- 1Department of Psychiatry, Firtst Hospital/First Clinical Medical College of Shanxi Medical University, Taiyuan, China
- 2School of Life Science, Shanxi University, Taiyuan, China
- 3Department of Chemistry and Biochemistry, Laurentian University, Sudbury, ON, Canada
Hydrogen sulfide (H2S) is a gasotransmitter along with nitric oxide and carbon oxide, which is involved in plant growth and development as well as biotic and abiotic stress resistance. In a previous study, we reported that mitogen-activated protein kinases, especially MPK4, are important downstream components of H2S involved in alleviating cold stress; however the underlying mechanism is unclear. In this study, we determined that the ability of H2S to alleviate cold stress is impaired in mpk4 mutants, but not in the upstream mek2 and crlk1 mutants. MPK4 was basically persulfidated, and NaHS (H2S donor) further increased the persulfidation level of MPK4. MEK2 was not persulfidated by H2S. NaHS treatments increased the MPK4 activity level nearly tenfold. The persulfidation signal of MPK4 did not disappear after eight cystein residues in MPK4 were site-mutated, respectively. Above all, our results suggested that H2S alleviates cold stress directly by persulfidating MPK4 and increasing the MPK4 kinase activity.
Introduction
Hydrogen sulfide (H2S) is a colorless and combustible gas with an odor similar to that of rotten eggs. H2S is toxic at high concentrations; however, at low concentrations, H2S plays important roles in both animals and plants as a gasotransmitter, along with nitric oxide and carbon oxide (Wang, 2012; Wang, 2014). H2S participates in the plant growth and development, as well as biotic and abiotic stress responses, including those to cold and drought. In proteins, H2S modifies cysteines (Cys) by persulfidation, which alters the -SH of Cys to -SSH, to regulate the activity or cell location of a protein. Persulfidation was first reported in mice, in which about 10%–25% of liver proteins, including glyceraldehyde-3-phosphate dehydrogenase (GAPDH), actin and tubulin, are persulfidated under physiological conditions. Persulfidation increases the activtiy of GAPDH and actin polymerization (Mustafa et al., 2009). In plants, there are also reports of persulfidated proteins (Aroca et al., 2015; Aroca et al., 2017). Aroca et al. reported that 2015 proteins in Arabidopsis seedlings were persulfidation. They accounted for 5% of the total proteins that participate in important plant processes, such as carbon metabolism, responses to abiotic and biotic stresses, growth and development, and RNA translation (Aroca et al., 2017).
Mitogen-activated protein kinases (MAPKs) are crucial components of cell signaling transduction in eukaryotes. MAPKs consist of three cascades: MEKK, MEK and MPK which phosphorylate and thereby activated next cascade respectively. When the cell membrane received the signal from outside, the receptor on the membrane was activated firstly, then MEKK→MEK→MPK cascades was activated, MPK activated downstream target such as enzymes, transcription factor leading to cell response (Šamajová et al., 2013). MAPK is involved in a wide range of physiological processes in plants including growth and development, and biotic and abiotic stress responses. MEKK1-MEK2-MPK4 plays a positive role in cold- and salt-stress responses in Arabidopsis (Teige et al., 2004). CRLK1, a calcium/calmodulin-regulated receptor-like kinase located in the plasma membrane, is the upstream of this cascade and interacts with MEKK1. Consequently, MEKK1 is phosphorylated when CRLK1 is activated by calcium signals under cold-stress conditions (Yang et al., 2010a; Yang et al., 2010b; Furuya et al., 2013).
Cold stress is one of the common environmental stresses for plants, and has many adverse effects on plant, which affects the physiological function of plant cell, restricts plant growth and development, and even causes plant death. It is meaningful to study how plants respond to cold stress (Ding et al., 2019). H2S plays important role in plant response to cold stress. In a previous study we found that MAPKs especially MPK4 are important downstream components in alleviating cold stress. MPK4 is required for H2S to alleviate damage to roots caused by cold stresses and regulate stomatal movement. However, the mechanisms underlying these functions remain unknown (Du et al., 2019). Here, we first studied the ability of H2S alleviate cold stress in mpk4, mek2 and crlk1 mutants using root-tip bending experiments. The effects of H2S on the persulfidation of MEK2 and MPK4 are assessed by biotin-switch assays revealed that MPK4, not MEK2, is persulfidated by H2S. Consequently, we determined the effects of H2S on the kinase activity of MPK4. Finally we investigated the persulfidation signal of Cys-site mutanted MPK4 to determine which Cys residues are important for persulfidation.
