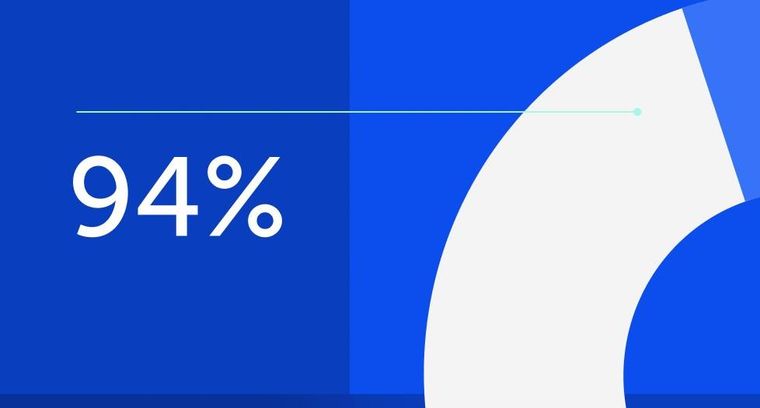
94% of researchers rate our articles as excellent or good
Learn more about the work of our research integrity team to safeguard the quality of each article we publish.
Find out more
ORIGINAL RESEARCH article
Front. Microbiol., 12 February 2025
Sec. Infectious Agents and Disease
Volume 16 - 2025 | https://doi.org/10.3389/fmicb.2025.1544124
Background: Our study examined the composition of the intestinal microflora in a hospitalized patient with AP symptoms treated several months earlier for diverticulitis. The therapeutic intervention necessitated Hartmann's procedure, culminating in colostomy creation.
Aims: Employing a thorough microbiological analysis we attempted to demonstrate whether the microflora isolated from the peripancreatic fluid exhibited a stronger correlation with the contents of the stoma or with the rectal swab. Additionally, we sought to determine the association between later onset of AP and diverticulitis.
Methods: Following clinical materials from the patient in the initial phase of AP were collected: rectal swab, colostomy bag contents (in the publication referred to as stoma content/stool) and peripancreatic fluid. Microbiological analysis was performed, including classic culture methodology, NGS techniques, and genotyping methodologies. Furthermore, the effect of bile on the shift in the population of selected bacterial species was examined.
Results: The NGS technique confirmed greater consistency in bacteria percentage (phyla/family) between stoma content and peripancreatic fluid. In both samples, a clear dominance of the Proteobacteria phyla (over 75%) and the Enterobacteriaceae family was demonstrated. Moreover, NGS verified the presence of the Fusobacteriota phylum and Fusobacteriaceae family only in rectal swabs, which may indicate a link between this type of bacteria and the etiology of diverticulitis. We observed that Escherichia coli 33 isolated from stool exhibited active gaseous metabolite production (mainly hydrogen).
Conclusions: The abundant production of hydrogen may substantially impact enzymatic processes, inducing specific alterations in disulfide bonds and trypsin inactivation. Our investigation alludes to the conceivable active involvement of bile in effecting qualitative and quantitative modifications in the peripancreatic microbiota composition, establishing a correlation between released bile and bacterial generation of gaseous metabolites.
According to the most recent epidemiological data and literature, a marked surge in the incidence of acute pancreatitis (AP) among middle-aged and elderly populations in developed nations has become apparent (Pendharkar et al., 2017). Notably, regions with a high AP prevalence (exceeding 30 cases per 100,000) include the United States and Canada. In contrast, European countries exhibit AP prevalence ranging from 29 to 12 cases per 100,000 inhabitants annually (Petrov and Yadav, 2019). Until now, gastroenterologists, surgeons, and epidemiologists have subscribed to the prevailing belief that prominent predisposing factors for AP encompass gallbladder and bile duct dysfunction, alcohol misuse, immunosuppressive agents, a stress-laden lifestyle, improper dietary practices, escalating obesity rates, abdominal traumas, and parathyroid gland hyperfunction (Wang et al., 2013). Nevertheless, gallstones persist as the foremost etiological factor in AP (constituting 45% of the cases), closely followed by alcohol misuse (20% of the total number of cases) (Boxhoorn et al., 2020; Hu et al., 2021).
Contemporary investigations by specific research cohorts have recently postulated an association between AP, ongoing intestinal inflammation, and surgical interventions performed on the intestines, such as those involving a stoma creation (Jessen et al., 2021).
AP manifests through both localized and systemic inflammatory reactions, classified into distinct categories as mild AP (MAP), moderately severe AP (MSAP), and severe AP (SAP), as per the 2012 revised Atlanta Classification proposed by Banks et al. (2012). The overall mortality rate associated with AP is generally reported at 1%−3%, yet in cases where SAP is present, the mortality rate can escalate markedly, reaching up to 30% (Hu et al., 2021; Petrov et al., 2010).
Previously established knowledge underscores the negligible involvement of the human digestive tract microflora in MAP and MSAP onset. This assertion rests upon the infrequency of microbiological analyses conducted on clinical samples derived from the peripancreatic space in individuals presenting with the initial stages of pancreatitis. Moreover, when such investigations are undertaken, microbial cultures are either negative or contaminated with many species characteristic of the gut microbiota. For MAP patients, the condition typically follows a self-limiting course with conservative management. In instances of MSAP, neither percutaneous nor surgical interventions are typically undertaken unless there are discernible clinical symptoms suggestive of potential infection. In cases of SAP, bacteria isolated from the peripancreatic space aggressively contribute to ongoing inflammatory processes, swift patient deterioration, tissue necrosis, sepsis, multiorgan failure, and fatality (Patel et al., 2021; Boumitri et al., 2017). Notably, Gram-negative rods (Enterobacterales), alongside Gram-positive cocci (Enterococcus faecalis and Enterococcus faecium), are frequently isolated from necrotizing pancreatitis in SAP patients. In this context, the predominance of Gram-negative rods is particularly implicated as a determinant leading to patient fatality (Patel et al., 2021; Zhu et al., 2019).
Currently, utilizing NGS, the capacity to scrutinize alterations in the microbiome across distinct stages of pancreatitis (encompassing MAP, MSAP, and SAP) has significantly increased. The applicability of NGS analyses extends comprehensively to all gastrointestinal tract samples, even in the aftermath of antibiotic administration, owing to its exclusive reliance on bacterial DNA originating from viable and non-viable bacterial entities.
In our investigative pursuits, we meticulously examined the compositional dynamics of the intestinal microbiota in a hospitalized patient presenting with AP symptoms. This particular patient, previously treated for diverticulitis a few months prior, was slated for the removal of a colostomy following the Hartmann procedure.
Facilitated by an exhaustive microbiological analysis, incorporating classical culture methodology, NGS techniques, and genotyping methodologies, we attempted to elucidate whether the microbiota isolated from the peripancreatic space demonstrated a stronger correlation with the stoma contents or the rectal microbiota. Through comparative assessment of these intestinal microbiotas, our objective was to discern potential associations between subsequent occurrences of acute pancreatitis and a medical history featuring diverticulitis or colostomy procedures.
Furthermore, we sought to investigate the impact of freshly secreted bile on the qualitative and quantitative composition of gas metabolites produced by a specific strain of Escherichia coli, thus expanding our understanding of the intricate interplay between bile constituents and the microbiota in the context of AP.
The microbiological analyses encompassed the procurement of samples (rectal swab, stoma content/stool, and peripancreatic fluid) from a 39-year-old individual presenting with MSAP. The patient's admission to the Surgery Department of the University Hospital at Jagiellonian University Medical College occurred in February 2020, prompted by abdominal pain indicative of large bowel obstruction resulting from acute diverticulitis. The therapeutic intervention necessitated Hartmann's procedure, culminating in the creation of colostomy. After hospital discharge (the patient's condition was satisfactory), a scheduled date for restoring digestive tract continuity was established.
