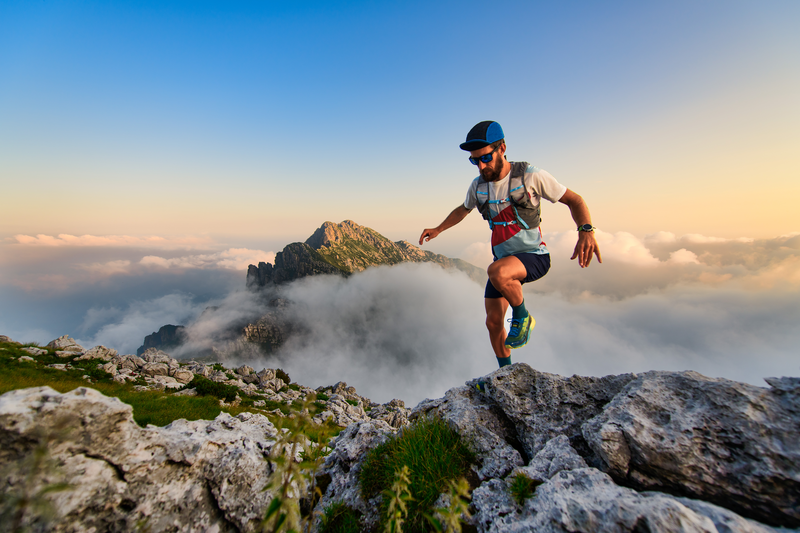
95% of researchers rate our articles as excellent or good
Learn more about the work of our research integrity team to safeguard the quality of each article we publish.
Find out more
ORIGINAL RESEARCH article
Front. Microbiol. , 25 March 2025
Sec. Microorganisms in Vertebrate Digestive Systems
Volume 16 - 2025 | https://doi.org/10.3389/fmicb.2025.1554484
Introduction: Dietary fiber plays a crucial role in maintaining gastrointestinal health. However, its protective effects on the intestinal health of calves remain to be fully elucidated. This study aimed to investigate the impact of dietary fiber supplementation on the intestinal microbiota of pre-weaned calves and its potential role in modulating microbial metabolic pathways.
Methods: A randomized controlled trial was conducted, enrolling 135 calves that were randomly assigned into three groups: (1) inulin supplementation, (2) psyllium husk powder (PHP) supplementation, and (3) a control group receiving no dietary fiber. Fecal microbiota samples were collected from calves without diarrhea at five time points (0, 7, 14, 28, and 56 days of age). Metagenomic sequencing was performed to analyze microbial composition and functional pathways. Additionally, a differential analysis of carbohydrate-active enzymes (CAZymes) was performed to evaluate the effect of dietary fiber on carbohydrate metabolism enzyme activity within the intestinal microbiota.
Results: Calves supplemented with dietary fiber exhibited a significant increase in the abundance of Bifidobacterium and Prevotella compared to the control group. These bacterial genera contributed to intestinal protection by modulating secondary bile acid metabolism and flavonoid metabolism pathways. CAZymes differential analysis revealed an increased abundance of carbohydrate metabolism enzymes in response to dietary fiber supplementation, with distinct microbial community compositions observed among different fiber treatments. Notably, at 56 days of age, calves fed PHP harbored intergeneric symbiotic clusters comprising Clostridium, Prevotella, and Bacteroides, suggesting a cooperative microbial network that may contribute to intestinal homeostasis.
Discussion: The findings of this study highlight the beneficial effects of dietary fiber on calf intestinal microbiota, particularly in enhancing microbial diversity and enzymatic activity related to carbohydrate metabolism. The observed microbial symbiosis in PHP-fed calves suggests a potential role in maintaining intestinal homeostasis. These insights provide a theoretical foundation for optimizing dietary interventions to promote gut health in calves during the transition period. Further research is warranted to explore the mechanistic interactions between dietary fiber, gut microbiota, and host health outcomes.
The health of calves is the foundation of a successful dairy farming industry. However, diarrhea remains a serious global challenge, contributing substantially to calf mortality and culling rates. Improving intestinal health helps calves fight off infections, grow stronger, and survive early-life challenges (Malmuthuge and Guan, 2017; Du et al., 2023). Research has demonstrated that calves experiencing diarrhea before weaning often face long-term effects, including decreased reproductive efficiency, reduced 305-day mature equivalent milk production (305ME) during their first lactation, and slower average daily gain (ADG) (Abuelo et al., 2021). According to the United States National Animal Health Monitoring System (NAHMS), nearly one-third of pre-weaned calves (33.8%) experience at least one illness, and over half of these cases (50.9%) are caused by digestive problems, such as diarrhea (NAHMS, 2021). Digestive issues are even more serious in newborn calves, causing about 32% of all deaths during this stage (Cho and Yoon, 2014; Urie et al., 2018). These numbers indicate how critical it is to maintain a healthy digestive system in young calves, especially for their long-term health and reproductivity.
Intestinal health is fundamental to the overall physiological health, with the gut microbiota playing a critical role in both maintaining the structure and function of the intestinal epithelium and the regulating immune responses (Zhou M. et al., 2024). A balanced intestinal environment provides a defense system, preventing harmful microorganisms from entering the bloodstream, maintaining immune balance to avoid overactivity, and strengthening the intestinal barrier through complex interactions among microbial communities, their metabolites, and epithelial cells (Kayama et al., 2020; Yue et al., 2023; Zhou P. et al., 2024). Additionally, the intestinal microbiota occupies ecological niches within the gastrointestinal tract, restricting space and resources available to pathogens, which helps maintain intestinal homeostasis (Dablool et al., 2024; Fauzia et al., 2024). The gut microbiota plays a significant role during early life, as it forms the basis for the development of both intestinal mucosal and systemic immune systems (Rodríguez et al., 2015). This early microbial ecosystem is highly dynamic, undergoing rapid changes that coincide with critical phases of intestinal and immune system maturation, particularly in pre-weaned calves (Hennessy et al., 2020). However, disruptions to the gut microbiota during this formative stage can lead to long-term dysbiosis, which increases susceptibility to chronic health issues (Belkaid and Hand, 2014; Sarkar et al., 2021). Thus, fostering the growth of a healthy intestinal microbiota during this key developmental window in calves is essential for reinforcing the intestinal barrier, maintaining gastrointestinal health, and laying the foundation for their long-term growth and resilience.
Dietary fiber has been widely recognized for its important role in maintaining gastrointestinal health (Elihasridas et al., 2023). A previous study demonstrated the ability of fiber to stimulate gastrointestinal motility, preserve the integrity of the intestinal mucosa, and facilitate the colonization and fermentation of beneficial microbiota, which resulted in the production of short-chain fatty acids (Gill et al., 2021; Jiajun et al., 2024). Furthermore, fiber supplementation has emerged as an effective intervention for mitigating antibiotic-induced dysbiosis by modulating the redox potential of the intestine (Penumutchu et al., 2023). Of the various dietary fibers, inulin has been shown to enhance the abundance of Bifidobacterium in the gut microbiota and increase the concentration of fecal short-chain fatty acids (Le Bastard et al., 2020a; Chen et al., 2023). Similarly, psyllium husk has been shown to promote the growth of butyrate-producing bacteria, improve stool consistency through enhanced water absorption, and fortify the intestinal barrier by reducing proinflammatory cytokines IL-1 and IL-6, as well as serum tryptophan levels (Hu et al., 2023; Lai et al., 2023; Garg et al., 2024). These findings underscore the potential of inulin and psyllium husk as functional dietary fibers that can effectively regulate the composition of the gut microbiota, reinforce the intestinal barrier integrity, and optimize stool quality. Thus, both inulin and psyllium husk are promising strategies for enhancing the gastrointestinal health of pre-weaned calves.
Our study aimed to fill this knowledge gap regarding the potential of inulin and psyllium husk as beneficial modulators of the gut microbiota of pre-weaned calves. We performed a feeding trial to assess how inulin and psyllium husk influence the intestinal microbiota composition and function in pre-weaned calves. Fecal samples were collected at multiple time points to track microbial changes and evaluate interactions within the microbial community. These findings provide valuable insights into the mechanisms by which these dietary fibers support gut health and reduce diarrhea risk in pre-weaned calves.
This study was conducted at a dairy farm in Hebei Province, China. A total of 136 healthy newborn Holstein female calves were selected based on health criteria developed by McGuirk and Peek (2014). These criteria evaluate calf health by checking their mental state, nasal and eye discharge, and coughing. All calves received colostrum shortly after birth and were individually housed in pens.
