- Institute of Biochemistry and Molecular Medicine, University of Bern, Bern, Switzerland
In mammalian cells, the L-type amino acid transporters (LATs) LAT1 (SLC7A5) and LAT2 (SLC7A8) form heterodimeric amino acid transporters (HATs) with the ancillary protein 4F2hc and are involved in the cellular uptake of specific amino acids. The HAT 4F2hc-LAT1 is found upregulated in various cancer cell types, while 4F2hc-LAT2 is a transporter for non-cancer cells. Preclinical studies have highlighted that 4F2hc-LAT1 plays an important role in tumor progression representing a valid anticancer target. Consequently, current research is focusing on the development of potent and specific human 4F2hc-LAT1 inhibitors. On the other hand, 4F2hc-LAT2 is emerging as target of other diseases, thus also gaining clinical interest. To determine affinity and specificity of substrates and inhibitors for 4F2hc-LAT1 or 4F2hc-LAT2, robust transport cell assays are indispensable. We have optimized and validated a transport assay using cells of the methylotrophic yeast Pichia pastoris stably overexpressing the human HATs 4F2hc-LAT1 or -LAT2, and the LATs LAT1 or LAT2 alone. The radioligand [3H]L-leucine was used as reporter and the substrates L-leucine, triiodothyronine (T3) and thyroxine (T4) as well as the inhibitors BCH and JPH203 (KYT-0353) for assay validation. Obtained half-maximal inhibitory concentrations also provided new insights, e.g., into the LAT specificity of the potent inhibitor JPH203 and on the potency of the thyroid hormones T3 and T4 to inhibit transport through human 4F2hc-LAT2. The LAT1 and LAT2 assays are of particular interest to determine possible implications and influences of 4F2hc in ligand binding and transport. In summary, the presented assays are valuable for characterization of ligands, e.g., towards 4F2hc-LAT1 specificity, and can also be applied for compound screening. Finally, our established approach and assay would also be applicable to other HATs and LATs of interest.
Introduction
Amino acids have diverse and essential roles in cell function, e.g., for protein synthesis, metabolism, signal transduction, neural transmission, and cellular growth and proliferation. Transport of amino acids across biological membranes is mediated by amino acid transporters, which are embedded in lipid bilayers of cells (Christensen, 1990; McGivan and Pastor-Anglada, 1994). Malfunction, absence or overexpression of amino acid transporters can affect homeostasis in the body leading to human diseases. The solute carrier (SLC) superfamily includes currently eleven families containing amino acid transporters (Kandasamy et al., 2018). The SLC7 family of amino acid transporters consists of fifteen genes and is split into two subgroups: the cationic amino acid transporters (CATs) and the L-type amino acid transporters (LATs) (Verrey et al., 2004; Fotiadis et al., 2013). CATs comprise the SLC7A1-A4 and SLC7A14 genes, and LATs the SLC7A5-A11, Slc7a12, SLC7A13, and Slc7a15 genes (Fotiadis et al., 2013). In contrast to CATs, LATs are not glycosylated. For correct trafficking to the plasma membrane in mammalian cells, LATs associated with type II membrane N-glycoproteins from the SLC3 family, i.e., 4F2hc (SLC3A2; CD98) and rBAT (SLC3A1) (Palacin and Kanai, 2004). These ancillary proteins (the heavy chains) are covalently connected to the corresponding LATs (the light subunits) through a conserved disulfide bridge to form heterodimeric amino acid transporters (HATs) (Chillaron et al., 2001; Wagner et al., 2001; Palacin and Kanai, 2004; Verrey et al., 2004; Fotiadis et al., 2013). The light subunits are the catalytic subunits of HATs (Reig et al., 2002; Rosell et al., 2014; Napolitano et al., 2015).
LAT1 (SLC7A5) and LAT2 (SLC7A8) are isoforms of the system L of amino acid transporters requiring the heavy chain 4F2 (4F2hc) for functional expression at the plasma membrane (Kanai et al., 1998; Pineda et al., 1999; Segawa et al., 1999). Furthermore, we recently showed that 4F2hc can modulate the substrate affinity and specificity of the light chains LAT1 and LAT2 (Kantipudi et al., 2020). In addition to these two LAT specific functions, the ancillary protein 4F2hc has multifunctional roles such as in cell adhesion, cell fusion, integrin signaling and regulation of macrophage activation via galectin-3 (Fenczik et al., 1997; Tsurudome and Ito, 2000; Feral et al., 2005; MacKinnon et al., 2008). 4F2hc-LAT1 is expressed in different tissues and organs (e.g., brain, ovary, placenta and testis), and in relatively high levels at the blood-brain barrier and in several types of tumors (Fotiadis et al., 2013; Scalise et al., 2018; Häfliger and Charles, 2019). The location and high expression levels make 4F2hc-LAT1 an interesting vehicle for drug delivery into the brain and for cancer cell targeting (Häfliger and Charles, 2019; Puris et al., 2020). In cancer cells, 4F2hc-LAT1 provides neutral and essential amino acids for nutrition and regulation of the mTOR signaling pathway (Nicklin et al., 2009). Thus, inhibition of this HAT represents a valid approach to block migration and invasion of cancer cells, and to induce apoptosis. In contrast, 4F2hc-LAT2 is ubiquitously expressed in the human body and highly expressed in polarized epithelia suggesting a major role of this HAT in transepithelial transport of amino acids (Bröer, 2008; Fotiadis et al., 2013). Thus, both transporters have evolved towards specific functions, e.g., LAT1 for uptake of specific amino acids into growing cells, and LAT2 towards normal cell-type and transcellular amino acid transport.
LAT1 and LAT2 are sodium-independent transporters that exchange substrates across membranes with a one-to-one stoichiometry (Verrey et al., 2004; Fotiadis et al., 2013). The substrate specificities of both HATs are comparable, but 4F2hc-LAT2 accepts in addition to large neutral also small neutral amino acids (Pineda et al., 1999; Rossier et al., 1999; Meier et al., 2002). Other substrates of 4F2hc-LAT1 and -LAT2 represent amino acid derivatives such as the thyroid hormones T3 and T4 (Friesema et al., 2001; Zevenbergen et al., 2015). The compound 2-aminobicyclo-(2,2,1)-heptane-2-carboxylic acid (BCH) (Kim et al., 2008) was described as specific inhibitor of system L inhibiting both, 4F2hc-LAT1 and -LAT2 (Kanai et al., 1998; Segawa et al., 1999). On the other hand, the tyrosine-based JPH203 (KYT-0353) molecule was reported as a competitive, potent and highly specific 4F2hc-LAT1 inhibitor with strong inhibitory effects on the growth of different cancer cells (Oda et al., 2010; Yun et al., 2014; Häfliger et al., 2018). Therefore, transport inhibitors with high specificity towards 4F2hc-LAT1 but not -LAT2 represent promising drug candidates for cancer therapy and diagnosis. In crescentic glomerulonephritis pathogenesis, LAT2 was shown to be upregulated activating the mTORC1 pathway (Kurayama et al., 2011). Thus, LAT2-specific inhibitors might also be interesting and considered therapeutically for crescentic glomerulonephritis and other emerging LAT2-related diseases. Towards discovery of potent and selective inhibitors against 4F2hc-LAT1 or -LAT2, robust assays for ligand screening and functional characterization using cells overexpressing corresponding LATs separately are crucial. Establishment of mammalian cell lines for stable expression of 4F2hc-LAT1 and -LAT2 is not straight-forward since most host cell lines express HATs endogenously. As a consequence, the activity of the exogenous LAT is difficult to distinguish from the endogenous one and the limited endogenous pool of 4F2hc is used for both, endogenous and exogenous LATs, thus introducing ambiguities in the assays. Khunweeraphong et al. reported the establishment of stable HEK293 cell lines expressing exogenous LAT1 or LAT2, and using endogenous 4F2hc of the cells to form HATs (Khunweeraphong et al., 2012). HEK293 cells indicated reduced backgrounds of amino acid transport, e.g., reduced contamination of endogenous LAT1 activity (Khunweeraphong et al., 2012), and other advantages compared to the murine S2 cells previously used in a similar endeavor (Morimoto et al., 2008).
