- 1Institute of Antibiotics, Huashan Hospital, Fudan University, Shanghai, China
- 2State Key Laboratory of Biotherapy and Cancer Center, West China Hospital, Sichuan University, Chengdu, Sichuan, China
- 3Clinical Pharmacology Research Center, Huashan Hospital, Fudan University, Shanghai, China
- 4Qilu Pharmaceutical Co., Ltd., Jinan, Shandong, China
Introduction: Pralurbactam (FL058) is a novel β-lactamase inhibitor with good inhibitory activity on class A, C, and D β-lactamases. This study aimed to evaluate the pharmacokinetic/pharmacodynamic (PK/PD) relationship of pralurbactam/meropenem in a neutropenic murine thigh infection model.
Methods: After 2-h infection, neutropenic mice was treated with meropenem every 2 h alone or in combination with pralurbactam at different dosing frequencies for 24 h, and the colony count in the thighs was determined before and after treatment. The maximum effect model was fit to the PK/PD relationship to determine the PK/PD index and targets for pralurbactam in combination with meropenem resulting in a static effect and 1-log10 kill.
Results: The plasma drug concentration-time data demonstrated that the PK profiles of pralurbactam were consistent with a one-compartment model. Pralurbactam demonstrated a linear PK profile in mice plasma. The percent time of free drug above 1 mg/L (%fT > 1 mg/L) was the PK/PD index that best described the bacterial killing effect of pralurbactam/meropenem over 24 h. When the PK/PD index %fT > 1 mg/L reached 38.4% and 63.6%, pralurbactam/meropenem combination would achieve bacteriostatic effect and 1-log10 reduction against Klebsiella pneumoniae in thigh bioburden, respectively.
Conclusion: These PK/PD data derived from mouse thigh infection models will be used to inform the optimal dosing regimen of pralurbactam/meropenem combination in clinical trials.
1 Introduction
The clinical use of antimicrobial agents has achieved great success in the treatment of infectious diseases. However, inappropriate use and even abuse of antimicrobial agents have imposed increasing pressure on the pathogenic microorganisms and induce the emergence of antimicrobial resistance due to various mechanisms, which allows the antibiotic-resistant bacteria to escape from killing and so leads to the failure of antimicrobial treatment. One important mechanism of antimicrobial resistance is the production of β-lactamases, which can hydrolyze β-lactam (BL) antibiotics directly. The evolvement of β-lactamases has directly led to the increasing resistance and spread of multidrug-resistant bacteria because of structural variations of the emerging enzymes. According to the results of Antimicrobial Resistance Surveillance System in China (CHINET) (Guo et al., 2022), about 73% of the clinical isolates collected from hospitals across China in 2021 were Gram-negative bacteria, about 60% of which were Enterobacterales. The prevalent β-lactamases-producing Enterobacterales (especially Klebsiella pneumoniae) are difficult to treat in clinical practice because they are resistant to nearly all BLs, including carbapenems such as meropenem and imipenem. The commonly used β-lactamase inhibitors (BLIs) such as clavulanic acid, tazobactam, and sulbactam are effective only against class A and D enzymes but not so active on the emerging carbapenemases such as KPC and NDM (Wang et al., 2018). Several novel BL/BLI combinations have been developed in clinical trials to address the issue of carbapenemase-producing Enterobacterales strains (Drawz et al., 2014).
Pralurbactam (FL058) is a novel BLI with a diazabicyclo structure similar to avibactam that is active against class A, C, and D β-lactamases in vitro (Sharma et al., 2016). Results of the in vitro study showed that pralurbactam in combination with meropenem might be a potential treatment for KPC- and/or OXA-48-producing Enterobacterales infection (Huang et al., 2024a). An in vitro susceptibility study (to be published) demonstrated that pralurbactam alone exhibited notable inhibitory effects on Escherichia coli and meropenem combined with 4 μg/ml pralurbactam had significantly lower the minimum inhibitory concentration (MIC) for NDM-producing E. coli with the MIC90 of 0.5 mg/L and exhibited partial inhibitory activity against NDM-producing K. pneumoniae with MIC50 and MIC90 values of 0.25 and 4 mg/L, respectively. Moreover, a completed phase I clinical trial showed that pralurbactam exhibited good safety, tolerance, and PK profiles (Huang et al., 2024b).
Pharmacokinetic/pharmacodynamic (PK/PD) models are usually used to simulate the PK process of antibiotics in human body due to the flexibility and convenience in experimental design (Drawz et al., 2014). The in vitro PD data combined with the in vivo PK data of different pralurbactam/meropenem combinations can be used to profile the free threshold concentration (CT) of pralurbactam, PK/PD index, and the target values of pralurbactam/meropenem combinations in animal infection models. In this study, we explored the PK/PD profile of pralurbactam/meropenem combinations in the neutropenic murine thigh infection models caused by different β-lactamases-producing Enterobacterales strains. The PK/PD index and target value for predicting the efficacy of pralurbactam/meropenem obtained from this study will be used to support the dose selection and breakpoint setting in clinical trials (Huang et al., 2024b).
2 Materials and methods
2.1 Compounds
Pralurbactam (batch nos.: B0220E01 and B0220E01K) was provided by Qilu Pharmaceutical Co. Ltd. Meropenem (batch no.: 2416C) was obtained from Japan Sumitomo Pharmaceutical Co. Cyclophosphamide (CTX, batch no.: 19111925) was provided by Jiangsu Hengrui Medicine Co., Ltd.
2.2 Strains
The study used a total of six strains of KPC-, NDM-, or OXA-producing Enterobacterales (three strains of K. pneumoniae and four strains of E. coli), which were provided by Microbiology Division, Institute of Antibiotics, Huashan Hospital, Fudan University. Quality control strains are selected for each bacteria. The MICs of pralurbactam, meropenem, and pralurbactam/meropenem combination against the strains are shown in Supplementary Table 1.
