- 1Genetics Department, Faculty of Basic Science, Shahrekord University, Shahrekord, Iran
- 2Medical Plants Research Center, Basic Health Sciences Institute, Shahrekord University of Medical Sciences, Shahrekord, Iran
This study aimed to screen native methionine gamma-lyase (L-methioninase) producing bacteria from soil samples and optimize the culture media for enhanced enzyme production using statistical design. Three bacteria, Pseudomonas mosselii, Ralstonia solanacearum, and Cytobacillus kochii, were identified as novel L-methioninase producers, which alternative source of L-methioninase for cancer treatment could be utilized alongside other therapeutic agents. The bacteria were isolated from various garden soils and cultured on a modified M9 medium and screened by Nessler reagent. According to Bergey’s manual of systematic bacteriology, identification tests determined the morphological, physiological, and biochemical characterizations. Further identification was performed using the analysis of the 16 s rDNA gene sequences using PCR and universal bacterial primers. The optimization of medium constituents for L-methioninase production was performed in two steps using Response Surface Methodology (RSM). The first step used the “one factor at a time” method to screen and identify critical medium components for L-methioninase production. The second step used the Box–Behnken design to assess quadratic effects and two-way interactions between variables and determine the response’s nonlinear nature. The study found that three isolates produced L-methioninase, namely Pseudomonas mosselii spp.MN02 (GenBank PP431975), Ralstonia solanacearum spp.MN02 (GenBank PP431636), and Cytobacillus kochii spp.MN02 (GenBank PP432622). Among these, Pseudomonas mosselii spp.MN02 produced the highest amount of L-methioninase and was therefore chosen for enzyme production optimization process. The maximum L-methioninase production of 1.5 ± 0.1 U/mL was obtained at a pH 6, and the best nitrogen source was yeast extract (1% concentration). The influence of different carbon sources revealed that glucose was the best carbon source for L-methioninase production (3.25 ± 0.1 U/mL). The optimization experiments using the Box–Behnken design predicted that L-methioninase would have an activity of 12.56 U/mL under optimal conditions, including 2% glucose, 2% yeast extract, pH 6, and temperature at 30°C. In conclusion, this study presents a promising new methods for identifying potential L-methioninase producers and optimizing the culture medium for more enzyme production by microbial fermentation. This could pave the way for developing a drug that assists in human cancers treatment.
1 Introduction
Methionine gamma-lyase or L-methioninase is an enzyme dependent on pyridoxal 5′-phosphate as cofactor. L-methioninase is an enzyme that depletes methionine from the blood, so cancer cells move toward cell death due to a lack of methionine. It is a Hoffman effect (Abozeid, 2023). This enzyme’s mechanism of action is that methionine’s anti-tumor mechanism is due to the loss of the function of molecules synthesized from methionine. According to research and newly published articles, methioninase is effective on all types of cancer (Siblini et al., 2022). In addition, methionine gama-lyase (MGL) has antibiotic properties, which can also be used to inhibit the growth of many warm bacteria and use positive and negative pathogenics. Due to the mechanism of action of methioninase in the antibiotic properties related to thiocyanates, this enzyme does not create antibiotic resistance (Raboni et al., 2024).
L-methioninase is found in nearly all organisms except mammals. Most bacteria, protozoans, archaea, and plants produce intracellular L-methioninase, while fungal sources produce extracellular L-methioninase (Irajie et al., 2021).
L-asparaginase and L-arginine are drugs widely used to treat lymphosarcoma, acute lymphoblastic leukemia, Hodgkin’s disease, and other types of tumors. However, one of the issues with its administration is that it can cause anaphylactic reactions (Kaiser, 2020). L-methioninase, on the other hand, does not have this problem. To produce L-methioninase, researchers have tried using fungi and bacteria as sources. While using fungi to produce L-methioninase is an attractive option, the risk of anaphylactic reactions caused by fungal sources is a concern. Selecting bacteria as producers of L-methioninase is an exciting prospect (Sahoo and Sahoo, 2021).