Several days before the planned restorative procedure on June 22, 2020, the patient had experienced a recurrence of severe abdominal pain accompanied by sweating, obstruction, and no colostomy bag contents. AP was confirmed upon conducting blood tests, abdominal ultrasound, and abdominal computed tomography. Imaging studies further identified acute-phase peripancreatic fluid collection. Given the patient's deteriorating general condition, a transfer to the intensive care unit (ICU) ensued for intensified therapeutic measures. In the ICU, a comprehensive array of clinical specimens was systematically collected for meticulous microbiological scrutiny, including blood, urine, rectal swabs, stoma content/stool, and peripancreatic fluid (obtained percutaneously).
Following the initial blood culture, wherein solely Staphylococcus epidermidis was detected (with suspicion of sample contamination), the persistent elevation in body temperature, reaching up to 39 degrees Celsius, prompted the initiation of broad-spectrum empirical antibiotic therapy. The regimen included Ciprofloxacin (three doses of 400 mg), Metronidazole (three doses of 500 mg), Xifaxan (three doses of 200 mg), and Diflucan (two doses of 200 mg). Subsequent blood culture analysis revealed the isolation of Staphylococcus capitis methicillin-resistant coagulase negative staphylococci (MSCNS), exhibiting sensitivity to isoxazolyl penicillins, combinations of penicillins with beta-lactamase inhibitors, cephalosporins (excluding ceftazidime, cefixime, ceftibuten), and carbapenems. Consequently, the antibiotic protocol was modified to incorporate Meropenem (three doses of 2 g), Metronidazole (three doses of 500 mg), Xifaxan (three doses of 200 mg), and Diflucan (two doses of 200 mg).
Throughout this therapeutic course, the patient received oral and parenteral nutrition with commendable tolerance, as evidenced by colostomy discharge. Continuous intravenous patient-controlled intraocular pressure (IOP) anesthesia was maintained. Noteworthy improvements, characterized by a reduction in inflammatory parameters and an enhanced health condition, were discerned on July 6, 2020. Subsequently, the patient (in a favorable condition) was transferred back to the Surgery Department of the University Hospital at Jagiellonian University Medical College in Kraków for ongoing therapeutic interventions.
Microbiological sample collection was conducted following the Declaration of Helsinki and approved by the Bioethics Committee of the Jagiellonian University (opinion number KBET 1072.6120.279).
The specimens acquired from the patient (rectal swab, stoma content/stool, and peripancreatic fluid) were meticulously processed during the onset of exacerbated MSAP clinical manifestation. The Department of Microbiology at Jagiellonian University Medical College conducted qualitative and quantitative microbiological cultures. Post-collection, the materials were transported in sterile containers on dry ice within 2 h. Subsequently, the samples were suspended in Schaedler's liquid medium (Becton, Dickinson and Company, Sparks, MD, USA), precisely weighed, and finely ground using sterile mortars and glass beads. Decimal dilution methodology was employed, and the diluted samples were plated on appropriate agar media.
Distinct agar media were utilized for specific bacterial groups:
• McConkey agar (Oxoid Ltd., Basingstoke, Hampshire, UK) for Enterobacteriaceae,
• Columbia blood agar with 5% sheep blood for streptococci (Oxoid Ltd., Basingstoke, UK),
• BBL Enterococcosel agar (BD, Franklin Lakes, USA) for enterococci,
• Rogosa Agar (Merck, Darmstadt, Germany) for lactobacilli,
• Sabouraud Agar (Merck, Darmstadt, Germany) for Candida spp.,
• Schaedler Agar with 5% sheep blood (BD, Franklin Lakes, USA) for anaerobes (Bacteroides, Clostridium, Fusobacterium),
• TOS Propionate Agar Base (Liofilchem, Roseto degli Abruzzi, Italy) for bifidobacteria.
Incubation durations were set at 37°C for 24 h for aerobic bacteria and 48–72 h for anaerobic bacteria. Colony-forming units (CFU) were computed per 1 g or 1 ml, contingent on the sample type, and converted to percentages for comparative analysis with sequencing data.
Bacterial species identification was authenticated through biochemical tests (API, bioMerieux, l'Etoile, France) and mass spectrometry (MALDI Biotyper, Bruker Scientific LLC, Billerica, MA, USA), adhering to the manufacturer's guidelines. Applying the updated MALDI Biotyper for In Vitro Diagnostic (MBT IVD) Library of mass spectra further ensured precision in bacterial identification.
All samples analyzed by classical microbial culture were parallelly evaluated by the NGS method. Sequencing was performed in the Center of Postgraduate Medical Education laboratory in Warsaw (CPME). Bacterial DNA was isolated from crushed clinical samples suspended in Schadler liquid using the commercial QIAamp DNA Stool Mini Kit and QIAamp DNA Mini Kit (Qiagen; Hilden, Germany), according to the manufacturer's protocol. Metagenomic analysis of the intestinal and pancreatic microflora was performed using hypervariable fragments of the 16S rRNA gene. Sequencing was performed using Ion 16SMetagenomics Kit (Thermo Fisher Scientific, Waltham, MA, USA) on the Ion Torrent Personal Genome Machine (PGM) platform. Briefly, DNA was subjected to the amplification of 16S rRNA libraries. The concentration of each 16S library was determined by bioanalyzer Agilent 2100 and High Sensitivity DNA Analysis Kit (Agilent® Bioanalyzer®, Santa Clara, CA, USA).
The barcoded libraries were prepared using the Ion PGM Template OT2 400 Kit (Fisher Scientific, Waltham, MA, USA) and the Ion OneTouch 2 System (Fisher Scientific, Waltham, MA, USA). A maximum of 32 barcoded 16S samples were sequenced on an Ion 318 v2 chip (Fisher Scientific, Waltham, MA, USA) using the Ion PGM Sequencing 400 Kit (Fisher Scientific, Waltham, MA, USA) according to the manufacturer's instructions.
Unmapped bam files from the PGM were converted into fastq with SamToFastq script (Picard Tools), and the sequences were filtered with a fastq_quality_filter from FASTX-Toolkit (Picard2018toolkit available at http://broadinstitute.github.io/picard/). Bacterial taxa were identified with Mothur version 1.38 (doi: 10.1128/AEM.01541-09). Sequences with more than 10 bases in a homopylymer and with a quality smaller than 20 on a sliding window of 50 bases were removed. Chimeric sequences were removed with chimera vsearch algorithm, implemented in mothur. The 16S rRNA sequences were classified by the Wang method, using the Silva bacterial 16S rRNA database version 138 as a template (doi: 10.1093/nar/gks1219).
Furthermore, in analyzing samples utilizing the NGS method, the CPME laboratory consistently employs standardized S1 and S2 stool specimens obtained from asymptomatic individuals as controls. Notably, the donors (with a median age of 35) refrained from using antibiotics and oral probiotics before stool collection and maintained a normal Body Mass Index (BMI).
Employing the Pulsed-field Gel Electrophoresis (PFGE) technique, the genomic DNA profiles of select bacterial species were meticulously juxtaposed. This analysis facilitated the discernment of the dynamic shifts within the bacterial flora at distinct anatomical levels of the gastrointestinal tract in the patient diagnosed with MSAP. The comparative DNA profiling focused on bacterial species (E. coli and E. faecalis), recurrently isolated from diverse ecological niches.
Bacterial cell suspension and plug preparation adhered to established methodologies outlined in prior publications for E. coli (Ejrnaes et al., 2006) and E. faecalis (Saeedi et al., 2002). Subsequently, E. coli strains' plugs underwent digestion with 25 U of XbaI restriction enzyme (Thermo Fisher Scientific, USA). At the same time, those of E. faecalis were subjected to 25 U SmaI, following the manufacturer's guidelines. All isolates underwent analysis utilizing the CHEF-DR III apparatus (Bio-Rad, Hercules, USA). The electrophoretic conditions for E. coli were defined as follows: initial switch time 2.0 s, final switch time 35.0 s, run time 21 h, gradient 6 V/cm, temperature 14°C. For E. faecalis strains, electrophoresis adhered to the subsequent conditions: initial switch time 5.0 s, final switch time 35.0 s, run time 20 h, gradient 6 V/cm, temperature 14°C. The resulting banding patterns were analyzed rigorously through the GelCompar software (Applied Math, Kortrijk, Belgium).