2.3 Neutropenic murine thigh infection models
Mice (SPF grade, female, 25–30 g, 6–7 weeks) were provided by Beijing Huafukang Biotechnology Co. The animal study was reviewed and approved by the Animal Ethics Committee of State Key Laboratory of Biotherapy, Sichuan University (approval letter No.: 20190923035). All experimental animals from the study were intraperitoneally injected with cyclophosphamide (solution using 0.9% sodium chloride injection to achieve a concentration of 15 mg/ml) at a dose of 150 mg/kg at 4 days and 1 day prior to the day of infection to form immunocompromised mouse models. The hair on both thighs of the mice was shaved off 2 h before inoculation. The injection site was disinfected with 75% alcohol before intramuscular injection and dried with skimmed cotton. The mouse was challenged by inoculum of 1 × 108 CFU/ml of the test strain via injection of 50 μl of bacterial solution into both thighs. The time was referred to as t = 0 h, 2 h after the mice were infected. The control mice were euthanized at this time to record the initial bacterial colonies by taking the thigh muscle. The specific dosage of pralurbactam or meropenem for each dosing regimen was intraperitoneally injected (t = 0 h). At 26 h after the mice were infected (t = 24 h), the mice (experimental group) in each dosage cohort were euthanized to record the bacterial load to calculate CFU change (Δlog10 CFU).
2.4 Quantification
The ultra-high performance liquid chromatography-tandem mass spectrometry (UPLC-MS/MS) system consisted of an LC-30A UPLC (Shimadzu Corporation, Japan) equipped with an Atlantis® T3 (3 μm, 2.1 × 100 mm) column, liquidity, and the cleaning solution mixed with formic acid, ammonium acetate, and acetonitrile, an API5500 mass spectrometer (AB SCIEX, USA). The retention times of chromatographic peaks and mass-to-charge ratios were obtained by MRM scanning pralurbactam (m/z 383.2 → 303.0), meropenem (m/z 384.1 → 141.2), internal standards pralurbactam-D4 (m/z 387.3 → 307.1), and meropenem-D6 (m/z 390.1 → 147.2) for peak identification. The drug concentration in the samples was calculated using Watson LIMS (version 7.5).
The UPLC-MS/MS method used to measure pralurbactam and meropenem concentrations in mice plasma was validated accurately in terms of selectivity, interaction, matrix effect, calibration curve, linearity, precision, accuracy, recovery, and stability. Briefly, the lower limit of quantification (LLOQ) was 0.125 mg/L for pralurbactam and meropenem. At this LLOQ level, the intra- and inter-assay precision was 3.5%–9.0% and 6.3% for pralurbactam, 5.0%–10.7% and 14.3% for meropenem. The overall recovery of pralurbactam and meropenem was 81.2% and 58.6%, respectively, and the corresponding coefficient of variation (CV) was 8.0% and 5.4%. This assay method was applied to quantify pralurbactam and meropenem for PK study.
2.5 PK studies
The administration method for pralurbactam or meropenem (dissolved in sterile water) is single-dose intraperitoneal injection. Mice were injected intraperitoneally with pralurbactam alone (5, 50, and 500 mg/kg), meropenem alone (50 and 100 mg/kg) and pralurbactam/meropenem combination (50/100 and 250/100 mg/kg). Five mice were randomly selected for anesthesia and orbital blood sampling at each time-point (0.083, 0.25, 0.5, 1, 2, 3, 4, and 6 h) after dosing. A blood sample (600–800 μl) was obtained from the mouse immediately. Each mouse selected for blood sampling at each time point was euthanized immediately. The concentrations of pralurbactam and meropenem in plasma samples were determined using the validated UPLC-MS/MS method. PK parameters (including non-compartmental parameter linear fitting and compartmental simulation) of pralurbactam and meropenem in plasma were calculated using Phoenix WinNonlin (version 8.1, Certara Corporation). The compartmental PK parameters of pralurbactam were used to calculate the fCmax, fAUC0–24, and %fT > CT. The free fraction of pralurbactam was 100% based on in vitro mouse plasma.
2.6 Pralurbactam combined with meropenem in PD studies
Different dosing regimens will be evaluated in established mouse thigh infection models to assess their effectiveness in killing bacteria, using the change in bacterial count in the mice thigh over 0–24 h post-administration. Five mice will be used for each dosing regimen in in vivo model to reduce intra-group variability.
The dose-fractionation experiment was performed in the infection models caused 1 strains of K. pneumoniae (17-R1-16), where the dose of meropenem was fixed in 100 mg/kg and the dosing interval was q2h. The dosing interval of pralurbactam changed at q2h, q4h, and q8h, where the daily dose of pralurbactam increased at 0–2,400 mg/kg as specified in the treatment protocol. The dose-escalation experiment was investigated in the infection models established by two strains of K. pneumoniae and four strains of E. coli, where the dose of meropenem was fixed in 100 mg/kg for K. pneumoniae and 50 mg/kg for E. coli and the dosing interval was q2h. The dosing interval of pralurbactam was fixed in q2h, where the dose of pralurbactam increased at 0, 5, 10, 25, and 100 mg/kg as specified in the treatment protocol (different in K. pneumoniae and E. coli). The dosing regimens are shown in Supplementary Table 2.
2.7 PK/PD analyses
The correlation between the PK/PD index (%fT > CT, fAUC, fCmax) and the bactericidal effect (Δlog10CFU) of pralurbactam in the presence of fixed dose of meropenem and different dosing regimens was fitted separately by employing a non-linear sigmoidal maximum-effect (Emax) model in Equation 1 (Phoenix WinNonlin, version 8.1, Certara). The value of PK/PD index corresponding to stasis (Δlog10CFU = 0) and 1-log10 kill (Δlog10CFU = 1) effects was derived for pralurbactam.
E was the pharmacodynamic index (ΔLog10CFU). E0 was the baseline value. Emax was the maximum bactericidal effect in vivo. X was the PK/PD index value in vivo. EC50 was the PK/PD index value when the bactericidal effect was 50%. γ was the slope of the curve.
