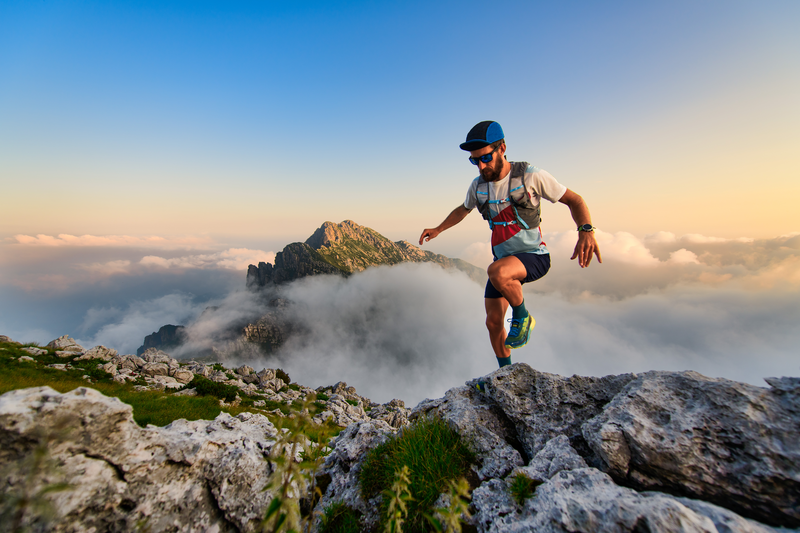
95% of researchers rate our articles as excellent or good
Learn more about the work of our research integrity team to safeguard the quality of each article we publish.
Find out more
REVIEW article
Front. Microbiol. , 10 January 2025
Sec. Infectious Agents and Disease
Volume 15 - 2024 | https://doi.org/10.3389/fmicb.2024.1519906
The presence of carbapenem-resistant Acinetobacter baumannii (CRAb) has become one of the leading causes of life-threatening, hospital-acquired infections globally, especially with a notable prevalence in intensive care units (ICUs). The cross-transmission of microorganisms between patients and the hospital setting is crucial in the development of CRAb colonization and subsequent infections. Recent studies indicate that colonization typically precedes infection, suggesting the effectiveness and necessity of preventing CRAb colonization as a primary method to lower infection risks. As CRAb infections tend to draw more attention due to their severe symptoms and poor outcomes, understanding the link between colonization and infection is equally vital. To establish a foundation for prevention and control strategies against CRAb colonization in ICUs, we present a comprehensive review of research pertaining to CRAb in ICUs. This encompasses an analysis of the resistance mechanisms and epidemiological characteristics of CRAb, a discussion on associated risk factors, adverse outcomes, and an evaluation of detection methods and preventive strategies.
The bacterium Acinetobacter baumannii is as an opportunistic human pathogen that predominantly infects critically ill patients worldwide. In recent years, the emergence and dissemination of carbapenem-resistant non-fermenting Gram-negative bacilli (NFGNB) in ICUs pose a substantial threat in hospitals (Agarwal et al., 2017; Kousouli et al., 2019). CRAb ranks among the top five global pathogens in terms of attributable mortality due to antibiotic-resistant infections. It is estimated to be the leading pathogen causing mortality attributable to antibiotic resistance in South-East Asia, East Asia, and Oceania (Antimicrobial Resistance Collaborators, 2022a; European Antimicrobial Resistance Collaborators, 2023). In 2019, it caused more than 57,700 deaths and 1.5 million DALYs (deaths and disability-adjusted life-years) (Antimicrobial Resistance Collaborators, 2022b). A study focusing on European, Eastern Mediterranean, and African populations revealed that CRAb had a pooled incidence of 41.7 cases per 1,000 patients, and accounts for 13.6% of hospital acquired infections in ICUs (Ayobami et al., 2019). And the resistance rate of CRAb showed a significantly upward trend (Lăzureanu et al., 2016; He et al., 2020). Data from the China Antimicrobial Resistance Surveillance System (CHINET) showed that the resistance rates of A. baumannii strains to meropenem and imipenem increased from 30.1% and 39.0% in 2005 to 71.5% and 72.3% in 2021 (Liu et al., 2022). Severe infections caused by CRAb have become a challenging clinical problem. The World Health Organization (WHO) listed CRAb as one of the bacteria urgently needing antibiotics in its 2017 publication “Global Priority List of Antibiotic-Resistant Bacteria”(World Health Organization, 2017), and maintain its critical status until 2024 (World Health Organization, 2024). Besides, A. baumannii causes a range of nosocomial infections across multiple anatomical sites. Most commonly, A. baumannii infections manifest as ventilator-associated pneumonia or central line-associated blood stream infections (Harding et al., 2018). Previous retrospective studies found a significantly higher sputum isolation rate of CRAb in ICUs compared to non-ICUs, and 80% of CRAb in ICUs were isolated from sputum samples, accounting for over 50% of all carbapenem-resistant NFGNB (Neves et al., 2016; He et al., 2020).
Differentiating between colonization and infection is challenging. Generally, CRAb colonization is defined as the presence of the bacteria on the skin, mucosa, open wounds, secretions, or indwelling medical devices without any associated clinical symptoms or signs of infection (fever, elevated white blood count, elevated inflammatory markers, and abnormal imaging) (Moghnieh et al., 2016; Neves et al., 2016; Bartal et al., 2022; Pascale et al., 2022).
In fact, the colonization of CRAb often occurs earlier than the occurrence of infection, prior studies have described the presence of colonization as a factor associated with the development of A. baumannii infections. Latibeaudiere et al. (2015) found that patients with positive rectal or respiratory secretion cultures were eight times more likely to develop CRAb infections. This finding remained significant even after adjusting for other variables such as sex, mechanical ventilation, and exposure to cephalosporins (Latibeaudiere et al., 2015). Other research data has shown that early colonization often leads to an increase in mortality, indicating the occurrence of poor prognosis (Zheng et al., 2021). This suggests that intervention during the early colonization phase of CRAb may help improve patients’ long-term prognosis. However, due to difficulties in defining colonization and infection, and regional differences in ICUs conditions, research and attention in this field are currently limited. This article aims to summarize literatures associated to CRAb colonization, integrate bacterial microbial characteristics, drug resistance, hazards, and prevention/intervention measures, provide certain warnings and guidance for clinical medicine, and advocate for attention to drug-resistant bacteria colonization.
Acinetobacter baumannii, a Gram-negative coccobacillus, measuring (0.6∼1.0) micrometers by (1.0∼1.6) micrometers, are mostly rod-shaped with blunt rounded ends, scattered or arranged in pairs, without spores or flagella, and the colonies are grayish-white, round, smooth, and have neatly defined edges form on a blood agar plate. A. baumannii is recognized for its adaptability and resilience in various environments. As a non-glucose fermenting and strictly aerobic bacterium, it can exist as a colonizing organism on human skin and other body sites, while also persisting in healthcare environments due to its robust biofilm formation (Figure 1). It withstands dry conditions effectively and harbors resistance determinants against antibiotics and commonly used disinfectants, increasing its propensity to contaminate hospital settings. Compared to other pathogens, it can survive for more extended periods on surfaces, including hospital fixtures, utensils, equipment, invasive medical devices, and personnel (Nutman et al., 2016; Odih et al., 2022).
For Gram-negative bacteria, common resistance mechanisms encompass enzymatic hydrolysis by β-lactamases, overexpression of drug efflux pumps, and mutations in antibiotic target sites (Li et al., 2015; Arzanlou et al., 2017). The mechanisms of carbapenem resistance in A. baumannii can generally be categorized into four distinct groups: alterations in penicillin-binding proteins, loss of outer membrane porins, overexpression of efflux pumps, and synthesis of carbapenem-hydrolyzingβ-lactamases (Nguyen and Joshi, 2021), while the last group is reported to be the most important one (Mugnier et al., 2009; Higgins et al., 2013).
The OXA-type carbapenem-hydrolyzing class D β-lactamases [oxacillinases (OXAs)] constitute a diverse family, mainly including blaOXA-23-like, blaOXA-40-like, blaOXA-58-like, blaOXA-143-like, and blaOXA-235-like (Nowak and Paluchowska, 2016; Juan et al., 2019). Their expression could be enhanced by the insertion of an upstream IS, which enhances expression by providing a strong promoter, causing high resistance levels (Mugnier et al., 2009; Sen and Joshi, 2016).
