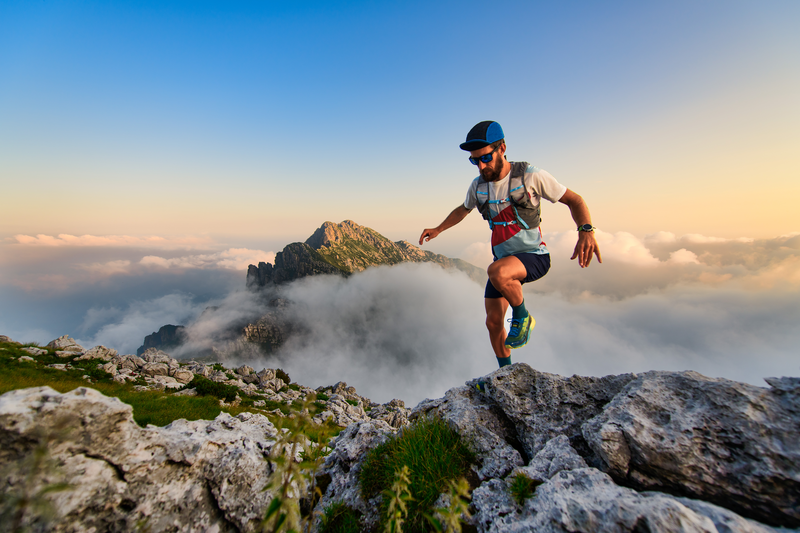
95% of researchers rate our articles as excellent or good
Learn more about the work of our research integrity team to safeguard the quality of each article we publish.
Find out more
ORIGINAL RESEARCH article
Front. Microbiol. , 22 January 2025
Sec. Terrestrial Microbiology
Volume 16 - 2025 | https://doi.org/10.3389/fmicb.2025.1510904
This article is part of the Research Topic Microbe Empowering Green Agriculture and Boosting Productivity and Quality View all 11 articles
Background: Intercropping Saccharum spp. (sugarcane) with the fungus Dictyophora indusiata together with bagasse amendment represents an innovative circular agriculture method that can enhance soil health, boost sugarcane yields, and increase farm profitability. Understanding the process by which D. indusiata degrades bagasse is key to optimizing this method.
Aims: This study aims to clarify the microbial and metabolic processes involved in bagasse degradation by D. indusiata in the sugarcane intercropping system.
Methods: Chemical composition analysis, metabarcoding sequencing, and metabolomic profiling were conducted on D. indusiata-degraded bagasse (DIBA) and naturally degraded bagasse (BA).
Results: Analysis of chemical composition revealed that only acid detergent fiber (ADF) and crude protein content differed significantly between the DIBA and BA treatments. Metabarcoding sequencing showed that DIBA significantly altered the bacterial and fungal communities, reducing microbial diversity. Metabolomic analysis indicated an enhancement of biological metabolism, particularly carbohydrate breakdown, in the DIBA treatment. Key metabolites, such as glucose, cellobiose, and D-mannose, were more abundant in DIBA samples. In addition, unique metabolites such as L-alanine, serine, and oxaloacetate were detected in the DIBA treatment, suggesting more efficient bagasse degradation compared with natural processes.
Conclusion: The use of macrofungi such as D. indusiata can play a pivotal role in circular agriculture by transforming agricultural waste into valuable soil amendments. Future research should focus on the long-term impact of this system on soil quality and crop yield, as well as the underlying mechanisms, to further optimize intercropping systems and the use of fungi in agricultural waste management.
The sustainable production of food has long been an important goal for mankind, but this goal is increasingly challenged by the unabated growth of the human population and the intensification of climate change (Zhang et al., 2018). Large quantities of fertilizer have been applied to accommodate the growing demand for food. However, the excessive application of fertilizer has caused deleterious effects on both crop production (for example, decreased yields) and the environment (such as environmental pollution and reductions in soil biodiversity) in China as well as in several other developing countries (Xu et al., 2020; Cui et al., 2020; Li et al., 2019; Vitousek et al., 2009). There is thus a pressing need to develop approaches that provide some of the beneficial effects of fertilizer application without compromising agricultural productivity or damaging the soil environment (Duan et al., 2022). Bagasse is a plant residue that remains after the extraction of sugar from crops such as sugarcane, and inorganic nutrients [e.g., phosphorus (P) and potassium (K)] required for plant growth are abundant in bagasse (Sharma et al., 2015). It is therefore often used as an auxiliary fertilizer in sugarcane fields after harvest and sugar extraction (Seleiman and Kheir, 2018). The application of bagasse is considered an effective strategy for alleviating the deleterious effects of excessive fertilizer application and more generally for achieving the goals of ecological agriculture, which aims to enhance production while promoting ecological sustainability. Bagasse application has been shown to enhance soil health by increasing the abundance of various soil nutrients (Sharma et al., 2015; Seleiman and Kheir, 2018; Bento et al., 2019) and pH (Mohamed et al., 2019). Moreover, bagasse application promotes the activity of soil microbes and increases the diversity of soil microbial communities (Ullah et al., 2020; Bhadha et al., 2020).
Dictyophora indusiata (DI), which is in the family Phallaceae, is an economically valuable, edible mushroom that is used in Chinese cuisine; it is also widely used as a Chinese medicinal material (Wang et al., 2021). Several culture substrates can be used to grow DI and improve soil nutrients, including sugarcane bagasse (Tong et al., 2021). Thus, the application of bagasse to sugarcane fields can enhance sugarcane production and promote soil health.
Building on this, a previous study conducted an intercropping experiment with D. indusiata and sugarcane using bagasse as a substrate. The findings revealed that growing D. indusiata in bagasse, intended as a fertilizer, not only reduced soil nitrogen loss by inhibiting soil urease synthesis (Duan et al., 2023) but also promoted the formation of a “white root” symbiosis with sugarcane, increasing flavonoid accumulation in the roots and significantly boosting sugarcane yield (Duan et al., 2024). These results suggest that degradation of bagasse by D. indusiata offers more soil and crop benefits than does natural degradation. Understanding the bagasse degradation process is crucial for optimizing this intercropping method and advancing circular agricultural techniques. However, the specifics of how D. indusiata degrades bagasse during field cultivation remain unclear, limiting further development of this ecological cultivation method.
