- 1School of Biotechnology and Food Engineering, Anyang Institute of Technology, Anyang, China
- 2College of Animal Science and Technology, Tarim University, AIar, China
Introduction: Fowl adenovirus serotype 4 (FAdV-4) is a highly contagious viral pathogen of global significance that affects various avian species. It primarily infects poultry and wild birds, leading to avian inclusion body hepatitis (IBH) and hepatitis-hydropericardium syndrome (HHS). The development of rapid diagnostic tools for detecting FAdV-4 is crucial for effective disease control and eradication efforts.
Methods: In this study, we developed a recombinase polymerase amplification (RPA) combined with CRISPR/Cas12a assay, specifically targeting the FAdV-4 Hexon gene. RPA and CRISPR/Cas12a reagents were added to the bottom and lid of the test tube at once, allowing the detection process to occur within a single reaction tube. This approach reduced contamination.
Results: The RPA-CRISPR/Cas12a detection method can identify as few as 10 copies of the genome per reaction, demonstrating 100% sensitivity comparable to that of fluorescence PCR (qPCR). This approach exhibits high specificity for FAdV-4, with no cross-reactivity observed with other FAdV serotypes or common avian pathogens. Additionally, the agreement rate between the results of RPA-CRISPR/Cas12a and qPCR for detecting clinical samples is as high as 97.5%.
Discussion: Therefore, the RPA-CRISPR/Cas12a assay presents a promising alternative for the simple, sensitive, and specific identification of FAdV-4.
Introduction
FAdV, or fowl adenovirus, belongs to the Adenoviridae family and the avian adenovirus genus (Schachner et al., 2018; Wang and Zhao, 2019). Through cross-neutralization and restriction enzyme assays, avian adenoviruses have been classified into five species: A, B, C, D, and E, comprising a total of 12 serotypes (Hess, 2000). Notably, FAdV-1-induced adenoviral gizzard erosion (AGE) is marked by macroscopic lesions in the affected gizzards, including inflammation and ulceration; FAdV-4 is associated with pericardial effusion hepatitis syndrome (HHS); and FAdV-8 causes inclusion body hepatitis (IBH) in chickens (Mo, 2021).
The disease primarily spreads horizontally through the fecal-oral route, although some studies suggest that vertical transmission may also occur. In China, HHS cases were sporadic before 2015; however, since July 2015, the disease has surged dramatically, with reports emerging from multiple provinces, including Chongqing, Hebei, Guangdong, Shandong, Jiangsu, Hubei, Liaoning, Sichuan, and Zhejiang (Wang et al., 2019; Jiang et al., 2019; Yuming et al., 2020; Li et al., 2017; Chen et al., 2019; Yu et al., 2019). In China, FAdV-4 has emerged as the serotype with the highest infection rate among adenoviruses.
Typical features of HHS include the accumulation of transparent or pale yellow fluid in the pericardium, liver swelling with fragility and discoloration, as well as focal necrosis and petechial hemorrhage (Asthana et al., 2013). Histopathological examination reveals characteristic changes in the liver, including small multifocal necrosis and alkaline inclusions in liver cells. Additionally, renal tubular epithelial cell necrosis, infiltration of mononuclear cells in the alveolar wall, and necrosis with inflammatory cell infiltration in the spleen are observed (Asthana et al., 2013; Sun et al., 2019).
Chickens between the ages of 3 to 6 weeks are especially vulnerable to FAdV-4 infection, with mortality rates ranging from 30% to 80% (Kumar et al., 1997). Besides, FAdV-4 infection can lead to a significant reduction in weight gain, as well as negative impacts on various production parameters, resulting in substantial economic losses (Grafl et al., 2012).
The molecular biology detection methods for adenoviruses include polymerase chain reaction (PCR), real-time quantitative PCR, restriction fragment length polymorphism polymerase chain reaction (PCR-RFLP), and loop-mediated isothermal amplification (LAMP) (Wang et al., 2017a; Zhai et al., 2019). While the sensitivity and specificity of molecular biology detection methods have significantly improved, traditional detection methods do have some drawbacks. They often require expensive equipment and skilled operators, and samples may need special processing, increasing operational complexity and time costs.
CRISPR/Cas is a bacterial adaptive immune system that has gained significant attraction in recent years due to its rapid detection capabilities (Wang and Doudna, 2023). The CRISPR-associated protein (Cas) functions by cleaving foreign nucleic acids, guided by programmable CRISPR RNA (CrRNA) that boasts high nuclease activity. Key Cas subtypes include Cas9, Cas12, Cas13, and Cas14. CRISPR/Cas12a (also known as CRISPR/Cpf1) belongs to the second family of Cas enzymes. It recognizes specific protospacer-adjacent motifs (PAMs) that are rich in thymine (T) nucleotides and catalyzes the maturation of its CrRNA. Cas12a specifically targets and cleaves complementary double-stranded DNA while demonstrating robust non-specific trans-cleavage activity toward single-stranded DNA (ssDNA), which generates fluorescent signals. The target double-stranded DNA can be obtained through recombinase polymerase amplification (RPA). RPA is an isothermal amplification method that is quick, cost-effective, and does not require specialized equipment (Kellner et al., 2019). As a result, RPA-CRISPR/Cas12a has become widely utilized for virus, bacteria and parasite detection (Qian et al., 2021; Lin et al., 2023; Xiong et al., 2022; Liu et al., 2021; Zhang et al., 2021).
The purpose of this study is to develop a novel diagnostic method that combines RPA and CRISPR/Cas12a technology, providing a fast and accurate approach for early diagnosis of FAdV-4.
Materials and methods
Viruses
Avian influenza virus (AIV), Newcastle disease virus (NDV), Infectious bursal disease virus (IBDV), Marek's disease virus (MDV) and Infectious laryngotracheitis virus (ILTV) were preserved in our laboratories. The details of these viruses have been detailed in one of our previous studies (Talwar et al., 2021). FAdV-1, FAdV-2, FAdV-3, FAdV-4, FAdV-5, FAdV-6, FAdV-7, FAdV-8a, FAdV-8b, FAdV-9, FAdV-10, and FAdV-11 were purchased from China Institute of Veterinary Drug Control (Beijing, China).
Preparation of DNA molecular standards
To facilitate primer design, we obtained the Hexon gene sequences from various FAdV-4 strains through the NCBI database: isolate YN2109 (OQ605505.1), PDS (KU647685.1), YN1605 (OQ605508.1), WS (KU647686.1), CG-D (KU647683.1), XZ (KU647687.1), ZK(KU647689.1), YZ (KU647688.1). Using DNAMAN v5.0 software, these sequences were compared, and the conserved region of the Hexon gene was cloned by the following method.
Nucleic acids from the FAdV-4 strain were obtained utilizing a DNA/RNA extraction kit from Tiangen (Beijing, China). To amplify the conserved Hexon gene via PCR, we designed a specific pair of primers: the forward primer (5′-GCCCACCCGAAATGTCACGA-3′) and the reverse primer (5′-CCGGTCGGGCAGCTCGACCA-3′). The forward primer and reverse primer are located at positions 243-262bp and 1028-1047bp of the Hexon gene, respectively, with a target gene length of 805bp. The PCR was conducted following the protocols outlined in the PCR Kit Manual (Takara, Beijing, China). The PCR procedure involved an initial denaturation at 95°C for 3 min, followed by denaturation at 95°C for 30 s, annealing at 55°C for 1 min, and extension at 72°C for 30 s, with a total of 30 cycles run; finally, a final extension step was conducted at 72°C for 5 min.
