- 1Key Laboratory of Freshwater Aquatic Genetic Resources, Ministry of Agriculture and Rural Affairs, Shanghai Ocean University, Shanghai, China
- 2National Demonstration Center for Experimental Fisheries Science Education, Shanghai Ocean University, Shanghai, China
- 3Shanghai Engineering Research Center of Aquaculture, Shanghai Ocean University, Shanghai, China
- 4Shanghai Collaborative Innovation for Aquatic Animal Genetics and Breeding, Shanghai Ocean University, Shanghai, China
- 5National Experimental Teaching Demonstration Centre for Aquatic Sciences, Shanghai Ocean University, Shanghai, China
- 6Department of Biology, University of Nebraska at Omaha, Omaha, NE, United States
Understanding the diversity and function of fish gut microbiomes has advanced substantially, yet many aspects remain poorly understood, particularly the interplay among microbiota, host species, and environmental factors in the context of conservation. This review explores the composition and abundance of gut bacterial communities in key aquaculture fish groups—cyprinids, ictalurids (catfish), salmonids, and cichlids (tilapia)—alongside the model organism zebrafish, across diverse geographic regions. The findings highlight environmental habitats and host species as primary determinants of gut microbiome structure, offering a global perspective on these microbial communities. Across all fish groups, the phyla Firmicutes, Fusobacteria, and Proteobacteria consistently dominated, while temperate, sub-equatorial, and sub-tropical regions exhibited the highest microbiome diversity, underscoring the contribution of taxonomic and environmental factors. The gut bacterial diversity of farm-raised fish shows a significant divergence from that of wild-caught fish, reflecting the impacts of ecological and management differences. Understanding the dynamic responses of fish gut microbiota is vital for guiding conservation efforts, safeguarding aquatic biodiversity, and advancing sustainable aquaculture practices. Future research should leverage innovative techniques and integrative approaches, both experimental and theoretical, to uncover the functional roles of microbiomes and predict their responses to environmental changes. Expanding geographic and taxonomic coverage will be critical for creating a comprehensive framework to inform global aquaculture and conservation strategies. Collectively, this perspective highlights the transformative potential of microbiome research in addressing global challenges in aquaculture and conservation biology.
Introduction
Microbiomes play critical roles in maintaining ecosystem health, nutrient cycling, and climate regulation (Lennon et al., 2023). Within aquatic environments, fish, the most diverse group of vertebrates, are host to complex microbial communities that significantly impact their physiology and the health of the surrounding environment (Lorgen-Ritchie et al., 2023). The fish gut microbiome presents an invaluable window into host-microbiota-environment interactions, offering insights with direct implications for aquaculture and conservation. The composition of fish gut microbiota is shaped by a combination of environmental, biological, and behavioral factors, which collectively influence microbial communities across diverse fish species (Nayfach et al., 2021; Bertoncin et al., 2022). These microbiomes are sensitive to environmental conditions, including water temperature, oxygen levels, pH, and salinity, as well as intrinsic factors such as feeding behaviors, life stages, and various anthropogenic influences (Liu et al., 2016; Li et al., 2017a; Du et al., 2019; Zotta et al., 2019; Huang et al., 2020; Mukherjee et al., 2020). The dynamic interplay between fish and their gut microbiota plays a crucial role in shaping fish communities, making this an important area of study for better understanding and managing aquatic biodiversity.
Beyond contributing to essential ecosystem functions, such as nutrient cycling and climate regulation, gut microbiota are also critical for individual health. Alterations in microbial communities can affect phenotypic traits, immune mechanisms, and animal fitness in response to climate change, as physiological functions originate mostly from the gut (Dinan and Cryan, 2016; Mohajeri et al., 2018; Sepulveda and Moeller, 2020). Microbiomes are leveraged to enhance fatty acid production in muscle tissue and improve fish development (Eichmiller et al., 2016; Stephens et al., 2016; Mohajeri et al., 2018; Aldars-García et al., 2021; Asnicar et al., 2021; Chen et al., 2021; Zhang et al., 2022; Yin et al., 2023). The gut microbiome helps protect the intestinal barrier, prevent the overgrowth of opportunistic pathogens, and modulate the host immune system, all of which are crucial for maintaining fish health (Merrifield and Rodiles, 2015; Llewellyn et al., 2016; Nohesara et al., 2023). Conversely, disruption of the microbial balance can result in the proliferation of harmful bacteria, leading to disease outbreaks in aquaculture settings (Talwar et al., 2018; Vargas-albores et al., 2021; Wang et al., 2021). Therefore, understanding and manipulating the fish gut microbiome has become an important strategy for developing sustainable and disease-resistant aquaculture practices.
This review investigates how microbial abundance varies in response to temperature, habitat, and taxonomic differences across major fish groups in global aquaculture. We focus on cyprinids, ictalurids (catfish), salmonids, and cichlids (tilapia), which are economically significant aquaculture species (Lu and Luo, 2020). Additionally, we compare the gut microbiomes of farmed fish, which are raised in controlled environments with standardized diets, with those of wild-caught fish, which interact directly with their natural habitats, to understand how these contrasting conditions influence fish gut microbiomes. This comparative approach will help identify key environmental and dietary factors shaping the gut microbiome and highlight bacterial groups particularly sensitive to these variables. By highlighting these variations and their underlying causes, this review offers valuable insights into the role of the gut microbiome in promoting fish resilience and health under changing environmental conditions. These insights are essential for informing conservation strategies and optimizing sustainable aquaculture practices worldwide.
Fish gut microbiomes varying across habitats, climatic zones, and feeding behaviors
Gut microbiota composition varies among fish taxa, with hosts from the same taxonomic group generally exhibiting more similar gut microbiota than those from different groups; however, biological factors such as feeding habits can lead to remarkable differences within taxa (Huang et al., 2020). Distinct gut microbiome compositions were observed across different fish groups, with Proteobacteria, Fusobacteria, and Firmicutes being the most prevalent (Figures 1A, B). While Actinobacteria was present in cyprinids, salmonids, cichlids, and zebrafish, it was not reported in the catfish group (Figure 1B). Fish species exhibit distinct feeding behaviors across water layers, which shape their gut microbiome composition. Cyprinids, ictalurids (catfish), salmonids, cichlids, and zebrafish, ranging from bottom dwellers to surface feeders, display microbiota variations based on diet and ecological niches (Ang and Petrell, 1998; Rahman et al., 2008; Ramesh and Kiran, 2016; Thomas and Opeh, 2018). The variation in microbiota phyla highlights the impact of feeding behaviors on gut microbiome diversity (Figures 1B, C), emphasizing ecological adaptation (Sinha and Jones, 1967; Magoulick and Lewis, 2002; Watzin et al., 2008).