Materials and Methods
Plant Materials and Growth Conditions
A Ds insertion mutant of mpk4 of Landsberg background (Ler) was kindly provided by John Mundy of Copenhagen University, in which Ds was integrated eight nucleotides upstream of the first intron of MPK4, and the expression of MPK4 was not detectable (Petersen et al., 2000). A T-DNA insertion mutants of mek2 (Garlic-511-H01.b.1a.Lb3Fa) of Columbia background (Col) (Teige et al., 2004) was provided by Markus Teige of Vienna University. A T-DNA insertion mutants of crlk1 (SALK-016240C) of Col (Yang et al., 2010a) was bought from ABRC. Wild type (WT) Arabidopsis of Ler and Col were used as control, respectively. The primers for genotyping are listed in Supplementary Table S1.
The growth conditions were described previously (Jin et al., 2011). Briefly, the seeds were grown in the pots containing a 1:1:1 soil:perlite:vermiculite (v:v:v) mixture or on the plates containing 1/2 Murashige and Skoog (MS) medium after been sterilizing with 75% ethyl alcohol and 6% NaClO. The seedlings were grown at 23°C, with 60% relative humidity and a 16/8 h (light/dark) photoperiod with 160 μEmm−2s−1 light illumination.
Root Tip-Bending Experiment
The root tip-bending experiments were performed as previously described (Du et al., 2017). Briefly, the seedlings were grown on 1/2 MS plates for 7 days. The treatment group was fumigated with 5 μM NaHS (H2S donor), while the control group was not fumigated. Then, the seedlings were transferred to new 1/2 MS plates and put into cold (4°C) or normal (23°C) conditions vertically and inversely. All the growth condition parameters of growth except temperature were the same.
Molecular Cloning and Construction of Expression Vectors
The CDS of MEK2 was PCR amplified and cloned downstream of a GST tag using EcoRI and XhoI restriction site in pGEX4T1. The CDS of MPK4 was PCR amplified and cloned downstream of a His6 tag using NheI and BamHI restriction sites in pET28a. For site-mutated MPK4 and MEK2EE, primers with corresponding mutated sites (PM) were designed, PM/PF (forward primer of CDS) and PM/PR (reverse primer of CDS) were used to generate the mutant PCR fragment using the pET28a-MPK4 or pGEX4T1-MEK2 plasmid as the template. It was important to determine whether to use PM or its complement. Then, two PCR products were used as templates to generate the mutated MPK4 or MEK2EE using the PF/PR as primers. Cystein was substituted with alanine in MPK4, and MEK2EE were generated by changing both putative phosphorylation sites to glutamate residues (T220E and T226E), which resulted in a constitutively active kinase. The vectors were transformed into Escherichia coli DH5α competent cells, and the sequences were confirmed by sequencing. The primers used are listed in Supplementary Table S1
Recombinant Protein Expression and Detection
pGEX4T1-MEK2 and pGEX4T1-MEK2EE were transformed into E. coli strain JM109. pET28a-MPK4 and its Cys-mutant vector were transformed into E. coli strain BL21. A single clone was incubated in 5 ml LB liquid medium supplemented with the appropriate antibiotics overnight at 37°C. Then, 2 ml solution was transferred into 100 ml LB liquid medium supplemented with appropriate antibiotics and incubated approxiamately 2.5 h at 37°C until the OD600 was 0.6–0.8. Then, 0.1 mM isopropyl β-D-1-thiogalactropyranoside was added to induce protein expression, and the E. coli cultures harboring MEK2 and MPK4 were incubated approximately 20 h at 28°C and 16°C, respectively. MEK2 and MEK2EE were purified using GST Selfinose resin and the MPK4 and Cys-mutant MPK4 were purified using Ni-NTA Sefinose resin. The purified protein was mixed with loading buffer containing β-mercaptoethanol and boiled for 10 min. 10% SDS-PAGE gel electrophoresis was performed and stained the gel with Coomassie Brilliant Blue.
Biotin-Switch Assay
The methods were described previously (Mustafa et al., 2009). Briefly, 0.2 mg purified protein was treated with 0-2000 μM NaHS as indicated in the legend at 4°C for 30 min. Then, acetone was added, and samples were incubated −20°C for 20 min to precipitate the proteins. The proteins were resuspended in 100 μL HEN buffer (250 mM Hepes-NaOH, pH 7.7, 1 mM EDTA and 0.1 mM neocuproine) after acetone was removed. Then 400 μL 20 mM methyl methanethiosulfonate (MMTS) buffer, which had been dissolved with HEN buffer with 2.5% SDS, were added, and samples were incubated at 50°C for 25 min with frequent vortexing to block free -SH. Acetone was added, and the samples were placed at −20°C for 20 min to remove the MMTS and precipitate the proteins. The 100 μL HEN buffer with 1% SDS was used to resuspend the proteins, followed by the addition of 30 μL 2 mM biotin-HDPD in dimethyl sulfoxide to label the -SSH of Cys. After incubation for 3 h at 25°C, acetone was added to remove the biotin-HDPD and precipitated the proteins. Proteins were resuspended in HEN buffer and loading buffer without β-mercaptoethanol was added. After boiling for 10min, the samples were subjected to 10% SDS-PAGE gel electrophoresis, in which Selstain SDS-PAGE gel was used. Protein bands could be observed under UV irradiation in Selstain SDS-PAGE gel and served as an indication of loading. Then the proteins were transferred to nitrocellulose membrane, and subjected to Western blotting. A biotin antibody was used in Western blotting. The persulfidation to load ratio was then densitometrically analyzed with the software ImageJ.