The methylotrophic yeast Pichia pastoris represents a well-established system for the expression of recombinant human membrane proteins (Byrne, 2015). Cholesterol and derivatives thereof were shown to play an important role in function and stability of LATs (Meury et al., 2014; Dickens et al., 2017; Cosco et al., 2020). Yeasts such as P. pastoris produce ergosterol (Nes et al., 1978) providing a valuable cholesterol derivate for interaction with heterologously expressed membrane proteins. Towards establishment of a robust assay for ligand screening and functional characterization, we have optimized, applied and validated a previously reported radioligand assay using P. pastoris overexpressing human 4F2hc-LAT1 or -LAT2, and the substrate [3H]L-leucine as radioligand. In contrast to the previously reported HEK293 cell assay (Khunweeraphong et al., 2012), 4F2hc is co-expressed with LAT1 or LAT2 in the Pichia-based assay, thus not limiting 4F2hc availability and boosting expression of HATs (Costa et al., 2013; Rosell et al., 2014; Kantipudi et al., 2020). Interestingly, and in absence of 4F2hc co-expression, Pichia is also able to express functionally the light subunits LAT1 and LAT2 alone (Costa et al., 2013; Rosell et al., 2014; Kantipudi et al., 2020). This allows evaluating possible contributions of the heavy chain 4F2hc on ligand binding and transport inhibition through selected substrates and inhibitors.
Materials and Methods
Cloning and Expression in P. pastoris of Human 4F2hc-LAT1, LAT1, 4F2hc-LAT2 and LAT2
Cloning of the human HATs and LATs into the pPICZB vector (Thermo Fisher Scientific, Waltham, MA, United States), electro-transformation of competent P. pastoris strain KM71H cells (Thermo Fisher Scientific, Waltham, MA, United States) and selection of clones with high protein expression levels was performed as described in detail previously: see for 4F2hc-LAT1 and LAT1 (Kantipudi et al., 2020), and for 4F2hc-LAT2 and LAT2 (Costa et al., 2013). Cell growth and expression conditions of Pichia clones overexpressing human 4F2hc-LAT1, LAT1, 4F2hc-LAT2 or LAT2, and untransformed P. pastoris KM71H cells (control) was conducted according to Kantipudi et al. (Kantipudi et al., 2020). Resulting cells were resuspended in transport buffer (150 mM choline chloride, 1 mM MgCl2, 1 mM CaCl2, 10 mM Tris-HEPES, pH 7.4) containing 50% (v/v) glycerol and the OD600 was adjusted to 40. Cells were stored at −18°C until further use.
[3H]L-Leucine Radioligand Transport Assay
For transport studies, 1 ml of thawed P. pastoris cells (OD600 40) expressing the corresponding transporter were diluted in 50 ml transport buffer and pelleted by centrifugation (3,000 × g, 15 min, room temperature). The pellet was then washed by resuspending it in 50 mL transport buffer and by repeating the washing procedure (centrifugation and resuspension) two times. Finally, the cell pellet was resuspended in 2 ml of transport buffer and incubated for 20 min at 30°C under agitation (300 rpm, Multitron, Infors HT, Bottmingen, Switzerland). The yeast suspension density was adjusted with transport buffer to an OD600 of 1.875 (4F2hc-LAT1, LAT1, 4F2hc-LAT2 or LAT2, and untransformed P. pastoris KM71H cells). All transport experiments were performed in a reaction volume of 100 µL. For time-dependent [3H]L-leucine uptake experiments (Figure 1), the reaction mixture contained 40 µL cell suspension and 60 µL substrate master mix [0.167 µM L-leucine spiked with [3H]L-leucine (American Radiolabeled Chemicals, St. Louis, MO, United States)] with a specific activity of 20 Ci/mmol resulting in a final L-leucine concentration of 0.1 µM. IC50 determinations of selected compounds for the transporters 4F2hc-LAT1, LAT1, 4F2hc-LAT2, or LAT2 were performed using 40 µL cell suspension, 50 µL of competitor solution at different concentrations and 10 µL substrate master mix [1 µM L-leucine spiked with [3H]L-leucine (American Radiolabeled Chemicals, St. Louis, MO, United States)] with a specific activity of 20 Ci/mmol resulting in a final L-leucine concentration of 0.1 µM. For L-leucine and BCH (2-aminobicyclo-(2,2,1)-heptane-2-carboxylic acid) IC50 experiments, the competitor solutions were at concentrations of 0.01−10,000 µM L-leucine (4F2hc-LAT1, LAT1, 4F2hc-LAT2, and LAT2), and 0.25−1,000 µM BCH (4F2hc-LAT1 and LAT1) and 1−5,000 µM BCH (4F2hc-LAT2 and LAT2)–see corresponding graphs in Figures 2, 3 for specific concentrations used. For determining the IC50 values of hydrophobic compounds such as JPH203 [(S)-2-amino-3-(4-((5-amino-2-phenylbenzo [d] oxazol-7-yl)-methoxy)-3,5-dichlorophenyl)-propanoic acid] also known as KYT-0353 (MedChemExpress, Monmouth Junction, NJ, United States), and the thyroid hormones T3 (triiodothyronine) and T4 (thyroxine) (Sigma, St. Louis, MO, United States), 40 µL of cell suspension was incubated with 50 µL of competitor solutions at different concentrations for 60 min at 25°C under agitation (500 rpm, Thermomixer compact, Eppendorf, Hamburg, Germany). The uptake was initiated by adding 10 µL substrate master mix [1 µM L-leucine spiked with [3H]L-leucine (American Radiolabeled Chemicals, St. Louis, MO, United States)] with a specific activity of 20 Ci/mmol to the preincubated 90 µL of reaction volume resulting in a final L-leucine concentration of 0.1 µM. Final ligand concentrations in 100 µL were 0.001−250 µM (JPH203, T3, and T4). The competitors were prepared in 100% (v/v) DMSO. The final DMSO concentration was always 0.5% (v/v), independently of which final substrate or inhibitor concentration was used in the assay. Control samples contained the same concentration of DMSO. Final OD600 values in uptake experiments were 0.75 for all cells (4F2hc-LAT1, LAT1, 4F2hc-LAT2 or LAT2 expressing cells) and untransformed P. pastoris KM71H cells. All transport reactions were done in 2 ml reaction tubes (Eppendorf, Hamburg, Germany) at 25°C under agitation (1,000 rpm, Thermomixer compact, Eppendorf, Hamburg, Germany). Uptakes were terminated after 10 min (uptake time) for all cells by adding 600 µL of pre-chilled transport buffer. Cells were rapidly separated from the buffer by transferring the stopped reactions on a 96-well 0.66 mm glass fiber filter plate (Corning FiltrEX, Corning, NY, United States) and vacuum filtration. Each well was washed with 2 ml of ice-cold transport buffer to remove free radioligand. The plate was then dried overnight at 37°C, and the backside was sealed with a BackSeal (PerkinElmer, Waltham, MA, United States). The trapped radioligand was released by addition of 200 µL scintillation cocktail (MicroScint 40, PerkinElmer, Waltham, MA, United States) to each well, and the plate topside was sealed with TopsealTM-A Plus (PerkinElmer, Waltham, MA, United States), followed by incubation for 30 min at 25°C and 1,000 rpm (Thermomixer compact, Eppendorf, Hamburg, Germany). Counts were measured in each well for 2 min with a scintillation counter (TopCount NXT, PerkinElmer, Waltham, MA, United States).