Following the PCR, the products were subjected to agarose gel electrophoresis for analysis and subsequently purified. The resulting purified gene fragment was then cloned into the pMD18-T vector according to the product manual (Takara, Beijing, China). The pMD-18T vector is a commonly used linear cloning vector characterized by the presence of multiple T (adenine) termini at both ends. During PCR amplification of DNA fragments, Taq DNA polymerase generates a poly-A tail at the 3′ end of the PCR product. In the ligation reaction, T4 DNA ligase is employed to join the poly-A terminated DNA fragment to the T termini of the pMD-18T vector. Once the ligation is complete, the resulting recombinant vector was transformed into competent cells DH5α. The authenticity of the recombinant plasmid was verified through nucleotide sequencing. The plasmid successfully inserted with the Hexon gene was named pMD-Hexon. Plasmid extraction was carried out using a reagent kit, and the concentration was determined using a Nano2000 (Thermo Scientific, MA, USA). The recombinant plasmids were utilized as DNA molecular standards.
RPA primer design
The design principles for RPA primers dictate a minimum length of 30 nucleotides, with typical primers ranging from 28 to 32 nucleotides. It is crucial to avoid excessive palindromic structures; the optimal range of G and C content is between 40% and 60%. Additionally, multiple G residues at the 5′ end of the primer should be minimized, while the 3′ end should ideally contain a higher proportion of G and C nucleotides. The target sequence for amplification typically ranges from 100 to 200 bp.
Based on the aforementioned guidelines, the amplification efficiency of the various primer pairs was assessed via agarose gel electrophoresis, allowing us to select the primer pair with optimal amplification efficiency for use in the CRISPR system. All primers and reporter molecules utilized in this study were synthesized by Sangon Biotech (Shanghai) Co., Ltd (Shanghai, China).
CrRNA design
CrRNA plays a crucial role in guiding the Cas12a protein recognition of the protospacer adjacent motif (PAM) which is characterized as TTTN (where N denotes any nucleotide). To design CrRNA, a specific sequence is selected followed by the PAM sequence. The CrRNA sequence includes a Hexon gene sequence (about 20 bp) and a scaffold sequence. In addition, a T7 promoter sequence is also added to the 5′ end of the CrRNA sequence. Three CrRNAs were designed. The sequences were subjected to BLAST analysis on the NCBI platform. The results indicated that the sequences exclusively aligned with the gene of FAdV-4, revealing no overlapping sequences in the genomes of other species or among other viruses.
The CrRNA sequence was synthesized and inserted into the pGEX-T vector, which was provided by Sangon (Shanghai, China). The plasmid containing the CrRNA sequence was in vitro transcribed by CleascripTM T7 RNA Polymerase according to the Kit manual (Yeasen Biotechnology, Shanghai, China). The obtained RNA was used for the subsequent experiments.
CrRNA screen
To assess the activity of CrRNAs, we performed a CRISPR/Cas12a detection analysis. The assay formulation included 0.5 μL of Cas12a protein (New England Biolab, MA, USA), 1 μL of CrRNA (2 μM), 2 μL of 10X NEBuffer 2.0 (New England Biolab, USA), 0.5 μL of a single-stranded DNA (ssDNA) probe (5′-FAM-TTATT-BHQ1-3′) as a reporter, and 2 μL of Hexon gene obtained from PCR. The reaction tube was placed under the Deao instrument (Guangzhou, China) to detect the fluorescence signal at a temperature of 39°C for 20 min. Fluorescence amplitude was recorded and utilized to evaluate the cleavage efficiency of the CrRNAs. Distilled deionized water (ddH2O) served as the negative control.
One-tube RPA-CRISPR/Cas12a assay
The one-tube RPA-CRISPR/Cas12a assay integrates a RPA reaction with Cas12a detection. The RPA reaction was performed using the RPA Basic kit (TwistDx, Maidenhead, United Kingdom), following the manufacturer's instructions. Each 50 μL RPA reaction comprised a mix of 2 μL of each RPA primer (10 μM), 29.5 μL of buffer, 1 μL of template (DNA molecular standard pMD-Hexon prepared above), and 2 μL of MgOAc (280 mM), all added to the bottom of a tube containing enzyme pellets.
For the Cas12a detection, the reagents-1 μL of Cas12a (10 μM), 1 μL of CrRNA (10 μM), 0.25 μL RNA inhibitor, 1 μL of DNA FQ reporter, and 2 μL of 10 × NEBuffer were placed on the inner surface of the tube lid. Throughout the entire process, the reagents were kept on ice. The tubes were carefully covered and incubated in a metal bath at 39°C for 20 min. Subsequently, the tubes were briefly centrifuged and transferred to a Deao incubator (Guangzhou, China) at 39°C for an additional 20 min. Fluorescent signals were captured at 30-s intervals. Generally, a sample is considered positive if it produces a clear amplification curve and the fluorescence value exceeds the threshold of the negative control; otherwise, the sample is deemed negative.
The experimental procedure is described in Figure 1.
Optimization of reaction conditions
In the one-tube RPA-CRISPR/Cas12a detection method, the specific concentrations of Cas12a protein and CrRNA are critically important for achieving optimal detection results. Firstly, the one-tube RPA-CRISPR/Cas12a assay was conducted using various CrRNA concentrations (1.5 μM, 1 μM, 0.5 μM, and 0.25 μM). The optimal CrRNA concentration was identified by analyzing the fluorescence values. Then, the one-tube RPA-CRISPR/Cas12a assay was conducted using the optimal CrRNA concentration, and different Cas12a concentrations (1.5 μM, 1 μM, 0.5 μM, and 0.25 μM) were evaluated as above.
Sensitivity test
The sensitivity of the one-tube RPA-CRISPR/Cas12a method was evaluated under the optimized conditions established above. The real-time one-tube RPA-CRISPR/Cas12a tests were carried out using the recombinant plasmid pMD-Hexon, which was diluted from 106 to 1010 copies/μL to serve as the template. Distilled deionized water (ddH2O) served as the negative control. The experiment was conducted in triplicate. In addition, the one-tube RPA-CRISPR/Cas12a method was compared with both the qPCR detection method and the basic RPA detection method. The qPCR detection method has been previously described (Wang et al., 2017a), while the details of the basic RPA detection method are outlined above.
Repetitive testing
Three DNA molecular standards with varying concentrations (1 × 106, 1 × 104, and 1 × 102) were used as templates. For each plasmid concentration, three replicates were conducted for intra-group repeatability. Simultaneously, each concentration underwent three independent tests for inter-group repeatability. The standard deviation (SD) and coefficient of variation (CV) for each dilution sample were calculated within and between groups to evaluate the method's reproducibility.
Specificity test
To verify the specificity of the one-tube RPA-CRISPR/Cas12a method for the detection of FAdV-4, the assay was used to detect various avian infectious pathogens. These pathogens are as follows: AIV, NDV, ILTV, MDV, IBDV, FAdV-1, FAdV-2, FAdV-3, FAdV-5, FAdV-6, FAdV-7, FAdV-8a, FAdV-8b, FAdV-9, FAdV-10, FAdV-11. FAdV-4 was included as the positive control, and ddH2O was used as the negative control. The experiment was conducted in triplicate.