The calves were divided into three groups: the control group (CON), the psyllium husk powder group (PHP), and the inulin group (INU). Details about the group assignments are shown in Table 1. Starting on the second day after birth, PHP group calves received psyllium husk powder, and INU group calves were supplemented with inulin. The control group did not receive any dietary fiber. The feeding schedule and composition of the feed are detailed in Table 1.
Fecal samples were collected on days 0, 7, 14, 28, and 56. These samples were stored at −80°C to maintain their quality for analysis. Fecal consistency scores were recorded daily for each calf throughout the trial. The scores ranged from 0 to 3 and were applied through observing the freshest feces visible on the bedding. A fecal consistency score of 2 or higher indicated “diarrhea” in a calf, while a score below 2 indicated a “healthy” calf. The duration of diarrhea was recorded when a calf exhibited signs of diarrhea, with the onset marked as the age in days when a diarrhea score of 2 was first recorded. The end of a diarrhea episode was identified as the day when the score was below 2. The duration of diarrhea was calculated as the lapse of time between the two dates, representing the occurrence of diarrhea in the calf (McGuirk, 2008). Nine healthy calves from each group that did not develop diarrhea during the study were selected for further analysis. These samples were used to examine how dietary fiber affected the gut microbiota of the calves.
In this study, complete microbial DNA was extracted from fecal samples using a magnetic soil and fecal DNA extraction kit (DP712, China TianGen Biotechnology Co., Ltd.). Approximately 0.25–0.5 g of each sample was mixed with lysis buffers and grinding beads, followed by mechanical disruption and thermal lysis. After centrifugation, the supernatant was transferred for further purification. DNA was then precipitated using binding buffer and magnetic beads, allowing selective adsorption of DNA. The beads were washed with ethanol-based buffers to remove contaminants, and DNA was finally eluted in Buffer TB. The purified DNA was stored at −20°C for downstream applications. Metagenomic sequencing was conducted on the Illumina NovaSeq 6000 platform, generating paired-end reads of 150 base pairs, by China TianGen Biotechnology Co., Ltd. The sequencing libraries were prepared using the TruSeq DNA Sample Prep Kit, with the 5′ adapter sequence: 5′-AATGATACGGCGACCACCGAGATCTACACTCTTTCCCTACACGACGCTCTTCCGATCT-3′ and the 3′ adapter sequence: 5′-GATCGGAAGAGCACACGTCTGAACTCCAGTCAC(index)ATCTCGTATGCCGTCTTCTGCTTG-3′.
After sequencing, low-quality reads were removed using Fastp (v0.24.4), yielding a total of 2,779,248,798 reads. Host DNA was removed using Bowtie2 (v2.5.2) within Kneaddata (v0.12.0), resulting in 1,586,617,206 clean reads, with an average of 9,775,668.787 clean reads per sample (58.17% of the total reads) (Supplementary Table S1).
Bioinformatic and statistical analyses were conducted using established methodologies (Liu et al., 2020). The raw data were subjected to quality control using Fastp (v0.23.4), followed by the removal of host sequences derived from NCBI (GenBank assembly ID: GCA_021347905.1, https://www.ncbi.nlm.nih.gov/datasets/genome/GCA_021347905.1/) using Bowtie2 (v2.5.2) within Kneaddata (v0.12.0) to obtain clean data. Subsequently, the clean data were assembled using megaHit (V1.2.9). Following read assembly, species annotation was conducted using Kraken2 (v2.1.3), while KEGG and CAZy database annotations (based on eggNOGDB version: 5.0.2) were performed using Diamond (v2.0.15) (Huang et al., 2018; Zhang et al., 2018; Zheng et al., 2023).
To analyze temporal changes in fecal microbiota composition, we assessed α diversity using the Shannon diversity index and Abundance-based Coverage Estimator (ACE) index. β diversity was evaluated using canonical principal coordinate analysis (CPCoA). Linear regression analysis was performed to assess the relationship between dietary fiber intervention and microbial diversity, with R2 values calculated for ACE and Shannon indices.
Metagenomic statistical analysis was conducted using R software version 4.3.1 (https://www.R-project.org/). The P-values for α diversity indices (ACE and Shannon) were calculated using ANOVA with post-hoc Tukey HSD test for multiple comparisons. The β diversity (distance values calculated using Chao) was analyzed with cPCoA, and P-values were computed using an ANOVA-like permutation test. For Spearman correlation analysis, P-values were not adjusted for multiple testing. Similarly, group comparisons of significantly different taxa and functional features (e.g., KEGG/CAZy) were performed without P-value correction. As this study is exploratory, unadjusted P-values were used to better reveal potential changes in the abundance and functionality of fecal microbiota in calves.
Visualization of α-diversity, β-diversity, and species stacked bar plots was performed using ggplot2 (Valero-Mora, 2010). The differential analysis of species and KEGG/CAZy database annotation results was performed using STAMP (statistical analysis of taxonomic and functional profiles, version: 2.1.3) and LEfSe (linear discriminant analysis effect size). Simple linear analysis was conducted using GraphPad Prism (v9.5.1), while Spearman correlation was calculated using WGCNA (https://cran.r-project.org/web/packages/WGCNA/index.html, Langfelder and Horvath, 2008). Co-occurrence networks were visualized using Gephi (https://gephi.github.io/, Bastian et al., 2009).
Dietary fiber supplementation significantly affected fecal microbiota diversity, with temporal variations in Shannon and ACE indices and R2 values exceeding 0.5 in linear regression analysis. At day 0, the structure of the fecal microbiota was relatively simple, with low α-diversity. However, the α-diversity of the fecal microbiota increased progressively with the calf's age. The fitted curves indicated that the ACE and Shannon indices in the INU and PHP groups were higher than those in the CON (control)group (Figures 1A, B). CPCoA revealed significant differences in the β-diversity of the fecal microbiota among the three groups over time (P < 0.01, Figures 1C–E). These findings suggest that the diversity and richness of the intestinal microbiota in pre-weaned calves changes significantly with increasing age.
Figure 1. Dynamic analysis of fecal microbial diversity in calves across different ages. (A, B) Trends in the Shannon diversity index or ACE diversity index of fecal micro-organisms in calves from 0 to 56 days of age treated with dietary fiber. (C–E) CPCoA analysis of fecal microbial β-diversity in calves 0–56 days old in CON, PHP, and INU groups.
Analysis of the Shannon and ACE indices revealed that at day 28, calves supplemented with psyllium husk powder had significantly higher Shannon and ACE indices, as compared to the control group (P < 0.05). In contrast, calves supplemented with inulin displayed no statistically significant differences from the control group, although the alpha diversity indices exhibited an increasing trend (Figure 2). CPCoA showed that intergroup differences became more pronounced at day 7, with an evident difference between the PHP and INU groups, indicating low similarity in the composition of the fecal microbiota between the two groups (Figures 3A, B). At day 14, significant differences in the composition of the fecal microbiota were found among the PHP, INU, and control groups (P < 0.01, Figure 3C). By day 28, the differences in the composition of the fecal microbiota among the three groups diminished (Figure 3D). However, at day 56, significant differences in the fecal microbiota composition were observed among calves supplemented with various dietary fibers (P < 0.05, Figure 3E). These findings suggested that dietary fiber supplementation in pre-weaned calves may alter the composition of the intestinal microbiota between days 14 and 56.
Figure 2. The impact of supplementation with psyllium husk powder and inulin on the α-diversity of the intestinal microbial community in neonatal calves. (A) Bacterial Shannon diversity index. (B) Bacterial ACE diversity index. (A, B) ANOVA (Analysis of Variance) with post-hoc Tukey HSD (Honestly Significant Difference) test, ns indicates no statistical difference, P < 0.05 indicates a significant statistical difference.
Figure 3. Assessment of the impact of psyllium husk powder and inulin supplementation on the β-diversity of the fecal microbiota in calves. (A–E) CPCoA analysis of fecal microbial β-diversity of CON, PHP, and INU calves at 0, 7, 14, 28, and 56 days. Group differences were calculated using the Chao distance, where P < 0.05 indicates a significant difference, and P < 0.01 indicates a highly significant difference.