Kinase Activity Assay
His-MPK4 (0.2 μg) was activated by incubating with MEK2EE (0.05 μg) in a reaction buffer (20 mM Tris-HCl, pH 7.5, 10 mM MgCl2, and 1 mM DTT) containing 50 μM ATP at room temperature for 0.5 h. Activated MPK4 was treated with NaHS at 4°C for 30 min and then incubated with MBP (1:10 enzyme substrate ration) in the reaction buffer containing 50 μM ATP at room temperature for 0.5 h. The control was not treated with NaHS. The MPK4 activity was calculated based on the conversion of ATP to ADP using an ADP-Glo Kinase Assay (Promega).
Statistical Analysis
Data are presented as the means ± standard error (SE). The determination of significant differences was performed with SPSS version 17.0 (SPSS, IBM, Chicago, IL, United States) using one way analysis of variance (ANOVA) followed by Duncan’s test or student’t-test as indicated in the figure legends. Different letters and asterisks represented significant differences among treatments. At least three independent experiments were performed.
Results
H2S Alleviates Cold Stress in an MEK2-Independent and MPK4-Dependent Way
In a previous study, we found that the function of H2S’s alleviation of cold stress requires MPK4. To determine whether H2S functions directly through MPK4 or MEK2, the cascade upstream of MPK4, we performed root tip-bending experiments using homozygous mek2 (Col) and mpk4 (Ler) seedlings (Supplementary Figure S1), and WT (Col) and WT (Ler) were used as control, respectively. Cold stress inhibits the growth of roots of WT, mek2 and mpk4 seedlings. NaHS pre-treatments alleviated the impairment of root growth caused by cold stress in WT and mek2 seedlings, but not in mpk4 seedlings (Figure 1).
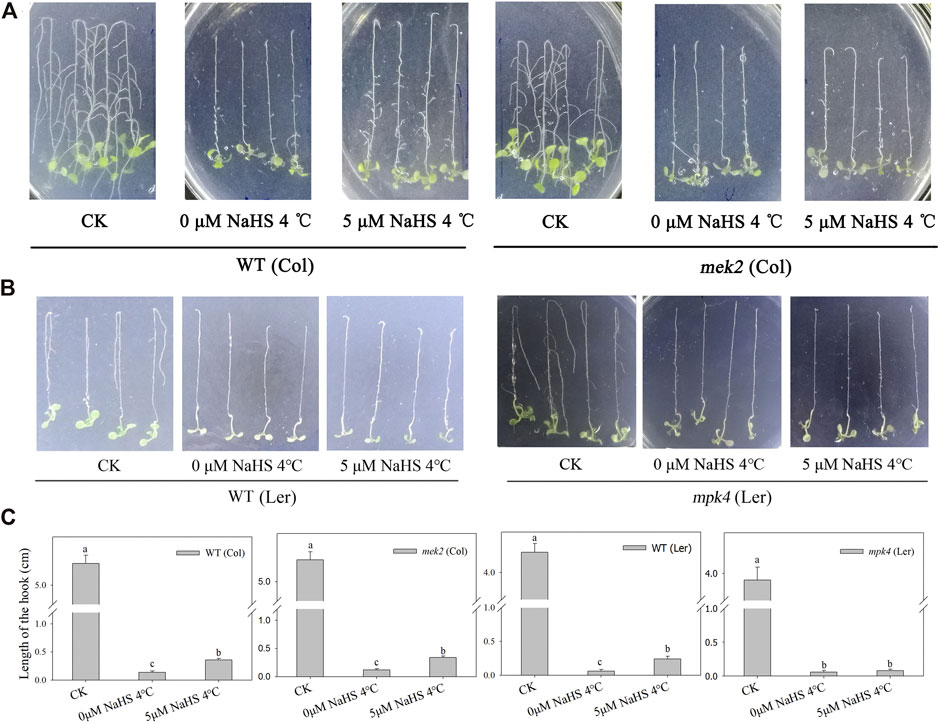
FIGURE 1. The alleviation effect of H2S in WT and mek2(A), and mpk4(B) under cold stress. (C). the quantitative analysis of hook of root bending in a and b. 1-week-old seedlings that grown on the 1/2 MS plates were fumigated by 5 μM NaHS for 12 h or not before transferred to 4°C inverserly. CK was still grown in 23°C inversely. The hook of root tip bending was observed 4 days later. Data are Mean ± SE and three independent experiment were repeated, different letters in c indicated significant differences among treatments (one way ANOVA p < 0.05).
