- 1Department of Biological Sciences, University of Bergen, Bergen, Norway
- 2Département Médicaments et Technologies pour la Santé (DMTS), Université Paris Saclay, CEA, INRAE, Bagnols-sur-Cèze, France
Keratin, one of the most recalcitrant and abundant proteins on Earth, constitutes a challenging and underutilized material for the poultry industry. Although it resists degradation by most commonly available enzymes, natural breakdown occurs through the action of certain fungi and bacteria. This process remains poorly understood, and only a few thermophilic and anaerobic bacteria are known to effectively degrade keratin. Some members of the genus Fervidobacterium have been demonstrated to be effective at degrading feather keratin under high temperatures and anoxic conditions. However, a comprehensive evaluation of their keratinolytic capabilities remains lacking, leaving their potential largely underexplored. In this study, we assessed the keratinolytic activity of all available Fervidobacterium strains. Six strains were active against this recalcitrant substrate, namely Fervidobacterium changbaicum CBS-1T, Fervidobacterium islandicum H-21T, Fervidobacterium pennivorans T, Fervidobacterium pennivorans DSM9078T, Fervidobacterium sp. GSH, and Fervidobacterium sp. 21710. These bacteria were used in a comparative proteomics analysis, grown with either glucose or chicken feathers as the sole carbon source. Similarly, the three most efficient strains, Fervidobacterium pennivorans T, Fervidobacterium sp. GSH, and Fervidobacterium islandicum H-21T underwent an in-depth comparative transcriptomics analysis. Among the numerous upregulated proteins and overexpressed genes identified when comparing feather-grown to glucose–grown cells, oxidoreductases and peptidases are key enzymes in the degradation process, suggesting their potential application in enzymatic keratinolytic cocktails for degrading feather keratin.
Introduction
Keratin, a structural protein found in the epidermis and outer protective layers of vertebrates, provides protection, insulation, and other mechanical functions. Beta-keratin, one of the main forms of keratin, is the principal component of several structures, such as scales, beaks, and feathers (Zhang and Fan, 2021). Chicken feather keratin is rich in cysteine, glutamine, proline, and serine, and its composition is similar to that of other feather keratins (Saravanan and Dhurai, 2012). Thus, keratin is one of the most abundant proteins on Earth, with millions of tons of feathers produced annually as a by-product of the food industry (Shavandi et al., 2017; Chen et al., 2022), indicating both its prevalence and persistence in the environment. Characterized by a high cysteine content, typically ranging from 7 to 13%, the structure of keratin is strengthened by hydrogen and disulfide bonds, making it chemically stable (Lange et al., 2016; Shavandi et al., 2017) and resistant to most conventional hydrolytic enzymes commonly used for protein degradation (Sypka et al., 2021; Chen et al., 2022).
Considered a biowaste, feathers are a major concern for the food industry and constitute an underutilized residue that is traditionally transformed into low-value products, such as feather meal or fertilizers (De Oliveira Martinez et al., 2020), or even burned or discarded (Sahoo et al., 2017; Chen et al., 2022). Conventional keratin extraction methods typically disrupt the structure of wool or feathers, leading to alterations in the composition and generation of pollutants (Shavandi et al., 2017). Although keratin degradation occurs in nature, this reaction is slow, and the details of the degradation process are still to be fully elucidated at molecular level (Daroit and Brandelli, 2014; Sahoo et al., 2017). Keratin degradation has been hypothesized to involve the cleavage of disulfide bonds by oxidoreductases, followed by the combined action of endo-and exo-proteases and other enzymes. However, the details of the process at molecular level remain unknown (Lange et al., 2016; Shavandi et al., 2017; Qiu et al., 2020).
Certain groups of microorganisms, most of which are mesophilic aerobes, have been reported to break down feather keratin (Daroit and Brandelli, 2014; Sahni et al., 2015; Srivastava et al., 2020). Interestingly, only a few are anaerobic and thermophilic or hyperthermophilic, such as strains of the genus Fervidobacterium, belonging to the Thermotogota phylum. All members of this taxon have an external sheath-like membrane called the toga, which is a defining characteristic of this phylum (Huber et al., 1986; Bhandari and Gupta, 2014). All of them are thermophilic or hyperthermophilic and fermentative rods with optimal temperatures range of 65–80°C (Huber et al., 1990; Andrews and Patel, 1996; Friedrich and Antranikian, 1996; Cai et al., 2007; Podosokorskaya et al., 2011; Kanoksilapatham et al., 2016) that use various sugars and proteinaceous substrates as carbon and energy sources (Conners et al., 2006). Several strains of the Fervidobacterium genus can degrade feather keratin at high temperatures under anaerobic conditions, meaning that their enzymatic machinery is accordingly adapted to function in these conditions, and highlighting their biological and biotechnological relevance (Huber et al., 1990; Friedrich and Antranikian, 1996; Kanoksilapatham et al., 2016; Javier-Lopez et al., 2022; Wang et al., 2024). Moreover, degradation of recalcitrant compounds is more efficient at high temperatures, what makes thermophiles more attractive compared to their mesophilic counterparts (Cowan et al., 2024), explaining why thermophilic enzymes are widely used in industry (Atif et al., 2024). Their genomes are approximately two megabases in size, with a G + C content ranging from 32 to 46% mol (Javier-Lopez et al., 2022). Despite the discovery and characterization of a few keratinases (Kluskens et al., 2002; Kim et al., 2004; Godde et al., 2005; Lee et al., 2015), the feather-degrading potential of this group remains largely underexplored. In this context, the metabolic versatility of the Fervidobacterium group, their thermophilic features, and the increasing availability of thermostable proteases for the degradation of proteinaceous biowaste from agriculture and fisheries have huge potential for biotechnological and industrial applications.
Unraveling how biological systems function and elucidating key molecular mechanisms have become more attainable with the advent of genomics, transcriptomics, and proteomics (Armengaud, 2016). Next-generation proteomics can be applied to whole cells, as well as proteins secreted in the milieu (Armengaud et al., 2012), offering insights into enzymes and catalysts produced and exported by bacteria.
To the best of our knowledge, comparative proteomic and transcriptomic studies on Fervidobacterium representatives are lacking. To uncover novel enzymes involved in keratin degradation and gain insights into the associated metabolic pathways, the keratinolytic capabilities of all available Fervidobacterium strains were evaluated in this study, and the cellular and exo-proteomes of the most efficient strains grown with chicken feathers or glucose were analyzed. Finally, the transcriptomes of the three representative strains were established. This multiomics study provides a comprehensive overview of the functionality of this thermophilic bacterial genus and highlights its potential for keratin degradation.
Materials and methods
Experimental design and strains used in this work
All available isolates of the genus Fervidobacterium were included in this study: Fervidobacterium pennivorans T (CP050868) and Fervidobacterium sp. GSH (CP126982), both isolated and described by our research group in Bergen (Javier-Lopez et al., 2022); Fervidobacterium pennivorans DSM 9078T (CP003260), Fervidobacterium nodosum Rt17-B1T (CP000771), Fervidobacterium sp. DSM 13770 (CP126498), Fervidobacterium islandicum H-21T (CP126499), Fervidobacterium sp. DSM 21710 (CP126500), Fervidobacterium changbaicum CBS-1T (CP026721), Fervidobacterium riparium 1445tT (CP009277) and Fervidobacterium gondwanense DSM13020T (CP126501), acquired from the German Collection of Microorganisms and Cell Cultures (Leibniz Institute DSMZ)1; Fervidobacterium thailandense FC2004T (CP140110), obtained through the Japan Collection of Microorganisms (JCM).2
The keratinolytic efficiencies of these strains were assessed based on their ability to degrade chicken feather keratin under anaerobic and thermophilic conditions. The experimental procedure included inoculating each strain into flasks containing plain mineral medium in the presence of a chicken feather and incubating the cultures at their optimal temperature based on the DSMZ guidelines. The cultures were monitored for 72 h, during which the integrity of the feathers was visually inspected. The keratinolytic strains underwent proteomic analysis to identify and quantify the potential enzymes involved in keratin degradation. Finally, transcriptome profiles of the three most efficient degradative strains were compared.
Medium preparation and cultivation
The organisms used in this study were cultured using a uniform Mineral Medium for Freshwater bacteria (MMF). This medium consisted of an initial mineral formulation, trace elements, and vitamins supplemented with yeast extract, glucose, or a chicken feather as carbon sources. The mineral composition of MMF contained, per liter: NaCl, 1 g; MgSO4·7H2O, 0.3 g; KCl, 0.3 g; NH4Cl, 0.5 g; CaCl2·2H2O, 0.1 g; and KH2PO4, 0.3 g. Ten milliliters of the trace elements solution SL-10 (Koblitz et al., 2022) was added. The required amount of yeast extract was at least of 0.5 grams per liter (Friedrich and Antranikian, 1996). The mixture was sterilized by autoclaving at 121°C for 20 min. After cooling to 60°C, while flushing with sterile nitrogen gas, 10 mL of a vitamin solution was added. The composition of the vitamin solution was, per liter: 4-aminobenzoic acid, 8 mg; D(+) biotin, 2 mg; nicotinic acid, 20 mg; Ca-D(+) pantothenic acid, 10 mg; pyridoxamine·2HCl, 30 mg; thiamine dichloride, 20 mg; and vitamin B12, 10 mg. Furthermore, 2 mL of 25% cysteine-HCl solution was added as a reducing agent.
The pH was adjusted to 7.1 ± 0.1 with 1 M HCl, and the medium was transferred to sterile 20 mL serum flasks using the Hungate technique (Hungate, 1950; Miller and Wolin, 1974). Each flask was capped with butyl rubber corks and secured with aluminum seals. Glucose was added as a carbon source to a final concentration of 5 g/L from a sterile anaerobic stock using a syringe.
Feather degradation assessment
To assess the keratinolytic efficiency of the organisms, the bacteria were incubated with MMF medium enriched with 0.5 g/L yeast extract and native chicken feathers (15 ± 5 mg). The feathers were washed with a solution of ethanol:methanol (1:1) to eliminate lipids, feces and other organic debris, and autoclaved (121°C, 20 min.), as previously described (Javier-Lopez et al., 2022).
The cultures were incubated at the optimal temperature for each strain, as recommended by the German Collection of Microorganisms and Cell Cultures (Leibniz Institute, DSMZ). These were: F. changbaicum CBS-1T (80°C), F. islandicum H-21T (65°C), F. pennivorans T (65°C), F. pennivorans DSM 9078T (65°C), F. sp. GSH (65°C), F. sp. 21710 (70°C), F. riparium 1445T (65°C), F. thailandense FC2004T (80°C), F. sp. 13770 (65°C), F. nodosum Rt17-B1T (70°C), F. gondwanense DSM 13020T (65°C).
Feather degradation assay
Although there is no standardized procedure for assessing keratin degradation (Qiu et al., 2020), one of the most reliable and straightforward methods is to calculate the difference in the weight of the substrate before and after incubation (De Oliveira Martinez et al., 2020). Accordingly, a quantitative feather degradation assay was designed to measure and compare the keratinolytic activity of the three most efficient strains, F. pennivorans T, Fervidobacterium sp. GSH and F. islandicum H-21T. The cultures were incubated for 72 h at the optimal temperature for each microorganism: 70°C for F. pennivorans T and Fervidobacterium sp. GSH and 65°C for F. islandicum H-21T. The experiment was performed in triplicate using chicken breast feathers as substrates. The feathers were weighed and placed aseptically in serum flasks that were previously gassed with sterile nitrogen. Next, 20 mL of sterile MMF medium enriched with yeast extract (0.5 g/L) was added to each flask and inoculated with 1 mL of a dense bacterial culture of the respective strains. After 0 (initial time), 12, 24, 36, 48, 60 and 72 h the cultures were aseptically filtered through a 5 mm pore Whatman syringe filter. The filter was washed with 70% ethanol to remove any residual media or biological material and dried at 65°C to a constant weight.