In this study, the 29 most abundant species were listed at the genus and species levels, and the remaining species with lower relative abundances were classified under the “Others” category. Relative abundance analysis at the genus level showed that the relative abundance of Bacteroides in the INU and PHP groups was lower than that in the control group at day 7. As the calf aged, the relative abundance of Bacteroides in the INU and PHP groups gradually increased, and after 28 days, the relative abundance of Bacteroides exhibited a trend of surpassing that of the control group (Figure 4A, Supplementary Figure S1). The relative abundance of Enterococcus tended to decrease during calf development, and the rate of decrease in the INU and PHP groups was slower than that in the control group. In addition, the relative abundance of Enterococcus in the PHP group was significantly increased as compared to that in the control and INU groups at day 28 (Figure 4A, Supplementary Figure S2A). The relative abundance of Bifidobacterium was significantly greater at days 7 and 14 after birth than at days 28 and 56. The relative abundance of Bifidobacterium in the INU group was substantially greater than that in the control group at days 7, 14, and 28. The relative abundance of Bifidobacterium in the PHP group was slightly lower than that in the INU group at days 7 and 14 (Figure 4A, Supplementary Figure S1).
Figure 4. Analysis of the fecal microbiota at the genus and species levels in neonatal calves. (A) Stacked bar chart showing the relative abundance of species at the genus level. (B) Comparison of specific genera, including Lactococcus and Ruminococcus on day 7, Prevotella on day 28 and day 56, Dorea on day 28, and Klebsiella on day 56. (C) Stacked bar chart illustrating the relative abundance of species at the species level. (D) Differential abundance of Bifidobacterium species in calves of different ages and treatments (CON group, INU group, and Psyllium husk group). (C, D) Intergroup differences were calculated using the Kruskal–Wallis test, where P < 0.05 indicates a significant difference and P < 0.01 indicates a highly significant difference.
Further analysis at the species level showed that at day 7 post-birth, the relative abundance of Bifidobacterium pseudopodium in the PHP group was significantly increased, as compared to that in the INU and control groups (P < 0.01). At day 14 after birth, the relative abundance of Bifidobacterium pseudocatenulatum in the INU group was greater than that in the PHP and control groups (P = 0.053). At day 28 post-birth, the relative abundances of Bifidobacterium longum, Bifidobacterium pseudocatenulatum, and Bifidobacterium breve in the INU group were substantially increased, as compared to those in the PHP and controls groups (P < 0.05, Figures 4C–D). The relative abundance of Prevotella in the intestine of neonatal calves was initially low, but gradually increased as the calves grew and developed (Figure 4A, Supplementary Figure S1). Moreover, on day 28, the relative abundance of Prevotella in the INU group was significantly greater than that in the control and PHP groups (P < 0.01). On day 56 after birth, the relative abundance of Prevotella in both the INU and PHP groups was significantly greater than that in the control group (P < 0.01, Figure 4B). Additionally, the relative abundance of Lactococcus in the control group was significantly lower than that in the INU and PHP groups (P < 0.05) on day 7. On day 288, the relative abundance of Dorea in the control group was significantly greater than that in the INU and PHP groups (P < 0.05), and on day 56, the relative abundance of Klebsiella in the control group was significantly increased, as compared to that in the INU and PHP groups (P < 0.05). Furthermore, on day 7, the relative abundance of Ruminococcus in the PHP group was significantly greater than that in the control and INU groups (P < 0.05, Figure 4B).
Further analysis at the species level revealed that on day 28, the relative abundance of Blautia hansenii in the INU group was significantly greater than that in the control and PHP groups (P < 0.05). In addition, the relative abundance of Enterococcus faecium in the PHP group was significantly increased as compared to that in the control and INU groups (P < 0.05). In contrast, the relative abundance of Dorea sp. CAG:317 in the control group was significantly greater than that in the INU and PHP groups (P < 0.05, Supplementary Figure S1B). On day 56, the relative abundance of Siphoviridae sp. in the control group was significantly increased as compared to that in the INU and PHP groups (P < 0.05), and the relative abundance of Sharpea azabuensis in the INU group was considerably greater than that in the control group (P < 0.05). In comparison, the relative abundance of Sharpea azabuensis in the PHP group was greater than that in the control group, but lower than that in the INU group. Additionally, the relative abundance of Clostridium perfringens in the control and INU groups was substantially lower than that in the PHP group (P < 0.05) (Supplementary Figure S1C).
LEfSe (linear discriminant analysis effect size) analysis of the gut microbiota from calves supplemented with psyllium husk powder and inulin demonstrated significant differences at 7 days after birth. The abundance of Ruminococcus in the intestine of calves supplemented with psyllium husk powder significantly differed from that in the control group, while the abundance of Streptococcus in the INU group was significantly different (all P < 0.05, LDA > 2.0). In addition, there were significant differences in the abundances of the genera Pectobacterium, Acinetobacter, and Vibrio in the intestines of calves in the control group, as compared with those in the PHP and INU groups (all P < 0.05, LDA > 2.0). At day 28 after birth, the primary differential bacterial communities in the intestines of calves supplemented with psyllium husk powder were Agathobaculum, Roseburia, Paenibacillus, and Intestinibacillus (all P < 0.05, LDA > 2.0). In contrast, the major differential bacterial communities in the intestines of calves supplemented with inulin were Prevotella, Streptococcus, and Phocaeicola (all P < 0.05, LDA > 2.0). At day 56 post-birth, Prevotella was revealed as a significantly differential bacterial genus in the intestines of calves supplemented with psyllium husk powder (all P < 0.05, LDA > 4.0), and Lactobacillus also showed significant differences (all P < 0.05, LDA > 2.0). In the intestines of calves supplemented with inulin, Sharpea was found to be the primary bacterial group (all P < 0.05, LDA > 2.0, Figure 5).
Figure 5. LEfSe analysis showing the differences in the microbiota composition at the genus level among the three groups (P < 0.05 and LDA > 2).
LEfSe analysis at the species level revealed that Bifidobacterium pseudolongum was significantly differentially abundant in the intestinal tract of 7-day-old calves supplemented with inulin (all P < 0.05, LDA > 2.0). As the calves developed to 28 days of age, Clostridium sp-WCA-389-WT-23D1, Agathobaculum desmolans, and Megamonas funiformis were identified as the major differential microbiota in the PHP group, while Prevotella copri and Prevotella sterecorea in the Prevotella genus, Bacteroides coprocola, Bacteroidaceae bacterium, and Bacteroides stercori in the Bacteroides genus, and Bifidobacterium longum and Bifidobacterium pseudocatenulatum in the Bifidobacterium genus were identified as the differential microbiota in the INU group. Additionally, Dorea sp. CAG:317 and Dorea phocaeensiss were identified as a differential species in the control group (all P < 0.05, LDA > 2.0). Prevotella sp. P2-180 and Prevotella stereorea in the Prevotella genus were identified as differential species in the PHP group up until the calves reached 56 days of age. The primary differential species in the INU group was Sharpea azabuensis (all P < 0.05, LDA > 2.0, Supplementary Figure S2).
At day 28 post-birth, the abundance of genes involved in the flavone and flavonol biosynthesis pathways in the PHP group was significantly increased, as compared to that in the control group (P < 0.05). However, at day 56 after birth, the flavone and flavonol biosynthesis pathways demonstrated an increase compared to those in the control group, but the difference was not statistically significant (Figures 6A, C–D). In the INU group, there was a slight increase in the flavone and flavonol biosynthesis pathways, as compared to that in the control group, but it was not statistically significant. However, the secondary bile acid biosynthesis pathway in the gut microbiota of calves in the INU group was significantly decreased, as compared to the control group (P < 0.05, Figure 6B).
Figure 6. Illustrates the differential functions of the intestinal microbiota in the CON group, INU group, and Psyllium husk group. (A) Abundance of flavone and flavonol biosynthesis on day 28. (B) Abundance of secondary bile acid biosynthesis on day 28. (C) Abundance of flavone and flavonol biosynthesis on day 56. (D) Abundance of flavone and flavonol biosynthesis in the CON group and Psyllium husk group on day 56. *Data analysis was conducted using ANOVA.
We then conducted a simple linear analysis on the differential species and functions and found that on day 28, there was a significant negative correlation between Bifidobacterium spp. in the INU group and secondary bile acid biosynthesis pathway (P = 0.0159, R2 = 0.3491, Figure 7A). Further analysis at the species level revealed that B. longum was not significantly correlated with the secondary bile acid biosynthesis (P = 0.0710, R2 = 0.2142), but B. breve was significantly negatively correlated with secondary bile acid biosynthesis (P = 0.0354, R2 = 0.2791, Figures 7C, D). On day 56, there was a significant positive correlation between the abundance of Prevotella in the PHP group and the flavone and flavonol biosynthesis pathway (P = 0.0390, R2 = 0.2543, Figure 7B).