Quantitative microbiological culture allowed the determination of bacteria types that numerically dominated the remaining bacterial populations. Potentially pathogenic strains from the Enterobacteriaceae, Enterococaceae, and Fusobacteriaceae families cultured from rectal swabs and stoma content/stool were subjected to further tests. Selected virulence factors responsible mainly for lytic and adherent properties were analyzed for these bacterial species.
In the case of E. coli, the PCR reaction was used to check the presence of the following genes: yggG and stcE (encoding zinc-dependent metalloproteases), hlyF (encoding hemolysin), iucC (aerobactin siderophore biosynthesis protein), iutA (aerobactin receptor), ibeA (adhesion to eukaryotic cells), neu (colibactin), pks+ (polyketide-peptide genotoxin known as colibactin) and sfa/foc (determinants for S fimbrial adhesins). The investigation into the presence of virulence genes within the Enterococcus population (asa1, gelE, hyl, cylA, and esp), responsible for the production of aggregation substance, gelatinase, hyaluronidase, cytolysin, and enterococcal surface protein, was systematically undertaken.
In the case of the Fusobacterium population, two genes (fadA and fap2) were analyzed. FadA protein mediates adhesion to and invasion into epithelial and endothelial cells. Fap2 is responsible for coding outer membrane proteins that induce cell death.
According to the manufacturer's instructions, bacterial DNA isolations were performed using the Genomic Mini kit (A&A Biotechnology, Gdynia, Poland). The PCR reactions were done in a BioRad (California, USA) thermocycler according to previously described methodologies (Kuan et al., 2011; Brandal et al., 2012; Yamamoto et al., 1995; Kaczmarek et al., 2012; Liu et al., 2021; Johnson and Stell, 2000; Vankerckhoven et al., 2004; Kashani et al., 2020; Kaplan et al., 2010). A list of all primers used in the study is presented in Table 1.
In the subsequent phase of our in vitro investigations, we systematically examined the impact of varied concentrations of freshly prepared bile (Dehydrate Fresh Bile—Difco Oxgall, Becton-Dickinson, Poland) on the proliferation dynamics of bacterial strains isolated from diverse sample origins within a single patient diagnosed with MSAP.
To execute this, in the formulation of species-specific solid growth media, dehydrated fresh bile was incorporated, resulting in distinct concentrations within the solidified agar: 5, 10, 20, 50, 100, and 200 g/L. The control group consisted of identical culture media lacking the addition of bile. The cultures were cultivated on conventional Petri dishes, 9 cm diameter each, containing 25 ml of agar, and subjected to growth conditions tailored to the unique requirements of individual strains.
The assessment of bacterial growth rates was conducted through a semi-quantitative scale, wherein 3 denoted heavy growth, 2 indicated moderate growth, 1 represented poor growth, and 0 signified an absence of any discernible growth.
Selected bacterial strains (potentially pathogenic strains from the Enterobacteriaceae, Enterococaceae, and Fusobacteriaceae families) were assessed for their ability to release gaseous post-culture metabolites depending on the presence or absence of bile addition in the growth medium. Gaseous bacterial metabolites were collected in suitable 0.6 l gas sampling bags (Tedlar gas sampling bag; Jensen Inert Products, NW, Coral Springs, Florida) for gas chromatography measurements. The bags were tightly connected (using appropriate valves—Figure 1) with a 250 ml culture flask filled with 230 ml of the appropriate solid medium (selection of the medium and culture conditions adapted to the strain—described in the materials and methods). A selected concentration of bile, i.e., 20 g/L, was added to each of these media. This concentration was chosen after analyzing the bile tolerance results of all tested bacteria. Flasks with the same agar media without adding bile served as controls.
Figure 1. Percentage of microbiota distribution by phylum and family level by the NGS method and by the classical culture method. (A) Phylum level—NGS; (B) phylum level—classical culture method; (C) family level—NGS; (D) family level—classical culture method.
Subsequently, 10 ml of a liquid 24-h culture of selected bacteria (population number was about 1 × 108 CFU/ml) was poured onto the surface of the solidified agar in individual flasks. The culture inoculum flasks were securely connected to a specialized gas-collection bag through valves. The cultivation process spanned 5 days, following which qualitative and quantitative analyses of gas samples were conducted utilizing a GC-MS chromatograph QP2020 (Shimadzu, Kyoto, Japan) equipped with a thermal conductivity detector (TCD). An MS line was equipped with an HP-PLOT/U column (Agilent, J&W, 0.530 mm diameter, 30 m length). A TCD line used to analyze hydrogen concentration was equipped with a 5 Å molecular sieve-packed column. The MS line was supplied with a helium carrier gas with a flow rate of 377.5 ml·min−1, while the TCD line was supplied with a nitrogen carrier gas with a 15 ml·min−1 flow rate. The oven temperature during measurements was set at 35°C. Before each measurement, the molecular sieve and the chromatographic column were conditioned at 150°C for 600 s. Quantitative analyses were performed on calibration curves based on certified gas mixtures supplied by Air Liquide Poland. Calibration curves and quantitative analyses were performed on areas of peaks related to particular gases.
Based on the classical culture method, we isolated 28 viable bacterial strains from one MSAP patient (11 from rectal swabs and 17 from stoma content/stool). However, we did not detect any viable bacteria in the peripancreatic fluid (the cumulative result is shown in Table 2).
Table 2. Bacteria isolated from a patient with MSAP by using qualitative and quantitative classical culture method.
However, NGS confirmed the presence of bacterial DNA in all samples, including peripancreatic fluid, which would also indicate the presence of bacteria or bacterial DNA in this place (Figures 1A, C).
Additionally, the NGS technique verified a greater abundance of phylum and bacteria families in rectal swabs compared to stoma content/stool and peripancreatic fluid, with these two last samples showing more remarkable similarity to each other both in phylum (Proteobacteria—over 75%, Bacteroidota—over 12%, Firmicutes—about 10%) and in family percentage distribution (Enterobacteriaceae—about 75%, Bacteroidaceae—about 12% and Ruminococcaceae and Lachnospiraceae—about 5%). Furthermore, NGS verified the presence of the Fusobacteriota phylum and the Fusobacteriaceae family, constituting ~10% of the rectal swab composition. The other two samples did not identify these microbial components—stoma content/stool and peripancreatic fluid. The concordance of these findings was corroborated through culture methods, where viable Fusobacterium nucleatum was exclusively identified in the rectal swab.
Utilizing the NGS method, a comparative analysis of the microflora distribution percentage at both the phylum and family levels was facilitated in stool samples obtained from two healthy donors, S1 and S2 (internal laboratory controls). This comparative assessment was conducted in relation to the stoma content/stool derived from a patient diagnosed with MSAP. We observed fascinating differences; firstly, in healthy control donors, the distribution of phylum is dominated mainly by anaerobic and microaerophilic bacteria, i.e., Bacteroidota (over 42%), then Firmicutes (over 30%), Proteobacteria (about 5%; Figure 2A).
Figure 2. Percentage distribution of microbiota by phylum and family level determined by the NGS method. (A) Phylum level—NGS. (B) Family level—NGS; S1—healthy control patient 1, S2—healthy control patient 2, MSAP- moderately severe acute pancreatitis.
In the case of the MSAP patient, aerobic flora had a prevailing presence, notably marked by the dominance of the Proteobacteria phylum, comprising ~77% of the microbial composition. Subsequently, the Bacteroidota phylum accounted for ~12%, followed by the Firmicutes phylum at ~10%. Furthermore, within the Proteobacteria group, the Enterobacteriaceae family, representing aerobic Gram-negative bacilli, emerged as the predominant family.
This observed shift suggests a potential alteration in oxidative-reductive (ox-redox) conditions within the digestive tract. Specifically, the ongoing inflammatory process may have induced a transition from anaerobic to aerobic conditions. Noteworthy is the significant change in the ratio between the two phyla, with anaerobic Bacteroidota surpassing aerobic Proteobacteria at 8:1 in the control group. In contrast, in the MSAP patient, the ratio shifted markedly to almost 1:7, indicating a noteworthy shift in microbial composition (Figures 2A, B).
