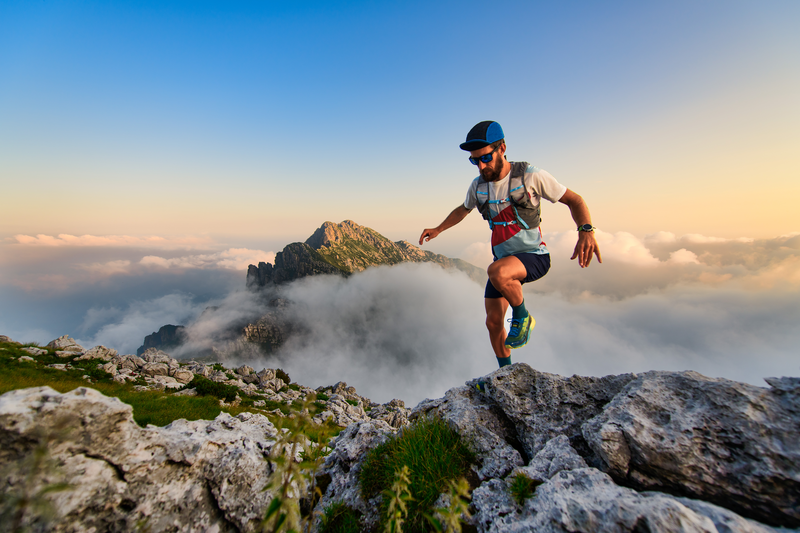
94% of researchers rate our articles as excellent or good
Learn more about the work of our research integrity team to safeguard the quality of each article we publish.
Find out more
REVIEW article
Front. Mol. Biosci. , 04 February 2021
Sec. Molecular Diagnostics and Therapeutics
Volume 7 - 2020 | https://doi.org/10.3389/fmolb.2020.636738
This article is part of the Research Topic Small Molecules and Peptide-Based Candidates as Therapeutics and Vaccines for COVID-19 Pandemic View all 31 articles
Since December 2019, SARS-CoV-2 (COVID-19), novel corona virus has caused pandemic globally, with rise in the number of cases and death of the patients. Vast majority of the countries that are dealing with rise in the active cases and death of patients suffering from novel corona viruses COVID-19 are trying to content the virus by isolating the patients and treating them with the approved antiviral that have been previously used in treating SARS, MERS, and drugs that are used to treat other viral infections. Some of these are under clinical trials. At present there are no therapeutically effective antiviral present and there are no vaccines or drugs available that are clinically approved for treating the corona virus. The current strategy is to re-purpose the available drugs or antiviral that can minimise or reduce the burden of the health care emergencies. In this article the reuse of antiviral, US-FDA approved drugs, plant based therapeutic, anti-malarial, anti-parasitic, anti–HIV drugs and the traditional medicines that are being currently used in treating the symptoms of COVID–19 patients is discussed emphasis is also given on the treatment using monoclonal antibodies. The present article provides the therapeutic strategies that will qualify as one of the best available treatment for the better management of the COVID–19 patients in order to achieve medical benefits.
Viruses are obligate parasites which mean they require host cells to reproduce and increase their number; they cannot replicate on their own. These viruses contain single or double stranded DNA or RNA as their genetic material. Viruses are infectious non-cellular particles (López-García and Moreira, 2012). These are mostly contagious and are known to infect almost all the living cells. These are tiny particles that cannot be observed under a normal microscope due to their sub-micron sizes that range from 150–200 nm. A virion is a functional virus that contains genetic material enclosed in an envelope, and the surface proteins help the virus infect the host cell and enter the host cell (Lodish et al., 2000).
Coronaviruses (CoVs) can infect mammals and other species. They are the largest group of viruses and its name is derived from Latin meaning “crown” or “wreath.” This is about the appearance of the surface that has a lipid membrane embedded with club-shaped projections that give the image or appearance of a stellar corona (Masters, 2006). These viruses belong to the order Nidovirales, family Coronaviridae, and subfamily Orthocoronavirinae. The subfamily Orthocoroanavirinae is divided further into four subgroups which include alpha, beta, gamma, and delta CoVs. The viruses belonging to the order Nidovirales have a 5′ capped, enveloped, single-stranded positive sense RNA virus with an unusual large RNA genome (∼30 kb) with sizes ranging from 80–120 nm (Belouzard et al., 2012). Generally, these viruses have spikes protruding from the surface and replicate in a unique way (Weiss and Navas-Martin, 2005). The RNA of this virus acts like messenger RNA and synthesizes two long proteins that are needed by the virus to replicate when it infects the cell. The SARS-CoV-2 genome encodes many proteins like the nucleocapsid protein, spike (S) protein, envelope (E) protein, and membrane protein (M) that are required for the replication into the host cell. It also codes for the coronavirus main protease that plays an important role in the gene expression and cleaves polyprotein-related proteins (Graham et al., 2008; Chang et al., 2020).
Since late December 2019 the world has being experiencing the problem of increasing cases of COVID-19. The SARS infection first appeared in Wuhan in the Hubei province of China. As of December 2020, there are around 78.7 million active cases and 1.73 million deaths across the world. It has emerged on six continents and has increased exponentially in some countries like the US, Brazil, and India. It was at first called a novel coronavirus belonging to Coronaviridae, beta coronavirus genera and subgenus sarbecovirus and this novel coronavirus was officially named by the International Committee on Taxonomy of Viruses (ICTV) as SARS-CoV-2 (Graham et al., 2008) due to its similarities with the SARS (severe acute respiratory syndrome) outbreak in 2003 (Graham et al., 2008). The World Health Organization (WHO) announced it as COVID-19 on 11 Feb 2020 and declared a public health emergency on 30 Jan 2020.
SARS-CoV-2 is an airborne virus highly contagious with an ability to transfer. As the current evidence suggests, SARS-CoV-2 is transmitted through respiratory mucus or saliva droplets (with droplet size > 5–10 µm in diameter) either from direct contact with an infected person coughing, sneezing, or talking from a distance of 1 meter or less or as droplet nuclei (particle < 5 µm in diameter) that remain active for a considerably long period of time and can spread to other people within 1 m of distance. Indirectly, the virus can spread through contact with surfaces that have been exposed to the virus which may make its way through the nose, mouth, or conjunctiva (Chang et al., 2020; Prasad and Prasad, 2020). It may be suggested that the face, nose, and eyes are often ignored by people as the portal entry for the COVID-19 infection. New evidence on the virus suggests that gastrointestinal symptoms are due to the presence of the viral RNA of this virus or the live infectious particles of the virus found in the feces suggesting that fecal-oral transmission may be one of the other possible routes of transmission of this virus (Coronaviridae Study Group of the International Committee on Taxonomy of, 2020). It is quite intriguing to know that there is no vertical transmission of this virus as evident from the two case studies conducted by (Hindson, 2020; WHO, 2020).
A large number of patients infected with COVID-19 are either asymptomatic or show mild symptoms and recover from the sickness. The clinical interpretations are fever, dry cough, and shortness of breath (Chen et al., 2020b) There are reports on patients experiencing anosmia, dysgeusia, and diarrhea (Fan et al., 2020). However, the mortality rate and the chance for the need of ICU care increases in elderly patients over 60 years of age and patients with underlying conditions like hypertension and diabetes (Shah et al., 2020).
There are no approved vaccines or any specific antiviral therapies available for COVID-19 (Lau et al., 2005). Therefore, the only preventive options left are to give supportive, symptomatic care, social distancing, and quarantine (Coleman and Frieman, 2014). It is a well-known fact that it takes months and years to develop a vaccine from scratch. Hence, researchers are constantly looking for options to manage or treat patients with drugs that are approved and used in treating other diseases like SARS, MERS, or the influenza virus within acceptable safety limits. Some anti-malarial and antiviral treatments have shown some promising results in the management of COVID-19. Some of the drugs that are approved for human disease have shown an antiviral effect by either blocking the viral enzyme which prevents its entry, or by blocking the replication of the genome that is necessary for the formation of the virus particle (Zumla et al., 2016).
In the present article, we have summarized the current therapeutic strategies, vaccines, and other diagnostic options that are being used in the management of COVID-19 and to shed some light on the intervention measures that could be taken to control the outbreak. This article provides information on the therapeutic strategies that will help to address the issues of the pandemic and control them.
The following pharmacotherapeutic agents are used currently in the treatment and management of COVID-19. Most of the drugs are repurposed agents that are used for the treatment of the disease (Table 1).
Hydroxychloroquine (Figure 1) and chloroquine (2) have been widely used as antimalarial drugs caused by the plasmodium species. Both chloroquine and hydroxychloroquine are the derivatives of the organic compound that belong to the quinoline group (Goel and Gerriets, 2020). It is one of the active chemical constituents found in the bark of the Cinchona officinalis plant. The antimalarial drugs chloroquine and hydroxychloroquine can also be used in treating amebiasis (Knight, 1980), in certain autoimmune diseases like rheumatoid arthritis (Freedman and Steinberg, 1960) and in lupus erythematosus syndrome (Liu et al., 2020b). Chloroquine and its derivative hydroxychloroquine exhibit antimalarial activity by inhibiting the enzyme heme polymerase in the trophozoites resisting the conversion of heme to hemozoin resulting in the accumulation of heme which is toxic to the parasite and kills it (Coronado et al., 2014). These antimalarial agents exert an antiviral effect by multifactorial mechanisms like increasing the pH of the intracellular vacuole and by interfering in the degradation of the protein pathways and modifying the ACE2 receptor glycosylation (Savarino et al., 2006; Sahraei et al., 2020). Angiotensin-converting enzyme 2 (ACE2) is a membrane bound protein and receptor for SARS-CoV. It facilitates its entry into the host cell by binding to the spike (S) protein of the virus resulting in the fusion of the viral and host membrane (Dimitrov, 2003; Li et al., 2003; Simmons et al., 2004). In treating a viral infection like SARS-CoV, it is of utmost importance to prevent the binding of the spike (S) protein of the virus to the ACE 2 by blocking it (Yeung et al., 2006). Chloroquine is being commercialized for its antimalarial activity and used as a drug for autoimmune diseases. It is also known to have a broad spectrum antiviral effect (Yan et al., 2013) and due to its inhibitory effect on ACE2, it is known to be a SARS-CoV inhibitor (Vincent et al., 2005). Chloroquine and hydroxychloroquine have been studied intensively both in vitro and in vivo for its antiviral effect on SARS-CoV. Initial in vitro studies showed some promising positive results (Gautret et al., 2020a). However, there are no consistent reports available on the clinical trials of these drugs (Molina et al., 2020). CQ seems to be more effective in vitro in controlling 2019-nCoV, and it may be considered for the cases of human suffering from COVID-19 (Wang et al., 2020a). A similar inhibitory activity of CQ for SARS-CoV-2 was observed in vero E6 cells at a micromolar level (EC50 = 1.13 µM) and was found to inhibit at both the entry and post entry stages in vero E6 cells (Wang et al., 2020a). CQ was found to be effective in inhibiting the aggravation of the virus when compared with the control group in 100 COVID-19 patients. It was also found to reduce the disease and cause negative results among positive COVID-19 patients (Gao et al., 2020). In a press briefing, the state council of China stated that chloroquine phosphate was found to be markedly effective in controlling the deteriorating condition of COVID-19 patients in many of the clinical trial centers in China in Feb 2020 (Gao et al., 2020). The (Guideline for the Prevention, Mar 17, 2020) recommends: for patients aged between 18–65 (adults), a dose of 500 mg twice daily for 7 days; for patients who weigh more than 50 kg, 500 mg twice daily for 2 days; for patients less than 50 kg, 500 mg once daily for 5 days; and is prohibited to patients with cardio problems due to its cardiotoxicity. However, more attention should be paid to the side effects of CQ on patients based on the prior observations made in patients that were treated for other viral diseases and due to its cardiotoxicity. There are several online reports and media reports available regarding the benefits of CQ As of now, many clinical trials are under way in evaluating its effectiveness and its safety in treating COVID-19 patients (NIH, 2020).
FIGURE 1. Chemical structures of pharmacotherapeutic agents used in the management of COVID-19 as repurposing agent.
Hydroxychloroquine (HCQ) is an antimalarial and immunosuppressive drug. In vitro studies found that HCQ was more effective in inhibiting SARS-CoV-2 than CQ (Yao et al., 2020). At present, there are many clinical trials underway on the efficacy of HCQ on COVID-19. As mentioned earlier, the clinical studies are inconsistent. A clinical study done by Dr. Didier Raoult revealed that patients treated with HCQ and azithromycin (3) showed 100% recovery of the COVID-19 patients and tested negative within 6 days of treatment (data not published). Due to its positive in vitro results on SARS-CoV-2, it was used on a large scale for COVID-19 patients in many countries including the USA. Other countries like South Korea and China that are affected by the pandemic have issued or underlined the guidelines for the therapeutic use of HCQ in treating COVID-19 patients. The Comprehensive Treatment and Management of COVID-19: Expert Consensus Statement from Shanghai recommends the use of hydroxychloroquine without dosage recommendation. The Peking University developed a PBPK model, and based on the result of this model, they recommended an oral dose for COVID-19 patients (Qu et al., 2020).