Figure 7. Presents the simple linear analysis of differential genera and differential functions. (A) The correlation between the Bifidobacterium genus and the secondary bile acid metabolism pathway on day 28. (B) The correlation between the Prevotella genus and flavone and flavonol biosynthesis on day 56. (C) The correlation between B. longum and secondary bile acid biosynthesis on day 28. (D) The correlation between B. breve and secondary bile acid biosynthesis on day 28.
CAZymes families have been identified as vigorous predictors of animal diet. In order to investigate the potential influence of various dietary fibers on the gut microbiota of pre-weaned calves, we conducted STAMP (statistical analysis of taxonomic and functional profiles) differential analysis of CAZymes functions across the three groups of calves. We found that as the calves developed, the functions of the gut microbiota in the INU and PHP groups gradually increased, as compared to those of the control group. On day 14 of calf development, the INU group exhibited significant differences in the CAZymes function of their microbiota, as compared to the control group, with 6 CHs (Carbohydrate Hydrolases), 1 CBM (Carbohydrate-Binding Module), and 1 GT (Glycosyltransferases). By day 28 of calf development, the INU group showed further significant differences, with 10 CHs, 4 CBMs, 1 GT, and 1 PL (Polysaccharide Lyases). After the calves reached 56 days of age, the INU group exhibited substantial significant differences, with 20 CHs, 1 GT, and 2 CEs (Carbohydrate Esterases) (Supplementary Figure S3). At 14 days of age, calves in the PHP group exhibited significant differences from those in the control group, with 3 CHs, 3 GTs, and 2 CBMs. These significant differences increased by 28 days of age, with 5 CHs, 2 GTs, 3 CBMs, and 1 CE. At 56 days of age, the function of the gut microbiota from calves in the PHP group significantly differed from that in the control group, with 16 CHs, 3 GTs, and 2 PLs (Supplementary Figure S4).
To investigate the potential associations between the intestinal microbiota of pre-weaned calves and differential functions of CAZymes in calves, we conducted a correlation analysis between the intestinal microbiota and differential functions of CAZymes in calves at D56. We found that regardless of whether the calves were fed inulin or psyllium husk powder, the functions of CAZymes in the gut microbiota of the calves were mostly positively correlated with bacterial genera and species (Figures 8A, B). There was a significant correlation between the intestinal microbiota of the Sharpea genus Sharpea azabuensis and GH43_28.hmm in calves fed inulin (P < 0.01, R > 0.25). The abundances of the Bacteroidales bacterium Oil-RF-744-WCA-WT-10 were significantly correlated with the abundances of GH5_2.hmm, GH43_5.hmm, and GH5_21.hmm (P < 0.05, R > 0.25). There was a substantial correlation between the abundance of the Prevotella genus Prevotella sp. P2-180 and that of GT2.hmm in the gut microbiota of calves fed psyllium husk powder (P < 0.05, R > 0.25). Prevotella stereorea exhibited a significant correlation with GH26.hmm (P < 0.05, R > 0.25). Candidatus Gastranaerophilales showed a highly significant correlation with 5 GHs (P < 0.01, R > 0.25), a significant correlation with 4 GHs (P < 0.05), and a significant correlation with PL10.hmm and GT92.hmm (P < 0.05, R > 0.25).
Figure 8. Association analysis of differential gut microbiota and CAZy differential functions in 56-day-old calves fed different dietary fibers. (A) Correlation between differential gut microbiota and CAZy differential functions in calves fed inulin for 56 days. (B) Correlation between Differential Gut Microbiota and CAZy Differential Functions in Calves Fed Psyllium Husk for 56 Days. (A, B) Correlation calculation using the Pearson algorithm, *P < 0.05, **P < 0.01, ***P < 0.001.
We analyzed the symbiotic relationships within the intestinal microbiota of 56-day-old calves and found that calves supplemented with inulin displayed weak intergenus associations, with only the Prevotella, Clostridium, and Bacteroides genera showing relatively strong intragenus correlations (Figure 9A). Conversely, calves supplemented with psyllium husk powder displayed strong intergenus associations within their intestinal microbiota. We also observed that Prevotella, Clostridium, Firmicutes, Bacteroides, and Candidatus Gastranaerophilales formed an intergenus symbiotic cluster, while Bacteroides, Faecalibacterium, and Ruminococcus formed another intergenus symbiotic cluster. Additionally, the abundances of Prevotella, Clostridium, and Firmicutes exhibited relatively high intra-genus correlations (Figure 9B).
Figure 9. Co-occurrence network of intestinal microbiota at the species level in (A) 56-day-old calves supplemented with inulin and (B) 56-day-old calves supplemented with Psyllium husk. Each node represents a species, and the edges represent a significant co-occurrence relationship. The top 400 correlating nodes are included in the graph (those with correlations <400 are the nodes with the highest correlation).
Diarrhea is a major health challenge in neonatal calves, causing high morbidity, mortality, and economic losses, while dietary fiber supports gastrointestinal health by alleviating diarrhea, constipation, and inflammation (Foster and Smith, 2009; Shoaib et al., 2016; Urie et al., 2018; Belorio and Gómez, 2021). Our findings suggest that supplementing neonatal calves with psyllium husk powder or inulin accelerates the establishment of a beneficial intestinal microbiota. Twenty-eight days after birth, calves in the psyllium husk group exhibited significantly greater microbial diversity and richness as compared to the control group. Furthermore, we found that the intestinal microbiota transitioned between days 14 and 28, highlighting a critical period of microbial maturation and underscoring the potential of dietary fiber intervention in supporting intestinal health during early development.
To explore the effects of psyllium husk powder and inulin on the gut microbiota of calves, we employed metagenomic analysis to assess variations in distinct microbial communities within the intestines of calves across various developmental stages. During the early stages of preweaning calf development, alterations in the gut microbiota induced by feeding psyllium husk powder or inulin were not prominent. However, Ruminococcus and Streptococcus were identified as differential microbial communities in the intestines of calves supplemented with dietary fiber. It has been previously reported that Ruminococcus is a critical symbiotic bacterium and butyrate-producing bacterium capable of fermenting plant fiber to produce short-chain fatty acids (La Reau and Suen, 2018). Moreover, Ruminococcus is a vital producer of butyrate, a beneficial short-chain fatty acid. During the early stages of calf development, supplementation with psyllium husk powder resulted in an increased abundance of Ruminococcus, suggesting that Ruminococcus could ferment psyllium husk powder to produce short-chain fatty acids. At day 28, as the calf intestinal microbiota diversity increased, beneficial bacteria, such as Agathobaculum, Roseburia, and Prevotella, became the dominant differential genera in the intestines of calves supplemented with psyllium husk powder and inulin. By day 56, the gut microbiota had stabilized. In calves supplemented with psyllium husk powder, Prevotella and Lactobacillus were the dominant genera. Additionally, Ruminococcus, Agathobaculum, and Roseburia—known butyrate producers—played a crucial role in providing energy for intestinal epithelial cells, supporting calf development (Rivière et al., 2016). Additionally, butyrate displays immunomodulatory and anti-inflammatory properties, aiding in preserving intestinal barrier function, which is crucial for enhancing resistance to diarrhea in neonatal calves. Furthermore, numerous studies have shown that feeding dietary fiber, such as inulin, increases the abundance of the Bifidobacterium genus. Concurrently, Bifidobacterium interacts with butyrate-producing bacteria, thereby promoting butyrate production. In this study, calves fed psyllium husk powder, exhibited a substantial increase in the abundance of Bifidobacterium pseudolongum at day 7, while calves fed inulin showed significant differences in the abundances of Bifidobacterium breve, Bifidobacterium longum, and Bifidobacterium pseudocatenulatum at days 14, 28, and 56, respectively. This finding indicates that both psyllium husk powder and inulin may have bifidogenic effects. In the intestines of calves fed psyllium husk powder, a significant increase in the abundance of the Bifidobacterium genus was not observed, but differences in the butyrate-producing bacteria were evident. We theorize that psyllium husk powder stimulates an increase in the abundance of butyrate-producing bacteria through other pathways, thereby promoting butyrate production.