A recent study reported an update on the molecular epidemiology and global distribution of carbapenemase encoding genes. Among all international clones (IC) IC1–IC8, they found IC2 predominated with 196 of 313 isolates (62.6%) was spread over all participating regions, ranging from 10% in Latin America to 70% in North America and about 80% in Africa, Asia, and Europe. The most frequently encountered carbapenemase-encoding genes were blaOXA-23-like and blaOXA-40-like, present in 234 of 300 (74.8%) and 56 of 300 isolates (17.9%), respectively. While the number of blaOXA-40-like-positive isolates was considerably higher in Latin and North America than in the remaining regions with nearly 32% of all American isolates (Müller et al., 2023). Overall, the predominance of blaOXA-23-like producing CRAb mainly representing IC2 has been reported worldwide (Higgins et al., 2010; Eigenbrod et al., 2019; Hamidian and Nigro, 2019).
Numerous factors contribute to the colonization of CRAb, highlighting the complexity of this healthcare challenge. In our prior research, we revealed patients testing positive for CRAb were more likely to have cardiovascular diseases, type II diabetes mellitus, solid tumors and suspected sepsis, and this seems to be explained by impairment of structure and function of certain body systems and immunosuppression (Xu et al., 2019a; Zheng et al., 2021). Other certain patient-associated factors like prolonged hospitalization, a history of previous hospitalization, and the severity of the underlying disease can also be linked to CRAb colonization (Thoma et al., 2022). In addition to patient disease-related factors, there still two main opportunities for the occurrence and dissemination of colonization events, namely the environment and the medical treatment.
As we mentioned before, A. baumannii possesses tenacity to persist in environments for prolonged periods, and this explains one of the main reasons for the occurrence of CRAb colonization. It is largely believed that two attributes, drug resistance and environmental persistence, have enabled A. baumannii to thrive in the nosocomial environment (Roca et al., 2012). Commonly, healthcare environments include prolonged periods of desiccation and routine disinfection regimes. Like their resistance to antibiotics, A. baumannii has evolved to withstand these environmental stresses (Wong et al., 2017). Desiccation resistance, which is the ability to maintain viability under dry conditions, varies amongst clinical isolates of A. baumannii, with some isolates remaining viable for almost 100 days (Harding et al., 2018). Besides, the biofilm formation also help increase tolerance to extracellular stresses (Greene et al., 2016a; Greene et al., 2016b).
Patients colonized with CRAb serve as carriers, contaminating their immediate surroundings like drawer handles of supply carts, floors, infusion pumps and ventilator buttons, etc. (Thom et al., 2011; Alfandari et al., 2014; Latibeaudiere et al., 2015; Shimose et al., 2016; Weiss et al., 2016). Ng et al. (2018) reported environmental contamination present in 5 of 18 (28%) of the rooms housing patients with CRAb, by collecting surface swabs of the environment inside and outside the ward. Uwingabiye et al. (2017) also observed a genetic resemblance in 80 of 83 (96.4%) of all isolates between environmental and clinical, showing that the clonal spread of environmental A. baumannii isolates is related to that of clinical isolates recovered from colonized or infected patients. Interestingly, some studies found a significantly difference in the degree of contamination based on the occupant’s anatomic source of A. baumannii (Rosa et al., 2014). After sampling the air and environmental surface swab daily for up to 10 days, Shimose et al. (2016) found the positive rate of air samples in rooms with rectally colonized patients is nearly three times (38.3% vs. 13.1%) that of patients with respiratory colonization. And the positive rate of environmental samples also showed the same trend (15.5% for patients with rectally colonization, 9.5% for patients with respiratory colonization) (Shimose et al., 2016).
Cross-transmission triggered by medical personnel contacting patients without proper disinfection forms critical cause of CRAb colonization/infection within ICUs wards (Russotto et al., 2015). Inadequate compliance with personal protective equipment (PPE) and hand hygiene are common contributing factors (Thoma et al., 2022). Instances like failure to remove gloves post-contact with the patient’s surrounding environment, coupled with the inadequate performance of the hand-hygiene action, are associated with heightened cross-contamination issues (Pires et al., 2017). Therefore, admitting patients who are CRAb carriers into ICUs certainly pose a nosocomial infection risk to other susceptible individuals (Maamar et al., 2018; Al-Hamad et al., 2020).
Neves et al. (2016) found that previous antibiotic treatment significantly contributed to the risk factors associated with CRAb colonization. Munoz-Price et al. (2016) found that once a patient is exposed to carbapenems, even if the disease severity is adjusted, the risk of colonization increases fourfold, and the impact will persist. Our team’s previous research also showed that the use of broad-spectrum antibiotics for over 7 days was an independent predictor of lower respiratory CRAb infection/colonization in mechanically ventilated patients. The CRAb detection rate in patients with antimicrobial combination therapy was 2.5% higher than non-antimicrobial combination therapy patients (Xu et al., 2019a). The reason for this phenomenon seems to be explained by adaptive resistance (Skiada et al., 2011). Previous studies have shown that multiple genes mediate this process, like the production of OXA-23 and TEMoneira leading to β-lactam resistance (Desmoulin et al., 2024), and the mutations in pmrCAB and lpxA gene may explain the adaptive resistance to polymyxins (Barin et al., 2013). Besides, overuse of antibiotics for presumed bacterial co-infection or secondary infection may also lead to the development and spread of bacterial resistance (Langford et al., 2020). Table 1 lists some antibiotics that may influence the CRAb colonization.
Previous studies have discovered a strong association between the use of invasive devices and CRAb colonization in a hospital environment (Moghnieh et al., 2016). Patients who receive supportive treatments like renal replacement therapy, longer invasive ventilation, and vasopressor treatment have been proved to have a high tendency for CRAb colonization (Zheng et al., 2021). The rate of lower respiratory CRAb infection/colonization in tracheotomy patients is 1.996 times higher than that in patients with tracheal intubation (Xu et al., 2019a). Neves et al. (2016) observed that invasive devices comprise a significant proportion of risk factors associated with CRAb colonization. Meschiari et al. (2021a) found the use of permanent devices and the use of invasive devices in a hospital environment significantly associated to risk of rectal colonization with CRAb. Among these were the use of vascular catheters, such as central venous catheterization (CVC) or the use of peripherally inserted central catheter (PICC) or Midline, urinary catheter (UC), nasogastric tube (NG), and mechanical ventilation (MV). Other factors that were significantly associated were tracheostomy and percutaneous endoscopic gastrostomy (PEG), which respectively determined an elevated risk for rectal CRAb colonization of 10.5 and 11 times (Meschiari et al., 2021a).
Overall, the above factors increase the risk of CRAb implantation in ICUs patients. In order to obtain much better prognosis, we should comprehensively understand the current colonization risk associated to environment and treatment, and much more efforts are needed urgently (Figure 2).
Figure 2. Risk factors of CRAb colonization. The figure shows main factors for the occurrence and dissemination of colonization events.
The harm induced by CRAb infection poses challenges for all doctors. Generally, the process of bacterial infection consists of three steps. First, bacteria invade or colonize initial sites of infection. Second, bacteria overcome host barriers, such as immune responses, and disseminate from initial body sites to the bloodstream. Third, bacteria adapt to survive in the blood and blood-filtering organs. Previous studies have confirmed that colonization precedes infection. There is evidence suggesting that colonization of A. baumannii in seemingly harmless body parts such as armpits, pharynx, and gastrointestinal tract in ICUs may precede subsequent infections (Ayats et al., 1997). Numerous studies indicate that colonization is a crucial risk factor for subsequent infections (Moghnieh et al., 2016; Gorrie et al., 2017; Karampatakis et al., 2018). In fact, the burden of colonization, expressed as the presence of CRAb at different sites, and the homeostasis alteration in particular organs, such as the gut and the lungs, may favor bacterial translocation and subsequent CRAb infection (Cogliati Dezza et al., 2023). And this process is mediated by multiple mechanisms including bacterial invasiveness and toxicity (iron uptake, siderophore, immune evasion, and biofilm formation) (Xiao et al., 2022), immunosuppression, multisite colonization, burden of comorbidities and mechanical ventilation, etc.