Macrofungi play a crucial role in the degradation of bagasse and other organic materials (Ahmed et al., 2018). First, they secrete a variety of enzymes, such as cellulases, hemicellulases, and ligninases (Al-Dhabi et al., 2021). These enzymes break down the complex components of bagasse, including cellulose, hemicellulose, and lignin. For example, cellulases hydrolyze cellulose into smaller sugar molecules, which can then be used by the fungus and other microorganisms (Wilson, 2011; Lynd Lee et al., 2002). Hemicellulases act on hemicellulose to release various sugars and other compounds (Saha, 2003). Ligninases degrade lignin, a complex polymer that is difficult to decompose (Laca et al., 2019). As the degradation process proceeds, macrofungi and associated microorganisms consume the degraded products for their growth and metabolism (Lu et al., 2020). This leads to the release of nutrients such as nitrogen, phosphorus, and potassium, which can be absorbed by plants and contribute to soil fertility. In addition, the degradation process can also modify the physical and chemical properties of bagasse, making it more accessible to other microorganisms and promoting the overall activity of the soil microbial community (Liu et al., 2023). Understanding the process by which large fungi degrade bagasse can provide valuable insights for improving intercropping methods and promoting the development of sustainable sugarcane cultivation techniques. By optimizing the degradation process, we can enhance the efficiency of nutrient release from bagasse, improve soil health, and increase crop productivity.
Understanding the microbial diversity and metabolic processes involved in bagasse degradation by D. indusiata is key to optimizing this process. Recent advances in second-generation sequencing technologies, particularly metabarcoding sequencing, have enabled the detailed analysis of microbial diversity in macro environments (Duan and Bau, 2021a; Duan and Bau, 2021b; Duan et al., 2023). In addition, derivatization-based metabolomic analysis using Gas Chromatography - Tandem Mass Spectrometry (GC–MS/MS) has emerged as a powerful tool for studying complex metabolites (Duan et al., 2022; Duan et al., 2023). Therefore, combining metabarcoding sequencing and GC–MS/MS-based metabolomics holds promise for revealing the characteristics of D. indusiata-mediated bagasse degradation.
This study aimed to clarify the characteristics of bagasse degraded by D. indusiata in a sugarcane intercropping system. By analyzing the chemical composition, fungal and bacterial diversity, and metabolomic profiles of bagasse samples cultivated with or without D. indusiata, we aimed to provide insights into this degradation process. Notably, our approach integrating multiple advanced techniques to dissect the complex interactions between the macrofungus, microbial communities, and metabolites during bagasse degradation is unprecedented, which sets a new benchmark for future research in this area and offers a novel perspective to optimize circular agricultural practices.
The experiment was performed in a field in Laibin City, Guangxi, China (23°16′N, 108°24′E) from February to August 2022. Two treatments were applied: (1) Bagasse process, in which crushed, air-dried bagasse was added to the soil between the sugarcane rows; and (2) Bagasse + Dictyophora process, in which crushed, air-dried bagasse mixed with D. indusiata strains (variety: Gutian-1; Duan et al., 2023; obtained via wheat-grain fermentation with 35 d) was applied between the rows, all treatments were maintained at high soil moisture (Figure 1A). The locally sourced sugarcane variety GT42 was used throughout the experiment, planted with an interrow spacing of approximately 50 cm and a row depth of 30 cm. The detailed intercropping model settings are consistent with those in the research published in the early stage of this study (Duan et al., 2023).
Figure 1. The experimental approach used in this study. (A) Sugarcane and Dictyophora indusiata intercropping. The image in the upper right shows an aerial view of the field and the division of treatments within the field. The three images in the lower left show sugarcane rows from the different treatments [Control, naturally degraded bagasse (BA) and D. indusiata-degraded bagasse (DIBA)]. (B) Appearance of the bagasse in the BA and DIBA treatments. (C) Flowchart of analyses performed in this study.
After 60 days of cultivation, when the sugarcane plants had reached about 50 cm in height, five semi-rectangular areas were marked for the treatments. The control and bagasse processes were each duplicated, whereas the Bagasse + Dictyophora process was performed in a single area at the center of the site (Figure 1A). The fruiting bodies of D. indusiata matured after roughly 60 days, and soil moisture was maintained at a high level during this period.
As shown in Figures 1A,B, five random points were selected in both the adjacent naturally degraded bagasse (BA) and D. indusiata-degraded bagasse (DIBA) areas as biological replicates, as Figure 1 Shown. Bagasse samples were collected by removing the top layer and sampling, and avoid mixing soil and root material, at a depth of 2–10 cm (for each point in the Figure 1A, bagasse from five separate sub-points was mixed for each sample, i.e., totaling 25 samples per treatment). All samples were frozen, ground into powder, packed into sterile cryostorage tubes, and preserved in liquid nitrogen.
After air-drying, the bagasse samples were crushed. The contents of plant cellulose, hemicellulose, lignin, acid detergent fiber (ADF), and neutral detergent fiber (NDF) were determined using the paradigm washing method combined with a crude fiber analyzer as described previously (Kong et al., 2017). The plant crude protein content was measured using sulfuric acid–catalyst digestion followed by the Kjeldahl nitrogen method (Shang et al., 2022).
To extract DNA from bagasse samples (3 g), we used the HiPure Soil DNA Kit (Magen, Guangzhou, China) according to the manufacturer’s protocol as described previously (Duan et al., 2022). The 16S rDNA V3–V4 region of the ribosomal RNA gene was amplified by polymerase chain reaction (PCR) using the primers 341F (5′-CCTACGGGNGGCWGCAG-3′) and 806R (5′-GGACTACHVGGGTATCTAAT-3′) for bacteria (Guo et al., 2017) and the primers ITS3_KYO2 (5′-GATGAAGAACGYAGYRAA-3′) and ITS4 (5′-TCCTCCGCTTATTGATATGC-3′) for fungi (Duan et al., 2022). Sequencing was performed on the Illumina NovaSeq 6,000 platform and involved paired-end sequencing of pooled purified metabarcoding libraries in equimolar ratios according to standard protocols (These steps were performed by Gene Denovo Co., Ltd., Guangzhou, China). Classification of sequences into representative operational taxonomic units (OTUs) was performed using a naïve Bayesian model with The Ribosomal Database Project classifier (version 2.2) (Wang et al., 2007). Annotation was based on the SILVA (16S rRNA metabarcoding data) (Pruesse et al., 2007) and UNITE (ITS metabarcoding data) (Nilsson et al., 2019) (version 8.0) databases. All figures were generated using R software, with the VennDiagram package (version 1.6.16) used to compare OTUs among groups (Chen and Boutros, 2011). The Shannon index and other alpha diversity indices were calculated using QIIME (version 1.9.1) (Caporaso et al., 2010). Principal component analysis (PCA) was performed to examine variations in OTU composition among experimental groups using the vegan R package.1 Statistically significant differences between treatments were determined using Welch’s t-test performed with the vegan package. The FAPROTAX database (Functional Annotation of Prokaryotic Taxa; version 1.0) (Louca et al., 2016) was used to predict ecologically relevant bacterial functions, and FUNGuild (Nguyen et al., 2016) (version 1.0) was used to predict the functional groups (guilds) of fungi.