The weight of the remaining feathers was recorded, and the efficiency of feather degradation was calculated by comparing the final weight to the initial value.
Genome annotations
Both proteomes and transcriptomes were mapped to the annotated genomes of the previously mentioned species. The Prokaryotic Genome Annotation Pipeline (PGAP) version 2023-05-17. build6771 (Tatusova et al., 2016)3 was used to predict genes and other features in the genomes of Fervidobacterium sp. GSH (CP126982) and F. islandicum H-21T (CP126499). The genome annotation of F. pennivorans T (CP050868) already available in Genbank was used.
Proteomics
Sample preparation
The bacteria were inoculated into flasks containing 15 mL of sterile mineral MMF medium with 0.5% glucose or a chicken feather. Biological triplicates were then incubated for 24 (glucose culture) or 48 h (feather culture) at the optimal temperature for each strain. The cells were harvested by centrifuging at 4°C for 10 min at 5,000 g. The pellets were weighted and mixed with 6 μL of Laemmli buffer per milligram of pellet, while the supernatants were filtered through 0.2 μm disk filters to eliminate any remaining cells. The filtered fraction was then concentrated using Amicon centrifugal filters (10 kDa cut-off) for 25 min at 5,000 g and 4°C. Then, 100 μL of this concentrated supernatant was transferred to a tube along with 100 μL of Laemmli buffer. Both pellets and concentrated supernatants mixed with Laemmli buffer were denatured by boiling at 99°C for 10 min.
For each sample, a volume of 20 μL of extract was subjected to electrophoresis on a NuPAGE 4–12% Bis-Tris (Invitrogen) gel for 5 min at 200 V in MES buffer (Invitrogen). The proteins were then stained with ready-to-use Coomassie SimplyBlue SafeStain (Thermo Fisher Scientific), destained with MilliQ water washes, excised as a single polyacrylamide band, treated, and proteolyzed with trypsin, as previously described (Rubiano-Labrador et al., 2014).
Tandem mass spectrometry and data interpretation
For each sample, the resulting tryptic peptides (15 out of 50 μL) were analyzed using tandem mass spectrometry with an Exploris 480 high-resolution tandem mass spectrometer (Thermo electron) coupled to a Vanquish Neo UHPLC in conditions similar to those previously described (Charlier et al., 2024). Briefly, peptides were desalted online with a PepMap 100 C18 pre-column and resolved on a reverse-phase Acclaim PepMap 100 C18 column (Thermo Fisher Scientific) at a flow rate of 250 nL/min with a 90 min gradient (5–25% B), followed by a 5 min gradient (25–40% B) with mobile phases A (0.1% HCOOH/100% H2O) and B (0.1% HCOOH/99.9%CH3CN). The mass spectrometer was operated in data-dependent acquisition mode with a Top20 strategy consisting of cycles of a full scan of peptide ions, followed by sequential selection of each of the 20 most intense precursors in the high-energy collisional dissociation cell, their fragmentation, and MS/MS scans of the resulting fragments. Only peptide ions with a charge state of 2+ or 3+ were selected for dissociation, with a dynamic exclusion of 10 s. Full-scan mass spectra from 350 to 1,500 m/z were acquired at a resolution of 120,000, whereas MS/MS scans were recorded at a resolution of 15,000. Peptide-to-spectrum assignment was performed with the Mascot software v2.5.1 (Matrix Science) against the annotated genome database of each specific strain.
Full-trypsin specificity with up to two missed cleavages allowed, fixed modification of carbamidomethylated cysteine, mass tolerances of 5 ppm for the precursors, and 0.02 Da for peptide fragments were selected as parameters. Methionine oxidation and asparagine and glutamine deamidation were selected as variable modifications. Peptide matches with a MASCOT peptide score below a p-value of 0.05 were considered. Proteins with at least two different peptides were selected, and their quantities were estimated using spectral counts. The false discovery rate for protein identification was <1%, as estimated using the MASCOT reverse decoy database option. Spectral counts were compared between conditions after standard normalization using the T-Fold method as previously described (Gouveia et al., 2020), selecting proteins that satisfied |T-fold| (≥1.5) and p-value (≤0.05) as significantly up-and downregulated. Volcano plots were drawn to visualize the results using the Ggplot2 (v 3.5.1) (Wickham, 2016) library in R.
Functional analyses
The upregulated proteins identified in the studied strains were submitted to the Kyoto Encyclopedia of Genes and Genomes (KEGG) (Kanehisa and Goto, 2000; Kanehisa, 2019; Kanehisa et al., 2022), annotated and KO codes assigned with BlastKOALA and their global metabolism and pathways were identified, analyzed and compared using the Reconstruct tool in KEGG Mapper.
Transcriptomics
RNA isolation and purification
Fervidobacterium pennivorans T, Fervidobacterium sp. GSH and F. islandicum H-21T were grown in triplicate with either glucose (0.5%) or native chicken breast feathers. After 18 (glucose and feather samples) or 40 h (feather cultures), the cells were harvested via centrifugation at 4°C for 10 min at 5,000 g, and total RNA was purified using the protocol described in the RNeasy Mini Kit from Qiagen. The RNA concentration was measured using a NanoDrop™ One/OneC spectrophotometer, and RNA integrity assessed using an Agilent 2,100 Bioanalyzer System (Agilent Technologies, California, USA) based on the calculation of the RNA integrity number (RIN). RNA samples were stored at −80°C until further analysis.
Complementary (cDNA) synthesis, sequencing and assembly
The rRNA was depleted, and cDNA was synthesized and sequenced using Illumina technologies at Eurofins Genomics facilities, Constance, Baden, Germany.4 Low-quality reads (PHRED score < 30) and adapters were trimmed using CLC Workbench Genomics v23.0.5.5 The filtered reads were assembled and mapped to the annotated genomes of F. pennivorans T, Fervidobacterium sp. GSH and F. islandicum H-21T with CLC Workbench Genomics v23.0.5, using the following parameters: mismatch cost = 2, insertion cost = 2, deletion cost = 3, length fraction = 0.8, and maximum number of hits per read = 10.
Differential gene expression
Gene count normalization and differential gene expression analyses were performed using the DESeq2 package (v1.43.1) in Bioconductor hosted in R (version 2023.09.0 + 463). The analysis was performed separately for each strain, comparing total gene expression levels between the glucose and feather cultures at 18 and 40 h of incubation. After applying a variance-stabilizing transformation (VST), genes with a |fold change| > 1.5 and a false discovery rate (FDR) < 0.05 were considered significantly over-and under-expressed, respectively. The significant genes were subset and the results visualized using heatmaps drawn with the R package pheatmap v1.0.12.6
Results
Feather degradation assessment
The keratinolytic potential of all 11 Fervidobacterium strains available was assessed using feather degradation tests. Temperature conditions were set individually for each strain according to their optimal growth temperature. The results in Table 1 were obtained after incubating the cultures for 72 h in MMF medium using chicken feathers as the sole carbon source. Among the strains tested, only F. nodosum Rt17-B1T and F. gondwanense DSM 13020T showed no visible signs of feather degradation. Fervidobacterium riparium 1445tT, Fervidobacterium sp. 13770, and Fervidobacterium thailandense FC2004T exhibited only partial feather degradation. The remaining six strains–F. changbaicum CBS-1T, F. islandicum H-21T, F. pennivorans T, F. pennivorans DSM 9078T, F. pennivorans GSH and Fervidobacterium sp. 21710–completely degraded feather within 72 h. F. pennivorans T, F. pennivorans GSH, and F. islandicum H-21T were particularly efficient in degrading most of the feathers after only 48 h and were thus selected for further investigation in degradation assays and transcriptomics. Examples of complete, partial and negative feather degradation are displayed in Supplementary Figure S1.
A quantitative degradation assay was performed to quantify and compare the keratinolytic activity of the three most efficient strains of fervidobacteria, namely F. pennivorans T, F. pennivorans GSH, and F. islandicum H-21T. Figure 1 shows the percentage of feather material remaining after 12, 24, 36, 48, 60 and 72 h of incubation. Feather weight loss was expressed as a percentage of the original weight. By the 12 h mark, there was almost no variation in weight loss, and the degradation became clearly noticeable after this time point. After 24 h, F. pennivorans T had broken down more than 30 ± 13% of the feather material, and F. pennivorans GSH had degraded approximately 20 ± 3%. The slowest strain at this time point was F. islandicum H-21T, with around 7 ± 1% degradation. At this time point, the degradation rate of all three strains significantly increased until 60 h, then slowed, except for F. pennivorans T, the most efficient strain, which reached a plateau after only 48 h. At 60 h, a stationary phase in feather degradation was reached for all three strains, but feather degradation could still be measured until the end of the experiment, at 72 h. At the conclusion of the assay, F. islandicum H-21T and F. pennivorans T had degraded most of the feather, with only 15 ± 7% and 17 ± 5% remaining, respectively. F. pennivorans GSH could only break down until 28 ± 2% of the feather after a 72-h incubation.
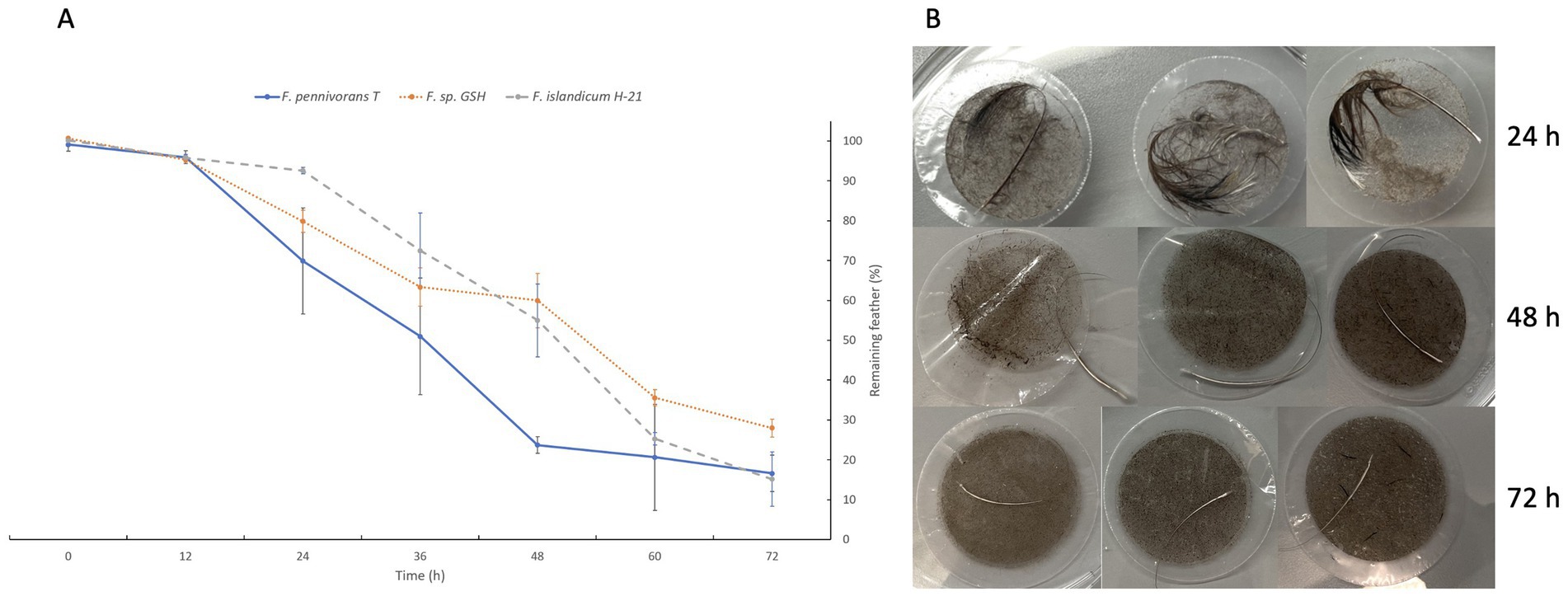
Figure 1. Quantitative feather degradation assay showing the difference in weight measured in percentage of remaining feathers after 12, 24, 36, 48, and 72 h of incubation. (A) Assay results for Fervidobacterium pennivorans T (blue), Fervidobacterium pennivorans GSH (orange), and Fervidobacterium islandicum H-21T (gray). (B) Feather degradation by F. pennivorans T after 24, 48, and 72 h of incubation, as revealed by filtering through a 5 mm-pore syringe filter (triplicates).