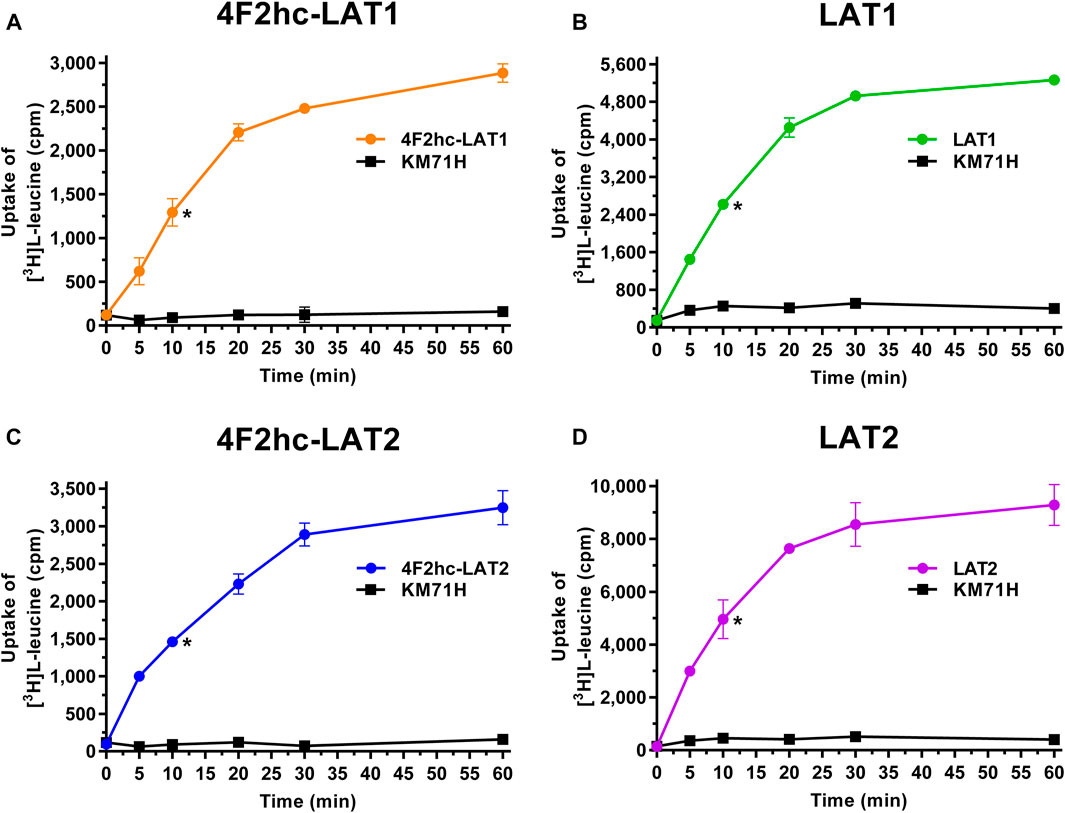
FIGURE 1. Time-dependent uptake of 0.1 µM [3H]L-leucine into P. pastoris KM71H cells expressing 4F2hc-LAT1 [(A); orange], LAT1 [(B); green], 4F2hc-LAT2 [(C); blue], or LAT2 [(D); violet]. [3H]L-leucine uptake into untransformed P. pastoris KM71H cells is shown in black. Asterisks indicate the 10 min time points located in the linear regimes chosen for the subsequently presented transport inhibition experiments (Figures 2–6). Data points are represented as mean ± standard deviation from a representative experiment in triplicate. If not visible, error bars are smaller than symbols.
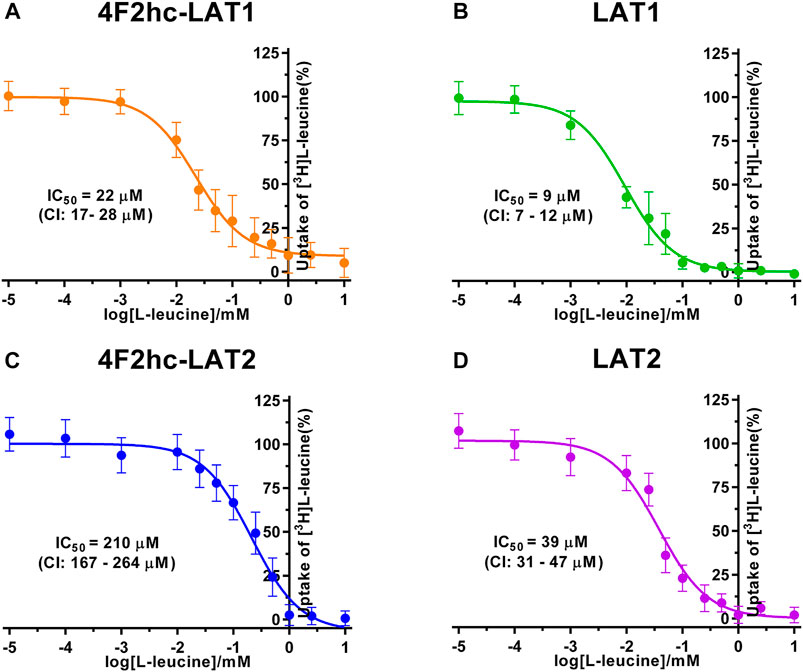
FIGURE 2. IC50 determination of L-leucine for human 4F2hc-LAT1 [(A); orange], LAT1 [(B); green], 4F2hc-LAT2 [(C); blue] and LAT2 [(D); violet] using P. pastoris KM71H cells expressing the corresponding transporter variants. Determined IC50 values and 95% confidence intervals (CIs) are indicated. Mean ± standard deviation of normalized data from three independent experiments, each at least in triplicate, are shown. If not visible, error bars are smaller than symbols.