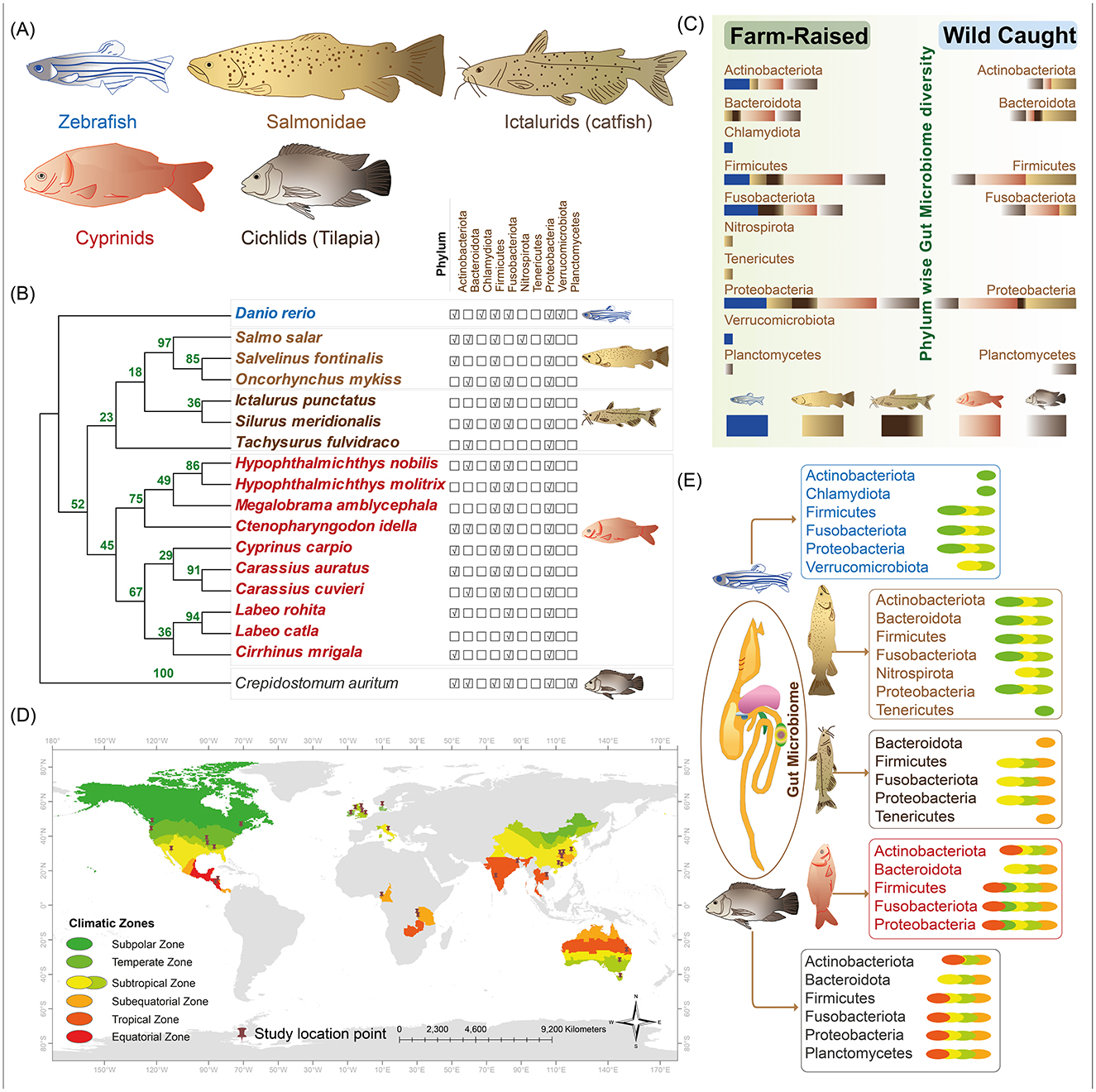
Figure 1. Gut microbiome diversity in four fish groups, farm-raised vs. wild-caught, and across climatic zones. (A) Fish groups and model species: four key fish groups commonly used in aquaculture—cyprinids, ictalurids (catfish), salmonids, and cichlids (tilapia)—are depicted, along with the model organism zebrafish. (B) Gut microbiome composition across different fish groups: phylogenetic relationships among the fish species are shown on the right, alongside dominant bacterial phyla associated with each fish species shown on the left. (C) Microbiome differences between farmed-raised and wild-caught fish: a bar chart illustrates the proportion of each bacterial phylum (represented by bar widths), with bar colors indicating specific fish groups. (D) Sampling sites and climate zones: sampling locations from reviewed studies are mapped globally, with climate zones differentiated by distinct colors. (E) Dominant bacterial phyla and climatic associations: dominant bacterial phyla in various fish groups are shown in relation to climate zones, with the same color scheme as (D).
Studies also revealed the composition of fish gut microbiomes varies based on habitat characteristics and geomorphology, with factors like salinity and the differences between nearshore littoral and offshore profundal zones significantly influencing microbial diversity (Zotta et al., 2019; Huang et al., 2020; Sylvain et al., 2020; Kim et al., 2021; Shang et al., 2021). Farm-raised fishes in controlled environments show higher microbiome abundance, especially of Firmicutes, Fusobacteria, and Proteobacteria, compared to wild-caught fishes, where Firmicutes and Proteobacteria are most abundant (Figure 1C, Table 1).
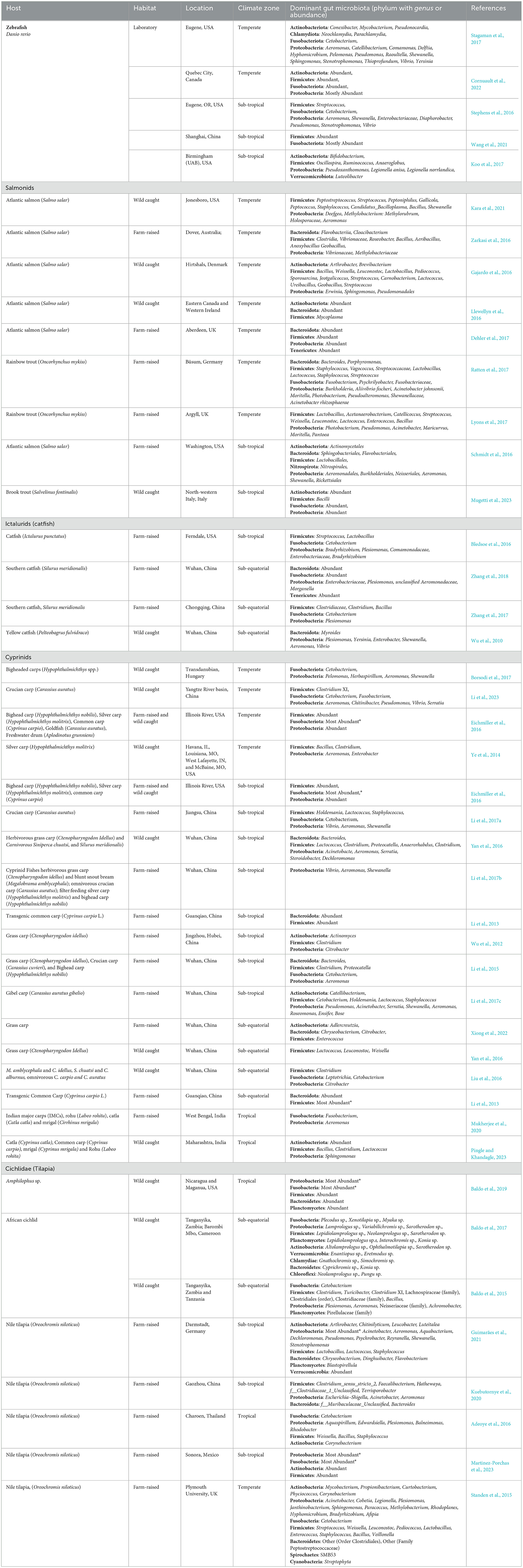
Table 1. Microbial community composition and abundance in zebrafish, salmonidae, ictalurids (catfish), cichlids (tilapia), and cyprinids across various geographic and climate zones.