Evaluation of the effect of clinical sample testing
From 2020 to 2024, a total of 40 clinical samples were collected from chickens suspected of FAdV infection in a chicken breeder in Yangling, Shanxi province, China. FAdV-4 can lead to symptoms such as hepatitis and pericardial effusion, prompting this study to collect tissue samples from the heart and liver for detection. One heart sample and one liver sample were collected from each chicken, resulting in a total of 40 samples from 20 chickens. These samples were collected for laboratory diagnostics and not specifically for our research purposes. Sample collection operation was approved by the institutional animal care and use committee (IACUC) of Anyang Institute of Technology, Anyang, China. After the samples were collected, the internal organs were chopped using scissors, and 1 mL of buffer solution was added for every 1 g of tissue to prepare a homogenate. To enhance the release of virus particles, the samples underwent three freeze-thaw cycles in a refrigerated homogenization environment. The supernatant was then obtained through high-speed centrifugation, followed by DNA extraction using Qiagen AllPrep DNA/RNA Mini Kit (QIAGEN China, Shanghai, China) following the instructions in the product manual. Finally, the extracted DNA was analyzed by the RPA-CRISPR/Cas12a method, and the results were compared with those obtained using the qPCR method.
Data analysis
GraphPad Prism 8.0 was utilized for data analysis and processing. The Student's t-test was employed to assess significant differences between groups. A p < 0.05 indicated significant differences, while a p > 0.05 suggested no significant differences. Additionally, a p < 0.01 denoted highly significant differences.
Results
Sequence alignment
The sequences of the RPA primers and CrRNA were shown in Table 1. Because the CrRNA2 performed well in the following section, only the sequence of CrRNA2 was analyzed. The analysis of the homology of Hexon genes among different FAdV-4 strains was displayed in Figure 2A. There is only one base difference among the nine FAdV-4 strains. The analysis of the homology of Hexon genes among different FAdV serotypes (FAdV-1 to FAdV-11) was displayed in Figure 2B. As show in Figure 2B, the sequence differences among different FAdV serotypes are highly significant. The primers and CrRNA sequence of FAdV-10 shows the highest homology with FAdV-4, differing by five bases.
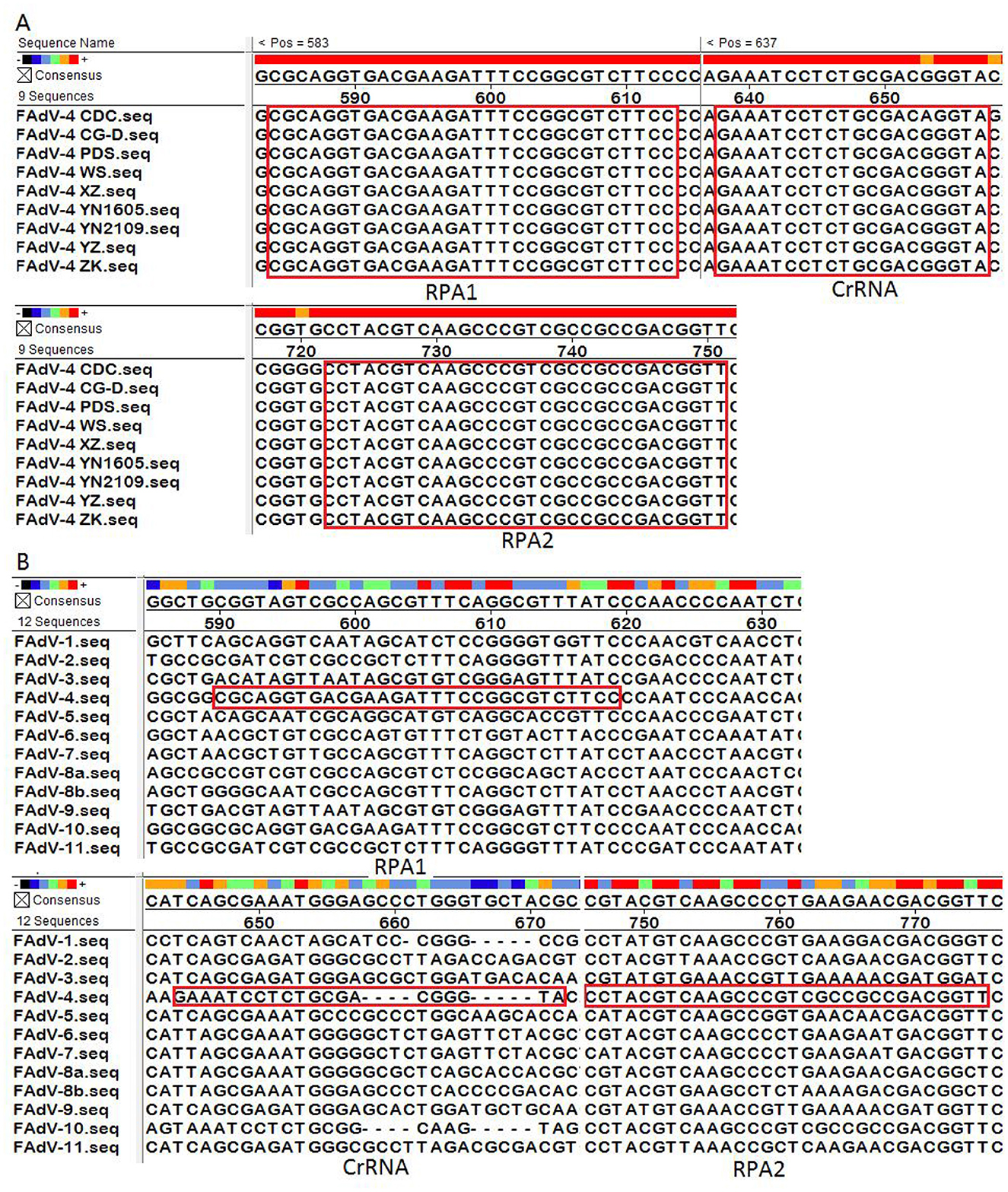
Figure 2. Sequence alignment. (A) The analysis of the homology of Hexon genes among different FAdV-4 strains; (B) The analysis of the homology of Hexon genes among different FAdV serotypes (FAdV-1 to FAdV-11). The RPA primers (RPA1 and RPA2) and CrRNA were marked in the red box.
CrRNA screen
The screening of CrRNA will utilize the constructed plasmid pMD-Hexon as a template for detection. The assay was conducted separately using the three CrRNAs (CrRNA1, CrRNA2 and CrRNA3). The fluorescence levels of the reaction products were assessed. As illustrated in Figure 3, results demonstrate that all three CrRNAs generate fluorescence curves. Notably, the fluorescence values for CrRNA2 and CrRNA3 surpassed that of CrRNA1. Meanwhile, the fluorescence values for CrRNA2 and CrRNA3 were the same as each other. Consequently, CrRNA2 was selected for subsequent experiments. The alignment of the CrRNA2 sequence was illustrated in Figure 2A.
