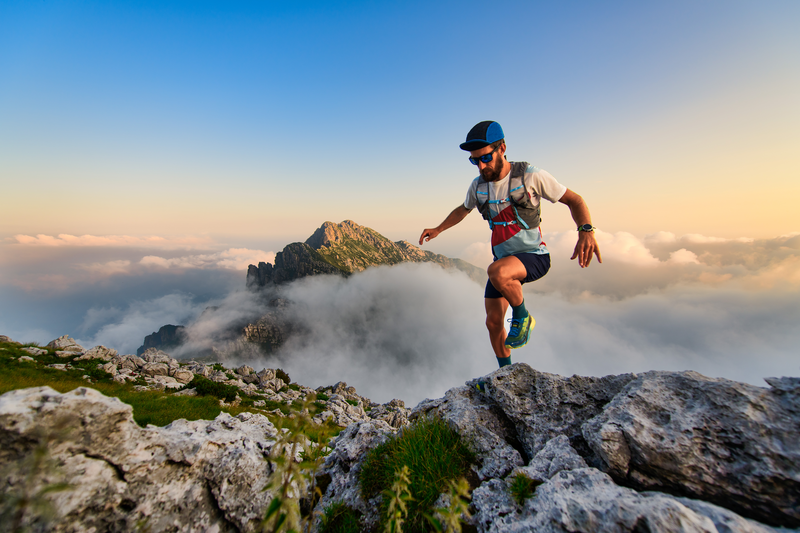
95% of researchers rate our articles as excellent or good
Learn more about the work of our research integrity team to safeguard the quality of each article we publish.
Find out more
REVIEW article
Front. Mol. Biosci. , 22 December 2020
Sec. Molecular Diagnostics and Therapeutics
Volume 7 - 2020 | https://doi.org/10.3389/fmolb.2020.606779
This article is part of the Research Topic Small Molecules and Peptide-Based Candidates as Therapeutics and Vaccines for COVID-19 Pandemic View all 31 articles
COVID-19 has resulted in a pandemic after its first appearance in a pneumonia patient in China in early December 2019. As per WHO, this global outbreak of novel COVID-19 has resulted in 28,329,790 laboratory-confirmed cases and 911,877 deaths which have been reported from 210 countries as on 12th Sep 2020. The major symptoms at the beginning of COVID-19 are fever (98%), tussis (76%), sore throat (17%), rhinorrhea (2%), chest pain (2%), and myalgia or fatigue (44%). Furthermore, acute respiratory distress syndrome (61.1%), cardiac dysrhythmia (44.4%), shock (30.6%), hemoptysis (5%), stroke (5%), acute cardiac injury (12%), acute kidney injury (36.6%), dermatological symptoms with maculopapular exanthema (36.1%), and death can occur in severe cases. Even though human coronavirus (CoV) is mainly responsible for the infections of the respiratory tract, some studies have shown CoV (in case of Severe Acute Respiratory Syndrome, SARS and Middle East Respiratory Syndrome, MERS) to possess potential to spread to extra-pulmonary organs including the nervous system as well as gastrointestinal tract (GIT). Patients infected with COVID-19 have also shown symptoms associated with neurological and enteric infection like disorders related to smell/taste, loss of appetite, nausea, emesis, diarrhea, and pain in the abdomen. In the present review, we attempt to evaluate the understanding of basic mechanisms involved in clinical manifestations of COVID-19, mainly focusing on interaction of COVID-19 with gut-brain axis. This review combines both biological characteristics of the virus and its clinical manifestations in order to comprehend an insight into the fundamental potential mechanisms of COVID-19 virus infection, and thus endorse in the advancement of prophylactic and treatment strategies.
There have been three outbreaks of human pathogenic coronavirus (CoV) in the 21st century namely, severe acute respiratory syndrome CoV (SARS-CoV) in 2003, Middle East respiratory syndrome CoV (MERS-CoV) in 2012 and new CoV disease 2019 (COVID-19) (first reported in China in 2019), causing transmission globally, which has caused global public health problems and economic development related challenges (Stadler et al., 2003; Cui et al., 2019; Lu et al., 2020; Memish et al., 2020; Wu et al., 2020a; Zhou et al., 2020a). The COVID-19 pandemic has resulted in 28,329,790 laboratory-confirmed cases and 911,877 deaths across 210 countries as on 12th Sep 2020, as per World Health Organization (WHO) (Organization and Organization, 2020). With the help of electron microscopy, it has been found that the virus possesses an envelope with round or oval particles called spikes (60–140 nm diameter) (Ahn et al., 2020; Fan et al., 2020). The genome sequences of novel CoV-2019 were related to SARS-CoV belonging to beta-CoV family and it also showed ~79% and ~50% similarity to SARS-CoV and MERS-CoV, respectively (Lu et al., 2020). Although SARS-CoV-2 is known to be infectious with a basic reproduction number (R0) of 3.77 (SARS, R0 = 3–5) (Li et al., 2020b; Yang et al., 2020b), most of the cases are mild and have a low mortality rate except among older patients (≥60 years of age) and patients with pre-existing comorbidities like hypertension, diabetes mellitus, and cardiovascular diseases (Baghizadeh Fini, 2020; Chen et al., 2020; Contini et al., 2020). It has been observed that many patients suffering from SARS CoV-2 infection are also associated with gastrointestinal and neurological symptoms. In SARS CoV infections, the intestinal inflammation is mainly modulated by the ACE2 receptor mediated mechanisms (that mainly regulate the gut-microbial flora) and the similar mechanism may be implicated in SARS CoV-2 infections as well (Yang et al., 2020a). Disturbances of gut-microbial flora may be a factor behind the CNS symptoms like confusion and delirium. The present review is an attempt to underpin the possible interplay between COVID-19, ACE2 and CNS and GIT symptoms.
