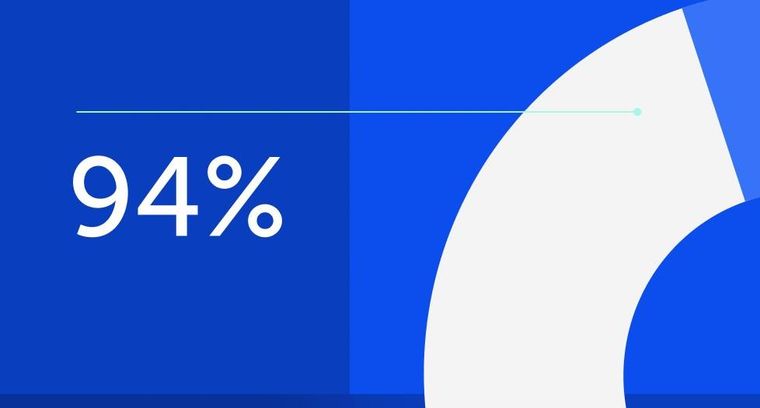
94% of researchers rate our articles as excellent or good
Learn more about the work of our research integrity team to safeguard the quality of each article we publish.
Find out more
ORIGINAL RESEARCH article
Front. Microbiol., 12 February 2025
Sec. Phage Biology
Volume 16 - 2025 | https://doi.org/10.3389/fmicb.2025.1496919
Background: Mastitis is a widespread disease on a global scale, significantly impacting the dairy industry. Mastitis in dairy cattle is caused by over 150 different bacteria, with Staphylococcus aureus (S. aureus) playing a significant role in financial losses, problems with animal welfare, and challenges with food safety. Phage treatment is thus being investigated as an effective replacement for reducing contaminants and illnesses caused by bacteria. In this study, we identified a phage UHP46, that effectively targets mastitis-causing S. aureus.
Methods: S. aureus S46 was used to screen for the wastewater lytic phages. The isolated lytic phage UHP46, which formed clear plaques and spots, was further characterized.
Results: Phage UHP46, belonging to the Herelleviridae family, forms clear, circular plaques in bacterial lawn. UHP46 showed stability under various range of temperature and pH levels, with maximum activity observed at pH 7 and temperature 37°C. Genomic analysis revealed that phage UHP46 is a dsDNA virus with an approximate genome size of 139,731 bp, and it encodes 72 proteins with known functions and 136 hypothetical proteins. One-step growth curve analysis indicated latent period of approximately 20 mins and burst size of about 27 progeny/cell. In organic stability test, UHP46 showed stability in DMSO and acetone. Furthermore, it effectively inhibited S. aureus growth for up to 16 h, suggesting its suitability for therapeutic applications against S. aureus infections.
Conclusion: These findings suggest that phage UHP46 could serve as a promising alternative to antibiotics for managing S. aureus- induced mastitis, contributing to dairy production and improved animal health.
Bovine mastitis, recognized by mammary gland’s inflammation, results from microbial infection or physical injury (Zhao and Lacasse, 2008). It is responsible for poor quality and reduced yield of milk, which accounts for substantial economic loss in dairy industries (Moschovas et al., 2023). Bovine mastitis is predicted to have an annual cost of failure of approximately 147 dollars linked to each cow, globally, with culling and milk yield associated losses making up 11–18 percent of the profit margin coming from each cow (Hogeveen et al., 2019). Decreased milk production due to mammary tissue damage results in 70% of the total losses (Zhao and Lacasse, 2008).
Mastitis is caused by a variety of pathogens, with staphylococci, enterobacteria, and streptococci accounting for the majority of infections (Haxhiaj et al., 2022). Mastitis is generally divided into two forms, clinical and subclinical infections (Cao et al., 2023), and the pathology might be environmental or contagious, based on the method of transmission and primary reservoir (Gomes et al., 2016). The main environmental pathogens causing mastitis include Klebsiella spp., Escherichia coli, and Streptococcus uberis. On the other hand, Streptococcus agalactiae and S. aureus are the examples of contagious microorganisms, and their main source is the infected mammary gland of cows (Gomes and Henriques, 2016). In dairy animals, S. aureus is most commonly associated with mastitis, which is hard to treat and also presents high risk of reoccurrence. Researchers have recognized S. aureus as the leading cause of mastitis causing pathogen due to its various characteristics such as higher antibiotic resistance, significant virulence factors, and the capacity to induce long-term infections (Hoque et al., 2018). S. aureus uses diverse virulence factors such as different toxins (exfoliative toxins Eta, Etb, toxic shock syndrome toxin-1 TSST-1, and enterotoxins SEA to SEQ), cell surface structures (intercellular adhesion genes A and D, and clumping factor A clfA), and immune evasion molecules (coagulase, protein A, leucocidins, and haemolysins) (Monistero et al., 2018).
Frequent mastitis infections in dairy animals lead to the increased use of antibiotics. The unregulated and unchecked consumption of antibiotics results in the development and spread of resistant bacteria (White and McDermott, 2001). Moreover, bacteria residing intracellularly within the mammary gland are challenging to treat due to their limited contact with antibiotics. In case of S. aureus, the current rate of cure with licensed antibiotics (such as pirlimycin) is about 10–30% (Gomes and Henriques, 2016). In addition, ability of pathogenic bacteria to grow as biofilms presents a key factor attributed to recurrence of mastitis infections and antibiotic resistance (Olson et al., 2002). Therefore, continuous rise in antibiotic-resistant bacteria urges scientists to develop alternative therapeutic strategies, and phage therapy has shown high efficacy in eradicating resistant pathogens (Li and Zhang, 2014). Bacteriophages, commonly called as phages, are the viruses which target bacterial cells by delivering their genomic content inside the host (Jamal et al., 2019). Phages are classified as lysogenic or lytic on the basis of their life cycle (Inal, 2003). They are found in almost every environment, for instance, ocean water is believed to have approximately 107 phage particles per milliliter (Wommack and Colwell, 2000), and total estimated phage count in biosphere is about 1031 (Mushegian, 2020). Phages offer several advantages over antibiotics based on their high host specificity, which allows them to protect the normal microflora (Kutateladze and Adamia, 2010).
The potential of phages has been investigated against S. aureus as biocontrol agents in food products (O’Flaherty et al., 2005; Wen et al., 2023), and as therapeutic agents in controlling mastitis (Geng et al., 2020). A number of investigations have proved that phages possess strong bactericidal potential against MDR strains of S. aureus (Varela-Ortiz et al., 2018), and phage testing in animal models infected with S. aureus has also shown promising result (Breyne et al., 2017). Continuous growth of phages in natural environments allow them to exhibit significant genetic diversity and highly dynamic nature, which ultimately allows researchers to explore different phages for MDR bacteria (Hatfull et al., 2022). Accordingly, the main aim of this study was to investigate the efficacy of lytic phages against mastitis-causing S. aureus, and determine the effect of different environmental conditions on the activity of phage. The genomic analysis of phage was also performed to better understand its phylogenetic association and structural components.