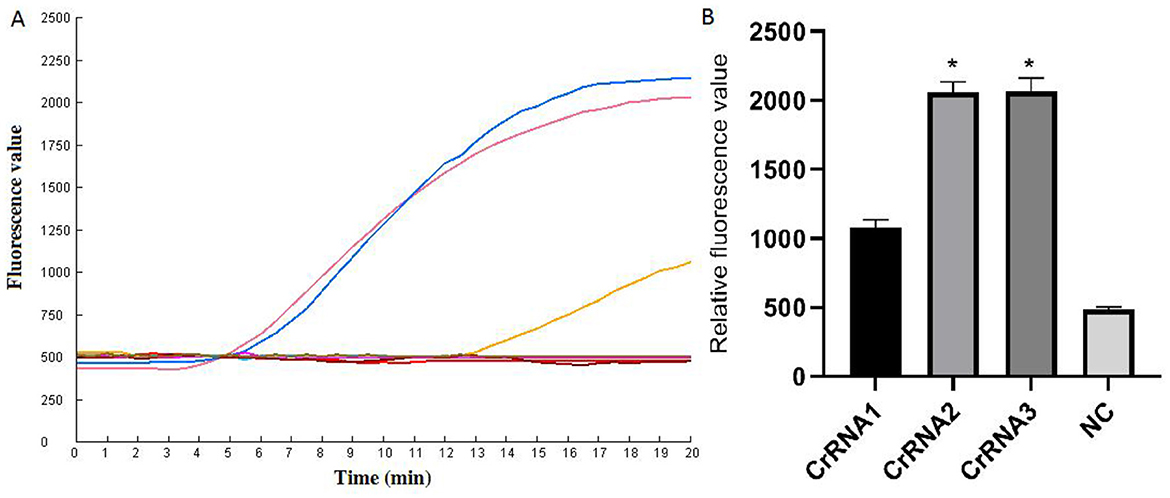
Figure 3. Screening of the optimal CrRNA. (A) Evaluation of the effect of the CrRNAs by the Cas12a detection assay. (B) The tests were conducted in triplicate. *Represents a p-value less than 0.05.
Reaction conditions
Various concentrations of CrRNA and Cas12a were assessed. The results demonstrated that fluorescence intensity increased with increasing concentrations of Cas12a (1 μM, 0.5 μM, 0.25 μM). However, no significant differences in peak fluorescence intensity were observed among the higher concentrations of Cas12a (2 μM, 1.5 μM, 1 μM). Therefore, a Cas12a concentration of 1 μM was selected for subsequent assays (Figures 4A, B).
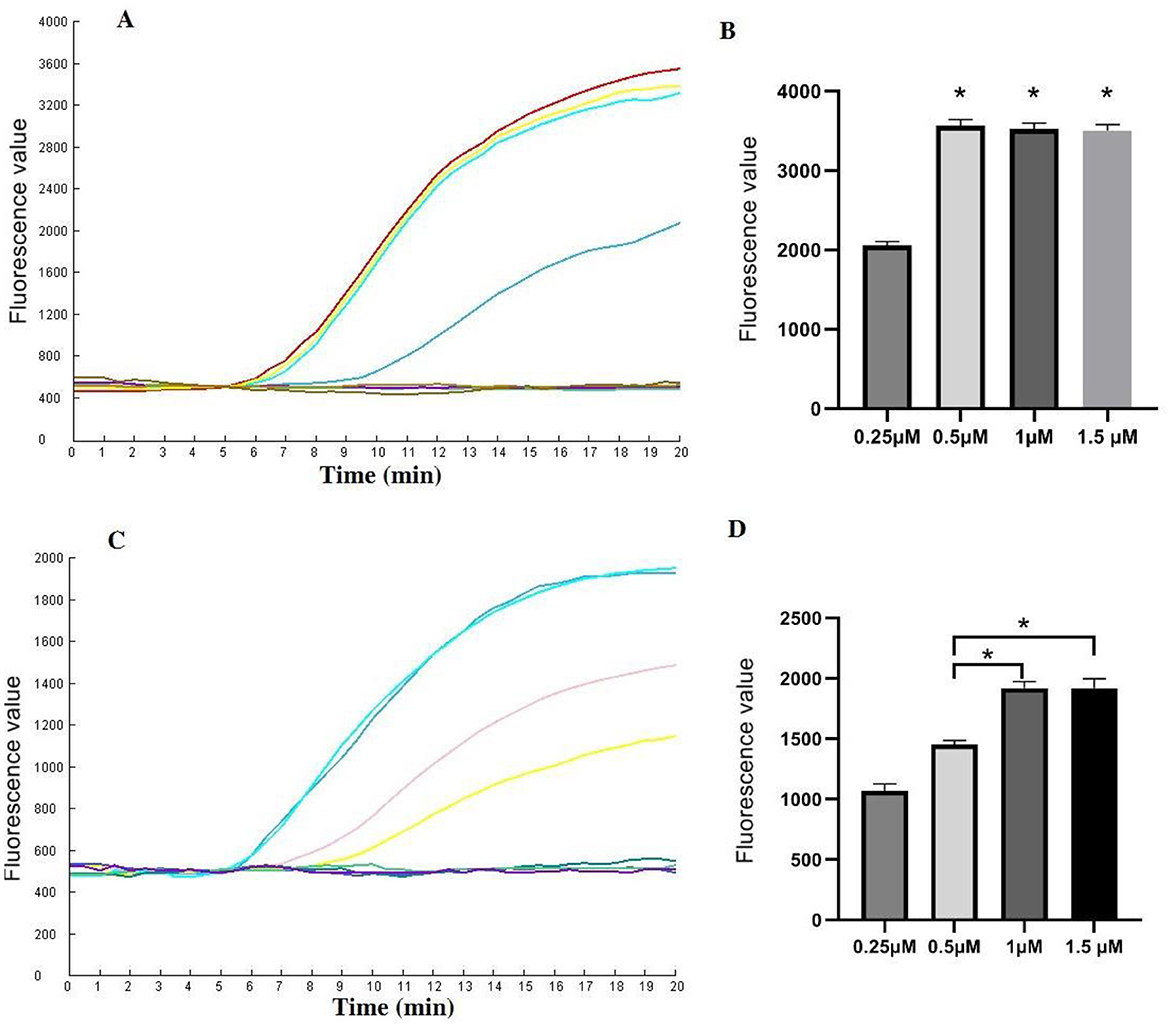
Figure 4. Evaluation of the CrRNA and Cas12a concentrations in the one-tube RPA-CRISPR/Cas12a test. (A, B) Different CrRNA concentrations were tested at 0.25 μM, 0.5 μM, 1 μM and 1.5 μM. The tests were conducted in triplicate; (C, D) Cas12a concentrations were evaluated at 0.25 μM, 0.5 μM, 1 μM and 1.5 μM. *Represents a p-value less than 0.05.
Next, the optimal concentration of CrRNA was investigated. As illustrated in Figures 4C, D, the use of higher concentrations of CrRNA (1 μM, 0.5 μM, 0.25 μM) accelerated the time required to achieve exponential amplification. When the CrRNA concentration exceeded 1 μM, there were no significant differences in the time to achieve exponential amplification. Consequently, a CrRNA concentration of 1 μM was chosen for the follow-up experiments.