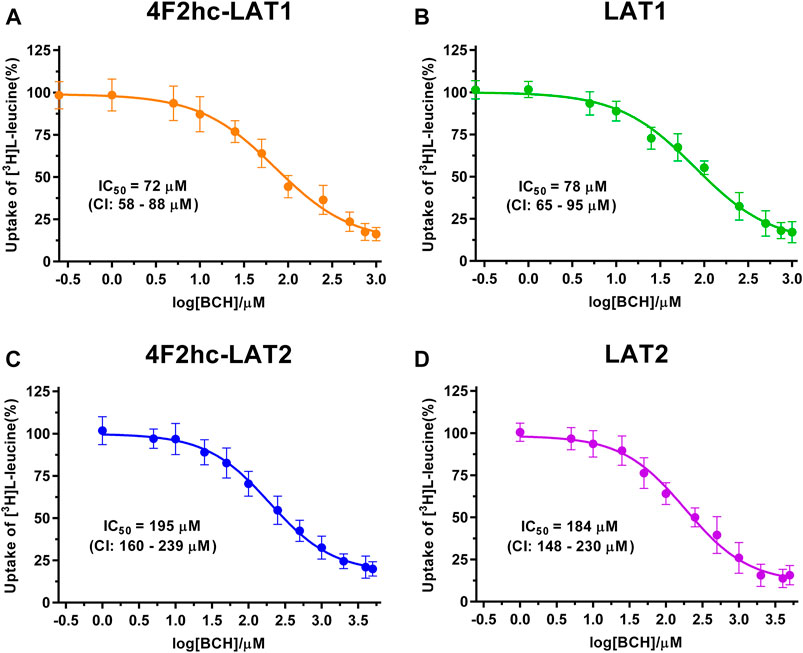
FIGURE 3. IC50 determination of BCH for human 4F2hc-LAT1 [(A); orange], LAT1 [(B); green], 4F2hc-LAT2 [(C); blue] and LAT2 [(D); violet] using P. pastoris KM71H cells expressing the corresponding transporter variants. Determined IC50 values and 95% confidence intervals (CIs) are indicated. Mean ± standard deviation of normalized data from three independent experiments, each at least in triplicate, are shown. If not visible, error bars are smaller than symbols.
Data Analysis, Curve Fitting and Statistics
Experiments were performed at least in triplicate. For data analysis, the signal of the untransformed P. pastoris cells was subtracted from the transporter signal to obtain the net transport signal. Data from three independent experiments were taken. In each of these experiments, the net transport signals were averaged and the half maximal inhibitory concentration (IC50) values of homologous (L-leucine) and heterologous (BCH, JPH203, T3, and T4) L-leucine transport competition experiments were determined by fitting a sigmoidal model curve to these data. Every experimental data point was then individually normalized using the corresponding upper plateau values (i.e., the fitted upper plateau value that corresponds to 100%). Single, normalized data points from the three independent experiments were averaged and a sigmoidal model curve was fitted to the data in order to obtain the IC50 values. Prism6 (GraphPad Software) was used for data analysis.
Results
Human 4F2hc-LAT1, LAT1, 4F2hc-LAT2 or LAT2 were expressed in the methylotrophic yeast P. pastoris. We showed in previous reports that not only the HATs 4F2hc-LAT1 and -LAT2, but also the light subunits LAT1 and LAT2 in absence of ancillary protein are properly folded, correctly trafficked to the plasma membrane and functional in P. pastoris (Rosell et al., 2014; Kantipudi et al., 2020).
Transport activities were determined by measuring the uptake of the substrate [3H]L-leucine into Pichia cells at OD600 0.75 expressing the corresponding HAT or LAT. Time-course experiments showed clear HAT- and LAT-dependent transport activities, which were much higher than the [3H]L-leucine uptake into untransformed host cells (Figure 1). In all cases, saturation of the transport process was observed. Differences in radioligand transport (Figure 1) are due to different expression levels of corresponding recombinant LATs as estimated from Vmax/OD values using previously determined kinetic parameters (Kantipudi et al., 2020). Uptake assay times of 10 min (i.e., in the linear regimes–time points indicated by asterisks in Figure 1) and corresponding Pichia cells at OD600 0.75 were taken for the subsequently presented experiments with 4F2hc-LAT1, LAT1, 4F2hc-LAT2, and LAT2 (Figures 2–6).
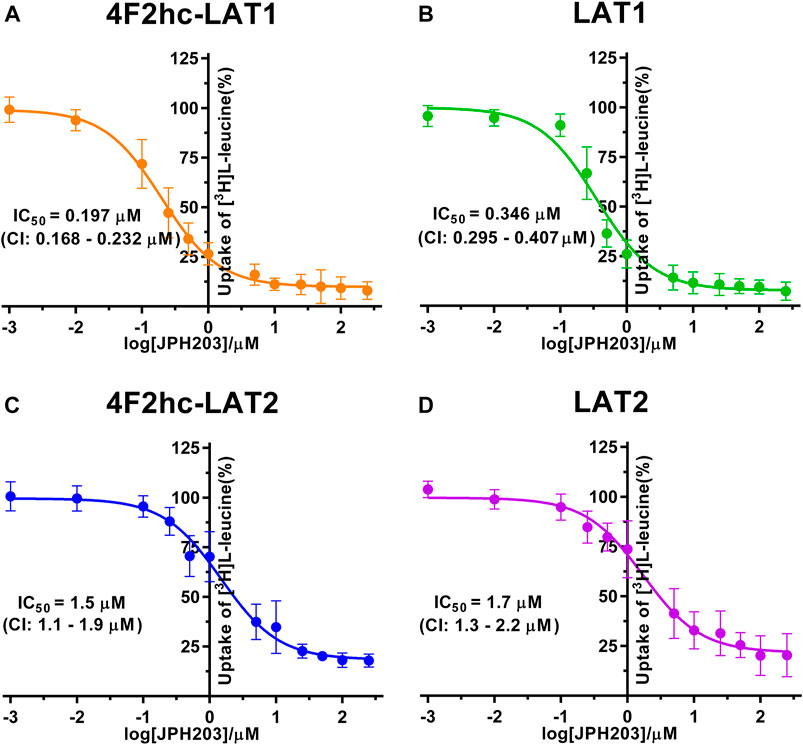
FIGURE 4. IC50 determination of JPH203 for human 4F2hc-LAT1 [(A); orange], LAT1 [(B); green], 4F2hc-LAT2 [(C); blue] and LAT2 [(D); violet] using P. pastoris KM71H cells expressing the corresponding transporter variants. Determined IC50 values and 95% confidence intervals (CIs) are indicated. Mean ± standard deviation of normalized data from three independent experiments, each at least in triplicate, are shown. If not visible, error bars are smaller than symbols.