Shotgun proteomics
Differential analysis
The six most active keratinolytic fervidobacteria (F. changbaicum CBS-1T, F. islandicum H-21T, F. pennivorans T, F. pennivorans DSM 9078T, F. pennivorans GSH and Fervidobacterium sp. 21710) and F. gondwanense DSM 13020T as a non-degradative (negative control) strain were subjected to a dual shotgun proteomic study, in which the cellular proteome and exoproteome were established (a list of proteins with statistical analysis is available as a separate Excel file in File S2). More than 80% of the approximately 2,000 proteins detected in each of the seven proteomes were identified, most of which were identified in the cellular proteome, with more than 1,250 proteins identified per strain (Table 2), whereas only approximately 200 proteins on average belonged to the exoproteome fraction. Approximately 160 proteins were found upregulated in the feather cultures of all strains, most of which were in the cellular fraction. The number of downregulated proteins identified was notably higher, with more than 200 proteins in each strain, except for Fervidobacterium sp. 21710, which had only 151 downregulated proteins. Surprisingly, the non-keratinolytic bacterium F. gondwanense DSM13020T showed the highest number of upregulated proteins, with a total of 76 proteins identified.
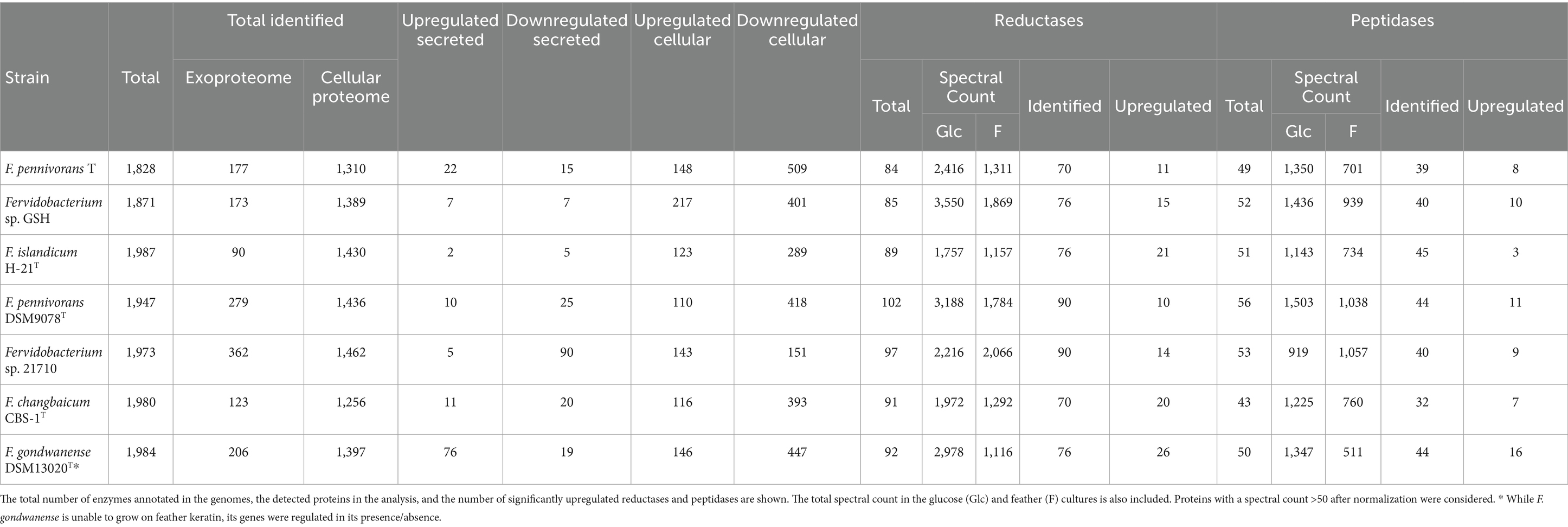
Table 2. Proteomics overview of the number of proteins detected with at least 2 peptides and numbers of significantly up-and downregulated proteins.
Differential analysis is shown in Figure 2 in the form of volcano plots, where protein abundances were compared between feather and glucose conditions. Proteins were classified as upregulated or downregulated based on a |fold change| threshold of 1.5 and a significance level (p-value) below 0.05. Across all strains, the statistically underrepresented proteome was more abundant, indicating that the proteome of cells grown in feather conditions was less diverse and, thus, more specialized than the proteome of cells grown on glucose. For most strains, the number of significantly downregulated proteins was between two and three times more abundant in the feather condition than in the glucose condition, except for Fervidobacterium sp. 21710, which had almost the same number of differential proteins in both fractions.
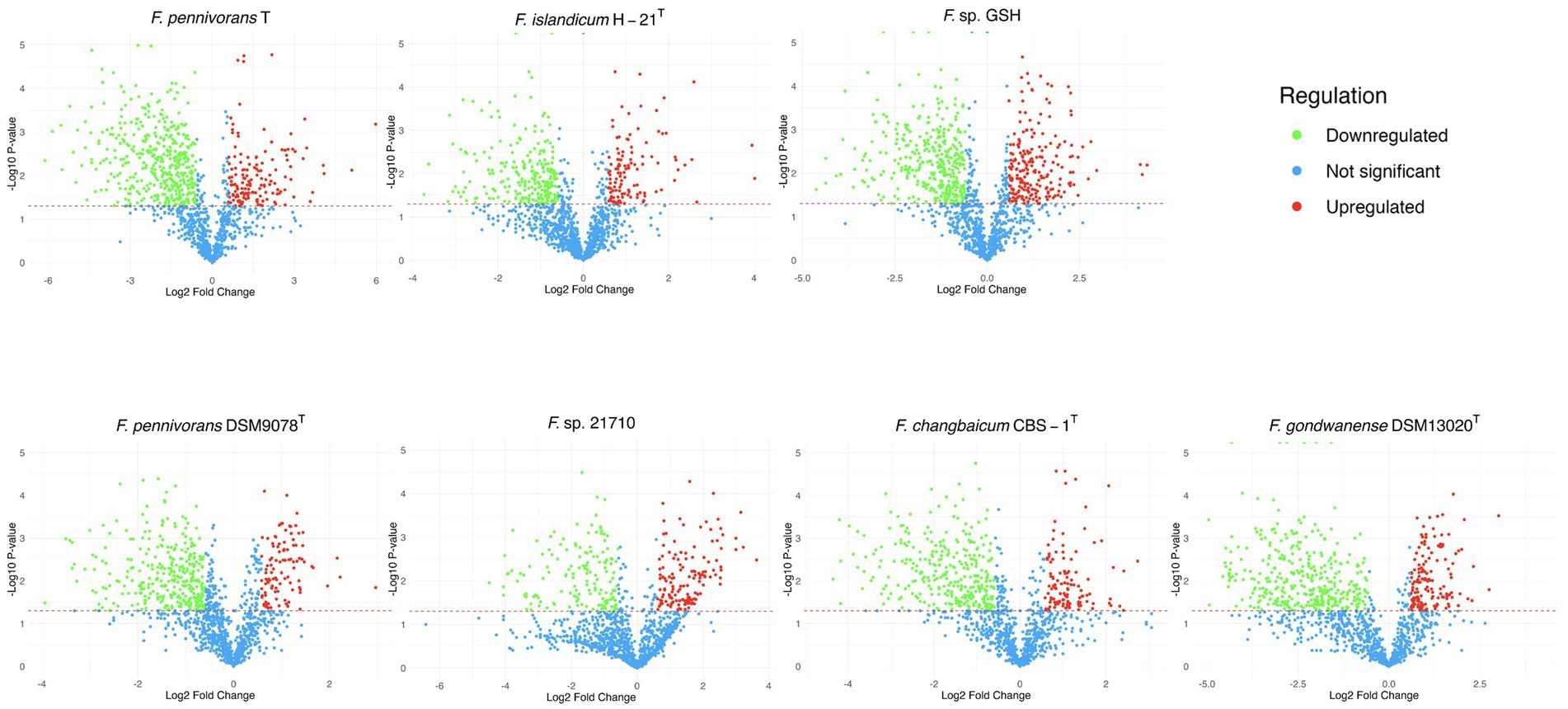
Figure 2. Volcano plot representations of the proteins identified in the cellular proteome of the described strains. The green dots represent the under-detected proteins in the feather condition compared to the glucose condition, and the red dots indicate the over-detected ones, with a |fold| threshold of 1.5. The statistical threshold was set at 0.05, with those proteins not significantly modified in terms of abundance, i.e., with higher p-values, as well as non-regulated proteins, indicated in blue.
Genome annotation of these strains revealed that each strain encoded approximately 100 reductases and 50 peptidases. Proteomic analysis identified >70 reductases and at least 40 peptidases, except for F. changbaicum CBS-1T and F. pennivorans T, with 32 and 39 detected peptidases, respectively (Table 2). The strain with the highest number of upregulated reductases and peptidases was again the non-keratinolytic strain F. gondwanense DSM13020T, with 26 and 16, respectively, approximately 35% of the total of both types of enzymes. Among the keratinolytic strains, F. islandicum H-21T showed the highest number of upregulated reductases (21), accounting for 28% of the total identified reductases. This contrasts with the sparse number of differentially detected peptidases, with only three being the lowest among all the strains studied. The fraction of upregulated reductases in the other strains ranged from 10 (11% of the total identified) for F. pennivorans DSM9078T to 20 (29% of the total identified) for F. changbaicum CBS-1T. The number of upregulated peptidases was lower, even more so than that of the reductases, ranging from 11 in F. pennivorans DSM9078T (25% of the total identified) to 7 in changbaicum CBS-1T (22% of the total identified). The accession numbers of the upregulated peptidases and reductases identified in each strain are listed in Supplementary Table S1.
KEGG functional analysis
The sequences of significantly upregulated proteins in F. pennivorans T, Fervidobacterium sp. GSH and F. islandicum H-21T were analyzed using the BlastKOALA (KEGG Orthology and Links Annotation) tool. A total of 111 proteins (69.8% of sequences) from the dataset of F. pennivorans T, 137 (62.8% of sequences) from Fervidobacterium sp. GSH, and 81 (64.8%) from F. islandicum H-21T were successfully annotated. The largest KEGG category was related to the carbohydrate metabolism pathways, with 19 entries from F. pennivorans T and 26 from Fervidobacterium sp. GSH, and 18 from F. islandicum H-21T. Additionally, amino acid metabolism pathways included 15 proteins from F. pennivorans T and 11 proteins from Fervidobacterium sp. GSH and eight from F. islandicum H-21T (Supplementary Figure S2).
Keratinolytic and non-keratinolytic strains comparison
The sequences of the upregulated peptidases identified in the most active strains, F. pennivorans T, Fervidobacterium sp. GSH and F. islandicum H-21T, were blasted against the proteome of F. gondwanense DSM13020T, a non-keratinolytic member of Fervidobacterium, using the protein sequences of F. pennivorans T. A total of 20 proteins were considered in this analysis. Six of them (QIV79356.1, QIV78721.1, QIV79147.1, QIV78659.1, QIV78935.1 and QIV78782.1) were not detected in F. gondwanense. The amino acid identity of these proteins ranged from 22.7 to 88.6%, with a median value of 80.4%. Interestingly, orthologues of three peptidases previously categorized as “true” keratinases (Qiu et al., 2020) (QIV78374.1, QIV78926.1 and QIV78937.1) were strongly downregulated in F. gondwanense. Furthermore, the amino acid identity of QIV78926.1 and QIV78937.1 compared to the orthologues of F. gondwanense was 55.3 and 54.9%, respectively (Table 3).