Relevant studies were retrieved from Science Direct, Medline, Public Library of Science, Mendeley, PubMed, Springer Link, and Google Scholar. We used multiple keywords like “microbiota,” “microbiome,” “microbial communities,” “gut-microbiota,” “SARS-CoV-2 infection,” “COVID-19 infection,” “Pathogenesis of COVID-19 infection,” “SARS CoV-2 transmission,” “SARS-CoV-2 mediated gastrointestinal infection,” “COVID-19 infection associated psychotic problems,” “COVID-19 infection associated cerebrovascular changes,” “Relationship of gut microbiota with brain” or “COVID-19 mediated inflammatory response,” in combination with “Cytokine storm,” “Immunogenic profile,” “SARS-CoV-2 mediated dysbiosis and dysbiosis altered mRNA profile,” “Immunomodulatory response changes brain physiology” individually and in combination for literature search. The overall method of article screening and selection criteria are depicted in Figure 1. The articles in English language are used to compile the information. We also screened the reference list of the articles retrieved in order to find articles that remained unidentified by initial search strategy.
As per WHO, COVID-19 is the third episode of spillover of an animal CoV to humans in the last 20 years that has caused major epidemic (Yang et al., 2020b). The main route of transmission of SARS-CoV-2 is through aerosolized droplets (like other CoV), additionally, the infection may also be transmitted through direct physical contact, mother-to-child (García, 2020; Harapan et al., 2020). The nucleic acid of SARS-CoV-2 has also been found in fecal specimens that suggest the potentiality of the gastrointestinal tract (GIT) in the transmission, even though it requires further studies in order to consider it as an established fact (Holshue et al., 2020). The incubation period of SARS-CoV-2 continues to be 5–14 days as per a retrospective study (Lu et al., 2020); however, a more recent study suggests the incubation period to be 24 days (Izzetti et al., 2020). At the beginning of COVID-19 pandemic, the main symptoms were fever (98%), tussis (76%), sore throat (17%), rhinorrhea (2%), chest pain (2%), and myalgia or fatigue (44%). Further, acute respiratory distress syndrome (61.1%), cardiac dysrhythmia (44.4%), shock (30.6%), hemoptysis (5%), stroke (5%), acute cardiac injury (12%), acute kidney injury (36.6%), dermatological symptoms with maculopapular exanthema (36.1%) and death can occur in severe cases (Figure 2) (Jiang et al., 2020; Kandeel and Al-Nazawi, 2020).
Although human CoV leads to infections of the respiratory tract, some studies have also demonstrated its potentiality to spread into extra-pulmonary organs including the nervous system and the GIT, as observed in cases of SARS and MERS (Kannan et al., 2020). As per recent reports, COVID-19 patients have also presented with neurological and enteric infection related symptoms such as disorders related to smell/taste, loss of appetite, nausea, emesis, pain in the abdomen, and diarrhea (Kotwani and Gandra, 2020; Lake, 2020).
Pharmacologically, enough evidence has been collected to establish the involvement of several important pathological molecular mechanisms in the spread of SARS-CoV-2 via aerosolized droplets into the lungs. The genome sequence of SARS-CoV-2 consists of 29 kb bases and 12 protein-coding regions (1ab, 1a, S, 3a, 4, M, 6, 7a, 7b, 8, N, 10) (Walls et al., 2020). Spike (S) protein mediates the entry of SARS-CoV-2 into the host cells. This protein comprises of two functional subunits–S1 (for attaching with the host cell) and S2 (helps in the fusion with the cellular membranes) (Li et al., 2020,a). The protein S of many CoVs are separated at the border between S1 and S2 subunits, which stay bound non-covalently in prefusion conformation (Walls et al., 2020). The S1 subunit (distal) carries receptor-binding domain and helps in stabilizing the prefusion state of membrane-anchored S2 subunit, which also acts as receptor for fusion (Liu et al., 2020a,b). The protein S for all CoVs is further cleaved by proteases of the host at the S2′ position, which is situated upstream to the fusion peptide. Hence, entry of CoV into the cells that are susceptible is a harmonious and complex process, which needs an intensive mechanism of binding of receptors and the proteolytic dispensation of the protein S that helps in the promotion of virus-cell fusion (Liu et al., 2020c). The most common entry mechanism of a virus into the host cell is via endocytosis, which is a receptor-mediated process. It is believed that the receptor, angiotensin-converting enzyme-2 (ACE2; protein found on surface of cells), and TMPRSS2 (a gene that encode for transmembrane protease enzyme and belong to serine protease family) are expressed in lung tissues and epithelial cells, which are employed by CoV to establish infection in the lungs (Ozma et al., 2020; Porfidia and Pola, 2020). There is also a known fact that ACE2 receptors are expressed on heart, esophageal, ileal, renal tissues at a level higher than that in the alveolar cells. This suggests the involvement of multi-organ systems in severe SARS-CoV-2 infection (Rico-Mesa et al., 2020). The virus binds with ACE2 receptors (present on the plasma membranes of the epithelial cells) of host cells particularly located on the respiratory tract and upper esophagus (Shanmugaraj et al., 2020). The SARS-CoV-2 can also infect cell types like stratified epithelial cells and enterocytes of colon and ileum. In addition, virus also infects cholangiocytes, proximal tubule cells of nephrons, urothelial cells of the urinary bladder, and myocardial cells of heart (Shen et al., 2020). It is known that the in vitro isolation of SARS-CoV-2 usually require 6 days in cell lines like Vero-E6 and Huh-7, but virus infects the epithelial cells lines within 96 h. However, the SARS-CoV-2 does not affect T cells, CD4 or ACE2 cells (Sifuentes-Rodríguez and Palacios-Reyes, 2020) and thus not damaging the immune systems. Moreover, nucleotide sequencing analysis revealed seven major genetic variations occurred in SARS CoV-2 indicating that the ongoing human infection may be a recent incident. These genetic variations may change the virus to remerge as more virulent to infect digestive, circulatory, urogenital, and central nervous system apart from respiratory tract infections (Hussain et al., 2020).