PFGE was performed for all E. coli (n = 5) and E. faecalis (n = 3) strains isolated from both rectal swabs and stoma content/stool. Analysis of E. coli isolates revealed three different genetic profiles (pulsotypes), which confirmed three separate E. coli strains (10, 25, and 33).
E. coli 18 had an identical profile as E. coli 10, and E. coli 14 had an identical profile as E coli 33. Among three analyzed E. faecalis isolates, two strains isolated from stoma content/stool (E. faecalis 19 and E. faecalis 26) had the same pulsotypes, while E. faecalis 34 isolated from rectal swabs had a unique genetic profile. Detailed results are presented in Figure 3.
Figure 3. Dendrogram of PFGE patterns showing the genetic relatedness of E. coli strains (A) and E. faecalis strains (B). The scale shows the percentage of similarity among different strains. Dice coefficient and unweighted pair group method with arithmetic mean UPGMA was used for cluster analysis; isolates with more than 95% similarity were clustered together as identical. Escherichia coli 14 and 33, 10 and 18 were identical pulsotypes. Among the Enterococcus faecalis strains, 19 and 26 were identical pulsotypes.
In all E. coli strains, we detected the presence of genes for hemolysin (hlyF), aerobactin (iutA), aerobactin siderophore biosynthesis protein (iucC), and polyketide-peptide genotoxin known as colibactin (pks+).
Strains of E. faecalis possessed genes encoding hydrolysis of gelatin, collagen, casein, hemoglobin (gels), and a gene responsible for increasing biofilm production (esp).
We detected fadA gene, responsible for adhesion to and invasion into epithelial and endothelial cells in the F. nucleatum strain isolated from a rectal swab. The detailed virulence profile of all the tested strains is shown in Table 3.
Our in vitro investigations examined the impact of varying concentrations of fresh bile on the growth rates of strains isolated from diverse sample origins within a single patient diagnosed with MSAP. Notably, strains belonging to the Enterococcus spp. exhibited the highest tolerance to bile, showcasing resilience across a broad spectrum of concentrations ranging from 5 to 200 g/L. Conversely, F. nucleatum displayed comparatively lower resistance, manifesting limited growth, particularly at a bile concentration of 50 g/L.
Interestingly, all tested strains demonstrated robust growth at a bile concentration of 20 g/L, signifying a pivotal threshold. Consequently, this concentration was strategically chosen for subsequent studies, owing to its consistent and favorable impact on the growth behavior of the microbial strains under examination.
The capacity for generating gaseous metabolites was assessed across all extant bacterial strains delineated in Table 4. Among these, the E. coli 33 sourced from stoma content/stool and E. coli 14 obtained from rectal swabs demonstrated proliferation within the medium containing 20 g/L bile. These strains exhibited a sufficient production of gaseous metabolites, evidenced by visible inflation of the collection bag, as depicted in Figure 4. However, thrice-repeated gas measurements showed that E. coli 33 released a markedly higher quantity of gas metabolites than E. coli 14. Consequently, we proceeded exclusively with the E. coli 33 for subsequent chromatographic examinations. Conversely, no discernible gaseous emissions were noted within the gas sampling bags from the remaining bacterial strains enumerated in Table 4.
Figure 4. The effect of bile on gaseous metabolite production. (Left) E.coli 33 was grown on MacConkey agar medium with 20 g/L bile—a visible gas-filled bag. (Right) The same strain of E. coli 33 is grown on MacConkey agar with no bile—much lower gas production was observed.
The gas chromatogram, acquired utilizing the TCD and featuring a retention time (tR) of 1.14 min, reveals conspicuous peaks indicative of hydrogen presence in the scrutinized samples (refer to Figure 5). Evident chromatographic patterns signify a heightened concentration of hydrogen within the analyzed samples, ranging from 13.78% (v1) to 23.02% (v2), as delineated in Table 5. The cumulative volume of the collected gas phase was slightly higher in the system incorporating bile. The average hydrogen production rate by E. coli 33 within MacConkey agar manifested as 3.12 and 5.43 ml/day in electrolytes absent and present with bile, respectively. We deduce that the inclusion of bile in the system increases hydrogen production, stemming from the formation of the hydrogen gas phase due to the combination of atomic hydrogen within MacConkey agar.
Table 5. The total volume of gas samples produced after 6 days by E. coli 33 placed in MacConkey agar and MacConkey agar containing bile.
The Mass Spectrometry (MS) chromatograms revealed the presence of nitrogen and carbon dioxide in the examined samples. Nitrogen in the gas sample derived from a “dead space” within the MacConkey agar during the initial stages of the experiment. Conversely, CO2 was generated by E. coli 33. The intensity of nitrogen peaks suggests a closely comparable concentration irrespective of the applied environmental conditions. A parallel scenario was noted for CO2, with concentrations reaching 27.71 and 26.77% for v1 and v2, respectively. The generation of CO2 in MacConkey agar, devoid and in the presence of bile, amounted to 6.28 and 6.31 ml/day, respectively.
The gut microbiota composition is inherently individualized, exhibiting a distinctive profile for each person. On a normative basis, it encompasses an excess of 1,500 distinct species and spans over 50 phyla. The superior abundance is typically attributed to Bacteroidetes, succeeded by Firmicutes, along with other prevalent phyla, including Proteobacteria, Actinomyces, Actinobacteria, Fusobacteria, and Verrucomicrobia (Qin et al., 2010).
In recent years, there has been a surge in comprehensive investigations on intestinal microbiota composition among individuals afflicted with diverse diseases, encompassing acute, chronic, and autoimmune conditions (Nagata et al., 2022; De Luca and Shoenfeld, 2019; Li et al., 2020).
Shifts in the gut microbiota may also be involved in the pathogenesis of pancreatic disease (including AP), chronic pancreatitis, or pancreatic cancer (PC). Developing screening methods for early pancreatic disease diagnosis is a priority. Metabolic modifications that occur in the functioning of the pancreas affect the composition of the intestinal microbiota. Hence, potential non-invasive biomarkers can be sought among the fecal bacteria and their metabolic products. Such investigations are being carried out toward PC diagnosis (Nagata et al., 2022; Kartal et al., 2022), early hepatocellular carcinoma diagnosis (Li et al., 2020; Ren et al., 2019), and differentiating patients with pancreatic ductal adenocarcinoma and autoimmune pancreatitis (Zhou et al., 2021).
The material commonly employed for investigating the gastrointestinal microbiota (relying on analyzing the 16S rRNA bacterial gene sequence and culturing techniques) predominantly comprises stool samples. However, our study placed particular significance on peripancreatic fluid as the primary material. Herein, we successfully demonstrated the presence of bacterial DNA utilizing NGS despite the absence of viable bacterial growth on the culture medium. This underscores the unique utility of peripancreatic fluid in our research, allowing for a comprehensive exploration of microbial genetic material even in instances where traditional culture methods prove inconclusive.
Obtaining stool samples from patients with gastrointestinal diseases, including AP, can be challenging because patients frequently follow a restricted dietary regime and suffer from constipation. Microbiological cultures can yield falsely negative results when patients undergo broad-spectrum antibiotic therapy. Other materials, such as biopsies, are limited; they are available in selected diseases, e.g., necrotizing pancreatitis. Challenges associated with procuring materials for microbiological assessment may occasionally be misleading and questionable.
The current study by Bassis et al. (2017) indicates the possibility of using the NGS technique for microbiological analysis of rectal swabs as an appropriate alternative for patients with acute pancreatitis, for whom obtaining other clinical samples, including stools, is a significant problem.
The structure of the bacterial communities in the Bassis study was similar between freshly passed stool and rectal swabs collected at different time points in each patient. Rectal swabs are a relatively uncomplicated sample to collect, do not require patient preparation, and can be easily transported to the laboratory. Therefore, rectal swabs may be an acceptable and practical substitute for stool collection and fecal microbiota analysis, which our study confirms.
In our investigation, the patient had previously experienced diverticulitis 6 months prior, subsequently undergoing Hartmann's surgery involving colostomy. By applying NGS analysis on bacterial microflora, we successfully showcased a correlation between the microbial structures found in samples from the stoma and those in the peripancreatic fluid, which may indicate the migration of bacteria from the intestinal lumen, where the diverticulitis process was taking place, to the pancreas and peripancreatic space (resulting in acute pancreatitis). However, the progression was relatively slow, as several months passed from the establishment of the stoma to the manifestation of AP symptoms.