Recent study demonstrated the process of biofilm formation from CRAb colonization to infection. Generally, biofilm production relies on the initial reversible bacterial attachment to a surface in response to environmental stimuli (Toyofuku et al., 2016). Early adhesion is essential in the colonization process and in establishing an A. baumannii infection. Recent researches found the CsuA/BABCDE chaperon-usher assembly system and the two-component system BfmRS are critical in biofilm formation, while the AdeABC, AdeIJK, and AdeFGH RND-type efflux systems play a central role in the initial stages of adhesion, surface colonization, and biofilm maturation in A. baumannii (Coyne et al., 2011). In the final stage, the cells within the biofilm disperse and colonize new surfaces (Cavallo et al., 2023; Figure 3).
Figure 3. Multiple mechanisms of CRAb from colonization to infection. The figure illustrates the mechanisms involved in CRAb from colonization to infection, including bacterial invasiveness and toxicity (iron uptake, siderophore, immune evasion, and biofilm formation), immunosuppression, multisite colonization, burden of comorbidities and mechanical ventilation, etc.
According to our research, when compared to non-colonized patients, CRAb colonized patients had significantly higher APACHE II scores and 6-month mortality rates (68.8% vs. 43.5%; P < 0.001), and longer ICUs stays (Zheng et al., 2021), and this finding corroborates earlier studies. Latibeaudiere et al. (2015) showed that patients carrying this pathogen found on surveillance cultures during their ICUs stay were at a 16.3 times higher risk of developing CRAb infections. Bacterial colonization frequently results in subsequent respiratory and digestive tract infections, and hospital infections (Maamar et al., 2018). Munoz-Price et al. (2016) found that CRAb colonization is the strongest variable associated with subsequent clinical infections of CRAb. The APACHE II score is strongly correlated with the acquisition of A. baumannii, leading to higher mortality rates and longer hospital stays, imposing a substantial burden on patient prognosis and treatment costs. The likelihood of subsequent bloodstream infections in patients carrying CRAb is seven times higher than in non-CRAb colonized patients (OR = 7.41, 95% CI = 2.39–22.92), and the mortality rate in CRAb colonized patients is 50%. Compared with non-CRAb colonized patients (OR = 1.84, 95%; CI = 0.69–4.90), the risk of death in CRAb colonized patients is almost two times higher (Odih et al., 2022). According to another research, the patients with CRAb colonization still show a high crude mortality rate, even if without a subsequent infection onset (Cogliati Dezza et al., 2023). Besides, a recent metagenomic research found the correlation between CRAb colonization/infection and respiratory tract microbiome dysbiosis. The results showed that the relative abundance of Acinetobacter increased in the order CRAb non-colonization, colonization and infection, while the α and β diversity of the lower respiratory tract microbiome was opposite. These dynamic evolution of pulmonary microbiota could promote the occurrence of infection and leading a poor prognosis (Xiao et al., 2022).
In addition, for bloodstream infection, it often occurs in patients with low immune function or serious basic diseases, such as long-term use of immunosuppressants, malignant tumors or diabetes. CRAb can enter the bloodstream through skin wounds, intravascular catheters, and other routes, leading to bacteremia or sepsis. Therefore, we may need to pay more attention to colonized patients with immunosuppression or invasive treatment. Overall, whether acquired during the stay in ICUs or imported, colonization by CRAb is a significant risk factor for serious nosocomial infection, causing prolonged ICUs hospitalization duration, elevated patient costs, and lower overall survival (Phu et al., 2017; Kousouli et al., 2019; Liu et al., 2020; Zhen et al., 2020; Ejaz et al., 2021).
CRAb colonization is a critical step before the onset of hospital-acquired infections and presents significant risks. Intervention and prevention of colonization issues should be implemented as early as possible.
Currently available strategies for reducing the transmission of healthcare–associated pathogens include a comprehensive bundle of measures (Rutala and Weber, 2019; Meschiari et al., 2021b; Seok et al., 2021). These include the development of evidence-based policies and procedures, the selection of suitable cleaning and disinfecting agents, and the education of staff across various departments, including environmental services, patient care equipment, and nursing. Compliance monitoring is also crucial, focusing on the thoroughness of cleaning and the proper use of products, with feedback mechanisms such as just-in-time coaching. Furthermore, the adoption of “no-touch” room decontamination technologies is instrumental in ensuring compliance with contact and enteric precautionary measures for patients (Rutala and Weber, 2019). Considering the possibilities in practical operation of preventing CRAb colonization, we will select a few points to discuss in detail.
Proper environmental disinfection and sterilization are important measures to reduce CRAb colonization. A study found the level of contamination is related to the patient’s colonization load (Nutman et al., 2016). Through the collection of swabs from the patient’s oral mucosa and rectum, along with sponge swabs from the patient’s skin and surrounding environment, it was discovered a positive correlation between the patient colonization score and the environmental contamination score (r = 0.63, P < 0.001). In fact, high-touch surfaces such as ventilators, control panels, infusion pumps and bedrails are considered to be important objects in the potential chain of transmission from one patient to another (Ng et al., 2018). Therefore, environmental management is of great significance.
Previous studies have shown that the cleanliness of the patient’s surroundings has been proven to be effective in reducing Acinetobacter colonization or infections (Lerner et al., 2020; Li et al., 2021). Using aerosolized hydrogen peroxide (aHP) system or manual hypochloride cleaning after discharge of a known CRAb-carrier decreased room contamination by 78% or 85%, respectively (Lerner et al., 2020). Other studies have shown that daily disinfection and care for patients can also reduce CRAb colonization/infection and improve long-term prognosis. In ICUs with high prevalence of CRAb, daily use of 2% chlorhexidine gluconate bath can reduce cross transmission of CRAb between patients (Hong et al., 2018; Metan et al., 2020; Suh et al., 2021). Chung et al. (2015) revealed a 51.8% reduction of CRAb acquisition rates in the medical ICU with CRAb endemicity during a 12-month chlorhexidine bathing period. And the CRAb contamination of environment, like patient gowns, bed rails, staff gowns, keyboards, and monitors was also significantly reduced during the chlorhexidine bathing period (Chung et al., 2015).
However, the frequent use of disinfectants has led to strains less susceptible to these agents (Biswas et al., 2018), while relevant studies showed the qacE and qac△E1 genes of CRAb isolates associated with a higher minimum inhibitory concentration and less susceptible to benzalkonium bromide and chlorhexidine gluconate (Guo and Li, 2019). Besides, enhanced environmental cleaning alone may be insufficient to curb the acquisition of CRAb in a highly endemic ICU. Simply increasing the frequency of cleaning without enhancement of other bundle interventions for CRAb reduction did not lead to a significant reduction in CRAb acquisition (Seok et al., 2021). Multiple factors like invasive therapies (Seok et al., 2021) and unique biological characteristics, particularly for isolates forming biofilms (Espinal et al., 2012), causing re-contamination during the disinfection interval. This reminds us to improve the cleaning standards for patients receiving different treatments, and pay more attention to the quality of single cleaning and the interval between two cleanings, with the goal of refining cleaning procedures to effectively diminish clinical exposure.
Enhancing the sensitivity and efficiency of detection would be a good way to decrease the colonization risk of CRAb. Previous evidence from simulation models demonstrates the potential benefits of active surveillance (Lee et al., 2011). One of the model has demonstrated that as screening sensitivity approaches 90%, the reduction in transmissions, infections, and deaths can reach 78% (with a range of 77%–80%), accompanied by cost savings between 22% and 53%, when the prevalence of carriage is between 2% and 6% (Coyle et al., 2014). Besides, since the actual treatment plan largely depends on the patient’s test results, false-positive test results or empirical antibiotic misuse due to diminished testing efficiency are leading contributors to some CRAb colonization cases. Especially during the COVID-19 pandemic, patients overload caused delayed culture and drug sensitivity results in microbiological laboratories, leading to inappropriate antibiotic treatment and resulting in widespread CRAb colonization in ICUs (Abelenda-Alonso et al., 2020; Vaughn et al., 2021). Therefore, improve detection technology is an effective strategy to alleviate CRAb colonization caused by unreasonable treatment.