Following the guidelines set out by the National Mastitis Council (NMC 2001), milk samples were aseptically collected from dairy farms in the regions of Khyber Pakhtunkhwa, Pakistan (NMC 2001). A milk sample (1 mL) was transferred to a California Mastitis Test (CMT) paddle, and the same amount of CMT reagent (Immucell, United States) was added. The mixture was gently swirled for 20–30 s, after which results were recorded according to the protocol. The CMT-positive milk samples were further processed for microbiological analysis. To isolate S. aureus from milk, samples were enriched by incubating 1 mL of milk with 5 mL of Brain Heart Infusion broth and incubated the mixture for 24 h at 37°C (Hegde et al., 2013). An aliquot of each milk sample (20–100 μL) was spread on Mannitol Salt agar to isolate Gram-positive bacteria. Gram staining, coagulase, catalase, Voges-Proskauer (VP), urease, indole, and methyl Red tests were performed to confirm the presence of S. aureus (Talaiekhozani et al., 2015). Using the phenol-chloroform isoamyl alcohol method, genomic DNA was extracted from all biochemically verified S. aureus strains. Additionally, 16S rDNA sequence analysis was carried out to identify microbes (Saruta et al., 1997). In accordance with CLSI 2019 standards, the Kirby-Bauer disk diffusion assay was used to evaluate the antibiotic susceptibility profile of the S. aureus isolates (Humphries et al., 2019).
Water samples were collected from various sources, including wastewater and animal farms, to obtain S. aureus specific phage. The water samples were centrifuged at 21,380 × g for 15 min to separate the phage from other particles. Then the supernatant was filtered through 0.45 μm syringe filter, and the filtrates were stored at 4°C for further processing (Emencheta et al., 2022).
A bovine S. aureus strain, S46, isolated from raw milk was cultured on Nutrient agar and incubated at 37°C for 24 h. After the incubation, colonies were transferred to Nutrient broth and incubated further for 24 h. Then, 20 mL of bacterial culture was mixed with 25 mL of wastewater supernatant. An additional 20 mL of Nutrient broth was added to the mixture, which was then incubated at 37°C for 24 h at 200 rpm in a shaking incubator (Heidolph Unimax 1010).
After incubation, the mixture was centrifuged at 7,024 × g for 15 min at 4°C. The supernatant was filtered through 0.22 μm syringe filter. For the initial confirmation of phage in the sample, spot assay was done. Briefly, the bacterial lawn was prepared by mixing 3 to 5 mL of 0.7% semi-solid agar (containing peptone 5 g/L, beef extract 3 g/L, sodium chloride 5 g/L, agar 7 g/L) with 100 μL of an early exponential phase bacterial culture. This mixture was then poured onto pre-prepared Nutrient agar plates, spread evenly to cover the surface, and allowed to dry for 3–5 min. Using a pipette, 10 μL aliquots of phage samples were spotted on the agar overlay surface. The plates were incubated at 37°C for up to 24 h. Clear zone on the bacterial lawn was presumptive of specific phage presence. Plaque assay was carried out for purification and subsequent quantification of phage (Alvi et al., 2020).
Phage was purified by picking a single clear plaque with the help of a sterile tip followed by re-suspension in SM buffer. The phage was re-propagated by mixing the suspension with the log phase host bacteria followed by centrifugation and filtration. Through double layer agar method, plaque assay was performed 10–12 times, and the phage titer was expressed as plaque-forming units per milliliter (PFU/mL). The isolated phage was stored in Nutrient broth at 4°C for short-term storage, and − 80°C for long-term storage with 50% glycerol added (Alvi et al., 2020).
Morphological features of phage UHP46 were determined using transmission electron microscopy (TEM). Briefly, the purified phage lysate (15 μL) was loaded on to carbon coated copper grids, allowed to dry, and treated with phosphotungstic acid (PTA). After removing the excess liquid, the stained copper grid was observed under FEI T20 transmission electron microscope (FEI, Hillsboro, OR).
In vitro reduction of bacterial growth by phage was determined using a bacterial growth reduction assay. UHP46 (3 × 107 PFU/mL) was added to S46 strain cultured in Nutrient broth at multiplicity of infection (MOI) of 1, and the mixture was incubated with shaking at 37°C. The bacterial growth reduction ability of UHP46 was monitored by measuring optical density at 600 nm (OD600) every 2 h, with the growth of S46 control, compared over a 16-h period (Khawaja et al., 2016). The data was analyzed through non-parametric Mann–Whitney test using GraphPad prism software, and the p-values were calculated using a T-test.
The pH stability of UHP46 was evaluated by adjusting SM buffer to pH levels of 2, 4, 6, 7, 8, 10, and 12 using either 1 M HCl or 1 M NaOH, with a known titer of 4.0 × 107 PFU /mL. The phage was then suspended in 1 mL of adjusted SM buffer and incubated for 1 h. The pH was then neutralized by preparing serial dilutions in SM buffer (pH 7) and the phages were enumerated by double layer agar method (Hammerl et al., 2014). For thermal stability assessment, 1 mL aliquots of UHP46 having viral titer of 9 × 107 PFU/mL were exposed independently to a range of temperatures (20, 25, 30, 37, 45, 55, 65, 75, and 85°C) for 1 h. Phage titer was calculated through plaque assay afterward to evaluate temperature effects on stability (Asif et al., 2020). The data for pH and thermal stability of UHP46 was analyzed using GraphPad prism software, and the p-values were calculated using a one-way ANOVA test.
One-step growth curve assay for UHP46 was performed as previously described (Vukotic et al., 2020). The experiment was performed at an MOI of 0.01 (3 × 109 CFU/mL and 3 × 107 PFU/mL). The bacteria and phage were added in equal volumes (500 μL each) and incubated at 37°C for 5 min followed by centrifugation at 13,000 × g for 1 min. The supernatant was discarded and the pellet was suspended in 100 mL Nutrient broth. During the subsequent 30-min incubation (starting from the point of dilution), 1 mL sample was collected at every 5 min interval and the phage titer was determined by double layer agar overlay method. The experiment was repeated three times, and burst size was subsequently calculated.
The stability of UHP46 in various organic solvents was determined by the method previously described by Fikadu et al. (2024) with some modifications. After mixing an equal volume of phage UHP46 with ethanol, methanol, DMSO, acetone and xylene, the mixture was incubated for 1 h at 37°C. As a control, SM buffer was mixed with phage in equal volumes. Once the phage and solvent combination had been incubated, the phage titer was determined using the double agar-layer technique (Fikadu et al., 2024).
The host range of UHP46 against several pathogenic strains of S. aureus was determined by modifying the approach by Alvi et al. (2020). The activity of UHP46 was checked using the double agar overlay method, and the plates were examined to determine if any inhibition zones had formed. The inhibition zones were categorized as clear, turbid, or absent. The activity of the phage was tested against 29 S. aureus strains isolated from bovine mastitis milk.