The design and optimization of biological processes are vital for improving proficiency and economic bioprocessing. Response Surface Methodology (RSM) is an exploited modeling procedure to establish the association between the responses and the independent variables. It can analyze the impacts of each variable itself or in combination via regression analysis. So far, Bax-Behken and RSM have been widely used to optimize the construction of many enzymes (Banihashemi et al., 2016).
This study mainly focuses on screening for L-methionine-producing bacteria from soil and then optimizing the culture media for enhanced enzyme production using statistical design. This is the first report of the three bacteria included Pseudomonas mosselii, Ralstonia solanacearum, and Cytobacillus kochii as a novel L-methioninase producer, which could be an optimistic alternative resource for cancer treatment.
2 Materials and methods
2.1 Sample collection
The soils were collected from various gardens in Iran, placed in sterile bags, and transported to the laboratory, where they were refrigerated at 4°C for further experimentation.
2.2 Screening for L-methionine producers
To begin with, various soil samples were cultured on a Nutrient Agar medium to isolate bacteria. Subsequently, a pure bacterial culture was suspended in 20 mL of modified M9 medium broth containing 0.1% methionine. This solution was then incubated at 30°C for 72 h at 120 rpm.
2.3 Enzyme assay
L-methioninas activity evaluated by Nessler’s method (Wriston and Yellin, 1973). 72-h bacterial cultures were centrifuged at 20124 RCF for 10 min, and a clear supernatant was kept for enzyme assay. 100 μL of supernatant transported to the sterile 1.5 mL microtube with 900 μL 0.5%W/V L-methionine (substrate) in Tris–HCl buffer (50 mM and pH 7.2). After incubation at 30°C for 60 min, 100 μL 15% trichloroacetic acid was added for stop the reaction and centrifuged at 8944 RCF for 10 min. The ammonia liberation in the supernatant was determined using the colorimetric technique by adding 200 Nessler regent in 200 μL supernatant and distilled water. The sample was first vortexed for 15 s and incubated at room temperature for 10 min, and finally, the absorption (OD) was measured at 425 nm. The ammonia released in the reaction was calculated based on the standard curve obtained using ammonium chloride. One unit of L-methioninase activity is defined as the amount of enzyme that releases 1 μL of ammonia per minute at 37°C.
2.4 Molecular identification of bacteria
The morphological, physiological, and biochemical characterizations of the best L-methionine-producing isolates (according to the highest yield of enzyme production at the screening stage) were determined by identification tests according to Bergey’s manual of systematic bacteriology (Sneath et al., 1986). For further identification, the analysis of the 16 s rDNA gene sequence using universal bacterial primers, 5′-CAGCCGCGGTAATAC-3′ as the forward primer and 5′-ACGGGCGGTGTGTAC-3′ as the reverse primer. Trench sequencing was employed to analyze 16S rDNA sequences After that, the phylogenic method for identifying bacterial isolates was used using the UPGMA algorithm tree in the Clustal W server.
2.5 Optimization of essential components
The optimization of medium constituents for L-methioninase production by the selected bacterium was performed in two steps. MINITAB software conducted all experimental designs and data analysis (version 20, PA, United States).
2.5.1 Identification of essential nutrient components
Optimization of the conditions and compositions of the culture medium was carried out using the method of “one factor at a time,” in which many media components were tested to obtain the best results. In this research, the carbon and nitrogen sources (each at 0.2, 0.4, 0.6, and 0.8% w/v) as well as temperature (25°C, 30°C, 32°C, 37°C, and 40°C) and pH (Raboni et al., 2024; Irajie et al., 2021; Kaiser, 2020; Sahoo and Sahoo, 2021; Banihashemi et al., 2016; Wriston and Yellin, 1973; Box and Behnken, 1960; Selim et al., 2015) were assessed to screen and identify the critical medium components for L-methioninase production. It should be noted that all experiments were performed in the M9 medium, and the best results of the factors in each step were applied in the next step.
2.5.2 Optimization of screened components
The next stage in the L-methioninase production optimization was to determine the most favorable level of each critical, independent variable as determined by the “one factor at a time” methodology. An experimental design, such as the Box–Behnken design (Box and Behnken, 1960), a fraction of the full factorial was used. Unlike the “one factor at a time,” this design assessed the quadratic effects and two-way interactions between the variables and determined the response’s nonlinear nature. The four independent variables were designed using MINITAB software (version 20, PA, United States). Experiments were performed in triplicate, and mean values were given. Finally, the final optimum experimental parameters were calculated using the Minitab Response Surface Optimizer function, which determined the best combination of each parameter.