3 Results
3.1 PK profiles of pralurbactam
The plasma concentration-time curves after intraperitoneal injection of single dose of pralurbactam alone, and pralurbactam/meropenem are shown in Figure 1. The regression analysis of PK parameters based on non-compartmental models confirmed that the Cmax or AUCinf of pralurbactam were dose proportional according to concentration data. The linear fitting equation of pralurbactam Cmax and AUCinf to dose was y = 1.049x = 0.118 (weighed R2 = 0.992), and y = 1.077x = 0.876 (weighed R2 = 0.999), respectively. According to different pralurbactam or meropenem concentrations in mice plasma obtained using different dosing regimens, the pharmacokinetic process of pralurbactam and meropenem in mice could be described by one-compartment model (PK parameters in Table 1).
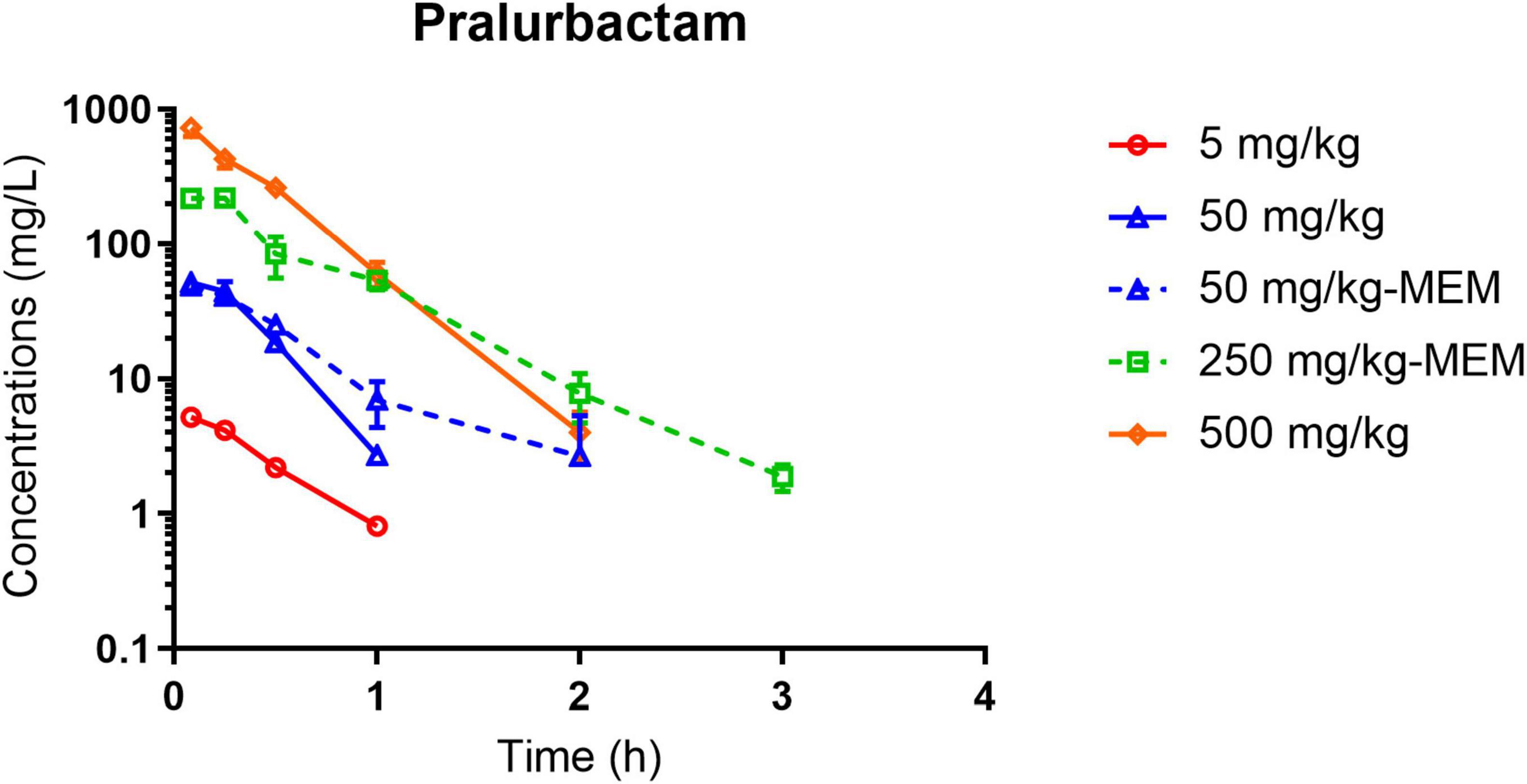
Figure 1. Pralurbactam plasma concentration-time curves after intraperitoneal injection of single ascending doses of pralurbactam or combined with meropenem in mouse thigh infection models. Solid lines indicate pralurbactam alone and dashed lines denote pralurbactam combined with meropenem. The dose of meropenem (combined with pralurbactam) was fixed at 100 mg/kg. MEM, meropenem.
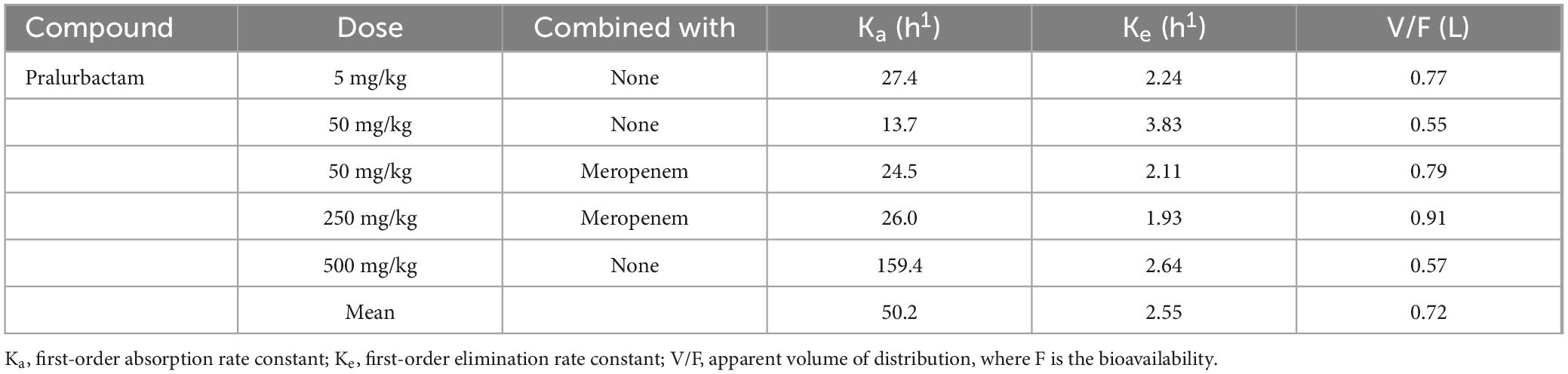
Table 1. Pharmacokinetic parameters of pralurbactam in mice plasma estimated by the one-compartment model.