The stability of phage UHP46 in heat-treated milk was evaluated as previously described, with some modifications (Ávila et al., 2023). Briefly, raw milk was sterilized by heating the milk to 110°C for 20 min and subsequently exposing it to UV light at 245 nm for 15 min to remove contaminants. An initial check for bacterial load in milk using serial dilution and culturing on MSA media was performed. Sterile test tubes containing 1 mL milk with a final phage titer of 2 × 109 PFU/mL were incubated at 4°C, 25°C, and 37°C. After 4 h and 24 h, phage aliquots were taken for double-layer agar assay to determine the phage titer.
The phenol-chloroform-isoamyl alcohol (PCI) method was used to extract phage DNA (Sambrook and Russell, 2006). For this purpose, DNase I (1 U) and RNase A (100 μg) were mixed with a 1 mL aliquot of filtered phage lysate (4.6 × 1012 PFU/mL), and the mixture was incubated for 4 h at 37°C. The mixture was placed in a water bath at 75°C for 10 min in order to denature the enzyme (DNase and RNase). Next, 50 μL of 10% SDS, 2.5 μL of proteinase K (20 mg/mL), and 40 μL of 0.5 M EDTA were added to the mixture. The sample was then incubated for 1 h at 55°C, with 20-min intervals of gentle mixing. After this, two microcentrifuge tubes containing equal amounts of lysate and PCI were centrifuged for 10 min at 12,000 × g. The top layer was carefully removed and transferred to a new microcentrifuge tube after centrifugation. Next, 50 μL of 3 M sodium acetate and 1 mL of ice-chilled 95% ethanol were added to each tube. After placing on ice for 5 min, the tubes were centrifuged again for 10 min at 12,000 × g. Subsequently, the pellet was rinsed with 500 μL of 70% ethanol and centrifuged at 12,000 × g for 5 min. The tubes were inverted and allowed to dry on blotting paper after the supernatant was disposed. After that, the DNA pellet was dissolved for 15–20 min in 50 μL of nuclease-free water.
Whole-genome sequencing was performed commercially by Macrogen Korea on an Illumina sequencing platform. SPAdes (4.0.0) was employed in assembling sequencing reads obtained after sequencing of whole genome. RAST server was used to annotate the assembled genome (Aziz et al., 2008). The predicted open reading frames (ORFs) were further analyzed through Interproscan,1 and BLASTp.2 Proteins containing transmembrane helices and signal peptides were identified using TMHMM,3 and SignalIP-5.0.4 Genes encoding for tRNAs were scanned by using tRNAscan-SE v. 2.0.5 Isoelectric point and molecular weight of the UHP46 proteins was calculated through the Expassy ProtParam tool.6 The PhageTerm program, which is part of Galaxy CPT Public7 was used to identify genomic termini and predict the head packaging mechanism employed by phages. BACPHLIP, integrated in Galaxy CPT Public (see Footnote 7) was used to assess the virulence score of UHP46. UHP46 genome was uploaded to the NCBI GenBank database with assigned accession number PP995776. VICTOR database8 and MEGA119 were used for phylogenetic analysis of UHP46 compared with other related phages. Furthermore, PhageAI10 and Phagenomics11 were used to identify genomic features of UHP46. Antibiotic resistance, and virulence associated genes in UHP46 genome were screened by using ResFinder 4.6.0,12 and VirulenceFinder 2.0,13 respectively.
Naturally occurring strains of S. aureus were identified to determine the most effective phage for the controlling or preventing bovine mastitis. S. aureus isolates were obtained from raw milk samples collected from dairy farms with cases of clinical mastitis and antibiotic susceptibility profile of the S. aureus isolates is presented in Table 1. A virulent phage UHP46 specific to S. aureus S46 was isolated from wastewater, showing clear spots on the bacterial lawn (Figure 1A). UHP46 produced transparent, circular plaques with a diameter of 1–2 mm, as seen in Figure 1B. The single plaque was propagated for further physiological and genetic analysis, after purification based on their morphology. TEM analysis indicated that UHP46 has a long, tail 170 nm in length, and icosahedral head with approximately 52 nm in diameter (Figure 1C). Based on these morphological features, UHP46 can be classified as a member of Siphoviridae family of the class Caudovirales phage.
Figure 1. Phage UHP46 plaques on a S. aureus lawn. (a) The spot test showed a distinct lysis region. (b) After the purified phages were plated on double-layer agar, clear plaques were observed. (c) Transmission electron microscopic image of UHP46 shows long tail, with an icosahedral head.
The phage activity against S. aureus was assessed over a 16-h period as shown in Figure 2. Initial OD600 values were 0.036 for the control (bacteria only) and 0.034 for the phage-treated sample. Significant differences in bacterial growth emerged over time. After 6 h, OD600 was 0.4 for the control and 0.2 for the phage-treated sample. At 16 h, the control reached an OD600 of 1.08 while the phage-treated sample was 0.44. These results demonstrate that phages effectively reduced bacterial growth, indicating their therapeutic potential against S. aureus.
Figure 2. Assay for bacterial growth reduction for UHP46, showing a reduction in S. aureus growth in the presence of the UHP46 phage in comparison to the uninfected bacterial cells (control). Results are the mean values from three independent experiments. The reduction of bacteria in the phage treated group is significant having a p-value of 0.005.
UHP46 demonstrated stability at 20, 25, 30, 37, and 45°C, with a significant titer decrease at 55°C as seen in Figure 3 (p-value < 0.0001). Although UHP46 remained stable at a temperature range of 20–55°C, phages held at 55°C showed the lowest stability, while phages stored at and below 37°C showed the maximum stability.
Figure 3. Effect of temperature on stability of phage UHP46. Results are the mean values with SD indicated by error bars from three independent experiments. There is no significant difference in the phage viability from 20 to 37°C.
Phage UHP46 demonstrated stability at various pH values (4, 6, 7, 8, and 10) as shown in Figure 4. The optimum pH for phage UHP46 was found to be 6–8. At pH 10, a two-log decrease in titer of UHP46 was observed whereas a significant decrease in titer was noticed at pH 4 and 12 (p-value < 0.0001). However, no viable phage was found at pH 2.
Figure 4. Effect of pH on stability of phage UHP46. Results are the mean values with SD indicated by error bars from three independent experiments. A significant decrease in the phage titer was observed at pH 2 and 12.
Following the infection of the host cell, a one-step growth curve was established to assess the proliferative ability of phage UHP46. As illustrated in Figure 5, phage UHP46 exhibited a latent period of 20 min and a burst size of 27 ± 1 virions per cell.
Figure 5. The latent period, or the interval between adsorption and the start of the first burst, was measured in the one-step growth curve assay of phage UHP46. The ratio of the total number of released phage particles to the total number of infected bacterial cells during the latent phase was used to calculate the burst size.