This was later confirmed by a clinical trial with 62 patients, where 31 received HCQ in a dose of 400 mg/d, 200 mg twice daily for 4 days while 31 other patients were the control group. After five days, the recovery period for the patients in the HCQ group was shortened and they showed significant recovery from the symptoms of fever and cough; further, it was observed that 81% of the patients showed improvement in the condition of pneumonia in the HCQ group compared to 55% in the control group. It should be mentioned here that the patients who were critically ill were from the control group, which confirms the therapeutic use of HCQ (Qu et al., 2020; Guideline for the Prevention, Mar 17, 2020). In another study, 36 COVID-19 patients were given HCQ with azithromycin. A full and rapid clearance of the virus was observed. The effect was more significant when given in combination with azithromycin then HCQ alone. It is still not clear how azithromycin enhances the efficacy, but it is known to inhibit the virus in severe respiratory infections (Megarbane and Scherrmann, 2020). The results were not similar in a small study of 11 COVID-19 patients conducted by (Molina et al., 2020) using the same combination of HCQ and azithromycin. This study was with a small number of patients, and the control group was from another clinical center, so the study was not a randomized controlled trial. This paper also did not undergo peer review and was published within 24 hours of its submission. In order to confirm its results, an expanded clinical trial with correct protocols should be followed. In another clinical trial, 42 COVID-19 patients were recruited. Of these 42 treated patients, six were excluded from the study (three patients were admitted to ICU, one died, one showed an adverse drug reaction, and one refused to take the medication) (Gautret et al., 2020b). The remaining 36 patients were taken into consideration for the final analysis. Twenty patients were given HCQ 200 mg orally three times daily with six of these patients given azithromycin along with HCQ to enhance the efficacy of HCQ. The patients who refused to take the drug were treated as the control which was from the other hospital. They found that six of the 36 patients showed no symptoms, 22 of the remaining patients complained of a sore throat and the remaining eight patients had signs of pneumonia. The primary goal of the clinical trial was the clearance of the virus, and the secondary was the serial viral load, clinical follow up, and the adverse effect of the drug. Both drugs have long QT intervals and increase the chances of tip torsion arrhythmia which may lead to death. Hence, a thorough evaluation is required (Malviya, 2020). In another observational study, 80 patients with mild COVID-19 infection were given hydroxychloroquine 200 mg three times daily for ten days along with azithromycin 500 mg orally for one day followed by 250 mg daily for four days (Gautret et al., 2020b). It was observed that out of the 80 patients, one died, one was admitted to the ICU, and the rest showed rapid recovery with a rapid decrease in the viral load in nasopharyngeal which was negative on day 7 with 83% and 93% on day 9, and the respiratory viral culture showed negative results with 97.5% on day 5. The hospital stay was reduced to five days. This study too had limitations as this was an observational study without any comparative group. And, the beneficial effects of this study cannot be evaluated as the study was on patients with mild symptoms. A pilot study was conducted in which they recruited 30 patients who were randomized into two groups of 15 each with one group receiving 400 mg of HCQ daily along with supportive care and the other group receiving only supportive care (Chen et al., 2020c). They observed a negative clearance of the virus on day 7 by RT-PCR and one patient suffered from severe symptoms. However, it was observed that there was no significant difference between the two groups in the clearance of the virus; with respect to clinical and radiological parameters, both groups performed equally the same. They concluded that the study was good, but a larger group should be included to evaluate the effectiveness of HCQ on COVID-19 patients. This paper was published in a Chinese journal and it is not clear whether it was peer reviewed. In a retrospective study of 368 patients hospitalized due to COVID-19 (Magagnoli et al., 2020), the patients were divided into 3 groups with the first group consisting of 97 patients who were given HCQ alone, the second group consisting of 113 patients who were given HCQ and azithromycin, and the third group consisting of 158 with no HCQ. In this study, only two primary result data were taken into consideration, one the death rate and the other the use of mechanical ventilation. The mortality rate was 27.8%, 22.1%, and 11.4% in HCQ alone, HCQ + azithromycin and no HCQ, respectively. The use of mechanical ventilation was 13.3%, 6.9%, and 14.1% in the same three groups, respectively. It was observed that the death rate was higher in the HCQ group then no HCQ group, and there was no significant difference in the use of ventilation in all three groups. The authors observed no beneficial effect of HCQ alone or with azithromycin or no HCQ on ventilation. An increase in the mortality rate was observed in the HCQ alone treated group. This study raises the credibility of the efficacy of HCQ for COVID-19. More randomized clinical control trials are required to address the issue. There are a large number of trials going on or reported (Borba et al., 2020; Mahevas et al., 2020; Molina et al., 2020; Tang et al., 2020) but none of them provide clear-cut data on whether to use or not use HCQ in treating COVID-19.
Even the results on the prophylactic role of HCQ for health care workers (Chatterjee et al., 2020) and individuals with a high risk of exposure to COVID-19 (Boulware et al., 2020) showed contradictory results. Another placebo controlled trial testing HCQ on post exposure for prophylaxis effect (Boulware et al., 2020) showed no significant difference in the occurrence of COVID-19 like symptoms between participants that received HCQ [49 of 414 (11.8%)] and those who received a placebo [58 of 407 (14.3%)]. It was also observed that the side effects were more in the HCQ participants than the placebo ones (40.1% in HCQ participants and 16.8% in placebo participants) but the side effects were without any adverse reaction. From their study, they concluded that HCQ could not prevent the occurrence of COVID-19 after exposure for high or moderate risk individuals. The study had certain limitations as most of the participants including the health care workers were unable to assess testing and the diagnosis was based on the symptoms that were more similar to the disease. The Indian Council on Medical Research (ICMR) studied the pre-prophylaxis effect of HCQ on health care workers (Boulware et al., 2020; Chatterjee et al., 2020). They concluded that there was a significant decline in the odds of getting infected by the health care workers who were given a maintenance dose of HCQ. A dose response relationship was seen between the rate of exposure and reduction in the infection. These two drugs are immunomodulators and are not immune suppressants (WHO, 2017). Hence, these drugs have no adverse effect related to an infection or cancer (Lazarus et al., 2006; Schrezenmeier and Dorner, 2020). The most common adverse side effects associated with these two drugs are nausea, vomiting, and abdominal discomfort (Srinivasa et al., 2017), but CQ and HCQ may cause cardiotoxicity, myopathy, and retinopathy (Costedoat-Chalumeau et al., 2007; Abdel-Hamid et al., 2008; Chatre et al., 2018; Jorge et al., 2018) and have long QTc intervals (Costedoat-Chalumeau et al., 2007; WHO, 2017; Chatre et al., 2018). Though the margin of safety for HCQ is significant (WHO, 2017; Ursing et al., 2020), in seriously ill COVID-19 patients, it causes cardiac problems and death related to it (Guastalegname and Vallone, 2020) which may be due to the high dose (Borba et al., 2020) in combination with azithromycin which increases the QT intervals (Malviya, 2020) and due to the patients’ underlying conditions (WHO, 2017; Chatre et al., 2018).
In the present scenario, the role of CQ or HCQ in the management of COVID-19 is a dynamic phenomenon, and as the results of the clinical trials become available, their role may change. As of now, there are many clinical trials underway, and researchers are awaiting the results. The clinical trials should include a large number of participants with different age groups and different underlying conditions. They should also include patients who are asymptomatic, mild symptomatic, and severely ill. These studies should have varying drug doses. In vitro studies have shown that these two drugs have antiviral properties, but the main question is how far these in vitro studies can be transformed or utilized in clinical studies (Rathi et al., 2020; Taccone et al., 2020). So far the clinical trials are not convincing enough for the use of HCQ in the management of COVID-19 patients . Depending on the results of the clinical trials available at this time, the experts are against the use of HCQ in the management of COVID-19 patients. Despite all of these contradictory opinions, there has been widespread advocacy to use HCQ mainly due to the fear that surrounded around COVID-19 and media/social forces rather than scientific facts (Cohen, 2020). Question arises on the safety of these both drugs, CQ and HCQ, and there is a thin line when it comes to the safety. Hence, if used indiscriminately and without proper supervision, they may lead to severe side effects mainly related to a craniological effect. If the drug is used under supervision as a prophylactic agent and as mentioned or advocated by ICMR as safe and encouraging (Chatterjee et al., 2020) with good positive results, the drugs would be a blessing in blocking the virus. Otherwise, it may be an antimalarial that can undergo clinical trials.
Remdesivir (4) was developed as an antiviral agent by an American biotechnology company called Gilead Science to treat the Ebola virus. Remdesivir is a prodrug that is metabolized to GS 441524, a nucleoside analogue, which inhibits the RNA dependent enzyme RNA polymerase (Gordon et al., 2020). This affects the function of the RNA polymerase that inhibits the viral enzyme endonuclease involved in the proofreading activity resulting in a decrease in the production of viral RNA (Agostini et al., 2018). Remdesivir exhibits a broad spectrum of antiviral activity and has shown encouraging results against SARS-CoV and MERS-CoV infections. Due to its inhibitory activity, many physicians have recommended the use of remdesivir in countries like the United States, Europe, and Japan (Bloomberglaw.com, 2020). In patients suffering from SARS-CoV, remdesivir has not been recommended by drug regulatory authorities. An in vitro clinical isolate of SARS-CoV showed that remdesivir exhibited significant inhibitory activity. There are studies available on the efficacy of remdesivir. There are many clinical trials underway to test the efficacy of remdesivir on COVID-19. In China, two clinical trials were started to study the efficacy of remdesivir on SARS-CoV-2. At Capital Medical University, a randomized quadruple-blind placebo controlled phase III clinical trial was registered to determine the efficacy of remdesivir on patients showing mild and moderate symptoms of a COVID-19 infection (NCT04252664, (ClinicalTrials.gov., 2020a)). A second trial on patients with advanced SARS-CoV-2 was registered at the same center (ClinicalTrials.gov., 2020b). In both the trials, on day one patients were administered with 200 mg of remdesivir, followed by 100 mg for 9 days. In both trials, the main aim was to note the time taken for the clinical trials, time taken to bring the fever to normal, saturation of oxygen, respiratory rate, and relief of cough within 72 hours.
Similarly, almost at the same time, the first COVID-19 case was reported in the United States (Holshue et al., 2020). The patient was suffering from a fever for four days which was later confirmed as COVID-19. When his condition worsened on the seventh day, he was given remdesivir by IV and his condition improved with no adverse side effect (Holshue et al., 2020). The clinical state of the patient improved the next day, but it was confounding to analyze the impact or the efficacy of remdesivir because the patient received simultaneous treatments of acetaminophen, ibuprofen, guaifenesin, vancomycin, cefepime, and supplemental oxygen. Later during the same period, 12 patients were confirmed with SARS-CoV-2 (Kujawski et al., 2020). Of the 12 patients, seven were hospitalized. Upon the worsening of their condition, three patients were given remdesivir (on compassionate grounds). The treatment was given for 4–10 days. Each patient received 200 mg of remdesivir IV on day one followed by 100 mg/day. It was observed that all the patients showed transient gastrointestinal symptoms like nausea, vomiting, gastroparesis, or rectal bleeding. Even though all the patient reported the symptoms, the treatment was continued until improvement (Kujawski et al., 2020). As the study was a small size and did not have any control group, it is hard to summarize the effect of remdesivir or the safety of this drug on COVID-19 patients. Funded by the National Institute of Allergies and Infectious Disease (NIAID), the NIH developed a study on the existing Chinese clinical trial in consultation with the WHO. The study was a double blind, randomized placebo controlled phase III trial which was aimed to evaluate the safety and efficacy of remdesivir in comparison with a placebo (ACTT-1 ClinicalTrials.gov number, NCT04280705) (clinicaltrials.gov, 2020). At present, the patients are being enrolled and the severity of the patients’ conditions is noted based on an eight-point ordinal scale along with secondary outcomes. An estimate of 75 clinical trials is expected to participate in this study across the United States and is expected for the primary completion by April 2023.