Metabolite analysis was performed using a previously published method [6; 15]. Approximately 0.5 g of bagasse sample was mixed with 1 mL of methanol: isopropanol: water (3: 3: 2 v/v/v), vortexed for 3 min, and subjected to ultrasound for 20 min. After centrifugation at 12,000 r/min and 4°C for 3 min, the supernatant was combined with 0.020 mL internal standard (10 μg/mL) in a sample vial and evaporated under nitrogen. The residue was freeze-dried in a lyophilizer, derivatized by mixing with 0.1 mL methoxy-amine hydrochloride in pyridine (0.015 g/mL), and incubated at 37°C for 2 h. Then, 0.1 mL bis (trimethylsilyl) trifluoroacetamide (BSTFA) [with 1% trimethylchlorosilane (TMCS)] was added, and the mixture was kept at 37°C for 30 min after vortexing. Approximately 0.2 mL of the derivatization solution was diluted with n-hexane to 1 mL, filtered through a 0.22-μm organic phase syringe filter, stored at −20°C, and analyzed within 24 h.
Metabolites were analyzed by gas chromatography–mass spectrometry (GC–MS) on an Agilent 8,890 gas chromatograph coupled to a 5977B mass spectrometer with a DB-5MS column (30 m length × 0.25 mm i.d. × 0.25 μm film thickness, J&W Scientific, United States). Helium was used as the carrier gas at a 1.2 mL/min flow rate. Samples were injected in front inlet mode with a split ratio of 5:1, and the injection volume was 1 μL. The oven temperature protocol consisted of an initial hold at 40°C for 1 min, ramping to 100°C at 20°C/min, further ramping to 300°C at 15°C/min, and a final hold at 300°C for 5 min. All samples were scanned in scan mode with ion source and transfer line temperatures of 230°C and 280°C, respectively.
Statistical analysis involved unsupervised PCA using the prcomp function in R, with data scaled to unit variance prior to PCA. Metabolites with a variable importance in projection (VIP) ≥ 1 and absolute log2 (fold change) (log2FC) ≥ 1 were considered to differ significantly between the BA and DIBA treatments. VIP values were obtained from orthogonal partial least squares discriminant analysis (OPLS-DA) results, which included score plots and permutation plots generated with the R package Metabo-AnalystR. Prior to OPLS-DA, the original data were log2-transformed and mean-centered, and a permutation test (200 permutations) was performed to prevent overfitting.
We first analyzed differences in the chemical composition of bagasse from the BA and DIBA treatments. As shown in Figure 2, among the six measured indicators, only ADF (with mean values of 52.99% for BA and 51.2% for DIBA) and crude protein (with mean values of 6.18 g/100 g for BA and 5.31 g/100 g for DIBA) showed significant differences (p < 0.05). We concluded that DI degradation and natural degradation had relatively similar effects on the overall chemical composition of bagasse.
Figure 2. Chemical composition of bagasse from the BA and DIBA treatments (n = 3). The vertical axis units are percentage for neutral detergent fiber (NDF), acid detergent fiber (ADF), hemicellulose, cellulose, and lignin and g/100 g for crude protein. Data are expressed as mean ± SD. Numbers above the bars indicate the significance of differences between the two treatments based on Welch’s t-test. ns, p > 0.05. NDF, neutral detergent fiber. ADF, acid detergent fiber.
To explore the microbial processes involved in DI degradation of bagasse, we performed metabarcoding sequencing of replicated bagasse samples from the BA and DIBA treatments, targeting 16S rRNA for bacteria and ITS for fungi. The sequencing generated 2,086,246 tags, with an average N50 length of 447 bp for bacteria and 361 bp for fungi. The range of OTUs across biological replicates (n = 5) was 2,296–3,419 for bacteria (mean 2,996) and 427–640 for fungi (mean 519), as detailed in Supplementary Tables S1, S2. PCA plots showed clear clustering of BA and DIBA samples from both the bacterial and fungal communities, with bacterial resolution (Figure 2A, PC1 76.72% and PC2 8.03%) and fungal resolution (Figure 2E, PC1 92.84% and PC2 4.47%) both exceeding 75%, indicating high separation accuracy. Venn diagrams of the OTUs revealed a total of 2,625 bacterial OTUs (746 unique to BA, 609 unique to DIBA, and 1,270 shared) and 689 fungal OTUs (200 unique to BA, 136 unique to DIBA, and 353 shared; Figures 3B,F). Alpha diversity analysis (Supplementary Table S3) showed that the observed species (Sob) index (Figure 3C) and the Shannon index (Figure 3D) for bacteria were significantly higher in BA (means 3,211 and 9.37) than in DIBA (means 2,782 and 8.88; p < 0.05), indicating that bacterial diversity was higher in BA. For fungi Shannon index (Supplementary Table S4), there was no significant difference in the Sob index between the BA (mean 532) and DIBA (mean 507) treatments, but the Shannon index was significantly higher in the BA treatment (mean 4.69) than in the DIBA treatment (mean 3.67) (p < 0.05; Figure 3G). Overall, these results suggest that DI degradation of bagasse significantly altered both bacterial and fungal diversity, leading to a decrease in alpha diversity.
Figure 3. OTUs and alpha diversity of bacteria and fungi in bagasse from the BA and DIBA treatments (n = 5). (A) Principal Component Analysis (PCA) plot of bacterial OTUs. (B) Venn diagram of bacterial OTUs. (C) Observed species (Sob) index of bacteria in the naturally degraded bagasse (BA) and D. indusiata-degraded bagasse (DIBA) treatments. (D) Shannon index of bacteria in the BA and DIBA treatments. (E) PCA plot of fungal OTUs. (F) Venn diagram of fungal OTUs. (G) Sob index of fungi in the BA and DIBA treatments. (H) Shannon index of fungi in the BA and DIBA treatments. In all boxplots, the top and bottom whiskers indicate the maximum and minimum values, the top margin of the box indicates the upper quartile, the lower margin indicates the lower quartile, and the central line represents the mean.