UHP46 organic solvent stability under similar exposure conditions to xylene, methanol, ethanol, acetone, and DMSO was observed. Control group exhibited a baseline viability of 1.78 × 1010 PFU/mL, with methanol resulting in 7.5 × 109 PFU/mL, xylene showing 1.63 × 1010 PFU/mL, ethanol displaying 6.4 × 109 PFU/mL, acetone yielding 1.4 × 1010 PFU/mL, and DMSO resulting in 1.73 × 1010 PFU/mL (Figure 6). These distinct responses highlight the differential susceptibility of UHP46 to the tested solvents, providing valuable insights into their viability under varied exposure conditions.
Figure 6. Stability of S. aureus phage UHP46 at different polar and non-polar organic solvents. Results are the mean values with SD indicated by error bars from three independent experiments.
Host range analysis of UHP46 demonstrated its lytic activity against 13.7% (4/29) of tested S. aureus strains. The analysis revealed clear zones in four of the tested strains, indicating susceptibility to the phage. The detailed results are shown in Table 2. Phage UHP46 was found to be highly selective and specific to a limited number of S. aureus isolates.
UHP46 was found stable at 4°C, 25°C, and 37°C with no significant reduction in phage titer up to 24 h as shown in Figure 7.
Figure 7. Stability of UHP46 phage in milk. Results are the mean values with SD indicated by error bars from three independent experiments.
UHP46 genome is a double stranded DNA molecule, which is 139,731 bp long and contains G + C content of 31.8%. It has 208 ORFs, among which 72 genes correspond to proteins with identified functions, while 136 ORFs were found uncharacterized, designated as hypothetical. Proteins with predicted functions were included in various categories such as nucleotide metabolism (DNA primase/helicase, RNA polymerase subunit, exonuclease and endonuclease, DNA polymerase), packaging and structural (major capsid protein, tail lysin, tail sheath protein, portal, tail lysin, tail tube, and tape measure and major tail proteins, adsorption-associated tail protein, baseplate protein), and lytic proteins (holin, lysin) (Figure 8). Lytic machinery of UHP46 consists of two phage lysins (encoded by ORF 81 and 82) and one holin encoded by ORF 161. ORF 126 and 31 encode the largest and smallest proteins (hypothetical), with estimated size of ~147 kDa, and ~ 3.8 kDa, respectively. Also, no genes encoding for tRNAs were detected in UHP46 genome. Moreover, 23 genes were found to contain transmembrane helices, while signal P cleavage sites were detected in two genes only (ORF 42 and 60). UHP46 is predicted to be type T5 phage having redundant ends, containing a direct terminal repeat (DTR) region of 12,857 bp. Genome-wide BLAST analysis showed that the UHP46 genome is highly similar to Staphylococcus phage ZCSS1 (MW430345), with 97.61% identity and 99% genome coverage. Phylogenetic analysis also revealed the close association of UHP46 with other Staphylococcus phages such as, vB SscM 1 (NC 047767), and vB SscM 2 (KX171213) (Figure 9A). Furthermore, there were no genes found coding for integrase, or antimicrobial resistance and virulence factors. Phage AI demonstrated that UHP46 belongs to order Caudovirales, family Herelleviridae, and genus Sciuriunavirus. The lytic nature of phage was further confirmed by the higher virulence scores of 0.85, and 98.83 generated by BACPHLIP, and phage AI, respectively. Phylogenetic analysis performed on the basis of amino acid sequences of large terminase and major capsid protein also showed close association of UHP46 with other Staphylococcus phages such as ZCSS1 (MW430345) (Figures 9B,C).
Figure 8. The genome map of UHP46. Circles represent (from the outside) the ORFs, G + C content, GC skew, and genome map scaled in kbp. Putative functional categories are displayed in different colors; lysis (red), structural proteins (dark blue), replication and regulation (brown), and additional function proteins (light blue).
Figure 9. Phylogenetic analysis of UHP46 based on (A) complete genome compared with related phages, and nucleotide sequences of (B) large terminase subunit, and (C) major capsid protein showing relatedness with other phages. The maps were created by using VICTOR database and MEGA11.
In this work, lytic phage UHP46 was isolated from sewage samples and characterized biochemically. In addition, genomic analysis of UHP46 was performed to determine its suitability as an anti-Staphylococcal against bovine mastitis isolates. Over a 16-h test period, UHP46 effectively reduced the growth of S. aureus. The growth of the bacteria in the presence of phage after 16 h was equal to 3 h growth without phage (Figure 2). This explains the significance of the phage UHP46 to control the S. aureus. Previously, phage SPW has demonstrated strong bacteriolytic activity against S. aureus by inhibiting its growth up to 60 h (Kala et al., 2014). One key feature of potential therapeutic phages is to target highly specific pathogenic bacteria, however, high specificity leads to comparatively narrow host range (Zhang et al., 2015). Phage UHP46 showed a narrow host range, infecting only 13.7% of the tested S. aureus strains. However, the host range of phage can be further improved through receptor modifications, or by using cocktail of phages as reported previously (Abedon et al., 2021; Dunne et al., 2021).
The physio-chemical factors such as temperature, and pH affect phages’ infectivity, storage viability, and incidence which is crucial for the success of phage therapy (Pradeep et al., 2022). Phage UHP46 showed good viability at various temperatures (20–55°C), while viability at and below 37°C was found to be maximum (Figure 3). Kraushaar et al. (2013) also reported good stability of three S. aureus phages (PSa1, PSa2, and PSa3) up to 50°C after 1-h treatment at thermal stress (Kraushaar et al., 2013). Moreover, UHP46 demonstrated stability at various pH values (4–10), with maximum phage activity observed at neutral pH (Figure 4). These results are similar to another study which reported stability of S. aureus phage vB_SauS_IMEP5 at pH 3–12 and inactivity of phage at pH 2 (Zhang et al., 2017). Moreover, WV phage showed highest viability at 6–7 pH range, and decreased stability at pH 3–4 (Jiang et al., 2021). Hence, tolerance of phage UHP46 to different environmental conditions indicates its potential to work effectively at normal or near normal body temperature and pH. Furthermore, different organic solvents are used in phage formulations, which necessitates the understanding of any potential harmful effects of these reagents on the stability of phages (Cao et al., 2023). Phage UHP46 retained activity in xylene, acetone, and DMSO, while stability was declined significantly in alcohols including methanol and ethanol. In a similar study, Oduor et al. (2020) reported the inactivation of S. aureus phages Stab20, Stab21, Stab22, and Stab23 in ethanol solution with concentration above 25% vol/vol (Oduor et al., 2020). The viability of E. coli phages was also reported to decrease after exposure to alcohols (Yamashita et al., 2000; Padmesh et al., 2023). It has been suggested that water-miscible organic solvents like alcohol may disrupt the hydrophobicity-hydrophilicity balance of the phage surface proteins, leading to disruption of phage structure and reduced viable phage titer (Cao et al., 2023). Phage stability in DMSO has been shown to vary significantly. The effect of DMSO treatment on two P. aeruginosa phages was investigated, with PPaMa3/19 maintaining 87.7% stability, while PPaMa4/19 lost infectivity with only 4.8% stability (Majdani and Hatefirad, 2022). Phage UHP46, however, remained viable over DMSO treatment, indicating its potential to sustain in environments where DMSO is used as a solvent or preservative. Phage UHP46 also showed good stability in acetone as reported in previous similar studies (Pallavi et al., 2021). Moreover, UHP46 was found stable after treatment with xylene, indicating its potential to be preserved in diverse range of solvents. The stability profile of UHP46 in above solvents may help in the selection of appropriate solvents for the optimization of phage formulation in therapeutic applications. Furthermore, UHP46 showed good stability in milk over a 24-h test period which indicates its potential to be used in milk applications. Similarly, significant stability of Salmonella phage ZCSE6 was reported when the milk was stored at 4°C for 6 days (Abdelsattar et al., 2021). Burst size and the latent period is an important criterion for phage to be used as therapeutic. Phages with the low latent period are considered good. UHP46 proliferates effectively in the targeted S. aureus strain, exhibiting latent period of 20 min, and a burst size of 27 ± 1 progeny/cell. In another study, S. aureus phage JD419 demonstrated burst size of 33 phages generated per cell (Feng et al., 2021).