Professor Cao Bin at the China-Japan Friendship Hospital in a clinical study suggested that remdesivir does not have any significant beneficial role or has no antiviral effect on patients suffering for severe SARS-CoV-2 (Wang et al., 2020c). However, there are several studies on the efficacy of remdesivir in COVID-19 patients. The results of the clinical trials from around the world suggest that remdesivir is known to reduce the symptoms and the mortality rate in patients on ventilation in intensive care units (Grein et al., 2020). Meanwhile the results of several clinical trials conducted in Chicago suggest that remdesivir is known to have a beneficial effect in early COVID-19 patients due to reduction in lung damage (Williamson et al., 2020). The results published on the use of remdesivir in severe COVID-19 patients suggest that 68% of severely affected patients have reduced symptoms and 13% mortality. Although the results are encouraging, it needs to be confirmed in a randomized, placebo controlled clinical trial on COVID-19 patients (Grein et al., 2020). The efficacy and safety of remdesivir has not been confirmed yet. It is available in several ongoing clinical trials for adults and non-pregnant patients, but on compassionate grounds the use of remdesivir is limited to pregnant women and individuals <18 years of age suffering from severe COVID-19. Although there is no contradiction indicated in the clinical trials, people with liver and kidney impairment must be treated or taken care of with caution (Guo et al., 2020). Under the compassionate use program, patients with confirmed COVID-19 have been given remdesivir in places like the US, Europe, and Japan (Amirian and Levy, 2020). Some of the initial studies in China have shown that remdesivir has no role in the clinical improvement of patients (Wang et al., 2020c). Results of the clinical trials on the adaptive COVID-19 Trial (ACTT-1) showed the recovery time was reduced significantly in the treatment group compared to the control (11 days compared to 15 days with a ratio rate of recovery 1.32; 95% confidence interval [CI], 1.12 to 1.55; p < 0.001) (Beigel et al., 2020). Although there are no results to validate that remdesivir decreases SARS-CoV-2 or RNA viral loads or reduces the mortality rate (Wang et al., 2020c), earlier positive results encourage the use of remdesivir. Furthermore, the results of the trials are still pending to validate the present results.
Based on the findings, the United States Food and Drug Administration (US-FDA) has approved the emergency use of remdesivir in hospitalized COVID-19 patients. With no other drug being approved by the US-FDA for use in the treatment of SARS-CoV-2, remdesivir may lessen the mortality, morbidity, and the burden of the global pandemic (FDA-News, 2020).
Favipiravir (T-705) (5) is a purine nucleic acid analog. In RNA viruses, favipiravir is known to inhibit the RNA dependent RNA polymerase (RdRp). After phosphoribosylation and phosphorylation, it forms favipiravir-RT which terminates the elongation of the RNA strand by binding to the RdRp (Sangawa et al., 2013). Due to its antiviral activity, favipiravir was licensed to treat influenza in Japan in 2014 (Hayden and Shindo, 2019). It is also known to be effective against several other viruses like Ebola, influenza, norovirus, chikungunya, and enterovirus (De Clercq, 2019). Favipiravir is shown to have teratogenic and embryotoxic effects and should be avoided during pregnancy and lactation (Nagata et al., 2015). In vitro studies on E6 vero cells suggest that favipiravir was able to interfere with the function of SARS-CoV–2 (EC50 = 61.88 Μm) (Wang et al., 2020a). The results encouraged more than 10 clinical trials which are underway or registered and patients are being recruited for the study. The initial results from one of the trials (ChiCTR2000029600) showed some promising results in terms of virus clearance which was short (4 days vs. 11 days) and the chest image (91.43% vs. 62.22%) when compared to the control group (with lopinavir and ritonavir). In 45 cases, the adverse reaction in comparison with the control group was lower (Cai et al., 2020). Another randomized clinical trial at the hospital at Wuhan University (ChiCTR2000030254) concluded that the patients treated with favipiravir (for 120 patients) showed a significant recovery rate (7 days) as compared to the control which was treated with umifenovir (for 120 patients). They concluded that the group treated with favipiravir were significantly better than the control group (Chen et al., 2020a). Encouraged by the positive results from the clinical trials and the availability of the drug, experts have recommended that the drug be included in the guidelines of National Health Commission of the People’s Republic of China.
Lopinavir and ritonavir (LPV/r) (6, 7) is an FDA approved oral combination drug that is used in the treatment of HIV-1. As an antiretroviral protease inhibitor, lopinavir is metabolized in the liver where ritonavir, a CYP3A4 inhibitor, is also a protease inhibitor and in combination with lopinavir, it is used to boost its antiviral activity and its bioavailability (De Clercq, 2009). It was used in the outbreak of SARS in 2003 due to its antiviral activity. Therefore, when there was a SARS-CoV-2 pandemic, LPV/r was considered as one of the best options to treat SARS-CoV-2. In vero E6 cells, lopinavir is known to inhibit the replication of SARS-CoV-2 with EC50 of 26.63 mM (Choy et al., 2020). In another in vitro study, Kang et al. showed that LPV/r significantly inhibited the activity of SARS-CoV-2 with a concentration of 7/1.75 mg/ml (Kang et al., 2020). In one of the clinical studies on hospitalized COVID-19 patients, the analysis showed that the early administration of LPV/r resulted in a short virus shedding (Yan et al., 2020). However, the results were not similar in the randomized clinical trial which showed that LPV/r had no beneficial effect on COVID-19 patients. In another randomized clinical trial in China, the combination drug was given twice daily for two weeks to adult patients with severe COVID-19. It was observed that there was no significant beneficial effect to the patients when compared to the control (standard care) group (Cao et al., 2020). In the same study, due to an adverse effect, only 14% of the patients could complete the treatment. Similar lack of benefit in the patients was observed in the clinical trial conducted in UK (unpublished data)(Randomized Evaluation of COVID-19 Therapy). The study resulted in terminating the recruitment of patients for the treatment. The treatment of LPV/r has adverse side effects like anorexia, nausea, abdominal discomfort, diarrhea, or acute gastritis, along with the risk of liver damage, inflammation of pancreas, more severe skin eruptions, and also the interaction of the drug with the CYP3A inhibition was observed in the clinical trials. These adverse reactions raised concerns related to the high dose and long term use of LPV/r to improve the clinical condition of the patients (Cao et al., 2020). In context to the above-mentioned adverse effects, there are serious concerns related to kidney injury, and secondary infection was less in those who were not receiving treatment. There are very limited data available on the use of lopinavir/ritonavir in treating COVID-19 patients. Further trials involving patients with severe COVID-19 symptoms and large control clinical trials are needed, and patients with underlying conditions need to be involved to study the role of lopinavir/ritonavir.
Arbidol (8), an indole-derivative molecule, has been approved as a prophylactic and to treat influenza and other respiratory infections caused by a virus (Blaising et al., 2014). Arbidol is being used in Russia and China against an upper respiratory tract infection due to the influenza virus A and B. Arbidol has inhibitory activity against diseases such as hepatitis B and C (Boriskin et al., 2008). Antiviral activity against SARS pathogens has been reported, and in vitro antiviral activity of the derivative arbidol mesylate (derivative of arbidol) showed a five times higher reduction in the reproduction of SARS then arbidol (Khamitov et al., 2008). There is a report available on the in vitro study on the efficacy of arbidol against COVID-19 (Lu, 2020). Arbidol exerts antiviral activity by inhibiting hemagglutinins which is a protein on the membrane of the virus which binds to the sialic acid receptor on human cells and makes its entry into the human cell by endocytosis and prevents the virus from being infective. It also induces the production of interferon and exhibits broad antiviral activity (Blaising et al., 2014). (Deng et al., 2020) observed that in SARS-CoV-2 patients taking a combination drug of arbidol and LPV/r, the negative rate increased in 7 and 14 days and the CT scan of the chest improved when compared to the oral LPV/r combination. However, it has to be noted that no improvement in the symptoms due to LPV/r or arbidol was observed. The clinical trial at the Shanghai Public Health Clinical Center observed more adverse reaction related to the gastro intestine (Jun et al., 2020). These contradictory results may be due to a small trial size and the timing of medication. A clinical trial is underway in China on post exposure prophylaxis in a population with a high risk of COVID-19. In this study, health care staffs were included (ChiCTR2000029592). The outcome of this result may throw light on the efficacy of arbidol on the early treatment and prevention of COVID-19.
Ribavirin (9), a guanosine nucleoside, is a synthetic antiviral drug. It exhibits its antiviral activity by inhibiting the enzyme inosine monophosphate dehydrogenase and shutdowns the progress of the replication of RNA and DNA viruses that leads to the destruction of the genome RNA. Currently, this drug has been approved and used in the treatment of chronic hepatitis C (Koren et al., 2003). It is known to inhibit viral replication in MERS-CoV when given to patients in combination with other antiviral drugs. It was observed that ribavirin when administered in combination with interferon alfa-2a, 70% of the patients who received this combination were alive for 14 days when compared to 29% in the control. In in vitro studies, ribavirin exhibited antiviral activity against the respiratory syncytial virus and influenza and para influenza virus, and due to its antiviral activity, ribavirin has been recommended by the US-FDA to treat patients with respiratory syncytial virus, where it had no antiviral activity against SARS-CoV (Koren et al., 2003). With respect to SARS-CoV-2, there is new evidence that suggests that ribavirin may decrease the replication of the virus by inhibiting PLpro (Wu et al., 2020). Recently in an open label randomized clinical trial (phase II), patients treated with a ribavirin, LPV/r, and interferon-1b combination had viral shedding more quickly when compared to patients with LPV/r (Fujii et al., 2004; Hung et al., 2020). As this study lacked a placebo group and critically ill patients, and it lacked ribavirin and a IFN-ß-1b monotherapy group, it was difficult to assess the use of this drug alone and it needs to be further studied. It has to be noted that ribavirin has more adverse reactions, such as hemolytic anemia (Fujii et al., 2004), hypocalcemia, and hypomagnesemia (Koren et al., 2003; Tai, 2007). It is being currently investigated in combination with other antivirals for therapy, but there are no reports available on the use of ribavirin alone in the treatment of COVID-19.
Approved by the US-FDA (United States Food and Drug Administration), Disulfiram (10) is used in aversion therapy for alcohol. It inhibits the enzyme aldehyde dehydrogenase in the liver. The in vitro study has shown that disulfiram inhibits PLpro papain–like protease in SARS-CoV and MERS-CoV (Lin et al., 2018). In vitro studies have shown that GRL0617, a targeted PLpro inhibitor, blocked the replication in SARS-CoV (Ratia et al., 2008). This compound is in its preclinical study, but it may be considered in the development of an anti-coronavirus drug. So far there are no clinical data available on the efficacy of disulfiram in COVID-19 treatments (McCreary and Pogue, 2020), but there is theoretical evidence that supports the repurposing of disulfiram.
Imatinib (11), also known as Gleevec, is an inhibitor of oral tyrosine kinase that has antiviral activity against Ebola and poxviruses (Coleman et al., 2016). In SARS and MERS-CoV, Abelson tyrosine kinase 2 (ABl2) is required for replication, and imatinib targets this ABl2. Imatinib inhibits MERS-CoV and SARS-CoV infections in in-vitro studies and with significantly low cellular toxicity (Dyall et al., 2014). There are no clinical data available at present and some are yet to be published, but the result of the in vitro studies suggest that a clinical trial or study would be beneficial.
Baricitinib (12) is used in the treatment of rheumatoid arthritis. It inhibits Janus kinase (JAK), binds to the adaptor associated kinase-1(AAK1) enzyme that is involved in the clathrin-mediated endocytosis, and also stops the cytokine storm (Russell et al., 2020). Based on the computational studies, baricitinib was identified as one of the promising candidates in the treatment of SARS-CoV-2. In a pilot study, they compared baricitinib and LPV/r with the standard therapy given to COVID-19 patients. This study showed that baricitinib decreased the viral entry and the cytokine storm (Mehta et al., 2020). It also showed significant improvement in fever, dyspnea, and hypoxia (Cantini et al., 2020). This drug seems to be one of the promising antiviral protease inhibitors which may be explored further for SARS-CoV-2.