Differential analysis of KEGG pathways identified a significant increase in flavone and flavonol biosynthesis pathways in calves supplemented with psyllium husk powder. As the calves reached 56 days of age, the activity of the flavone and flavonol biosynthesis pathway was elevated compared to the control group, but the difference was not statistically significant. Compared with the control group, the secondary bile acid biosynthesis pathway in the calves, supplemented with inulin, was significantly decreased. Furthermore, simple linear regression analysis indicated that the abundance of Bifidobacterium at day 28 was significantly correlated with the secondary bile acid biosynthesis pathway in calves supplemented with inulin. At the species level, B. breve was significantly correlated with the secondary bile acid biosynthesis pathway, while B. longum exhibited some correlation with the secondary bile acid biosynthesis pathway, although the correlation was not statistically significant. These results indicate that the increased abundance of Bifidobacterium partially reduces the activity of the secondary bile acid biosynthesis pathway. Previous studies have demonstrated that the metabolic products synthesized by the secondary bile acid biosynthesis pathway are related with the development of fatty liver, obesity, and colorectal cancer in humans and animals (Jia et al., 2021; Liu et al., 2022). Current research indicates that deoxycholic acid, a metabolic product involved in the secondary bile acid biosynthesis pathway, adversely alters the barrier function of the intestinal epithelium (Stenman et al., 2013; Liu et al., 2018; Zhuang et al., 2024). Our study suggests that supplementing pre-weaned calves with inulin enriches Bifidobacterium during the early development of the intestinal barrier, which inhibits the secondary bile acid biosynthesis pathway to protect intestinal health and promotes intestinal recovery, thereby reducing the incidence of calf diarrhea.
When the calves reached 56 days of age, the structure of their gut microbiota closely resembled that of adult cattle. Our investigation revealed substantial differences at day 56 in the abundance of Prevotella between calves supplemented with psyllium husk powder and those in the control group. Through species-level LEfSe analysis, we identified Prevotella sp. P2-180 and Prevotella stereorea as the primary differential strains. Further simple linear regression analysis identified a significant positive correlation between the abundance of Prevotella, as well as the flavone and flavonol biosynthesis pathways. This study confirms that both flavone and flavonol biosynthesis, both of which produce flavonoid compounds that play various roles in the gastrointestinal tract, are essential metabolic pathways in the gut microbiota. These pathways perform essential functions, including maintaining intestinal barrier integrity, modulating intestinal immune system, regulating intestinal hormone secretion, promoting beneficial microbial communities, inhibiting harmful endotoxin production, and positively regulating beneficial fatty acid production (Oteiza et al., 2018). Furthermore, prior research suggests that intestinal microbiotas abundant in Prevotella possess an enhanced capacity for the utilization of complex polysaccharides (Chen et al., 2017), which aligns with our findings, indicating a significant increase in Prevotella during the later stages of preweaning in calves supplemented with psyllium husk powder or inulin, as compared to the control group. Prevotella is an intestinal probiotic strongly correlated with the production of total short-chain fatty acids. A previous in vitro study suggested that supplementing a diet with flavonoids and dietary fibers that produced SCFAs (Short-chain fatty acids) significantly enriched Prevotella (Lucas et al., 2018). This preliminary study also suggested that flavonoids could promote SCFAs production via Prevotella. We speculated that supplementation with psyllium husk powder in calves at 28 days of age gradually activated the flavone and flavonol biosynthesis pathways in the intestinal microbial community, leading to the production of flavonoids. These flavonoids promote the colonization of Prevotella in the intestines of calves, as well as the production of SCFAs. Both Prevotella and SCFAs protected the health of post-weaning calf intestinal epithelial cells, maintained intestinal metabolic health, and preserved intestinal mucosal barrier integrity, thereby maintaining intestinal homeostasis (Xiong et al., 2019; Blaak et al., 2020; Silva et al., 2020).
The physiological functions of the bovine digestive system differ considerably from those of monogastric animals. Adult cows rely on the gastrointestinal microbiota to ferment cellulose and other substrates, producing volatile fatty acids to meet their energy requirements. The digestive system of pre-weaned calves is similar to that of monogastric animals, but as weaning approaches, the rumen of calves begins to progressively develop, transitioning toward that of adult cattle. Our study demonstrated significant differences at day 56 in the intestinal microbiota of calves fed inulin, particularly in the abundance of the Sharpea genus and Bacteroidales bacterium Oil-RF-744-WCA-WT-10. These differences were significantly correlated with enzymes from the GH43 and GH5 families. Previous research has suggested that the Sharpea genus is an important producer of lactic acid and can work synergistically with other associated genera to promote the production of butyrate (Kamke et al., 2016; Lin et al., 2019; Zhang et al., 2022). Additionally, this genus stabilizes the ecosystem of the intestinal microbiota, leading to increased production of butyrate for intestinal epithelial cell function, maintaining intestinal barrier integrity, and laying the foundation for the maturation of the calf's digestive system.
In calves supplemented with Psyllium husk, the Prevotella genus was the primary differential species at day 56. Pearson correlation analysis revealed significant correlations between the Prevotella genus and enzyme families, including GT2 and GH26. Candidatus Gastranaerophilales bacteria were found to be significantly correlated with the enzyme families GH5, GH10, GH26, GH28, GH43, GH53, GH67, GH115, GT92, and PL10. Previous studies have indicated that Candidatus Gastranaerophilales bacteria contribute to the production of indole, which can be converted into indole-3-propionic acid (IPA). IPA has been shown to play an anti-inflammatory role in the gastrointestinal tract and throughout the body, thus protecting the intestinal health of calves and reducing diarrhea (Zhao et al., 2019; Rosario et al., 2021).
According to the CAZy database (https://www.CAZy.org) classification, enzymes involved in carbohydrate metabolism are categorized as glycosyl hydrolases (GHs), polysaccharide lyases (PLs), carbohydrate esterases (CEs), and glycosyl transferases (GTs). These enzymes display substrate specificity in carbohydrate degradation (Helbert et al., 2019). Previous studies have demonstrated that the intake of different forms of carbohydrates can promote specific bacterial taxa and alter the CAZymes profile in the intestine (Cronin et al., 2021; Ye et al., 2022). In this study, the intestinal microbiota of calves, supplemented with fructooligosaccharides (FOSs) and psyllium husk powder before weaning, showed increased levels of multiple CAZymes families, as compared with those in the control group. Specifically, at day 56 the FOSs group showed significant increases in enzyme families GH5, GH13, GH26, GH30, GH32, GH43, GH51, GH53, GH67, GH73, GH98, and CE13, as compared to the control group, while the PHP group showed significant increases in families GT2, GT5, GT92, GH5, GH10, GH13, GH26, GH28, GH43, GH51, GH53, GH67, GH115, PL10, and PL11, as compared to the control group. We hypothesized that due to the different stimulating effects of FOS as a single-substrate dietary fiber and psyllium husk powder, as a complex dietary fiber on the CAZymes of the intestinal microbiota, these CAZymes could influence polysaccharide digestion, which supported the dietary structure transition in calves after weaning. Additionally, the promotion of different intestinal bacterial populations in calf intestines may be facilitated by various substrates.
Our study found that supplementation with different dietary fibers significantly influenced the fecal microbiota composition and functional metabolism in calves. In the PHP group, the dominant symbiotic bacterial community at the genus level comprised Prevotella, Clostridium, and Firmicutes, exhibiting a unique metabolic synergy. Studies have shown that certain Clostridium and Firmicutes strains (e.g., Clostridium sp. CAG:914, Clostridium sp. CAG:302, Firmicutes bacterium CAG:321, and Firmicutes bacterium CAG:884) are negatively correlated with short-chain fatty acids (SCFAs) levels (Zhao et al., 2022), suggesting that these strains may act as SCFAs consumers. In contrast, Prevotella strains (e.g., Prevotella sp. TF2-5 and Prevotella sp. P5-50) decompose plant fibers to generate SCFAs (Accetto and Avguštin, 2019), while Prevotella sp. P4-76 enhances mucin-layer carbohydrate metabolism and promotes succinate production, which is subsequently converted into propionate (an SCFA) via the succinate-propionate pathway (Le Bastard et al., 2020b). A key role of psyllium husk powder lies in its ability to stimulate mucin production (Martellet et al., 2022), thereby providing abundant metabolic substrates for Prevotella strains, significantly enhancing their carbohydrate-degrading capacity and increasing SCFA and succinate production. For instance, Prevotella sp. AM42-24 exhibits polysaccharide degradation and fermentation capabilities in the rumen (Shinkai et al., 2022), while Prevotella multisaccharivorax, a dominant species in the calf gastrointestinal tract, primarily ferments carbohydrates to produce acetate and succinate (Wang et al., 2023), further highlighting the central metabolic role of Prevotella in the PHP group. These metabolic products are subsequently utilized by specific Firmicutes and Clostridium strains, forming a mutualistic symbiotic relationship: Prevotella acts as an SCFA “producer”, while certain Firmicutes and Clostridium strains function as “consumers”, depending on these metabolic products for growth. This producer-consumer synergy contributes to the stabilization of the intestinal microenvironment (Figure 10). In contrast, the INU group exhibited a weaker symbiotic relationship among Prevotella, Clostridium, and Firmicutes, lacking the same level of metabolic synergy observed in the PHP group. Therefore, psyllium husk plays a crucial role in modulating gut microbiota structure and function by promoting mucin production, enhancing Prevotella metabolic activity, and supporting the utilization of SCFAs by downstream microbial communities. This mechanism not only reveals the intrinsic symbiotic interactions within the PHP group but also provides a scientific basis for dietary fiber interventions aimed at improving gut health, highlighting the unique potential of psyllium husk in maintaining intestinal microbial homeostasis.