3.2 PD results of pralurbactam/meropenem in murine thigh infection models
The neutropenic murine thigh infection models were established using the strains of K. pneumoniae and E. coli that produce different types of carbapenemase (KPC, OXA, or NDM). The bacterial killing curves for different dosing regimens were plotted based on the bacterial counts obtained at various time points (Figure 2). The change in bacteriality from pre- and 24 h after dosing was used as the pharmacodynamic evaluation indicator. PK/PD relationship of pralurbactam/meropenem combination was investigated in the models by dose fractionation (Figure 2A) and increasing the dose of pralurbactam in the combinations (Figures 2B, C). The bactericidal effect of pralurbactam/meropenem combination was related to the daily dose and dosing frequency of pralurbactam when fixed dosing of meropenem. Shorter dosing interval or higher dose of pralurbactam could boost the bacteriostatic effect of each treatment. The combination of at least 25 mg/kg pralurbactam (fixed 100 mg/kg q2h of meropenem) could inhibit the growth of all the test strains of K. pneumoniae (17-R1-16, 20-W2-70, and ATCC BAA-1705) more effectively. It is surprising that dosing 50 mg/kg meropenem alone (without pralurbactam) exhibits significant growth inhibition on two strains of E. coli (18-W40-096 and ATCC BAA-2452). The two strains of E. coli were excluded PK/PD analyses.
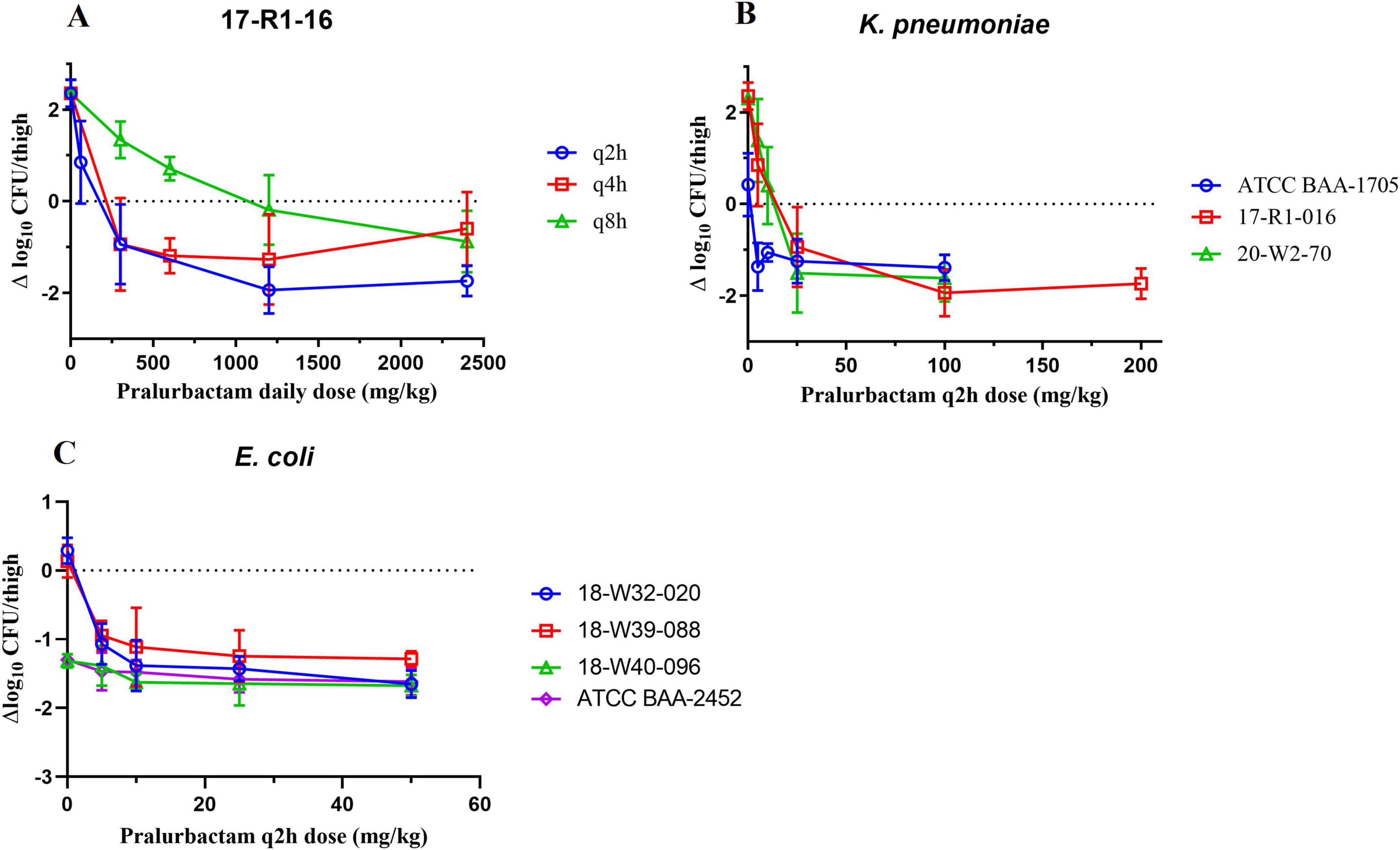
Figure 2. Bactericidal effect of pralurbactam combined with meropenem in terms of pralurbactam daily dose and dose fractionation (A), and caused by Klebsiella pneumoniae (B) or Escherichia coli (C) after increasing doses of pralurbactam. The dose of meropenem was fixed in 100 mg/kg for K. pneumoniae and 50 mg/kg for E. coli. The dosing interval was q2h.