CRLK1-MEKK1-MEK2-MPK4 is a known cascade in cold-signal transduction, and we obtained homozygous crlk1 (Col) seeds (Supplementary Figure S1) and performed root tip-bending experiements to confirm the targets of H2S—mediated cold stress alleviation. NaHS alleviated the damage caused by 4°C treatments in both WT and crlk1 (Col) seedlings (Supplementary Figure S2).
MPK4, but not MEK2, is Persulfidated
H2S modifies the proteins through persulfidation to regulate the proteins’ function. Consequently, we investigated the persulfidation of MPK4 and MEK2. Firstly, we cloned the CDS sequence of MPK4 into the pET28a vector, purified recombinant His-tagged MPK4 (41.36 kD, Supplementary Figure S3) and performed biotin-switch assays. MPK4 was basically persulfidated and NaHS treatments further increased MPK4 persulfidation (Figure 2A).
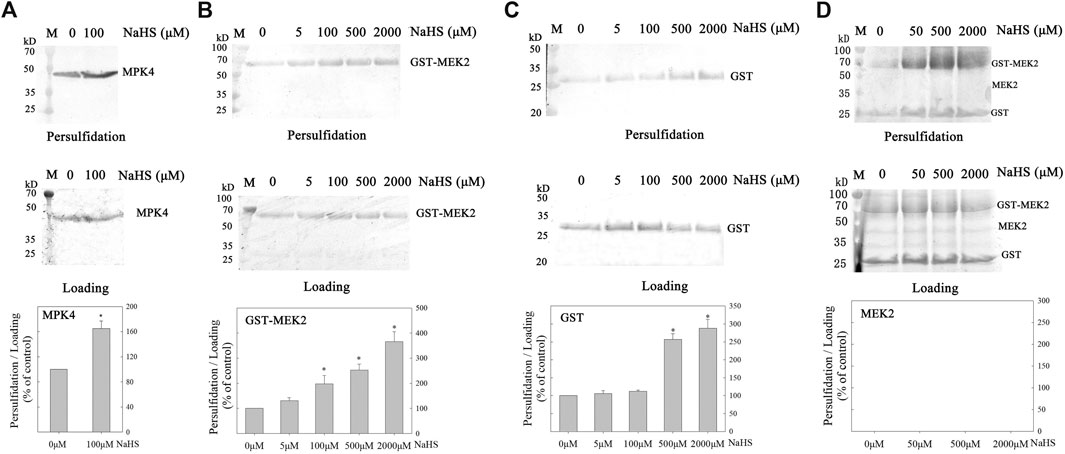
FIGURE 2. The effect of H2S on persulfidation of MPK4 and MEK2. The effect of H2S on persulfidation of MPK4 (A), GST-MEK2 (B), GST (C) and MEK2 (D). MPK4, GST-MEK2, GST and MEK2 were incubated with NaHS of different concentration as indicated in the Figure in 4°C for 30 min, and subjected to biotin-switch assay, in which 10% SDS-PAGE gel and biotin antibody were used. Quantification anlysis was performed using ImageJ. Data are Mean ± SE, and three independent experiment were repeated. *indicated significant differences compared with control (Student’ t-test, p < 0.05). M: Marker.
Then, we constructed the GST-tagged MEK2 cDNA plasmid pGEX4T1-MEK2 (Supplementary Figure S4A) and transfected it into E. coil from which recombinant GST-MEK2 was purified (65.93 kD, Supplementary Figure S4B). The GST-MEK2 was subjected to a biotin-switch assay. The NaHS treatment significantly increased the persulfidation level of GST-MEK2, and NaHS induced GST-MEK2 persulfidation in a concentration-dependent manner (Figure 2B). Because GST contains Cys residues, we then detected the persulfidation level of GST (26 kD) and found that it was also persulfidated by NaHS (Figure 2C). To exclude interference by the GST-tag, we digested the GST-MEK2 with Thrombin, which separates GST from MEK2, leaving the MEK2 protein (39.93 kD, Supplementary Figure S4B). Then, the persulfidation level of MEK2 was analyzed. MEK2 itselft didn’t show persulfidation signals and the NaHS treatment did not increase the persulfidation of MEK2 (Figure 2D).
H2S Induces MPK4 Activity
Then, we determined the role of H2S in the MPK4 activity using ADP-Glo agent, which allowed the kinase activity to be indicated by the ATP-ADP conversion ratio. The ADP produced in the kinase reaction increased after 5 μM and 100 μM NaHS treatments, indicating that the MPK4 kinase activity level increased after the NaHS treatment (Figure 3).