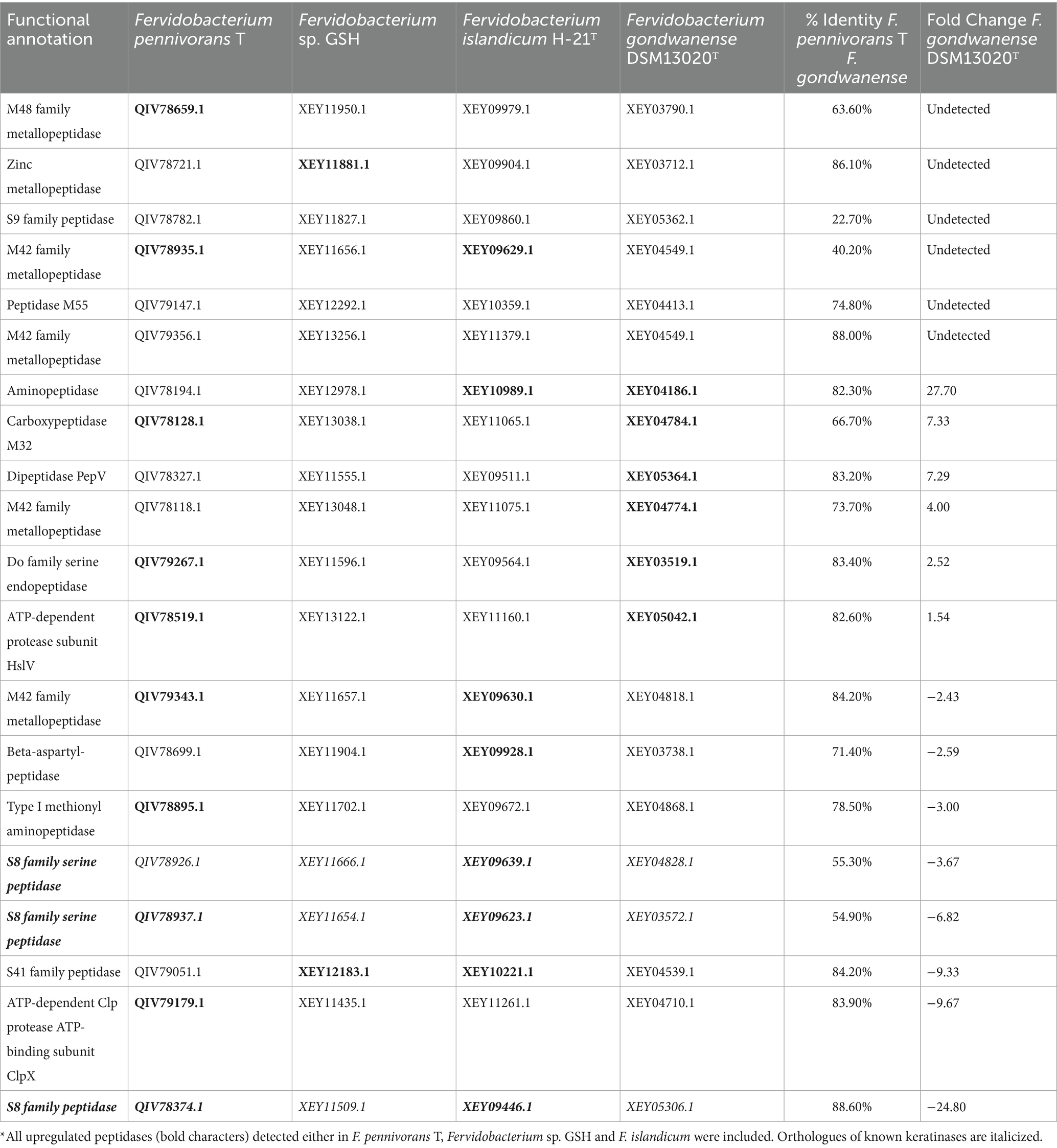
Table 3. Peptidase comparison across the most active strains and the non-keratinolytic F. gondwanense*.
Transcriptomics
Deep mRNA sequencing (62.3 GB data) led to high coverage per sample: 2,813 × for F. pennivorans T and 2,361 × for Fervidobacterium sp. GSH, and 2,141 × for F. islandicum H-21T. More than 90% of the reads mapped to annotated genomes for all samples, except for two replicates with slightly lower values. In general, the broken pairs remained below 2% for all the samples (Supplementary Tables S2–S4). Following normalization and statistical analysis with DESeq2, 1,716 genes (FDR < 0.05) were identified in F. pennivorans T and 1,398 in Fervidobacterium sp. GSH, and 953 in F. islandicum H-21T. Less than half of these genes were overexpressed (Fold >1.5) in F. pennivorans T and Fervidobacterium sp. GSH (728 and 665, respectively), whereas 502 genes were overexpressed in F. islandicum H-21T compared with the feather- and glucose-grown cells. Heatmaps drawn using the 50 most overexpressed and the 50 most underexpressed genes of the three strains are shown in Figure 3, which shows strong genetic reprogramming in the three strains to adapt to the carbon source. Furthermore, the expression of some genes was higher after 18 h of growth, whereas others were more abundant after 40 h, indicating that some genes were switched on and off earlier than others when these strains were growing with chicken feathers.
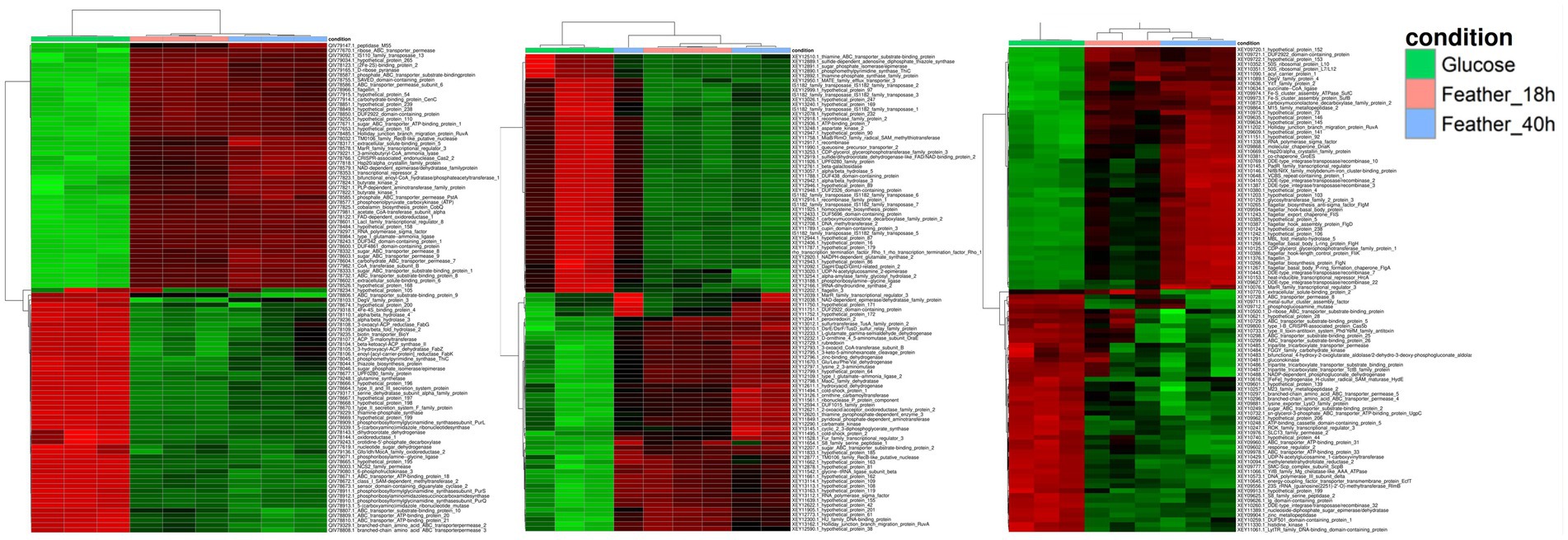
Figure 3. Heatmap representation with the most promiment genes among the significantly expressed features of F. pennivorans T, Fervidobacterium sp. GSH, and F. islandicum H-21T. The columns represent the different samples, corresponding to the substrates used in the experiment: glucose and chicken feathers. The rows indicate the relative abundance of the genes, from high (red) to low expression (green).
Peptidases and oxidoreductases
The expression of 47 genes annotated as peptidases or proteases was significantly (FDR < 0.05) modified in the F. pennivorans T transcriptome, 19 of which were overexpressed in either the 18 or 40 h samples.
Several enzymes with keratinolytic potential have been identified, including metallopeptidases (QIV78118.1, QIV79325.1, QIV79356.1, QIV79147.1, QIV78781., QIV78721.1, QIV78572.1, and QIV79192.1), carboxypeptidases (QIV79319.1), and serine peptidases (QIV78782.1, QIV78937.1). For Fervidobacterium sp. GSH, 36 peptidases or proteases were identified in the transcriptome. A total of 21 of these enzymes were significantly overexpressed in the 18 or 40 h samples. Among these enzymes, metallopeptidases (XEY13048.1, XEY13256.1, XEY12292.1, XEY11656.1, XEY12216.1, XEY12589.1 and XEY12665.1) or serine peptidases (XEY11827.1, XEY11654.1 and XEY11509.1). Finally, 27 of these enzymes were identified in the proteome of F. islandicum H-21T (FDR < 0.05), 14 of which were overexpressed in the samples harvested after 18 or 40 h. Several overexpressed metallopeptidases (XEY11075.1, XEY09864.1, XEY10359.1 and XEY10721.1) and serine peptidases (XEY09860.1 and XEY09564.1) were also found in this bacterium. Notably, three of these genes were overexpressed in feather-grown cells of all three strains: two metallopeptidases of the M42 and M55 subfamilies, a serine S9 family protease, and the dipeptidase PepV. In addition, a gene annotated as an ATP-dependent Clp protease ATP-binding subunit was overexpressed in all three strains.
Supplementary Table S5 shows the most overexpressed and most upregulated peptidases, proteases and oxidoreductases detected across the analyzed strains in both Transcriptomics and Proteomics analyses. This table also includes the fold-changes, Enzyme Commission (EC) numbers and the amino acid percentage identity.
Only a fraction of the statistically significant peptidases and proteases detected in these three strains is overexpressed in the presence of feathers, suggesting that not all peptidases of these strains are necessary for keratin degradation. Some of them had high expression levels only at one of the kinetic points, that is, after 18 or 40 h of incubation, with only a few of these genes found to be active throughout the entire incubation period in feather cultures.
Keratin degradation begins with the action of different oxidoreductases. A total of 76 of these genes were detected in the transcriptome of F. pennivorans T, 38 of which were overexpressed. Regarding Fervidobacterium sp. GSH, 39 overexpressed reductases within a total of 65 significant ones were identified, a similar number compared to F. pennivorans T. Finally, 46 reductases were identified among the significant genes in F. islandicum H-21T, 29 of which were overexpressed in the feather cultures, a lower count compared with the other two strains. Seven reductases were overexpressed in all three strains, suggesting that they were important for the reaction. These enzymes were identified as Glu/Leu/Phe/Val dehydrogenase, 2-oxoacidacceptor oxidoreductase subunit alpha, NAD (P)/FAD-dependent oxidoreductase, NADH-quinone oxidoreductase subunit NuoF, SDR family oxidoreductase, L-threonine 3-dehydrogenase, 2,4-diaminopentanoate dehydrogenase, and 2-hydroxyacid dehydrogenase.