Next, we examined the most abundant taxa of bacteria and fungi present in bagasse from the BA and DIBA treatments. The top five most abundant bacterial phyla (Figure 4A) were Proteobacteria (25.04% in BA, 43.59% in DIBA), Bacteroidetes (17.51, 10.16%), Patescibacteria (11.26, 10.16%), Acidobacteria (5.18, 12.13%), and Chloroflexi (13.53, 3.03%). The top three most abundant fungal phyla (Figure 4D) were Ascomycota (68.71% in BA, 26.77% in DIBA), Basidiomycota (18.92, 70.86%), and Ciliophora (9.26, 0.85%). When bacteria in the BA and DIBA treatments were compared, the five bacterial genera with the most significant differences in relative abundance (Figure 4C) were SM1A02 (5.14% in BA, 0.85% in DIBA), Burkholderia-Caballeronia-Paraburkholderia (0.15, 3.47%), Ohtaekwangia (3, 0.28%), Acidocella (0.11, 2.88%), and Terrimonas (2.02, 0.96%). Likewise, the three fungal families (Figure 4F), with the greatest differences in relative abundance were Phallaceae (5.97% in BA, 61.46% in DIBA), Lasiosphaeriaceae (36.84, 4.73%), and Sordariales_fam_Incertae_sedis (0.92, 6.61%).
Figure 4. Bacterial and fungal taxa and functions in bagasse from the BA and DIBA treatments. (A) Relative abundance of bacterial phyla in naturally degraded bagasse (BA) and D. indusiata-degraded bagasse (DIBA) samples. (B) Functional annotations of bacterial OTUs obtained from Functional Annotation of Prokaryotic Taxa (FAPROTAX) database. (C) The five bacterial taxa with the greatest difference in abundance between BA and DIBA treatments. Significant differences in mean abundance were assessed using Welch’s t-test. (D) Relative abundance of fungal phyla in BA and DIBA samples. (E) Functional annotations of fungal OTUs obtained from the FunGuild database. (F) The three fungal taxa with the greatest difference in abundance between BA and DIBA treatments. Significant differences in mean abundance were assessed using Welch’s t-test.
Finally, we examined the functional roles of the identified microorganisms. Using the FAPROTAX database (Figure 4B), we annotated bacterial OTUs and found that the three functional modules with the largest differences in abundance between BA and DIBA samples were chemoheterotrophy (8.11% in BA, 11.66% in DIBA), aerobic chemoheterotrophy (7.5, 11.2%), and cellulolysis (0.52, 1.43%). An analysis of fungal OTUs, annotated using the FunGuild database (Figure 4E), revealed similar functional modules, with the largest differences also observed in chemoheterotrophy, aerobic chemoheterotrophy, and cellulolysis.
We next performed a metabolomic analysis to further characterize the process of bagasse degradation by DI. A total of 100 metabolites were detected, including acids (22), alcohols (12), amines (3), aromatics (3), carbohydrates (22), esters (4), heterocyclic compounds (4), ketones (1), lipids (18), nitrogen compounds (1), phenols (1), and others (9).
We first analyzed the overall patterns of metabolite abundance in BA and DIBA samples using PCA. As shown in Figure 5A, PC1 (69.6%) and PC2 (7.67%) together explained 77.27% of the variance, and the treatments were clearly separated along PC1. Most of the identified metabolites, including those from key categories such as acids, carbohydrates, and lipids, had higher abundances in DIBA samples than in BA samples (Figure 5B). For detailed information on all metabolites and their relative abundances, please refer to Supplementary Table S5. These findings suggest that the addition of DI to bagasse-amended soil significantly may increase the intensity of biological metabolism, accelerating bagasse degradation.
Figure 5. Composition and abundance of metabolites in BA and DIBA samples. (A) Principal Component Analysis (PCA) plot of metabolite abundance in naturally degraded bagasse (BA) and D. indusiata-degraded bagasse (DIBA) samples. (B) Heatmap showing the Z-score-normalized abundance values of individual metabolites from 12 classes in BA and DIBA samples. Each column corresponds to a sample, and each row corresponds to a metabolite.
We identified metabolites whose abundance differed significantly between the BA and DIBA treatments. As shown in Tables 1, 46 of the 100 detected metabolites exhibited significant differences in abundance between the BA and DIBA samples. The three metabolites with the greatest increase in abundance in DIBA compared with BA were 4-aminobutanoic acid (Log2FC = 15.7), β-gentiobiose octamethyl (Log2FC = 11.75), and D-galactose 2 (Log2FC = 9.27). Notably, 17 of the 22 detected carbohydrate metabolites (77.27%) were significantly more abundant in DIBA, suggesting that carbohydrate and energy metabolism were particularly stimulated during the process of bagasse degradation by DI.
Table 1. Forty-six differentially abundant metabolites between the naturally degraded bagasse (BA) and D. indusiata-degraded bagasse (DIBA) treatments.
To further investigate the metabolites associated with bagasse degradation by DI, we quantified the absolute concentrations of 33 carbohydrates, including 25 monosaccharides, 6 disaccharides, and 1 trisaccharide, in BA and DIBA samples. As shown in Table 2, we detected 17 carbohydrate metabolites, including 4 disaccharides and 13 monosaccharides. The carbohydrate that showed the greatest difference in concentration between BA and DIBA samples was glucose, with concentrations of 27.61 ± 1.27 mg/g in BA samples and 510.04 ± 38.37 mg/g in DIBA samples (Log2FC = 4.21). In addition, cellobiose, D-mannose, and D-galactose were detected only in the DIBA samples. Sucrose was the only carbohydrate whose concentration was higher in BA samples (24.43 ± 1.41 mg/g) than in DIBA samples (6.66 ± 0.25 mg/g; Log2FC = −1.87). These findings suggest that the presence of DI favors the breakdown of disaccharides into monosaccharides for further metabolism, potentially enhancing the efficiency of sugar degradation. Detailed metabolite information is provided in Supplementary Table S6.
Table 2. Concentrations of carbohydrate metabolites in naturally degraded bagasse (BA) and D. indusiata-degraded bagasse (DIBA) samples (n = 5).