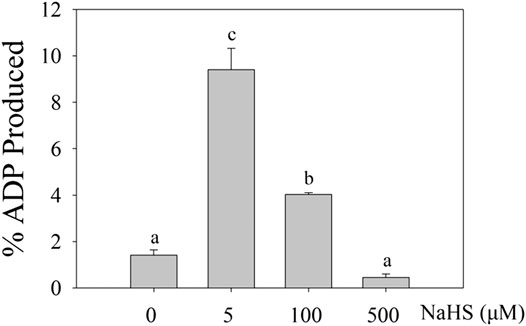
FIGURE 3. The effect of H2S on the activity of MPK4. The 0.2 μg purified MPK4 was first activated by 0.05 μg purified MEK2EE, then treated with 0 μM, 5 μM, 100μM and 500 μM NaHS in 4°C for 30 min. Activated MPK4 was incubated with MBP (1:10 enzyme substrate ration), and the activity of MPK4 was calculated by the conversion of ATP to ADP using ADP-Glo Kinase Assay (Promega). Data are Mean ± SE and three independent experiments were repeated, different letters indicated significant differences among treatments (one way ANOVA p < 0.05).
The Persulfidation of MPK4 did not Disappeared After Cys Residues was Mutated
Persulfidation modifications occur on the Cys residues, therefore, we mutated Cys residues in MPK4 to determine the important Cys residues involved in the persulfidation of MPK4. There are eight Cys residues in MPK4 (at the 6th, 58th, 146th, 181st, 218th, 232nd, 325th, and 341st positions), and all of them were site-mutated to alanine, respectively. Then, the biotin-switch assay was performed. However, the persulfidation signal was detected in all eight Cys residues in MPK4, which had no significant difference with WT MPK4 (Figure 4).
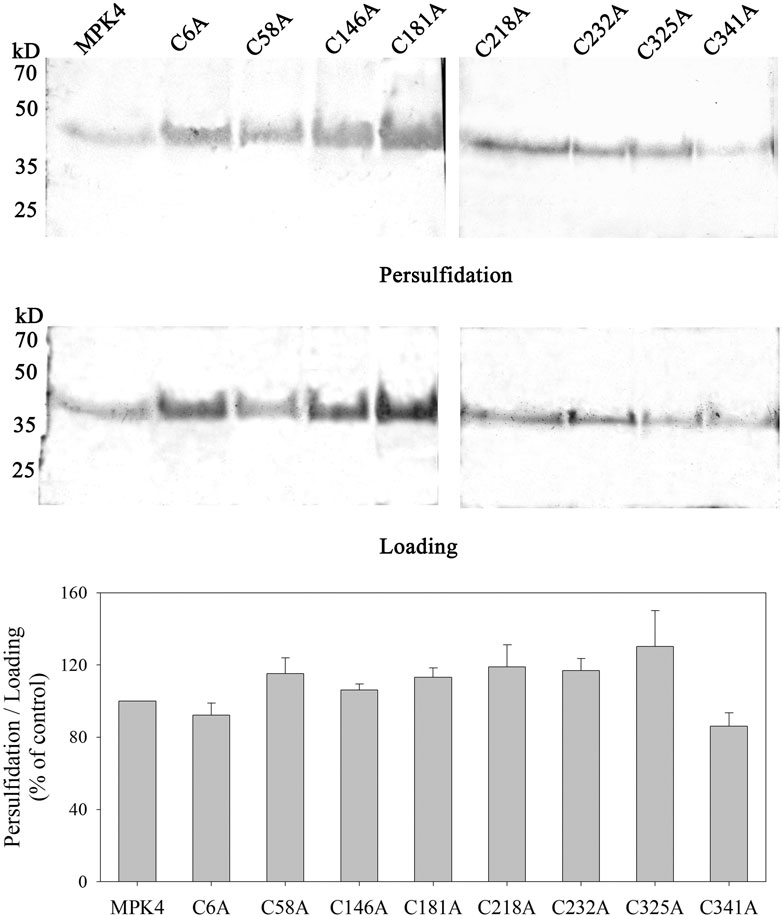
FIGURE 4. Persulfidation signal of MPK4 with and without Cys mutation. The eight Cys residues in MPK4 were site-mutated to alanine including cysteine 6, 58, 146, 181, 218, 232, 325 and 341, WT MPK4 protein and site-mutated MPK4 proteins were subjected to biotin-switch assay, in which 10% SDS-PAGE gel and biotin antibody were used. Quantification anlysis was performed using ImageJ. Data are Mean ± SE, and three independent experiment were repeated. The significance of difference was analyzed by student’ t-test. M: Marker.