Notably, more than half of the reductases of F. pennivorans T and Fervidobacterium sp. GSH were switched on after only 18 h of incubation, whereas only a few were overexpressed after 40 h of incubation; thus, they can be considered late-induced reductases. This suggests that more reductases are activated during the early stages of bacterial growth. F. islandicum H-21T did not exhibit a clear pattern. Most of the overexpressed reductases identified in this strain were found after 48 h of incubation, with only a few that could be considered early induced reductases. This behavior may also explain the longer time required by this bacterium to adapt to changes in the carbon source and fully degrade feathers.
Among the most abundant proteins in the supernatants of feather cultures, both peptidases and reductases were detected, along with other enzymes such as specific ABC substrate-binding transporters. Additionally, the proteins found upregulated in the three most active strains and their functional annotations are available in a separate Excel file as Supplementary Table S6.
Proteomics and transcriptomics integration
A total of 164 overrepresented features of F. pennivorans T were identified by combining the results of transcriptomic and proteomic analyses. The interactions between these features were analyzed using the STRING tool. A network with 325 edges with an estimated p-value lower than 1.0e−16 was obtained. This network indicates a high number of statistically relevant interactions, that is, biologically relevant connections. Among the clusters identified using STRING, a group of features related to lysine degradation stood out, with 10 and 8 features annotated in the STRING cluster CL: 3375 (FDR = 0.03) and the KEGG pathway fpe00310 (FDR = 0.003), respectively. In addition, 15 features were assigned to the STRING cluster CL: 2832 (FDR = 0.03), which is related to bacterial extracellular solute binding (Figure 4).
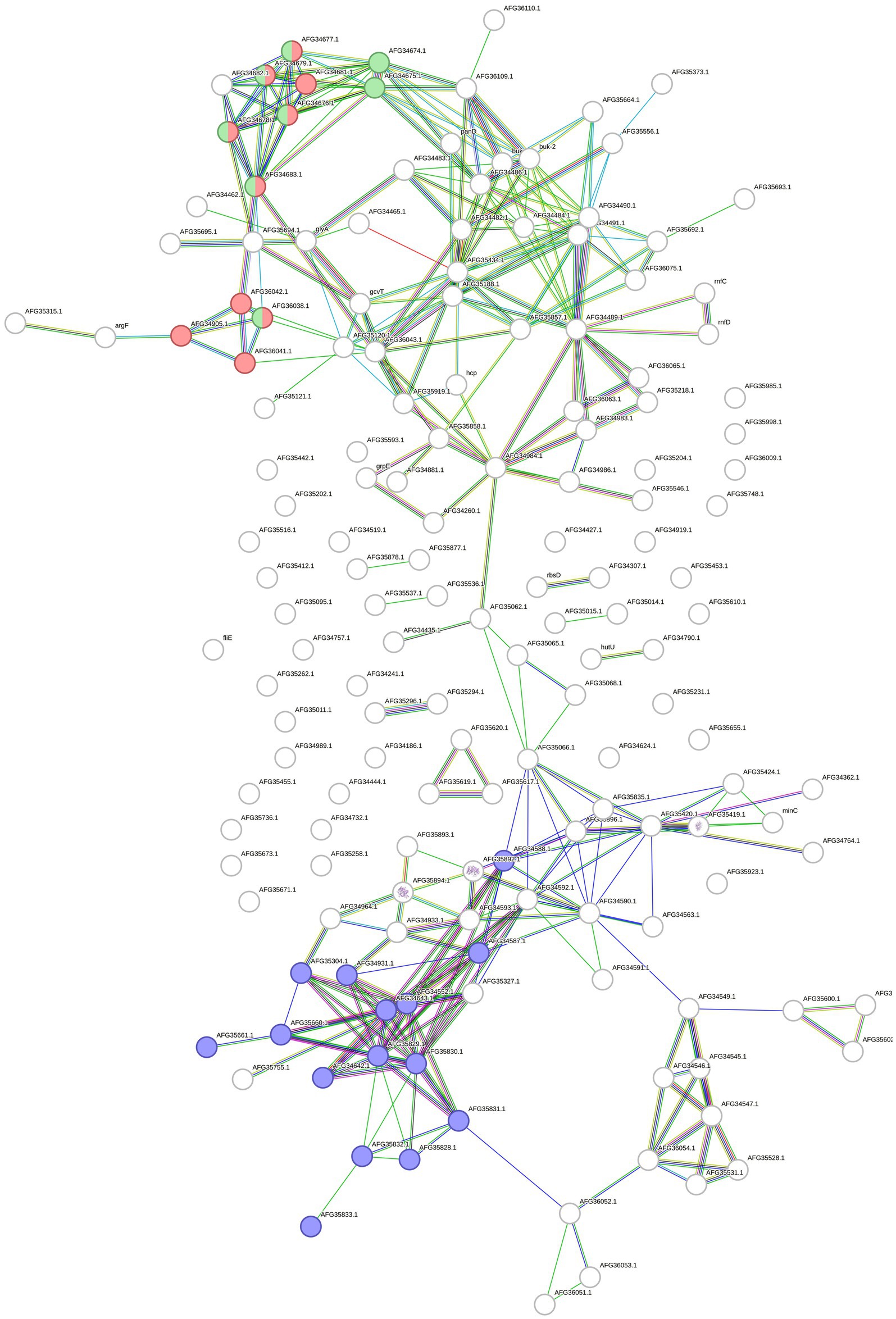
Figure 4. Protein and gene network made with STRING. The network contains all the upregulated proteins and genes identified in F. pennivorans T when growing the bacterium with a chicken feather, compared to cultures grown with glucose. The circles represent the features, and the lines indicate the connections found by STRING. Features related to lysine degradation are colored in red (STRING cluster CL:3375) and green (KEGG pathway fpe00310), and those assigned to extracellular solute binding (STRING cluster CL:2832) are colored in blue.
For Fervidobacterium sp. GSH, lysine degradation (fpe00310, FDR 0.00047), and glycine, serine, and threonine metabolism (fpe00260, FDR 0.0017) pathways were significant. Additionally, several biological processes annotated in the Gene Ontology database were detected, some of which were related to keratin and protein degradation processes. Overall, 384 edges were created in the network over the expected number of 209 edges with a p-value lower than 1.0e−16 (Figure 5).
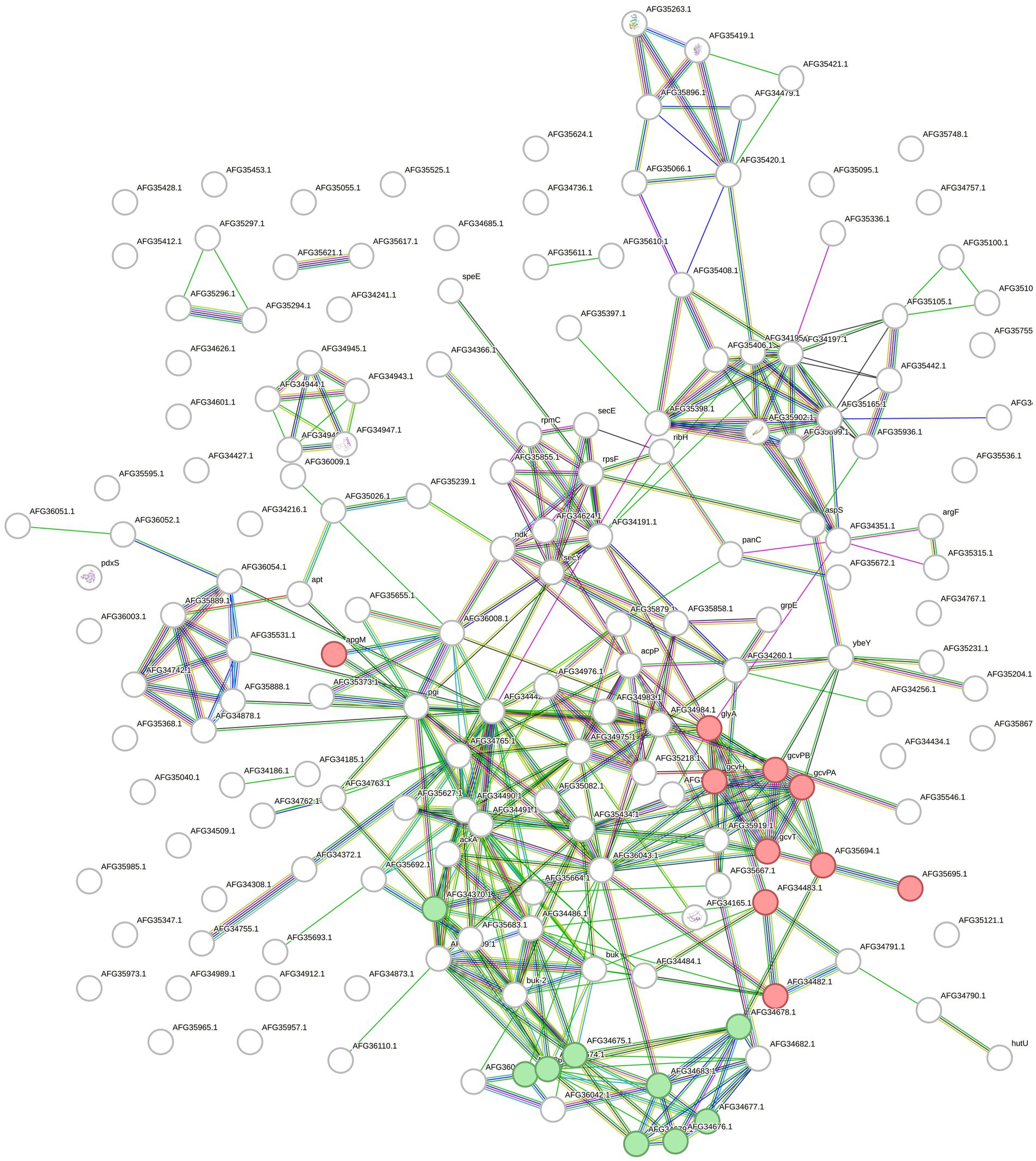
Figure 5. STRING network built with the upregulated and overexpressed features identified in F. sp. GSH. Overexpressed genes and upregulated proteins when growing the cells with chicken feathers were used. Green colored circles correspond to features in the lysine degradation pathway (fpe00310) and the red ones to glycine, serine and threonine metabolism (fpe00260), both from KEGG database.
Finally, for F. islandicum H-21T, 74 selected features provided a STRING network with 60 edges (p = 7.71e-05). In this case, 11 features of the oxidoreductase activity STRING-related cluster (CL: 934) were annotated with a significant FDR of 0.0207. Five features of the lysine degradation pathway in KEGG (fia00310) were identified and annotated with an FDR of 0.017 (Figure 6).