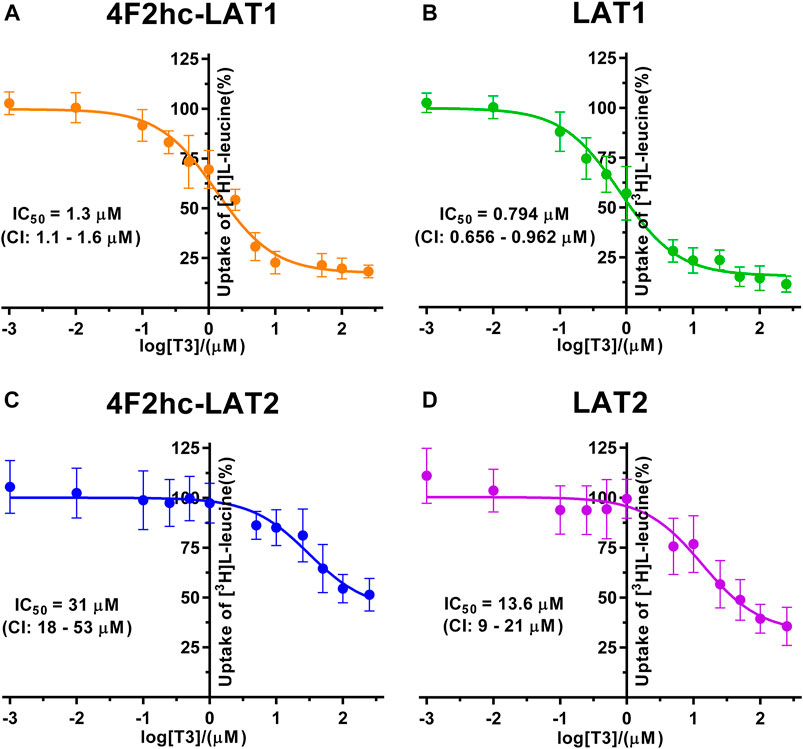
FIGURE 5. IC50 determination of T3 for human 4F2hc-LAT1 [(A); orange], LAT1 [(B); green], 4F2hc-LAT2 [(C); blue] and LAT2 [(D); violet] using P. pastoris KM71H cells expressing the corresponding transporter variants. Determined IC50 values and 95% confidence intervals (CIs) are indicated. Mean ± standard deviation of normalized data from three independent experiments, each at least in triplicate, are shown. If not visible, error bars are smaller than symbols.
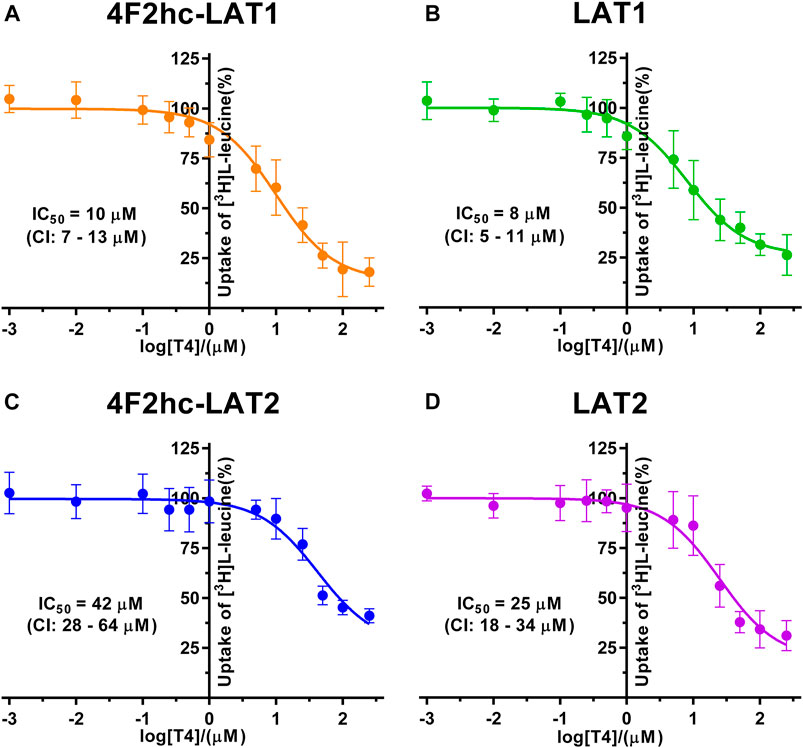
FIGURE 6. IC50 determination of T4 for human 4F2hc-LAT1 [(A); orange], LAT1 [(B); green], 4F2hc-LAT2 [(C); blue] and LAT2 [(D); violet] using P. pastoris KM71H cells expressing the corresponding transporter variants. Determined IC50 values and 95% confidence intervals (CIs) are indicated. Mean ± standard deviation of normalized data from three independent experiments, each at least in triplicate, are shown. If not visible, error bars are smaller than symbols.
We determined the half-maximal inhibitory concentrations (IC50s) of L-leucine by homologous competition for all four transporter variants. Obtained IC50 values were 22 µM (4F2hc-LAT1), 9 µM (LAT1), 210 µM (4F2hc-LAT2), and 39 µM (LAT2) (Figure 2).
As previously reported (Kantipudi et al., 2020), comparison of IC50s indicated a modulatory effect of the heavy subunit 4F2 on light subunits, which is most striking between 4F2hc-LAT2 and LAT2 (Figure 2). After validation of the yeast-cell based transport assay with the substrate L-leucine (Figures 1, 2), we pursued validation using the described system L transport inhibitor BCH (Kim et al., 2008) and the 4F2hc-LAT1 specific inhibitor JPH203 (Oda et al., 2010). We obtained IC50 values of 72 µM (4F2hc-LAT1), 78 µM (LAT1), 195 µM (4F2hc-LAT2), and 184 µM (LAT2) for BCH (Figure 3). These results indicated that the compound BCH inhibits both human HATs being about 2.7-fold more specific for 4F2hc-LAT1. Interestingly, and in contrast to L-leucine (Figure 2), LAT1 and LAT2 in absence of 4F2hc had comparable IC50s as their heterodimeric forms (Figure 3) indicating no significant influence of the ancillary protein on BCH binding to LATs of HATs.
For the second inhibitor, i.e., JPH203, IC50 values of 0.197 µM (4F2hc-LAT1), 0.346 µM (LAT1), 1.5 µM (4F2hc-LAT2) and 1.7 µM (LAT2) were obtained using our yeast cell-based assay (Figure 4). Clearly, the compound JPH203 is with its three-digit nanomolar IC50 value potent and about 7.6-fold more specific for 4F2hc-LAT1 compared with 4F2hc-LAT2. As observed also with BCH, IC50 values of JPH203 were comparable for 4F2hc-LAT1 and LAT1, and 4F2hc-LAT2 and LAT2, indicating no significant effect of the ancillary protein on inhibitor binding.
The thyroid hormones triiodothyronine (T3) and thyroxine (T4) represent substrates of 4F2hc-LAT1 and -LAT2 (Friesema et al., 2001; Zevenbergen et al., 2015). We selected these amino acid derivatives as substrates for further validation of our P. pastoris cell-based transport assay. For triiodothyronine, we obtained IC50 values of 1.3 µM (4F2hc-LAT1), 0.794 µM (LAT1), 31 µM (4F2hc-LAT2), and 13.6 µM (LAT2) (Figure 5). The inhibitory effect of T3 was considerably more pronounced for 4F2hc-LAT1 and LAT1 compared with 4F2hc-LAT2 and LAT2, e.g., about 24-fold lower IC50 value for 4F2hc-LAT1 in comparison with 4F2hc-LAT2.
Finally, we determined the IC50s of the thyroid hormone thyroxine using our yeast cell-based transport assay and obtained IC50 values of 10 µM (4F2hc-LAT1), 8 µM (LAT1), 42 µM (4F2hc-LAT2), and 25 µM (LAT2) (Figure 6). Similar to triiodothyronine, the thyroxine hormone also competed with [3H]L-leucine uptake through 4F2hc-LAT1, LAT1, 4F2hc-LAT2, and LAT2. The transport inhibitory effect and thus specificity of T4 was about four-times higher for 4F2hc-LAT1 compared with 4F2hc-LAT2. A comparable trend was observed for the light subunits LAT1 and LAT2. The presence of the heavy chain 4F2 had no marked or only a weak effect on the IC50 values of 4F2hc-LAT1 (10 µM) and 4F2hc-LAT2 (42 µM) compared to the light subunits LAT1 (8 µM) and LAT1 (25 µM) alone.