Discussion
As a gas signal molecule, H2S has many physiological functions, such as promoting seed germination (Li et al., 2012), fruit ripening (Muñoz-Vargas et al., 2018), lateral root formation (Mei et al., 2017) and participating in stress response. H2S persulfidates proteins to regulate their functions. Chen et al. reported that H2S positively regulates abscisic acid signaling through persulfidation of SnRK2.6 in guard cells (Chen et al., 2020). Shen et al. reported that H2S persulfidated NADPH oxidase enhancing its ability to produce reactive oxygen species (Shen et al., 2020). Aroca et al. reported that there were 2015 persulfidated proteins, including MPK4, in 30-day-old Arabidopsis WT seedlings (Aroca et al., 2017). In our previous studies, we reported that H2S alleviates cold stress through MAPK signals, especially those of MPK4, in Arabidopsis, however, the underlying mechanism remain elusive. In this study, we explored whether MPK4 is the direct target of H2S in the alleviation of cold stress and whether this involves the persulfidation of MAPK.
The important roles of MAPKs in H2S’s physiological functions have been reported in several studies. The expression level of TaMPK4 increased after NaCl + H2S treatment compared with NaCl alone in wheat seedlings (Guo et al., 2019). The MAPK expression level was up-regulated by H2S treatment, while it decreased in the presence of an H2S biosynthesis inhibitor or H2S scavenger in cucumber roots under nitrate-stress conditions (Qi et al., 2019). Here, we used root tip-bending experiments to study the roles of MEK2 and MPK4 in the H2S cold-stress alleviation process, and the results showed that the alleviation required MPK4 but not MEK2 (Figure 1). As shown in Supplementary Figure S2 the ability of H2S to alleviate cold stress was not impaired in crlk1, suggesting that CRLK1 is not required. The results indicated that MPK4 is the target of H2S in the process of H2S alleviating the damage caused by cold stress.
The physiological range of H2S was estimated to be 10–100 μM in health animals and humans (Wang, 2012), therefore we treated the purified-protein of MPK4 and MEK2 with NaHS at 0–100 μM and higher concentration to detect the persulfidation of H2S to the MPK4 and MEK2. The results showed that MPK4 was basically persulfidated, and the 100 μM NaHS treatment increased the MPK4 persulfidation level (Figure 2A). MEK2 is an upstream component of MPK4 in cold-signaling transduction, and MEK2 was not persulfidated (Figure 2). Thus, MPK4, not MEK2, is persulfidated by H2S, and MPK4 is the direct target of H2S-mediated persulfidation. Our results showed that MPK4, GST-MEK2 and GST were basically persulfidated. Endogenous persulfidation can be observed in many proteins without H2S treatment, and H2S treatment increase the persulfidation level, such as GADPH, Tublin in HEK293 cell (Mustafa et al., 2009), MEK1 in human endothelial cells (Zhao et al., 2014), and RBOHD in Arabidopsis (Shen et al., 2020). We infer that there are multiple Cys residues in the protein, and some of them are persulfidation without H2S treatment. H2S treatment can persulfidate the Cys at a specific site therefore increase the persulfidation level of the protein.
Zhao et al. reported that H2S persulfidated MEK1; therefore, it increased the acitvity of MEK1, leading to PARP-1 activation, which attenuates DNA damage (Zhao et al., 2014). The effects of H2S on the activities of other MAPK members have not been reported in plants. Here, our result showed that H2S increased the MPK4 kinase acitivity (Figure 3).
We also investigated the Cys sites that were persulfidated in MPK4. Thus, we mutated the Cys residues in MPK4 and performed biotin switch assays using Cys-mutated MPK4. The persulfidation level of MPK4 did not disappeared after the Cys residues were mutated (Figure 4). In most reports, the persulfidation occurred on a single Cys residue. For example, H2S persulfidated MEK1 at Cys residue 341 (Zhao et al., 2014), H2S persulfidated pyruvate carboxylase at Cys265 (Ju et al., 2015), and mutations of Cys341 and Cys265 abolished the basal MEK1 and pyruvate carboxylase activity levels, respectively. Recently, however, more and more reports have found that persulfidation occurs on multiple Cys residues in a protein. Saha et al. reported that of the 11 Cys in specificity protein 1 (SP1), two were poteintial sites of persulfidation: Cys68 and Cys755 (Saha et al., 2016). Shen et al. reported that CysC44 and Cys205 were persulfiated in L-CYSTEINE DESULFHYDRASE1 (DES1) upon NaHS treatment, and RESPIRATORY BURST OXIDASE HOMOLOG PROTEIN D (RBOHD) is specific persulfidated at both Cys825 and Cys890 (Shen et al., 2020). Thus, the persulfidation modification may occur on more than one Cys in MPK4.
In this study, we investigated the functions of H2S in cold stress and explored the underlying mechanisms. We found that H2S alleviates cold stress through MPK4, not MEK2 or CRLK1, and that H2S increases the MPK4 persulfidation and improve the kinase activity level. However, we did not identify the Cys sites of persulfidation in MPK4, possibly because the persulfidation of MPK4 occurred on two or more Cys residues. In future studies, we will analyze the protein structure of MPK4 and use mass spectrometry to investigate this subject, which will be a very interesting study.