Based on the genomic analysis, UHP46 is a member of the Herelleviridae family. Herelleviridae (formerly a part of Myoviridae) phages infecting S. aureus strains are commonly used for therapeutic purposes due to their effective lysis ability against MDR pathogens (Kornienko et al., 2023). The genome of UHP46 contains 139,731 bp, which is within the range of other Herelleviridae phages characterized against S. aureus, such as the 137,950 and 138,307 bp genomes of KSAP7 and KSAP11, respectively (Kitamura et al., 2020). Whole genome comparison revealed that UHP46 has 95–97% nucleotide sequence identity to Staphylococcus phages vB_SscM-1 (NC_047767.1), vB_SscM-2 (KX171213.1), and ZCSS1 (MW430345.1). The UHP46 genome carries terminal redundancies in the form of 12,857 bp direct terminal repeats, indicating that UHP46 follows T5 phage packaging model (long DTR) as reported in case of Staphylococcus phage vB_SauM_VL10 (Lerdsittikul et al., 2024). In this packaging system, terminase recognizes the specific sequences on the phage DNA where DTR are generated (Garneau et al., 2017), and it also minimizes the possibility of the unwanted host transduction of virulence or AMR genes (Pradal et al., 2023). In fact, UHP46 genome showed no virulence, toxin or antibiotic resistance genes, suggesting its potential to be used safely in clinical trials.
The majority of the UHP46 encoded proteins are hypothetical. This is commonly observed in annotated phage genomes, and the possible reasons may include the low characterization of phage proteins, and the wide phage diversity (Pradal et al., 2023). The UHP46 genes with predicted biological functions could be categorized into different groups such as replication and metabolism-associated genes, lysis genes, and structural genes. Multiple genes involved in replication and metabolism were identified in the UHP46 genome including RNA polymerase, and DNA polymerase which suggest that UHP46 is independent of the host machinery for transcription and DNA replication. Additionally, ORF142 was predicted to be a bifunctional protein DNA helicase/primase which is responsible for the unwinding and catalytic synthesis of DNA (Nazir et al., 2022). ORF72 encodes HNH endonuclease, which have been reported to play crucial role in infection, reproduction, and life cycle of phages (Kala et al., 2014). UHP46 genome also contains genes encoding RusA-like Holliday junction resolvase (ORF145) and VSR endonuclease (ORF111), which cleave the phosphodiester bonds between the DNA nucleotides, and these proteins are presumed to provide nucleotide monomers required for phage DNA synthesis (Yang et al., 2021).
Among the ORFs, different UHP46 genes were predicted to be involved in the host cell lysis. ORF81 and 82 encode for phage lysin, N-acetylmuramoyl-L-alanine amidase, which disrupts the bacterial peptidoglycan. The UHP46 genome also contains a holin gene, two tail proteins with lysin activity, and a tail lysin. Double-stranded DNA tailed phages (Caudovirales) employ a cassette consisting of holin and endolysin to kill bacterial cells (Wu et al., 2021). Holins enable endolysins to reach the peptidoglycan layer of bacterial cell wall by creating pores in the cell membrane of bacteria. As a result, new phage progeny is released to further infect the other bacterial cells (Lu et al., 2020). Moreover, the G + C content of UHP46 (31.8%) phage was also comparable to that of other S. aureus phages such as vB_SauS_SA2 (31.9%) (Wang et al., 2019). Additionally, two genes encoding terminase large and small subunit proteins were found in the UHP46 genome, and both subunits are considered to form a complete terminase (Liao et al., 2008). Terminase large subunit, a DNA packaging protein, is used for phylogenetic classification of phages due to its universally conserved sequence (Mardiana et al., 2022). Phylogenetic analysis of UHP46 large terminase and major capsid protein indicated its close association with the Staphylococcus phage ZCSS1 (MW430345), which is also classified in Herelleviridae family.
This study investigated the possibility of using the virulent phage UHP46 as a therapeutic agent against S. aureus strains sourced from bovine milk. Morphological and genomic analyses indicated that phage UHP46 resembles members of the Herelleviridae family and lacks any toxin or virulence genes. Based on these findings, we conclude that phage UHP46 could be an effective phage application for targeting S. aureus in bovine mastitis.
The datasets presented in this study can be found in online repositories. The names of the repository/repositories and accession number(s) can be found at: https://www.ncbi.nlm.nih.gov/genbank/, PP995776.
SN: Investigation, Methodology, Writing – original draft. IK: Conceptualization, Investigation, Supervision, Writing – review & editing. JM: Conceptualization, Funding acquisition, Investigation, Methodology, Project administration, Resources, Supervision, Writing – review & editing. MJ: Supervision, Writing – review & editing. IA: Supervision, Writing – review & editing. AA: Investigation, Methodology, Writing – original draft. AmU: Investigation, Methodology, Writing – original draft. ArU: Investigation, Methodology, Writing – review & editing. WS: Investigation, Methodology, Writing – original draft. HK: Investigation, Methodology, Writing – original draft. AK: Conceptualization, Data curation, Software, Supervision, Writing – review & editing.
The author(s) declare that financial support was received for the research, authorship, and/or publication of this article. The authors extend their appreciation to the Higher Education Commission (HEC), Pakistan for financing this study under the project NRPU (14494).
The authors declare that the research was conducted in the absence of any commercial or financial relationships that could be construed as a potential conflict of interest.
All claims expressed in this article are solely those of the authors and do not necessarily represent those of their affiliated organizations, or those of the publisher, the editors and the reviewers. Any product that may be evaluated in this article, or claim that may be made by its manufacturer, is not guaranteed or endorsed by the publisher.