Figure 10. Schematic representation of symbiotic gut microbiota and metabolic pathways in calves (Created in https://BioRender.com).
In conclusion, this study investigated the effects of inulin and psyllium husk powder supplementation on the composition of the intestinal microbiota in pre-weaned calves, as well as the KEGG and CAZymes functions of the intestinal microbiota. This study found that both inulin and psyllium husk powder increased the abundance of the intestinal microbiota to a certain extent, while also promoting the colonization of beneficial bacteria, such as Bifidobacterium, Prevotella, and Sharpea at various stages before weaning. It is theorized that Bifidobacterium protects the intestinal epithelium and preserves intestinal health by inhibiting the activity of the secondary bile acid metabolism pathway, whereas Prevotella produces flavonoid metabolites to preserve intestinal health by promoting the flavonoid metabolism pathway. Furthermore, supplementation with various dietary fibers resulted in upregulation of CAZymes in the calf intestinal microbiota, indicating that dietary fiber supplementation during the calf gastrointestinal transition stage may affect the carbohydrate degradation capacity of the intestines, which warrants further investigation into its influence on subsequent production performance. This study demonstrated the potential of supplementing pre-weaned calves with dietary fiber to improve the structure of the calf intestinal microbiota, promote the colonization of beneficial microorganisms, and protect intestinal health, thus providing deeper insights into the regulatory effects of various dietary fibers on the calf intestinal microbiota.
The raw data supporting the findings of this study have been deposited in the National Microbiology Data Center (NMDC) under the Project ID: NMDC10019387. These data are publicly accessible and provide comprehensive information for reproducing the results reported in this study. Researchers interested in further analyses or validations are encouraged to utilize this dataset.
The animal study was approved by China Agricultural University Laboratory Animal Welfare and Animal Experimental Ethical Review Committee. The study was conducted in accordance with the local legislation and institutional requirements.
WL: Data curation, Methodology, Software, Visualization, Writing – original draft. XY: Data curation, Investigation, Methodology, Supervision, Writing – review & editing. YG: Data curation, Writing – review & editing. XZ: Formal analysis, Writing – review & editing. KS: Formal analysis, Writing – review & editing. HZ: Methodology, Writing – review & editing. ZD: Data curation, Methodology, Writing – review & editing. DL: Investigation, Resources, Writing – review & editing. JC: Supervision, Writing – review & editing. CM: Project administration, Resources, Writing – review & editing.
The author(s) declare that financial support was received for the research and/or publication of this article. This research was supported by China Agriculture University of Veterinary Medicine.
DL was employed by Hebei Shounong Modern Agricultural Technology Co., LTD.
The remaining authors declare that the research was conducted in the absence of any commercial or financial relationships that could be construed as a potential conflict of interest.
The author(s) declare that no Gen AI was used in the creation of this manuscript.
All claims expressed in this article are solely those of the authors and do not necessarily represent those of their affiliated organizations, or those of the publisher, the editors and the reviewers. Any product that may be evaluated in this article, or claim that may be made by its manufacturer, is not guaranteed or endorsed by the publisher.
The Supplementary Material for this article can be found online at: https://www.frontiersin.org/articles/10.3389/fmicb.2025.1554484/full#supplementary-material
305ME, 305-day Mature Equivalent Milk Production; ADG, Average Daily Gain; NAHMS, National Animal Health Monitoring System; CAZy, Carbohydrate-Active EnZYmes; INU, Inulin Group; PHP, Psyllium Husk Powder Group; CON, Control Group; ACE, Abundance-based Coverage Estimator; CPCoA, Canonical Principal Coordinate Analysis; ANOVA, Analysis of Variance; HSD, Honest Significant Difference; LDA, Linear Discriminant Analysis; LEfSe, Linear Discriminant Analysis Effect Size; SCFA, Short-Chain Fatty Acids; IPA, Indole-3-Propionic Acid; CAZymes, Carbohydrate-Active Enzymes; CHs, Glycosyl Hydrolases; GTs, Glycosyl Transferases; CBMs, Carbohydrate-Binding Modules; PLs, Polysaccharide Lyases; CEs, Carbohydrate Esterases; KEGG, Kyoto Encyclopedia of Genes and Genomes; STAMP, Statistical Analysis of Taxonomic and Functional Profiles; WGCNA, Weighted Correlation Network Analysis.
Abuelo, A., Cullens, F., and Brester, J. L. (2021). Effect of preweaning disease on the reproductive performance and first-lactation milk production of heifers in a large dairy herd. J. Dairy Sci. 104, 7008–7017. doi: 10.3168/jds.2020-19791
Accetto, T., and Avguštin, G. (2019). The diverse and extensive plant polysaccharide degradative apparatuses of the rumen and hindgut Prevotella species: a factor in their ubiquity? Syst. Appl. Microbiol. 42, 107–116. doi: 10.1016/j.syapm.2018.10.001
Bastian, M., Heymann, S., and Jacomy, M. (2009). “Gephi: an open source software for exploring and manipulating networks,” in: Proceedings of the International AAAI Conference on Web and Social Media, 361–362.