Discovered in 1975, Ivermectin (13) came into use in 1981 (Vercruysse and Rew, 2002; Mehlhorn, 2008). It is approved by FDA to treat, prevent and control the river blindness (onchocerciasis) that is common in population. Ivermectin is also used in the treatment of Strongyloidosis, enterobiasis, Trichuris trichura, Loa Loa, Scabies, human lice, malaria. Under in–vitro conditions Ivermectin is known to exhibits broad spectrum of antiviral activity against large number of viruses (Mastrangelo et al., 2012; Lundberg et al., 2013; Azeem et al., 2015; Gotz et al., 2016). As mentioned above COVID–19 is a ssRNA virus that is similar to SARS coronavirus (SARS–CoV). Recent in-vitro studies on the antiviral activity of Ivermectin against SARS CoV –2 revealed that it can inhibit the viral replication. It reduced the virus load by 5000 fold in vero hSLAM cells submerged with single dose of Ivermectin for period of 48 hour, however increase in exposure period up to 72 hour did not show any effect in the reduction of the viral load. In addition, there was no side effect of this drug at any given point of time (Choudhary and Sharma, 2020). How it exhibits its antiviral activity is not known, it probably exhibits its antiviral activity same way has it does on other different viruses. It inhibits the import of host and viral proteins into the nucleus. Integrase protein of the virus and importin IMP/1 heterodimer is necessary for the IN nuclear import which enhances the infection. During infection majority of the RNA viruses rely on the IMP/1, Ivermectin increases its antiviral potentials by inhibiting the import (Caly et al., 2020; Choudhary and Sharma, 2020). Study on the clinical efficacy of ivermectin was studied by (Rajter et al., 2020) patients hospitalized in four board health hospital in Florida was studied a total of 280 patients was involved (from march 15 to 11 may 2020). Out of 280 patients, 173 patients were treated with ivermectin and 107 without, most patients in both the group received HCQ or azithromycin or both of them. The results showed that the patients treated with ivermectin had significantly lower mortality rate especially in patients with ventilator support and who required inspired oxygen in comparison to control (15.0% vs 25.2%). But this study has short comings this finding must be confirmed by randomized clinical trial. In another study carried out in India on the association between ivermectin prophylaxis and infection of COVID-19 among health care works. A reduction of 73 % in COVID-19 infection was observed in health care works for the following one month. In this study the health care workers were given two–dose of ivermectin prophylaxis at dose of 300 μg/kg with a gap of 72 hours (Behera et al., 2020). This study was not peer reviewed and further clinical trials are required so that it can be used in large scale or to be used for clinical trial. In another retrospective study conducted on hospitalized patients receiving Ivermectin in Spain showed no improvement (Camprubi et al., 2020). They observe that there was no clinical improvement and microbiological outcomes in the severe COVID-19 patients who received 200 μg/kg in comparison to similar group of patients who did not receive ivermectin, It must be noted that the ivermectin treatment was given later in the later stage of the disease or infection. This study has some limitations, randomized clinical trials are necessary to evaluate the high doses. In another randomized clinical trial involving patients with mild to moderate symptoms and who tested positive for COVID–19 was studied (Chowdhury et al., 2020) in Bangladesh Chakoria Upazilla Health Complex. In this study the patients were divided into two group, group A received single dose of 200 µgm/kg + Doxycycline 100 mg BID for 10 days and Group B received 400 mg of HCQ on 1st day and subsequent 9 days they received 200 mg + Azithromycin 500 g for 5 days. Time taken for negative PCR test and full symptomatic recovery was measured in both the groups. Patients in group A (Ivermectin + Doxycline ) showed more promising trend towards recovery then group B. It was observed that the patients in group A showed negative result at a mean day of 8.93 days and full symptomatic recovery at 5.93 days and 55.10 % were symptom free by 5th day. Whereas group B showed negative result at mean of 6.99 days and were symptom free by 9.33 days. These results were not statistically significant between the difference in time for negative PCR and between symptom free and was not peer reviewed. Although the results are promising but more test results are awaited to be used as one of the therapeutic agents.
Passive immunity through antibodies can be used in the treatment of SARS-CoV-2 patients. This is done by using convalescent plasma from recovered patients. The convalescent plasma from infected patients contain antibodies that give immunity to the patients either by binding to the infectious particle-like virus and neutralizing it or through other pathways mediated by antibodies such as antibody-dependent cellular cytotoxicity (Casadevall and Pirofski, 2020) by phagocytosis and complement activation. This therapy has shown encouraging results in other infectious diseases like measles, poliomyelitis, mumps, and influenza (Casadevall and Pirofski, 2020). In 2003 during the SARS-CoV-1 outbreak, convalescent plasma therapy was used to evaluate its efficiency. It resulted in significantly higher 22-day discharge in treated patients when compared to control groups (Cheng et al., 2005), but there are other studies that were inconclusive (Stockman et al., 2006). In South Korea during the MERS outbreak, two of the three patients who received this convalescent plasma were able to neutralize the antibody activity (Ko et al., 2018). Based on the promising outcome of the previous studies or experience, the US-FDA facilitated the use of convalescent plasma on compassionate grounds in critically ill COVID-19 patients (USFDA, 2020). In the case of emerging pandemics where vaccines and antiviral drugs are unavailable, the WHO has authorized the use of convalescent plasma. The study on the efficacy and safety of convalescent plasma was conducted on COVID-19 patients in United States. The preliminary results were encouraging with respect to clinical improvement as 36% of the patients’ demonstrated improvement in 7 days and 76% of the patients improved or were discharged from the hospital. Also, there were no adverse effects reported by the patients, which makes it a safe therapy for severely ill COVID-19 patients (Salazar et al., 2020). Similar results were observed in a study involving 5,000 patients, and < 1% of the adverse effects were observed during the first four days post-infusion with 14.9% mortality (seven-day incidence) (Joyner et al., 2020). In another study involving 39 severely ill COVID-19 patients, Liu et al. found that patients who got convalescent plasma had an improved survival rate in non-intubated patients than in intubated patients (Liu et al., 2020c). In an open label randomized clinical trial involving 103 severely ill COVID-19 patients given convalescent plasma, 52% of the patients showed clinical improvement in 28 days when compared to the control group with 43%. It was noted that there was no difference in the mortality rate in both groups (28 days), but there was a higher negative PCR conversion rate in the treatment group (87.2%) than the control group (37.5%) (Li et al., 2020). This study was terminated due to a decrease in COVID-19 cases. In another study with five patients, (Shen et al., 2020) reported that there were clinical improvements in the patients. However, the efficacy of the treatment could not be assessed due to the small size of the study, but these encouraging results prompt the investigator to study more rigorously the efficacy of convalescent plasma as a possible treatment. A number of clinical trials are underway, and others have been submitted to the US-FDA to test the efficacy of convalescent plasma. These studies may throw some light on the efficacy of the treatment.
Protein molecules released by the host cell in response to viral infection heighten the immune system of the host and combat the virus. Interferon alfacon-1 is a synthetic type 1 interferon with a 166 amino acid sequence synthesized by genetic engineering (Melian and Plosker, 2001). Interferon alfacon-1 is an antiviral and anticancer agent. It has a therapeutic effect on leukemia, melanoma, HIV/AIDS related Kaposi’s sarcoma, and hepatitis C (Schlossberg and Samuel, 2017). It was found to be effective in SARS-CoV and was tested for COVID-19. In a non-randomized trial, COVID-19 patients were treated with interferon in combination with corticosteroids. Patients treated with interferon alfacon-1 and steroids had a better clinical outcome than patients treated with steroids alone without interferon alfacon-1 (Loutfy et al., 2003). There are in vitro studies to show the inhibitory activity of interferon alfacon-1 on SARS-CoV-2. The result of interferon-beta-1b in a combination therapy has been encouraging and clinical trials are underway to evaluate the use of type-1 interferon as an adjunctive therapy.
Cyclosporine A (14) is an immunosuppressant that has been widely used in autoimmune disorders and transplantations. The in vitro studies have shown that cyclosporine A inhibits SARS and other coronaviruses replication. The mechanism of antiviral activity is not known, but it might be due to the inhibition of cyclophilin protein pathways including SARS-CoV. It may be beneficial for COVID-19 patients, but its use is limited due to its toxic effect. There are some studies where a small number of kidney transplant patients who changed to or continued cyclosporine A during COVID-19 treatment showed no harmful effects (Molyvdas and Matalon, 2020).
Methylprednisolone (15) is an immunosuppressive and anti-inflammatory agent approved by the US-FDA. Due to its anti-inflammatory activity at low doses, corticosteroids like methylprednisolone has been tested for a variety of viral infections, but the clinical benefits remain divisive. The use of corticosteroid in RSV infected children had no clinical benefits (Corneli et al., 2007). It also appeared to be harmful in other viral infections like hepatitis and cerebral malaria (McGee and Hirschmann, 2008). A comparative study on the effect of corticosteroids (including methylprednisolone) with a placebo involving 6,548 patients showed that corticosteroids were associated with an increase in mortality (Ni et al., 2019). A systemic review of the use of corticosteroids in SARS revealed that in four studies the use of corticosteroids was harmful including a decrease in viral clearance and drug complications while 25 other studies were inconclusive (Stockman et al., 2006). Many clinical studies on the effect of corticosteroids on viral infections showed that they have no clinical benefits, so the WHO does not recommend the use of corticosteroids in the treatment of viral pneumonia or ARDS. Regarding COVID-19, there is no convincing evidence to show that methylprednisolone has no therapeutics benefits. However, several clinical trials are underway to study the safety and efficacy.
Dexamethasone (16) is a steroid and due to its anti-inflammatory and immunosuppressant activity, it is being used in diseases like asthma, allergies, and autoimmune diseases like lupus and rheumatoid arthritis (Shrestha et al., 2019; Elkharwili et al., 2020; Wang et al., 2020b). There are no data available on the role played by dexamethasone in the cure of COVID-19 patients, but there is evidence to show that the survival and mortality rate in severely ill COVID-19 patients improves when treated with dexamethasone. Preliminary results of the randomized phase II and III clinical trials showed that dexamethasone reduced deaths in severely ill COVID-19 patients. Out of 11,320 patients, 2,104 were randomized to receive dexamethasone treatment for 10 days, 4,321 received usual cares, and the rest were given standard care. It was found that the mortality rate reduced by one third in patients with ventilation and in patients who required oxygen by one fifth. Dexamethasone had no therapeutic benefit for patients with milder cases and who did not receive oxygen support (Horby et al., 2020) . Currently, there are 14 clinical trials going on, and eight of them are expected to complete their study by 2020. The results may be beneficial in combating this pandemic in severely ill patients.
During the SARS outbreak in 2003, Chinese traditional medicine was used to treat and prevent the disease (Zhang, 2003). Traditional medicine of China was part of the prevention program to include several Chinese herbal medicine formulas to prevent infection in adults and children. It was observed that the Shufeng Jiedu and Lianhua Qingwen capsules played an important role in the prevention and treatment of influenza A (H1N1). There are also studies to show that Yu ping feng powder has an antiviral, anti-inflammatory, and immunoregulatory effect (Du et al., 2015). A large scale randomized trial found that Yinqiao powder with other heat-clearing formulas could reduce the time of a fever in patients with H1N1 virus infection (Wang et al., 2011). It is also suggested that traditional Chinese medicine may be beneficial in a high risk population that is exposed to COVID-19, such as medical staff, family members, and people who come in contact with COVID-19 patients. However, the efficacy and safety of Chinese traditional formulas on COVID-19 patients needs to be confirmed by clinical trials.
In India, Neem (Azadirachta indica L.) is considered as a traditional plant, and the leaves, seeds, bark, flowers, and roots are used to cure various diseases. The active constituent of neem, like Nimbolide has been explored for its pharmacological properties and is used in treating various diseases such as cancer, diabetes, and inflammatory diseases (Elumalai et al., 2012). In an experimental model, Nimbolide was found to inhibit TNF–α, and suppress the nuclear translocation of p65 NF-κB, HDAC-3, and the cytokine storm, so it may have some beneficial effects in the SARS-CoV-2 infection because of its antiviral activity or directly by controlling the cytokine storm. It may also have clinical significance for inflammation during a viral infection. Some of the other natural products that have been used in various diseases and that show antiviral activity against herpes virus 1 and 2 is Withaferin A found in the medicinal plant Withania sominifera from Ashwagandha (Grover et al., 2011). It may have or show encouraging results in COVID-19 treatment.
Belonging to the triterpenoids group, Saikosaponins are extracted from various plants like Scrophularia scorodonia, Heteromorpha spp, and Bupleurum spp and is known to exert antiviral activity against Corona virus 229E by inhibiting the penetration and attachment of the virus (Cheng et al., 2006). Molecular docking of Saikosaponins B4 suggests that this compound may be used as a spike protein inhibitor and could be a possible therapeutic agent in the treatment of COVID-19.
Quercetin (17) is a plant flavanol that belongs to polyphenols and is used as a pharmacological agent for inflammation and cancer (Jeong et al., 2009). It has shown antiviral activity by inhibiting viral replication and by inhibiting its entry in the Dengue virus. It also exhibited antiviral activity against SARS-CoV by inhibiting its entry into a host cell. It may have antiviral activity against SARS-CoV-2. It has been approved by the US-FDA to use it as an active ingredient in drugs. All these natural and traditional compounds or ingredients need to be clinically tested against COVID-19 for possible use in this pandemic.
Large number of monoclonal antibodies is being used as therapeutic agents and also for diagnostic purposes. USFDA has approved the use of monoclonal antibodies to treat cancer and autoimmune diseases. Few monoclonal antibodies is being used in the treatment of SARS-CoV-2, to name few Bevacizumad (NCT04305106), Tocilizumab (NCT04317092), Meplazumab (NCT04275245) etc. Tian et al. (2020) reported that the human monoclonal antibody like CR3022 that is specific to SARS-CoV has the ability to bind to the SARS-CoV-2 RBD (KD of 6.3 nM) and the epitope did not overlap with ACE2 binding sites in SARS CoV–2 RBD. Making it an clinically effective candidate for treating SARS CoV-2. In developing a new monoclonal therapeutic agent for treating SARS-CoV-2, scientists target the spike proteins, ACE2 binding sites . Large number of monoclonal antibodies is tested against SARS-CoV and these monoclonal antibodies may be effective against COVID–19 (Tian et al., 2020).