Sensitivity
The recombinant plasmid pMD-Hexon was serially diluted and used as a template for sensitivity detection in the RPA-CRISPR/Cas12a method, while the established PCR method served as a comparison. As illustrated in Figure 5, the real-time RPA-CRISPR/Cas12a method can detect as low as 10 copies/μL. When utilizing template concentrations ranging from 10 to 106 copies, the method exhibits distinct exponential amplification curves. The fluorescence signal is detectable within a time frame of 5–15 min, indicating the method's rapid responsiveness in identifying target pathogen across this concentration range. The sensitivity of the detection method developed in this study is comparable to that of the qPCR method. However, when using template concentrations of 10 to 106 copies in qPCR, the time range for fluorescence amplification signals to appear is around 20–35 min. The data collected from three runs with the DNA standards were analyzed using semi-logarithmic regression in GraphPad Prism 5.0. The analysis demonstrated that this assay is reproducible (Figure 5C). However, the limit of detection (LoD) for the basic RPA was 103 copies/μL, which is 100 times lower than that of the one-tube RPA-CRISPR/Cas12a method (Figure 5D).
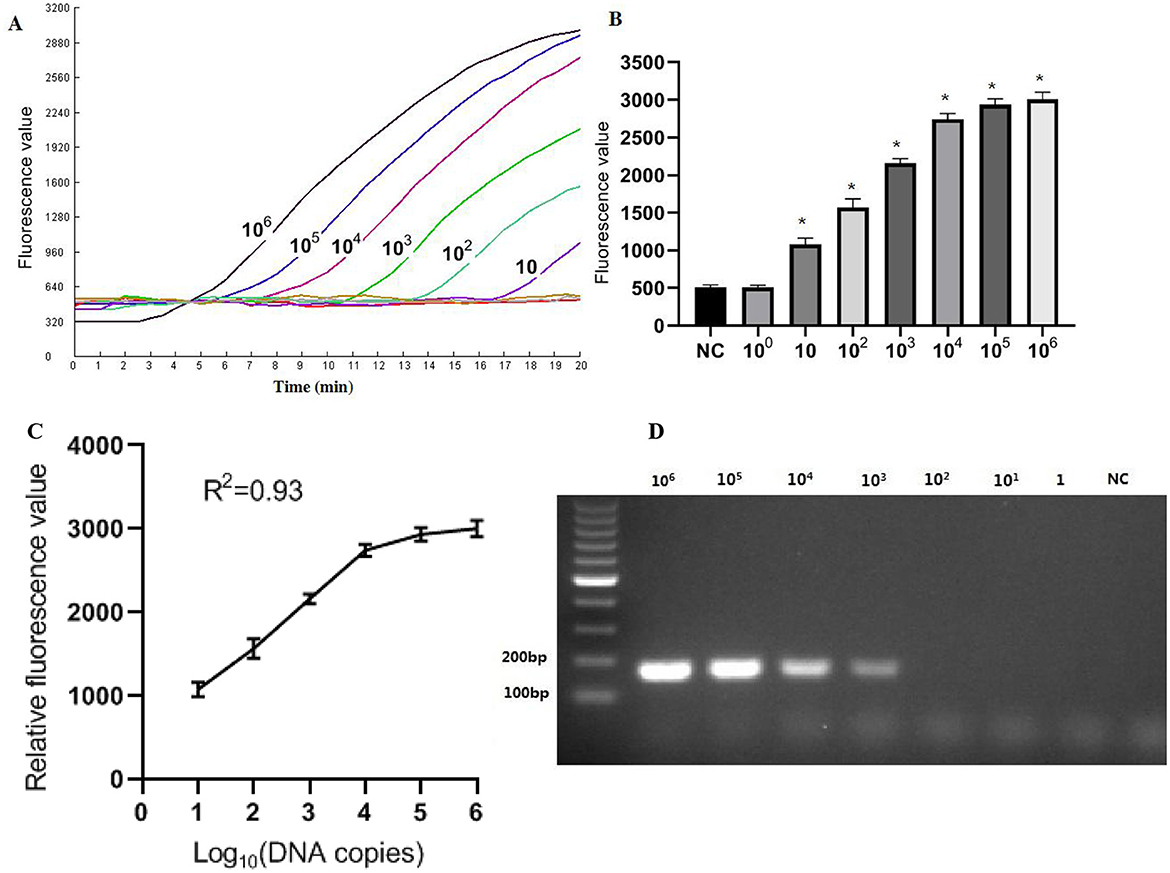
Figure 5. Sensitivity results based on fluorescence detection. (A) To determine the minimum detection of the RPA-CRISPR/Cas12a assay, ten-fold dilution standards were detected as templates by the method. (B) The tests were conducted in triplicate. (C) The LoD of the basic RPA assay. (D) Semi-logarithmic regression of the data collected from 3 runs using the DNA standards was analyzed by GraphPad Prism 5.0. *Represents a p-value less than 0.05.
Repetitive testing
The results indicated that the intra-group coefficients of variation for the different concentrations of molecular standards were 3.0%, 2.7%, and 7.5%, while the inter-group coefficients of variation were 1.9%, 4.0%, and 7.0% (Table 2). Both the intra-group and inter-group coefficients of variation (CV) were below 10%, demonstrating that the method established in this experiment exhibits good reproducibility.
Specificity test
As illustrated in Figure 6, only FAdV-4 tested positive by the one-tube RPA-CRISPR/Cas12a method. All the other viruses (AIV, NDV, ILTV, MDV, IBDV, FAdV-1, FAdV-2, FAdV-3, FAdV-5, FAdV-6, FAdV-7, FAdV-8a, FAdV-8b, FAdV-9, FAdV-10, FAdV-11) tested negative by the assay. These results demonstrate that the one-tube RPA-CRISPR/Cas12a method exhibited high specificity, as only the target virus produced positive result, with no cross-reactivity observed with other common pathogens.
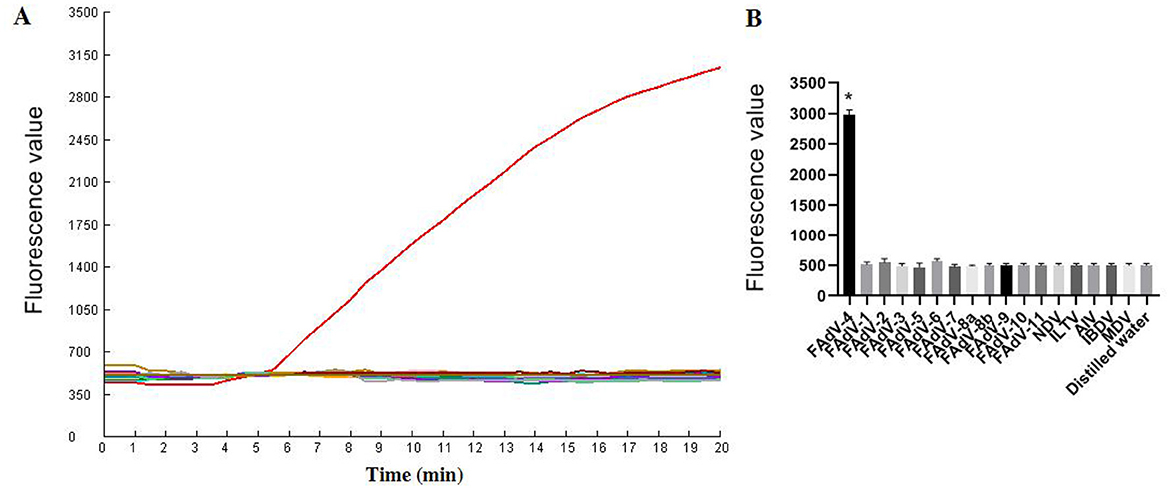
Figure 6. Specificity results based on fluorescence detection. (A) FAdV-4 and other common avain viruses (AIV, NDV, ILTV, MDV, IBDV, FAdV-1, FAdV-2, FAdV-3, FAdV-5, FAdV-6, FAdV-7, FAdV-8a, FAdV-8b, FAdV-9, FAdV-10, FAdV-11) were detected by the RPA CRISPR/Cas12a assay; (B) The tests were conducted in triplicate. *Represents a p-value less than 0.05.