The clinical characteristics as well as the epidemiology of disorders related to GIT in COVID-19 patients has been analyzed by various researchers. Different studies report that various percentage of COVID-19 patients were affected by GIT related disorders like emesis, nausea, and diarrhea. In one study, a total of 651 COVID-19 patients enrolled before they were treated with antivirals or antibiotics and it was observed that 11.4% of the patients suffered from at least one common symptom related to the GIT namely diarrhea (Jin et al., 2020). In this study, the disorders related to intestines persisted for a median of 4 days and preceded symptoms related to the respiratory system. Symptoms related to GIT were comparatively higher among critically ill COVID-19 patients (23%) than in mild COVID-19 cases (8%) (Sohrabi et al., 2020). Several studies involving varying numbers of COVID-19 patients (n = 254, 59, 204, 58, 138, and 1099) suggested development of disorders related to the GIT in 26, 25.4, 18.6, 11, 13.7, and 8.7% of the COVID-19 patients, respectively (Borah et al., 2020; Cheung et al., 2020; Lin et al., 2020; Pan et al., 2020; Wang et al., 2020a; Zhou et al., 2020b). Other studies carried out in the United States and Europe enrolling varying number of patients (n = 278, 318, and 40) showed GIT related signs and symptoms in 35, 61, and 55% patients, respectively. The pooled data of prevalence of GIT symptoms including anorexia (26.8%), diarrhea (12.5%), nausea/emesis (10.2%), and pain/discomfort in the abdomen was seen in a meta-analysis study that included 4243 COVID-19 patients across 6 countries (Tian et al., 2020). SARS-CoV and SARS-CoV-2 have nearly 80% resemblance and hence, infections of GIT that mainly mediated through ACE2 receptors was expected. ACE2 receptors are expressed in enterocytes of the small intestine, and its rate of binding affinity influences the rate of infectivity of the virus. In addition, in comparison with the previous SARS-CoV outbreaks, SARS-CoV-2 RNA was detectable in the stool samples though it was not found in the respiratory tract (Velavan and Meyer, 2020).
In SARS-CoV infections, the intestinal inflammation is mainly modulated by ACE2 receptor mediated mechanism and therefore, its disruption by SARS-CoV-2 may result in diarrhea and other GIT related complications. Recruiting inflammatory cells from the bone marrow, systemic or local production of inflammatory cytokines like interleukin (IL)-6, tumor necrosis factor-alpha (TNF-α), IL-1β, 2,7,8,9,10,17, monocyte chemotactic protein 1 (MCP1), macrophage inflammatory protein 1A (MIP1A), macrophage inflammatory protein 1B (MIP1B), macrophage inflammatory protein 3A (MIP3A), elevated levels of procalcitonin, increased levels of ferritin d-dimer, C-reactive protein (CRP), and activating JAK-STAT protein may also contribute in the etiology of the GIT complications (Wang et al., 2020b; Wu et al., 2020b). Further, the excessive secretion of cytokines (cytokine storm) along the GIT wall may result in tissue damage. This cellular damage may also be induced by viral replication, which might impart in the development of injury and inflammation of the gut epithelium. These inflammatory cytokines stimulate the vagus nerve to promote nausea, vomiting, and diarrhea that usually occur in complicated cases of COVID-19 infections (Wu et al., 2020c). A recent study carried out on complicated cases of COVID-19 revealed that infection with SARS-CoV-2 could alter the gut microbiota. This study reveals relatively increased cases of opportunistic infections of GIT that mainly caused by Streptococcus, Rothia, Veillonella, Erysipelothrix, Clostridium, and Actinomyces species in COVID-19 patients (Xiao et al., 2020).
The ACE2 receptors in the intestines are known to act as co-receptors for the intake of the nutrients including amino acid (AA) from food. Apart from controlling dietary AA homeostasis, ACE2 helps in regulating the development of gut-microbiota and innate immunity mechanisms (Yang et al., 2020a). Earlier reports reveals that the expression of AA transporter protein B0AT1 was not present in the small intestine of ACE2 mutant mice. Due to lack of expression of B0AT1 proteins in the intestines, the serum concentration of non-essential AA valine (Val), threonine (Thr), and tyrosine (Tyr) and the essential AA tryptophan (Trp) were significantly decreased in Ace2−/y mice (Ye et al., 2020). Further, these AAs (particularly Trp) acts as precursors for the synthesis of monoamine neurotransmitters serotonin [5-hydroxytyphtophan (5-HT)] and catecholamines in the brain. Furthermore, the rate of production of 5-HT and catecholamines was directly associated with their local substrate availability in the brain (Yu et al., 2020). This might further result in reduction in the production of 5-HT and catecholamines. Therefore, severe COVID-19 patients with GIT related complications might develop pathophysiological conditions like depression, delirium, and confusion.