Data Availability Statement
The raw data supporting the conclusions of this article will be made available by the authors, without undue reservation.
Author Contributions
XD, YP, and GY designed the experiments and wrote the manuscript. XD performed the experiments. YG constructed the pET28a-MPK4 vector. XD, YP, ZJ, ZL, DL, LZ, XM, SL, and YG analyzed the data. XD, YP, ZJ, ZL, DL, LZ, XM, GY, SL, and YG revised the manuscript. All authors approved the final manuscript and agreed to be accountable for all aspects of the work.
Funding
This work was funded by the National Natural Science Foundation of China (31972428), Foundation of China Scholarship Council (2020-1417) and the Shanxi Province Natural Science Foundation (201801D121191 and 201801D121197).
Conflict of Interest
The authors declare that the research was conducted in the absence of any commercial or financial relationships that could be construed as a potential conflict of interest.
Supplementary Material
The Supplementary Material for this article can be found online at: https://www.frontiersin.org/articles/10.3389/fmolb.2021.635470/full#supplementary-material.
References
Aroca, A., Benito, J. M., Gotor, C., and Romero, L. C. (2017). Persulfidation proteome reveals the regulation of protein function by hydrogen sulfide in diverse biological processes in Arabidopsis. J. Exp. Bot. 68, 4915–4927. doi:10.1093/jxb/erx294 |
Aroca, Á., Serna, A., Gotor, C., and Romero, L. C. (2015). S-sulfhydration: a cysteine posttranslational modification in plant systems. Plant Physiol. 168, 334–342. doi:10.1104/pp.15.00009 |
Chen, S., Jia, H., Wang, X., Shi, C., Wang, X., Ma, P., et al. (2020). Hydrogen sulfide positively regulates abscisic acid signaling through persulfidation of SnRK2.6 in guard cells. Mol. Plant 13, 732–744. doi:10.1016/j.molp.2020.01.004 |
Ding, Y., Shi, Y., and Yang, S. (2019). Advances and challenges in uncovering cold tolerance regulatory mechanisms in plants. New Phytol. 222, 1690–1704. doi:10.1111/nph.15696 |
Du, X., Jin, Z., Liu, D., Yang, G., and Pei, Y. (2017). Hydrogen sulfide alleviates the cold stress through MPK4 in Arabidopsis thaliana. Plant Physiol. Biochem. 120, 112–119. doi:10.1016/j.plaphy.2017.09.028 |
Du, X., Jin, Z., Zhang, L., Liu, X., Yang, G., and Pei, Y. (2019). H2S is involved in ABA-mediated stomatal movement through MPK4 to alleviate drought stress in Arabidopsis thaliana. Plant Soil 435, 295–307. doi:10.1007/s11104-018-3894-0
Furuya, T., Matsuoka, D., and Nanmori, T. (2013). Phosphorylation of Arabidopsis thaliana MEKK1 via Ca(2+) signaling as a part of the cold stress response. J. Plant Res. 126, 833–840. doi:10.1007/s10265-013-0576-0 |
Guo, T., Huina, D., Dongyun, M., Xin, H., Junfeng, H., Chenyang, W., et al. (2019). Exogenous hydrogen sulfde alleviates salt stress by improving antioxidant defenses and the salt overly sensitive pathway in wheat seedlings. Acta Physiol. Plant 41, 123. doi:10.1007/s11738-019-2918-6
Jin, Z., Shen, J., Qiao, Z., Yang, G., Wang, R., and Pei, Y. (2011). Hydrogen sulfide improves drought resistance in Arabidopsis thaliana. Biochem. Biophys. Res. Commun. 414, 481–486. doi:10.1016/j.bbrc.2011.09.090 |
Ju, Y., Untereiner, A., Wu, L., and Yang, G. (2015). H2S-induced S-sulfhydration of pyruvate carboxylase contributes to gluconeogenesis in liver cells. Biochim. Biophys. Acta. 1850, 2293–2303. doi:10.1016/j.bbagen.2015.08.003 |
Li, Z.-G., Gong, M., and Liu, P. (2012). Hydrogen sulfide is a mediator in H2O2-induced seed germination in Jatropha Curcas. Acta Physiol. Plant 34, 2207–2213. doi:10.1007/s11738-012-1021-z
Mei, Y., Chen, H., Shen, W., Shen, W., and Huang, L. (2017). Hydrogen peroxide is involved in hydrogen sulfide-induced lateral root formation in tomato seedlings. BMC Plant Biol. 17 (1), 162. doi:10.1186/s12870-017-1110-7 |