Finally, we quantified the absolute concentrations of 68 energy metabolism–related metabolites in bagasse from the BA and DIBA treatments. As shown in Table 3, we detected 17 metabolites, including amino acid derivatives (1), amino acids (7), nucleotides and their metabolites (5), organic acids and their derivatives (2), and phosphate sugars (1). The three metabolites with the greatest increases in concentration in DIBA compared with BA were threonine (49.95 ± 26.88 ng/g in BA, 2191.56 ± 143.96 ng/g in DIBA; Log2FC = 5.46), glutamine (145.89 ± 81.04 ng/g in BA, 3025.07 ± 246.72 ng/g in DIBA; Log2FC = 5.46), and D(+)-glucose (19717.77 ± 2172.52 ng/g in BA, 383091.1 ± 21446.91 ng/g in DIBA; Log2FC = 4.28). Four additional metabolites—L-asparagine, L-alanine, serine, and oxaloacetate—were detected only in the DIBA samples. Overall, the concentrations of most energy-related metabolites were significantly higher in DIBA samples than in BA samples, indicating that the presence of DI was associated with much higher metabolic intensity during bagasse degradation.
Table 3. Energy metabolism–related metabolites in bagasse from the naturally degraded bagasse (BA) and D. indusiata-degraded bagasse (DIBA) treatments (n = 5).
Interplanting sugarcane with D. indusiata is an innovative cultivation method that can improve soil health and sugarcane yield [15; 16]. This study aimed to better understand the consequences of this process for bagasse degradation in order to promote the improvement of this circular agricultural practice. This study examined the degradation of sugarcane bagasse under two conditions: natural degradation (BA) and degradation in the presence of D. indusiata (DIBA). Our analyses revealed key chemical, microbial, and metabolite differences between these two treatments, which suggested that DIBA was more effective than BA in terms of bagasse degradation. These findings will be useful for the continued development of agricultural practices based on bagasse application.
Analysis of chemical composition revealed significant differences in ADF and crude protein content between the BA and DIBA treatments, although there were no significant differences in other components such as NDF, hemicellulose, cellulose, and lignin. This result suggests that although DIBA and BA produce similar overall changes in the major structural components of bagasse, DIBA may exert more subtle effects, particularly on protein content. In general, macrofungi are effective in degrading both the NDF and ADF of crop straw. For example, one study showed that use of the fungus Phanerochete chrysosporium for biological delignification during fermentation of sugarcane top fiber increased the NDF degradation rate by 54.21% and the ADF degradation rate by 53.06% (Yanti et al., 2021). In addition, related studies have shown that white rot fungi such as Phanerochaete chrysosporium, Irpex lacteus, and Phlebia acerina and edible fungi such as Pleurotus ostreatus, Pleurotus eryngii, and Lentinula edodes can effectively degrade ADF and NDF in straw materials from oats and corn (Zheng et al., 2021; Atalar and Çetinkaya, 2020). Although there was no significant difference in NDF percentage between DIAB and BA samples in our study, the average percentages of both hemicellulose and lignin were lower in DIAB than in BA (Figure 1), consistent with previous reports that macrofungi are effective at degrading ADF and NDF. In addition, the significantly lower crude protein content in DIBA samples suggested that DIBA treatment stimulated the breakdown of proteins, potentially increasing the availability of nitrogen in the soil, as protein is the main source of organic nitrogen in most ecosystems (Jan et al., 2009). In an earlier report, we showed that intercropping with D. indusiata reduced nitrogen loss from the soil (Duan et al., 2023). Combining these results, we speculate that increased decomposition of crude protein may be an important aspect of the process by which the DIBA treatment promotes soil health.
Metabarcoding sequencing revealed significant differences in the bacterial and fungal communities of bagasse from the BA and DIBA treatments. DIBA significantly altered microbial diversity, leading to reduced bacterial and fungal alpha diversity compared with BA. This reduction in diversity, particularly the lower Shannon indices, suggests that D. indusiata cultivation can reduce microbial diversity during bagasse degradation. Our previous research also found that the diversity of soil and sugarcane root-associated bacteria and fungi decreased when D. indusiata was intercropped with sugarcane, consistent with the results of this study. We speculate that this may be because D. indusiata, as a dominant fungus, synthesizes antimicrobial metabolites that inhibit the growth of other microorganisms (Huang et al., 2011; Habtemariam, 2019). Notably, the increased abundance of Proteobacteria and Acidobacteria in DIBA, together with the enrichment of Basidiomycota fungi, suggests that DIBA may promote specific microbial taxa that could facilitate the breakdown of complex bagasse materials. Interestingly, our previous study on the diversity of endophytic bacteria in sugarcane roots also found that D. indusiata forms a symbiotic relationship with the roots that selectively cultivates specific bacteria (e.g., Bacillus spp.) (Duan et al., 2024), again consistent with our current findings. In the fungal community, the increase in Phallaceae and the dominance of Basidiomycota in DIBA underscore the importance of these fungal taxa in lignocellulose degradation. Because Basidiomycota are known for their lignin-degrading capabilities (Lundell et al., 2010), this taxonomic shift in DIBA may have contributed to more efficient degradation of lignocellulosic materials, consistent with the observed higher glucose concentrations in DIBA samples. Given the potential antimicrobial properties of D. indusiata, which study had reported that the water extract of D. indusiate can inhibit the growth of bacteria and fungi (Habtemariam, 2019), we hypothesize that D. indusiata may facilitate the degradation of bagasse by enriching the abundance of specific bacterial and fungal taxa.
Metabolomic analysis suggested that DIBA significantly enhanced biological metabolism, particularly the breakdown of carbohydrates. This was evident from the higher concentrations of key metabolites such as glucose, cellobiose, and D-mannose in DIBA compared with BA. The much higher concentration of glucose in DIBA samples (510.04 pg./g vs. 27.61 pg./g in BA) strongly suggests that the presence of D. indusiata promotes the enzymatic hydrolysis of polysaccharides into simpler sugars, which are then metabolized by the microbial community. The metabolism of large fungi typically demands a substantial amount of energy from their environment, resulting in significant activity of carbohydrate-related metabolism. Our previous research also found that carbohydrate metabolism in the soil increased significantly during intercropping of D. indusiata with sugarcane (Duan et al., 2023). A study of the interactions between fairy ring fungi and soil also revealed that large fungi promoted a significant increase in soil carbohydrate metabolites (Duan et al., 2022). In addition, the significantly higher concentrations of carbohydrate metabolites in DIBA samples, particularly monosaccharides like D-galactose and cellobiose, support the notion that the DIBA treatment is more effective at breaking down complex carbohydrates into simpler, readily metabolizable forms. This enhanced carbohydrate degradation is further supported by the higher abundance of cellulolytic functional modules in DIBA, as indicated by the FAPROTAX and FunGuild annotations.