The gut-brain axis is a two-way system that connects cognitive centers of the brain with peripheral working of the digestive tract (Figure 3). It is evident that changes in gut microbiota may have influence on the development of behavioral changes like depression, delirium/confusion. This is in turn most likely associated with the rate of absorption of Trp from the GIT and production of 5-HT in the brain tissues. (Yu et al., 2020). The gut microbiota or its metabolic end products stimulates vagus nerve in order to transmit the impulses to the solitary nucleus that also function as primary GIT sensory relay station, and then to the most vital centers such as thalamus, hypothalamus, locus ceruleus, amygdala, and periaqueductal gray (Al Omran and Aziz, 2014). These electrical impulses produced in the vagus nerve (as a result of stimulation of gut microbiota) will have an effect on 5-HT concentration in the brain tissues of both rodents and humans (Ressler and Mayberg, 2007). The gut microbiota influence the various neurotransmitter levels by stimulating the central nervous system and the gut via the synthesis of metabolites (Galland, 2014; Evrensel and Ceylan, 2015). These metabolites are the end products of normal bactrial flora of the GIT, which include short-chain fatty acids (SCFAs), bile acids, choline metabolites, lactate, and vitamins. These metabolites can directly or indirectly regulate the production of neurotransmitters (Galland, 2014). Immune cells and inflammatory mediators play various functions in the gut-brain axis communication ranging from physiological role in sleep and memory to pathophysiological role in neuropsychiatric conditions (Caspani et al., 2018). Litratures indicate that the patients with depression usually possess high concentrations of pro-inflammatory cytokines (for instance; IL-6, and TNF-α) as compared with normal individuals (Dantzer et al., 2008; Dowlati et al., 2009). These pro-inflammatory cytokines in the GIT may stiumulate vagus nerve in order to modulate central stress circuitry followed by activation of hypothalamic–pituitary–adrenal axis (Sternberg, 2006). Further, it is also known that patients suffering from depression may have abundance of Streptococcus, Rothia, Veillonella, Erysipelothrix, Clostridium, and Actinomyces species in their gut microbiota (Amirkhanzadeh Barandouzi et al., 2020) and it is established that an increased growth of Streptococcus species would raise the levels of IL-6 and TNF-α in humans (Jiang et al., 2015). This fact has been further supported by various studies wherein higher concentrations of pro-inflammatory cytokines (such as IL-1β and IL-6) and low concentrations of anti-inflammatory cytokines (like IL-4 and IL-10) were detected in patients enduring with depression (Berk et al., 2013; Wong et al., 2016). Additionally, the intestinal concentrations of 5-HT are retained by the enterochromaffin cells that possess tryptophan hydroxylase and stimulated by the metabolites of the gut like SCFA and bile acids (Annweiler et al., 2020; Bobker and Robbins, 2020). Moreover, it has been assumed that a reduction in levels of Trp might result in a decrease of 5-HT, which may lead to delirium development (Gunther et al., 2008). An increase in the levels of dopamine and development of delirium are inter-linked. Also, dopamine is linked to various metabolic pathways and Ca2+ channels which result in a marked elevation in the dopamine under an impaired oxidative condition. The Ca2+ influx in the cells results in an elevated dopamine production which results in uncoupling of oxidative phosphorylation mainly occur in the mitochondria of the brain parenchymal cells (Calcagno et al., 2020). The result is an elevated production of metabolites of dopamine that are toxic along with a decreased ATP production, which inhibits the catechol-O-methyl transferase (COMT) activity, an important enzyme that aids dopamine synthesis and breakdown in the prefrontal cortex (Gunther et al., 2008; Maldonado, 2008; Kamholz, 2010).
Figure 3. Organotropism of SARS CoV-2 associated pathogenic relationship of gut-brain axis and psychotic illness.
On the other hand, recently, a study showed resistance of norepinephrine (NE) transporter (NET) knockout mice toward depression-like behavioral changes, which are stress induced, as well as expression of brain neurotrophin which are seen in normal mice. Hence, depression and delirium can be caused due to reduced levels of NE and elevated concentrations of dopamine (Coleman et al., 2020; Das et al., 2020). Besides, CRP may lead to the stimulation of the formation of reactive oxygen species (ROS) which result in blood brain barrier disruption, thereby causes delirium. As per studies, a higher occurrence of delirium in post-operative hip surgery patients is associated with higher concentrations of CRP and IL-6 (Beloosesky et al., 2004; Macdonald et al., 2007). A prospective study was conducted wherein they measured the inflammatory biomarkers procalcitonin, and CRP in patients ventilated mechanically (McGrane et al., 2011). An association was found between delirium, less coma-free days, elevated levels of CRP and procalcitonin, which implicated inflammation as a vital mechanism in delirium pathophysiology.
In nutshell, there is a need to pay attention to patients presenting initial symptoms related to the digestive system particularly diarrhea during the diagnosis and management of SARS-CoV-2. In addition, on the occurrence of diarrhea or other related symptoms during treatment, the patient should receive a prompt integrative surveillance of psychotic results. Thus, the treatment regimen should include anti-diarrhea therapy along with the probiotics (necessary for reactivation of beneficial intestinal microbiota) that will help in reducing the psychotic consequences of the central nervous system. In addition to antiviral intervention, the treatment regimen should include rejuvenating electrolytes along with sufficient water intake. Further, future studies are required to obtain insights into association between SARS-CoV-2 and the gut-brain axis.
PS: conceptualization, writing—original draft, and funding acquisition. MM and AN: supervision and writing—review and editing. PD: conceptualization and writing—review and editing. MG, JS, and SK: writing—review and editing. All authors contributed to the article and approved the submitted version.