To treat autoimmune diseases like Rheumatoid arthritis and multiple myeloma, tocilizumab is used. This is a human recombinant IL-6 receptor (IL-6R) antibody and IL-6 receptors is involved in the activation of inflammatory and immunological modulators that is responsible in the respiratory collapse that is observed in the patients that are infected with COVID-19. It is also associated major side effects like allergy, liver toxicity and hyperlipidemia (Jones and Ding, 2010). At present, tocilizumab is under phase II clinical trials and is being tested for SARS-CoV-2 (NCT04317092) (Scott, 2017).
Sarilumab is another monoclonal antibody and is an IL-6 receptor that is under clinical trials for COVID–19 (NCT04315298). It is used in autoimmune disease like rheumatoid arthritis and suppresses the inflammation mediated by IL–6R. The therapeutic efficacy of the sarilumab along with remdesivir against SARS-CoV-2 patients is being tested under the clinical trials. Further clinical trials may be required to study its potential as the most effective therapeutic agent against SARS-CoV-2 (Pelechas et al., 2019; Lansdowne and Campbell, 2020).
In any given pandemic there will be a rush to develop vaccines to help the mankind. Vaccine development takes time as the vaccines must not only be protective but also safe. Unlike other drugs that are delivered into sick patients, vaccines are administered into healthy patients and require very high safety margins (Singh and Mehta, 2016). During the COVID-19 pandemic several different institute and Pharmaceutical companies are trying to develop the vaccines. As of August 11, 2020, 28 of these companies have advanced into clinical trials with Moderna, CanSino, the University of Oxford, BioNTech, Sinovac, Sinopharm, Anhui Zhifei Longcom, Inovio, Novavax, Vaxine, Zydus Cadila, Institute of Medical Biology, and the Gamaleya Research Institute having moved beyond their initial safety and immunogenicity studies (Chung et al., 2020). The vaccines are in clinical trial or in market as mentioned in Table 2. The Pfizer-BioNTech COVID-19 vaccine (BNT162b2) has not been approved or licensed by the U.S. Food and Drug Administration (FDA), but has been authorized for emergency use by FDA under an Emergency Use Authorization (EUA) to prevent Coronavirus Disease 2019 (COVID-19) for use in individuals 16 years of age and older. (www.cvdvaccine.com). As per the recent articles published indicate that the efficacy of the Pfizer vaccine was 52% after first dose and 95% after taking second dose (Mahase, 2020). The adverse drug reactions are also mentioned in the article. There were 4 deaths during the clinical trials (in the treatment and placebo group), as per the investigators these cases are not related to the vaccine. More clinical trails are in progress to launch these vaccines in the market. According to the some data the vaccines will be first available to the health care workers and people in need.
COVID-19 is causing a pandemic and there is an urgent therapeutic need to combat it. There is no potential antiviral drug or vaccine available to treat this virus. There is a global race to develop a vaccine, but in the present scenario to minimize the strain patients are given the current available therapeutics that has been used in previous viral infections or viral outbreaks. The patients are given the currently available antiviral depending on the prior antiviral experiences regarding SARS, MERS, influenza, and HIV virus, and these drugs have been evaluated in clinical studies or trials with patients. In this review, we have summarized the use of the current available drugs that have potential antiviral activity, and emphasis was given to the drugs that have circulated as having potential as an antiviral and anticipated to be beneficial in the treatment of COVID-19. However, most of the studies or the clinical trials were inconclusive or inconsistent in concluding the benefits of the repurposing of the available antiviral drugs. Some of the clinical trials are still ongoing and the results are pending which may be beneficial in treating or minimizing the symptoms of the virus. Some of the results are expected to be by the end of the year. Remdesivir proved to be beneficial from the clinical trial and has been recommended by the US-FDA for its use in the treatment of COVID-19 patients. Although some of the available antiviral have encouraging results, care must be taken and monitored clinically for the possible adverse side effects these drugs may pose. It should be kept in mind to monitor the effect of these drugs on critically ill patients or patients with ventilation and the effect it has on patients with mild symptoms or underlying conditions.
All authors listed have made a substantial, direct, and intellectual contribution to the work and approved it for publication.
The authors declare that the research was conducted in the absence of any commercial or financial relationships that could be construed as a potential conflict of interest.
Authors wish to thank Dean, Faculty of Pharmacy and Medical Sciences, Dean, Scientific Research, Al-Ahliyya Amman University, Amman Jordan for providing necessary facilities.
Abdel-Hamid, H., Oddis, C. V., and Lacomis, D. (2008). Severe hydroxychloroquine myopathy. Muscle. Nerve. 38 (3), 1206–1210. doi:10.1002/mus.21091
Agostini, M. L., Andres, E. L., Sims, A. C., Graham, R. L., Sheahan, T. P., Lu, X., et al. (2018). Coronavirus susceptibility to the antiviral remdesivir (GS-5734) is mediated by the viral polymerase and the proofreading exoribonuclease. mBio 9, e00221-18. doi:10.1128/mBio.00221-18
Amirian, E. S., and Levy, J. K. (2020). Current knowledge about the antivirals remdesivir (GS-5734) and GS-441524 as therapeutic options for coronaviruses. One Health 9, 100128. doi:10.1016/j.onehlt.2020.100128
Azeem, S., Ashraf, M., Rasheed, M. A., Anjum, A. A., and Hameed, R. (2015). Evaluation of cytotoxicity and antiviral activity of ivermectin against Newcastle disease virus. Pak. J. Pharm. Sci. 28 (2), 597–602.
Behera, P., Patro, B. K., Singh, A. K., Chandanshive, P. D., Ravi Kumar, S. R, Pradhan, S. K., et al. (2020). Role of ivermectin in the prevention of COVID-19 infection among healthcare workers in India: a matched case-control study. MedRxiv. doi:10.1101/2020.10.29.20222661
Beigel, J. H., Tomashek, K. M., Dodd, L. E., Mehta, A. K., Zingman, B. S., Kalil, A. C., et al. (2020). Remdesivir for the treatment of Covid-19-final report. N. Engl. J. Med. 383 (19), 1813–1826. doi:10.1056/NEJMoa2007764
Belouzard, S., Millet, J. K., Licitra, B. N., and Whittaker, G. R. (2012). Mechanisms of coronavirus cell entry mediated by the viral spike protein. Viruses 4 (6), 1011–1033. doi:10.3390/v4061011
Blaising, J., Polyak, S. J., and Pecheur, E. I. (2014). Arbidol as a broad-spectrum antiviral: an update. Antiviral. Res. 107, 84–94. doi:10.1016/j.antiviral.2014.04.006
Bloomberglaw.com Hundreds of virus patients allowed to try gilead’s ebola drug. Available at: https://news.bloomberglaw.com/pharma-and-life-sciences/hundreds-of-corona-patientsallowed-to-try-gileads-ebola-drug (Accessed March 27, 2020).
Borba, M., De Almeida Val, F., Sampaio, V. S., Alexandre, M. A., Melo, G. C., Brito, M., et al. (2020). Chloroquine diphosphate in two different dosages as adjunctive therapy of hospitalized patients with severe respiratory syndrome in the context of coronavirus (SARS-CoV-2) infection: preliminary safety results of a randomized, double-blinded, phase IIb clinical trial (clorocovid-19 study). MedRxiv. doi:10.1101/2020.04.07.20056424
Boriskin, Y. S., Leneva, I. A., Pecheur, E. I., and Polyak, S. J. (2008). Arbidol: a broad-spectrum antiviral compound that blocks viral fusion. Curr. Med. Chem. 15 (10), 997–1005. doi:10.2174/092986708784049658
Boulware, D. R., Pullen, M. F., Bangdiwala, A. S., Pastick, K. A., Lofgren, S. M., Okafor, E. C., et al. (2020). A randomized trial of hydroxychloroquine as postexposure prophylaxis for covid-19. N. Engl. J. Med. 383 (6), 517–525. doi:10.1056/NEJMoa2016638
Cai, Q., Yang, M., Liu, D., Chen, J., Shu, D., Xia, J., et al. (2020). Experimental treatment with favipiravir for covid-19: an open-label control study. Engineering, 6 (10), 1192–1198. doi:10.1016/j.eng.2020.03.007
Caly, L., Druce, J. D., Catton, M. G., Jans, D. A., and Wagstaff, K. M. (2020). The FDA-approved drug ivermectin inhibits the replication of SARS-CoV-2 in vitro. Antiviral Res. 178, 104787. doi:10.1016/j.antiviral.2020.104787
Camprubi, D., Almuedo-Riera, A., Marti-Soler, H., Soriano, A., Hurtado, J. C., Subira, C., et al. (2020). Lack of efficacy of standard doses of ivermectin in severe COVID-19 patients. PLoS One 15 (11), e0242184. doi:10.1371/journal.pone.0242184
Cantini, F., Niccoli, L., Matarrese, D., Nicastri, E., Stobbione, P., and Goletti, D. (2020). Baricitinib therapy in COVID-19: a pilot study on safety and clinical impact. J. Infect. 81 (2), 318–356. doi:10.1016/j.jinf.2020.04.017
Cao, B., Wang, Y., Wen, D., Liu, W., Wang, J., Fan, G., et al. (2020). A Trial of lopinavir-ritonavir in adults hospitalized with severe covid-19. N. Engl. J. Med. 382 (19), 1787–1799. doi:10.1056/NEJMoa2001282
Casadevall, A., and Pirofski, L. A. (2020). The convalescent sera option for containing COVID-19. J. Clin. Invest. 130 (4), 1545–1548. doi:10.1172/JCI138003
Chang, D., Xu, H., Rebaza, A., Sharma, L., and Dela Cruz, C. S. (2020). Protecting health-care workers from subclinical coronavirus infection. Lancet Respir. Med. 8 (3), e13. doi:10.1016/S2213-2600(20)30066-7
Chatre, C., Roubille, F., Vernhet, H., Jorgensen, C., and Pers, Y. M. (2018). Cardiac complications attributed to chloroquine and hydroxychloroquine: a systematic review of the literature. Drug. Saf. 41 (10), 919–931. doi:10.1007/s40264-018-0689-4
Chatterjee, P., Anand, T., Singh, K. J., Rasaily, R., Singh, R., Das, S., et al. (2020). Healthcare workers & SARS-CoV-2 infection in India: a case-control investigation in the time of COVID-19. Indian J. Med. Res. 151 (5), 459–467. doi:10.4103/ijmr.IJMR_2234_20
Chen, C., Zhang, Y., Huang, J., Yin, P., Cheng, Z., Wu, J., et al. (2020a). Favipiravir versus arbidol for COVID-19: a randomized clinical trial. MedRxiv. doi:10.1101/2020.03.17.20037432
Chen, H., Guo, J., Wang, C., Luo, F., Yu, X., Zhang, W., et al. (2020b). Clinical characteristics and intrauterine vertical transmission potential of COVID-19 infection in nine pregnant women: a retrospective review of medical records. Lancet 395 (10226), 809–815. doi:10.1016/S0140-6736(20)30360-3
Chen, J., Liu, D., Liu, L., Liu, P., Xu, Q., Xia, L., et al. (2020c). A pilot study of hydroxychloroquine in treatment of patients with moderate COVID-19. Zhejiang Da Xue Xue Bao Yi Xue Ban 49 (2), 215–219. doi:10.3785/j.issn.1008-9292.2020.03.03
Chen, J., Qi, T., Liu, L., Ling, Y., Qian, Z., Li, T., et al. (2020d). Clinical progression of patients with COVID-19 in Shanghai, China. J. Infect. 80 (5), e1–e6. doi:10.1016/j.jinf.2020.03.004
Chen, X., Zheng, F., Qing, Y., Ding, S., Yang, D., Lei, C., et al. (2020e). Epidemiological and clinical features of 291 cases with coronavirus disease 2019 in areas adjacent to Hubei, China: a double-center observational study. MedRxiv. doi:10.1101/2020.03.03.20030353
Chen, Z., Hu, J., Zhang, Z., Jiang, S., Han, S., Yan, D., et al. (2020f). Efficacy of hydroxychloroquine in patients with COVID-19: results of a randomized clinical trial. MedRxiv. doi:10.1101/2020.03.22.20040758
Cheng, P. W., Ng, L. T., Chiang, L. C., and Lin, C. C. (2006). Antiviral effects of saikosaponins on human coronavirus 229E in vitro. Clin. Exp. Pharmacol. Physiol. 33 (7), 612–616. doi:10.1111/j.1440-1681.2006.04415.x
Cheng, Y., Wong, R., Soo, Y. O., Wong, W. S., Lee, C. K., Ng, M. H., et al. (2005). Use of convalescent plasma therapy in SARS patients in Hong Kong. Eur. J. Clin. Microbiol. Infect. Dis. 24 (1), 44–46. doi:10.1007/s10096-004-1271-9
Choudhary, R., and Sharma, A. K. (2020). Potential use of hydroxychloroquine, ivermectin and azithromycin drugs in fighting COVID-19: trends, scope and relevance. New Microbes New Infect. 35, 100684. doi:10.1016/j.nmni.2020.100684
Chowdhury, A. T. M. M., Shahbaz, M., Karim, M. R., Islam, J., Guo, D., and He, S. (2020). A randomized trial of ivermectin-doxycycline and hydroxychloroquine-azithromycin therapy on COVID19 patients. Research Square doi:10.21203/rs.3.rs-38896/v1
Choy, K. T., Wong, A. Y., Kaewpreedee, P., Sia, S. F., Chen, D., Hui, K. P. Y., et al. (2020). Remdesivir, lopinavir, emetine, and homoharringtonine inhibit SARS-CoV-2 replication in vitro. Antiviral Res. 178, 104786. doi:10.1016/j.antiviral.2020.104786
Chung, Y. H., Beiss, V., Fiering, S. N., and Steinmetz, N. F. (2020). COVID-19 vaccine frontrunners and their nanotechnology design. ACS Nano 14 (10), 12522–12537. doi:10.1021/acsnano.0c07197
Clinicaltrials.gov (2020). Comparison with the placebo controlled (NCT04280705): a multicenter, adaptive, randomized blinded controlled trial of the safety and efficacy of investigational therapeutics for the treatment of COVID-19 in hospitalized adults. Available at: https://clinicaltrials.gov/ct2/history/NCT04280705?A=10&B=16&C=Side-by-Side (Accessed November 30, 2020).