Culture and polymerase chain reaction (PCR) are traditionally considered the most common methods for pathogen epidemiological screening. Recently, researchers have used loop-mediated isothermal amplification (LAMP) technology to detect A. baumannii and carbapenem antibiotic resistance. They found LAMP to be a promising method for early detection (Garciglia-Mercado et al., 2021; Sharma and Gaind, 2021). LAMP’s sensitivity is 10 times higher than PCR (Garciglia-Mercado et al., 2021), and apart from LAMP, whole-genome sequencing (WGS) is also a valuable tool in epidemiological research (Ng et al., 2018; Venditti et al., 2019). In fact, high sequencing costs and lacking professional testing technicians resulting in only some regions having the conditions to implement the test that affect the widely used of WGS. Perhaps establishing a convenient and efficient testing industry chain can better solve the problems such as cost and specimen transportation, thereby better serving surrounding medical institutions. Overall, LAMP appears to be an effective screening method for CRAB epidemiological investigations, effectively balancing sensitivity with detection costs (Hong et al., 2018).
Furthermore, there is a need to stress hospital awareness education for healthcare personnel and enhance guidelines compliance such as hand hygiene. Previous researches had indicated that enhanced adherence to hand hygiene protocols correlates with a reduction of healthcare-associated infections in general and multidrug-resistant organisms (Lotfinejad et al., 2021). Although hand hygiene might seem simple, compliance in healthcare settings has always been far from ideal worldwide (Gould et al., 2017). In a related study, we found that hand hygiene compliance before patient contact is relatively low, indicating a disregard of hospital infection prevention and control principles by healthcare personnel, and this leads high occurrence of nasal pathogenic bacteria colonization among ICUs medical staff (Xu et al., 2019b). Actually, available evidence shows that compliance with hand hygiene recommendations during healthcare delivery remains suboptimal around the world, with an average of 59.6% compliance levels in intensive care units up to 2018, and extreme differences between high income and low income countries (64.5% vs. 9.1%) (de Kraker et al., 2022). Besides, some research found the seniority of medical workers also correlated with the incidence and prevalence of CRAb acquisition. In our previous research, we found that nursing staff with lower seniority are more prone to bacterial colonization. This could be explained that despite receiving standardized training, junior nursing staff often possess less experience or are prone to making more errors, which consequently leads to their performance not matching the excellence demonstrated by their senior counterparts (Xu et al., 2019b; Seok et al., 2021). Therefore, further optimization of hand hygiene education and supervision is urgently needed.
World Health Organization designed “The My 5 Moments for Hand Hygiene approach,” which helped to highlight hand hygiene indications to facilitate understanding, training, and monitoring in a broad range of healthcare settings worldwide. This concept has gained universal acceptance and serves as the foundation of WHO’s multimodal strategy for enhancing hand hygiene. However, the accumulated evidence has suggested that multimodal promotion strategies are more effective than single interventions in changing healthcare workers’ behavior, considering the complex and multifactorial determinants of hand hygiene compliance (Allegranzi et al., 2013; Zingg et al., 2015). Bundled interventions that incorporating the multimodal hand hygiene improvement strategies include additional measures such as goal setting, reward incentives, and accountability, have demonstrated promising outcomes in boosting compliance (Luangasanatip et al., 2015). In summary, more implementation strategies should be combined with different regions and cultures to better motivate and supervise clinical workers.
Unreasonable medical practice is also an important risk factor for the occurrence of CRAb colonization, so it is important to follow strict antibiotic use guidelines and to carry out adequate assessment before performing invasive procedures. In addition, strict and standardized disinfection and aseptic operations are also important guarantees for preventing bacterial colonization and infection.
Honestly, according to a guideline reported by ESCMID-EUCIC (European Committee of Infection Control-European Society of Clinical Microbiology and Infectious Diseases), there is not sufficient evidence suggesting the positive effects brought by decolonization intervention (Tacconelli et al., 2019). However, some measures significantly increased the 14-day eradication of CRAb that including daily 4% chlorhexidine body wash, inhaled colistin (160 mg twice daily), colistin sulfate [50 mg (salt) four times daily] and tobramycin (80 mg four times daily) (Agustí et al., 2002; Borer et al., 2007; Kuo et al., 2012; Chen et al., 2014). Based on the current situation, we believe that there will be more research guiding a better response to the challenge brought by CRAb colonization in the future (Figure 4).
Figure 4. Proposal bundle to prevent CRAb colonization. The figure summarized four aspects of preventive measures.
Carbapenem-resistant Acinetobacter baumannii colonization, as a relatively overlooked topic in the current research field of drug-resistant bacteria, has received increasing attention in recent years. It is undeniable that early intervention in the occurrence and development of colonization issues is extremely important and urgent. Preventing the colonization of CRAb can better improve the patient’s prognosis, shorten hospitalization time, and save related medical expenses; on the other hand, it also saves valuable medical resources, enabling clinical workers to carry out medical work more efficiently. In addition to more efficient detection techniques, there is still a lack of research on whether specific clones are responsible for both environmental colonization and ICUs infection (Jiang et al., 2022). But we advocate that more clinical workers should pay attention to the impact of CRAb colonization and provide more comprehensive ideas for subsequent individualized treatment.
SZ: Visualization, Writing – original draft, Writing – review & editing. JX: Supervision, Visualization, Writing – review & editing. YNL: Writing – review & editing. WL: Visualization, Writing – review & editing. YHL: Writing – review & editing. MP: Writing – review & editing. MY: Writing – review & editing. HH: Writing – review & editing. YC: Writing – review & editing. XZ: Writing – review & editing. HW: Conceptualization, Funding acquisition, Supervision, Writing – review & editing.
The author(s) declare financial support was received for the research, authorship, and/or publication of this article. This work was supported by the National Natural Science Foundation of China (grant no. 82072231), Natural Science Foundation of Shandong Province (ZR2023YQ068), and Taishan Scholars Program of Shandong Province (award no. tsqn202103165).
We would like to thank all participants in preparing this review. Besides, Figures 1–4 were created with BioRender.com.
The authors declare that the research was conducted in the absence of any commercial or financial relationships that could be construed as a potential conflict of interest.
The authors declare that no Generative AI was used in the creation of this manuscript.
All claims expressed in this article are solely those of the authors and do not necessarily represent those of their affiliated organizations, or those of the publisher, the editors and the reviewers. Any product that may be evaluated in this article, or claim that may be made by its manufacturer, is not guaranteed or endorsed by the publisher.
CRAb, carbapenem-resistant Acinetobacter baumannii; ICUs, intensive care units; A. baumannii, Acinetobacter baumannii; NFGNB, non-fermenting Gram-negative bacilli; CHINET, China Antimicrobial Resistance Surveillance System; WHO, World Health Organization; ARGs, antibiotic resistance genes; PPE, personal protective equipment; PCR, polymerase chain reaction; LAMP, loop-mediated isothermal amplification; WGS, whole-genome sequencing.
Abelenda-Alonso, G., Padullés, A., Rombauts, A., Gudiol, C., Pujol, M., Alvarez-Pouso, C., et al. (2020). Antibiotic prescription during the COVID-19 pandemic: A biphasic pattern. Infect. Control Hosp. Epidemiol. 41, 1371–1372.
Agarwal, S., Kakati, B., Khanduri, S., and Gupta, S. (2017). Emergence of carbapenem resistant non-fermenting gram-negative bacilli isolated in an ICU of a tertiary care hospital. J. Clin. Diagn. Res. 11, Dc04–Dc07. doi: 10.7860/jcdr/2017/24023.9317
Agustí, C., Pujol, M., Argerich, M. J., Ayats, J., Badía, M., Domínguez, M. A., et al. (2002). Short-term effect of the application of selective decontamination of the digestive tract on different body site reservoir ICU patients colonized by multi-resistant Acinetobacter baumannii. J. Antimicrob. Chemother. 49, 205–208. doi: 10.1093/jac/49.1.205
Alfandari, S., Gois, J., Delannoy, P. Y., Georges, H., Boussekey, N., Chiche, A., et al. (2014). Management and control of a carbapenem-resistant Acinetobacter baumannii outbreak in an intensive care unit. Med. Mal. Infect. 44, 229–231.