This project was funded by the Deanship of Scientific Research, King Faisal University, Saudi Arabia (Project No. 180009).
The authors declare that the research was conducted in the absence of any commercial or financial relationships that could be construed as a potential conflict of interest.
The authors gratefully acknowledge the support of Deanship of Scientific Research, King Faisal University, Saudi Arabia (Project No. 180009).
Ahn, D. G., Shin, H. J., Kim, M. H., Lee, S., Kim, H. S., Myoung, J., et al. (2020). Current status of epidemiology, diagnosis, therapeutics, and vaccines for novel coronavirus disease 2019 (COVID-19). J. Microbiol. Biotechnol. 30, 313–324. doi: 10.4014/jmb.2003.03011
Al Omran, Y., and Aziz, Q. (2014). The brain-gut axis in health and disease. Adv. Exp. Med. Biol. 817, 135–153. doi: 10.1007/978-1-4939-0897-4_6
Amirkhanzadeh Barandouzi, Z., Starkweather, A., Henderson, W., Gyamfi, A., and Cong, X. (2020). Altered composition of gut microbiota in depression: a systematic review. Front. Psychiatry 11:541. doi: 10.3389/fpsyt.2020.00541
Annweiler, C., Cao, Z., Wu, Y., Faucon, E., Mouhat, S., Kovacic, H., et al. (2020). Counter-regulatory ‘Renin-Angiotensin’ system-based candidate drugs to treat COVID-19 diseases in SARS-CoV-2-infected patients. Infect. Disord. Drug Targets 20, 407–408. doi: 10.2174/1871526520666200518073329
Baghizadeh Fini, M. (2020). What dentists need to know about COVID-19. Oral Oncol. 105:104741. doi: 10.1016/j.oraloncology.2020.104741
Beloosesky, Y., Grinblat, J., Pirotsky, A., Weiss, A., and Hendel, D. (2004). Different C-reactive protein kinetics in post-operative hip-fractured geriatric patients with and without complications. Gerontology 50, 216–222. doi: 10.1159/000078350
Berk, M., Williams, L. J., Jacka, F. N., O'neil, A., Pasco, J. A., Moylan, S., et al. (2013). So depression is an inflammatory disease, but where does the inflammation come from? BMC Med. 11:200. doi: 10.1186/1741-7015-11-200
Bobker, S. M., and Robbins, M. S. (2020). COVID-19 and headache: a primer for trainees. Headache 60, 1806–1811. doi: 10.1111/head.13884
Borah, P., Deb, P. K., Deka, S., Venugopala, K. N., Singh, V., Mailavaram, R. P., et al. (2020). Current scenario and future prospect in the management of COVID-19. Curr. Med. Chem. doi: 10.2174/0929867327666200908113642
Calcagno, N., Colombo, E., Maranzano, A., Pasquini, J., Keller Sarmiento, I. J., Trogu, F., et al. (2020). Rising evidence for neurological involvement in COVID-19 pandemic. Neurol. Sci. 41, 1339–1341. doi: 10.1007/s10072-020-04447-w
Caspani, G., Corbet Burcher, G., Garralda, M. E., Cooper, M., Pierce, C. M., Als, L. C., et al. (2018). Inflammation and psychopathology in children following PICU admission: an exploratory study. Evid. Based Ment. Health 21, 139–144. doi: 10.1136/ebmental-2018-300027
Chen, H., Guo, J., Wang, C., Luo, F., Yu, X., Zhang, W., et al. (2020). Clinical characteristics and intrauterine vertical transmission potential of COVID-19 infection in nine pregnant women: a retrospective review of medical records. Lancet 395, 809–815. doi: 10.1016/S0140-6736(20)30360-3
Cheung, K. S., Hung, I. F. N., Chan, P. P. Y., Lung, K. C., Tso, E., Liu, R., et al. (2020). Gastrointestinal manifestations of SARS-CoV-2 infection and virus load in fecal samples from a Hong Kong cohort: systematic review and meta-analysis. Gastroenterology 159, 81–95. doi: 10.1053/j.gastro.2020.03.065
Coleman, J. J., Manavi, K., Marson, E. J., Botkai, A. H., and Sapey, E. (2020). COVID-19: to be or not to be; that is the diagnostic question. Postgrad. Med. J. 96, 392–398. doi: 10.1136/postgradmedj-2020-137979
Contini, C., Di Nuzzo, M., Barp, N., Bonazza, A., De Giorgio, R., Tognon, M., et al. (2020). The novel zoonotic COVID-19 pandemic: an expected global health concern. J. Infect. Dev. Ctries 14, 254–264. doi: 10.3855/jidc.12671
Cui, J., Li, F., and Shi, Z. L. (2019). Origin and evolution of pathogenic coronaviruses. Nat. Rev. Microbiol. 17, 181–192. doi: 10.1038/s41579-018-0118-9
Dantzer, R., O'connor, J. C., Freund, G. G., Johnson, R. W., and Kelley, K. W. (2008). From inflammation to sickness and depression: when the immune system subjugates the brain. Nat. Rev. Neurosci. 9, 46–56. doi: 10.1038/nrn2297
Das, G., Mukherjee, N., and Ghosh, S. (2020). Neurological insights of COVID-19 pandemic. ACS Chem. Neurosci. 11, 1206–1209. doi: 10.1021/acschemneuro.0c00201