3.3 PK/PD analyses
Sigmoid curves were fitted by regression analysis with PK/PD index expressed as (i) the fraction of the dosing interval above a free threshold concentration (%fT > CT), (ii) the area under the free drug concentration-time curve (fAUC), and (iii) the maximum free drug concentration (fCmax). The fitting results of the correlation between the three PK/PD indexes for pooled K. pneumoniae and E. coli are shown in Figure 3. The PK/PD relationship of pralurbactam/meropenem was best described by %fT > CT (with CT = 1 mg/L) followed by fAUC and fCmax. The R2 is significantly higher than other values (Table 2, 0.733 vs. others). The separate Emax model fitting for K. pneumoniae and E. coli is shown in Supplementary Figure 1, respectively. The Emax model fitting for the five strains is shown in Supplementary Figure 2. The estimated values of Emax model parameters are shown in Table 2. When the PK/PD index %fT > 1 mg/L reached 38.4% and 63.6%, pralurbactam/meropenem combination would achieve bacteriostatic effect and 1-log10 reduction against K. pneumoniae (KPC and OXA) in thigh bioburden, respectively (Table 3). %fT > 1 mg/L for pralurbactam/meropenem combination that achieved a static effect, and 1-log10 kill in colony count against E. coli (NDM) were 1.8 and 40.1, respectively (Table 3).
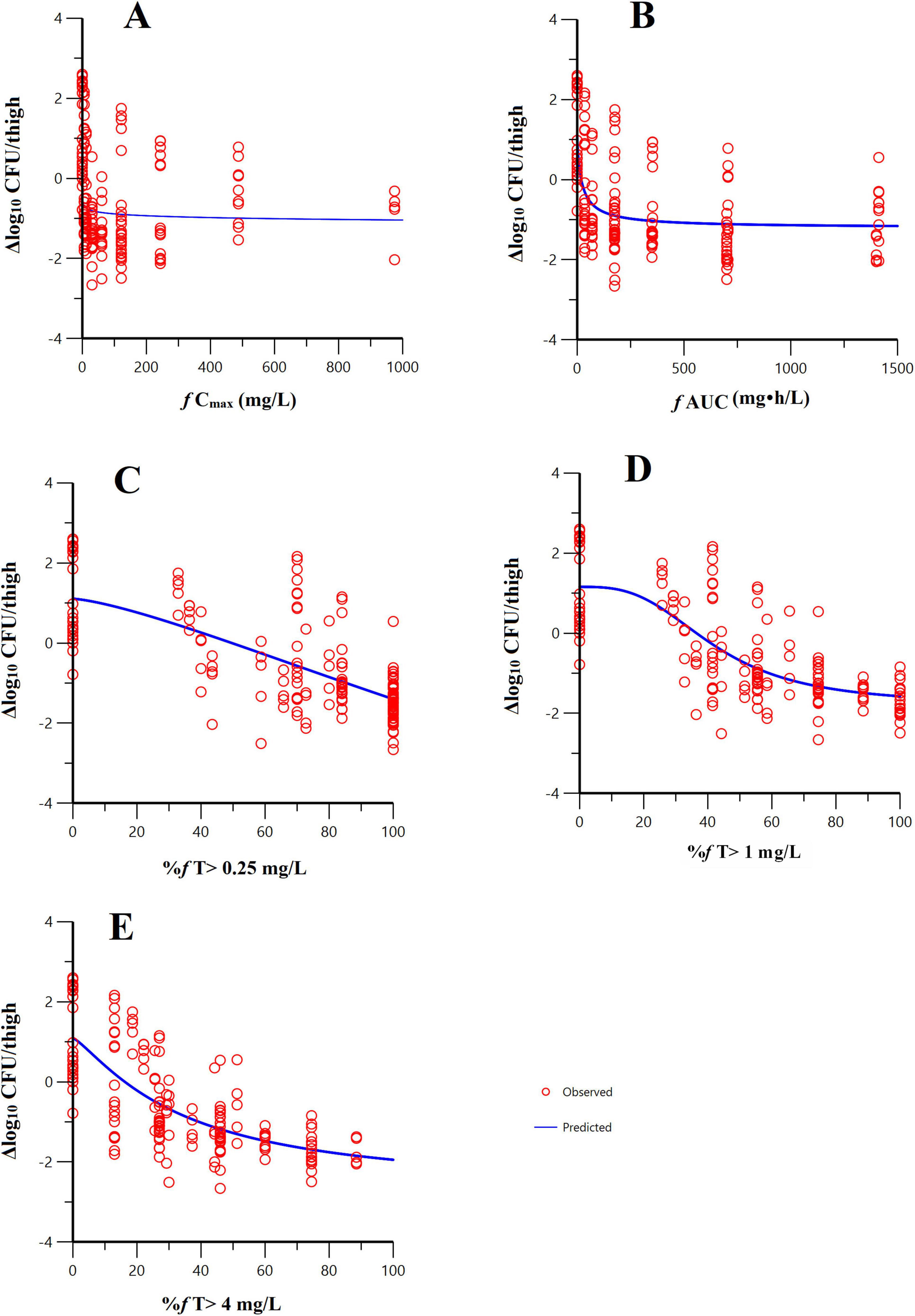
Figure 3. Emax model fitting of the pharmacokinetic/pharmacodynamic (PK/PD) index-bactericidal effect of pralurbactam (combined with meropenem). PK/PD index: (A) f AUC; (B) f Cmax; (C) %fT > 0.25 mg/L; (D) %fT > 1 mg/L; (E) %fT > 4 mg/L. The hollow circles represent the observed values and the solid lines represent the predicted values. It was evident that the PK/PD relationship of pralurbactam (combined with meropenem) was best described by %fT > 1 mg/L.