Discussion
A cell-based transport assay using the methylotrophic yeast P. pastoris overexpressing the human HATs 4F2hc-LAT1 or -LAT2, and LATs LAT1 or LAT2, and the radiolabeled substrate [3H]L-leucine was optimized and validated using selected substrates and inhibitors. In contrast to our previous study (Kantipudi et al., 2020), we adjusted uptake time and OD600 for the four stably expressing Pichia clones to same conditions, i.e., to 10 min and OD600 0.75 (Figure 1). For comparison and validation of the adjusted uptake conditions, IC50s were determined for the substrate L-leucine (Figure 2)–the here studied transporters have high affinities for L-leucine relative to the other proteinogenic amino acids (Kantipudi et al., 2020). The obtained IC50s for L-leucine (Figure 2) were comparable to previously published values using oocytes (Kanai et al., 1998; Friesema et al., 2001; Yanagida et al., 2001), and P. pastoris cells at OD600 10 (4F2hc-LAT1, 4F2hc-LAT2, and LAT1) and OD600 3 (LAT2), and uptake times of 10 min (4F2hc-LAT1, 4F2hc-LAT2, and LAT1) and 2 min (LAT2) (Kantipudi et al., 2020). The modulatory effect of the heavy chain 4F2 on the L-leucine affinity of light subunits, this being most pronounced for 4F2hc-LAT2 and LAT2 (Figures 2C,D), was also observed in line with our previous report (Kantipudi et al., 2020). Newly, we tested with the P. pastoris cell-based assay, described transport inhibitors, i.e., the system L and 4F2hc-LAT1 uptake inhibitors BCH (Kim et al., 2008) and JPH203 (Oda et al., 2010), respectively. The IC50s of BCH for the two HATs were comparable with values from the literature (Table 1) and almost identical to the IC50s from corresponding LATs in absence of 4F2hc (Figure 3). The latter indicated no contribution of the ancillary protein on BCH binding to light subunits. JPH203 was described as a competitive, potent and highly specific 4F2hc-LAT1 inhibitor (Endou et al., 2008; Oda et al., 2010). The authors of these two studies determined IC50s of 0.14 µM (Oda et al., 2010), and 0.19 and 0.2 µM (Endou et al., 2008) for human LAT1 HATs expressed in S2 cells derived from mouse renal proximal tubules (Morimoto et al., 2008) (Table 1). The IC50 of 0.197 µM for 4F2hc-LAT1 obtained with the here presented assay (Figure 4A) is similar to the values (0.14, 0.19, and 0.2 µM) determined for the same HAT using S2 cells (Table 1). Furthermore, most kinetic parameters for JPH203 and 4F2hc-LAT1 determined using other cell types than S2 cells were also in good agreement with the IC50 value from P. pastoris cells (Table 1). However, a marked difference is found between the IC50 of JPH203 determined for human 4F2hc-LAT2 using the P. pastoris (1.5 µM; Figure 4C) and the S2 cell-based assays (>10 µM (Oda et al., 2010) and almost no inhibition at 10 µM (Endou et al., 2008)). Furthermore, Oda et al. indicated an IC50(S2-LAT2)/IC50(S2-LAT1) ratio of >500, which would be indicative of a high specificity of JPH203 towards human 4F2hc-LAT1 and not 4F2hc-LAT2 (Oda et al., 2010). In strong contrast, the IC50(Pichia-4F2hc-LAT2)/IC50(Pichia-4F2hc-LAT1) ratio was only about 7.6, indicating a significantly more moderate specificity of JPH203 towards 4F2hc-LAT1. This finding was further corroborated independently using Pichia cells expressing the light subunits alone, which yielded a comparable IC50(Pichia-LAT2)/IC50(Pichia-LAT1) ratio of about 4.9. Future studies on the specificity of JPH203 towards 4F2hc-LAT1 and -LAT2 will clarify this ambiguity. For further validation of our yeast cell-based transport assay, we tested amino acid derivatives, specifically the thyroid hormones T3 and T4, which are substrates of 4F2hc-LAT1 and -LAT2 (Friesema et al., 2001; Zevenbergen et al., 2015). For 4F2hc-LAT1, T3 reflected a higher potency in transport inhibition than T4 (Figures 5A, 6A) in line with previous studies (Table 1). No kinetic parameters were found in the literature for T3 and T4 with 4F2hc-LAT2 for comparison. Thus, our IC50 for T3 and T4 represent first 4F2hc-LAT2 and LAT2 specific kinetic values (Figures 5C,D,6C,D). Based on the obtained IC50s, the ancillary protein 4F2hc did not have an important influence on the binding of the two hormones to the light subunits (Figures 5, 6).
Conclusion
We have provided a robust yeast cell-based transport assay for the functional characterization of human 4F2hc-LAT1 and -LAT2, and LAT1 and LAT2 substrates and inhibitors. P. pastoris cells expressing HATs are valuable for determining kinetic parameters of ligands, e.g., potency and selectivity of transport inhibitors towards 4F2hc-LAT1 and -LAT2. Such kinetic information will help evaluating the structure-activity relationship of new ligands. Moreover, assays performed with yeast cells expressing light subunits provide kinetic parameters that, when compared with those of corresponding HATs, are of interest towards identification of possible effects of the ancillary protein 4F2hc on ligand affinity and specificity. First kinetic parameters on T3 and T4 were successfully provided for human 4F2hc-LAT2 and LAT2 using the here presented yeast cell-based transport assay. Finally, the established approach and assay could also be used for compound screening and applied to other HATs and LATs of interest.
Data Availability Statement
The raw data supporting the conclusions of this article will be made available by the authors, without undue reservation.
Author Contributions
DF conceived and designed the study. SK performed the experiments, collected and analyzed the data. SK and DF wrote the manuscript. DF obtained the funding. SK and DF read, and approved the submitted version.
Funding
This research was funded by the University of Bern, the Swiss National Science Foundation and the Swiss National Centre of Competence in Research (NCCR) TransCure.
Conflict of Interest
The authors declare that the research was conducted in the absence of any commercial or financial relationships that could be construed as a potential conflict of interest.
Acknowledgments
We thank Sara Bonetti and Jean-Marc Jeckelmann for valuable feedback on the manuscript.