Dowlati, Y., Herrmann, N., Swardfager, W., Liu, H., Sham, L., Reim, E., et al. (2009). A meta-analysis of cytokines in major depression. Biol. Psychiatry 67, 446–457. doi: 10.1016/j.biopsych.2009.09.033
Evrensel, A., and Ceylan, M. E. (2015). The gut-brain axis: the missing link in depression. Clin. Psychopharmacol. Neurosci. 13, 239–244. doi: 10.9758/cpn.2015.13.3.239
Fan, H., Tang, X., Song, Y., Liu, P., and Chen, Y. (2020). Influence of COVID-19 on cerebrovascular disease and its possible mechanism. Neuropsychiatr. Dis. Treat 16, 1359–1367. doi: 10.2147/NDT.S251173
Galland, L. (2014). The gut microbiome and the brain. J. Med. Food 17, 1261–1272. doi: 10.1089/jmf.2014.7000
García, L. F. (2020). Immune response, inflammation, and the clinical spectrum of COVID-19. Front. Immunol. 11:1441. doi: 10.3389/fimmu.2020.01441
Gunther, M. L., Morandi, A., and Ely, E. W. (2008). Pathophysiology of delirium in the intensive care unit. Crit. Care Clin. 24, 45–65. doi: 10.1016/j.ccc.2007.10.002
Harapan, H., Itoh, N., Yufika, A., Winardi, W., Keam, S., Te, H., et al. (2020). Coronavirus disease 2019 (COVID-19): a literature review. J. Infect. Public Health 13, 667–673. doi: 10.1016/j.jiph.2020.03.019
Holshue, M. L., Debolt, C., Lindquist, S., Lofy, K. H., Wiesman, J., Bruce, H., et al. (2020). First case of 2019 novel coronavirus in the United States. N. Engl. J. Med. 382, 929–936. doi: 10.1056/NEJMoa2001191
Hussain, S., Pottathil, S., Islam, M., Chohan, M., and Rasool, S. (2020). Analysis of codon usage and nucleotide bias in middle east respiratory syndrome coronavirus genes. Evol. Bioinform. 16:117693432091886. doi: 10.1177/1176934320918861
Izzetti, R., Nisi, M., Gabriele, M., and Graziani, F. (2020). COVID-19 transmission in dental practice: brief review of preventive measures in Italy. J. Dent. Res. 99, 1030–1038. doi: 10.1177/0022034520920580
Jiang, F., Deng, L., Zhang, L., Cai, Y., Cheung, C. W., and Xia, Z. (2020). Review of the clinical characteristics of coronavirus disease 2019 (COVID-19). J. Gen. Intern. Med. 35, 1545–1549. doi: 10.1007/s11606-020-05762-w
Jiang, W., Wu, N., Wang, X., Chi, Y., Zhang, Y., Qiu, X., et al. (2015). Dysbiosis gut microbiota associated with inflammation and impaired mucosal immune function in intestine of humans with non-alcoholic fatty liver disease. Sci. Rep. 5:8096. doi: 10.1038/srep08096
Jin, X., Lian, J. S., Hu, J. H., Gao, J., Zheng, L., Zhang, Y. M., et al. (2020). Epidemiological, clinical, and virological characteristics of 74 cases of coronavirus-infected disease 2019 (COVID-19) with gastrointestinal symptoms. Gut 69, 1002–1009. doi: 10.1136/gutjnl-2020-320926
Kamholz, B. (2010). Update on delirium: diagnosis, management, and pathophysiology. Psychiatr. Ann. 40, 52–62. doi: 10.3928/00485718-20091229-05
Kandeel, M., and Al-Nazawi, M. (2020). Virtual screening and repurposing of FDA approved drugs against COVID-19 main protease. Life Sci. 251:117627. doi: 10.1016/j.lfs.2020.117627
Kannan, S., Shaik Syed Ali, P., Sheeza, A., and Hemalatha, K. (2020). COVID-19 (Novel Coronavirus 2019)–recent trends. Eur. Rev. Med. Pharmacol. Sci. 24, 2006–2011. doi: 10.26355/eurrev_202002_20378
Kotwani, A., and Gandra, S. (2020). Potential pharmacological agents for COVID-19. Indian J. Public Health 64, S112–S116. doi: 10.4103/ijph.IJPH_456_20
Lake, M. A. (2020). What we know so far: COVID-19 current clinical knowledge and research. Clin. Med. (Lond.) 20, 124–127. doi: 10.7861/clinmed.2019-coron
Li, H., Liu, S. M., Yu, X. H., Tang, S. L., and Tang, C. K. (2020a). Coronavirus disease 2019 (COVID-19): current status and future perspectives. Int. J. Antimicrob. Agents 55:105951. doi: 10.1016/j.ijantimicag.2020.105951
Li, Q., Guan, X., Wu, P., Wang, X., Zhou, L., Tong, Y., et al. (2020b). Early transmission dynamics in Wuhan, China, of novel coronavirus-infected pneumonia. N. Engl. J. Med. 382, 1199–1207. doi: 10.1056/NEJMoa2001316
Li, Y. C., Bai, W. Z., and Hashikawa, T. (2020). The neuroinvasive potential of SARS-CoV2 may play a role in the respiratory failure of COVID-19 patients. J. Med. Virol. 92, 552–555. doi: 10.1002/jmv.25728
Lin, L., Jiang, X., Zhang, Z., Huang, S., Zhang, Z., Fang, Z., et al. (2020). Gastrointestinal symptoms of 95 cases with SARS-CoV-2 infection. Gut 69, 997–1001. doi: 10.1136/gutjnl-2020-321013
Liu, Q., Wang, R. S., Qu, G. Q., Wang, Y. Y., Liu, P., Zhu, Y. Z., et al. (2020a). Gross examination report of a COVID-19 death autopsy. Fa Yi Xue Za Zhi 36, 21–23. doi: 10.12116/j.issn.1004-5619.2020.01.005