Belkaid, Y., and Hand, T. W. (2014). Role of the microbiota in immunity and inflammation. Cell 157, 121–141. doi: 10.1016/j.cell.2014.03.011
Belorio, M., and Gómez, M. (2021). Psyllium: a useful functional ingredient in food systems. Crit. Rev. Food Sci. Nutr. 62, 527–538. doi: 10.1080/10408398.2020.1822276
Blaak, E., Canfora, E., Theis, S., Frost, G., Groen, A., Mithieux, G., et al. (2020). Short chain fatty acids in human gut and metabolic health. Benef. Microb. 11, 411–455. doi: 10.3920/BM2020.0057
Chen, N., Liu, Y., Wei, S., Zong, X., Zhou, G., Lu, Z., et al. (2023). Dynamic changes of inulin utilization associated with longitudinal development of gut microbiota. Int. J. Biol. Macromol. 229, 952–963. doi: 10.1016/j.ijbiomac.2022.12.318
Chen, T., Long, W., Zhang, C., Liu, S., Zhao, L., and Hamaker, B. R. (2017). Fiber-utilizing capacity varies in Prevotella-versus Bacteroides-dominated gut microbiota. Sci. Rep. 7:2594. doi: 10.1038/s41598-017-02995-4
Cho, Y. I., and Yoon, K. J. (2014). An overview of calf diarrhea—infectious etiology, diagnosis, and intervention. J. Vet. Sci. 15, 1–17. doi: 10.4142/jvs.2014.15.1.1
Cronin, P., Joyce, S. A., O'Toole, P. W., and O'Connor, E. M. (2021). Dietary fibre modulates the gut microbiota. Nutrients 13:1655. doi: 10.3390/nu13051655
Dablool, A. S., Atwah, B., Alghamdi, S., Momenah, M. A., Saleh, O., Alhazmi, N., et al. (2024). Could Paenibacillus xylanexedens MS58 be an ecofriendly antibiotic in poultry production? Impacts on performance, blood biochemistry, gut microbiota and meat quality. Pak. Vet. J. 44. doi: 10.29261/pakvetj/2024.180
Du, W., Wang, X., Hu, M., Hou, J., Du, Y., Si, W., et al. (2023). Modulating gastrointestinal microbiota to alleviate diarrhea in calves. Front. Microbiol. 14:1181545. doi: 10.3389/fmicb.2023.1181545
Elihasridas, Z. M., Ningrat, R. W. S., Erpomen, P. E. M., and Makmur, M. (2023). In Vitro Nutrient Digestibility and Ruminal Fermentation Characteristics of Ammoniated and Fermented Treatment of Soaked and Unsoaked Cymbopogon nardus Waste. doi: 10.47278/journal.ijvs/2022.204
Fauzia, A., Roni, P., Novirman, J., and Hanannaisa, S. (2024). Exploring the impact of processed cassava peel on microbial dynamics and in vitro nutrient digestibility in ruminant diets. Int. J. Vet. Sci. 13, 463–470. doi: 10.47278/journal.ijvs/2023.119
Foster, D. M., and Smith, G. W. (2009). Pathophysiology of diarrhea in calves. Vet. Clin. North Am. Food Anim. Pract. 25, 13–36. doi: 10.1016/j.cvfa.2008.10.013
Garg, P., Garg, P. K., and Bhattacharya, K. (2024). Psyllium husk positively alters gut microbiota, decreases inflammation, and has bowel-regulatory action, paving the way for physiologic management of irritable bowel syndrome. Gastroenterology 166, 545–546. doi: 10.1053/j.gastro.2023.11.019
Gill, S. K., Rossi, M., Bajka, B., and Whelan, K. (2021). Dietary fibre in gastrointestinal health and disease. Nat. Rev. Gastroenterol. Hepatol. 18, 101–116. doi: 10.1038/s41575-020-00375-4
Helbert, W., Poulet, L., Drouillard, S., Mathieu, S., Loiodice, M., Couturier, M., et al. (2019). Discovery of novel carbohydrate-active enzymes through the rational exploration of the protein sequences space. Proc. Natl. Acad. Sci. 116, 6063–6068. doi: 10.1073/pnas.1815791116
Hennessy, M. L., Indugu, N., Vecchiarelli, B., Bender, J., Pappalardo, C., Leibstein, M., et al. (2020). Temporal changes in the fecal bacterial community in Holstein dairy calves from birth through the transition to a solid diet. PLoS ONE 15:e0238882. doi: 10.1371/journal.pone.0238882
Hu, D., Liu, W., Yu, W., Huang, L., Ji, C., Liu, X., et al. (2023). Psyllium seed husk regulates the gut microbiota and improves mucosal barrier injury in the colon to attenuate renal injury in 5/6 nephrectomy rats. Renal Fail. 45:2197076. doi: 10.1080/0886022X.2023.2197076
Huang, L., Zhang, H., Wu, P., Entwistle, S., Li, X., Yohe, T., et al. (2018). dbCAN-seq: a database of carbohydrate-active enzyme (CAZyme) sequence and annotation. Nucleic Acids Res. 46, D516–D521. doi: 10.1093/nar/gkx894
Jia, W., Wei, M., Rajani, C., and Zheng, X. (2021). Targeting the alternative bile acid synthetic pathway for metabolic diseases. Prot. Cell 12, 411–425. doi: 10.1007/s13238-020-00804-9
Jiajun, H., Zhenzhou, W., Liu, Y., Li, L., Guoqiang, Z., Dangdang, W., et al. (2024). Effects of dietary supplementation with Clostridium butyricum on rumen fermentation, rumen microbiota and feces in beef cattle. Kafkas Üniversitesi Veteriner Fakültesi Dergisi 30, 325–333. doi: 10.9775/kvfd.2023.31114
Kamke, J., Kittelmann, S., Soni, P., Li, Y., Tavendale, M., Ganesh, S., et al. (2016). Rumen metagenome and metatranscriptome analyses of low methane yield sheep reveals a Sharpea-enriched microbiome characterised by lactic acid formation and utilisation. Microbiome 4, 1–16. doi: 10.1186/s40168-016-0201-2
Kayama, H., Okumura, R., and Takeda, K. (2020). Interaction between the microbiota, epithelia, and immune cells in the intestine. Ann. Rev. Immunol. 38, 23–48. doi: 10.1146/annurev-immunol-070119-115104
La Reau, A. J., and Suen, G. (2018). The ruminococci: key symbionts of the gut ecosystem. J. Microbiol. 56, 199–208. doi: 10.1007/s12275-018-8024-4
Lai, H., Li, Y., He, Y., Chen, F., Mi, B., Li, J., et al. (2023). Effects of dietary fibers or probiotics on functional constipation symptoms and roles of gut microbiota: a double-blinded randomized placebo trial. Gut Microbes 15:2197837. doi: 10.1080/19490976.2023.2197837
Langfelder, P., and Horvath, S. (2008). WGCNA: an R package for weighted correlation network analysis. BMC Bioinform. 9, 1–13. doi: 10.1186/1471-2105-9-559
Le Bastard, Q., Chapelet, G., Birgand, G., Hillmann, B. M., Javaudin, F., Hayatgheib, N., et al. (2020b). Gut microbiome signatures of nursing home residents carrying Enterobacteria producing extended-spectrum β-lactamases. Antimicrob. Resist. Infect. Control 9:107. doi: 10.1186/s13756-020-00773-y
Le Bastard, Q., Chapelet, G., Javaudin, F., Lepelletier, D., Batard, E., and Montassier, E. (2020a). The effects of inulin on gut microbial composition: a systematic review of evidence from human studies. Eur. J. Clin. Microbiol. Infect. Dis. 39, 403–413. doi: 10.1007/s10096-019-03721-w
Lin, L., Xie, F., Sun, D., Liu, J., Zhu, W., and Mao, S. (2019). Ruminal microbiome-host crosstalk stimulates the development of the ruminal epithelium in a lamb model. Microbiome 7, 1–16. doi: 10.1186/s40168-019-0701-y
Liu, L., Dong, W., Wang, S., Zhang, Y., Liu, T., Xie, R., et al. (2018). Deoxycholic acid disrupts the intestinal mucosal barrier and promotes intestinal tumorigenesis. Food Funct. 9, 5588–5597. doi: 10.1039/C8FO01143E
Liu, Y., Zhang, S., Zhou, W., Hu, D., Xu, H., and Ji, G. (2022). Secondary bile acids and tumorigenesis in colorectal cancer. Front. Oncol. 12:813745. doi: 10.3389/fonc.2022.813745
Liu, Y.-X., Qin, Y., Chen, T., Lu, M., Qian, X., Guo, X., et al. (2020). A practical guide to amplicon and metagenomic analysis of microbiome data. Prot. Cell 12, 315–330. doi: 10.1007/s13238-020-00724-8
Lucas, S., Omata, Y., Hofmann, J., Böttcher, M., Iljazovic, A., Sarter, K., et al. (2018). Short-chain fatty acids regulate systemic bone mass and protect from pathological bone loss. Nat. Commun. 9:55. doi: 10.1038/s41467-017-02490-4
Malmuthuge, N., and Guan, L. L. (2017). Understanding the gut microbiome of dairy calves: opportunities to improve early-life gut health1. J. Dairy Sci. 100, 5996–6005. doi: 10.3168/jds.2016-12239
Martellet, M. C., Majolo, F., Ducati, R. G., Volken de Souza, C. F., and Goettert, M. I. (2022). Probiotic applications associated with psyllium fiber as prebiotics geared to a healthy intestinal microbiota: a review. Nutrition 103–104:111772. doi: 10.1016/j.nut.2022.111772
McGuirk, S. M. (2008). Disease management of dairy calves and heifers. Vet. Clin. North Am. Food Anim. Pract. 24, 139–153. doi: 10.1016/j.cvfa.2007.10.003