Temperature is another key factor influencing gut microbiome composition across climate zones. Fish from warmer environments often display greater microbial diversity, with temperature playing a crucial role in shaping species-specific responses (Wong and Rawls, 2012; Kokou et al., 2018). For example, yellow-tail kingfish showed higher gut microbiota richness at 26°C than at 20°C (Soriano et al., 2018), while turbot exhibited greater diversity at 20°C (Guerreiro et al., 2016). In rainbow trout, higher temperatures were associated with a reduction in Firmicutes (Huyben et al., 2018), and in salmon, higher temperatures led to a decrease in Acinetobacter and an increase in pathogenic Vibrio (Ley et al., 2008). Such variations underscore important role of temperature in influencing microbiota, particularly in temperature-sensitive fish (Chevalier et al., 2015). Among those climatic zones, fish from the subtropical region displayed the highest microbial diversity, with tropical, temperate, and sub-equatorial regions following diversity levels (Figures 1D, E, Table 1).
Gut microbiome: an indicator for fish conservation and management strategies
The gut microbiome is increasingly recognized as an important indicator of environmental health and the adaptability of fish populations, offering valuable insights for conservation efforts (Soh et al., 2024). Factors such as geographic location, exposure to contaminants, urbanization, and the introduction of invasive species can significantly disrupt the gut microbiome, impacting essential processes like digestion, metabolism, immunity, and overall health (Zhu et al., 2021a; Clough et al., 2023; Lennon et al., 2023; Lorgen-Ritchie et al., 2023). Such disruptions complicate conservation strategies, particularly for endangered species, by altering microbial diversity and reducing adaptability. Shifts in microbial diversity in response to environmental pollutants or habitat changes can provide early warnings of ecological imbalance, facilitating timely conservation interventions (Zhu et al., 2021a).
Invasive species, such as Nile tilapia, illustrate how microbiome diversity can confer competitive advantages. Compared to native fish, invasive tilapia display higher gut microbial alpha diversity, reduced interspecies microbial competition, and enhanced food utilization, supporting niche expansion and local adaptation (Gu et al., 2020). Similarly, bighead carp and silver carp, major aquaculture species in East Asia, have become invasive in North America, where hybridization in the Mississippi River Basin (MRB) has further increased their adaptability (Wang et al., 2020). Studies suggest that hybrids benefit from a diversified gut microbiome, which, along with genomic adaptability, may facilitate invasion by supporting survival and local adaptation (Wang et al., 2020; Zhu et al., 2021b). These findings underscore the critical role of the gut microbiome in driving ecological success and adaptability in invasive species. As environmental pressures intensify, understanding the interactions between host genetics and microbiome diversity will be essential for managing invasive populations and protecting native biodiversity.
Managing gut microbiota in captive breeding programs can improve reintroduction success rates by enhancing the resilience and ecological fitness of released fish in their natural habitats (Zhu et al., 2021a). Microbiome-based interventions, including probiotics and prebiotics, hold considerable promise for enhancing resilience and adaptability in both farmed and wild fish, reducing mortality rates and reinforcing conservation outcomes (Jin Song et al., 2019; Vargas-albores et al., 2021; de Jonge et al., 2022). This approach is essential for species recovery, as a well-balanced gut microbiome strengthens adaptability, immune function, and overall health in reintroduced populations, enabling them to thrive in challenging environments.
Advancing sustainable aquaculture and implementing effective conservation strategies for vulnerable fish populations hinges on a comprehensive understanding of gut microbiome dynamics. Research has shown that gut microbiota plays a fundamental role in host resilience, immune function, and adaptation to environmental changes, all of which are critical for both farmed and wild fish (Fonseca and Fuentes, 2023; Zhu and Wang, 2023). Fish migration and breeding activities are closely associated with variations in gut microbiome composition, as they drive physiological and environmental changes (Llewellyn et al., 2016; Hamilton et al., 2019; Liu et al., 2021). As conservation and aquaculture practices increasingly incorporate microbiome management, this could become a powerful tool for sustaining biodiversity, aiding species recovery, and supporting sustainable aquaculture.
Embracing microbiome-based solutions strengthens the health and adaptability of individual species and contributes to the stability of entire aquatic ecosystems. Such microbiome-focused approaches have the potential to transform conservation practices by enhancing species survival, mitigating the impacts of invasive species, and restoring ecosystem health. By integrating these strategies, conservation efforts can foster resilient and balanced aquatic environments, paving the way for the long-term sustainability of our aquatic ecosystems.
Discussion and future directions
This review provides a global perspective on the gut microbiome of four major aquaculture fish groups: cyprinids, ictalurids (catfish), cichlids (tilapia), and salmonids. It highlighted microbial composition and diversity across geographic regions and contrasting farmed and wild environmental conditions (Figure 1). By focusing on these important species, we identified dominant phyla and critical patterns that consistently appear across studies. Notably, samples from temperate, sub-equatorial, and subtropical zones exhibited the highest microbial diversity, emphasizing the interplay between taxonomic and environmental factors in shaping the microbiome (Figure 1E, Table 1). These findings underscore the adaptive significance of the gut microbiome in supporting essential functions such as digestion, immunity, and overall health, thereby enhancing the resilience and productivity of aquaculture species.
Limitations and opportunities
While this review provides valuable insights, it is important to acknowledge its limitations. The selection of species and groups, while reasonable, does not fully represent the diversity of fish (Riera and Baldo, 2020). This limitation may restrict the generalizability of our findings, as different species and habitats harbor distinct microbiome compositions. Even within a single taxonomic group, various species inhabiting different niches can possess diverse gut microbiomes, which might have been underestimated in our review. Future research should aim to expand the analysis to include all species and taxonomic groups studied.
Additionally, the focus on microbial composition at the phylum level, while informative, represents a much higher taxonomic resolution. Comparisons at the genus or even at the species level would offer more detailed and biologically meaningful insights, particularly for understanding functional relationships within the microbiome. Furthermore, variability in sampling methods, sequencing depth, and environmental contexts across the reviewed studies introduces potential biases. This heterogeneity complicates cross-study comparisons and limits the ability to draw broad, definitive conclusions. A meta-analysis of raw sequencing data could address these inconsistencies, yielding more robust and statistically validated insights.
A geographic bias in sampling is evident, with most studies conducted in North America and East Asia, while regions such as Central Europe and Africa are underrepresented (Figure 1D, Table 1). This imbalance, similar to patterns observed in the Earth Microbiome Project (Gilbert et al., 2018), underscores the need for increased geographic diversity to achieve a truly global perspective on fish gut microbiomes. Expanding research efforts to include diverse regions and underrepresented species will be crucial for creating a comprehensive and unbiased framework for understanding and leveraging microbiome data in aquaculture and conservation.
Addressing microbiome complexities with innovative, integrated approaches
The study of fish gut microbiomes is inherently complex due to the various influencing variables, such as diet, water quality, temperature, and salinity, which complicate efforts to isolate specific factors affecting microbial community structure (Egerton et al., 2018). The gut microbiome interacts dynamically with the host and the environment, requiring research to move beyond simple associations to uncover complex causal relationships (Xiong et al., 2019). The functional impact of the microbiome depends on the entire ecological network, where diet, environmental factors, and microbial composition interact in interdependent ways (Talwar et al., 2018; Diwan et al., 2022).