ClinicalTrials.gov (2020a). Mild/moderate 2019-nCoV remdesivir RCT. Available at: https://clinicaltrials.gov/ct2/show/NCT04252664 (Accessed April 10, 2020).
ClinicalTrials.gov (2020b). Severe 2019-nCoV remdesivir RCT. Available at: https://clinicaltrials.gov/ct2/show/NCT04257656 (Accessed April 10, 2020).
Cohen, M. S. (2020). Hydroxychloroquine for the prevention of Covid-19-searching for evidence. N. Engl. J. Med. 383 (6), 585–586. doi:10.1056/NEJMe2020388
Coleman, C. M., and Frieman, M. B. (2014). Coronaviruses: important emerging human pathogens. J. Virol. 88 (10), 5209–5212. doi:10.1128/JVI.03488-13
Coleman, C. M., Sisk, J. M., Mingo, R. M., Nelson, E. A., White, J. M., and Frieman, M. B. (2016). Abelson kinase inhibitors are potent inhibitors of severe acute respiratory syndrome coronavirus and middle east respiratory syndrome coronavirus fusion. J. Virol. 90 (19), 8924–8933. doi:10.1128/JVI.01429-16
Corneli, H. M., Zorc, J. J., Mahajan, P., Shaw, K. N., Holubkov, R., Reeves, S. D., et al. (2007). A multicenter, randomized, controlled trial of dexamethasone for bronchiolitis. N. Engl. J. Med. 357 (4), 331–339. doi:10.1056/NEJMoa071255
Coronado, L. M., Nadovich, C. T., and Spadafora, C. (2014). Malarial hemozoin: from target to tool. Biochim. Biophys. Acta. 1840 (6), 2032–2041. doi:10.1016/j.bbagen.2014.02.009
Coronaviridae Study Group of the International Committee on Taxonomy of Viruses (2020). The species Severe acute respiratory syndrome-related coronavirus: classifying 2019-nCoV and naming it SARS-CoV-2. Nat. Microbiol. 5 (4), 536–544. doi:10.1038/s41564-020-0695-z
Costedoat-Chalumeau, N., Hulot, J.S., Amoura, Z., Leroux, G., Lechat, P., Funck-Brentano, C., et al. (2007). Heart conduction disorders related to antimalarials toxicity: an analysis of electrocardiograms in 85 patients treated with hydroxychloroquine for connective tissue diseases. Rheumatology 46 (5), 808–810. doi:10.1093/rheumatology/kel402
De Clercq, E. (2009). Anti-HIV drugs: 25 compounds approved within 25 years after the discovery of HIV. Int. J. Antimicrob. Agents 33 (4), 307–320. doi:10.1016/j.ijantimicag.2008.10.010
De Clercq, E. (2019). New nucleoside analogues for the treatment of hemorrhagic fever virus infections. Chem. Asian J. 14 (22), 3962–3968. doi:10.1002/asia.201900841
Deng, L., Li, C., Zeng, Q., Liu, X., Li, X., Zhang, H., et al. (2020). Arbidol combined with LPV/r versus LPV/r alone against Corona virus disease 2019: a retrospective cohort study. J. Infect. 81 (1), e1–e5. doi:10.1016/j.jinf.2020.03.002
Dimitrov, D. S. (2003). The secret life of ACE2 as a receptor for the SARS virus. Cell 115 (6), 652–653. doi:10.1016/s0092-8674(03)00976-0
Du, C. Y., Zheng, K. Y., Bi, C. W., Dong, T. T., Lin, H., and Tsim, K. W. (2015). Yu Ping Feng San, an ancient chinese herbal decoction, induces gene expression of anti-viral proteins and inhibits neuraminidase activity. Phytother. Res. 29 (5), 656–661. doi:10.1002/ptr.5290
Dyall, J., Coleman, C. M., Hart, B. J., Venkataraman, T., Holbrook, M. R., Kindrachuk, J., et al. (2014). Repurposing of clinically developed drugs for treatment of Middle East respiratory syndrome coronavirus infection. Antimicrob. Agents Chemother. 58 (8), 4885–4893. doi:10.1128/AAC.03036-14
Elkharwili, D. A., Ibrahim, O. M., Elazab, G. A., and Elrifaey, S. M. (2020). Two regimens of dexamethasone versus prednisolone for acute exacerbations in asthmatic Egyptian children. Eur. J. Hosp. Pharm. 27 (3), 151–156. doi:10.1136/ejhpharm-2018-001707
Elumalai, P., Gunadharini, D. N., Senthilkumar, K., Banudevi, S., Arunkumar, R., Benson, C. S., et al. (2012). Induction of apoptosis in human breast cancer cells by nimbolide through extrinsic and intrinsic pathway. Toxicol. Lett. 215 (2), 131–142. doi:10.1016/j.toxlet.2012.10.008
Fan, C., Lei, D., Fang, C., Li, C., Wang, M., Liu, Y., et al. (2020). Perinatal transmission of COVID-19 Associated SARS-CoV-2: should we worry?. Clin. Infect. Dis.ciaa226, doi:10.1093/cid/ciaa226
FDA-News (2020). Remdesivir EUA letter of authorization. Available at: https://www.fda.gov/media/137564/download (Accessed May 1, 2020).
Freedman, A., and Steinberg, V. L. (1960). Chloroquine in rheumatoid arthritis; a double blindfold trial of treatment for one year. Ann. Rheum. Dis. 19, 243–250. doi:10.1136/ard.19.3.243
Fujii, T., Nakamura, T., and Iwamoto, A. (2004). Current concepts in SARS treatment. J. Infect. Chemother. 10 (1), 1–7. doi:10.1007/s10156-003-0296-9
Gao, J., Tian, Z., and Yang, X. (2020). Breakthrough: Chloroquine phosphate has shown apparent efficacy in treatment of COVID-19 associated pneumonia in clinical studies. Biosci. Trends 14 (1), 72–73. doi:10.5582/bst.2020.01047
Gautret, P., Lagier, J. C., Parola, P., Hoang, V. T., Meddeb, L., Mailhe, M., et al. (2020a). Hydroxychloroquine and azithromycin as a treatment of COVID-19: results of an open-label non-randomized clinical trial. Int. J. Antimicrob. Agents 56 (1), 105949. doi:10.1016/j.ijantimicag.2020.105949
Gautret, P., Lagier, J. C., Parola, P., Hoang, V. T., Meddeb, L., Sevestre, J., et al. (2020b). Clinical and microbiological effect of a combination of hydroxychloroquine and azithromycin in 80 COVID-19 patients with at least a six-day follow up: a pilot observational study. Travel Med. Infect. Dis. 34, 101663. doi:10.1016/j.tmaid.2020.101663
Gordon, C. J., Tchesnokov, E. P., Woolner, E., Perry, J. K., Feng, J. Y., Porter, D. P., et al. (2020). Remdesivir is a direct-acting antiviral that inhibits RNA-dependent RNA polymerase from severe acute respiratory syndrome coronavirus 2 with high potency. J. Biol. Chem. 295 (20), 6785–6797. doi:10.1074/jbc.RA120.013679
Gotz, V., Magar, L., Dornfeld, D., Giese, S., Pohlmann, A., Hoper, D., et al. (2016). Influenza A viruses escape from MxA restriction at the expense of efficient nuclear vRNP import. Sci. Rep. 6, 23138. doi:10.1038/srep23138
Graham, R. L., Sparks, J. S., Eckerle, L. D., Sims, A. C., and Denison, M. R. (2008). SARS coronavirus replicase proteins in pathogenesis. Virus. Res. 133 (1), 88–100. doi:10.1016/j.virusres.2007.02.017
Grein, J., Ohmagari, N., Shin, D., Diaz, G., Asperges, E., Castagna, A., et al. (2020). Compassionate use of remdesivir for patients with severe Covid-19. N. Engl. J. Med. 382 (24), 2327–2336. doi:10.1056/NEJMoa2007016
Grover, A., Agrawal, V., Shandilya, A., Bisaria, V.S., and Sundar, D. (2011). Non-nucleosidic inhibition of herpes simplex virus DNA polymerase: mechanistic insights into the anti-herpetic mode of action of herbal drug withaferin A. BMC Bioinformatics 12 (13), S22. doi:10.1186/1471-2105-12-S13-S22
Guastalegname, M., and Vallone, A. (2020). Could chloroquine /hydroxychloroquine be harmful in coronavirus disease 2019 (COVID-19) treatment?. Clin. Infect. Dis. 71 (15), 888–889. doi:10.1093/cid/ciaa321
Guideline for the Prevention (2020). “Chinese clinical guidance for COVID-19 pneumonia diagnosis and treatment,” in ACC News Story. The National Health Commission of the People’s Republic of China [in Chinese].
Guo, Y. R., Cao, Q. D., Hong, Z. S., Tan, Y. Y., Chen, S. D., Jin, H. J., et al. (2020). The origin, transmission and clinical therapies on coronavirus disease 2019 (COVID-19) outbreak-an update on the status. Mil. Med. Res. 7 (1), 11. doi:10.1186/s40779-020-00240-0
Hayden, F. G., and Shindo, N. (2019). Influenza virus polymerase inhibitors in clinical development. Curr. Opin. Infect. Dis. 32 (2), 176–186. doi:10.1097/QCO.0000000000000532
Hindson, J. (2020). COVID-19: faecal-oral transmission?. Nat. Rev. Gastroenterol. Hepatol. 17 (5), 259. doi:10.1038/s41575-020-0295-7
Holshue, M. L., DeBolt, C., Lindquist, S., Lofy, K. H., Wiesman, J., Bruce, H., et al. (2020). First case of 2019 novel coronavirus in the United States. N. Engl. J. Med. 382 (10), 929–936. doi:10.1056/NEJMoa2001191
Horby, P., Lim, W. S., Emberson, J. R., Mafham, M., Bell, J. L., Linsell, L., et al. (2020). Dexamethasone in hospitalized patients with Covid-19-preliminary report. N. Engl. J. Med. doi:10.1056/NEJMoa2021436
Huang, M., Yang, Y., Shang, F., Zheng, Y., Liang, L., Wenjing, Z., et al. (2020). Early and critical care in severe patients with COVID-19 infection in Jiangsu Province, China: a descriptive study, SSRN Elect. J., doi:10.2139/ssrn.3551429
Hung, I. F., Lung, K. C., Tso, E. Y., Liu, R., Chung, T. W., Chu, M. Y., et al. (2020). Triple combination of interferon beta-1b, lopinavir-ritonavir, and ribavirin in the treatment of patients admitted to hospital with COVID-19: an open-label, randomised, phase 2 trial. Lancet 395 (10238), 1695–1704. doi:10.1016/S0140-6736(20)31042-4
Jeong, J. H., An, J. Y., Kwon, Y. T., Rhee, J. G., and Lee, Y. J. (2009). Effects of low dose quercetin: cancer cell-specific inhibition of cell cycle progression. J. Cell. Biochem. 106 (1), 73–82. doi:10.1002/jcb.21977
Jones, G., and Ding, C. (2010). Tocilizumab: a review of its safety and efficacy in rheumatoid arthritis. Clin. Med. Insights. Arthritis. Musculoskelet. Disord. 3, 81–89. doi:10.4137/CMAMD.S4864
Jorge, A., Ung, C., Young, L. H., Melles, R. B., and Choi, H. K. (2018). Hydroxychloroquine retinopathy-implications of research advances for rheumatology care. Nat. Rev. Rheumatol. 14 (12), 693–703. doi:10.1038/s41584-018-0111-8
Joyner, M. J., Wright, R. S., Fairweather, D., Senefeld, J. W., Bruno, K. A., Klassen, S. A., et al. (2020). Early safety indicators of COVID-19 convalescent plasma in 5000 patients. J. Clin. Invest. 130 (9), 4791–4797. doi:10.1172/JCI140200
Jun, C., Yun, L., Xiuhong, X., Ping, L., Feng, L., Tao, L., et al. (2020). Efficacies of lopinavir/ritonavir and abidol in the treatment of novel coronavirus pneumonia. Chin. J. Epidemiol. 38, E008. doi:10.3760/cma.j.cn311365-20200210-00050
Kang, C. K., Seong, M. W., Choi, S. J., Kim, T. S., Choe, P. G., Song, S. H., et al. (2020). In vitro activity of lopinavir/ritonavir and hydroxychloroquine against severe acute respiratory syndrome coronavirus 2 at concentrations achievable by usual doses. Korean J. Intern. Med. 35 (4), 782–787. doi:10.3904/kjim.2020.157
Khamitov, R. A., Loginova, S., Shchukina, V. N., Borisevich, S. V., Maksimov, V. A., and Shuster, A. M. (2008). Antiviral activity of arbidol and its derivatives against the pathogen of severe acute respiratory syndrome in the cell cultures. Vopr. Virusol. 53 (4), 9–13.