Al-Hamad, A., Pal, T., Leskafi, H., Abbas, H., Hejles, H., Alsubikhy, F., et al. (2020). Molecular characterization of clinical and environmental carbapenem resistant Acinetobacter baumannii isolates in a hospital of the Eastern Region of Saudi Arabia. J. Infect. Public Health 13, 632–636. doi: 10.1016/j.jiph.2019.08.013
Allegranzi, B., Gayet-Ageron, A., Damani, N., Bengaly, L., McLaws, M. L., Moro, M. L., et al. (2013). Global implementation of WHO’s multimodal strategy for improvement of hand hygiene: A quasi-experimental study. Lancet Infect. Dis. 13, 843–851. doi: 10.1016/s1473-3099(13)70163-4
Antimicrobial Resistance Collaborators (2022a). The burden of bacterial antimicrobial resistance in the WHO European region in 2019: A cross-country systematic analysis. Lancet Pub. Heal. 7, e897–e913. doi: 10.1016/s2468-2667(22)00225-0
Antimicrobial Resistance Collaborators (2022b). Global burden of bacterial antimicrobial resistance in 2019: A systematic analysis. Lancet 399, 629–655. doi: 10.1016/s0140-6736(21)02724-0
Arzanlou, M., Chai, W. C., and Venter, H. (2017). Intrinsic, adaptive and acquired antimicrobial resistance in Gram-negative bacteria. Essays Biochem. 61, 49–59. doi: 10.1042/ebc20160063
Ayats, J., Corbella, X., Ardanuy, C., Domínguez, M. A., Ricart, A., Ariza, J., et al. (1997). Epidemiological significance of cutaneous, pharyngeal, and digestive tract colonization by multiresistant Acinetobacter baumannii in ICU patients. J. Hosp. Infect. 37, 287–295.
Ayobami, O., Willrich, N., Harder, T., Okeke, I. N., Eckmanns, T., and Markwart, R. (2019). The incidence and prevalence of hospital-acquired (carbapenem-resistant) Acinetobacter baumannii in Europe, Eastern Mediterranean and Africa: A systematic review and meta-analysis. Emerg. Microbes. Infect. 8, 1747–1759. doi: 10.1080/22221751.2019.1698273
Barin, J., Martins, A. F., Heineck, B. L., Barth, A. L., and Zavascki, A. P. (2013). Hetero- and adaptive resistance to polymyxin B in OXA-23-producing carbapenem-resistant Acinetobacter baumannii isolates. Ann. Clin. Microbiol. Antimicrob. 12:15. doi: 10.1186/1476-0711-12-15
Bartal, C., Rolston, K. V. I., and Nesher, L. (2022). Carbapenem-resistant Acinetobacter baumannii: Colonization, infection and current treatment options. Infect. Dis. Ther. 11, 683–694. doi: 10.1007/s40121-022-00597-w
Biswas, D., Tiwari, M., and Tiwari, V. (2018). Comparative mechanism based study on disinfectants against multidrug-resistant Acinetobacter baumannii. J. Cell. Biochem. 119, 10314–10326. doi: 10.1002/jcb.27373
Borer, A., Gilad, J., Porat, N., Megrelesvilli, R., Saidel-Odes, L., Peled, N., et al. (2007). Impact of 4% chlorhexidine whole-body washing on multidrug-resistant Acinetobacter baumannii skin colonisation among patients in a medical intensive care unit. J. Hosp. Infect. 67, 149–155. doi: 10.1016/j.jhin.2007.07.023
Cavallo, I., Oliva, A., Pages, R., Sivori, F., Truglio, M., Fabrizio, G., et al. (2023). Acinetobacter baumannii in the critically ill: Complex infections get complicated. Front. Microbiol. 14:1196774. doi: 10.3389/fmicb.2023.1196774
Chen, Y. M., Fang, W. F., Kao, H. C., Chen, H. C., Tsai, Y. C., Shen, L. S., et al. (2014). Influencing factors of successful eradication of multidrug-resistant Acinetobacter baumannii in the respiratory tract with aerosolized colistin. Biomed. J. 37, 314–320. doi: 10.4103/2319-4170.132879
Cheng, V. C., Chen, J. H., So, S. Y., Wong, S. C., Yan, M. K., Chau, P. H., et al. (2015). Use of fluoroquinolones is the single most important risk factor for the high bacterial load in patients with nasal and gastrointestinal colonization by multidrug-resistant Acinetobacter baumannii. Eur. J. Clin. Microbiol. Infect. Dis. 34, 2359–2366. doi: 10.1007/s10096-015-2489-4
Chung, Y. K., Kim, J. S., Lee, S. S., Lee, J. A., Kim, H. S., Shin, K. S., et al. (2015). Effect of daily chlorhexidine bathing on acquisition of carbapenem-resistant Acinetobacter baumannii (CRAB) in the medical intensive care unit with CRAB endemicity. Am. J. Infect. Control 43, 1171–1177. doi: 10.1016/j.ajic.2015.07.001
Cogliati Dezza, F., Covino, S., Petrucci, F., Sacco, F., Viscido, A., Gavaruzzi, F., et al. (2023). Risk factors for carbapenem-resistant Acinetobacter baumannii (CRAB) bloodstream infections and related mortality in critically ill patients with CRAB colonization. JAC Antimicrob. Resist. 5:dlad096. doi: 10.1093/jacamr/dlad096
Coyle, J. R., Kaye, K. S., Taylor, T., Tansek, R., Campbell, M., Hayakawa, K., et al. (2014). Effectiveness and cost of implementing an active surveillance screening policy for Acinetobacter baumannii: A Monte Carlo simulation model. Am. J. Infect. Control 42, 283–287. doi: 10.1016/j.ajic.2013.09.027
Coyne, S., Courvalin, P., and Périchon, B. (2011). Efflux-mediated antibiotic resistance in Acinetobacter spp. Antimicrob. Agents Chemother. 55, 947–953. doi: 10.1128/aac.01388-10
de Kraker, M. E. A., Tartari, E., Tomczyk, S., Twyman, A., Francioli, L. C., Cassini, A., et al. (2022). Implementation of hand hygiene in health-care facilities: Results from the WHO hand hygiene self-assessment framework global survey 2019. Lancet Infect. Dis. 22, 835–844. doi: 10.1016/s1473-3099(21)00618-6
Desmoulin, A., Sababadichetty, L., Kamus, L., Daniel, M., Feletti, L., Allou, N., et al. (2024). Adaptive resistance to cefiderocol in carbapenem-resistant Acinetobacter baumannii (CRAB): Microbiological and clinical issues. Heliyon 10:e30365. doi: 10.1016/j.heliyon.2024.e30365
Eigenbrod, T., Reuter, S., Gross, A., Kocer, K., Günther, F., Zimmermann, S., et al. (2019). Molecular characterization of carbapenem-resistant Acinetobacter baumannii using WGS revealed missed transmission events in Germany from 2012-15. J. Antimicrob. Chemother. 74, 3473–3480. doi: 10.1093/jac/dkz360
Ejaz, H., Ahmad, M., Younas, S., Junaid, K., Abosalif, K. O. A., Abdalla, A. E., et al. (2021). Molecular epidemiology of extensively-drug resistant Acinetobacter baumannii sequence type 2 Co-Harboring bla NDM and bla OXA from clinical origin. Infect. Drug Resist. 14, 1931–1939.
Espinal, P., Martí, S., and Vila, J. (2012). Effect of biofilm formation on the survival of Acinetobacter baumannii on dry surfaces. J. Hosp. Infect. 80, 56–60. doi: 10.1016/j.jhin.2011.08.013
European Antimicrobial Resistance Collaborators (2023). The burden of antimicrobial resistance in the Americas in 2019: A cross-country systematic analysis. Lancet Reg. Health Am. 25:100561. doi: 10.1016/j.lana.2023.100561
Freire, M. P., Van Der Heijden, I. M., do Prado, G. V., Cavalcante, L. S., Boszczowski, I., Bonazzi, P. R., et al. (2014). Polymyxin use as a risk factor for colonization or infection with polymyxin-resistant Acinetobacter baumannii after liver transplantation. Transpl. Infect. Dis. 16, 369–378. doi: 10.1111/tid.12210
Garciglia-Mercado, C., Gaxiola-Robles, R., Ascencio, F., Grajales-Muñiz, C., Soriano Rodríguez, M. L., Silva-Sanchez, J., et al. (2021). Antibacterial effect of acetic acid during an outbreak of carbapenem-resistant Acinetobacter baumannii in an ICU (II). J. Infect. Dev. Ctries 15, 1167–1172.
Gorrie, C. L., Mirceta, M., Wick, R. R., Edwards, D. J., Thomson, N. R., Strugnell, R. A., et al. (2017). Gastrointestinal carriage is a major reservoir of Klebsiella pneumoniae infection in intensive care patients. Clin. Infect. Dis. 65, 208–215.