To address these challenges, the holobiont approach and multi-omics techniques should be employed. Future research on fish can follow the lead of large-scale microbiome initiatives, such as the Human Microbiome Project (Turnbaugh et al., 2007), to advance fish microbiome studies. For instance, the holobiont model has been used to explore how microbiomes interact with host genomes to drive adaptability and invasiveness in hybrid bighead and silver carp within the Mississippi River Basin (Wang et al., 2020; Zhu et al., 2021b). The integration of multi-omics data can uncover the functional roles of microbiomes in fish health and adaptation by linking microbial genes and metabolic pathways to host physiological traits, such as digestion efficiency, immunity, and stress tolerance.
Beyond experimental and field research, computational modeling and theoretical studies are crucial for enhancing our understanding of microbiomes as integral components of complex ecological networks (Kumar et al., 2019). Modeling microbial interaction networks, predicting the responses of these networks to environmental changes, and simulating diverse scenarios to assess the far-reaching impacts of the microbiome on host resilience and aquaculture productivity are promising avenues for future work. By combining experimental and computational approaches, researchers can unravel the intricate interdependencies among microbiomes, host, and environments, providing deeper insights into ecosystem functioning and advancing practical applications.
Perspectives in aquaculture and conservation
Aquaculture practices, such as integrated pond fish farming and natural fish germplasm resource conservation, have been pivotal in sustainable aquaculture in regions like China (Li et al., 1990; Lu et al., 1997, 2020). Microbiome is likely to play a crucial role in these processes. Comparative analyses of gut microbial communities between natural and pond-cultured populations, as well as within the same pond ecosystem, could unveil the underlying mechanisms.
Further studies on the role of the microbiome in fish nutrition and health are necessary to enhance aquaculture productivity and promote healthier aquatic ecosystems. Developing microbiome-targeted feeds enriched with prebiotics, probiotics, or synbiotics can stimulate beneficial gut microbes and improve fish growth and disease resistance. For example, utilizing probiotics and incorporating alternative protein sources, such as insect meals, can enhance gut health and optimize aquaculture practices (Fonseca and Fuentes, 2023; Hasan et al., 2023). Feed formulations should be optimized based on microbiome profiles to improve nutrient uptake and resilience to pathogens. Insights from studies on wild and hybrid species can inform the design of functional feeds and strategies to enhance aquaculture productivity (Reshma et al., 2018; Cui et al., 2022).
Applying microbiome research to improve recirculating aquaculture systems by optimizing microbial communities in biofilters and water systems is crucial (Rurangwa and Verdegem, 2015; Mugwanya et al., 2021). Studying sediment and water column microbiomes to enhance nutrient recycling and minimize environmental impact is also essential. Promoting integrated multi-trophic aquaculture systems that leverage microbiome interactions across species can contribute to sustainable aquaculture practices (Troell et al., 2009).
Microbiome research is critical for understanding and maintaining wild fish populations. Future research should study the microbiomes of wild fish populations to understand their role in species health and ecosystem stability. Using microbiome monitoring to support species reintroduction programs and mitigate the impacts of invasive species is crucial. Enhancing biodiversity conservation by protecting critical microbial symbionts associated with endangered species is imperative.
Conclusion
The fish gut microbiome represents a promising frontier for advancements in aquaculture and conservation biology. By harnessing the power of microbiome research, we can develop more sustainable aquaculture practices, enhance the resilience of wild fish populations, and safeguard the delicate balance of aquatic ecosystems. Interdisciplinary approaches and innovative technologies will pave the way for transformative solutions to global challenges in aquaculture sustainability and biodiversity conservation.
Author contributions
NK: Writing – original draft, Writing – review & editing. NL: Writing – review & editing. JK: Writing – review & editing. HC: Writing – review & editing. GL: Writing – review & editing, Conceptualization, Writing – original draft. JW: Conceptualization, Writing – original draft, Writing – review & editing. CW: Conceptualization, Writing – original draft, Writing – review & editing.
Funding
The author(s) declare financial support was received for the research, authorship, and/or publication of this article. CW is grateful for funding support from the National Natural Science Foundation of China under grant number: 32172959. GL acknowledges funding support from the Mississippi River Basin Panel on Aquatic Nuisance Species for the Invasive Carp Genetics Project.
Acknowledgments
This review is dedicated in part to the late Louise Bernatchez, our esteemed mentor and long-time collaborator, and Sifa Li for his pioneering work in conserving the four major Chinese carp species. We extend our gratitude to L. Zhu for his foundational contributions and for inviting us to undertake this review. We also thank our lab members for their insightful comments and invaluable contributions.
Conflict of interest
The authors declare that the research was conducted in the absence of any commercial or financial relationships that could be construed as a potential conflict of interest.
Generative AI statement
The author(s) declare that Gen AI was used in the creation of this manuscript. We used generative AI tools to help with English and improve language.
Publisher's note
All claims expressed in this article are solely those of the authors and do not necessarily represent those of their affiliated organizations, or those of the publisher, the editors and the reviewers. Any product that may be evaluated in this article, or claim that may be made by its manufacturer, is not guaranteed or endorsed by the publisher.