References
Bröer, S. (2008). Amino Acid Transport across Mammalian Intestinal and Renal Epithelia. Physiol. Rev. 88 (1), 249–286. doi:10.1152/physrev.00018.2006
Byrne, B. (2015). Pichia pastoris as an Expression Host for Membrane Protein Structural Biology. Curr. Opin. Struct. Biol. 32, 9–17. doi:10.1016/j.sbi.2015.01.005
Chillarón, J., Roca, R., Valencia, A., Zorzano, A., and Palacín, M. (2001). Heteromeric Amino Acid Transporters: Biochemistry, Genetics, and Physiology. Am. J. Physiology-Renal Physiol. 281, F995–F1018. doi:10.1152/ajprenal.2001.281.6.f995
Choi, D. W., Kim, D. K., Kanai, Y., Wempe, M. F., Endou, H., and Kim, J.-K. (2017). JPH203, a Selective L-type Amino Acid Transporter 1 Inhibitor, Induces Mitochondria-dependent Apoptosis in Saos2 Human Osteosarcoma Cells. Korean J. Physiol. Pharmacol. 21 (6), 599–607. doi:10.4196/kjpp.2017.21.6.599
Christensen, H. N. (1990). Role of Amino Acid Transport and Countertransport in Nutrition and Metabolism. Physiol. Rev. 70 (1), 43–77. doi:10.1152/physrev.1990.70.1.43
Cosco, J., Scalise, M., Colas, C., Galluccio, M., Martini, R., Rovella, F., et al. (2020). ATP Modulates SLC7A5 (LAT1) Synergistically with Cholesterol. Sci. Rep. 10 (1), 16738. doi:10.1038/s41598-020-73757-y
Costa, M., Rosell, A., Álvarez-Marimon, E., Zorzano, A., Fotiadis, D., and Palacín, M. (2013). Expression of Human Heteromeric Amino Acid Transporters in the Yeast Pichia pastoris. Protein Expr. Purif. 87 (1), 35–40. doi:10.1016/j.pep.2012.10.003
Dickens, D., Chiduza, G. N., Wright, G. S. A., Pirmohamed, M., Antonyuk, S. V., and Hasnain, S. S. (2017). Modulation of LAT1 (SLC7A5) Transporter Activity and Stability by Membrane Cholesterol. Sci. Rep. 7, 43580. doi:10.1038/srep43580
Endou, H., Kanai, Y., Saito, K., and Oda, K. (2008). Aromatic Amino Acid Derivative with LAT1 Inhibitory Activity, LAT1 Inhibitor Containing the Same and Method for Producing the Same. Patent Publication No. WO2008081537 International Application No. PCT/JP2006/326233.
Fenczik, C. A., Sethi, T., Ramos, J. W., Hughes, P. E., and Ginsberg, M. H. (1997). Complementation of Dominant Suppression Implicates CD98 in Integrin Activation. Nature 390 (6655), 81–85. doi:10.1038/36349
Feral, C. C., Nishiya, N., Fenczik, C. A., Stuhlmann, H., Slepak, M., and Ginsberg, M. H. (2005). CD98hc (SLC3A2) Mediates Integrin Signaling. Proc. Natl. Acad. Sci. 102 (2), 355–360. doi:10.1073/pnas.0404852102
Fotiadis, D., Kanai, Y., and Palacín, M. (2013). The SLC3 and SLC7 Families of Amino Acid Transporters. Mol. Aspects Med. 34 (2-3), 139–158. doi:10.1016/j.mam.2012.10.007
Friesema, E. C. H., Docter, R., Moerings, E. P. C. M., Verrey, F., Krenning, E. P., Hennemann, G., et al. (2001). Thyroid Hormone Transport by the Heterodimeric Human System L Amino Acid Transporter. Endocrinology 142 (10), 4339–4348. doi:10.1210/endo.142.10.8418
Häfliger, P., and Charles, R.-P. (2019). The L-type Amino Acid Transporter LAT1-An Emerging Target in Cancer. Ijms 20 (10), 2428. doi:10.3390/ijms20102428
Häfliger, P., Graff, J., Rubin, M., Stooss, A., Dettmer, M. S., Altmann, K.-H., et al. (2018). The LAT1 Inhibitor JPH203 Reduces Growth of Thyroid Carcinoma in a Fully Immunocompetent Mouse Model. J. Exp. Clin. Cancer Res. 37 (1), 234. doi:10.1186/s13046-018-0907-z
Kanai, Y., Segawa, H., Miyamoto, K.-i., Uchino, H., Takeda, E., and Endou, H. (1998). Expression Cloning and Characterization of a Transporter for Large Neutral Amino Acids Activated by the Heavy Chain of 4F2 Antigen (CD98). J. Biol. Chem. 273 (37), 23629–23632. doi:10.1074/jbc.273.37.23629
Kandasamy, P., Gyimesi, G., Kanai, Y., and Hediger, M. A. (2018). Amino Acid Transporters Revisited: New Views in Health and Disease. Trends Biochem. Sci. 43 (10), 752–789. doi:10.1016/j.tibs.2018.05.003
Kantipudi, S., Jeckelmann, J.-M., Ucurum, Z., Bosshart, P. D., and Fotiadis, D. (2020). The Heavy Chain 4F2hc Modulates the Substrate Affinity and Specificity of the Light Chains LAT1 and LAT2. Ijms 21 (20), 7573. doi:10.3390/ijms21207573
Khunweeraphong, N., Nagamori, S., Wiriyasermkul, P., Nishinaka, Y., Wongthai, P., Ohgaki, R., et al. (2012). Establishment of Stable Cell Lines with High Expression of Heterodimers of Human 4F2hc and Human Amino Acid Transporter LAT1 or LAT2 and Delineation of Their Differential Interaction with alpha-Alkyl Moieties. J. Pharmacol. Sci. 119 (4), 368–380. doi:10.1254/jphs.12124fp
Kim, C. S., Cho, S.-H., Chun, H. S., Lee, S.-Y., Endou, H., Kanai, Y., et al. (2008). BCH, an Inhibitor of System L Amino Acid Transporters, Induces Apoptosis in Cancer Cells. Biol. Pharm. Bull. 31 (6), 1096–1100. doi:10.1248/bpb.31.1096
Kurayama, R., Ito, N., Nishibori, Y., Fukuhara, D., Akimoto, Y., Higashihara, E., et al. (2011). Role of Amino Acid Transporter LAT2 in the Activation of mTORC1 Pathway and the Pathogenesis of Crescentic Glomerulonephritis. Lab. Invest. 91 (7), 992–1006. doi:10.1038/labinvest.2011.43
MacKinnon, A. C., Farnworth, S. L., Hodkinson, P. S., Henderson, N. C., Atkinson, K. M., Leffler, H., et al. (2008). Regulation of Alternative Macrophage Activation by Galectin-3. J. Immunol. 180 (4), 2650–2658. doi:10.4049/jimmunol.180.4.2650
McGivan, J. D., and Pastor-Anglada, M. (1994). Regulatory and Molecular Aspects of Mammalian Amino Acid Transport. Biochem. J. 299 (Pt 2), 321–334. doi:10.1042/bj2990321
Meier, C., Ristic, Z., Klauser, S., and Verrey, F. (2002). Activation of System L Heterodimeric Amino Acid Exchangers by Intracellular Substrates. EMBO J. 21 (4), 580–589. doi:10.1093/emboj/21.4.580
Meury, M., Costa, M., Harder, D., Stauffer, M., Jeckelmann, J.-M., Brühlmann, B., et al. (2014). Detergent-induced Stabilization and Improved 3D Map of the Human Heteromeric Amino Acid Transporter 4F2hc-LAT2. PLoS ONE 9, e109882. doi:10.1371/journal.pone.0109882