1. ^https://www.ebi.ac.uk/interpro/search/sequence/
2. ^https://blast.ncbi.nlm.nih.gov/Blast.cgi?PROGRAM=blastp&PAGE_TYPE=BlastSearch&LINK_LOC=blasthome
3. ^https://dtu.biolib.com/DeepTMHMM
4. ^https://services.healthtech.dtu.dk/services/SignalP-5.0/
5. ^https://lowelab.ucsc.edu/cgi-bin/tRNAscan-SE2.cgi
6. ^https://web.expasy.org/protparam/
7. ^https://cpt.tamu.edu/galaxy-pub
8. ^https://ggdc.dsmz.de/victor.php#
9. ^https://www.megasoftware.net/dload_win_beta
11. ^https://phagenomics.net/phagenomics_portal/
Abdelsattar, A. S., Safwat, A., Nofal, R., Elsayed, A., Makky, S., and El-Shibiny, A. (2021). Isolation and characterization of bacteriophage Zcse6 against Salmonella spp.: phage application in milk. Biologics 1, 164–176. doi: 10.3390/biologics1020010
Abedon, S. T., Danis-Wlodarczyk, K. M., and Wozniak, D. J. (2021). Phage cocktail development for bacteriophage therapy: toward improving Spectrum of activity breadth and depth. Pharmaceuticals 14:1019. doi: 10.3390/ph14101019
Alvi, I. A., Asif, M., Tabassum, R., Aslam, R., Abbas, Z., and Rehman, S. U. (2020). RLP, a bacteriophage of the family Podoviridae, rescues mice from bacteremia caused by multi-drug-resistant Pseudomonas aeruginosa. Arch. Virol. 165, 1289–1297. doi: 10.1007/s00705-020-04601-x
Asif, M., Alvi, I. A., Tabassum, R., and Rehman, S. U. (2020). Tac1, an unclassified bacteriophage of the family Myoviridae infecting Acinetobacter baumannii with a large burst size and a short latent period. Arch. Virol. 165, 419–424. doi: 10.1007/s00705-019-04483-8
Ávila, M., Sánchez, C., Calzada, J., Mayer, M. J., Berruga, M. I., López-Díaz, T. M., et al. (2023). Isolation and characterization of new bacteriophages active against clostridium tyrobutyricum and their role in preventing the late blowing defect of cheese. Food Res. Int. 163:112222. doi: 10.1016/j.foodres.2022.112222
Aziz, R. K., Bartels, D., Best, A. A., Dejongh, M., Disz, T., Edwards, R. A., et al. (2008). The Rast server: rapid annotations using subsystems technology. BMC Genomics 9:75. doi: 10.1186/1471-2164-9-75
Breyne, K., Honaker, R. W., Hobbs, Z., Richter, M., Żaczek, M., Spangler, T., et al. (2017). Efficacy and safety of a bovine-associated Staphylococcus aureus phage cocktail in a murine model of mastitis. Front. Microbiol. 8:2348. doi: 10.3389/fmicb.2017.02348
Cao, Y., Khanal, D., Kim, J., Chang, R. Y. K., Byun, A. S., Morales, S., et al. (2023). Stability of bacteriophages in organic solvents for formulations. Int. J. Pharm. 646:123505. doi: 10.1016/j.ijpharm.2023.123505
Dunne, M., Prokhorov, N. S., Loessner, M. J., and Leiman, P. G. (2021). Reprogramming bacteriophage host range: design principles and strategies for engineering receptor binding proteins. Curr. Opin. Biotechnol. 68, 272–281. doi: 10.1016/j.copbio.2021.02.006
Emencheta, S., Attama, A., Ezeibe, E., and Onuigbo, E. (2022). Isolation of Escherichia Coli phages from waste waters. Hosts Viruses 9, 12–18. doi: 10.17582/journal.hv/2022/9.2.1.7
Feng, T., Leptihn, S., Dong, K., Loh, B., Zhang, Y., Stefan, M. I., et al. (2021). Jd419, a Staphylococcus aureus phage with a unique morphology and broad host range. Front. Microbiol. 12:602902. doi: 10.3389/fmicb.2021.602902
Fikadu, A., Amankwah, S., Alemu, B., Alemu, Y., Naga, A., Tekle, E., et al. (2024). Isolation and phenotypic characterization of virulent bacteriophages against multidrug-resistant Escherichia coli and its phage-resistant variant from sewage sources. Infect. Drug Resist. 17, 293–303. doi: 10.2147/IDR.S441085
Garneau, J. R., Depardieu, F., Fortier, L.-C., Bikard, D., and Monot, M. (2017). PhageTerm: a tool for fast and accurate determination of phage termini and packaging mechanism using next-generation sequencing data. Sci. Rep. 7:8292. doi: 10.1038/s41598-017-07910-5
Geng, H., Zou, W., Zhang, M., Xu, L., Liu, F., Li, X., et al. (2020). Evaluation of phage therapy in the treatment of Staphylococcus aureus-induced mastitis in mice. Folia Microbiol. 65, 339–351. doi: 10.1007/s12223-019-00729-9
Gomes, F., and Henriques, M. (2016). Control of bovine mastitis: old and recent therapeutic approaches. Curr. Microbiol. 72, 377–382. doi: 10.1007/s00284-015-0958-8
Gomes, F., Saavedra, M. J., and Henriques, M. (2016). Bovine mastitis disease/pathogenicity: evidence of the potential role of microbial biofilms. Pathog. Dis. 74:ftw006. doi: 10.1093/femspd/ftw006
Hammerl, J. A., Jäckel, C., Alter, T., Janzcyk, P., Stingl, K., Knüver, M. T., et al. (2014). Reduction of Campylobacter jejuni in broiler chicken by successive application of group II and group III phages. PLoS One 9:e114785. doi: 10.1371/journal.pone.0114785
Hatfull, G. F., Dedrick, R. M., and Schooley, R. T. (2022). Phage therapy for antibiotic-resistant bacterial infections. Annu. Rev. Med. 73, 197–211. doi: 10.1146/annurev-med-080219-122208
Haxhiaj, K., Wishart, D. S., and Ametaj, B. N. (2022). Mastitis: what it is, current diagnostics, and the potential of metabolomics to identify new predictive biomarkers. Dairy 3, 722–746. doi: 10.3390/dairy3040050
Hegde, R., Isloor, S., Prabhu, K. N., Shome, B. R., Rathnamma, D., Suryanarayana, V. V. S., et al. (2013). Incidence of subclinical mastitis and prevalence of major mastitis pathogens in organized farms and unorganized sectors. Indian J. Microbiol. 53, 315–320. doi: 10.1007/s12088-012-0336-1
Hogeveen, H., Steeneveld, W., and Wolf, C. A. (2019). Production diseases reduce the efficiency of dairy production: a review of the results, methods, and approaches regarding the economics of mastitis. Ann. Rev. Resour. Econ. 11, 289–312. doi: 10.1146/annurev-resource-100518-093954