Through quantitative metabolomic analysis of carbohydrate and energy metabolites, we identified several metabolites that were unique to the DIBA treatment, including D-mannose, L-alanine, serine, and oxaloacetate. D-mannose is a naturally occurring bioactive monosaccharide, a C-2 epimer of glucose, and a component of various polysaccharides in plants (Wang et al., 2022). L-alanine and serine are key components for protein synthesis, and oxaloacetate is an intermediate in the tricarboxylic acid cycle, which plays important roles in regulating mitochondrial function, gluconeogenesis, the urea cycle, and amino acid synthesis (Yu and Sivitz, 2020). The presence of these unique monosaccharides and amino acid metabolites in DIBA also suggests that DIBA has an enhanced capacity to degrade bagasse compared with BA.
This study provides evidence that the cultivation of D. indusiata alongside sugarcane enhances the degradation of bagasse, suggesting that such intercropping is an effective method for improving soil health and promoting sustainable agriculture. DIBA not only altered the microbial community but also appeared to promote the breakdown of complex carbohydrates, leading to a higher abundance of metabolites essential for soil fertility. These findings suggest that the use of macrofungi like D. indusiata could help to advance circular agriculture by converting agricultural waste into valuable soil amendments. Future research should explore the long-term effects of DIBA on soil quality and crop yield, as well as the underlying mechanisms responsible for its selective enrichment of microbial taxa. Understanding these processes could lead to further optimization of intercropping systems and to the more widespread use of fungi in agricultural waste management.
The original contributions presented in the study are included in the article/Supplementary material, further inquiries can be directed to the corresponding authors.
MD: Conceptualization, Formal analysis, Investigation, Methodology, Software, Visualization, Writing – original draft, Writing – review & editing. XW: Formal analysis, Funding acquisition, Investigation, Visualization, Writing – original draft, Writing – review & editing. SL: Investigation, Writing – review & editing. HH: Investigation, Writing – review & editing. XL: Investigation, Writing – review & editing. YL: Investigation, Writing – review & editing. CL: Investigation, Writing – review & editing. BF: Investigation, Writing – review & editing. JC: Investigation, Writing – review & editing. DZ: Investigation, Writing – review & editing. ZC: Conceptualization, Investigation, Resources, Supervision, Writing – review & editing. ZW: Conceptualization, Funding acquisition, Investigation, Supervision, Writing – review & editing.
The author(s) declare that financial support was received for the research, authorship, and/or publication of this article. This work was jointly funded by the National Natural Science Foundation of China [grant no. NNSFC 32260715]; Central Government Guides Local Funds for Science and Technology Development [grant no. GuiKe ZY21195033]; Guangxi Major Science and Technology Project [grant no. GuiKe AA22117004]; Guangxi Academy of Agricultural Sciences Fund (Guinongke 2025YP065, GuiNongKeMeng 202403–1-5, GXAAS-WCJT 2024SC01, GXGHTY2023SC01); Chinese Academy of Sciences Foresight Strategic Science and Technology Project (XDA0450302); Guangxi Special Crop Experimental Station (TS202223); Special Project of Basic Scientific Research Business of Guangxi Academy of Agricultural Sciences (Guinongke 2021JM90).
We thank TopEdit (www.topeditsci.com) for linguistic assistance during the preparation of this manuscript.
The authors declare that the research was conducted in the absence of any commercial or financial relationships that could be construed as a potential conflict of interest.
The author(s) declare that no Gen AI was used in the creation of this manuscript.
All claims expressed in this article are solely those of the authors and do not necessarily represent those of their affiliated organizations, or those of the publisher, the editors and the reviewers. Any product that may be evaluated in this article, or claim that may be made by its manufacturer, is not guaranteed or endorsed by the publisher.
The Supplementary material for this article can be found online at: https://www.frontiersin.org/articles/10.3389/fmicb.2025.1510904/full#supplementary-material
1. ^version 2.5.3; http://CRAN.R-project.org/package=vegan; 2022.11.9
Ahmed, P. M., Soro, M. R., de Figueroa, L. I., and Pajot, H. F. (2018). Agro-industrial wastewaters bioremediation by Ligninolytic macrofungi, strategies for bioremediation of organic and inorganic pollutants. Boca Raton, FL: CRC Press, 1–28.
Al-Dhabi, N. A., Arasu, M. V., Lekshmi, R., Ignacimuthu, S., Rajakrishnan, R., Duraipandiyan, V., et al. (2021). Potential biotechnological applications of enzymes from macrofungi, advances in macrofungi. Boca Raton, FL: CRC Press, 177–197.
Atalar, A., and Çetinkaya, N. (2020). Treatment of corn straw with Pleurotus ostreatus, Pleurotus eryngii and Lentinula edodes to improve the digestibility of the lignocellulosic complex. J. Anatol. Environ. Anim. Sci. 5, 765–771. doi: 10.35229/jaes.812010
Bento, L. R., Castro, A. J. R., Moreira, A. B., Ferreira, O. P., Bisinoti, M. C., and Melo, C. A. (2019). Release of nutrients and organic carbon in different soil types from hydrochar obtained using sugarcane bagasse and vinasse. Geoderma 334, 24–32. doi: 10.1016/j.geoderma.2018.07.034
Bhadha, J. H., Xu, N., Khatiwada, R., Swanson, S., and LaBorde, C. (2020). Bagasse: a potential organic soil amendment used in sugarcane production: SL477/SS690, 8/2020. EDIS 2020:5. doi: 10.32473/edis-ss690-2020
Caporaso, J. G., Kuczynski, J., Stombaugh, J., Bittinger, K., Bushman, F. D., Costello, E. K., et al. (2010). QIIME allows analysis of high-throughput community sequencing data. Nat. Methods 7, 335–336. doi: 10.1038/nmeth.f.303