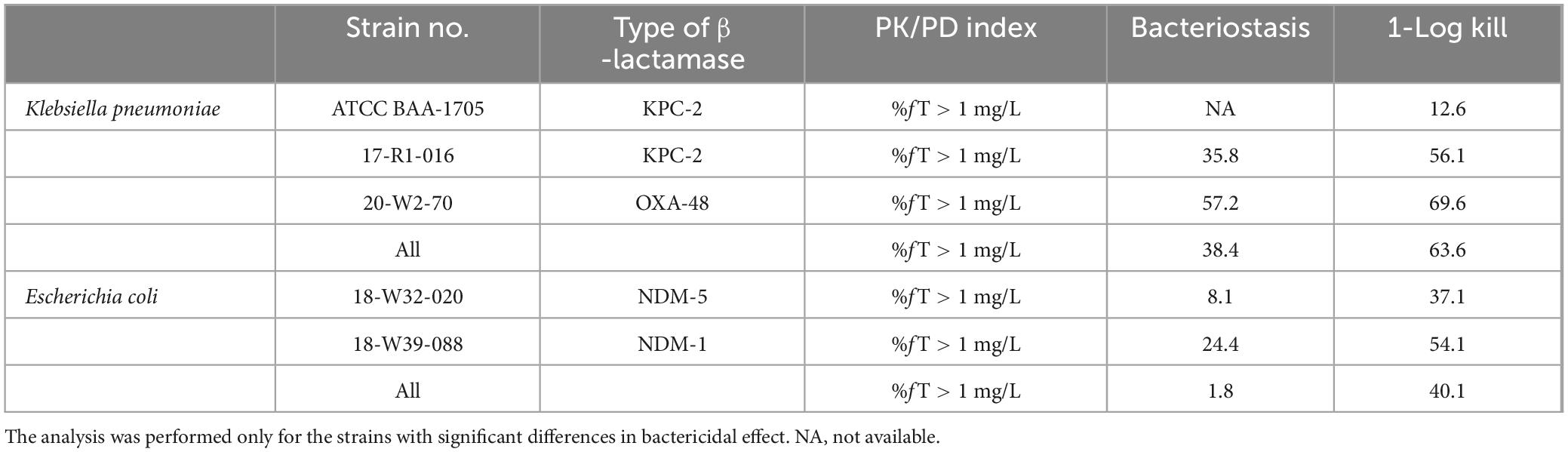
Table 3. Targets of the pharmacokinetic/pharmacodynamic indexes for pralurbactam (combined with meropenem).
4 Discussion
In this study, the PK parameters of pralurbactam were calculated for each dosage cohort using Phoenix WinNonlin software based on the mean plasma concentrations at each sampling point in mice. The mean values of the PK parameters were used to simulate the potential PK/PD indices. Another method, the PK parameters could be estimated by the population PK (PPK) model using NONMEM software based on the pooled plasma concentrations. The estimated typical values of Ke and V/F based on the population PK model of pralurbactam were generally consistent with the classical mean values of Ke (PPK: 2.34 h1 vs. classical PK: 2.55 h1) and V/F (PPK: 0.719 L vs. classical PK: 0.72 L). Ka estimate was close to the median value (PPK: 29.1 h1 vs. classical PK: 26.0 h1). Ka could not be obtained accurately because the peak concentration was reached at the first sampling time point (5 min after intraperitoneal injection of pralurbactam). The classical Ka varied greatly between dosage cohorts (range from 13.7 to 159.4 h1). However, the effect of Ka variation on PK/PD index could be negligible, and the inter-mice variation did not have significant effect on the result of PK parameters.
Most drugs are eliminated more rapidly in mice than in human body. Multiple doses are required in mice to simulate the absorption, distribution, metabolism, and excretion process in human body. A dose of 50 mg/kg q2h pralurbactam intraperitoneally injected in mice approximates 500 mg q8h pralurbactam intravenous 2 h-infusion in humans (%fT > 1 mg/L: 88% vs. 86%) (Huang et al., 2024b). A dose of 100 mg/kg of meropenem q2h in mice produces a free drug time above 8 mg/L similar to that with 2 g administered q8h by a 2-h infusion in humans, and 50 mg/kg q2h in mice similar to 1 g q8h by a 2-h infusion in humans (Sabet et al., 2018). Monogue et al. (2018) reported that meropenem dosed at 50 mg/kg at 0 h, 8 mg/kg at 2.5 h, and 5 mg/kg at 4.5 h in mice humanized pharmacokinetic profiles compared to human meropenem doses (1 g q8h by a 0.5-h infusion).
In vitro studies of pralurbactam proposed %fT > CT (CT = 1 mg/L) as the best PK/PD index to predict the in vivo bactericidal effect of pralurbactam/meropenem combination (Huang et al., 2024a). The in vitro target value was lower because of possible host and bacterial factors (PK/PD 1-log kill target in vitro: 48 vs. in vivo: 63.6). Much slower growth rate in vivo and the influence of the host might lead to this discrepancy (Zhang et al., 2022). Differences in PK profiles between animals and humans require further simulations. Lung infection model is more suitable to investigate the in vivo bactericidal activity of pralurbactam/meropenem combination against β-lactamases-producing K. pneumoniae because of different drug exposure and the permeability between lung and thigh tissues. It was reported that the PK/PD target obtained in thigh infection model was higher than lung infection model (Berkhout et al., 2016). The thigh model appeared more conservative and stringent values. Further experiments are required to confirm the antimicrobial activity of different pralurbactam/meropenem combinations in mouse lung infection models.
The time above threshold %fT > (CT = 1 mg/L) is typically considered the best PK/PD index to predict the bactericidal activity of avibactam, another BLI with a diazabicyclo structure, in the latest studies (Berkhout et al., 2016). However, the conclusion is inconsistent in different animal models and different BLs because AUC may also play a role (MacGowan et al., 2017; Mavridou et al., 2015). The proposal of “time above instantaneous MIC” may explain the differences (Nichols et al., 2022; Bhagunde et al., 2012), but it is impossible to monitor the instantaneous drug concentration in blood in clinical practice. The best PK/PD index of pralurbactam is different from that of other BLIs (Supplementary Table 3; Berkhout et al., 2016; Nichols et al., 2018; Wu et al., 2018; Mavridou et al., 2015; Griffith et al., 2019). The PK/PD target of the same BLI may be different in various infection models such as hollow fiber infection model, thigh infection model, or lung infection model. No clinical PK/PD target values have been reported for pralurbactam. PK/PD study in subsequent clinical trials is also required to refine the exposure-response of pralurbactam/meropenem combinations in humans.