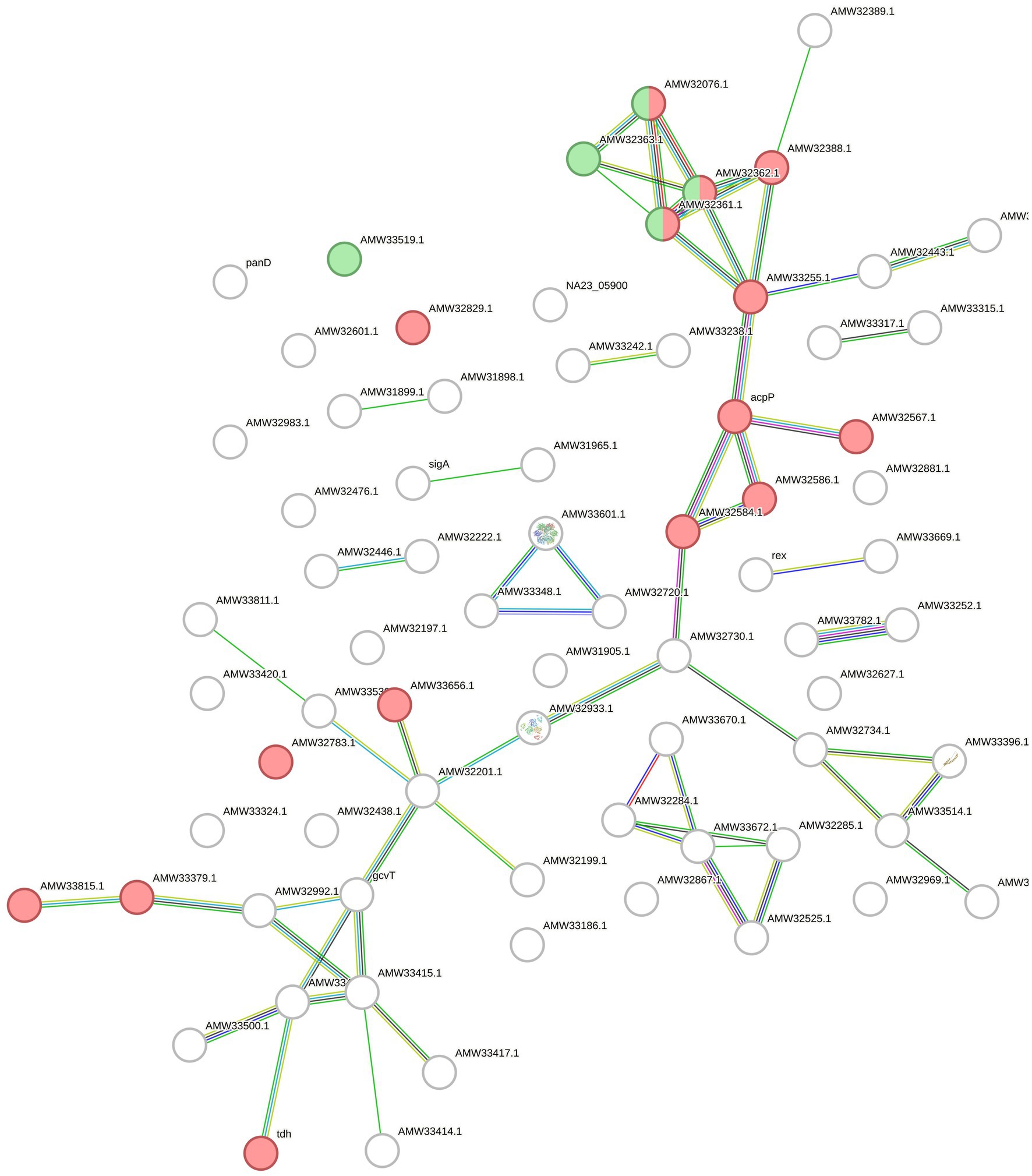
Figure 6. STRING network with the upregulated proteins and overexpressed genes of F. islandicum H-21T. The circles represent the features of the bacterium annotated by STRING and the lines indicate the interactions among them. The features of the CL:934 cluster (Mixed, incl. Oxidoreductase activity, and Butanoate metabolism, red) and of the fia00310 KEGG Pathway (Lysine degradation, green) are highlighted.
A model of keratin degradation
A hypothetical model for keratin degradation is presented in Figure 7, showing overrepresented candidate oxidoreductases, peptidases and specific peptide binding proteins and transporters of F. pennivorans T in the feather cultures. The location (extra-or intracellular) of the enzymes reflects their presence in the upregulated exo-or cellular proteome. The exo-and endopeptidase assignation is based on MEROPS database. The process starts when cells of F. pennivorans T bind to the feather. At this point, cell-bound and extracellular features would start the reaction. Both extracellular oxidoreductases (QIV79098.1, QIV79321.1), exo- (QIV79356.1), endo- (QIV79267.1) and oligopeptidases (QIV78327.1) that could potentially participate in this step are shown. Smaller peptides would be captured by specific substrate binding proteins (QIV77877.1, QIV78038.1) and transferred into the cytoplasm by specific permeases [QIV77875.1, a dipeptide transporter, as predicted by sequence comparison (Consortium, 2024)]. Then, cellular oxidoreductases (QIV78122.1, QIV78923.1) would reduce the disulfide bonds and a combined action of cellular exo- (QIV79147.1), endo- (QIV78374.1) and oligopeptidases (QIV78128.1) would decompose the peptides and finish the degradation.
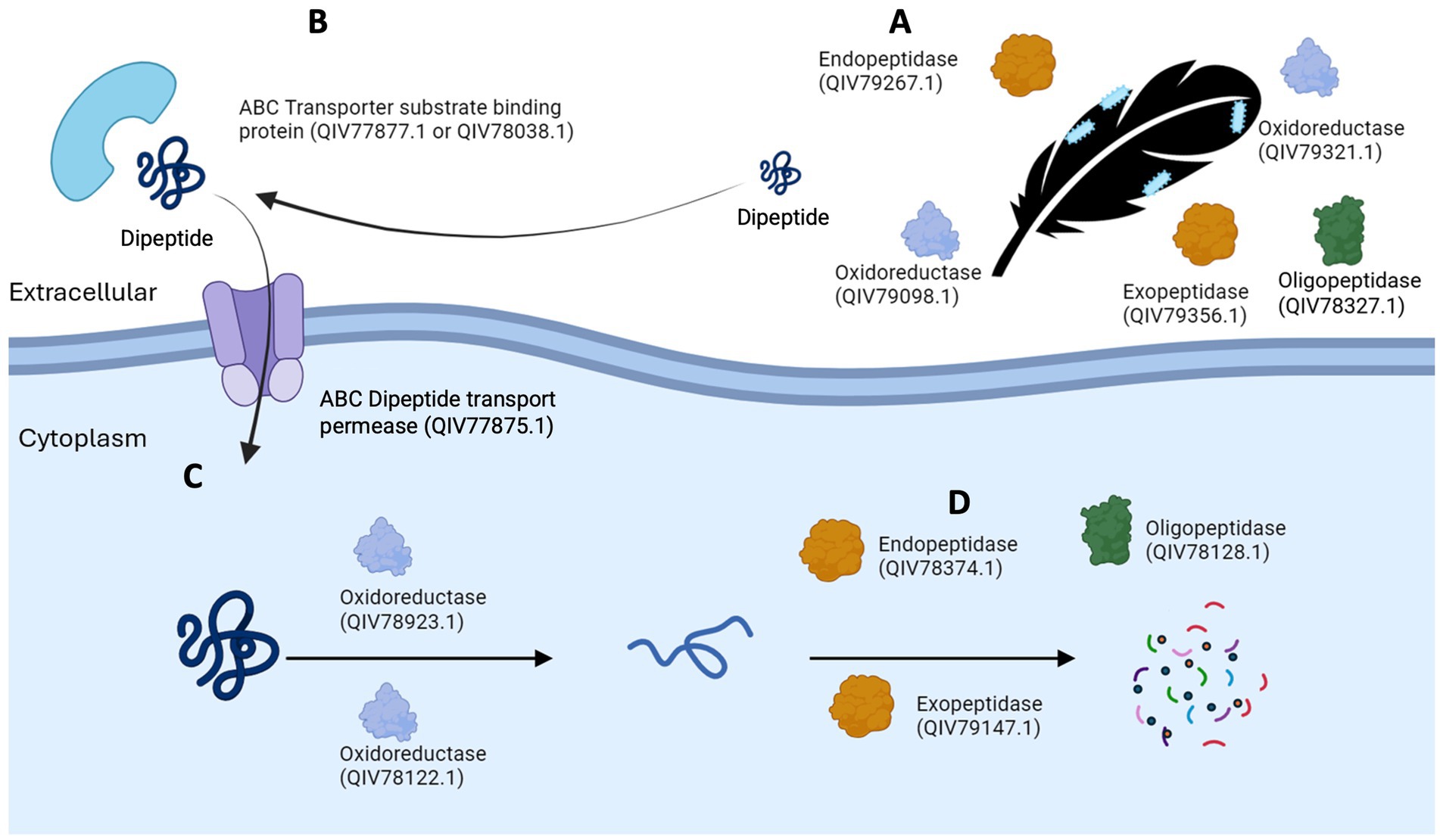
Figure 7. A model of keratin degradation by Fervidobacterium, made with upregulated enzymes identified in F. pennivorans T. Candidate peptidases and oxidoreductases start the reaction outside the cell (A), specific peptide binding proteins (B) and transporters introduce smaller peptides into the cytoplasm (C), where the combined action of cellular oxidoreductases, exo-, endo-and oligopeptidases complete the reaction.
Discussion
Keratin is a robust and recalcitrant protein that is slowly degraded despite its high occurrence in nature. While several microorganisms can break down feather keratin, only a few are thermophilic, and a minority are anaerobic (Daroit and Brandelli, 2014; Sahni et al., 2015; Srivastava et al., 2020). This study assessed the keratinolytic capabilities of all available isolates in the genus Fervidobacterium, a group of bacteria known to count with keratinolytic members, and investigated and compared their transcriptomes and proteomes when grown with glucose or chicken feathers to highlight the key molecular players for feather degradation.
Among the studied organisms, only F. nodosum Rt17-B1T and F. gondwanense DSM13020T showed no evidence of keratin degradation. F. riparium 1445tT, F. thailandense FC2004T and Fervidobacterium sp. 13770 exhibited partial activity, as they broke down a portion of the feathers with which they were cultured, but the degradation halted at some point, rendering the reaction incomplete. The six remaining strains, F. changbaicum CBS-1T, F. islandicum H-21T, F. pennivorans T, F. pennivorans DSM9078T, Fervidobacterium sp. GSH and Fervidobacterium sp. 21710 could degrade feather keratin completely within 72 h. Thus, 6 out of 11 Fervidobacterium strains displayed clear keratinolytic activity, demonstrating the potential of this group of bacteria for applications in feather and keratin degradation reactions. Among the aforementioned strains, three showed high activity: F. islandicum H-21T, F. pennivorans T, and Fervidobacterium sp. GSH, being F. pennivorans T particularly efficient as it could degrade a chicken feather almost completely after 48 h at 70°C.
Between 70 and 92% of the total proteins in bacteria were identified using label-free shotgun proteomics. Of these, 10–15% were significantly more abundant in feather cultures, most of them detected in the cellular proteome, indicating that this fraction of proteins was upregulated in the presence of feathers. Surprisingly, F. gondwanense DSM13020T had a high number of upregulated proteins despite its inability to damage the integrity of feathers. This indicated that the growth of this bacterium halted when the yeast extract supplement in the culture was depleted. A comparison of the upregulated peptidases across the most active strains with the peptidases of F. gondwanense DSM13020T showed that several of these enzymes in the latter were downregulated in the feather cultures, suggesting deficiencies in the regulation of these enzymes in F. gondwanense DSM13020T, which may explain the inability of this organism to attack feather keratin. In particular, the “true” keratinases QIV78374.1, QIV78926.1 and QIV78937.1 were strongly downregulated in F. gondwanense whereas clearly upregulated in the keratinolytic strains. These three enzymes shared a sequence identity of 88.6, 54.9 and 55.3%, respectively and compared with their orthologues in F. pennivorans T, suggesting potential deficiencies in their sequence and/or structure, particularly in QIV78926.1 and QIV78937.1. So, these three enzymes may be required for successful feather degradation and can be good candidates to be further explored as keratinolytic enzymes. Furthermore, genus Fervidobacterium has undergone several horizontal gene transfer events (Nelson et al., 1999; Frock et al., 2010; Cuecas et al., 2017) and shows an intricated evolutionary history (Javier-López et al., 2024), which might have resulted in the loss or truncation of some of these enzymes.