References
Adeoye, A. A., Yomla, R., Jaramillo-Torres, A., Rodiles, A., Merrifield, D. L., and Davies, S. J. (2016). Combined effects of exogenous enzymes and probiotic on Nile tilapia (Oreochromis niloticus) growth, intestinal morphology and microbiome. Aquaculture 463, 61–70. doi: 10.1016/j.aquaculture.2016.05.028
Aldars-García, L., Chaparro, M., and Gisbert, J. P. (2021). Systematic review: the gut microbiome and its potential clinical application in inflammatory bowel disease. Microorganisms 9:977. doi: 10.3390/microorganisms9050977
Ang, K. P., and Petrell, R. J. (1998). Pellet wastage, and subsurface and surface feeding behaviours associated with different feeding systems in sea cage farming of salmonids. Aquac. Eng. 18, 95–115. doi: 10.1016/S0144-8609(98)00026-0
Asnicar, F., Berry, S. E., Valdes, A. M., Nguyen, L. H., Piccinno, G., Drew, D. A., et al. (2021). Microbiome connections with host metabolism and habitual diet from 1,098 deeply phenotyped individuals. Nat. Med. 27, 321–332. doi: 10.1038/s41591-020-01183-8
Baldo, L., Pretus, J. L., Riera, J. L., Musilova, Z., Bitja Nyom, A. R., and Salzburger, W. (2017). Convergence of gut microbiotas in the adaptive radiations of African cichlid fishes. ISME J. 11, 1975–1987. doi: 10.1038/ismej.2017.62
Baldo, L., Riera, J. L., Salzburger, W., Barluenga, M., and Costa, R. (2019). Phylogeography and ecological niche shape the cichlid fish gut microbiota in central American and African lakes. Front. Microbiol. 10, 1–19. doi: 10.3389/fmicb.2019.02372
Baldo, L., Riera, J. L., Tooming-Klunderud, A., Albà, M. M., and Salzburger, W. (2015). Gut microbiota dynamics during dietary shift in eastern African cichlid fishes. PLoS ONE 10, 1–23. doi: 10.1371/journal.pone.0127462
Bertoncin, A. P. D. S., Tramonte, R. P., Pinha, G. D., Gentilin-Avanci, C., de Oliveira, M. V. C., and Mormul, R. P. (2022). On the significance of wetlands: three decades of aquatic macroinvertebrate monitoring programs in a Neotropical floodplain. Acta Limnol. Brasil. 34:4721. doi: 10.1590/s2179-975x4721
Bledsoe, J. W., Peterson, B. C., Swanson, K. S., and Small, B. C. (2016). Ontogenetic characterization of the intestinal microbiota of channel catfish through 16S rRNA gene sequencing reveals insights on temporal shifts and the influence of environmental microbes. PLoS ONE 11, 1–22. doi: 10.1371/journal.pone.0166379
Borsodi, A. K., Szabó, A., Krett, G., Felföldi, T., Specziár, A., and Boros, G. (2017). Gut content microbiota of introduced bigheaded carps (Hypophthalmichthys spp.) inhabiting the largest shallow lake in Central Europe. Microbiol. Res. 195, 40–50. doi: 10.1016/j.micres.2016.11.001
Chen, L., Wang, D., Garmaeva, S., Kurilshikov, A., Vich Vila, A., Gacesa, R., et al. (2021). The long-term genetic stability and individual specificity of the human gut microbiome. Cell 184, 2302–2315.e12. doi: 10.1016/j.cell.2021.03.024
Chevalier, C., Stojanović, O., Colin, D. J., Suarez-Zamorano, N., Tarallo, V., Veyrat-Durebex, C., et al. (2015). Gut microbiota orchestrates energy homeostasis during cold. Cell 163, 1360–1374. doi: 10.1016/j.cell.2015.11.004
Clough, J., Schwab, S., and Mikac, K. (2023). Gut microbiome profiling of the endangered Southern Greater Glider (Petauroides volans) after the 2019–2020 Australian Megafire. Animals 13:3583. doi: 10.3390/ani13223583
Cornuault, J. K., Byatt, G., Paquet, M. E., De Koninck, P., and Moineau, S. (2022). Zebrafish: a big fish in the study of the gut microbiota. Curr. Opin. Biotechnol. 73, 308–313. doi: 10.1016/j.copbio.2021.09.007
Cui, X., Zhang, Q., Zhang, Q., Zhang, Y., Chen, H., Liu, G., et al. (2022). Research progress of the gut microbiome in hybrid fish. Microorganisms 10, 1–21. doi: 10.3390/microorganisms10050891
de Jonge, N., Carlsen, B., Christensen, M. H., Pertoldi, C., and Nielsen, J. L. (2022). The gut microbiome of 54 mammalian species. Front. Microbiol. 13, 1–11. doi: 10.3389/fmicb.2022.886252
Dehler, C. E., Secombes, C. J., and Martin, S. A. M. (2017). Environmental and physiological factors shape the gut microbiota of Atlantic salmon parr (Salmo salar L.). Aquaculture 467, 149–157. doi: 10.1016/j.aquaculture.2016.07.017
Dinan, T. G., and Cryan, J. F. (2016). Mood by microbe: towards clinical translation. Genome Med. 8, 36–38. doi: 10.1186/s13073-016-0292-1
Diwan, A. D., Harke, S. N., Gopalkrishna, A., and Panche, A. N. (2022). Aquaculture industry prospective from gut microbiome of fish and shellfish: an overview. J. Anim. Physiol. Anim. Nutr. 106, 441–469. doi: 10.1111/jpn.13619
Du, F., Li, Y., Tang, Y., Su, S., Yu, J., Yu, F., et al. (2019). Response of the gut microbiome of Megalobrama amblycephala to crowding stress. Aquaculture 500, 586–596. doi: 10.1016/j.aquaculture.2018.10.067
Egerton, S., Culloty, S., Whooley, J., Stanton, C., and Ross, R. P. (2018). The gut microbiota of marine fish. Front. Microbiol. 9, 1–17. doi: 10.3389/fmicb.2018.00873
Eichmiller, J. J., Hamilton, M. J., Staley, C., Sadowsky, M. J., and Sorensen, P. W. (2016). Environment shapes the fecal microbiome of invasive carp species. Microbiome 4, 1–13. doi: 10.1186/s40168-016-0190-1
Fonseca, F., and Fuentes, J. (2023). Editorial: microbiome dynamics as biomarkers of welfare status in aquatic species. Front. Physiol. 14, 1–2. doi: 10.3389/fphys.2023.1276351
Gajardo, K., Jaramillo-torres, A., Kortner, T. M., and Merrifield, D. L. (2016). Alternative protein sources in the diet modulate microbiota and functionality in the distal intestine of Atlantic salmon (Salmo salar). Appl. Environ. Microbiol. 83:e02615–16. doi: 10.1128/AEM.02615-16
Gilbert, J. A., Jansson, J. K., and Knight, R. (2018). Earth microbiome project and global systems biology. mSystems 3, 1–4. doi: 10.1128/mSystems.00217-17
Gu, D., Yu, F. D., Hu, Y. C., Wang, J. W., Xu, M., Mu, X. D., et al. (2020). The species composition and distribution patterns of non-native fishes in the main rivers of South China. Sustainability 12, 1–15. doi: 10.3390/su12114566
Guerreiro, I., Enes, P., Rodiles, A., Merrifield, D., and Oliva-Teles, A. (2016). Effects of rearing temperature and dietary short-chain fructooligosaccharides supplementation on allochthonous gut microbiota, digestive enzymes activities and intestine health of turbot (Scophthalmus maximus L.) juveniles. Aquac. Nutr. 22, 631–642. doi: 10.1111/anu.12277