Gould, D. J., Moralejo, D., Drey, N., Chudleigh, J. H., and Taljaard, M. (2017). Interventions to improve hand hygiene compliance in patient care. Cochrane. Database Syst. Rev. 9:Cd005186. doi: 10.1002/14651858.CD005186.pub4
Greene, C., Vadlamudi, G., Newton, D., Foxman, B., and Xi, C. (2016a). The influence of biofilm formation and multidrug resistance on environmental survival of clinical and environmental isolates of Acinetobacter baumannii. Am. J. Infect. Control 44, e65–e71. doi: 10.1016/j.ajic.2015.12.012
Greene, C., Wu, J., Rickard, A. H., and Xi, C. (2016b). Evaluation of the ability of Acinetobacter baumannii to form biofilms on six different biomedical relevant surfaces. Lett. Appl. Microbiol. 63, 233–239. doi: 10.1111/lam.12627
Guo, J., and Li, C. (2019). Molecular epidemiology and decreased susceptibility to disinfectants in carbapenem-resistant Acinetobacter baumannii isolated from intensive care unit patients in central China. J. Infect. Public Health 12, 890–896. doi: 10.1016/j.jiph.2019.06.007
Hamidian, M., and Nigro, S. J. (2019). Emergence, molecular mechanisms and global spread of carbapenem-resistant Acinetobacter baumannii. Microb. Genom. 5:e000306. doi: 10.1099/mgen.0.000306
Harding, C. M., Hennon, S. W., and Feldman, M. F. (2018). Uncovering the mechanisms of Acinetobacter baumannii virulence. Nat. Rev. Microbiol. 16, 91–102. doi: 10.1038/nrmicro.2017.148
He, S., Li, Z., Yang, Q., Quan, M., Zhao, L., and Hong, Z. (2020). Resistance trends among 1,294 nosocomial Acinetobacter baumannii Strains from a tertiary general hospital in China, 2014 - 2017. Clin. Lab. 66. doi: 10.7754/Clin.Lab.2019.190629
Higgins, P. G., Dammhayn, C., Hackel, M., and Seifert, H. (2010). Global spread of carbapenem-resistant Acinetobacter baumannii. J. Antimicrob. Chemother. 65, 233–238. doi: 10.1093/jac/dkp428
Higgins, P. G., Pérez-Llarena, F. J., Zander, E., Fernández, A., Bou, G., and Seifert, H. (2013). OXA-235, a novel class D β-lactamase involved in resistance to carbapenems in Acinetobacter baumannii. Antimicrob. Agents Chemother. 57, 2121–2126. doi: 10.1128/aac.02413-12
Hong, J., Jang, O. J., Bak, M. H., Baek, E. H., Park, K.-H., Hong, S. I., et al. (2018). Management of carbapenem-resistant Acinetobacter baumannii epidemic in an intensive care unit using multifaceted intervention strategy. Korean J. Intern. Med. 33, 1000–1007.
Hu, H., Wang, Y., Sun, J., Wang, Y., Zhou, J., Shi, Q., et al. (2024). Risk factors and molecular epidemiology of intestinal colonization by carbapenem-resistant Gram-negative bacteria in patients with hematological diseases: A multicenter case-control study. Microbiol. Spectr. 12:e0429923. doi: 10.1128/spectrum.04299-23
Jiang, Y., Ding, Y., Wei, Y., Jian, C., Liu, J., and Zeng, Z. (2022). Carbapenem-resistant Acinetobacter baumannii: A challenge in the intensive care unit. Front. Microbiol. 13:1045206. doi: 10.3389/fmicb.2022.1045206
Juan, C. H., Chuang, C., Chen, C. H., Li, L., and Lin, Y. T. (2019). Clinical characteristics, antimicrobial resistance and capsular types of community-acquired, healthcare-associated, and nosocomial Klebsiella pneumoniae bacteremia. Antimicrob. Resist. Infect. Control. 8:1. doi: 10.1186/s13756-018-0426-x
Karampatakis, T., Tsergouli, K., Iosifidis, E., Antachopoulos, C., Karapanagiotou, A., Karyoti, A., et al. (2018). Impact of active surveillance and infection control measures on carbapenem-resistant Gram-negative bacterial colonization and infections in intensive care. J. Hosp. Infect. 99, 396–404.
Kousouli, E., Zarkotou, O., Polimeri, K., Themeli-Digalaki, K., and Pournaras, S. (2019). Impact of bloodstream infections caused by carbapenem-resistant Gram-negative pathogens on ICU costs, mortality and length of stay. Infect. Prev. Pract. 1:100020.
Kuo, S. C., Lee, Y. T., Yang, S. P., Chen, C. P., Chen, T. L., Hsieh, S. L., et al. (2012). Eradication of multidrug-resistant Acinetobacter baumannii from the respiratory tract with inhaled colistin methanesulfonate: A matched case-control study. Clin. Microbiol. Infect. 18, 870–876. doi: 10.1111/j.1469-0691.2011.03682.x
Langford, B. J., So, M., Raybardhan, S., Leung, V., Westwood, D., MacFadden, D. R., et al. (2020). Bacterial co-infection and secondary infection in patients with COVID-19: A living rapid review and meta-analysis. Clin. Microbiol. Infect. 26, 1622–1629.
Latibeaudiere, R., Rosa, R., Laowansiri, P., Arheart, K., Namias, N., and Munoz-Price, L. S. (2015). Surveillance cultures growing carbapenem-Resistant Acinetobacter baumannii predict the development of clinical infections: A retrospective cohort study. Clin. Infect. Dis. 60, 415–422.
Lăzureanu, V., Poroşnicu, M., Gândac, C., Moisil, T., Bădiţoiu, L., Laza, R., et al. (2016). Infection with Acinetobacter baumannii in an intensive care unit in the Western part of Romania. BMC Infect. Dis. 16:95. doi: 10.1186/s12879-016-1399-0
Lee, B. Y., McGlone, S. M., Doi, Y., Bailey, R. R., and Harrison, L. H. (2011). Economic value of Acinetobacter baumannii screening in the intensive care unit. Clin. Microbiol. Infect. 17, 1691–1697. doi: 10.1111/j.1469-0691.2011.03491.x
Lerner, A. O., Abu-Hanna, J., Carmeli, Y., and Schechner, V. (2020). Environmental contamination by carbapenem-resistant Acinetobacter baumannii: The effects of room type and cleaning methods. Infect. Control Hosp. Epidemiol. 41, 166–171. doi: 10.1017/ice.2019.307
Li, X. Z., Plésiat, P., and Nikaido, H. (2015). The challenge of efflux-mediated antibiotic resistance in Gram-negative bacteria. Clin. Microbiol. Rev. 28, 337–418. doi: 10.1128/cmr.00117-14
Li, Y., Ge, H., Zhou, H., Zhou, W., Zheng, J., Chen, W., et al. (2021). Impact of environmental cleaning on the colonization and infection rates of multidrug-resistant Acinetobacter baumannii in patients within the intensive care unit in a tertiary hospital. Antimicrob. Resist. Infect. Control 10:4. doi: 10.1186/s13756-020-00870-y
Liu, C., Chen, K., Wu, Y., Huang, L., Fang, Y., Lu, J., et al. (2022). Epidemiological and genetic characteristics of clinical carbapenem-resistant Acinetobacter baumannii strains collected countrywide from hospital intensive care units (ICUs) in China. Emerg. Microbes Infect. 11, 1730–1741.
Liu, Y., Wang, Q., Zhao, C., Chen, H., Li, H., Wang, H., et al. (2020). Prospective multi-center evaluation on risk factors, clinical characteristics and outcomes due to carbapenem resistance in Acinetobacter baumannii complex bacteraemia: Experience from the Chinese antimicrobial resistance surveillance of nosocomial infections (CARES) network. J. Med. Microbiol. 69, 949–959.