Clinical sample testing results
Upon completion of the DNA extraction, the RPA-CRISPR/Cas12a assay was performed to assess the presence of FAdV-4 in the clinical samples. The results indicated that 18 out of the 40 samples tested positive for FAdV-4, demonstrating a high prevalence of the virus among the affected chickens. Among these 18 positive samples, 9 heart samples and 9 liver samples from 9 chickens were positive. This result indicates that the method has the same effect on detecting different samples. The samples were also analyzed using qPCR, revealing that out of the 18 samples that tested positive for RPA, real-time qPCR confirmed 17 samples as positive, with CT values ranging from 21 to 31; one sample returned a negative result. Conversely, among the 22 samples that tested negative via RPA, qPCR also identified them all as negative. The agreement rate between the qPCR assay and the RPA assay for clinical samples was 97.5% (Table 3).
Discussion
FAdV-4 is a highly contagious virus affecting various bird species, especially poultry and wild birds, causing avian inclusion body hepatitis (IBH) and hepatitis-hydropericardium syndrome (HHS). The development of rapid diagnostic tools for detecting FAdV-4 is essential for effective disease control and eradication efforts. In this study, we have developed a RPA assay in combination with CRISPR/Cas12a, specifically targeting the Hexon gene. The RPA-CRISPR/Cas12a assay can detect as few as 10 genomic copies per reaction, demonstrating high sensitivity comparable to qPCR. Additionally, the assay displays high specificity for FAdV-4, with no cross-reactivity observed with other common avian pathogens.
Numerous studies have explored a range of methods for detecting FAdV-4. PCR-RFLP involves cutting the amplified PCR product with specific endonucleases and then visualizing the results using gel electrophoresis (Raue and Hess, 1998; Mase et al., 2010). Raue et al. designed two pairs of primers targeting the conserved region of the Hexon gene in 1998. This was followed by amplification of the gene and digestion with the Hae II enzyme, allowing for the differentiation of 12 FAdV reference strains (Raue and Hess, 1998). Mase et al. (2010) also designed primers targeting the fiber-1 gene of FAdV-4 and FAdV-10. They utilized PCR-RFLP combined with the Alu I enzyme to differentiate between FAdV-4 and FAdV-10. However, these methods of amplification followed by digestion with endonucleases were relatively complex and may not be suitable for processing a large number of clinical samples.
Mase et al. (2021) established a routine gel-based PCR detection method enabling the simultaneous identification of FAdV-1, FAdV-2, FAdV-4, FAdV-8a, and FAdV-8b. Wang et al. (2017a) designed two primers and a probe based on the FAdV-4 Hexon gene sequence to establish a qPCR detection method, achieving a minimum detection limit of 10 copies/μL. Zhai et al. (2019) designed two pairs of primers based on the sequence of conserved region of the 52K gene and developed a LAMP assay for the detection of FAdV-4, with a detection limit of 10 copies/μL.
Despite the establishment of these detection methods, they are primarily suited for laboratory settings and are not ideal for regions with inadequate infrastructure and limited resources. This limitation poses significant challenges for effectively preventing and controlling FAdV-4 infection. To address these issues, this study developed a rapid detection method for FAdV-4 using RPA in combination with CRISPR/Cas12a, thus compensating for the aforementioned shortcomings.
Literature reviews have demonstrated that CRISPR/Cas12a has become a widely utilized and emerging diagnostic tool in veterinary clinical testing. Typically, before conducting CRISPR/Cas12a testing, the target gene is first amplified using nucleic acid amplification methods (Huang et al., 2023; Kostyusheva et al., 2022; Yin et al., 2024).
Conventional PCR and isothermal amplification methods, including RPA and LAMP, are effective techniques for amplifying target genes for DNA detection. However, PCR requires multiple thermal cycles in a thermal cycler for amplification. LAMP generally operates within a consistent temperature range of 60–66°C; however, its complex primer design and susceptibility to non-specific effects can hinder its suitability for on-site applications. In contrast, RPA allows for the simultaneous opening of DNA double strands and synthesis of complementary strands, enabling amplification to be completed in just 20 min, making it particularly well-suited for on-site deployment (Ma et al., 2020, 2023; Zhu et al., 2022; Ma et al., 2022; Tan et al., 2022; Li et al., 2018; Wang J. C. et al., 2017; Wang et al., 2017c,b). While RPA does carry a risk of non-specific amplification, the addition of CrRNA significantly enhances its specificity (Kellner et al., 2019).
The diagnostic method established in the present study operates within a single reaction tube. Initially, RPA is employed to amplify the target fragment, which can be incubated at 39°C for 20 min, with no special instrument requirements during the reaction process. Subsequently, The CRISPR/Cas12a components, positioned on the tube lid, were driven into the tube by centrifugal force, resulting in a cleavage reaction of the ssDNA reporter molecule. This detection occurs within a single reaction tube, allowing for analysis with a fluorescence detector without the need to open the tube. This approach not only simplifies the procedure but also minimizes the risk of potential aerosol contamination. Previous studies typically employed a two-step approach, separating RPA amplification from CRISPR/Cas12a detection (Liu et al., 2021; Zhao et al., 2023; Talwar et al., 2021). The two-step method can be cumbersome and susceptible to various influencing factors, potentially resulting in biased outcomes. In contrast, the one-tube method used in this study streamlines the process, reduces operational complexity, minimizes the risk of error, enhances detection efficiency, and supports broader application in clinical settings.
A RPA assay for detecting FAdV has been reported (Zhang et al., 2020). The amplification process was completed in just 14 min, marking a significant improvement over conventional PCR, which typically requires 98 min. However, similar to PCR, the RPA assay still necessitates agarose gel electrophoresis for result analysis, which takes ~30 min to prepare and run. Consequently, when comparing the gel-based RPA method to conventional PCR, there are no substantial advancements in operational convenience. In contrast, our study established a real-time assay that eliminates the need for electrophoresis following amplification, significantly enhancing efficiency. A previous study described a detection method that combines RPA with test strips for virus identification. While this approach is effective, it necessitates opening the reaction tube and transferring the RPA product to the test strip for detection. This process not only extends the overall experimental time but also heightens the risk of aerosol contamination (Jirawannaporn et al., 2022).
This new detection method for FAdV-4 is designed for on-site testing. Our study integrates RPA and CRISPR/Cas12a technology to develop a novel diagnostic method that offers an alternative assay for the early monitoring of FAdV-4 and the epidemiological investigation of local chicken populations. The real-time RPA-CRISPR/Cas12a assay is user-friendly, highly specific, and sensitive, requiring no specialized instruments or equipment. Its accessibility makes it particularly suitable for implementation in remote areas. Therefore, this research establishes a simple, efficient, rapid, and accurate method for detecting FAdV-4, representing a significant advancement in the prevention and control of this virus.