Guimarães, M. C., da Silva Guimarães, A. I. C., Natori, M. M., Alarcon, M. F. F., Dias, D. de. C., Ishikawa, C. M., et al. (2021). Oral administration of Bacillus subtilis and Lactobacillus plantarum modulates the gut microbiota and increases the amylase activity of Nile tilapia (Oreochromis niloticus). Aquacult. Int. 29, 91–104. doi: 10.1007/s10499-020-00612-2
Hamilton, E. F., Element, G., van Coeverden de Groot, P., Engel, K., Neufeld, J. D., Shah, V., et al. (2019). Anadromous arctic char microbiomes: bioprospecting in the high arctic. Front. Bioeng. Biotechnol. 7, 1–13. doi: 10.3389/fbioe.2019.00032
Hasan, I., Rimoldi, S., Saroglia, G., and Terova, G. (2023). Sustainable fish feeds with insects and probiotics positively affect freshwater and marine fish gut microbiota. Animals 13, 1–17. doi: 10.3390/ani13101633
Huang, Q., Sham, R. C., Deng, Y., Mao, Y., Wang, C., Zhang, T., et al. (2020). Diversity of gut microbiomes in marine fishes is shaped by host-related factors. Mol. Ecol. 29, 5019–5034. doi: 10.1111/mec.15699
Huyben, D., Sun, L., Moccia, R., Kiessling, A., Dicksved, J., and Lundh, T. (2018). Dietary live yeast and increased water temperature influence the gut microbiota of rainbow trout. J. Appl. Microbiol. 124, 1377–1392. doi: 10.1111/jam.13738
Jin Song, S., Woodhams, D. C., Martino, C., Allaband, C., Mu, A., Javorschi-Miller-Montgomery, S., et al. (2019). Engineering the microbiome for animal health and conservation. Exp. Biol. Med. 244, 494–504. doi: 10.1177/1535370219830075
Kara, J., Andres Bingdi, L., Lauren, E., Johnson Kevin, L., Kapuscinski Ashley, H., and Moerke Fangqiong Ling, J. H. K. (2021). Life stage and vaccination shape the gut microbiome of hatchery-reared Atlantic salmon (Salmo salar). bioRxiv 61, 109–118. doi: 10.1016/j.aquaculture.2024.741469
Kim, P. S., Shin, N. R., Lee, J. B., Kim, M. S., Whon, T. W., Hyun, D. W., et al. (2021). Host habitat is the major determinant of the gut microbiome of fish. Microbiome 9, 1–16. doi: 10.1186/s40168-021-01113-x
Kokou, F., Sasson, G., Nitzan, T., Doron-Faigenboim, A., Harpaz, S., Cnaani, A., et al. (2018). Host genetic selection for cold tolerance shapes microbiome composition and modulates its response to temperature. Elife 7, 1–21. doi: 10.7554/eLife.36398
Koo, H., Hakim, J. A., Powell, M. L., Kumar, R., Eipers, P. G., Morrow, C. D., et al. (2017). Metagenomics approach to the study of the gut microbiome structure and function in zebra fish Danio rerio fed with gluten formulated diet. J. Microbiol. Methods 135, 69–76. doi: 10.1016/j.mimet.2017.01.016
Kuebutornye, F. K. A., Wang, Z., Lu, Y., Abarike, E. D., Sakyi, M. E., Li, Y., et al. (2020). Effects of three host-associated Bacillus species on mucosal immunity and gut health of Nile tilapia, Oreochromis niloticus and its resistance against Aeromonas hydrophila infection. Fish Shellfish Immunol. 97, 83–95. doi: 10.1016/j.fsi.2019.12.046
Kumar, M., Ji, B., Zengler, K., and Nielsen, J. (2019). Modelling approaches for studying the microbiome. Nat. Microbiol. 4, 1253–1267. doi: 10.1038/s41564-019-0491-9
Lennon, J. T., Frost, S. D. W., Nguyen, N. K., Peralta, A. L., Place, A. R., and Treseder, K. K. (2023). Microbiology and climate change: a transdisciplinary imperative. mBio 14:22. doi: 10.1128/mbio.03335-22
Ley, R. E., Lozupone, C. A., Hamady, M., Knight, R., and Gordon, J. I. (2008). Worlds within worlds: evolution of the vertebrate gut microbiota. Nat. Rev. Microbiol. 6, 776–788. doi: 10.1038/nrmicro1978
Li, S. F., Wu, L., Wang, J., Chou, Q., and Chen, Y. (1990). Comprehensive Genetic Study of Chinese Carps. Shanghai: Shanghai Scientific and Technical Publishers.
Li, T., Li, H., Gatesoupe, F., She, R., and Lin, Q. (2017a). Bacterial signatures of “red-operculum” disease in the gut of crucian carp (Carassius auratus). Microb. Ecol. 74, 510–521. doi: 10.1007/s00248-017-0967-1
Li, T., Long, M., Gatesoupe, F. J., Zhang, Q., Li, A., and Gong, X. (2015). Comparative analysis of the intestinal bacterial communities in different species of carp by pyrosequencing. Microb. Ecol. 69, 25–36. doi: 10.1007/s00248-014-0480-8
Li, T., Long, M., Li, H., Gatesoupe, F., Zhang, X., and Waite, D. W. (2017b). Multi-omics analysis reveals a correlation between the host phylogeny, gut microbiota and metabolite profiles in cyprinid fishes. Front. Microbiol. 8:454. doi: 10.3389/fmicb.2017.00454
Li, X., Huang, X., Zhao, L., Cai, W., Yu, Y., and Zhang, J. (2023). Host habitat as a dominant role in shaping the gut microbiota of wild crucian carp (Carassius auratus). Fishes 8, 1–9. doi: 10.3390/fishes8070369
Li, X., Yan, Q., Xie, S., Hu, W., Yu, Y., and Hu, Z. (2013). Gut microbiota contributes to the growth of fast-growing transgenic common carp (Cyprinus carpio L.). PLoS One 8:64577. doi: 10.1371/journal.pone.0064577
Li, X., Zhou, L., Yu, Y., Ni, J., Xu, W., and Yan, Q. (2017c). Composition of gut microbiota in the gibel carp (Carassius auratus gibelio) varies with host development. Microb. Ecol. 74, 239–249. doi: 10.1007/s00248-016-0924-4
Liu, H., Guo, X., Gooneratne, R., Lai, R., Zeng, C., and Zhan, F. (2016). The gut microbiome and degradation enzyme activity of wild freshwater fishes influenced by their trophic levels. Sci. Rep. 6:24340. doi: 10.1038/srep24340
Liu, Y., Li, X., Li, J., and Chen, W. (2021). The gut microbiome composition and degradation enzymes activity of black Amur bream (Megalobrama terminalis) in response to breeding migratory behavior. Ecol. Evol. 11, 5150–5163. doi: 10.1002/ece3.7407
Llewellyn, M. S., McGinnity, P., Dionne, M., Letourneau, J., Thonier, F., Carvalho, G. R., et al. (2016). The biogeography of the atlantic salmon (Salmo salar) gut microbiome. ISME J. 10, 1280–1284. doi: 10.1038/ismej.2015.189
Lorgen-Ritchie, M., Uren Webster, T., McMurtrie, J., Bass, D., Tyler, C. R., Rowley, A., et al. (2023). Microbiomes in the context of developing sustainable intensified aquaculture. Front. Microbiol. 14, 1–16. doi: 10.3389/fmicb.2023.1200997
Lu, G., Li, S., and Bernatchez, L. (1997). Mitochondrial DNA diversity, population structure, and conservation genetics of four native carps within the Yangtze River, China. Can. J. Fish. Aquat. Sci. 54, 47–58. doi: 10.1139/f96-266
Lu, G., and Luo, M. (2020). Genomes of major fishes in world fisheries and aquaculture: status, application and perspective. Aquacult. Fish. 5, 163–173. doi: 10.1016/j.aaf.2020.05.004
Lu, G., Wang, C., Zhao, J., Liao, X., Wang, J., Luo, M., et al. (2020). Evolution and genetics of bighead and silver carps: native population conservation versus invasive species control. Evol. Appl. 13, 1351–1362. doi: 10.1111/eva.12982