In the rectal swab specimen obtained from a patient diagnosed with MSAP, a discernibly elevated microbial population was observed compared to samples derived from individuals in good health. Notably, there was a pronounced prevalence of aerobic bacteria belonging to the Proteobacteria genus, surpassing the presence of anaerobic and microaerophilic bacterial counterparts. This discovery holds significant scientific merit, particularly in light of Zhou et al.'s (2020) research, which concurrently demonstrated the selective enrichment of Proteobacteria in the intestinal microbiota of individuals affected with pancreatic cancer as opposed to their healthy counterparts.
The patient diagnosed with MSAP exhibited elevated relative abundances of potentially pathogenic bacteria (Enterobacteriaceae and Enterococcus), as revealed by both NGS and culture studies. In their recent investigation, Sexton et al. highlighted that any perturbations in the human microbiome have the potential to instigate immune processes, including inflammation, thereby contributing to the onset of diseases (Sexton et al., 2022).
The prevalence of aerobic bacteria in the intestinal milieu of AP patients may instigate an unfavorable redox potential, thereby impacting intestinal epithelial cells. Imbalances in oxidative stress can manifest as intestinal inflammation and compromise the integrity of the intestinal barrier. Notably, microorganisms, particularly Gram-negative rods (through endotoxin activity,) can incite inflammatory responses, enhance the recruitment of pro-inflammatory cells, stimulate cytokine secretion, elevate susceptibility to oxidative stress, perturb energy dynamics, and inflict damage to DNA, as elucidated by Serra et al. (2018).
The increase in the Proteobacteria population presumably causes a proliferation in the diversity of Enterobacteriaceae and Enterococcaceae, which is reflected in the isolation of three different E. coli and two different E. faecalis genotypes in the examined patient. All three isolated E. coli strains possessed the same set of virulence factors: hlyF (hemolysin-inducing autophagy in eukaryotic cells), iucC (aerobactin siderophore biosynthesis protein), iutA (gene helping in iron acquisition in E. coli by producing siderophores) and pks+ (gene coding genotoxin colibactin that induces DNA damage, cell cycle arrest and mutations in eukaryotic cells) (Sarowska et al., 2019). Similarly, both isolated E. faecalis strains exhibited the presence of the same virulence genes (gelE and esp). The gelatinase, encoded by the gelE gene, plays a crucial role in facilitating the translocation of Enterococcus faecalis across human enterocytes, thereby promoting microbial invasion. Simultaneously, the enterococcal surface protein (Esp) is implicated in the adhesion and colonization processes, underscoring its significance in the initial stages of host-microbe interactions (Kiruthiga et al., 2020). In the available literature, no reports show the relationship between specific virulence gene combinations in the mentioned bacterial species and their ability to cause AP. Nevertheless, all identified virulence factors of E. coli and E. faecalis in our study can be helpful for the bacteria to colonize, multiply, and survive in the peripancreatic space. In 2003, Ammori identified these two species (stemming from the intestinal microbiota) as contributors to necrotic infections in pancreatic tissue (Ammori, 2003). Moreover, Tan et al. (2015) showed a significant increase in the Enterobacteriaceae and Enterococcus population in patients with AP compared to the control group in their multi-hospital prospective clinical study. Therefore, the intestinal flora may play an essential role in the development of AP. Increased levels of potentially pathogenic bacteria, such as Enterobacteriaceae and Enterococcus, may contribute to intestinal microbiota dysbiosis and dysfunction of the intestinal barrier, eventually leading to bacterial translocation (Chen et al., 2011; Bauer et al., 2002; Manichanh et al., 2006). Studies have revealed that intestinal mucosal barrier injury is one of the significant complications of AP. A meta-analysis revealed that 59% of AP patients had associated intestinal barrier injury with increased intestinal mucosal permeability, leading to intestinal bacterial translocation, pancreatic tissue necrosis, and infection (Capurso et al., 2012). Also, in our in vitro studies conducted on human Caco-2 cell lines, we observed that an increased population of E. coli (over 1 × 107 CFU/ml) reduced the secretion of occludin and zonulin-tight junction proteins produced by intestinal epithelial cells, which may directly affect the intestinal barrier tightness (unpublished data).
Interestingly, using the NGS and the classical culture methods, we demonstrated the presence of many Fusobacteria in the rectal swab of a patient with MSAP, which we did not find in the stools of healthy donors (samples S1 and S2). F. nucleatum is a Gram-negative anaerobic bacterium that lives in the oral cavity and produces lipopolysaccharides and other virulence factors, including FadA (adhesin) and Fap2 (outer membrane protein), which play a significant role in causing local diseases. F. nucleatum promotes inflammation (a potent stimulator of inflammatory cytokines) and binds to or attacks many cell types, including oral and colon T cells, keratinocytes, and macrophages (Kinder Haake and Lindemann, 1997). The FadA adhesin enables F. nucleatum to bind host cells in the pancreatic environment by interacting with cadherins (Sun et al., 2019).
The interaction between F. nucleatum and natural killer (NK) cells is mediated by the Fab2 protein, which binds to TIGIT receptors on the NK cell surface. This binding event leads to the impairment of NK cell function, specifically by suppressing their natural cytotoxicity. Consequently, this bacterial interference renders NK cells unable to effectively target and attack cancer cells, as substantiated in colon cancer studies (Gur et al., 2015).
Contemporary medical literature has prominently explored the correlation between Fusobacterium species and acute intra-abdominal infections such as peritonitis, hepatic abscesses, and AP, mainly when linked to previous diverticulitis episodes (Brook and Frazier, 2000; Bawa et al., 2022). In our patient's case, isolating viable F. nucleatum rods from the rectal swab suggests a potential direct association of this bacterial species with a prior episode of acute diverticulitis. Bawa et al. (2022) documented a parallel clinical scenario where recurrent diverticulitis with abdominal pain was linked to F. nucleatum upon detailed microbial investigation. While confirmation is speculative, it is plausible that diverticulitis initiated by Fusobacterium could have disseminated or induced secondary dysbiosis in the peripancreatic space. This speculation gains support from (Del Castillo et al. (2019), who reported a heightened relative abundance of Fusobacterium spp. in samples from pancreatic cancer patients. Furthermore, Mitsuhashi et al. demonstrated correlations between the quantity of F. nucleatum and reduced survival rates in patients with pancreatic ductal adenocarcinoma (PDAC) (Mitsuhashi et al., 2015).
There is also promising research on using these microorganisms as biomarkers in colorectal cancer screening. Guo et al. (2018) showed that testing the ratio of F.nucleatum to Bifidobacterium allows the detection of colorectal cancer with 84.6% sensitivity and 92.3% specificity.
Consequently, monitoring the elevation in Fusobacterium numbers through comprehensive analyses such as NGS and traditional culture methods in rectal swabs and other relevant samples may be a valuable indicator for tracking inflammation progression. Such assessments could be markers for evaluating the risk of the patient's deteriorating condition and influencing survival outcomes.
Bile duct obstructions and gallstone migration cause gallstone-induced pancreatitis. Bile duct and/or pancreatic duct obstruction may lead to a local increase in bile pressure, resulting in irregular digestive enzyme activation and local inflammation. Consequently, it may damage pancreatic tissue and cause uncontrolled bile leakage into the peripancreatic space (Wang et al., 2009).
Bile is an essential substrate added to many bacterial media, which can directly influence the shift of the microbial balance toward species resistant to this factor. Streptococci of the Enterococcus genus and Gram-negative rods of the Escherichia genus are among the bacteria whose population growth and maintenance of population viability strictly depend on the presence of bile in the medium. Therefore, an increase in the E. coli population in the peripancreatic environment under the influence of bile may be a sufficient agent causing intestinal inflammatory process progression as it may lead to the accumulation of lipopolysaccharides (LPS). This endotoxin strongly stimulates immune system cells but may also lead to robust gaseous metabolite production [mainly hydrogen (H2)]. Based on our results, the production of gaseous metabolites under the influence of bile is a strain-dependent feature because only one strain of E. coli 33 isolated from stool (accumulated in the stoma bag) showed active production of gaseous metabolites. In contrast, the other three E. coli strains did not have such spectacular activities despite being cultured in the same conditions.