Data availability statement
The original contributions presented in the study are included in the article/supplementary material, further inquiries can be directed to the corresponding author.
Ethics statement
The animal study was approved by the Institutional Animal Care and Use Committee (IACUC) of Anyang Institute of Technology, Anyang, China. The study was conducted in accordance with the local legislation and institutional requirements.
Author contributions
LM: Writing – original draft, Writing – review & editing. XW: Writing – original draft, Writing – review & editing. MZha: Writing – original draft, Writing – review & editing. MZhu: Writing – original draft, Writing – review & editing.
Funding
The author(s) declare financial support was received for the research, authorship, and/or publication of this article. This study was supported by grants from Science and Technology Program of Henan Province (212102110090).
Conflict of interest
The authors declare that the research was conducted in the absence of any commercial or financial relationships that could be construed as a potential conflict of interest.
Generative AI statement
The author(s) declare that no Gen AI was used in the creation of this manuscript.
Publisher's note
All claims expressed in this article are solely those of the authors and do not necessarily represent those of their affiliated organizations, or those of the publisher, the editors and the reviewers. Any product that may be evaluated in this article, or claim that may be made by its manufacturer, is not guaranteed or endorsed by the publisher.
References
Asthana, M., Chandra, R., and Kumar, R. (2013). Hydropericardium syndrome: current state and future developments. Arch. Virol. 158, 921–931. doi: 10.1007/s00705-012-1570-x
Chen, L., Yin, L., Zhou, Q., Peng, P., Du, Y., Liu, L., et al. (2019). Epidemiological investigation of fowl adenovirus infections in poultry in China during 2015-2018. BMC Vet. Res. 15:271. doi: 10.1186/s12917-019-1969-7
Grafl, B., Aigner, F., Liebhart, D., Marek, A., Prokofieva, I., Bachmeier, J., et al. (2012). Vertical transmission and clinical signs in broiler breeders and broilers experiencing adenoviral gizzard erosion. Avian Pathol. 41, 599–604. doi: 10.1080/03079457.2012.740614
Hess, M. (2000). Detection and differentiation of avian adenoviruses: a review. Avian Pathol. 29, 195–206. doi: 10.1080/03079450050045440
Huang, M., Chen, Y., Zheng, L., and Yao, Y. F. (2023). Highly sensitive and naked-eye detection of herpes simplex virus type 1 using LAMP- CRISPR/Cas12 diagnostic technology and gold nanoparticles. Heliyon 9:e22146. doi: 10.1016/j.heliyon.2023.e22146
Jiang, Z., Liu, M., Wang, C., Zhou, X., Li, F., Song, J., et al. (2019). Characterization of fowl adenovirus serotype 4 circulating in chickens in China. Vet. Microbiol. 238:108427. doi: 10.1016/j.vetmic.2019.108427
Jirawannaporn, S., Limothai, U., Tachaboon, S., Dinhuzen, J., Kiatamornrak, P., Chaisuriyong, W., et al. (2022). Rapid and sensitive point-of-care detection of Leptospira by RPA-CRISPR/Cas12a targeting lipL32. PLoS Negl. Trop. Dis. 16:e0010112. doi: 10.1371/journal.pntd.0010112
Kellner, M. J., Koob, J. G., Gootenberg, J. S., Abudayyeh, O. O., and Zhang, F. (2019). SHERLOCK: nucleic acid detection with CRISPR nucleases. Nat. Protoc. 14, 2986–3012. doi: 10.1038/s41596-019-0210-2
Kostyusheva, A., Brezgin, S., Babin, Y., Vasilyeva, I., Glebe, D., Kostyushev, D., et al. (2022). CRISPR-Cas systems for diagnosing infectious diseases. Methods 203, 431–446. doi: 10.1016/j.ymeth.2021.04.007
Kumar, R., Chandra, R., Shukla, S. K., Agrawal, D. K., and Kumar, M. (1997). Hydropericardium syndrome (HPS) in India: a preliminary study on the causative agent and control of the disease by inactivated autogenous vaccine. Trop. Anim. Health Prod. 29, 158–164. doi: 10.1007/BF02633014
Li, J., Macdonald, J., and von Stetten, F. (2018). Review: a comprehensive summary of a decade development of the recombinase polymerase amplification. Analyst 144, 31–67. doi: 10.1039/C8AN01621F
Li, P. H., Zheng, P. P., Zhang, T. F., Wen, G. Y., Shao, H. B., Luo, Q. P., et al. (2017). Fowl adenovirus serotype 4: Epidemiology, pathogenesis, diagnostic detection, and vaccine strategies. Poult. Sci. 96, 2630–2640. doi: 10.3382/ps/pex087
Lin, Z., Sun, B., Yang, X., Jiang, Y., Wu, S., Lv, B., et al. (2023). Infectious disease diagnosis and pathogen identification platform based on multiplex recombinase polymerase amplification-assisted CRISPR-Cas12a system. ACS Infect. Dis. 9, 2306–2315. doi: 10.1021/acsinfecdis.3c00381
Liu, P., Wang, X., Liang, J., Dong, Q., Zhang, J., Liu, D., et al. (2021). A recombinase polymerase amplification-coupled Cas12a mutant-based module for efficient detection of streptomycin-resistant mutations in mycobacterium tuberculosis. Front. Microbiol. 12:796916. doi: 10.3389/fmicb.2021.796916
Ma, L., Lian, K., Zhu, M., Tang, Y., and Zhang, M. (2022). Visual detection of porcine epidemic diarrhea virus by recombinase polymerase amplification combined with lateral flow dipstrip. BMC Vet. Res. 18:140. doi: 10.1186/s12917-022-03232-5
Ma, L., Shi, H., Zhang, M., Song, Y., Zhang, K., Cong, F., et al. (2020). Establishment of a real-time recombinase polymerase amplification assay for the detection of avian reovirus. Front. Vet. Sci. 7, 551350. doi: 10.3389/fvets.2020.551350
Ma, L., Zhu, M., Meng, Q., Wang, Y., and Wang, X. (2023). Real-time detection of Seneca Valley virus by one-tube RPA-CRISPR/Cas12a assay. Front. Cell. Infect. Microbiol. 13:1305222. doi: 10.3389/fcimb.2023.1305222
Mase, M., Hiramatsu, K., Nishijima, N., Iseki, H., and Watanabe, S. (2021). Identification of specific serotypes of fowl adenoviruses isolated from diseased chickens by PCR. J. Vet. Med. Sci. 83, 130–133. doi: 10.1292/jvms.20-0400
Mase, M., Nakamura, K., and Imada, T. (2010). Characterization of Fowl adenovirus serotype 4 isolated from chickens with hydropericardium syndrome based on analysis of the short fiber protein gene. J. Vet. Diagn. Investigat. 22, 218–223. doi: 10.1177/104063871002200207
Mo, J. (2021). Historical investigation of fowl adenovirus outbreaks in South Korea from 2007 to 2021: a comprehensive review. Viruses 13:2256. doi: 10.3390/v13112256
Qian, W., Huang, J., Wang, X., Wang, T., and Li, Y. (2021). CRISPR-Cas12a combined with reverse transcription recombinase polymerase amplification for sensitive and specific detection of human norovirus genotype GII.4. Virology 564, 26–32. doi: 10.1016/j.virol.2021.09.008
Raue, R., and Hess, M. (1998). Hexon based PCRs combined with restriction enzyme analysis for rapid detection and differentiation of fowl adenoviruses and egg drop syndrome virus. J. Virol. Methods 73, 211–217. doi: 10.1016/S0166-0934(98)00065-2
Schachner, A., Matos, M., Grafl, B., and Hess, M. (2018). Fowl adenovirus-induced diseases and strategies for their control - a review on the current global situation. Avian Pathol. 47, 111–126. doi: 10.1080/03079457.2017.1385724
Sun, J., Zhang, Y., Gao, S., Yang, J., Tang, Y., Diao, Y., et al. (2019). Pathogenicity of fowl adenovirus serotype 4 (FAdV-4) in chickens. Infect. Genet. Evol. 75:104017. doi: 10.1016/j.meegid.2019.104017
Talwar, C. S., Park, K. H., Ahn, W. C., Kim, Y. S., Kwon, O. S., Yong, D., et al. (2021). Detection of infectious viruses using CRISPR-Cas12-based assay. Biosensors 11:301. doi: 10.3390/bios11090301
Tan, M., Liao, C., Liang, L., Yi, X., Zhou, Z., Wei, G., et al. (2022). Recent advances in recombinase polymerase amplification: Principle, advantages, disadvantages and applications. Front. Cell. Infect. Microbiol. 12:1019071. doi: 10.3389/fcimb.2022.1019071
Wang, J., Wang, J., Chen, P., Liu, L., and Yuan, W. (2017a). Development of a TaqMan-based real-time PCR assay for rapid and specific detection of fowl aviadenovirus serotype 4. Avian Pathol. 46, 338–343. doi: 10.1080/03079457.2016.1278428
Wang, J., Wang, J., Geng, Y., and Yuan, W. (2017b). A recombinase polymerase amplification-based assay for rapid detection of African swine fever virus. Can. J. Vet. Res. 81, 308−312.