Lyons, P. P., Turnbull, J. F., Dawson, K. A., and Crumlish, M. (2017). Phylogenetic and functional characterization of the distal intestinal microbiome of rainbow trout Oncorhynchus mykiss from both farm and aquarium settings. J. Appl. Microbiol. 122, 347–363. doi: 10.1111/jam.13347
Magoulick, D. D., and Lewis, L. C. (2002). Predation on exotic zebra mussels by native fishes: effects on predator and prey. Freshw. Biol. 47, 1908–1918. doi: 10.1046/j.1365-2427.2002.00940.x
Martinez-Porchas, M., Preciado-Álvarez, A., Vargas-Albores, F., Gracia-Valenzuela, M. H., Cicala, F., Martinez-Cordova, L. R., et al. (2023). Microbiota plasticity in tilapia gut revealed by meta-analysis evaluating the effect of probiotics, prebiotics, and biofloc. PeerJ 11, 1–24. doi: 10.7717/peerj.16213
Mohajeri, M. H., Brummer, R. J. M., Rastall, R. A., Weersma, R. K., Harmsen, H. J. M., Faas, M., et al. (2018). The role of the microbiome for human health: from basic science to clinical applications. Eur. J. Nutr. 57, 1–14. doi: 10.1007/s00394-018-1703-4
Mugetti, D., Pastorino, P., Beltramo, C., Audino, T., Arillo, A., Esposito, G., et al. (2023). The gut microbiota of farmed and wild brook trout (Salvelinus fontinalis): evaluation of feed-related differences using 16S rRNA gene metabarcoding. Microorganisms 11:71636. doi: 10.3390/microorganisms11071636
Mugwanya, M., Dawood, M. A., Kimera, F., and Sewilam, H. (2021). Biofloc systems for sustainable production of economically important aquatic species: a review. Sustainability 13:7255. doi: 10.3390/su13137255
Mukherjee, A., Rodiles, A., Merrifield, D. L., Chandra, G., and Ghosh, K. (2020). Exploring intestinal microbiome composition in three Indian major carps under polyculture system: a high-throughput sequencing based approach. Aquaculture 524:735206. doi: 10.1016/j.aquaculture.2020.735206
Nayfach, S., Roux, S., Seshadri, R., Udwary, D., Varghese, N., Schulz, F., et al. (2021). A genomic catalog of Earth's microbiomes. Nat. Biotechnol. 39, 499–509. doi: 10.1038/s41587-020-0718-6
Nohesara, S., Abdolmaleky, H. M., Zhou, J. R., and Thiagalingam, S. (2023). Microbiota-induced epigenetic alterations in depressive disorders are targets for nutritional and probiotic therapies. Genes 14:122217. doi: 10.3390/genes14122217
Pingle, S. A., and Khandagle, A. J. (2023). A metagenomic analysis of gut microbiome phylogeny among four economically important carp species from wild and aquaculture farms. J. Appl. Biol. Biotechnol. 11, 105–111. doi: 10.7324/JABB.2023.11512-1
Rahman, M. M., Verdegem, M. C. J., Nagelkerke, L. A. J., Wahab, M. A., and Verreth, J. A. J. (2008). Swimming, grazing and social behaviour of rohu Labeo rohita (Hamilton) and common carp Cyprinus carpio (L.) in tanks under fed and non-fed conditions. Appl. Anim. Behav. Sci. 113, 255–264. doi: 10.1016/j.applanim.2007.09.008
Ramesh, I., and Kiran, B. R. (2016). Food and feeding habits of catfish Clarias batrachus (Linn) in Bhadravathi Area, Karnataka. Int. J. Res. Environ. Sci. 2, 56–59. doi: 10.20431/2454-9444.0204006
Ratten, J., Beyer, M., Hasler, M., Laroche, J., and Schulz, C. (2017). The malleable gut microbiome of juvenile rainbow trout (Oncorhynchus mykiss): diet- dependent shifts of bacterial community structures. PLoS One 12:e0177735. doi: 10.1371/journal.pone.0177735
Reshma, K. J., Sumithra, T. G., Nair, A. V., Stefi Raju, V., Kishor, T. G., Sreenath, K. R., et al. (2018). An insight into the gut microbiology of wild-caught Mangrove Red Snapper, Lutjannus argentimaculatus (Forsskal, 1775). Aquaculture 497, 320–330. doi: 10.1016/j.aquaculture.2018.08.008
Riera, J. L., and Baldo, L. (2020). Microbial co-occurrence networks of gut microbiota reveal community conservation and diet-associated shifts in cichlid fishes. Anim. Microbiome 2:4. doi: 10.1186/s42523-020-00054-4
Rurangwa, E., and Verdegem, M. C. (2015). Microorganisms in recirculating aquaculture systems and their management. Rev. Aquacult. 7, 117–130. doi: 10.1111/raq.12057
Schmidt, V., Amaral-Zettler, L., Davidson, J., Summerfelt, S., and Good, C. (2016). Influence of fishmeal-free diets on microbial communities in atlantic salmon (Salmo salar) recirculation aquaculture systems. Appl. Environ. Microbiol. 82, 4470–4481. doi: 10.1128/AEM.00902-16
Sepulveda, J., and Moeller, A. H. (2020). The effects of temperature on animal gut microbiomes. Front. Microbiol. 11, 1–9. doi: 10.3389/fmicb.2020.00384
Shang, S., Ren, J., Wang, J., Xin, S., Xia, J., and Tang, X. (2021). High-throughput sequencing reveals significant diversity in the gut microbiomes of humpback (Chanodichthys dabryi) and crucian carp (Carassius carassius). Biologia 76, 655–662. doi: 10.2478/s11756-020-00591-y
Sinha, V. R. P., and Jones, J. W. (1967). On the food of the freshwater eels and their feeding relationship with the salmonids. J. Zool. 153, 119–137. doi: 10.1111/j.1469-7998.1967.tb05034.x
Soh, M., Tay, Y. C., Lee, C. S., Low, A., Orban, L., Jaafar, Z., et al. (2024). The intestinal digesta microbiota of tropical marine fish is largely uncultured and distinct from surrounding water microbiota. NPJ Biofilms Microbiomes 10, 1–15. doi: 10.1038/s41522-024-00484-x
Soriano, E. L., Ramírez, D. T., Araujo, D. R., Gómez-Gil, B., Castro, L. I., and Sánchez, C. G. (2018). Effect of temperature and dietary lipid proportion on gut microbiota in yellowtail kingfish Seriola lalandi juveniles. Aquaculture 497, 269–277. doi: 10.1016/j.aquaculture.2018.07.065
Stagaman, K., Burns, A. R., Guillemin, K., and Bohannan, B. J. M. (2017). The role of adaptive immunity as an ecological filter on the gut microbiota in zebrafish. ISME J. 11, 1630–1639. doi: 10.1038/ismej.2017.28
Standen, B. T., Rodiles, A., Peggs, D. L., Davies, S. J., Santos, G. A., and Merrifield, D. L. (2015). Modulation of the intestinal microbiota and morphology of tilapia, Oreochromis niloticus, following the application of a multi-species probiotic. Appl. Microbiol. Biotechnol. 99, 8403–8417. doi: 10.1007/s00253-015-6702-2
Stephens, W. Z., Burns, A. R., Stagaman, K., Wong, S., Rawls, J. F., Guillemin, K., et al. (2016). The composition of the zebrafish intestinal microbial community varies across development. ISME J. 10, 644–654. doi: 10.1038/ismej.2015.140
Sylvain, F. É., Holland, A., Bouslama, S., Audet-Gilbert, É., Lavoie, C., Luis Val, A., et al. (2020). Fish skin and gut microbiomes show contrasting signatures of host species and habitat. Appl. Environ. Microbiol. 86, 1–15. doi: 10.1128/AEM.00789-20