Knight, R. (1980). The chemotherapy of amoebiasis. J. Antimicrob. Chemother. 6 (5), 577–593. doi:10.1093/jac/6.5.577
Ko, J. H., Seok, H., Cho, S. Y., Ha, Y. E., Baek, J. Y., Kim, S. H., et al. (2018). Challenges of convalescent plasma infusion therapy in middle east respiratory coronavirus infection: a single centre experience. Antivir. Ther. 23 (7), 617–622. doi:10.3851/IMP3243
Koren, G., King, S., Knowles, S., and Phillips, E. (2003). Ribavirin in the treatment of sars: a new trick for an old drug? CMAJ 168 (10), 1289–1292.
Kujawski, S. A., Wong, K., Collins, J. P., Epstein, L., Killerby, M. E., Midgley, C. M., et al. (2020). First 12 patients with coronavirus disease 2019 (COVID-19) in the United States. MedRxiv. doi:10.1101/2020.03.09.20032896
Lansdowne, L. E., and Campbell, M. (2020). Remdesivir and sarilumab-COVID-19 clinical trials begin. Available at: https://www.technologynetworks.com/drug-discovery/blog/remdesivir-and-sarilumab-covid-19-clinical-trials-begin-332627 (Accessed March 28, 2020).
Lau, S. K., Woo, P. C., Li, K. S., Huang, Y., Tsoi, H. W., Wong, B. H., et al. (2005). Severe acute respiratory syndrome coronavirus-like virus in Chinese horseshoe bats. Proc. Natl. Acad. Sci. USA 102 (39), 14040–14045. doi:10.1073/pnas.0506735102
Lazarus, M. N., Robinson, D., Mak, V., Moller, H., and Isenberg, D. A. (2006). Incidence of cancer in a cohort of patients with primary sjogren’s syndrome. Rheumatology 45 (8), 1012–1015. doi:10.1093/rheumatology/kei281
Li, L., Zhang, W., Hu, Y., Tong, X., Zheng, S., Yang, J., et al. (2020). Effect of convalescent plasma therapy on time to clinical improvement in patients with severe and life-threatening COVID-19: a randomized clinical trial. JAMA 324 (5), 460–470. doi:10.1001/jama.2020.10044
Li, W., Moore, M. J., Vasilieva, N., Sui, J., Wong, S. K., Berne, M. A., et al. (2003). Angiotensin-converting enzyme 2 is a functional receptor for the SARS coronavirus. Nature 426 (6965), 450–454. doi:10.1038/nature02145
Lim, J., Jeon, S., Shin, H. Y., Kim, M. J., Seong, Y. M., Lee, W. J., et al. (2020). Case of the index patient who caused tertiary transmission of COVID-19 infection in Korea: the application of Lopinavir/Ritonavir for the treatment of COVID-19 infected pneumonia monitored by quantitative RT-PCR. J. Korean Med. Sci. 35 (6), e79. doi:10.3346/jkms.2020.35.e79
Lin, M. H., Moses, D. C., Hsieh, C. H., Cheng, S. C., Chen, Y. H., Sun, C. Y., et al. (2018). Disulfiram can inhibit MERS and SARS coronavirus papain-like proteases via different modes. Antiviral. Res. 150, 155–163. doi:10.1016/j.antiviral.2017.12.015
Liu, F., Xu, A., Zhang, Y., Xuan, W., Yan, T., Pan, K., et al. (2020a). Patients of COVID-19 may benefit from sustained Lopinavir-combined regimen and the increase of Eosinophil may predict the outcome of COVID-19 progression. Int. J. Infect. Dis. 95, 183–191. doi:10.1016/j.ijid.2020.03.013
Liu, J., Cao, R., Xu, M., Wang, X., Zhang, H., Hu, H., et al. (2020b). Hydroxychloroquine, a less toxic derivative of chloroquine, is effective in inhibiting SARS-CoV-2 infection in vitro. Cell Discov. 6, 16. doi:10.1038/s41421-020-0156-0
Liu, S. T. H., Lin, H.-M., Baine, I., Wajnberg, A., Gumprecht, J. P., Rahman, F., et al. (2020c). Convalescent plasma treatment of severe COVID-19: a matched control study. MedRxiv. doi:10.1101/2020.05.20.20102236
Lo, I. L., Lio, C. F., Cheong, H. H., Lei, C. I., Cheong, T. H., Zhong, X., et al. (2020). Evaluation of SARS-CoV-2 RNA shedding in clinical specimens and clinical characteristics of 10 patients with COVID-19 in Macau. Int. J. Biol. Sci. 16 (10), 1698–1707. doi:10.7150/ijbs.45357
Lodish, H., Berk, A., Zipursky, S. L., Matsudaira, P., Baltimore, D., and Darnell, J. (2000). Viruses: structure, function, and uses in molecular cell biology, New York, NY: H. Freeman & Co.
López-García, P., and Moreira, D. (2012). Viruses in biology. Evo. Edu. Outreach 5 (3), 389–398. doi:10.1007/s12052-012-0441-y
Loutfy, M. R., Blatt, L. M., Siminovitch, K. A., Ward, S., Wolff, B., Lho, H., et al. (2003). Interferon alfacon-1 plus corticosteroids in severe acute respiratory syndrome: a preliminary study. JAMA 290 (24), 3222–3228. doi:10.1001/jama.290.24.3222
Lu, H. (2020). Drug treatment options for the 2019-new coronavirus (2019-nCoV). Biosci. Trends. 14 (1), 69–71. doi:10.5582/bst.2020.01020
Lundberg, L., Pinkham, C., Baer, A., Amaya, M., Narayanan, A., Wagstaff, K. M., et al. (2013). Nuclear import and export inhibitors alter capsid protein distribution in mammalian cells and reduce venezuelan equine encephalitis virus replication. Antiviral Res. 100 (3), 662–672. doi:10.1016/j.antiviral.2013.10.004
Magagnoli, J., Narendran, S., Pereira, F., Cummings, T. H., Hardin, J. W., Sutton, S. S., et al. (2020). Outcomes of hydroxychloroquine usage in United States veterans hospitalized with COVID-19. Med. (NY) 1, 114. doi:10.1016/j.medj.2020.06.001
Mahase, E. (2020). Covid-19: Pfizer vaccine efficacy was 52% after first dose and 95% after second dose, paper shows. BMJ 371, m4826. doi:10.1136/bmj.m4826
Mahevas, M., Tran, V. T., Roumier, M., Chabrol, A., Paule, R., Guillaud, C., et al. (2020). Clinical efficacy of hydroxychloroquine in patients with covid-19 pneumonia who require oxygen: observational comparative study using routine care data. BMJ 369, m1844. doi:10.1136/bmj.m1844
Malviya, A. (2020). Ventricular arrhythmia risk due to chloroquine/hydroxychloroquine treatment for COVID-19: should it be given. Indian Heart J. 72 (2), 131–132. doi:10.1016/j.ihj.2020.04.006
Masters, P. S. (2006). The molecular biology of coronaviruses. Adv.Virus Res. 66, 193–292. doi:10.1016/S0065-3527(06)66005-3
Mastrangelo, E., Pezzullo, M., De Burghgraeve, T., Kaptein, S., Pastorino, B., Dallmeier, K., et al. (2012). Ivermectin is a potent inhibitor of flavivirus replication specifically targeting NS3 helicase activity: new prospects for an old drug. J Antimicrob. Chemother. 67 (8), 1884–1894. doi:10.1093/jac/dks147
McCreary, E. K., and Pogue, J. M. (2020). coronavirus disease 2019 treatment: a review of early and emerging options. Open Forum. Infect. Dis. 7 (4). ofaa105, doi:10.1093/ofid/ofaa105
McGee, S., and Hirschmann, J. (2008). Use of corticosteroids in treating infectious diseases. Arch. Intern. Med. 168 (10), 1034–1046. doi:10.1001/archinte.168.10.1034
Megarbane, B., and Scherrmann, J. M. (2020). Hydroxychloroquine and azithromycin to treat patients with COVID-19: both friends and foes?. J. Clin. Pharmacol. 60 (7), 808–814. doi:10.1002/jcph.1646
Mehta, P., McAuley, D. F., Brown, M., Sanchez, E., Tattersall, R. S., Manson, J. J., et al. (2020). COVID-19: consider cytokine storm syndromes and immunosuppression. Lancet 395 (10229), 1033–1034. doi:10.1016/S0140-6736(20)30628-0
Melian, E. B., and Plosker, G. L. (2001). Interferon alfacon-1: a review of its pharmacology and therapeutic efficacy in the treatment of chronic hepatitis C. Drugs 61 (11), 1661–169191. doi:10.2165/00003495-200161110-00009
Mo, P., Xing, Y., Xiao, Y., Deng, L., Zhao, Q., Wang, H., et al. (2020). Clinical characteristics of refractory COVID-19 pneumonia in Wuhan, China. Clin. Infect. Dis. ciaa 270. doi:10.1093/cid/ciaa270
Molina, J. M., Delaugerre, C., Le Goff, J., Mela-Lima, B., Ponscarme, D., Goldwirt, L., et al. (2020). No evidence of rapid antiviral clearance or clinical benefit with the combination of hydroxychloroquine and azithromycin in patients with severe COVID-19 infection. Med. Mal. Infect. 50 (4), 384. doi:10.1016/j.medmal.2020.03.006
Molyvdas, A., and Matalon, S. (2020). Cyclosporine: an old weapon in the fight against coronaviruses. Eur. Respir. J. 56 (5), 2002484. doi:10.1183/13993003.02484-2020
Nagata, T., Lefor, A. K., Hasegawa, M., and Ishii, M. (2015). Favipiravir: a new medication for the Ebola virus disease pandemic. Disaster. Med. Public Health Prep. 9 (1), 79–81. doi:10.1017/dmp.2014.151
Ni, Y. N., Chen, G., Sun, J., Liang, B. M., and Liang, Z.A. (2019). The effect of corticosteroids on mortality of patients with influenza pneumonia: a systematic review and meta-analysis. Crit. Care. 23 (1), 99. doi:10.1186/s13054-019-2395-8
NIH (2020). Chloroquine/hydroxychloroquine prevention of coronavirus disease (COVID-19) in the healthcare setting (COPCOV), Bethesda, MD: United States National Library of Medicine.