Wang, J., Zhang, Y., Zhang, R., Han, Q., Wang, J., Liu, L., et al. (2017c). Recombinase polymerase amplification assay for rapid detection of porcine circovirus 3. Mol. Cell. Probes 36, 58–61. doi: 10.1016/j.mcp.2017.09.001
Wang, J. C., Liu, L. B., Han, Q. A., Wang, J. F., and Yuan, W. Z. (2017). An exo probe-based recombinase polymerase amplification assay for the rapid detection of porcine parvovirus. J. Virol. Methods 248, 145–147. doi: 10.1016/j.jviromet.2017.06.011
Wang, J. Y., and Doudna, J. A. (2023). CRISPR technology: A decade of genome editing is only the beginning. Science 379:eadd8643. doi: 10.1126/science.add8643
Wang, K., Sun, H., Li, Y., Yang, Z., Ye, J., Chen, H., et al. (2019). Characterization and pathogenicity of fowl adenovirus serotype 4 isolated from eastern China. BMC Vet. Res. 15:373. doi: 10.1186/s12917-019-2092-5
Wang, Z., and Zhao, J. (2019). Pathogenesis of hypervirulent fowl adenovirus serotype 4: the contributions of viral and host factors. Viruses 11:741. doi: 10.3390/v11080741
Xiong, Y., Cao, G., Chen, X., Yang, J., Shi, M., Wang, Y., et al. (2022). One-pot platform for rapid detecting virus utilizing recombinase polymerase amplification and CRISPR/Cas12a. Appl. Microbiol. Biotechnol. 106, 4607–4616. doi: 10.1007/s00253-022-12015-9
Yin, X., Yang, L., Sun, X., Zheng, Q., Piao, Y., Hu, B., et al. (2024). Development and validation of sensitive and rapid CRISPR/Cas12-based PCR method to detect hazelnut in unlabeled products. Food Chem. 438:137952. doi: 10.1016/j.foodchem.2023.137952
Yu, G., Lin, Y., Dou, Y., Tang, Y., and Diao, Y. (2019). Prevalence of fowl adenovirus serotype 4 and co-infection by immunosuppressive viruses in fowl with hydropericardium hepatitis syndrome in Shandong Province, China. Viruses 11:517. doi: 10.3390/v11060517
Yuming, F., Sheng, Y., Wenyu, D., Shihong, C., Wenfeng, L., Wenjing, H., et al. (2020). Molecular characterization and phylogenetic analysis of fowl adenovirus serotype-4 from Guangdong Province, China. Vet. World 13, 981–986. doi: 10.14202/vetworld.2020.981-986
Zhai, X., Mei, X., Wu, X., Zuo, L., Zhou, L., Tian, Y., et al. (2019). A loop-mediated isothermal amplification coupling with a lateral flow dipstick for rapid and specific detection of fowl adenovirus serotype-4. J. Virol. Methods 270, 79–86. doi: 10.1016/j.jviromet.2019.04.026
Zhang, J., Liu, J., An, D., Fan, Y., Cheng, Z., Tang, Y., et al. (2020). A novel recombinase polymerase amplification assay for rapid detection of epidemic fowl adenovirus. Poult. Sci. 99, 6446–6453. doi: 10.1016/j.psj.2020.08.021
Zhang, W. S., Pan, J., Li, F., Zhu, M., Xu, M., Zhu, H., et al. (2021). Reverse transcription recombinase polymerase amplification coupled with CRISPR-Cas12a for facile and highly sensitive colorimetric SARS-CoV-2 detection. Anal. Chem. 93, 4126–4133. doi: 10.1021/acs.analchem.1c00013
Zhao, F., Wang, P., Wang, H., Liu, S., Sohail, M., Zhang, X., et al. (2023). CRISPR/Cas12a-mediated ultrasensitive and on-site monkeypox viral testing. Analyt. Meth. 15, 2105–2113. doi: 10.1039/D2AY01998A
Keywords: avian adenovirus type 4, Cas12a, recombinase polymerase amplification, detection, sensitivity
Citation: Ma L, Wang X, Zhang M and Zhu M (2025) Rapid detection of FAdV-4 by one-tube RPA-CRISPR/Cas12a assay. Front. Microbiol. 16:1541943. doi: 10.3389/fmicb.2025.1541943
Received: 10 December 2024; Accepted: 13 January 2025;
Published: 03 February 2025.
Edited by:
Leiliang Zhang, Shandong First Medical University and Shandong Academy of Medical Sciences, ChinaReviewed by:
Shubo Wen, Inner Mongolia Minzu University, ChinaMahasen Khudeir, University of Al-Qadisiyah, Iraq
Copyright © 2025 Ma, Wang, Zhang and Zhu. This is an open-access article distributed under the terms of the Creative Commons Attribution License (CC BY). The use, distribution or reproduction in other forums is permitted, provided the original author(s) and the copyright owner(s) are credited and that the original publication in this journal is cited, in accordance with accepted academic practice. No use, distribution or reproduction is permitted which does not comply with these terms.
*Correspondence: Mengjie Zhu, em1qMTU4NDAzNTQ5MzZAMTYzLmNvbQ==
†These authors have contributed equally to this work