, L. (2018). LINC00210 as a miR-328-5p Sponge Promotes Nasopharyngeal Carcinoma Tumorigenesis by Activating NOTCH3 Pathway. Biosci. Rep. 38 (6). doi:10.1042/bsr20181168
Zhang, T.-H., Liu, H.-C., Zhu, L.-J., Chu, M., Liang, Y.-J., Liang, L.-Z., et al. (2011). Activation of Notch Signaling in Human Tongue Carcinoma. J. Oral Pathol. Med. 40 (1), 37–45. doi:10.1111/j.1600-0714.2010.00931.x
Zhang, X., Liu, X., Luo, J., Xiao, W., Ye, X., Chen, M., et al. (2016b). Notch3 Inhibits Epithelial-Mesenchymal Transition by Activating Kibra-Mediated Hippo/YAP Signaling in Breast Cancer Epithelial Cells. Oncogenesis 5 (11), e269. doi:10.1038/oncsis.2016.67
Zhang, X., Shi, H., Yao, J., Li, Y., Gao, B., Zhang, Y., et al. (2020). FAM225A Facilitates Colorectal Cancer Progression by Sponging miR‐613 to Regulate NOTCH3. Cancer Med. 9 (12), 4339–4349. doi:10.1002/cam4.3053
Zhang, Y., Chen, B., Wang, Y., Zhao, Q., Wu, W., Zhang, P., et al. (2019). NOTCH3 Overexpression and Posttranscriptional Regulation by miR-150 Were Associated with EGFR‐TKI Resistance in Lung Adenocarcinoma. Oncol. Res. 27 (7), 751–761. doi:10.3727/096504018x15372657298381
Zhang, Z., Wang, H., Ikeda, S., Fahey, F., Bielenberg, D., Smits, P., et al. (2010). Notch3 in Human Breast Cancer Cell Lines Regulates Osteoblast-Cancer Cell Interactions and Osteolytic Bone Metastasis. Am. J. Pathol. 177 (3), 1459–1469. doi:10.2353/ajpath.2010.090476
Zhou, J.-X., Zhou, L., Li, Q.-J., Feng, W., Wang, P.-M., Li, E.-F., et al. (2016). Association between High Levels of Notch3 Expression and High Invasion and Poor Overall Survival Rates in Pancreatic Ductal Adenocarcinoma. Oncol. Rep. 36 (5), 2893–2901. doi:10.3892/or.2016.5079
Zhou, J., Zheng, X., Feng, M., Mo, Z., Shan, Y., Wang, Y., et al. (2019). Upregulated MMP28 in Hepatocellular Carcinoma Promotes Metastasis via Notch3 Signaling and Predicts Unfavorable Prognosis. Int. J. Biol. Sci. 15 (4), 812–825. doi:10.7150/ijbs.31335
Zhou, L., Zhang, N., Song, W., You, N., Li, Q., Sun, W., et al. (2013). The Significance of Notch1 Compared with Notch3 in High Metastasis and Poor Overall Survival in Hepatocellular Carcinoma. PLoS One 8 (2), e57382. doi:10.1371/journal.pone.0057382
Zhu, H., He, G., Wang, Y., Hu, Y., Zhang, Z., Qian, X., et al. (2019). Long Intergenic Noncoding RNA 00707 Promotes Colorectal Cancer Cell Proliferation and Metastasis by Sponging miR-206. Ott 12, 4331–4340. doi:10.2147/ott.S198140
Zhu, W., Liang, Q., Yang, X., Yu, Y., Shen, X., and Sun, G. (2017). Combination of Sorafenib and Valproic Acid Synergistically Induces Cell Apoptosis and Inhibits Hepatocellular Carcinoma Growth via Down-Regulating Notch3 and pAkt. Am. J. Cancer Res. 7 (12), 2503–2514. 29312803
Zong, Z. H., Liu, Y., Chen, S., and Zhao, Y. (2020). Circ_PUM1 Promotes the Development of Endometrial Cancer by Targeting the miR‐136/NOTCH3 Pathway. J. Cel Mol Med 24 (7), 4127–4135. doi:10.1111/jcmm.15069
Keywords: cancer, Notch3, mechanism, targeted therapy, cancer biology
Citation: Xiu M, Wang Y, Li B, Wang X, Xiao F, Chen S, Zhang L, Zhou B and Hua F (2021) The Role of Notch3 Signaling in Cancer Stemness and Chemoresistance: Molecular Mechanisms and Targeting Strategies. Front. Mol. Biosci. 8:694141. doi: 10.3389/fmolb.2021.694141
Received: 12 April 2021; Accepted: 27 May 2021;
Published: 14 June 2021.
Edited by:
Matteo Becatti, University of Firenze, ItalyReviewed by:
Parham Jabbarzadeh Kaboli, Southwest Medical University, ChinaShanchun Guo, Xavier University of Louisiana, United States
Copyright © 2021 Xiu, Wang, Li, Wang, Xiao, Chen, Zhang, Zhou and Hua. This is an open-access article distributed under the terms of the Creative Commons Attribution License (CC BY). The use, distribution or reproduction in other forums is permitted, provided the original author(s) and the copyright owner(s) are credited and that the original publication in this journal is cited, in accordance with accepted academic practice. No use, distribution or reproduction is permitted which does not comply with these terms.
*Correspondence: Fuzhou Hua, aHVhZnV6aG91QDEyNi5jb20=