Morimoto, E., Kanai, Y., Kim, D. K., Chairoungdua, A., Choi, H. W., Wempe, M. F., et al. (2008). Establishment and Characterization of Mammalian Cell Lines Stably Expressing Human L-type Amino Acid Transporters. J. Pharmacol. Sci. 108 (4), 505–516. doi:10.1254/jphs.08232fp
Napolitano, L., Scalise, M., Galluccio, M., Pochini, L., Albanese, L. M., and Indiveri, C. (2015). LAT1 Is the Transport Competent Unit of the LAT1/CD98 Heterodimeric Amino Acid Transporter. Int. J. Biochem. Cell Biol. 67, 25–33. doi:10.1016/j.biocel.2015.08.004
Nes, W. R., Sekula, B. C., Nes, W. D., and Adler, J. H. (1978). The Functional Importance of Structural Features of Ergosterol in Yeast. J. Biol. Chem. 253 (17), 6218–6225. doi:10.1016/s0021-9258(17)34602-1
Nicklin, P., Bergman, P., Zhang, B., Triantafellow, E., Wang, H., Nyfeler, B., et al. (2009). Bidirectional Transport of Amino Acids Regulates mTOR and Autophagy. Cell 136 (3), 521–534. doi:10.1016/j.cell.2008.11.044
Oda, K., Hosoda, N., Endo, H., Saito, K., Tsujihara, K., Yamamura, M., et al. (2010). L-type Amino Acid Transporter 1 Inhibitors Inhibit Tumor Cell Growth. Cancer Sci. 101 (2), 173–179. doi:10.1111/j.1349-7006.2009.01386.x
Palacín, M., and Kanai, Y. (2004). The Ancillary Proteins of HATs: SLC3 Family of Amino Acid Transporters. Pflugers Arch. 447 (5), 490–494. doi:10.1007/s00424-003-1062-7
Pineda, M., Fernández, E., Torrents, D., Estévez, R., López, C., Camps, M., et al. (1999). Identification of a Membrane Protein, LAT-2, that Co-expresses with 4F2 Heavy Chain, an L-type Amino Acid Transport Activity with Broad Specificity for Small and Large Zwitterionic Amino Acids. J. Biol. Chem. 274 (28), 19738–19744. doi:10.1074/jbc.274.28.19738
Puris, E., Gynther, M., Auriola, S., and Huttunen, K. M. (2020). L-Type Amino Acid Transporter 1 as a Target for Drug Delivery. Pharm. Res. 37 (5), 88. doi:10.1007/s11095-020-02826-8
Reig, N., Chillaron, J., Bartoccioni, P., Fernandez, E., Bendahan, A., Zorzano, A., et al. (2002). The Light Subunit of System Bo,+ Is Fully Functional in the Absence of the Heavy Subunit. EMBO J. 21, 4906–4914. doi:10.1093/emboj/cdf500
Ritchie, J., Peter, G., Shi, Y., and Taylor, P. (1999). Thyroid Hormone Transport by 4F2hc-IU12 Heterodimers Expressed in Xenopus Oocytes. J. Endocrinol. 163 (2), R5–R9. doi:10.1677/joe.0.163r005
Rosell, A., Meury, M., Alvarez-Marimon, E., Costa, M., Perez-Cano, L., Zorzano, A., et al. (2014). Structural Bases for the Interaction and Stabilization of the Human Amino Acid Transporter LAT2 with its Ancillary Protein 4F2hc. Proc. Natl. Acad. Sci. 111 (8), 2966–2971. doi:10.1073/pnas.1323779111
Rossier, G., Meier, C., Bauch, C., Summa, V., Sordat, B., Verrey, F., et al. (1999). LAT2, a New Basolateral 4F2hc/CD98-Associated Amino Acid Transporter of Kidney and Intestine. J. Biol. Chem. 274 (49), 34948–34954. doi:10.1074/jbc.274.49.34948
Scalise, M., Galluccio, M., Console, L., Pochini, L., and Indiveri, C. (2018). The Human SLC7A5 (LAT1): The Intriguing Histidine/large Neutral Amino Acid Transporter and its Relevance to Human Health. Front. Chem. 6, 243. doi:10.3389/fchem.2018.00243
Segawa, H., Fukasawa, Y., Miyamoto, K.-i., Takeda, E., Endou, H., and Kanai, Y. (1999). Identification and Functional Characterization of a Na+-independent Neutral Amino Acid Transporter with Broad Substrate Selectivity. J. Biol. Chem. 274 (28), 19745–19751. doi:10.1074/jbc.274.28.19745
Tsurudome, M., and Ito, Y. (2000). Function of Fusion Regulatory Proteins (FRPs) in Immune Cells and Virus-Infected Cells. Crit. Rev. Immunol. 20 (3), 30–196. doi:10.1615/CritRevImmunol.v20.i3.10
Verrey, F., Closs, E. I., Wagner, C. A., Palacin, M., Endou, H., and Kanai, Y. (2004). CATs and HATs: the SLC7 Family of Amino Acid Transporters. Pflugers Archiv Eur. J. Physiol. 447 (5), 532–542. doi:10.1007/s00424-003-1086-z
Wagner, C. A., Lang, F., and Bröer, S. (2001). Function and Structure of Heterodimeric Amino Acid Transporters. Am. J. Physiology-Cell Physiol. 281 (4), C1077–C1093. doi:10.1152/ajpcell.2001.281.4.C1077
Yanagida, O., Kanai, Y., Chairoungdua, A., Kim, D. K., Segawa, H., Nii, T., et al. (2001). Human L-type Amino Acid Transporter 1 (LAT1): Characterization of Function and Expression in Tumor Cell Lines. Biochim. Biophys. Acta (Bba) - Biomembranes 1514, 291–302. doi:10.1016/s0005-2736(01)00384-4
Yothaisong, S., Dokduang, H., Anzai, N., Hayashi, K., Namwat, N., Yongvanit, P., et al. (2017). Inhibition of L-type Amino Acid Transporter 1 Activity as a New Therapeutic Target for Cholangiocarcinoma Treatment. Tumour Biol. 39 (3), 101042831769454. doi:10.1177/1010428317694545
Yun, D.-W., Lee, S. A., Park, M.-G., Kim, J.-S., Yu, S.-K., Park, M.-R., et al. (2014). JPH203, an L-type Amino Acid Transporter 1-selective Compound, Induces Apoptosis of YD-38 Human Oral Cancer Cells. J. Pharmacol. Sci. 124 (2), 208–217. doi:10.1254/jphs.13154fp
Keywords: amino acid transporter, inhibitor, LAT1, LAT2, JPH203, Pichia pastoris, SLC7, Transport Assay
Citation: Kantipudi S and Fotiadis D (2021) Yeast Cell-Based Transport Assay for the Functional Characterization of Human 4F2hc-LAT1 and ‐LAT2, and LAT1 and LAT2 Substrates and Inhibitors. Front. Mol. Biosci. 8:676854. doi: 10.3389/fmolb.2021.676854
Received: 06 March 2021; Accepted: 06 May 2021;
Published: 28 May 2021.
Edited by:
Cesare Indiveri, University of Calabria, ItalyReviewed by:
Manuel Palacin, Institute for Research in Biomedicine, SpainAndreas H. Engel, University of Basel, Switzerland
Copyright © 2021 Kantipudi and Fotiadis. This is an open-access article distributed under the terms of the Creative Commons Attribution License (CC BY). The use, distribution or reproduction in other forums is permitted, provided the original author(s) and the copyright owner(s) are credited and that the original publication in this journal is cited, in accordance with accepted academic practice. No use, distribution or reproduction is permitted which does not comply with these terms.
*Correspondence: Dimitrios Fotiadis, dimitrios.fotiadis@ibmm.unibe.ch