What function can such hydrogen accumulation play in the inflamed peripancreatic space? The role of hydrogen accumulation in the inflamed peripancreatic space is not fully explained in current literature, particularly regarding the biological functions of gaseous metabolites generated by both host cells and bacteria during the initiation, maintenance, and resolution of inflammatory processes in the intestines. Some research groups exploring the biological functions of different gases have noted that hydrogen (H2), given its reducing properties, may undergo conversion into hydrogen sulfide (H2S). Considering that many biochemical processes can occur in human bodies, such amounts of hydrogen of a reductive nature may significantly affect enzymatic processes through specific changes in disulfide bonds and inactivation of trypsin (He et al., 2023). Mee et al. (1965) reported that atomic hydrogen might attack disulfide bonds, forming sulphydryl groups or hydrogen sulfide and destroying tryptophan (Wimmer and Zarevúcka, 2010). Hydrogen sulfide (H2S) exhibits dual roles, as at low physiological concentrations, it [alongside nitric oxide (NO) and carbon monoxide (CO)] can function as a signaling molecule that regulates vasodilation. This activity contributes to a reduction in blood pressure.
On the other hand, other authors indicate that H2S also adversely affects intestinal peristalsis, which is highly unfavorable in patients with AP. Moreover, H2S has a toxic effect on intestinal epithelial cells and causes clinical symptom exacerbation in patients with ulcerative colitis. H2S also exerts a negative impact on carcinogenesis processes. Studies in cell cultures and a mouse model have shown that colon cancer cells produce large amounts of H2S, which they then use to produce energy, divide, and grow the tumor. Hydrogen sulfide is synthesized by host cells in various physiological systems, including the circulation, gastrointestinal tract, and nervous system. Its production involves two enzymes, and vitamin B6 is a cofactor in this process. Studies, such as those conducted on rats with experimental sepsis induced by colon ligation and puncture, as well as toxic shock triggered by lipopolysaccharide administration, have confirmed an upsurge in H2S production within blood vessels, aligning with a significant decrease in blood pressure (Liu et al., 2017). The hydrogen sulfide generated, whether in its gaseous state or dissociated forms (HS–, S2–), can induce damage to the intestinal mucosa and exert cytotoxic effects on intestinal epithelial cells (Bełtowski, 2004).
Bacteria inhabiting the appropriate ecological niches do not necessarily penetrate deeply into the host's tissues as a living cell to initiate acute inflammation. Only bacterial post-culture metabolites such as LPSs, peptidoglycan, exopolysaccharide, enzymes, metalloproteases, or gases are sufficient for the occurrence of acute or chronic inflammatory processes.
Understanding the primary causes and mechanism of the pathogenesis and progression of AP is essential to facilitate early diagnosis and treatment and avoid a course leading to severe disease onset (Patel et al., 2021).
Our study indicates the possibility of active participation of bile in qualitative and quantitative changes in the composition of the microbiota in the peripancreatic space and the connection between released bile and the bacterial production of gaseous metabolites.
This study investigated the gastrointestinal microbiota of a single patient who developed acute pancreatitis following a prior episode of diverticulitis, a condition described by surgeons as exceptionally rare. Despite being a single clinical case, we were able to collect a substantial number of parallel samples, enabling a comparative analysis of classical culture methodologies and next-generation sequencing (NGS). Notably, we successfully cultured bacteria potentially implicated in the pathomechanism of acute pancreatitis, including Fusobacterium nucleatum and Escherichia coli, the latter producing gaseous metabolites in response to bile exposure. The role of these gaseous metabolites, particularly hydrogen, in either exacerbating or alleviating acute pancreatitis warrants further investigation through in vitro and in vivo studies involving larger patient cohorts across different stages of the disease.
The datasets presented in this study can be found in online repositories. The names of the repository/repositories and accession number(s) can be found here: https://www.ncbi.nlm.nih.gov/, PRJNA1114082.
The studies involving humans were approved by the study was conducted in accordance with the Declaration of Helsinki and approved by the Bioethics Committee of the Jagiellonian University (opinion number KBET 1072.6120.279). Written informed consent for all participants was obtained. The studies were conducted in accordance with the local legislation and institutional requirements. The participants provided their written informed consent to participate in this study. Written informed consent was obtained from the individual(s) for the publication of any potentially identifiable images or data included in this article.
AC: Data curation, Investigation, Methodology, Visualization, Writing – original draft, Writing – review & editing. EG: Data curation, Investigation, Methodology, Visualization, Writing – original draft, Writing – review & editing. AT-P: Investigation, Methodology, Visualization, Writing – original draft. NŻ-L: Methodology, Writing – original draft. MK: Methodology, Writing – original draft. AS: Investigation, Writing – original draft. KJ: Formal analysis, Software, Writing – original draft. KM: Methodology, Writing – original draft. KS: Methodology, Writing – original draft. AK: Validation, Writing – original draft. AP: Methodology, Writing – original draft. MS: Conceptualization, Funding acquisition, Project administration, Supervision, Writing – original draft.
The author(s) declare financial support was received for the research, authorship, and/or publication of this article. This research was funded by The National Science Center Poland, under Grant (No:2018/31/B/NZ6/02472) and by Jagiellonian University Medical College under Grant (no: N41/DBS/000699, N41/DBS/001023, and N41/DBS/00459).
The authors declare that the research was conducted in the absence of any commercial or financial relationships that could be construed as a potential conflict of interest.
The author(s) declare that no Gen AI was used in the creation of this manuscript.
All claims expressed in this article are solely those of the authors and do not necessarily represent those of their affiliated organizations, or those of the publisher, the editors and the reviewers. Any product that may be evaluated in this article, or claim that may be made by its manufacturer, is not guaranteed or endorsed by the publisher.
The Supplementary Material for this article can be found online at: https://www.frontiersin.org/articles/10.3389/fmicb.2025.1544124/full#supplementary-material
Ammori, B. J. (2003). Role of the gut in the course of severe acute pancreatitis. Pancreas 26, 122–129. doi: 10.1097/00006676-200303000-00006
Banks, P. A., Bollen, T. L., Dervenis, C., Gooszen, H. G., Johnson, C. D., Sarr, M. G., et al. (2012). Classification of acute pancreatitis-2012: revision of the Atlanta classification and definitions by international consensus. Gut 62, 102–111. doi: 10.1136/gutjnl-2012-302779
Bassis, C. M., Moore, N. M., Lolans, K., Seekatz, A. M., Weinstein, R. A., Young, V. B., et al. (2017). Comparison of stool versus rectal swab samples and storage conditions on bacterial community profiles. BMC Microbiol. 17:78. doi: 10.1186/s12866-017-0983-9
Bauer, T. M., Schwacha, H., Steinbrückner, B., Brinkmann, F. E., Ditzen, A. K., Aponte, J. J., et al. (2002). Small intestinal bacterial overgrowth in human cirrhosis is associated with systemic endotoxemia. Am. J. Gastroenterol. 97, 2364–2370. doi: 10.1111/j.1572-0241.2002.05791.x
Bawa, A., Kainat, A., Raza, H., George, T. B., Omer, H, and Pillai, A. C. (2022). Fusobacterium Bacteremia causing hepatic abscess in a patient with diverticulitis. Cureus 14:e26938. doi: 10.7759/cureus.26938
Bełtowski, J. (2004). Siarkowodór jako biologicznie aktywny mediator w układzie krazenia [Hydrogen sulfide as a biologically active mediator in the cardiovascular system]. Postepy Hig. Med. Dosw. 58, 285–291. Polish.