Chen, H., and Boutros, P. C. (2011). Venn diagram: a package for the generation of highly-customizable Venn and Euler diagrams in R. BMC Bioinformatics 12:35. doi: 10.1186/1471-2105-12-35
Cui, M., Zeng, L., Qin, W., and Feng, J. (2020). Measures for reducing nitrate leaching in orchards: a review. Environ. Pollut. 263:114553. doi: 10.1016/j.envpol.2020.114553
Duan, M., and Bau, T. (2021a). Initial sample processing can influence the soil microbial metabarcoding surveys, revealed by Leucocalocybe mongolica fairy ring ecosystem. Biotechnol. Biotechnol. Equip. 35, 1427–1438. doi: 10.1080/13102818.2021.1996272
Duan, M., and Bau, T. (2021b). Grassland fairy rings of Leucocalocybe mongolica represent the center of a rich soil microbial community. Braz. J. Microbiol. 52, 1357–1369. doi: 10.1007/s42770-021-00478-3
Duan, M., Li, X., Wu, X., Long, S., Huang, H., Li, Y., et al. (2024). Dictyophora indusiata and Bacillus aryabhattai improve sugarcane yield by endogenously associating with the root and regulating flavonoid metabolism. Front. Plant Sci. 15:1326917. doi: 10.3389/fpls.2024.1326917
Duan, M., Li, Y., Zhu, G., Wu, X., Huang, H., Qin, J., et al. (2023). Soil chemistry, metabarcoding, and metabolome analyses reveal that a sugarcane—Dictyophora indusiata intercropping system can enhance soil health by reducing soil nitrogen loss. Front. Microbiol. 14:990. doi: 10.3389/fmicb.2023.1193990
Duan, M., Long, Y., Fan, H., Ma, L., Han, S., Li, S., et al. (2022). Fenlong-ridging promotes microbial activity in sugarcane: a soil and root Metabarcoding survey. Agriculture 12:244. doi: 10.3390/agriculture12020244
Duan, M., Long, S., Wu, X., Feng, B., Qin, S., Li, Y., et al. (2023). Genome, transcriptome, and metabolome analyses provide new insights into the resource development in an edible fungus Dictyophora indusiata. Front. Microbiol. 14:1137159. doi: 10.3389/fmicb.2023.1137159
Duan, M., Lu, M., Lu, J., Yang, W., Li, B., Ma, L., et al. (2022). Soil chemical properties, metabolome, and Metabarcoding give the new insights into the soil transforming process of fairy ring Fungi Leucocalocybe mongolica. J. Fungi 8:680. doi: 10.3390/jof8070680
Duan, M., Lu, J., Yang, W., Lu, M., Wang, J., Li, S., et al. (2022). Metabarcoding and metabolome analyses reveal mechanisms of Leymus chinensis growth promotion by fairy ring of Leucocalocybe mongolica. J. Fungi 8:944. doi: 10.3390/jof8090944
Duan, M., Yang, C., Bao, L., Han, D., Wang, H., Zhang, Y., et al. (2023). Morchella esculenta cultivation in fallow paddy fields and drylands affects the diversity of soil bacteria and soil chemical properties. Front. Genet. 14:1251695. doi: 10.3389/fgene.2023.1251695
Guo, M., Wu, F., Hao, G., Qi, Q., Li, R., Li, N., et al. (2017). Bacillus subtilis improves immunity and disease resistance in rabbits. Front. Immunol. 8:354. doi: 10.3389/fimmu.2017.00354
Habtemariam, S. (2019). The chemistry, pharmacology and therapeutic potential of the edible mushroom Dictyophora indusiata (vent ex. Pers.) Fischer (Synn. Phallus indusiatus). Biomedicines 12:98. doi: 10.3390/biomedicines7040098
Huang, M., Chen, X., Tian, H., Sun, B., and Chen, H. (2011). Isolation and identification of antibiotic albaflavenone from Dictyophora indusiata (vent: Pers.) Fischer. J. Chem. Res. 35, 659–660. doi: 10.3184/174751911X13202334527264
Jan, M. T., Roberts, P., Tonheim, S. K., and Jones, D. L. (2009). Protein breakdown represents a major bottleneck in nitrogen cycling in grassland soils. Soil Biol. Biochem. 41, 2272–2282. doi: 10.1016/j.soilbio.2009.08.013
Kong, D., Fang, P., Wang, H., Chen, X., Yue, Y., Lv, F., et al. (2017). Thermal properties and conditioning temperature control of formula feeds containing high content of whey powder for weanling pigs. Trans. Chin. Soc. Agric. Eng. 33, 299–307. doi: 10.11975/j.issn.1002-6819.2017.16.039
Laca, A., Laca, A., and Díaz, M. (2019). “Hydrolysis: from cellulose and hemicellulose to simple sugars” in Second and third generation of feedstocks. eds. A. Basile and F. Dalena (Amsterdam, Netherlands: Elsevier), 213–240.
Li, S., Lei, Y., Zhang, Y., Liu, J., Shi, X., Jia, H., et al. (2019). Rational trade-offs between yield increase and fertilizer inputs are essential for sustainable intensification: a case study in wheat-maize cropping systems in China. Sci. Total Environ. 679, 328–336. doi: 10.1016/j.scitotenv.2019.05.085
Liu, H., Li, D., Huang, Y., Lin, Q., Huang, L., Cheng, S., et al. (2023). Addition of bacterial consortium produced high-quality sugarcane bagasse compost as an environmental-friendly fertilizer: optimizing arecanut (Areca catechu L.) production, soil fertility and microbial community structure. Appl. Soil Ecol. 188:104920. doi: 10.1016/j.apsoil.2023.104920
Louca, S., Parfrey, L. W., and Doebeli, M. (2016). Decoupling function and taxonomy in the global ocean microbiome. Science 353, 1272–1277. doi: 10.1126/science.aaf4507
Lu, H., Lou, H., Hu, J., Liu, Z., and Chen, Q. (2020). Macrofungi: a review of cultivation strategies, bioactivity, and application of mushrooms. Compr. Rev. Food Sci. Food Saf. 19, 2333–2356. doi: 10.1111/1541-4337.12602
Lundell, T. K., Mäkelä, M. R., and Hildén, K. (2010). Lignin-modifying enzymes in filamentous basidiomycetes – ecological, functional and phylogenetic review. J. Basic Microbiol. 50, 5–20. doi: 10.1002/jobm.200900338
Lynd Lee, R., Weimer Paul, J., van Zyl Willem, H., and Pretorius Isak, S. (2002). Microbial cellulose utilization: fundamentals and biotechnology. Microbiol. Mol. Biol. Rev. 66, 506–577. doi: 10.1128/MMBR.66.3.506-577.2002
Mohamed, I., Ali, M., Ahmed, N., and Chen, F. (2019). Cadmium immobilization and alleviation of its toxicity for soybean grown in a clay loam contaminated soil using sugarcane bagasse-derived biochar. Environ. Sci. Pollut. Res. 26, 21849–21857. doi: 10.1007/s11356-019-05501-7