The pathways involved in keratin degradation remain to be elucidated. It has been hypothesized that keratin degradation cannot be completed by a single hydrolytic enzyme, so keratinolytic organisms may possess and activate multiple and different enzymes to effectively decompose this recalcitrant molecule (Huang et al., 2015; Fellahi et al., 2016). Thus, current hypothesis is that this reaction requires at least oxidoreductases to cleave the disulfide bonds of the molecule and endo-and exopeptidases to hydrolyze the exposed peptide bonds (Lange et al., 2016; Shavandi et al., 2017; Qiu et al., 2020). Furthermore, it has been previously shown that feather degradation may start with physical binding of the bacteria to the surface of the feather, something already described in Fervidobacterium (Kang et al., 2020; Javier-Lopez et al., 2022) and other taxa (Jeong et al., 2010), highlighting the importance of intracellular and membrane-bound enzymes in the process.
Although fervidobacteria possess a similar total number of these enzymes, their regulation across bacteria in the presence of keratin varies. Surprisingly, F. gondwanense DSM13020T showed a higher number of upregulated peptidases and oxidoreductases than keratinolytic organisms, indicating that fervidobacteria may not make use of a large number of these enzymes for effective feather breakdown. The number of overrepresented peptidases identified in the transcriptomics and proteomics analyses was similar in the three studied strains. However, the number of overexpressed oxidoreductases, among the total number of these enzymes identified in the proteomes, was higher in the transcriptomics study, particularly in the early log phase, consistent with the hypothesis that oxidoreductases participate in the first steps of keratin degradation. Although many proteases have shown keratinolytic activity relying on the previous action of oxidoreductases, only a few can at least partially degrade keratin without accessory agents and have thus been categorized as “true keratinases” (Qiu et al., 2020). Two S8 proteases, one from Bacillus sp. AH-101 (Takami et al., 1990) and another from the fungus Onygena corvina (Huang et al., 2015), met these criteria. F. pennivorans T has three homologues of these keratinases: QIV78926.1, QIV78374.1 and QIV78937.1, respectively. QIV78374.1 was upregulated with a fold change of 7.68 in the proteomics analysis, and QIV78937.1 was overexpressed in both the transcriptomics and proteomics analyses, with fold changes of 1.76 and 3.08, respectively. Therefore, these three proteases are potential candidates for catalogs of true keratinases. While sources and mechanisms of action of keratinases are variate, with a wide distribution both in prokaryotic and eukaryotic organisms (Qiu et al., 2020), these three proteases can be classified as S8 serine endoproteases, a group of enzymes known to be involved in keratin degradation (Huang et al., 2015). An identical ortholog of QIV78937.1, termed fervidolysin (PDB 1R6V), has been described and expressed (Kluskens et al., 2002). Its 1.7 Å crystal structure showed four different domains, two sandwich domains, a 14 kDa propetide and a catalytic triad Asp41-His79-Ser260, composing a 58 kDa mature protein (Kim et al., 2004). An ortholog of the thermostable alkaline protease from Bacillus sp. AH-101was also identified in the upregulated proteome of F. pennivorans T (QIV78926.1). All these proteases are thermostable and active at high pH (10–12) (Qiu et al., 2020).
Furthermore, six peptidases which were overrepresented in the feather cultures of F. pennivorans T, Fervidobacterium sp. GSH and/or F. islandicum H-21T were also confirmed to be overexpressed in the presence of keratin in a previous work (Kang et al., 2020) on F. islandicum AW-1: QIV78895.1, QIV78374.1, QIV78699.1, QIV79319.1, QIV79051.1 and QIV79147.1. Current work is progressing on enzymatic assays including these and other proteases, showing promising results. Proteomic and transcriptomic results often show a low correlation (Gygi et al., 1999), especially in bacteria. Thus, it is challenging to combine the data from both approaches (Nie et al., 2006). Here, the STRING networks that merged the upregulated proteins and overexpressed genes showed robust and meaningful connections across the overrepresented features, suggesting that most of them were linked to the response of these organisms to the presence of feather keratin in the environment. However, it is worth noting that non-overrepresented features may also participate in the degradation process, as well as others whose current annotation is not accurately solved.
Conclusion
Based on the keratinolytic assessment of the 11 available strains of the genus Fervidobacterium, 6 strains, namely F. changbaicum CBS-1T, F. islandicum H-21T, F. pennivorans T, F. pennivorans DSM9078T, Fervidobacterium sp. GSH and Fervidobacterium sp. 21710 showed clear activity, completely degrading chicken breast feathers within 72 h at high temperatures (65–80°C). F. islandicum H-21T, F. pennivorans T, and Fervidobacterium sp. GSH were the most active organisms, with F. pennivorans disintegrating chicken feathers after 48 h at 70°C. The proteomics results revealed that only a small fraction of the proteome in the active strains responded to this condition and was upregulated in the presence of feathers, suggesting that these bacteria do not need major enzymatic machinery to break down feather keratin. A higher number of reductase-encoding genes were found in the early log phase of the overexpressed transcriptomes, implying the involvement of these enzymes in the initial stages of keratin degradation, congruent with the current keratin degradation hypothesis. Furthermore, three potential “true keratinases” were identified in F. pennivorans T: QIV78374.1, QIV78926.1, and QIV78937.1 but were downregulated in the feather cultures of the non-keratinolytic F. gondwanense DSM13020T. Homologs of these enzymes have already been cataloged as true keratinases, which are active even in the absence of helper oxidoreductases. The enzymes described here could expand the current catalog of available keratinolytic enzymes and may be integrated into industrial applications to help to mitigate the ecological and environmental challenges associated with feather waste accumulation.
Data availability statement
Mass spectrometry proteomics data are available from the ProteomeXchange Consortium via the PRIDE partner repository under the dataset identifiers PXD054267 and 10.6019/PXD054267 (Fervidobacterium pennivorans T), PXD054278, and 10.6019/PXD054278 (Fervidobacterium sp. GSH), PXD054282 and 10.6019/PXD054282 (Fervidobacterium pennivorans DSM 9078T), PXD054272 and 10.6019/PXD054272 (Fervidobacterium islandicum H-21T), and PXD054280 and 10.6019/PXD054280 (Fervidobacterium sp. DSM 21710), PXD054285 and 10.6019/PXD054285 (Fervidobacterium changbaicum CBS-1T), and PXD054269 and 10.6019/PXD054269 (Fervidobacterium gondwanense DSM13020T). The equivalence between the locus tag codes in the PRIDE tables and the accession numbers available in GenBank database is available in Supplementary Table S7.
Author contributions
RJ-L: Conceptualization, Data curation, Formal analysis, Investigation, Methodology, Visualization, Writing – original draft, Writing – review & editing. MK: Formal analysis, Investigation, Methodology, Writing – review & editing. JA: Formal analysis, Funding acquisition, Methodology, Supervision, Writing – original draft, Writing – review & editing. N-KB: Conceptualization, Funding acquisition, Project administration, Supervision, Writing – original draft, Writing – review & editing.
Funding
The author(s) declare that financial support was received for the research and/or publication of this article. This research was funded by the ERA-NET Cofund on Food Systems and Climate (FOSC) under the European Union’s Horizon 2020 Research and Innovation Program (grant number 862555), the Research Council of Norway (Norges Forskningsråd) (grant number 328955) and the Agence Nationale de la Recherche (grant number ANR-21-FOSC-0002-04).
Conflict of interest
The authors declare that the research was conducted in the absence of any commercial or financial relationships that could be construed as a potential conflict of interest.
Generative AI statement
The authors declare that no Generative AI was used in the creation of this manuscript.
Publisher’s note
All claims expressed in this article are solely those of the authors and do not necessarily represent those of their affiliated organizations, or those of the publisher, the editors and the reviewers. Any product that may be evaluated in this article, or claim that may be made by its manufacturer, is not guaranteed or endorsed by the publisher.
Supplementary material
The Supplementary material for this article can be found online at: https://www.frontiersin.org/articles/10.3389/fmicb.2025.1509937/full#supplementary-material
Footnotes
3. ^https://github.com/ncbi/pgap
4. ^https://eurofinsgenomics.eu/
References
Andrews, K. T., and Patel, B. K. C. (1996). Fervidobacterium gondwanense sp nov, a new thermophilic anaerobic bacterium isolated from nonvolcanically heated geothermal waters of the great Artesian Basin of Australia. Int. J. Syst. Bacteriol. 46, 265–269. doi: 10.1099/00207713-46-1-265
Armengaud, J. (2016). Next-generation proteomics faces new challenges in environmental biotechnology. Curr. Opin. Biotechnol. 38, 174–182. doi: 10.1016/j.copbio.2016.02.025
Armengaud, J., Christie-Oleza, J. A., Clair, G., Malard, V., and Duport, C. (2012). Exoproteomics: exploring the world around biological systems. Expert Rev. Proteomics 9, 561–575. doi: 10.1586/epr.12.52
Atif, F., Maqsood, N., Ali, W., Ali, W., and Irfan, M. (2024). Extremophiles and their enzymatic diversity and biotechnological potential. Syst. Microbiol. Biomanufactur. 4, 833–849. doi: 10.1007/s43393-024-00275-7
Bhandari, V., and Gupta, R. S. (2014). “The phylum Thermotogae” in The prokaryotes: Other major lineages of Bacteria and the Archaea. eds. E. Rosenberg, E. F. DeLong, S. Lory, E. Stackebrandt, and F. Thompson (Berlin, Heidelberg: Springer Berlin Heidelberg), 989–1015.