Hoque, M. N., Das, Z. C., Rahman, A. N. M. A., Haider, M. G., and Islam, M. A. (2018). Molecular characterization of Staphylococcus aureus strains in bovine mastitis milk in Bangladesh. Int. J. Vet. Sci. Med. 6, 53–60. doi: 10.1016/j.ijvsm.2018.03.008
Humphries, R. M., Abbott, A. N., and Hindler, J. A. (2019). Understanding and addressing CLSI breakpoint revisions: a primer for clinical laboratories. J. Clin. Microbiol. 57:e00203-19. doi: 10.1128/jcm.00203-19
Inal, J. M. (2003). Phage therapy: a reappraisal of bacteriophages as antibiotics. Arch. Immunol. Ther. Exp. 51, 237–244
Jamal, M., Bukhari, S., Andleeb, S., Ali, M., Raza, S., Nawaz, M. A., et al. (2019). Bacteriophages: an overview of the control strategies against multiple bacterial infections in different fields. J. Basic Microbiol. 59, 123–133. doi: 10.1002/jobm.201800412
Jiang, Y., Xu, Q., Jiang, L., and Zheng, R. (2021). Isolation and characterization of a lytic Staphylococcus aureus phage Wv against Staphylococcus aureus biofilm. Intervirology 64, 169–177. doi: 10.1159/000515282
Kala, S., Cumby, N., Sadowski, P. D., Hyder, B. Z., Kanelis, V., Davidson, A. R., et al. (2014). Hnh proteins are a widespread component of phage Dna packaging machines. Proc. Natl. Acad. Sci. 111, 6022–6027. doi: 10.1073/pnas.1320952111
Khawaja, K. A., Rauf, M., Abbas, Z., and Rehman, S. U. (2016). A virulent phage Jhp against Pseudomonas aeruginosa showed infectivity against multiple genera. J. Basic Microbiol. 56, 1090–1097. doi: 10.1002/jobm.201500764
Kitamura, N., Sasabe, E., Matsuzaki, S., Daibata, M., and Yamamoto, T. (2020). Characterization of two newly isolated Staphylococcus aureus bacteriophages from Japan belonging to the genus Silviavirus. Arch. Virol. 165, 2355–2359. doi: 10.1007/s00705-020-04749-6
Kornienko, M., Bespiatykh, D., Gorodnichev, R., Abdraimova, N., and Shitikov, E. (2023). Transcriptional landscapes of Herelleviridae bacteriophages and Staphylococcus aureus during phage infection: An overview. Viruses 15:1427. doi: 10.3390/v15071427
Kraushaar, B., Thanh, M. D., Hammerl, J. A., Reetz, J., Fetsch, A., and Hertwig, S. (2013). Isolation and characterization of phages with lytic activity against methicillin-resistant Staphylococcus aureus strains belonging to clonal complex 398. Arch. Virol. 158, 2341–2350. doi: 10.1007/s00705-013-1707-6
Kutateladze, M., and Adamia, R. (2010). Bacteriophages as potential new therapeutics to replace or supplement antibiotics. Trends Biotechnol. 28, 591–595. doi: 10.1016/j.tibtech.2010.08.001
Lerdsittikul, V., Apiratwarrasakul, S., Atithep, T., Withatanung, P., Indrawattana, N., Pumirat, P., et al. (2024). Isolation and characterisation of a novel Silviavirus bacteriophage promising antimicrobial agent against methicillin-resistant Staphylococcus aureus infections. Sci. Rep. 14:9251. doi: 10.1038/s41598-024-59903-w
Li, L., and Zhang, Z. (2014). Isolation and characterization of a virulent bacteriophage Spw specific for Staphylococcus aureus isolated from bovine mastitis of lactating dairy cattle. Mol. Biol. Rep. 41, 5829–5838. doi: 10.1007/s11033-014-3457-2
Liao, W., Song, S., Sun, F., Jia, Y., Zeng, W., and Pang, Y. (2008). Isolation, characterization and genome sequencing of phage Mztp02 from Bacillus thuringiensis Mz1. Arch. Virol. 153, 1855–1865. doi: 10.1007/s00705-008-0201-z
Lu, M., Liu, H., Lu, H., Liu, R., and Liu, X. (2020). Characterization and genome analysis of a novel Salmonella phage vB_SenS_Se1. Curr. Microbiol. 77, 1308–1315. doi: 10.1007/s00284-020-01879-7
Majdani, R., and Hatefirad, R. (2022). Isolation and characterization of two lytic bacteriophages against Pseudomonas aeruginosa and evaluation of their antibacterial effects on clinical isolates of Staphylococcus aureus and Escherichia coli in the northwest of Iran. Iran. J. Med. Microbiol. 16, 312–323. doi: 10.30699/ijmm.16.4.312
Mardiana, M., Teh, S.-H., Lin, L.-C., and Lin, N.-T. (2022). Isolation and characterization of a novel Siphoviridae phage, vB_AbaS_Tcup2199, Infecting Multidrug-Resistant Acinetobacter baumannii. Viruses 14:1240. doi: 10.3390/v14061240
Monistero, V., Graber, H. U., Pollera, C., Cremonesi, P., Castiglioni, B., Bottini, E., et al. (2018). Staphylococcus aureus isolates from bovine mastitis in eight countries: genotypes, detection of genes encoding different toxins and other virulence genes. Toxins 10:247. doi: 10.3390/toxins10060247
Moschovas, M., Pavlatos, G., Basdagianni, Z., Manessis, G., and Bossis, I. (2023). A cross-sectional study of risk factors affecting Milk quality in dairy cows. Animals 13:3470. doi: 10.3390/ani13223470
Mushegian, A. R. (2020). Are there 1031 virus particles on earth, or more, or fewer? J. Bacteriol. 202:e00052-20. doi: 10.1128/jb.00052-20
Nazir, A., Qi, C., Shi, N., Gao, X., Feng, Q., Qing, H., et al. (2022). Characterization and genomic analysis of a novel Drexlervirial bacteriophage Ime268 with lytic activity against Klebsiella pneumoniae. Infect. Drug Resist. 15, 1533–1546. doi: 10.2147/IDR.S347110
O’Flaherty, S., Coffey, A., Meaney, W., Fitzgerald, G., and Ross, R. (2005). Inhibition of bacteriophage K proliferation on Staphylococcus aureus in raw bovine milk. Lett. Appl. Microbiol. 41, 274–279. doi: 10.1111/j.1472-765X.2005.01762.x
Oduor, J. M. O., Kadija, E., Nyachieo, A., Mureithi, M. W., and Skurnik, M. (2020). Bioprospecting Staphylococcus phages with therapeutic and bio-control potential. Viruses 12:133. doi: 10.3390/v12020133
Olson, M. E., Ceri, H., Morck, D. W., Buret, A. G., and Read, R. R. (2002). Biofilm bacteria: formation and comparative susceptibility to antibiotics. Can. J. Vet. Res. 66, 86–92.