Boumitri, C., Brown, E., and Kahaleh, M. (2017). Necrotizing pancreatitis: current management and therapies. Clin. Endosc. 50, 357–365. doi: 10.5946/ce.2016.152
Boxhoorn, L., Voermans, R. P., Bouwense, S. A., Bruno, M. J., Verdonk, R. C., Boermeester, M. A., et al. (2020). Acute pancreatitis. Lancet 396, 726–734. doi: 10.1016/S0140-6736(20)31310-6
Brandal, L. T., Sekse, C., Lindstedt, B. A., Sunde, M., Løbersli, I., Urdahl, A. M., et al. (2012). Norwegian sheep are an important reservoir for human-pathogenic Escherichia coli O26:H11. Appl. Environ. Microbiol. 78, 4083–4091. doi: 10.1128/AEM.00186-12
Brook, I., and Frazier, E. H. (2000). Aerobic and anaerobic microbiology in intra-abdominal infections associated with diverticulitis. J. Med. Microbiol. 49, 827–830. doi: 10.1099/0022-1317-49-9-827
Capurso, G., Zerboni, G., Signoretti, M., Valente, R., Stigliano, S., Piciucchi, M., et al. (2012). Role of the gut barrier in acute pancreatitis. J. Clin. Gastroenterol. 46(Suppl), S46–S51. doi: 10.1097/MCG.0b013e3182652096
Chen, Y., Yang, F., Lu, H., Wang, B., Chen, Y., Lei, D., et al. (2011). Characterization of fecal microbial communities in patients with liver cirrhosis. Hepatology 54, 562–572. doi: 10.1002/hep.24423
De Luca, F., and Shoenfeld, Y. (2019). The microbiome in autoimmune diseases. Clin. Exp. Immunol. 195, 74–85. doi: 10.1111/cei.13158
Del Castillo, E., Meier, R., Chung, M., Koestler, D. C., Chen, T., Paster, B. J., et al. (2019). The microbiomes of pancreatic and duodenum tissue overlap and are highly subject specific but differ between pancreatic cancer and noncancer subjects. Cancer Epidemiol. Biomarkers Prev. 28, 370–383. doi: 10.1158/1055-9965.EPI-18-0542
Ejrnaes, K., Sandvang, D., Lundgren, B., Ferry, S., Holm, S., Monsen, T., et al. (2006). Pulsed-field gel electrophoresis typing of Escherichia coli strains from samples collected before and after pivmecillinam or placebo treatment of uncomplicated community-acquired urinary tract infection in women. J. Clin. Microbiol. 44, 1776–1181. doi: 10.1128/JCM.44.5.1776-1781.2006
Guo, S., Li, L., Xu, B., Li, M., Zeng, Q., Xiao, H., et al. (2018). A simple and novel fecal biomarker for colorectal cancer: ratio of fusobacterium nucleatum to probiotics populations, based on their antagonistic effect. Clin. Chem. 64, 1327–1337. doi: 10.1373/clinchem.2018.289728
Gur, C., Ibrahim, Y., Isaacson, B., Yamin, R., Abed, J., Gamliel, M., et al. (2015). Binding of the Fap2 protein of Fusobacterium nucleatum to human inhibitory receptor TIGIT protects tumors from immune cell attack. Immunity 42, 344–355. doi: 10.1016/j.immuni.2015.01.010
He, Y., Zhang, Q., Yu, X., Zhang, S., and Guo, W. (2023). Overview of microbial profiles in human hepatocellular carcinoma and adjacent nontumor tissues. J. Transl. Med. 21:68. doi: 10.1186/s12967-023-03938-6
Hu, X., Gong, L., Zhou, R., Han, Z., Ji, L., Zhang, Y., et al. (2021). Variations in gut microbiome are associated with prognosis of hypertriglyceridemia-associated acute pancreatitis. Biomelecules 11:695. doi: 10.3390/biom11050695
Jessen, B., Möhlig, M., Siegmund, B., and Treese, C. (2021). Recurrent pancreatitis in a 43-year-old patient with high-output stoma. Z Gastroenterol. 59, 865–868. doi: 10.1055/a-1524-3178
Johnson, J. R., and Stell, A. L. (2000). Extended virulence genotypes of Escherichia coli strains from patients with urosepsis in relation to phylogeny and host compromise. J. Infect. Dis. 181, 261–272. doi: 10.1086/315217
Kaczmarek, A., Budzyńska, A., and Gospodarek, E. (2012). Prevalence of genes encoding virulence factors among Escherichia coli with K1 antigen and non-K1 E. coli strains. J. Med. Microbiol. 61(Pt 10), 1360–1365. doi: 10.1099/jmm.0.044263-0
Kaplan, C. W., Ma, X., Paranjpe, A., Jewett, A., Lux, R., Kinder-Haake, S., et al. (2010). Fusobacterium nucleatum outer membrane proteins Fap2 and RadD induce cell death in human lymphocytes. Infect. Immun. 78, 4773–4778. doi: 10.1128/IAI.00567-10
Kartal, E., Schmidt, T. S. B., Molina-Montes, E., Rodríguez-Perales, S., Wirbel, J., Maistrenko, O. M., et al. (2022). A faecal microbiota signature with high specificity for pancreatic cancer. Gut 71, 1359–1372. doi: 10.1136/gutjnl-2021-324755
Kashani, N., Bezmin Abadi, A. T., Rahimi, F., and Forootan, M. (2020). FadA-positive Fusobacterium nucleatum is prevalent in biopsy specimens of Iranian patients with colorectal cancer. New Microbes New Infect. 34, 100651. doi: 10.1016/j.nmni.2020.100651
Kinder Haake, S., and Lindemann, R. A. (1997). Fusobacterium nucleatum T18 aggregates human mononuclear cells and inhibits their PHA-stimulated proliferation. J. Periodontol. 68, 39–44. doi: 10.1902/jop.1997.68.1.39
Kiruthiga, A., Padmavathy, K., Shabana, P., Naveenkumar, V., Gnanadesikan, S., Malaiyan, J., et al. (2020). Improved detection of esp, hyl, asa1, gelE, cylA virulence genes among clinical isolates of Enterococci. BMC Res. Notes 13:170. doi: 10.1186/s13104-020-05018-0
Kuan, C. S., Wong, M. T., Choi, S. B., Chang, C. C., Yee, Y. H., Wahab, H. A., et al. (2011). Klebsiella pneumoniae yggG gene product: a zinc-dependent metalloprotease. Int. J. Mol. Sci. 12, 4441–4455. doi: 10.3390/ijms12074441
Li, X. Y., He, C., Zhu, Y., and Lu, N. H. (2020). Role of gut microbiota on intestinal barrier function in acute pancreatitis. World J. Gastroenterol. 26, 2187–2193. doi: 10.3748/wjg.v26.i18.2187
Liu, K., Yang, X., Zeng, M., Yuan, Y., Sun, J., He, P., et al. (2021). The role of fecal Fusobacterium nucleatum and pks+ Escherichia coli as early diagnostic markers of colorectal cancer. Dis. Markers 2021:1171239. doi: 10.1155/2021/1171239
Liu, Y., Liao, R., Qiang, Z., and Zhang, C. (2017). Pro-inflammatory cytokine-driven PI3K/Akt/Sp1 signalling and H2S production facilitates the pathogenesis of severe acute pancreatitis. Biosci. Rep. 37:BSR20160483. doi: 10.1042/BSR20160483
Manichanh, C., Rigottier-Gois, L., Bonnaud, E., Gloux, K., Pelletier, E., Frangeul, L., et al. (2006). Reduced diversity of faecal microbiota in Crohn's disease revealed by a metagenomic approach. Gut 55, 205–211. doi: 10.1136/gut.2005.073817
Mee, L. K., Navon, G., and Stein, G. (1965). The action of atomic hydrogen on trypsin in aqueous solution. Biochim. Biophys. Acta 104, 151–159. doi: 10.1016/0304-4165(65)90230-8
Mitsuhashi, K., Nosho, K., Sukawa, Y., Matsunaga, Y., Ito, M., Kurihara, H., et al. (2015). Association of Fusobacterium species in pancreatic cancer tissues with molecular features and prognosis. Oncotarget 6, 7209–7220. doi: 10.18632/oncotarget.3109
Nagata, N., Nishijima, S., Kojima, Y., Hisada, Y., Imbe, K., Miyoshi-Akiyama, T., et al. (2022). Metagenomic identification of microbial signatures predicting pancreatic cancer from a multinational study. Gastroenterology 163, 222–238. doi: 10.1053/j.gastro.2022.03.054