During selection of the lead compounds, pralurbactam showed superior antimicrobial activity than avibactam, which is consistent with previously reported underlying mechanism of resistance to avibactam, including β-lactamase variants, changes in bacterial membrane permeability, and overexpression of efflux pumps (Xiong et al., 2022; Bush and Bradford, 2019), especially the blaKPC gene mutation. PD study demonstrated stronger inhibitory effect of pralurbactam combined with meropenem on KPC-producing bacteria, which corroborates with previous findings (unpublished data from pre-clinical trials). Antimicrobial susceptibility testing results showed that meropenem combined with pralurbactam could not inhibit NDM-producing K. pneumoniae, but could inhibit NDM-producing E. coli to some extent, which may be attributed to the partial activity of pralurbactam alone against E. coli (Huang et al., 2024a). The time-killing studies of different dosing regimens and dose-finding studies in dose-fractionation and dose-escalation experiments are warranted to clarify the most proper dosing regimens in clinical trials.
5 Conclusion
In conclusion, %fT > 1 mg/L was the PK/PD index that best described the bacterial killing effect of pralurbactam/meropenem over 24 h in mouse thigh infection model. When %fT > 1 mg/L reached 38.4% and 63.6%, pralurbactam/meropenem combination would achieve bacteriostatic effect and 1-log10 reduction against K. pneumoniae in thigh bioburden, respectively. These PK/PD data derived from mouse thigh infection models will be used to inform the optimal dosing regimen of pralurbactam/meropenem combination in clinical trials.
Data availability statement
The raw data supporting the conclusions of this article will be made available by the authors, without undue reservation.
Ethics statement
The animal study was approved by the Animal Ethics Committee of State Key Laboratory of Biotherapy, Sichuan University. The study was conducted in accordance with the local legislation and institutional requirements.
Author contributions
ZH: Formal analysis, Investigation, Software, Visualization, Writing – original draft. WL: Data curation, Investigation, Resources, Writing – original draft. RZ: Formal analysis, Investigation, Visualization, Writing – original draft. YL: Validation, Writing – original draft. XL: Methodology, Writing – original draft. XB: Methodology, Writing – original draft. SZ: Writing – review & editing. XMW: Writing – review & editing. NZ: Writing – review & editing. CG: Writing – review & editing. BG: Conceptualization, Project administration, Writing – review & editing. ZW: Project administration, Supervision, Writing – review & editing. JZ: Conceptualization, Funding acquisition, Supervision, Writing – review & editing. XJW: Conceptualization, Funding acquisition, Supervision, Writing – review & editing.
Funding
The author(s) declare financial support was received for the research, authorship, and/or publication of this article. This study was supported by the grants from the Major Research and Development Project of Innovative Drugs, Ministry of Science and Technology of China (2017ZX09304005), Shanghai Science and Technology Innovation Action Plan (22S11904702), and Qilu Pharmaceutical Co., Ltd.
Acknowledgments
We thank Xingjun Cheng, Xiuhua Huang, and other staff of State Key Laboratory of Biotherapy at Sichuan University for their assistance in the animal experiments.
Conflict of interest
SZ, XMW, NZ, and CG are employees of Qilu Pharmaceutical Co., Ltd.
The remaining authors declare that the research was conducted in the absence of any commercial or financial relationships that could be construed as a potential conflict of interest.
Generative AI statement
The authors declare that no Generative AI was used in the creation of this manuscript.
Publisher’s note
All claims expressed in this article are solely those of the authors and do not necessarily represent those of their affiliated organizations, or those of the publisher, the editors and the reviewers. Any product that may be evaluated in this article, or claim that may be made by its manufacturer, is not guaranteed or endorsed by the publisher.
Supplementary material
The Supplementary Material for this article can be found online at: https://www.frontiersin.org/articles/10.3389/fmicb.2024.1516979/full#supplementary-material
References
Berkhout, J., Melchers, M. J., van Mil, A. C., Seyedmousavi, S., Lagarde, C. M., Schuck, V. J., et al. (2016). Pharmacodynamics of ceftazidime and avibactam in neutropenic mice with thigh or lung infection. Antimicrob. Agents Chemother. 60, 368–375. doi: 10.1128/AAC.01269-15
Bhagunde, P., Chang, K. T., Hirsch, E. B., Ledesma, K. R., Nikolaou, M., and Tam, V. H. (2012). Novel modeling framework to guide design of optimal dosing strategies for beta-lactamase inhibitors. Antimicrob. Agents Chemother. 56, 2237–2240. doi: 10.1128/AAC.06113-11
Bush, K., and Bradford, P. A. (2019). Interplay between beta-lactamases and new beta-lactamase inhibitors. Nat. Rev. Microbiol. 17, 295–306. doi: 10.1038/s41579-019-0159-8
Drawz, S. M., Papp-Wallace, K. M., and Bonomo, R. A. (2014). New beta-lactamase inhibitors: a therapeutic renaissance in an MDR world. Antimicrob. Agents Chemother. 58, 1835–1846. doi: 10.1128/AAC.00826-13