Talwar, C., Nagar, S., Lal, R., and Negi, R. K. (2018). Fish gut microbiome: current approaches and future perspectives. Ind. J. Microbiol. 58, 397–414. doi: 10.1007/s12088-018-0760-y
Thomas, E. E., and Opeh, P. B. (2018). Food and feeding habits of black spot catfish (Auchenoglanis biscutatus) from Lower River Benue, Makurdi. Asian J. Fish. Aq. Res. 1, 1–5. doi: 10.9734/ajfar/2018/v1i3321
Troell, M., Joyce, A., Chopin, T., Neori, A., Buschmann, A. H., and Fang, J. G. (2009). Ecological engineering in aquaculture-potential for integrated multi-trophic aquaculture (IMTA) in marine offshore systems. Aquaculture 297, 1–9. doi: 10.1016/j.aquaculture.2009.09.010
Turnbaugh, P. J., Ley, R. E., Hamady, M., Fraser-Liggett, C. M., Knight, R., and Gordon, J. I. (2007). The human microbiome project. Nature 449, 804–810. doi: 10.1038/nature06244
Vargas-albores, F., Martínez-c, L. R., Martínez-porchas, M., and Cicala, F. (2021). Therapeutic modulation of fish gut microbiota, a feasible strategy for aquaculture? Aquaculture 544:737050. doi: 10.1016/j.aquaculture.2021.737050
Wang, A., Zhang, Z., Ding, Q., Yang, Y., Bindelle, J., Ran, C., et al. (2021). Intestinal Cetobacterium and acetate modify glucose homeostasis via parasympathetic activation in zebrafish. Gut Microbes 13, 1–15. doi: 10.1080/19490976.2021.1900996
Wang, J., Gaughan, S., Lamer, J. T., Deng, C., Hu, W., Wachholtz, M., et al. (2020). Resolving the genetic paradox of invasions: preadapted genomes and postintroduction hybridization of bigheaded carps in the Mississippi River Basin. Evol. Appl. 13, 263–277. doi: 10.1111/eva.12863
Watzin, M. C., Joppe-Mercure, K., Rowder, J., Lancaster, B., and Bronson, L. (2008). Significant fish predation on zebra mussels Dreissena polymorpha in Lake Champlain, U.S.A. J. Fish. Biol. 73, 1585–1599. doi: 10.1111/j.1095-8649.2008.02033.x
Wong, S., and Rawls, J. F. (2012). Intestinal microbiota composition in fishes is influenced by host ecology and environment. Mol. Ecol. 21, 3100–3102. doi: 10.1111/j.1365-294X.2012.05646.x
Wu, S., Gao, T., Zheng, Y., Wang, W., Cheng, Y., and Wang, G. (2010). Microbial diversity of intestinal contents and mucus in yellow catfish (Pelteobagrus fulvidraco). Aquaculture 303, 1–7. doi: 10.1016/j.aquaculture.2009.12.025
Wu, S., Wang, G., Angert, E. R., Wang, W., Li, W., and Zou, H. (2012). Composition, diversity, and origin of the bacterial community in grass carp intestine. PLoS ONE 7:30440. doi: 10.1371/journal.pone.0030440
Xiong, F., Chen, S., Jakovlić, I., Li, W., Li, M., Zou, H., et al. (2022). The role of intestinal microbiota in regulating the metabolism of bile acids is conserved across vertebrates. Front. Microbiol. 13, 1–13. doi: 10.3389/fmicb.2022.824611
Xiong, J. B., Nie, L., and Chen, J. (2019). Current understanding on the roles of gut microbiota in fish disease and immunity. Zool. Res. 40, 70–76. doi: 10.24272/j.issn.2095-8137.2018.069
Yan, Q., Li, J., Yu, Y., Wang, J., He, Z., Nostrand, J. D., et al. (2016). Environmental filtering decreases with fish development for the assembly of gut microbiota. Environ. Microbiol. 18, 4739–4754. doi: 10.1111/1462-2920.13365
Ye, L., Amberg, J., Chapman, D., Gaikowski, M., and Liu, W. T. (2014). Fish gut microbiota analysis differentiates physiology and behavior of invasive Asian carp and indigenous American fish. ISME J. 8, 541–551. doi: 10.1038/ismej.2013.181
Yin, J., Li, Y., Tian, Y., Zhou, F., Ma, J., Xia, S., et al. (2023). Obese Ningxiang pig-derived microbiota rewires carnitine metabolism to promote muscle fatty acid deposition in lean DLY pigs. Innovation 4:100486. doi: 10.1016/j.xinn.2023.100486
Zarkasi, K. Z., Taylor, R. S., Abell, G. C. J., Tamplin, M. L., Glencross, B. D., and Bowman, J. P. (2016). Atlantic salmon (Salmo salar L.) gastrointestinal microbial community dynamics in relation to digesta properties and diet. Microb. Ecol. 71, 589–603. doi: 10.1007/s00248-015-0728-y
Zhang, Y., Zhou, L., Xia, J., Dong, C., and Luo, X. (2022). Human microbiome and its medical applications. Front. Mol. Biosci. 8, 1–17. doi: 10.3389/fmolb.2021.703585
Zhang, Z., Li, D., Refaey, M. M., and Xu, W. (2017). High spatial and temporal variations of microbial community along the southern catfish gastrointestinal tract: insights into dynamic food digestion. Front. Microbiol. 8:1531. doi: 10.3389/fmicb.2017.01531
Zhang, Z., Li, D., Refaey, M. M., Xu, W., Tang, R., and Berry, D. (2018). Host age affects the development of southern catfish gut bacterial community divergent from that in the food and rearing water. Front. Microbiol. 9, 1–9. doi: 10.3389/fmicb.2018.00495
Zhu, L., and Wang, J. (2023). Editorial: community series in the wildlife gut microbiome and its implication for conservation biology, volume II. Front. Microbiol. 14:1329928. doi: 10.3389/fmicb.2023.1329928
Zhu, L., Wang, J., and Bahrndorff, S. (2021a). Editorial: the wildlife gut microbiome and its implication for conservation biology. Front. Microbiol. 12, 10–13. doi: 10.3389/fmicb.2021.697499
Zhu, L., Zhang, Z., Chen, H., Lamer, J. T., Wang, J., Wei, W., et al. (2021b). Gut microbiomes of bigheaded carps and hybrids provide insights into invasion: a hologenome perspective. Evol. Appl. 14, 735–745. doi: 10.1111/eva.13152
Keywords: fish gut microbiome, aquaculture species, microbial diversity, host association, environment impact, conservation biology
Citation: Kanika NH, Liaqat N, Chen H, Ke J, Lu G, Wang J and Wang C (2025) Fish gut microbiome and its application in aquaculture and biological conservation. Front. Microbiol. 15:1521048. doi: 10.3389/fmicb.2024.1521048
Received: 01 November 2024; Accepted: 09 December 2024;
Published: 07 January 2025.
Edited by:
Lifeng Zhu, Nanjing University of Chinese Medicine, ChinaReviewed by:
Yaqiu Liu, Chinese Academy of Fishery Sciences, ChinaWancai Xia, China West Normal University, China
Copyright © 2025 Kanika, Liaqat, Chen, Ke, Lu, Wang and Wang. This is an open-access article distributed under the terms of the Creative Commons Attribution License (CC BY). The use, distribution or reproduction in other forums is permitted, provided the original author(s) and the copyright owner(s) are credited and that the original publication in this journal is cited, in accordance with accepted academic practice. No use, distribution or reproduction is permitted which does not comply with these terms.
*Correspondence: Guoqing Lu, Z2x1M0B1bm9tYWhhLmVkdQ==; Jun Wang, d2FuZ2p1bkBzaG91LmVkdS5jbg==; Chenghui Wang, d2FuZ2NoQHNob3UuZWR1LmNu