Liu, W., Wang, J., Li, W., Zhou, Z., Liu, S., and Rong, Z. (2020b). Clinical characteristics of 19 neonates born to mothers with COVID-19. Front. Med. 14, 193–198. doi: 10.1007/s11684-020-0772-y
Liu, Y., Gayle, A. A., Wilder-Smith, A., and Rocklöv, J. (2020c). The reproductive number of COVID-19 is higher compared to SARS coronavirus. J. Travel Med. 27:taaa021. doi: 10.1093/jtm/taaa021
Lu, R., Zhao, X., Li, J., Niu, P., Yang, B., Wu, H., et al. (2020). Genomic characterisation and epidemiology of 2019 novel coronavirus: implications for virus origins and receptor binding. Lancet 395, 565–574. doi: 10.1016/S0140-6736(20)30251-8
Macdonald, A., Adamis, D., Treloar, A., and Martin, F. (2007). C-reactive protein levels predict the incidence of delirium and recovery from it. Age Ageing 36, 222–225. doi: 10.1093/ageing/afl121
Maldonado, J. R. (2008). Pathoetiological model of delirium: a comprehensive understanding of the neurobiology of delirium and an evidence-based approach to prevention and treatment. Crit. Care Clin. 24, 789–856. doi: 10.1016/j.ccc.2008.06.004
McGrane, S., Girard, T. D., Thompson, J. L., Shintani, A. K., Woodworth, A., Ely, E. W., et al. (2011). Procalcitonin and C-reactive protein levels at admission as predictors of duration of acute brain dysfunction in critically ill patients. Crit. Care 15:R78. doi: 10.1186/cc10070
Memish, Z. A., Perlman, S., Van Kerkhove, M. D., and Zumla, A. (2020). Middle East respiratory syndrome. Lancet 395, 1063–1077. doi: 10.1016/S0140-6736(19)33221-0
Organization, W. H., and Organization, W. H. (2020). Coronavirus Disease (COVID-2019) Situation Reports. World Health Organization.
Ozma, M. A., Maroufi, P., Khodadadi, E., Köse, S., Esposito, I., Ganbarov, K., et al. (2020). Clinical manifestation, diagnosis, prevention, and control of SARS-CoV-2 (COVID-19) during the outbreak period. Infez. Med. 28, 153–165.
Pan, L., Mu, M., Yang, P., Sun, Y., Wang, R., Yan, J., et al. (2020). Clinical characteristics of COVID-19 patients with digestive symptoms in Hubei, China: a descriptive, cross-sectional, multicenter study. Am. J Gastroenterol. 115, 766–773. doi: 10.14309/ajg.0000000000000620
Porfidia, A., and Pola, R. (2020). Venous thromboembolism in COVID-19 patients. J. Thromb. Haemost. 18, 1516–1517. doi: 10.1111/jth.14842
Ressler, K., and Mayberg, H. (2007). Targeting abnormal neural circuits in mood and anxiety disorders: from the laboratory to the clinic. Nat. Neurosci. 10, 1116–1124. doi: 10.1038/nn1944
Rico-Mesa, J. S., White, A., and Anderson, A. S. (2020). Outcomes in patients with COVID-19 infection taking ACEI/ARB. Curr. Cardiol. Rep. 22:31. doi: 10.1007/s11886-020-01291-4
Shanmugaraj, B., Siriwattananon, K., Wangkanont, K., and Phoolcharoen, W. (2020). Perspectives on monoclonal antibody therapy as potential therapeutic intervention for Coronavirus disease-19 (COVID-19). Asian Pac. J. Allergy Immunol. 38, 10–18. doi: 10.12932/AP-200220-0773
Shen, K. L., Yang, Y. H., Jiang, R. M., Wang, T. Y., Zhao, D. C., Jiang, Y., et al. (2020). Updated diagnosis, treatment, and prevention of COVID-19 in children: experts' consensus statement (condensed version of the second edition). World J. Pediatr. 16, 232–239. doi: 10.1007/s12519-020-00362-4
Sifuentes-Rodríguez, E., and Palacios-Reyes, D. (2020). COVID-19: the outbreak caused by a new coronavirus. Bol. Med. Hosp. Infant. Mex. 77, 47–53. doi: 10.24875/BMHIM.20000039
Sohrabi, C., Alsafi, Z., O'neill, N., Khan, M., Kerwan, A., Al-Jabir, A., et al. (2020). World Health Organization declares global emergency: a review of the 2019 novel coronavirus (COVID-19). Int. J. Surg. 76, 71–76. doi: 10.1016/j.ijsu.2020.02.034
Stadler, K., Masignani, V., Eickmann, M., Becker, S., Abrignani, S., Klenk, H. D., et al. (2003). SARS–beginning to understand a new virus. Nat. Rev. Microbiol. 1, 209–218. doi: 10.1038/nrmicro775
Sternberg, E. M. (2006). Neural regulation of innate immunity: a coordinated nonspecific host response to pathogens. Nat. Rev. Immunol. 6, 318–328. doi: 10.1038/nri1810
Tian, S., Hu, W., Niu, L., Liu, H., Xu, H., and Xiao, S. Y. (2020). Pulmonary pathology of early-phase 2019 novel coronavirus (COVID-19) pneumonia in two patients with lung cancer. J. Thorac. Oncol. 15, 700–704. doi: 10.1016/j.jtho.2020.02.010
Velavan, T. P., and Meyer, C. G. (2020). The COVID-19 epidemic. Trop. Med. Int. Health 25, 278–280. doi: 10.1111/tmi.13383