Patel, B. K., Patel, K. H., Bhatia, M., Iyer, S. G., Madhavan, K., Moochhala, S. M., et al. (2021). Gut microbiome in acute pancreatitis: a review based on current literature. World J. Gastroenterol. 27, 5019–5036. doi: 10.3748/wjg.v27.i30.5019
Pendharkar, S. A., Mathew, J., and Petrov, M. S. (2017). Age- and sex-specific prevalence of diabetes associated with diseases of the exocrine pancreas: a population-based study. Dig. Liver Dis 49, 540–544. doi: 10.1016/j.dld.2016.12.010
Petrov, M. S., Shanbhag, S., Chakraborty, M., Phillips, A. R., and Windsor, J. A. (2010). organ failure and infection of pancreatic necrosis as determinants of mortality in patients with acute pancreatitis. Gastroenterology 139, 813–820. doi: 10.1053/j.gastro.2010.06.010
Petrov, M. S., and Yadav, D. (2019). Global epidemiology and holistic prevention of pancreatitis. Nat. Rev. Gastroenterol. Hepatol. 16, 175–184. doi: 10.1038/s41575-018-0087-5
Qin, J., Li, R., Raes, J., Arumugam, M., Burgdorf, K. S., Manichanh, C., et al. (2010). A human gut microbial gene catalogue established by metagenomic sequencing. Nature 464, 59–65. doi: 10.1038/nature08821
Ren, Z., Li, A., Jiang, J., Zhou, L., Yu, Z., Lu, H., et al. (2019). Gut microbiome analysis as a tool towards targeted non-invasive biomarkers for early hepatocellular carcinoma. Gut 68, 1014–1023. doi: 10.1136/gutjnl-2017-315084
Saeedi, B., Hällgren, A., Jonasson, J., Nilsson, L. E., Hanberger, H., Isaksson, B., et al. (2002). Modified pulsed-field gel electrophoresis protocol for typing of enterococci. APMIS 110, 869–874. doi: 10.1034/j.1600-0463.2002.1101205.x
Sarowska, J., Futoma-Koloch, B., Jama-Kmiecik, A., Frej-Madrzak, M., Ksiazczyk, M., Bugla-Ploskonska, G., et al. (2019). Virulence factors, prevalence and potential transmission of extraintestinal pathogenic Escherichia coli isolated from different sources: recent reports. Gut Pathog. 11:10. doi: 10.1186/s13099-019-0290-0
Serra, N., Di Carlo, P., Gulotta, G., d' Arpa, F., Giammanco, A., Colomba, C., et al. (2018). Bactibilia in women affected with diseases of the biliary tract and pancreas. A STROBE guidelines-adherent cross-sectional study in Southern Italy. J. Med. Microbiol. 67, 1090–1095. doi: 10.1099/jmm.0.000787
Sexton, R. E., Uddin, M. H., Bannoura, S., Khan, H. Y., Mzannar, Y., Li, Y., et al. (2022). Connecting the human microbiome and pancreatic cancer. Cancer Metastasis Rev. 41, 317–331. Erratum in: Cancer Metastasis Rev. 2022 May 10. doi: 10.1007/s10555-022-10022-w
Sun, Z., Xiong, C., Teh, S. W., Lim, J. C. W., Kumar, S., Thilakavathy, K., et al. (2019). Mechanisms of oral bacterial virulence factors in pancreatic cancer. Front. Cell. Infect. Microbiol. 9:412. doi: 10.3389/fcimb.2019.00412
Tan, C., Ling, Z., Huang, Y., Cao, Y., Liu, Q., Cai, T., et al. (2015). Dysbiosis of intestinal microbiota associated with inflammation involved in the progression of acute pancreatitis. Pancreas 44, 868–875. doi: 10.1097/MPA.0000000000000355
Vankerckhoven, V., Van Autgaerden, T., Vael, C., Lammens, C., Chapelle, S., Rossi, R., et al. (2004). Development of a multiplex PCR for the detection of asa1, gelE, cylA, esp, and hyl genes in enterococci and survey for virulence determinants among European hospital isolates of Enterococcus faecium. J. Clin. Microbiol. 42, 4473–4479. doi: 10.1128/JCM.42.10.4473-4479.2004
Wang, G., Wen, J., Xu, L., Zhou, S., Gong, M., Wen, P., et al. (2013). Effect of enteral nutrition and ecoimmunonutrition on bacterial translocation and cytokine production in patients with severe acute pancreatitis. J. Surg. Res. 183, 592–597. doi: 10.1016/j.jss.2012.12.010
Wang, G.-J., Gao, C.-F., Wei, D., Wang, C., and Ding, S.-Q. (2009). Acute pancreatitis: etiology and common pathogenesis. World J. Gastroenterol. 15, 1427–1430. doi: 10.3748/wjg.15.1427
Wimmer, Z., and Zarevúcka, M. (2010). A review on the effects of supercritical carbon dioxide on enzyme activity. Int. J. Mol. Sci. 11, 233–253. doi: 10.3390/ijms11010233
Yamamoto, S., Terai, A., Yuri, K., Kurazono, H., Takeda, Y., Yoshida, O., et al. (1995). Detection of urovirulence factors in Escherichia coli by multiplex polymerase chain reaction. FEMS Immunol. Med. Microbiol. 12, 85–90. doi: 10.1111/j.1574-695X.1995.tb00179.x
Zhou, C. H., Meng, Y. T., Xu, J. J., Fang, X., Zhao, J. L., Zhou, W., et al. (2020). Altered diversity and composition of gut microbiota in Chinese patients with chronic pancreatitis. Pancreatology 20, 16–24. doi: 10.1016/j.pan.2019.11.013
Zhou, W., Zhang, D., Li, Z., Jiang, H., Li, J., Ren, R., et al. (2021). The fecal microbiota of patients with pancreatic ductal adenocarcinoma and autoimmune pancreatitis characterized by metagenomic sequencing. J. Transl. Med. 19:215. doi: 10.1186/s12967-021-02882-7
Keywords: acute pancreatitis, microbiome, microbiota, bile, NGS, gaseous metabolites, Fusobacterium nucleatum, Escherichia coli
Citation: Chmielarczyk A, Golińska E, Tomusiak-Plebanek A, Żeber-Lubecka N, Kulecka M, Szczepanik A, Jedlińska K, Mech K, Szaciłowski K, Kuziak A, Pietrzyk A and Strus M (2025) Microbial dynamics of acute pancreatitis: integrating culture, sequencing, and bile impact on bacterial populations and gaseous metabolites. Front. Microbiol. 16:1544124. doi: 10.3389/fmicb.2025.1544124
Received: 12 December 2024; Accepted: 10 January 2025;
Published: 12 February 2025.
Edited by:
George Grant, Independent Researcher, Aberdeen, United KingdomReviewed by:
Sudhir Kumar, Iowa State University, United StatesCopyright © 2025 Chmielarczyk, Golińska, Tomusiak-Plebanek, Żeber-Lubecka, Kulecka, Szczepanik, Jedlińska, Mech, Szaciłowski, Kuziak, Pietrzyk and Strus. This is an open-access article distributed under the terms of the Creative Commons Attribution License (CC BY). The use, distribution or reproduction in other forums is permitted, provided the original author(s) and the copyright owner(s) are credited and that the original publication in this journal is cited, in accordance with accepted academic practice. No use, distribution or reproduction is permitted which does not comply with these terms.
*Correspondence: Magdalena Strus, bWFnZGFsZW5hLnN0cnVzQHVqLmVkdS5wbA==
†These authors have contributed equally to this work
Disclaimer: All claims expressed in this article are solely those of the authors and do not necessarily represent those of their affiliated organizations, or those of the publisher, the editors and the reviewers. Any product that may be evaluated in this article or claim that may be made by its manufacturer is not guaranteed or endorsed by the publisher.
Research integrity at Frontiers
Learn more about the work of our research integrity team to safeguard the quality of each article we publish.