Cai, J. G., Wang, Y. P., Liu, D. B., Zeng, Y., Xue, Y. F., Ma, Y. H., et al. (2007). Fervidobacterium changbaicum sp nov., a novel thermophilic anaerobic bacterium isolated from a hot spring of the Changbai Mountains, China. Int. J. Syst. Evol. Microbiol. 57, 2333–2336. doi: 10.1099/ijs.0.64758-0
Charlier, P., Bourdin, V., N'Dah, D., Kielbasa, M., Pible, O., and Armengaud, J. (2024). Metaproteomic analysis of king Ghezo tomb wall (Abomey, Benin) confirms 19th century voodoo sacrifices. Proteomics. 24:e2400048. doi: 10.1002/pmic.202400048
Chen, H., Gao, S., Li, Y., Xu, H.-J., Li, W., Wang, J., et al. (2022). Valorization of livestock keratin waste: application in agricultural fields. Int. J. Environ. Res. Public Health 19:6681. doi: 10.3390/ijerph19116681
Conners, S. B., Mongodin, E. F., Johnson, M. R., Montero, C. I., Nelson, K. E., and Kelly, R. M. (2006). Microbial biochemistry, physiology, and biotechnology of hyperthermophilic Thermotoga species. FEMS Microbiol. Rev. 30, 872–905. doi: 10.1111/j.1574-6976.2006.00039.x
Consortium, T. U. (2024). UniProt: the universal protein knowledgebase in 2025. Nucleic Acids Res. 53, D609–D617. doi: 10.1093/nar/gkae1010
Cowan, D. A., Albers, S. V., Antranikian, G., Atomi, H., Averhoff, B., Basen, M., et al. (2024). Extremophiles in a changing world. Extremophiles 28:26. doi: 10.1007/s00792-024-01341-7
Cuecas, A., Kanoksilapatham, W., and Gonzalez, J. M. (2017). Evidence of horizontal gene transfer by transposase gene analyses in Fervidobacterium species. PLoS One 12:e0173961. doi: 10.1371/journal.pone.0173961
Daroit, D. J., and Brandelli, A. (2014). A current assessment on the production of bacterial keratinases. Crit. Rev. Biotechnol. 34, 372–384. doi: 10.3109/07388551.2013.794768
De Oliveira Martinez, J. P., Cai, G., Nachtschatt, M., Navone, L., Zhang, Z., Robins, K., et al. (2020). Challenges and opportunities in identifying and Characterising keratinases for value-added peptide production. Catalysts 10:184. doi: 10.3390/catal10020184
Fellahi, S., Chibani, A., Feuk-Lagerstedt, E., and Taherzadeh, M. J. (2016). Identification of two new keratinolytic proteases from a Bacillus pumilus strain using protein analysis and gene sequencing. AMB Express 6:42. doi: 10.1186/s13568-016-0213-0
Friedrich, A. B., and Antranikian, G. (1996). Keratin degradation by Fervidobacterium pennavorans, a novel thermophilic anaerobic species of the order Thermotogales. Appl. Environ. Microbiol. 62, 2875–2882. doi: 10.1128/aem.62.8.2875-2882.1996
Frock, A. D., Notey, J. S., and Kelly, R. M. (2010). The genus Thermotoga: recent developments. Environ. Technol. 31, 1169–1181. doi: 10.1080/09593330.2010.484076
Godde, C., Sahm, K., Brouns, S. J. J., Kluskens, L. D., van der Oost, J., de Vos, W. M., et al. (2005). Cloning and expression of islandisin, a new thermostable subtilisin from Fervidobacterium islandicum, in Escherichia coli. Appl. Environ. Microbiol. 71, 3951–3958. doi: 10.1128/AEM.71.7.3951-3958.2005
Gouveia, D., Grenga, L., Pible, O., and Armengaud, J. (2020). Quick microbial molecular phenotyping by differential shotgun proteomics. Environ. Microbiol. 22, 2996–3004. doi: 10.1111/1462-2920.14975
Gygi, S. P., Rochon, Y., Franza, B. R., and Aebersold, R. (1999). Correlation between protein and mRNA abundance in yeast. Mol. Cell. Biol. 19, 1720–1730. doi: 10.1128/MCB.19.3.1720
Huang, Y., Busk, P. K., Herbst, F.-A., and Lange, L. (2015). Genome and secretome analyses provide insights into keratin decomposition by novel proteases from the non-pathogenic fungus Onygena corvina. Appl. Microbiol. Biotechnol. 99, 9635–9649. doi: 10.1007/s00253-015-6805-9
Huber, R., Langworthy, T. A., König, H., Thomm, M., Woese, C. R., Sleytr, U. B., et al. (1986). Thermotoga maritima sp. nov. represents a new genus of unique extremely thermophilic eubacteria growing up to 90°C. Arch. Microbiol. 144, 324–333. doi: 10.1007/BF00409880
Huber, R., Woese, C. R., Langworthy, T. A., Kristjansson, J. K., and Stetter, K. O. (1990). Fervidobacterium-Islandicum Sp-Nov, a new extremely thermophilic Eubacterium belonging to the Thermotogales. Arch. Microbiol. 154, 105–111. doi: 10.1007/BF00423318
Hungate, R. E. (1950). The anaerobic mesophilic cellulolytic BACTERIA. Bacteriol. Rev. 14, 1–49. doi: 10.1128/br.14.1.1-49.1950
Javier-López, R., Geliashvili, N., and Birkeland, N.-K. (2024). Comparative genomics of Fervidobacterium: a new phylogenomic landscape of these wide-spread thermophilic anaerobes. BMC Genomics 25:1248. doi: 10.1186/s12864-024-11128-x
Javier-Lopez, R., Mandolini, E., Dzhuraeva, M., Bobodzhanova, K., and Birkeland, N. K. (2022). Fervidobacterium pennivorans subsp. keratinolyticus subsp. nov., a novel feather-degrading anaerobic thermophile. Microorganisms 11:22. doi: 10.3390/microorganisms11010022
Jeong, J.-H., Lee, O. M., Jeon, Y.-D., Kim, J.-D., Lee, N.-R., Lee, C.-Y., et al. (2010). Production of keratinolytic enzyme by a newly isolated feather-degrading Stenotrophomonas maltophilia that produces plant growth-promoting activity. Process Biochem. 45, 1738–1745. doi: 10.1016/j.procbio.2010.07.020
Kanehisa, M. (2019). Toward understanding the origin and evolution of cellular organisms. Protein Sci. 28, 1947–1951. doi: 10.1002/pro.3715
Kanehisa, M., Furumichi, M., Sato, Y., Kawashima, M., and Ishiguro-Watanabe, M. (2022). KEGG for taxonomy-based analysis of pathways and genomes. Nucleic Acids Res. 51, D587–D592. doi: 10.1093/nar/gkac963
Kanehisa, M., and Goto, S. (2000). KEGG: Kyoto encyclopedia of genes and genomes. Nucleic Acids Res. 28, 27–30. doi: 10.1093/nar/28.1.27
Kang, E., Jin, H.-S., La, J. W., Sung, J.-Y., Park, S.-Y., Kim, W.-C., et al. (2020). Identification of keratinases from Fervidobacterium islandicum AW-1 using dynamic gene expression profiling. Microb. Biotechnol. 13, 442–457. doi: 10.1111/1751-7915.13493
Kanoksilapatham, W., Pasomsup, P., Keawram, P., Cuecas, A., Portillo, M. C., and Gonzalez, J. M. (2016). Fervidobacterium thailandense sp nov., an extremely thermophilic bacterium isolated from a hot spring. Int. J. Syst. Evol. Microbiol. 66, 5023–5027. doi: 10.1099/ijsem.0.001463
Kim, J. S., Kluskens, L. D., de Vos, W. M., Huber, R., and van der Oost, J. (2004). Crystal structure of fervidolysin from Fervidobacterium pennivorans, a keratinolytic enzyme related to subtilisin. J. Mol. Biol. 335, 787–797. doi: 10.1016/j.jmb.2003.11.006
Kluskens, L. D., Voorhorst, W. G. B., Siezen, R. J., Schwerdtfeger, R. M., Antranikian, G., van der Oost, J., et al. (2002). Molecular characterization of fervidolysin, a subtilisin-like serine protease from the thermophilic bacterium Fervidobacterium pennivorans. Extremophiles 6, 185–194. doi: 10.1007/s007920100239
Koblitz, J., Halama, P., Spring, S., Thiel, V., Baschien, C., Hahnke, R. L., et al. (2022). MediaDive: the expert-curated cultivation media database. Nucleic Acids Res. 51, D1531–D 1538. doi: 10.1093/nar/gkac803
Lange, L., Huang, Y., and Busk, P. K. (2016). Microbial decomposition of keratin in nature-a new hypothesis of industrial relevance. Appl. Microbiol. Biotechnol. 100, 2083–2096. doi: 10.1007/s00253-015-7262-1
Lee, Y. J., Dhanasingh, I., Ahn, J. S., Jin, H. S., Choi, J. M., Lee, S. H., et al. (2015). Biochemical and structural characterization of a keratin-degrading M32 carboxypeptidase from Fervidobacterium islandicum AW-1. Biochem. Biophys. Res. Commun. 468, 927–933. doi: 10.1016/j.bbrc.2015.11.058
Miller, T. L., and Wolin, M. J. (1974). A serum bottle modification of the Hungate technique for cultivating obligate anaerobes. Appl. Microbiol. 27, 985–987. doi: 10.1128/am.27.5.985-987.1974
Nelson, K. E., Clayton, R. A., Gill, S. R., Gwinn, M. L., Dodson, R. J., Haft, D. H., et al. (1999). Evidence for lateral gene transfer between Archaea and bacteria from genome sequence of Thermotoga maritima. Nature 399, 323–329. doi: 10.1038/20601
Nie, L., Wu, G., and Zhang, W. (2006). Correlation between mRNA and protein abundance in Desulfovibrio vulgaris: a multiple regression to identify sources of variations. Biochem. Biophys. Res. Commun. 339, 603–610. doi: 10.1016/j.bbrc.2005.11.055
Podosokorskaya, O. A., Merkel, A. Y., Kolganova, T. V., Chernyh, N. A., Miroshnichenko, M. L., Bonch-Osmolovskaya, E. A., et al. (2011). Fervidobacterium riparium sp nov., a thermophilic anaerobic cellulolytic bacterium isolated from a hot spring. Int. J. Syst. Evol. Microbiol. 61, 2697–2701. doi: 10.1099/ijs.0.026070-0
Qiu, J., Wilkens, C., Barrett, K., and Meyer, A. S. (2020). Microbial enzymes catalyzing keratin degradation: classification, structure, function. Biotechnol. Adv. 44:107607. doi: 10.1016/j.biotechadv.2020.107607
Rubiano-Labrador, C., Bland, C., Miotello, G., Guérin, P., Pible, O., Baena, S., et al. (2014). Proteogenomic insights into salt tolerance by a halotolerant alpha-proteobacterium isolated from an Andean saline spring. J. Proteome 97, 36–47. doi: 10.1016/j.jprot.2013.05.020
Sahni, N., Sahota, P., and Phutela, U. (2015). Bacterial keratinases and their prospective applications: a review. Int. J. Curr. Microbiol. App. Sci. 4, 768–783.
Sahoo, D. K., Thatoi, H. N., Mitra, B., Mondal, K. C., and Mohapatra, P. K. D. (2017) in Advances in microbial keratinase and its potential applications. Microbial biotechnology: Volume 1. Applications in agriculture and environment. eds. J. K. Patra, C. N. Vishnuprasad, and G. Das (Singapore: Springer Singapore), 105–133.
Saravanan, K., and Dhurai, B. (2012). Exploration on the amino acid content and morphological structure in chicken feather fiber. J. Textile Apparel Technol. Manage. 7, 1–6.
Shavandi, A., Silva, T. H., Bekhit, A. A., and Bekhit, A. E.-D. A. (2017). Keratin: dissolution, extraction and biomedical application. Biomater. Sci. 5, 1699–1735. doi: 10.1039/C7BM00411G
Srivastava, B., Khatri, M., Singh, G., and Arya, S. K. (2020). Microbial keratinases: an overview of biochemical characterization and its eco-friendly approach for industrial applications. J. Clean. Prod. 252:119847. doi: 10.1016/j.jclepro.2019.119847
Sypka, M., Jodłowska, I., and Białkowska, A. M. (2021). Keratinases as versatile enzymatic tools for sustainable development. Biomol. Ther. 11:1900. doi: 10.3390/biom11121900
Takami, H., Akiba, T., and Horikoshi, K. (1990). Characterization of an alkaline protease from Bacillus sp. no. AH-101. Appl. Microbiol. Biotechnol. 33, 519–523. doi: 10.1007/BF00172544
Tatusova, T., DiCuccio, M., Badretdin, A., Chetvernin, V., Nawrocki, E. P., Zaslavsky, L., et al. (2016). NCBI prokaryotic genome annotation pipeline. Nucleic Acids Res. 44, 6614–6624. doi: 10.1093/nar/gkw569
Wang, Y., Qian, J., Shi, T., Wang, Y., Ding, Q., and Ye, C. (2024). Application of extremophile cell factories in industrial biotechnology. Enzym. Microb. Technol. 175:110407. doi: 10.1016/j.enzmictec.2024.110407
Wickham, H. (2016). Ggplot2: Elegant graphics for data analysis. Cham: Switzerland, Springer International Publishing.
Keywords: chicken feather, keratin, keratinase, oxidoreductase, peptidase, protease, proteomics, transcriptomics
Citation: Javier-López R, Kielbasa M, Armengaud J and Birkeland N-K (2025) Transcriptomic and proteomic insights into feather keratin degradation by Fervidobacterium. Front. Microbiol. 16:1509937. doi: 10.3389/fmicb.2025.1509937
Edited by:
Philippe M. Oger, UMR5240 Microbiologie, Adaptation et Pathogenie (MAP), FranceReviewed by:
Santosh Kumar Karn, Sardar Bhagwan Singh University, IndiaIlya V. Kublanov, Hebrew University of Jerusalem, Israel
Copyright © 2025 Javier-López, Kielbasa, Armengaud and Birkeland. This is an open-access article distributed under the terms of the Creative Commons Attribution License (CC BY). The use, distribution or reproduction in other forums is permitted, provided the original author(s) and the copyright owner(s) are credited and that the original publication in this journal is cited, in accordance with accepted academic practice. No use, distribution or reproduction is permitted which does not comply with these terms.
*Correspondence: Rubén Javier-López, cnViZW4uamF2aWVyLWxvcGV6QHVpYi5ubw==; Nils-Kåre Birkeland, bmlscy5iaXJrZWxhbmRAdWliLm5v