Padmesh, S., Singh, A., Chopra, S., Sen, M., Habib, S., Shrivastava, D., et al. (2023). Isolation and characterization of novel lytic bacteriophages that infect multi drug resistant clinical strains of Escherichia coli. Environ. Sci. Pollut. Res. 31, 57327–57337. doi: 10.1007/s11356-023-28081-z
Pallavi, B., Puneeth, T. G., Shekar, M., and Girisha, S. K. (2021). Isolation, characterization and genomic analysis of vB-AhyM-Ap1, a lytic bacteriophage infecting Aeromonas hydrophila. J. Appl. Microbiol. 131, 695–705. doi: 10.1111/jam.14997
Pradal, I., Casado, A., Del Rio, B., Rodriguez-Lucas, C., Fernandez, M., Alvarez, M. A., et al. (2023). Enterococcus faecium bacteriophage vB_EfaH_163, a new member of the Herelleviridae family, reduces the mortality associated with an E. faecium vanR clinical isolate in a galleria mellonella animal model. Viruses 15:179. doi: 10.3390/v15010179
Pradeep, A., Ramasamy, S., Veniemilda, J., and Vinodkumar, C. S. (2022). Effect of Ph and temperature variations on phage stability-a crucial prerequisite for phage therapy. Int. J. Pharm. Sci. Res. 13, 5178–5182. doi: 10.13040/IJPSR.0975-8232.13(12).5178-82
Sambrook, J., and Russell, D. W. (2006). Purification of nucleic acids by extraction with phenol:chloroform. CSH Protoc. 2006:pdb.prot4455. doi: 10.1101/pdb.prot4455
Saruta, K., Matsunaga, T., Kono, M., Hoshina, S., Ikawa, S., Sakai, O., et al. (1997). Rapid identification and typing of Staphylococcus aureus by nested Pcr amplified ribosomal Dna spacer region. FEMS Microbiol. Lett. 146, 271–278. doi: 10.1111/j.1574-6968.1997.tb10204.x
Talaiekhozani, A., Alaee, S., and Ponraj, M. (2015). Guidelines for quick application of biochemical tests to identify unknown bacteria. SSRN Electr. J. 2, 65–82. doi: 10.2139/ssrn.4101035
Varela-Ortiz, D. F., Barboza-Corona, J. E., González-Marrero, J., León-Galván, M. F., Valencia-Posadas, M., Lechuga-Arana, A. A., et al. (2018). Antibiotic susceptibility of Staphylococcus aureus isolated from subclinical bovine mastitis cases and in vitro efficacy of bacteriophage. Vet. Res. Commun. 42, 243–250. doi: 10.1007/s11259-018-9730-4
Vukotic, G., Obradovic, M., Novovic, K., Di Luca, M., Jovcic, B., Fira, D., et al. (2020). Characterization, antibiofilm, and depolymerizing activity of two phages active on carbapenem-resistant Acinetobacter baumannii. Front. Med. 7:426. doi: 10.3389/fmed.2020.00426
Wang, J., Zhao, F., Sun, H., Wang, Q., Zhang, C., Liu, W., et al. (2019). Isolation and characterization of the Staphylococcus aureus bacteriophage vB_SauS_Sa2. Aims Microbiol. 5, 285–307. doi: 10.3934/microbiol.2019.3.285
Wen, H., Yuan, L., Ye, J.-H., Li, Y.-J., Yang, Z.-Q., and Zhou, W.-Y. (2023). Isolation and characterization of a broad-spectrum phage Sapyzu11 and its potential application for biological control of Staphylococcus aureus. Qual. Assurance Safety Crops Foods 15, 32–48. doi: 10.15586/qas.v15i2.1238
White, D. G., and Mcdermott, P. F. (2001). Emergence and transfer of antibacterial resistance. J. Dairy Sci. 84, E151–E155. doi: 10.3168/jds.S0022-0302(01)70209-3
Wommack, K. E., and Colwell, R. R. (2000). Virioplankton: viruses in aquatic ecosystems. Microbiol. Mol. Biol. Rev. 64, 69–114. doi: 10.1128/MMBR.64.1.69-114.2000
Wu, Z., Zhang, Y., Xu, X., Ahmed, T., Yang, Y., Loh, B., et al. (2021). The Holin-Endolysin lysis system of the Op2-like phage X2 infecting Xanthomonas oryzae pv. Oryzae. Viruses 13:1949. doi: 10.3390/v13101949
Yamashita, M., Murahashi, H., Tomita, T., and Hirata, A. (2000). Effect of alcohols on Escherichia coil phages. Biocontrol Sci. 5, 9–16. doi: 10.4265/bio.5.9
Yang, M., Xia, Q., Du, S., Zhang, Z., Qin, F., and Zhao, Y. (2021). Genomic characterization and distribution pattern of a novel marine Om43 phage. Front. Microbiol. 12:651326. doi: 10.3389/fmicb.2021.651326
Zhang, L., Bao, H., Wei, C., Zhang, H., Zhou, Y., and Wang, R. (2015). Characterization and partial genomic analysis of a lytic Myoviridae bacteriophage against Staphylococcus aureus isolated from dairy cows with mastitis in mid-east of China. Virus Genes 50, 111–117. doi: 10.1007/s11262-014-1130-4
Zhang, Q., Xing, S., Sun, Q., Pei, G., Cheng, S., Liu, Y., et al. (2017). Characterization and complete genome sequence analysis of a novel virulent Siphoviridae phage against Staphylococcus aureus isolated from bovine mastitis in Xinjiang, China. Virus Genes 53, 464–476. doi: 10.1007/s11262-017-1445-z
Keywords: mastitis, Staphylococcus aureus, antibiotic resistance, bacteriophages, phage therapy
Citation: Najeeb S, Khan I, Muhammad J, Jahangir M, Alvi IA, Abbas A, Ullah A, Ullah A, Sajjad W, Khan H and Khan A (2025) Characterization and genomic analysis of a Herelleviridae bacteriophage UHP46 infecting mastitis-causing Staphylococcus aureus. Front. Microbiol. 16:1496919. doi: 10.3389/fmicb.2025.1496919
Received: 15 September 2024; Accepted: 27 January 2025;
Published: 12 February 2025.
Edited by:
Shan Goh, University of Hertfordshire, United KingdomReviewed by:
Gunaraj Dhungana, Meharry Medical College, United StatesCopyright © 2025 Najeeb, Khan, Muhammad, Jahangir, Alvi, Abbas, Ullah, Ullah, Sajjad, Khan and Khan. This is an open-access article distributed under the terms of the Creative Commons Attribution License (CC BY). The use, distribution or reproduction in other forums is permitted, provided the original author(s) and the copyright owner(s) are credited and that the original publication in this journal is cited, in accordance with accepted academic practice. No use, distribution or reproduction is permitted which does not comply with these terms.
*Correspondence: Javed Muhammad, amF2ZWQubXVoYW1tYWRAdW9oLmVkdS5waw==; Anza Abbas, YW56YWFiYmFzMzE5OEBnbWFpbC5jb20=
Disclaimer: All claims expressed in this article are solely those of the authors and do not necessarily represent those of their affiliated organizations, or those of the publisher, the editors and the reviewers. Any product that may be evaluated in this article or claim that may be made by its manufacturer is not guaranteed or endorsed by the publisher.
Research integrity at Frontiers
Learn more about the work of our research integrity team to safeguard the quality of each article we publish.