McGuirk, S. M., and Peek, S. F. (2014). Timely diagnosis of dairy calf respiratory disease using a standardized scoring system. Anim. Health Res. Rev. 15, 145–147. doi: 10.1017/S1466252314000267
NAHMS (2021). Morbidity and Mortality in U.S. Preweaned Dairy Heifer Calves NAHM Dairy 2014 Study Calf Component. NAHMS. Available online at: https://www.aphis.usda.gov/sites/default/files/morb-mort-us-prewean-dairyheifer-nahms-2014.pdf
Oteiza, P., Fraga, C. G., Mills, D., and Taft, D. (2018). Flavonoids and the gastrointestinal tract: local and systemic effects. Mol. Aspects Med. 61, 41–49. doi: 10.1016/j.mam.2018.01.001
Penumutchu, S., Korry, B. J., Hewlett, K., and Belenky, P. (2023). Fiber supplementation protects from antibiotic-induced gut microbiome dysbiosis by modulating gut redox potential. Nat. Commun. 14:5161. doi: 10.1038/s41467-023-40553-x
Rivière, A., Selak, M., Lantin, D., Leroy, F., and De Vuyst, L. (2016). Bifidobacteria and butyrate-producing colon bacteria: importance and strategies for their stimulation in the human gut. Front. Microbiol. 7:979. doi: 10.3389/fmicb.2016.00979
Rodríguez, J. M., Murphy, K., Stanton, C., Ross, R. P., Kober, O. I., Juge, N., et al. (2015). The composition of the gut microbiota throughout life, with an emphasis on early life. Microb. Ecol. Health Dis. 26:26050. doi: 10.3402/mehd.v26.26050
Rosario, D., Bidkhori, G., Lee, S., Bedarf, J., Hildebrand, F., Le Chatelier, E., et al. (2021). Systematic analysis of gut microbiome reveals the role of bacterial folate and homocysteine metabolism in Parkinson's disease. Cell Rep. 34:108807. doi: 10.1016/j.celrep.2021.108807
Sarkar, A., Yoo, J. Y., Valeria Ozorio Dutra, S., Morgan, K. H., and Groer, M. (2021). The association between early-life gut microbiota and long-term health and diseases. J. Clin. Med. 10:459. doi: 10.3390/jcm10030459
Shinkai, T., Ikeyama, N., Kumagai, M., Ohmori, H., Sakamoto, M., Ohkuma, M., et al. (2022). Prevotella lacticifex sp. nov., isolated from the rumen of cows. Int. J. Syst. Evol. Microbiol. 72:5278. doi: 10.1099/ijsem.0.005278
Shoaib, M., Shehzad, A., Omar, M., Rakha, A., Raza, H., Sharif, H. R., et al. (2016). Inulin: Properties, health benefits and food applications. Carbohyd. Polym. 147, 444–454. doi: 10.1016/j.carbpol.2016.04.020
Silva, Y. P., Bernardi, A., and Frozza, R. L. (2020). The role of short-chain fatty acids from gut microbiota in gut-brain communication. Front. Endocrinol. 11:508738. doi: 10.3389/fendo.2020.00025
Stenman, L. K., Holma, R., Eggert, A., and Korpela, R. (2013). A novel mechanism for gut barrier dysfunction by dietary fat: epithelial disruption by hydrophobic bile acids. Am. J. Physiol. Gastrointest. Liver Physiol. 304, G227–G234. doi: 10.1152/ajpgi.00267.2012
Urie, N. J., Lombard, J. E., Shivley, C. B., Kopral, C. A., Adams, A. E., Earleywine, T. J., et al. (2018). Preweaned heifer management on US dairy operations: Part V. Factors associated with morbidity and mortality in preweaned dairy heifer calves. J. Dairy Sci. 101, 9229–9244. doi: 10.3168/jds.2017-14019
Valero-Mora, P. M. (2010). ggplot2: elegant graphics for data analysis. J. Statist. Softw. Book Rev. 35, 1–3. doi: 10.18637/jss.v035.b01
Wang, J., Zhao, K., Li, M., Fan, H., Wang, M., Xia, S., et al. (2023). A preliminary study of the potential molecular mechanisms of individual growth and rumen development in calves with different feeding patterns. Microorganisms 11:2423. doi: 10.3390/microorganisms11102423
Xiong, Y.-J., Deng, Z.-B., Liu, J.-N., Qiu, J.-J., Guo, L., Feng, P.-P., et al. (2019). Enhancement of epithelial cell autophagy induced by sinensetin alleviates epithelial barrier dysfunction in colitis. Pharmacol. Res. 148:104461. doi: 10.1016/j.phrs.2019.104461
Ye, S., Shah, B. R., Li, J., Liang, H., Zhan, F., Geng, F., et al. (2022). A critical review on interplay between dietary fibers and gut microbiota. Trends Food Sci. Technol. 124, 237–249. doi: 10.1016/j.tifs.2022.04.010
Yue, S., Li, X., Qian, J., Du, J., Liu, X., Xu, H., et al. (2023). Impact of enzymatic hydrolyzed protein feeding on rumen microbial population, blood metabolites and performance parameters of lactating dairy cows. Pak. Vet. J. 43. doi: 10.29261/pakvetj/2023.081
Zhang, H., Yohe, T., Huang, L., Entwistle, S., Wu, P., Yang, Z., et al. (2018). dbCAN2: a meta server for automated carbohydrate-active enzyme annotation. Nucl. Acids Res. 46, W95–W101. doi: 10.1093/nar/gky418
Zhang, Z., Zhang, G., Zhang, S., and Zhao, J. (2022). Fructooligosaccharide reduces weanling pig diarrhea in conjunction with improving intestinal antioxidase activity and tight junction protein expression. Nutrients 14:512. doi: 10.3390/nu14030512
Zhao, G., Xiang, Y., Wang, X., Dai, B., Zhang, X., Ma, L., et al. (2022). Exploring the possible link between the gut microbiome and fat deposition in pigs. Oxid. Med. Cell. Long. 2022, 1–13. doi: 10.1155/2022/1098892
Zhao, Z.-H., Xin, F.-Z., Xue, Y., Hu, Z., Han, Y., Ma, F., et al. (2019). Indole-3-propionic acid inhibits gut dysbiosis and endotoxin leakage to attenuate steatohepatitis in rats. Exp. Mol. Med. 51, 1–14. doi: 10.1038/s12276-019-0304-5
Zheng, J., Ge, Q., Yan, Y., Zhang, X., Huang, L., and Yin, Y. (2023). dbCAN3: automated carbohydrate-active enzyme and substrate annotation. Nucl. Acids Res. 51, W115–W121. doi: 10.1093/nar/gkad328
Zhou, M., Zeng, Y., Zhou, W., Zhu, H., Xing, Y., Dong, X., et al. (2024). Supplementation of Laminaria japonica powder influence ruminal microbiota without affecting ruminal fermentation in bulls. Pak. Vet. J. 44. doi: 10.29261/pakvetj/2024.265
Zhou, P., Chen, C., Patil, S., and Dong, S. (2024). Unveiling the therapeutic symphony of probiotics, prebiotics, and postbiotics in gut-immune harmony. Front. Nutr. 11:1355542. doi: 10.3389/fnut.2024.1355542
Zhuang, K., Shu, X., Meng, W., and Zhang, D. (2024). Blended-protein changes body weight gain and intestinal tissue morphology in rats by regulating arachidonic acid metabolism and secondary bile acid biosynthesis induced by gut microbiota. Eur. J. Nutr. 63, 1605–1621. doi: 10.1007/s00394-024-03359-1
Keywords: calves, dietary fiber, gastrointestinal health, Bifidobacterium, Prevotella, microbial interactions, intestinal homeostasis
Citation: Lu W, Yi X, Ge Y, Zhang X, Shen K, Zhuang H, Deng Z, Liu D, Cao J and Ma C (2025) Effects of dietary fiber on the composition, function, and symbiotic interactions of intestinal microbiota in pre-weaned calves. Front. Microbiol. 16:1554484. doi: 10.3389/fmicb.2025.1554484
Received: 02 January 2025; Accepted: 07 March 2025;
Published: 25 March 2025.
Edited by:
Klibs N. Galvao, University of Florida, United StatesReviewed by:
Aisha Khatoon, University of Agriculture, Faisalabad, PakistanCopyright © 2025 Lu, Yi, Ge, Zhang, Shen, Zhuang, Deng, Liu, Cao and Ma. This is an open-access article distributed under the terms of the Creative Commons Attribution License (CC BY). The use, distribution or reproduction in other forums is permitted, provided the original author(s) and the copyright owner(s) are credited and that the original publication in this journal is cited, in accordance with accepted academic practice. No use, distribution or reproduction is permitted which does not comply with these terms.
*Correspondence: Jie Cao, Y2FvamllMDEwQDE2My5jb20=; Chong Ma, bWFjaF9jYXVAY2F1LmVkdS5jbg==
Disclaimer: All claims expressed in this article are solely those of the authors and do not necessarily represent those of their affiliated organizations, or those of the publisher, the editors and the reviewers. Any product that may be evaluated in this article or claim that may be made by its manufacturer is not guaranteed or endorsed by the publisher.
Research integrity at Frontiers
Learn more about the work of our research integrity team to safeguard the quality of each article we publish.