Lotfinejad, N., Peters, A., Tartari, E., Fankhauser-Rodriguez, C., Pires, D., and Pittet, D. (2021). Hand hygiene in health care: 20 years of ongoing advances and perspectives. Lancet Infect. Dis. 21, e209–e221. doi: 10.1016/s1473-3099(21)00383-2
Luangasanatip, N., Hongsuwan, M., Limmathurotsakul, D., Lubell, Y., Lee, A. S., Harbarth, S., et al. (2015). Comparative efficacy of interventions to promote hand hygiene in hospital: Systematic review and network meta-analysis. BMJ 351:h3728. doi: 10.1136/bmj.h3728
Maamar, E., Alonso, C. A., Ferjani, S., Jendoubi, A., Hamzaoui, Z., Jebri, A., et al. (2018). NDM-1- and OXA-23-producing Acinetobacter baumannii isolated from intensive care unit patients in Tunisia. Int. J. Antimicrob. Agents 52, 910–915.
Meschiari, M., Kaleci, S., Orlando, G., Selmi, S., Santoro, A., Bacca, E., et al. (2021a). Risk factors for nosocomial rectal colonization with carbapenem-resistant Acinetobacter baumannii in hospital: A matched case-control study. Antimicrob. Resist. Infect. Control 10:69. doi: 10.1186/s13756-021-00919-6
Meschiari, M., Lòpez-Lozano, J. M., Di Pilato, V., Gimenez-Esparza, C., Vecchi, E., Bacca, E., et al. (2021b). A five-component infection control bundle to permanently eliminate a carbapenem-resistant Acinetobacter baumannii spreading in an intensive care unit. Antimicrob. Resist. Infect. Control 10:123. doi: 10.1186/s13756-021-00990-z
Metan, G., Zarakolu, P., Otlu, B., Tekin, Ý, Aytaç, H., Bölek, E. Ç, et al. (2020). Emergence of colistin and carbapenem-resistant Acinetobacter calcoaceticus-Acinetobacter baumannii (CCR-Acb) complex in a neurological intensive care unit followed by successful control of the outbreak. J. Infect. Public Health 13, 564–570.
Moghnieh, R., Siblani, L., Ghadban, D., El Mchad, H., Zeineddine, R., Abdallah, D., et al. (2016). Extensively drug-resistant Acinetobacter baumannii in a Lebanese intensive care unit: Risk factors for acquisition and determination of a colonization score. J. Hosp. Infect. 92, 47–53.
Mugnier, P. D., Poirel, L., and Nordmann, P. (2009). Functional analysis of insertion sequence ISAba1, responsible for genomic plasticity of Acinetobacter baumannii. J. Bacteriol. 191, 2414–2418. doi: 10.1128/jb.01258-08
Müller, C., Reuter, S., Wille, J., Xanthopoulou, K., Stefanik, D., Grundmann, H., et al. (2023). A global view on carbapenem-resistant Acinetobacter baumannii. mBio 14:e0226023. doi: 10.1128/mbio.02260-23
Munoz-Price, L. S., Rosa, R., Castro, J. G., Laowansiri, P., Latibeaudiere, R., Namias, N., et al. (2016). Evaluating the impact of antibiotic exposures as time-dependent variables on the acquisition of carbapenem-resistant Acinetobacter baumannii. Crit. Care Med. 44, e949–e956.
Neves, F. C., Clemente, W. T., Lincopan, N., Paiăo, I. D., Neves, P. R., Romanelli, R. M., et al. (2016). Clinical and microbiological characteristics of OXA-23- and OXA-143-producing Acinetobacter baumannii in ICU patients at a teaching hospital. Braz. J. Infect. Dis. 20, 556–563.
Ng, D. H. L., Marimuthu, K., Lee, J. J., Khong, W. X., Ng, O. T., Zhang, W., et al. (2018). Environmental colonization and onward clonal transmission of carbapenem-resistant Acinetobacter baumannii (CRAB) in a medical intensive care unit: The case for environmental hygiene. Antimicrob. Resist. Infect. Control 7:51. doi: 10.1186/s13756-018-0343-z
Nguyen, M., and Joshi, S. G. (2021). Carbapenem resistance in Acinetobacter baumannii, and their importance in hospital-acquired infections: A scientific review. J. Appl. Microbiol. 131, 2715–2738. doi: 10.1111/jam.15130
Nowak, P., and Paluchowska, P. (2016). Acinetobacter baumannii: Biology and drug resistance - role of carbapenemases. Folia Histochem. Cytobiol. 54, 61–74. doi: 10.5603/FHC.a2016.0009
Nutman, A., Lerner, A., Schwartz, D., and Carmeli, Y. (2016). Evaluation of carriage and environmental contamination by carbapenem-resistant Acinetobacter baumannii. Clin. Microbiol. Infect. 22, 949.e945–949.e947. doi: 10.1016/j.cmi.2016.08.020
Odih, E. E., Irek, E. O., Obadare, T. O., Oaikhena, A. O., Afolayan, A. O., Underwood, A., et al. (2022). Rectal colonization and nosocomial transmission of carbapenem-resistant Acinetobacter baumannii in an intensive care unit, Southwest Nigeria. Front. Med. 9:846051. doi: 10.3389/fmed.2022.846051
Pascale, R., Bussini, L., Gaibani, P., Bovo, F., Fornaro, G., Lombardo, D., et al. (2022). Carbapenem-resistant bacteria in an intensive care unit during the coronavirus disease 2019 (COVID-19) pandemic: A multicenter before-and-after cross-sectional study. Infect. Control Hosp. Epidemiol. 43, 461–466.
Phu, V. D., Nadjm, B., Duy, N. H. A., Co, D. X., Mai, N. T. H., Trinh, D. T., et al. (2017). Ventilator-associated respiratory infection in a resource-restricted setting: Impact and etiology. J. Intensive Care 5:69.
Pires, D., Soule, H., Bellissimo-Rodrigues, F., Gayet-Ageron, A., and Pittet, D. (2017). Hand hygiene with alcohol-based hand rub: How long is long enough? Infect. Control Hosp. Epidemiol. 38, 547–552.
Rizk, N. A., Zahreddine, N., Haddad, N., Ahmadieh, R., Hannun, A., Bou Harb, S., et al. (2022). The impact of antimicrobial stewardship and infection control interventions on Acinetobacter baumannii resistance rates in the ICU of a tertiary care center in lebanon. Antibiotics 11:911. doi: 10.3390/antibiotics11070911
Roca, I., Espinal, P., Vila-Farrés, X., and Vila, J. (2012). The Acinetobacter baumannii oxymoron: Commensal hospital dweller turned pan-drug-resistant menace. Front. Microbiol. 3:148. doi: 10.3389/fmicb.2012.00148
Rosa, R., Depascale, D., Cleary, T., Fajardo-Aquino, Y., Kett, D. H., and Munoz-Price, L. S. (2014). Differential environmental contamination with Acinetobacter baumannii based on the anatomic source of colonization. Am. J. Infect. Control 42, 755–757. doi: 10.1016/j.ajic.2014.03.016
Russotto, V., Cortegiani, A., Raineri, S. M., and Giarratano, A. (2015). Bacterial contamination of inanimate surfaces and equipment in the intensive care unit. J. Intensive Care 3:54.
Rutala, W. A., and Weber, D. J. (2019). Best practices for disinfection of noncritical environmental surfaces and equipment in health care facilities: A bundle approach. Am. J. Infect. Control 47s, A96–A105. doi: 10.1016/j.ajic.2019.01.014
Sen, B., and Joshi, S. G. (2016). Studies on Acinetobacter baumannii involving multiple mechanisms of carbapenem resistance. J. Appl. Microbiol. 120, 619–629. doi: 10.1111/jam.13037
Seok, H., Jeon, J. H., Jung, J. H., Ahn, S. H., Seo, M., Cho, H. K., et al. (2021). Does enhanced environmental cleaning reduce carbapenem-resistant Acinetobacter baumannii colonization in the intensive care unit? Int. J. Infect. Dis. 109, 72–76.