Pelechas, E., Voulgari, P. V., and Drosos, A. A. (2019). Clinical evaluation of the safety, efficacy and tolerability of sarilumab in the treatment of moderate to severe rheumatoid arthritis. Ther. Clin. Risk Manag. 15, 1073–1079. doi:10.2147/TCRM.S167452
Prasad, A., and Prasad, M. (2020). SARS-CoV-2: the emergence of a viral pathogen causing havoc on human existence. J. Genet. 99. 37, doi:10.1007/s12041-020-01205-x
Qu, J.-M., Wang, C., and Cao, B. (2020). Guidance for the management of adult patients with coronavirus disease 2019. Chin. Med. J. 133(13), 1575-1594. doi:10.1097/CM9.0000000000000899
Rajter, J. C., Sherman, M. S., Fatteh, N., Vogel, F., Sacks, J., and Rajter, J. J. (2020). ICON (Ivermectin in COvid Nineteen) study: use of ivermectin is associated with lower mortality in hospitalized patients with COVID19. MedRxiv Preprint. doi:10.1101/2020.06.06.20124461
Raoult, D (2020). Hydroxychloroquine and azithromycin versus COVID-19: grift, conspiracy theories, and another bad study by didier raoult. Available at: https://sciencebasedmedicine.org/hydroxychloroquine-and-azithromycin-versus-covid-19/ (Accessed May 3 0, 2020)
Rathi, S., Ish, P., Kalantri, A., and Kalantri, S. (2020). Hydroxychloroquine prophylaxis for COVID-19 contacts in India. Lancet Infect. Dis. 20 (10), 1118–1119. doi:10.1016/S1473-3099(20)30313-3
Ratia, K., Pegan, S., Takayama, J., Sleeman, K., Coughlin, M., Baliji, S., et al. (2008). A noncovalent class of papain-like protease/deubiquitinase inhibitors blocks SARS virus replication. Proc. Natl. Acad. Sci. USA 105 (42), 16119–16124. doi:10.1073/pnas.0805240105
Russell, B., Moss, C., George, G., Santaolalla, A., Cope, A., Papa, S., et al. (2020). Associations between immune-suppressive and stimulating drugs and novel COVID-19-a systematic review of current evidence. Ecancermedicalscience 14, 1022. doi:10.3332/ecancer.2020.1022
Sahraei, Z., Shabani, M., Shokouhi, S., and Saffaei, A. (2020). Aminoquinolines against coronavirus disease 2019 (COVID-19): chloroquine or hydroxychloroquine. Int. J. Antimicrob. Agents 55 (4), 105945. doi:10.1016/j.ijantimicag.2020.105945
Salazar, E., Perez, K. K., Ashraf, M., Chen, J., Castillo, B., Christensen, P. A., et al. (2020). Treatment of Coronavirus disease 2019 (COVID-19) patients with convalescent plasma. Am. J. Pathol 190 (8), 1680–1690. doi:10.1016/j.ajpath.2020.05.014
Sangawa, H., Komeno, T., Nishikawa, H., Yoshida, A., Takahashi, K., Nomura, N., et al. (2013). Mechanism of action of T-705 ribosyl triphosphate against influenza virus RNA polymerase. Antimicrob. Agents. Chemother. 57 (11), 5202–5208. doi:10.1128/AAC.00649-13
Savarino, A., Di Trani, L., Donatelli, I., Cauda, R., and Cassone, A. (2006). New insights into the antiviral effects of chloroquine. Lancet Infect. Dis. 6 (2), 67–69. doi:10.1016/S1473-3099(06)70361-9
Schlossberg, D., and Samuel, R. (2017). INTRON A (interferon alpha) infergen (interferon alfacon‐1) injection intron A (interferon alfa‐2a) injection, in Antibiotics manual: a guide to commonly used antimicrobials. 2nd Edn (Hoboken, NJ: John Wiley & Sons).
Schrezenmeier, E., and Dorner, T. (2020). Mechanisms of action of hydroxychloroquine and chloroquine: implications for rheumatology. Nat. Rev. Rheumatol. 16 (3), 155–166. doi:10.1038/s41584-020-0372-x
Scott, L. J. (2017). Tocilizumab: a review in rheumatoid arthritis. Drugs 77 (17), 1865–1879. doi:10.1007/s40265-017-0829-7
Shah, A., Kashyap, R., Tosh, P., Sampathkumar, P., and O’Horo, J. C. (2020). Guide to understanding the 2019 novel coronavirus. Mayo. Clin. Proc. 95 (4), 646–652. doi:10.1016/j.mayocp.2020.02.003
Shen, C., Wang, Z., Zhao, F., Yang, Y., Li, J., Yuan, J., et al. (2020). Treatment of 5 critically Ill patients with COVID-19 with convalescent plasma. JAMA 323 (16), 1582–1589. doi:10.1001/jama.2020.4783
Shrestha, R., Kharel Sitaula, R., Karki, P., Joshi, S.N., and Rawal, S. (2019). Combined intravitreal bevacizumab and dexamethasone in bilateral lupus retinopathy. Int. Med. Case Rep. J. 12, 329–333. doi:10.2147/IMCRJ.S220499
Simmons, G., Reeves, J. D., Rennekamp, A. J., Amberg, S. M., Piefer, A. J., and Bates, P. (2004). Characterization of severe acute respiratory syndrome-associated coronavirus (SARS-CoV) spike glycoprotein-mediated viral entry. Proc. Natl. Acad. Sci. USA 101 (12), 4240–4245. doi:10.1073/pnas.0306446101
Singh, K., and Mehta, S. (2016). The clinical development process for a novel preventive vaccine: an overview. J. Postgrad. Med. 62 (1), 4–11. doi:10.4103/0022-3859.173187
Srinivasa, A., Tosounidou, S., and Gordon, C. (2017). Increased incidence of gastrointestinal side effects in patients taking hydroxychloroquine: a brand-related issue? J. Rheumatol. 44 (3), 398. doi:10.3899/jrheum.161063
Stockman, L.J., Bellamy, R., and Garner, P. (2006). SARS: systematic review of treatment effects. PLoS Med. 3 (9), e343. doi:10.1371/journal.pmed.0030343
Taccone, F. S., Gorham, J., and Vincent, J. L. (2020). Hydroxychloroquine in the management of critically ill patients with COVID-19: the need for an evidence base. Lancet Respir. Med. 8 (6), 539–541. doi:10.1016/S2213-2600(20)30172-7
Tai, D. Y. (2007). Pharmacologic treatment of SARS: current knowledge and recommendations. Ann. Acad. Med. Singap. 36 (6), 438–443.
Tang, W., Cao, Z., Han, M., Wang, Z., Chen, J., Sun, W., et al. (2020). Hydroxychloroquine in patients with mainly mild to moderate coronavirus disease 2019: open label, randomised controlled trial. BMJ 369, m1849. doi:10.1136/bmj.m1849
Tian, X., Li, C., Huang, A., Xia, S., Lu, S., Shi, Z., et al. (2020). Potent binding of 2019 novel coronavirus spike protein by a SARS coronavirus-specific human monoclonal antibody. Emerg. Microbes. Infect. 9 (1), 382–385. doi:10.1080/22221751.2020.1729069
Ursing, J., Rombo, L., Eksborg, S., Larson, L., Bruvoll, A., Tarning, J., et al. (2020). High-dose chloroquine for uncomplicated plasmodium falciparum malaria is well tolerated and causes similar QT interval prolongation as standard-dose chloroquine in children. Antimicrob. Agents Chemother. 64 (3). e01846–e01919. doi:10.1128/AAC.01846-19
USFDA (2020). US food and drug administration. recommendations for investigational COVID-19 convalescent plasma. NH: U. S.Food and Drug Administration.
Vercruysse, J., and Rew, R.S. (2002). Macrocyclic lactones in antiparasitic therapy. Oxon, United Kingdom: CABI Publication.
Vincent, M. J., Bergeron, E., Benjannet, S., Erickson, B.R., Rollin, P.E., Ksiazek, T.G., et al. (2005). Chloroquine is a potent inhibitor of SARS coronavirus infection and spread. Virol. J. 2, 69. doi:10.1186/1743-422X-2-69
Wang, C., Cao, B., Liu, Q. Q., Zou, Z. Q., Liang, Z. A., Gu, L., et al. (2011). Oseltamivir compared with the Chinese traditional therapy maxingshigan-yinqiaosan in the treatment of H1N1 influenza: a randomized trial. Ann. Intern. Med. 155 (4), 217–225. doi:10.7326/0003-4819-155-4-201108160-00005
Wang, M., Cao, R., Zhang, L., Yang, X., Liu, J., Xu, M., et al. (2020a). Remdesivir and chloroquine effectively inhibit the recently emerged novel coronavirus (2019-nCoV) in vitro. Cell Res. 30 (3), 269–271. doi:10.1038/s41422-020-0282-0
Wang, Q., Xu, B., Fan, K., Wu, J., and Wang, T. (2020b). Inflammation suppression by dexamethasone via inhibition of CD147-mediated NF-kappaB pathway in collagen-induced arthritis rats. Mol. Cell Biochem. 473 (1-2), 63–76. doi:10.1007/s11010-020-03808-5
Wang, Y., Zhang, D., Du, G., Du, R., Zhao, J., Jin, Y., et al. (2020c). Remdesivir in adults with severe COVID-19: a randomised, double-blind, placebo-controlled, multicentre trial. Lancet 395 (10236), 1569–1578. doi:10.1016/S0140-6736(20)31022-9
Weiss, S. R., and Navas-Martin, S. (2005). Coronavirus pathogenesis and the emerging pathogen severe acute respiratory syndrome coronavirus. Microbiol. Mol. Biol. Rev. 69 (4), 635–664. doi:10.1128/MMBR.69.4.635-664.2005
WHO (2017). “The cardiotoxicity of antimalarials,” in Proceedings of the WHO evidence review group meeting, Geneva, Switzerland, October13–14 (World Health Organization), 2016.
WHO (2020). Modes of transmission of virus causing COVID-19: implications for IPC precaution recommendations: Scientific brief. Geneva, Switzerland: World Health Organization.
Williamson, B. N., Feldmann, F., Schwarz, B., Meade-White, K., Porter, D. P., Schulz, J., et al. (2020). Clinical benefit of remdesivir in rhesus macaques infected with SARS-CoV-2. bioRxiv. doi:10.1101/2020.04.15.043166
Wu, C., Liu, Y., Yang, Y., Zhang, P., Zhong, W., Wang, Y., et al. (2020). Analysis of therapeutic targets for SARS-CoV-2 and discovery of potential drugs by computational methods. Acta. Pharm. Sin. B 10 (5), 766–788. doi:10.1016/j.apsb.2020.02.008
Yan, D., Liu, X. Y., Zhu, Y. N., Huang, L., Dan, B. T., Zhang, G. J., et al. (2020). Factors associated with prolonged viral shedding and impact of lopinavir/ritonavir treatment in hospitalised non-critically ill patients with SARS-CoV-2 infection. Eur. Respir. J. 56 (1). doi:10.1183/13993003.00799-2020
Yan, Y., Zou, Z., Sun, Y., Li, X., Xu, K. F., Wei, Y., et al. (2013). Anti-malaria drug chloroquine is highly effective in treating avian influenza A H5N1 virus infection in an animal model. Cell Res. 23 (2), 300–302. doi:10.1038/cr.2012.165
Yao, X., Ye, F., Zhang, M., Cui, C., Huang, B., Niu, P., et al. (2020). In vitro antiviral activity and projection of optimized dosing design of hydroxychloroquine for the treatment of severe acute respiratory syndrome coronavirus 2 (SARS-CoV-2). Clin. Infect. Dis. 71 (15), 732–739. doi:10.1093/cid/ciaa237
Yeung, K. S., Yamanaka, G. A., and Meanwell, N. A. (2006). Severe acute respiratory syndrome coronavirus entry into host cells: opportunities for therapeutic intervention. Med. Res. Rev. 26 (4), 414–433. doi:10.1002/med.20055
Zhang, S. P. (2003). WHO says SARS outbreak is over, but fight should go on. BMC 327, 70. doi:10.1136/bmj.327.7406.70-c
Keywords: COVID-19, hydroxychlorquine, chloroquine, lopinavir/ritonavir, remdesivir, favipiravir, arbidol, ivermectin
Citation: Naik RR and Shakya AK (2021) Therapeutic Strategies in the Management of COVID-19. Front. Mol. Biosci. 7:636738. doi: 10.3389/fmolb.2020.636738
Received: 01 December 2020; Accepted: 30 December 2020;
Published: 04 February 2021.
Edited by:
Balakumar Chandrasekaran, Philadelphia University, JordanReviewed by:
Kuldeep Singh, Integral University, IndiaCopyright © 2021 Naik and Shakya. This is an open-access article distributed under the terms of the Creative Commons Attribution License (CC BY). The use, distribution or reproduction in other forums is permitted, provided the original author(s) and the copyright owner(s) are credited and that the original publication in this journal is cited, in accordance with accepted academic practice. No use, distribution or reproduction is permitted which does not comply with these terms.
*Correspondence: Rajashri R. Naik, cnNoYXJyeUBhbW1hbnUuZWR1Lmpv
†These authors have contributed equally to this work and share first authorship
Disclaimer: All claims expressed in this article are solely those of the authors and do not necessarily represent those of their affiliated organizations, or those of the publisher, the editors and the reviewers. Any product that may be evaluated in this article or claim that may be made by its manufacturer is not guaranteed or endorsed by the publisher.
Research integrity at Frontiers
Learn more about the work of our research integrity team to safeguard the quality of each article we publish.