Griffith, D. C., Sabet, M., Tarazi, Z., Lomovskaya, O., and Dudley, M. N. (2019). Pharmacokinetics/pharmacodynamics of vaborbactam, a novel beta-lactamase inhibitor, in combination with meropenem. Antimicrob. Agents Chemother. 63, e01659–18. doi: 10.1128/AAC.01659-18
Guo, Y., Han, R., Jiang, B., Ding, L., Yang, F., Zheng, B., et al. (2022). In vitro activity of new beta-lactam-beta-lactamase inhibitor combinations and comparators against clinical isolates of gram-negative bacilli: results from the China antimicrobial surveillance network (CHINET) in 2019. Microbiol. Spectr. 10:e185422. doi: 10.1128/spectrum.01854-22
Huang, Z., Bian, X., Li, Y., Hu, J., Guo, B., Yang, X., et al. (2024a). In vitro pharmacokinetics/pharmacodynamics of FL058 (a novel beta-lactamase inhibitor) combined with meropenem against carbapenemase-producing enterobacterales. Front. Pharmacol. 15:1282480. doi: 10.3389/fphar.2024.1282480
Huang, Z., Yang, X., Jin, Y., Yu, J., Cao, G., Wang, J., et al. (2024b). First-in-human study to evaluate the safety, tolerability, and population pharmacokinetic/pharmacodynamic target attainment analysis of FL058 alone and in combination with meropenem in healthy subjects. Antimicrob. Agents Chemother. 68:e133023. doi: 10.1128/aac.01330-23
MacGowan, A., Tomaselli, S., Noel, A., and Bowker, K. (2017). The pharmacodynamics of avibactam in combination with ceftaroline or ceftazidime against beta-lactamase-producing enterobacteriaceae studied in an in vitro model of infection. J. Antimicrob. Chemother. 72, 762–769. doi: 10.1093/jac/dkw480
Mavridou, E., Melchers, R. J., van Mil, A. C., Mangin, E., Motyl, M. R., and Mouton, J. W. (2015). Pharmacodynamics of imipenem in combination with beta-lactamase inhibitor MK7655 in a murine thigh model. Antimicrob. Agents Chemother. 59, 790–795. doi: 10.1128/AAC.03706-14
Monogue, M. L., Giovagnoli, S., Bissantz, C., Zampaloni, C., and Nicolau, D. P. (2018). In vivo efficacy of meropenem with a novel non-beta-lactam-beta-lactamase inhibitor, nacubactam, against gram-negative organisms exhibiting various resistance mechanisms in a murine complicated urinary tract infection model. Antimicrob. Agents Chemother. 62, e02596–17. doi: 10.1128/AAC.02596-17
Nichols, W. W., Bradford, P. A., and Stone, G. G. (2022). The primary pharmacology of ceftazidime/avibactam: in vivo translational biology and pharmacokinetics/pharmacodynamics (PK/PD). J. Antimicrob. Chemother. 77, 2341–2352. doi: 10.1093/jac/dkac172
Nichols, W. W., Newell, P., Critchley, I. A., Riccobene, T., and Das, S. (2018). Avibactam pharmacokinetic/pharmacodynamic targets. Antimicrob. Agents Chemother. 62, e02446–17. doi: 10.1128/AAC.02446-17
Sabet, M., Tarazi, Z., Nolan, T., Parkinson, J., Rubio-Aparicio, D., Lomovskaya, O., et al. (2018). Activity of meropenem-vaborbactam in mouse models of infection due to KPC-producing carbapenem-resistant enterobacteriaceae. Antimicrob. Agents Chemother. 62, e01446–17. doi: 10.1128/AAC.01446-17
Sharma, R., Park, T. E., and Moy, S. (2016). Ceftazidime-avibactam: a novel cephalosporin/beta-lactamase inhibitor combination for the treatment of resistant gram-negative organisms. Clin. Ther. 38, 431–444. doi: 10.1016/j.clinthera.2016.01.018
Wang, Q., Wang, X., Wang, J., Ouyang, P., Jin, C., Wang, R., et al. (2018). Phenotypic and genotypic characterization of carbapenem-resistant enterobacteriaceae: data from a longitudinal large-scale CRE study in China (2012-2016). Clin. Infect. Dis. 67(suppl_2), S196–S205. doi: 10.1093/cid/ciy660
Wu, J., Racine, F., Wismer, M. K., Young, K., Carr, D. M., Xiao, J. C., et al. (2018). Exploring the pharmacokinetic/pharmacodynamic relationship of relebactam (MK-7655) in combination with imipenem in a hollow-fiber infection model. Antimicrob. Agents Chemother. 62, e02323–17. doi: 10.1128/AAC.02323-17
Xiong, L., Wang, X., Wang, Y., Yu, W., Zhou, Y., Chi, X., et al. (2022). Molecular mechanisms underlying bacterial resistance to ceftazidime/avibactam. Wires Mech. Dis. 14:e1571. doi: 10.1002/wsbm.1571
Keywords: pralurbactam, β-lactamase inhibitor, Klebsiella pneumoniae, Escherichia coli, pharmacokinetic/pharmacodynamic analysis, neutropenic thigh infection model
Citation: Huang Z, Li W, Zhang R, Li Y, Li X, Bian X, Zheng S, Wang X, Zhang N, Gao C, Guo B, Wang Z, Zhang J and Wu X (2024) Pharmacokinetic/pharma-codynamic study of pralurbactam (FL058) combined with meropenem in a neutropenic murine thigh infection model. Front. Microbiol. 15:1516979. doi: 10.3389/fmicb.2024.1516979
Received: 25 October 2024; Accepted: 06 December 2024;
Published: 17 December 2024.
Edited by:
Piotr Majewski, Medical University of Bialystok, PolandReviewed by:
Lautaro De Vedia, University of Buenos Aires, ArgentinaWenchao Mao, Zhejiang Hospital, China
Copyright © 2024 Huang, Li, Zhang, Li, Li, Bian, Zheng, Wang, Zhang, Gao, Guo, Wang, Zhang and Wu. This is an open-access article distributed under the terms of the Creative Commons Attribution License (CC BY). The use, distribution or reproduction in other forums is permitted, provided the original author(s) and the copyright owner(s) are credited and that the original publication in this journal is cited, in accordance with accepted academic practice. No use, distribution or reproduction is permitted which does not comply with these terms.
*Correspondence: Xiaojie Wu, d3V4aWFvamllQGZ1ZGFuLmVkdS5jbg==; Jing Zhang, emhhbmdqX2Z1ZGFuQGFsaXl1bi5jb20=
†These authors have contributed equally to this work and share first authorship