Muñoz-Vargas, M. A., González-Gordo, S., Cañas, A., López-Jaramillo, J., Palma, J. M., Corpas, F. J., et al. (2018). Endogenous hydrogen sulfide (H2S) is up-regulated during sweet pepper (Capsicum annuum L.) fruit ripening. In vitro analysis shows that NADP-dependent isocitrate dehydrogenase (ICDH) activity is inhibited by H2S and NO. Nitric Oxide 81, 36–45. doi:10.1016/j.niox.2018.10.002 |
Mustafa, A. K., Gadalla, M. M., Sen, N., Kim, S., Mu, W., Gazi, S. K., et al. (2009). H2S signals through protein S-sulfhydration. Sci. Signal 2, ra72. doi:10.1126/scisignal.2000464 |
Petersen, M., Brodersen, P., Naested, H., Andreasson, E., Lindhart, U., Johansen, B., et al. (2000). Arabidopsis map kinase 4 negatively regulates systemic acquired resistance. Cell 103, 1111–1120. doi:10.1016/s0092-8674(00)00213-0 |
Qi, Q., Guo, Z., Liang, Y., Li, K., and Xu, H. (2019). Hydrogen sulfide alleviates oxidative damage under excess nitrate stress through MAPK/NO signaling in cucumber. Plant Physiol. Biochem. 135, 1–8. doi:10.1016/j.plaphy.2018.11.017 |
Saha, S., Chakraborty, P. K., Xiong, X., Dwivedi, S. K., Mustafi, S. B., Leigh, N. R., et al. (2016). Cystathionine β-synthase regulates endothelial function via protein S-sulfhydration. FASEB J. 30, 441–456. doi:10.1096/fj.15-278648 |
Šamajová, O., Plíhal, O., Al-Yousif, M., Hirt, H., and Šamaj, J. (2013). Improvement of stress tolerance in plants by genetic manipulation of mitogen-activated protein kinases. Biotechnol. Adv. 31, 118–128. doi:10.1016/j.biotechadv.2011.12.002 |
Shen, J., Zhang, J., Zhou, M., Zhou, H., Cui, B., Gotor, C., et al. (2020). Persulfidation-based modification of cysteine desulfhydrase and the NADPH oxidase RBOHD controls guard cell abscisic acid signaling. Plant Cell 32, 1000–1017. doi:10.1105/tpc.19.00826 |
Teige, M., Scheikl, E., Eulgem, T., Dóczi, R., Ichimura, K., Shinozaki, K., et al. (2004). The MKK2 pathway mediates cold and salt stress signaling in Arabidopsis. Mol. Cell 15, 141–152. doi:10.1016/j.molcel.2004.06.023 |
Wang, R. (2014). Gasotransmitters: growing pains and joys. Trends Biochem. Sci. 39, 227–232. doi:10.1016/j.tibs.2014.03.003 |
Wang, R. (2012). Physiological implications of hydrogen sulfide: a whiff exploration that blossomed. Physiol. Rev. 92, 791–896. doi:10.1152/physrev.00017.2011 |
Yang, T., Chaudhuri, S., Yang, L., Du, L., and Poovaiah, B. W. (2010a). A calcium/calmodulin-regulated member of the receptor-like kinase family confers cold tolerance in plants. J. Biol. Chem. 285, 7119–7126. doi:10.1074/jbc.M109.035659 |
Yang, T., Shad Ali, G., Yang, L., Du, L., Reddy, A. S., and Poovaiah, B. W. (2010b). Calcium/calmodulin-regulated receptor-like kinase CRLK1 interacts with MEKK1 in plants. Plant Signal Behav. 5, 991–994. doi:10.1074/jbc.M109.035659 |
Keywords: hydrogen sulfide, Persulfidation, MPK4, MEK2, cold stress
Citation: Du X, Jin Z, Liu Z, Liu D, Zhang L, Ma X, Yang G, Liu S, Guo Y and Pei Y (2021) H2S Persulfidated and Increased Kinase Activity of MPK4 to Response Cold Stress in Arabidopsis. Front. Mol. Biosci. 8:635470. doi: 10.3389/fmolb.2021.635470
Received: 30 November 2020; Accepted: 01 February 2021;
Published: 11 March 2021.
Edited by:
Venkaiah Betapudi, United States Department of Homeland Security, United StatesReviewed by:
Vijay Pratap Singh, University of Allahabad, IndiaHongfang Jin, Peking University First Hospital, China
Francisco J. Corpas, Estación Experimental del Zaidín (EEZ), Spain
Copyright © 2021 Du, Jin, Liu, Liu, Zhang, Ma, Yang, Liu, Guo and Pei. This is an open-access article distributed under the terms of the Creative Commons Attribution License (CC BY). The use, distribution or reproduction in other forums is permitted, provided the original author(s) and the copyright owner(s) are credited and that the original publication in this journal is cited, in accordance with accepted academic practice. No use, distribution or reproduction is permitted which does not comply with these terms.
*Correspondence: Yanxi Pei, peiyanxi@sxu.edu.cn