Sharma, A., and Gaind, R. (2021). Development of loop-mediated isothermal amplification assay for detection of clinically significant members of acinetobacter calcoaceticus-baumannii complex and associated carbapenem resistance. Front. Mol. Biosci. 8:659256. doi: 10.3389/fmolb.2021.659256
Sharma, S., Das, A., Garg, R., Pramanik, S., Marndi, P., Singh, R., et al. (2022). Reservoir of carbapenem-resistant Acinetobacter baumannii in the hospital environment and colonization pressure: A surveillance-based study in indian intensive care unit. Microb. Drug Resist. 28, 1079–1086. doi: 10.1089/mdr.2022.0088
Shimose, L. A., Masuda, E., Sfeir, M., Berbel Caban, A., Bueno, M. X., dePascale, D., et al. (2016). Carbapenem-resistant Acinetobacter baumannii: Concomitant contamination of air and environmental surfaces. Infect. Control Hosp. Epidemiol. 37, 777–781. doi: 10.1017/ice.2016.69
Skiada, A., Markogiannakis, A., Plachouras, D., and Daikos, G. L. (2011). Adaptive resistance to cationic compounds in Pseudomonas aeruginosa. Int. J. Antimicrob. Agents 37, 187–193. doi: 10.1016/j.ijantimicag.2010.11.019
Suh, J. W., Kim, N. H., Lee, M. J., Lee, S. E., Chun, B. C., Lee, C. K., et al. (2021). Real-world experience of how chlorhexidine bathing affects the acquisition and incidence of vancomycin-resistant enterococci (VRE) in a medical intensive care unit with VRE endemicity: A prospective interrupted time-series study. Antimicrob. Resist. Infect. Control. 10:160.
Tacconelli, E., Mazzaferri, F., de Smet, A. M., Bragantini, D., Eggimann, P., Huttner, B. D., et al. (2019). ESCMID-EUCIC clinical guidelines on decolonization of multidrug-resistant Gram-negative bacteria carriers. Clin. Microbiol. Infect. 25, 807–817. doi: 10.1016/j.cmi.2019.01.005
Thom, K. A., Johnson, J. K., Lee, M. S., and Harris, A. D. (2011). Environmental contamination because of multidrug-resistant Acinetobacter baumannii surrounding colonized or infected patients. Am. J. Infect. Control 39, 711–715. doi: 10.1016/j.ajic.2010.09.005
Thoma, R., Seneghini, M., Seiffert, S. N., Vuichard Gysin, D., Scanferla, G., Haller, S., et al. (2022). The challenge of preventing and containing outbreaks of multidrug-resistant organisms and Candida auris during the coronavirus disease 2019 pandemic: Report of a carbapenem-resistant Acinetobacter baumannii outbreak and a systematic review of the literature. Antimicrob. Resist. Infect. Control. 11:12.
Toyofuku, M., Inaba, T., Kiyokawa, T., Obana, N., Yawata, Y., and Nomura, N. (2016). Environmental factors that shape biofilm formation. Biosci. Biotechnol. Biochem. 80, 7–12. doi: 10.1080/09168451.2015.1058701
Uwingabiye, J., Lemnouer, A., Roca, I., Alouane, T., Frikh, M., Belefquih, B., et al. (2017). Clonal diversity and detection of carbapenem resistance encoding genes among multidrug-resistant Acinetobacter baumannii isolates recovered from patients and environment in two intensive care units in a Moroccan hospital. Antimicrob. Resist. Infect. Control. 6:99. doi: 10.1186/s13756-017-0262-4
Vaughn, V. M., Gandhi, T. N., Petty, L. A., Patel, P. K., Prescott, H. C., Malani, A. N., et al. (2021). Empiric Antibacterial therapy and community-onset bacterial coinfection in patients hospitalized with coronavirus disease 2019 (COVID-19): A multi-hospital cohort study. Clin. Infect. Dis. 72, e533–e541.
Venditti, C., Vulcano, A., D’Arezzo, S., Gruber, C. E. M., Selleri, M., Antonini, M., et al. (2019). Epidemiological investigation of an Acinetobacter baumannii outbreak using core genome multilocus sequence typing. J. Global Antimicrob. Resist. 17, 245–249.
Weiss, N., Faugeras, F., Rohaut, B., Leconte, J., Lafeuille, E., Brossier, F., et al. (2016). Multidrug-resistant bacteria transmitted through high-density EEG in ICU. Seizure 37, 65–68. doi: 10.1016/j.seizure.2016.03.005
Wong, D., Nielsen, T. B., Bonomo, R. A., Pantapalangkoor, P., Luna, B., and Spellberg, B. (2017). Clinical and pathophysiological overview of acinetobacter infections: A century of challenges. Clin. Microbiol. Rev. 30, 409–447. doi: 10.1128/cmr.00058-16
Wong, S. C., Chen, J. H., Chau, P. H., So, S. Y., AuYeung, C. H., Yuen, L. L., et al. (2022). Gastrointestinal colonization of carbapenem-resistant Acinetobacter baumannii: What is the implication for infection control? Antibiotics 11:1297. doi: 10.3390/antibiotics11101297
World Health Organization (2017). ‘Prioritization of Pathogens to Guide Discovery, Research and Development of New Antibiotics for Drug-Resistant Bacterial Infections, Including Tuberculosis’. Geneva: World Health Organization.
World Health Organization (2024). ‘WHO Bacterial Priority Pathogens List, 2024: Bacterial Pathogens of Public Health Importance, to Guide Research, Development and Strategies to Prevent and Control Antimicrobial Resistance’. Geneva: World Health Organization.
Xiao, T., Guo, Q., Zhou, Y., Shen, P., Wang, Y., Fang, Q., et al. (2022). Comparative respiratory tract microbiome between carbapenem-resistant Acinetobacter baumannii colonization and ventilator associated Pneumonia. Front. Microbiol. 13:782210. doi: 10.3389/fmicb.2022.782210
Xu, N. N., Wang, H., and Zhou, M. (2019a). Analysis of risk factors and prevention strategies of extensive drug - resistant Acinetobacter baumannii infection and colonization in patients with mechanical ventilation. Zhongguo Xiaoduxue Zazhi 36, 439–442.
Xu, N. N., Zhou, M., Sun, E. H., Li, W., Qin, W. D., Zhang, F., et al. (2019b). Nasal pathogenic bacteria colonization and related risk factors among medical staff of intensive care unit. Chin. J. Crit. Care Intensive Care Med. 5, 3–8.
Zhen, X., Stålsby Lundborg, C., Sun, X., Gu, S., and Dong, H. (2020). Clinical and economic burden of carbapenem-resistant infection or colonization caused by Klebsiella pneumoniae, Pseudomonas aeruginosa, Acinetobacter baumannii: A multicenter study in China. Antibiotics 9:514. doi: 10.3390/antibiotics9080514
Zheng, Y., Xu, N. N., Pang, J. J., Han, H., Yang, H. N., Qin, W. D., et al. (2021). Colonization with extensively drug-resistant and prognosis in critically Ill patients: An observational cohort study. Front. Med. 8:667776. doi: 10.3389/fmed.2021.667776
Zingg, W., Holmes, A., Dettenkofer, M., Goetting, T., Secci, F., Clack, L., et al. (2015). Hospital organisation, management, and structure for prevention of health-care-associated infection: A systematic review and expert consensus. Lancet Infect. Dis. 15, 212–224. doi: 10.1016/s1473-3099(14)70854-0
Keywords: CRAb, colonization, risk factors, hazard, prevention
Citation: Zhang S, Xiao J, Li Y, Li W, Li Y, Pang M, Yan M, Han H, Cui Y, Zhang X and Wang H (2025) An integrative review on the risk factors, prevention, and control strategies for carbapenem-resistant Acinetobacter baumannii colonization in critically ill patients. Front. Microbiol. 15:1519906. doi: 10.3389/fmicb.2024.1519906
Received: 30 October 2024; Accepted: 24 December 2024;
Published: 10 January 2025.
Edited by:
Efthymia Giannitsioti, University General Hospital Attikon, GreeceReviewed by:
Polyxeni Karakosta, University General Hospital Attikon, GreeceCopyright © 2025 Zhang, Xiao, Li, Li, Li, Pang, Yan, Han, Cui, Zhang and Wang. This is an open-access article distributed under the terms of the Creative Commons Attribution License (CC BY). The use, distribution or reproduction in other forums is permitted, provided the original author(s) and the copyright owner(s) are credited and that the original publication in this journal is cited, in accordance with accepted academic practice. No use, distribution or reproduction is permitted which does not comply with these terms.
*Correspondence: Hao Wang, d2FuZ2hhbzM0QDEyNi5jb20=
†These authors have contributed equally to this work and share first authorship
Disclaimer: All claims expressed in this article are solely those of the authors and do not necessarily represent those of their affiliated organizations, or those of the publisher, the editors and the reviewers. Any product that may be evaluated in this article or claim that may be made by its manufacturer is not guaranteed or endorsed by the publisher.
Research integrity at Frontiers
Learn more about the work of our research integrity team to safeguard the quality of each article we publish.