Nguyen, N. H., Song, Z., Bates, S. T., Branco, S., Tedersoo, L., Menke, J., et al. (2016). FUNGuild: an open annotation tool for parsing fungal community datasets by ecological guild. Fungal Ecol. 20, 241–248. doi: 10.1016/j.funeco.2015.06.006
Nilsson, R. H., Larsson, K. H., Taylor, A. F. S., Bengtsson-Palme, J., Jeppesen, T. S., Schigel, D., et al. (2019). The UNITE database for molecular identification of fungi: handling dark taxa and parallel taxonomic classifications. Nucleic Acids Res. 47, D259–d264. doi: 10.1093/nar/gky1022
Pruesse, E., Quast, C., Knittel, K., Fuchs, B. M., Ludwig, W., Peplies, J., et al. (2007). SILVA: a comprehensive online resource for quality checked and aligned ribosomal RNA sequence data compatible with ARB. Nucleic Acids Res. 35, 7188–7196. doi: 10.1093/nar/gkm864
Saha, B. C. (2003). Hemicellulose bioconversion. J. Ind. Microbiol. Biotechnol. 30, 279–291. doi: 10.1007/s10295-003-0049-x
Seleiman, M. F., and Kheir, A. M. S. (2018). Saline soil properties, quality and productivity of wheat grown with bagasse ash and thiourea in different climatic zones. Chemosphere 193, 538–546. doi: 10.1016/j.chemosphere.2017.11.053
Shang, D., Gu, W., Zhai, Y., Ning, J., Mao, X., Sheng, X., et al. (2022). Solid sampling pyrolysis adsorption-desorption thermal conductivity method for rapid and simultaneous detection of N and S in seafood. Molecules 27:909. doi: 10.3390/molecules27248909
Sharma, S., Thind, H. S., Singh, Y., Singh, V., and Singh, B. (2015). Soil enzyme activities with biomass ashes and phosphorus fertilization to rice–wheat cropping system in the indo-Gangetic plains of India. Nutr. Cycl. Agroecosyst. 101, 391–400. doi: 10.1007/s10705-015-9684-7
Tong, L., Li, H., Liu, X., Li, B., Chen, L., Chen, G., et al. (2021). Effects of continuous cropping of Dictyophora on soil physical and chemical properties, microbial biomass and enzyme activity. J. Innov. Soc. Sci. Res. 8:6890. doi: 10.53469/jissr.2021.08(07).39
Ullah, Z., Ali, S., Muhammad, N., Khan, N., Rizwan, M., Khan, M. D., et al. (2020). Biochar impact on microbial population and elemental composition of red soil. Arab. J. Geosci. 13:757. doi: 10.1007/s12517-020-05671-6
Vitousek, P. M., Naylor, R., Crews, T., David, M. B., Drinkwater, L. E., Holland, E., et al. (2009). Agriculture. Nutrient imbalances in agricultural development. Science 324, 1519–1520. doi: 10.1126/science.1170261
Wang, Q., Garrity, G. M., Tiedje, J. M., and Cole, J. R. (2007). Naive Bayesian classifier for rapid assignment of rRNA sequences into the new bacterial taxonomy. Appl. Environ. Microbiol. 73, 5261–5267. doi: 10.1128/AEM.00062-07
Wang, J., Wen, X., Zhang, Y., Zou, P., Cheng, L., Gan, R., et al. (2021). Quantitative proteomic and metabolomic analysis of Dictyophora indusiata fruiting bodies during post-harvest morphological development. Food Chem. 339:127884. doi: 10.1016/j.foodchem.2020.127884
Wang, P., Zheng, Y., Li, Y., Shen, J., Dan, M., and Wang, D. (2022). Recent advances in biotransformation, extraction and green production of D-mannose. Curr. Res. Food Sci. 5, 49–56. doi: 10.1016/j.crfs.2021.12.002
Wilson, D. B. (2011). Microbial diversity of cellulose hydrolysis. Curr. Opin. Microbiol. 14, 259–263. doi: 10.1016/j.mib.2011.04.004
Xu, Q., Ling, N., Chen, H., Duan, Y., Wang, S., Shen, Q., et al. (2020). Long-term chemical-only fertilization induces a diversity decline and deep selection on the soil Bacteria. Korean J. Agric. Sci. 5:337. doi: 10.1128/mSystems.00337-20
Yanti, G., Jamarun, N., Elihasridas, E., and Astuti, T. (2021). Quality improvement of sugarcane top as animal feed with biodelignification by Phanerochaete chrysosporium Fungi on in-vitro digestibility of NDF, ADF, cellulose and hemicellulose. J. Phys. Conf. Ser. 1940:012063. doi: 10.1088/1742-6596/1940/1/012063
Yu, L., and Sivitz, W. I. (2020). Oxaloacetate mediates mitochondrial metabolism and function. Curr. Metabol. Syst. Biol. Form. 7, 11–23. doi: 10.2174/2213235X07666191008103247
Zhang, H., Li, Y., and Zhu, J. K. (2018). Developing naturally stress-resistant crops for a sustainable agriculture. Nat. Plants 4, 989–996. doi: 10.1038/s41477-018-0309-4
Keywords: sugarcane, bagasse, Dictyophora indusiata , metabarcoding, metabolomics
Citation: Duan M, Wu X, Long S, Huang H, Li X, Li Y, Li C, Feng B, Chen J, Zhong D, Chen Z and Wang Z (2025) Intercropping of Saccharum spp. with Dictyophora indusiata: effects on microbial communities and metabolite profiles during bagasse degradation. Front. Microbiol. 16:1510904. doi: 10.3389/fmicb.2025.1510904
Received: 15 October 2024; Accepted: 07 January 2025;
Published: 22 January 2025.
Edited by:
Tanveer Ahmad, Muhammad Nawaz Shareef University of Agriculture, PakistanReviewed by:
Muhammad Imtiaz, National Institute for Biotechnology and Genetic Engineering, PakistanCopyright © 2025 Duan, Wu, Long, Huang, Li, Li, Li, Feng, Chen, Zhong, Chen and Wang. This is an open-access article distributed under the terms of the Creative Commons Attribution License (CC BY). The use, distribution or reproduction in other forums is permitted, provided the original author(s) and the copyright owner(s) are credited and that the original publication in this journal is cited, in accordance with accepted academic practice. No use, distribution or reproduction is permitted which does not comply with these terms.
*Correspondence: Zhendong Chen, Y3pkMzgwOEBneGFhcy5uZXQ=; Zeping Wang, eWFoZW5nODMwNjE5QDE2My5jb20=
†These authors have contributed equally to this work
Disclaimer: All claims expressed in this article are solely those of the authors and do not necessarily represent those of their affiliated organizations, or those of the publisher, the editors and the reviewers. Any product that may be evaluated in this article or claim that may be made by its manufacturer is not guaranteed or endorsed by the publisher.
Research integrity at Frontiers
Learn more about the work of our research integrity team to safeguard the quality of each article we publish.