Walls, A. C., Park, Y. J., Tortorici, M. A., Wall, A., Mcguire, A. T., and Veesler, D. (2020). Structure, function, and antigenicity of the SARS-CoV-2 Spike glycoprotein. Cell 181, 281–292. doi: 10.1016/j.cell.2020.02.058
Wang, D., Hu, B., Hu, C., Zhu, F., Liu, X., Zhang, J., et al. (2020a). Clinical characteristics of 138 hospitalized patients with 2019 novel coronavirus-infected pneumonia in Wuhan, China. JAMA 323, 1061–1069. doi: 10.1001/jama.2020.1585
Wang, Y., Wang, Y., Chen, Y., and Qin, Q. (2020b). Unique epidemiological and clinical features of the emerging 2019 novel coronavirus pneumonia (COVID-19) implicate special control measures. J. Med. Virol. 92, 568–576. doi: 10.1002/jmv.25748
Wong, M. L., Inserra, A., Lewis, M. D., Mastronardi, C. A., Leong, L., Choo, J., et al. (2016). Inflammasome signaling affects anxiety- and depressive-like behavior and gut microbiome composition. Mol. Psychiatry 21, 797–805. doi: 10.1038/mp.2016.46
Wu, F., Zhao, S., Yu, B., Chen, Y. M., Wang, W., Song, Z. G., et al. (2020a). A new coronavirus associated with human respiratory disease in China. Nature 579, 265–269. doi: 10.1038/s41586-020-2008-3
Wu, J., Song, S., Cao, H. C., and Li, L. J. (2020b). Liver diseases in COVID-19: etiology, treatment, and prognosis. World J. Gastroenterol. 26, 2286–2293. doi: 10.3748/wjg.v26.i19.2286
Wu, Y., Xu, X., Chen, Z., Duan, J., Hashimoto, K., Yang, L., et al. (2020c). Nervous system involvement after infection with COVID-19 and other coronaviruses. Brain Behav. Immun. 87, 18–22. doi: 10.1016/j.bbi.2020.03.031
Xiao, H., Zhang, Y., Kong, D., Li, S., and Yang, N. (2020). The effects of social support on sleep quality of medical staff treating patients with coronavirus disease 2019 (COVID-19) in January and February 2020 in China. Med. Sci. Monit. 26:e923549. doi: 10.12659/MSM.923921
Yang, L., Tian, D., and Liu, W. (2020a). Strategies for vaccine development of COVID-19. Sheng Wu Gong Cheng Xue Bao 36, 593–604. doi: 10.13345/j.cjb.200094
Yang, Y., Lu, Q., Liu, M., Wang, Y., Zhang, A., Jalali, N., et al. (2020b). Epidemiological and clinical features of the 2019 novel coronavirus outbreak in China. medRxiv 2020.2002.2010.20021675. doi: 10.1101/2020.02.10.20021675
Ye, M., Ren, Y., and Lv, T. (2020). Encephalitis as a clinical manifestation of COVID-19. Brain Behav. Immun. 88, 945–946. doi: 10.1016/j.bbi.2020.04.017
Yu, N., Li, W., Kang, Q., Xiong, Z., Wang, S., Lin, X., et al. (2020). Clinical features and obstetric and neonatal outcomes of pregnant patients with COVID-19 in Wuhan, China: a retrospective, single-centre, descriptive study. Lancet Infect. Dis. 20, 559–564. doi: 10.1016/S1473-3099(20)30176-6
Zhou, P., Yang, X. L., Wang, X. G., Hu, B., Zhang, L., Zhang, W., et al. (2020a). A pneumonia outbreak associated with a new coronavirus of probable bat origin. Nature 579, 270–273. doi: 10.1038/s41586-020-2012-7
Keywords: SARS-CoV-2, COVID-19 infection, gut-brain axis, ACE2 receptor, microbiota, dysbiosis, cytokine storm
Citation: Shinu P, Morsy MA, Deb PK, Nair AB, Goyal M, Shah J and Kotta S (2020) SARS CoV-2 Organotropism Associated Pathogenic Relationship of Gut-Brain Axis and Illness. Front. Mol. Biosci. 7:606779. doi: 10.3389/fmolb.2020.606779
Received: 15 September 2020; Accepted: 30 November 2020;
Published: 22 December 2020.
Edited by:
Saeid Abu-Romman, Al-Balqa Applied University, JordanReviewed by:
Shantanu Bandopadhyay, Naraina Vidya Peeth Group of Institutions, IndiaCopyright © 2020 Shinu, Morsy, Deb, Nair, Goyal, Shah and Kotta. This is an open-access article distributed under the terms of the Creative Commons Attribution License (CC BY). The use, distribution or reproduction in other forums is permitted, provided the original author(s) and the copyright owner(s) are credited and that the original publication in this journal is cited, in accordance with accepted academic practice. No use, distribution or reproduction is permitted which does not comply with these terms.
*Correspondence: Pran Kishore Deb, cHJhbmtpc2hvcmUxQGdtYWlsLmNvbQ==; orcid.org/0000-0002-8650-2874
Disclaimer: All claims expressed in this article are solely those of the authors and do not necessarily represent those of their affiliated organizations, or those of the publisher, the editors and the reviewers. Any product that may be evaluated in this article or claim that may be made by its manufacturer is not guaranteed or endorsed by the publisher.
Research integrity at Frontiers
Learn more about the work of our research integrity team to safeguard the quality of each article we publish.