3 Results and discussion
3.1 Isolation and screening of L-methioninase-producing bacteria
The bacteria were cultured in a modified M9 medium, and the screening of bacteria produces L-methionine. We cultured 16 samples from different soil sources in broth-modified M9 medium for 72 h. The experiments were repeated three times, and the average values were used. The results show that we found three isolates that can produce L-methionine, and the B5s9 isolate had higher activity than the other isolate. B5s9 isolate could be considered an excellent L-methioninase producer.
3.2 Identification of the isolated strains
To identify the isolated strain, the results demonstrated that the strain B5s8 was a gram-negative motile bacillus in addition to indole production; however, this isolate could not ferment all the tested carbohydrates. In parallel, the widely used method of 16 S rRNA gene analysis was also applied, demonstrating that the almost complete sequence of 16 S rRNA gene had 95% homology with Pseudomonas mosselii strain MN02. Moreover, the phylogenetic tree was drawn (Figure 1). Therefore, according to the observations and analyses, the strain MN02 was identified as Pseudomonas mosselii. Another strain that we placed as producing the L-methioninase includes B2s8 and NB7s3.B2S8 was a gram-negative andNB7s3 was gram-positive, and we used 16 S rRNA gene was applied, however after a complete sequence of 16 S rRNA gene, respectively, had homology with Ralstonia solanacearum strain MN02 and Cytobacillus kochii strain MN02 and drowned phylogenetic tree (Figures 2, 3).
3.3 Screening important media components for L-methioninase production by Pseudomonas mosselii sp. MN02
L-methininase productions were assessed at various pH to discover the influence of pH. Maximum of L-methininase production of 1.5 ± 0.1 U/mL was obtained at a pH of 6. A decrease in the production of L-methioninase is observed in pH’s lower than 5 and higher than 8. Therefore, the optimum pH for Pseudomonas mosselii to produce L-methioninase has attained a pH of 6 (Figure 4A). In this regard, some scientists reported the best pH for L-methioninas production in other strains. Selim et al. reported that the best pH range for L-methioninase producing in Streptomyces sp. was 7–7.5 (Selim et al., 2015), and Mokham et al. reported that the best pH range for L-methioninase producing in Alcaligenes when increased pH from 4 to 6 (Mohkam et al., 2020). To study the influence of temperature for maximum production of L-methioninase was 32°C and L-methioninase production was 2.0 ± 0.1 U/mL. When we increased the temperature to more than 37°C, we could see an apparent decrease in enzyme production (Figure 4B).
Among various nitrogen sources, we tested two different types of nitrogen sources, including inorganic and organic. The review and result of this are in Figure 5A. We found that the best nitrogen source for Pseudomonas mosselii to produce L-methioninase was yeast extract at 1% concentration, and MN02 can produce 3.2 ± 0.1 U/mL L-methioninas. After the yeast extract, the tryptone was placed at a concentration of 0.5%. On the other hand, we can see lower production of the enzyme in (NH4)2SO4; it can be shown that producing of L-methioninase in Pseudomonas mosselii, was not dependent on L-methionine which means that these bacteria can produce the enzyme in the absent of L-methionine (Singh and Kharayat, 2018). Some other bacteria identified in the past, like these bacteria, were not dependent on L-methionine for enzyme production, like Alcaligenes (Mohkam et al., 2020). However, fungi can produce L-methininase when L-methionine is in their culture. This fungi like Trichoderma harzianum and Aspergillus spp. (Salim et al., 2019). Without needing L-methioninase, this feature helps us produce L-methioninas from Pseudomonas mosselii, a cheaper nitrogen source. The properties of P. mosselii, including its faster growth and production compared to fungi, simpler cultivation conditions that are typically easier and more cost-effective, easier genetic engineering capabilities, and fewer side effects, produce L-methioninase without L-methionine, make it a more suitable choice for producing the enzyme L-methioninase.
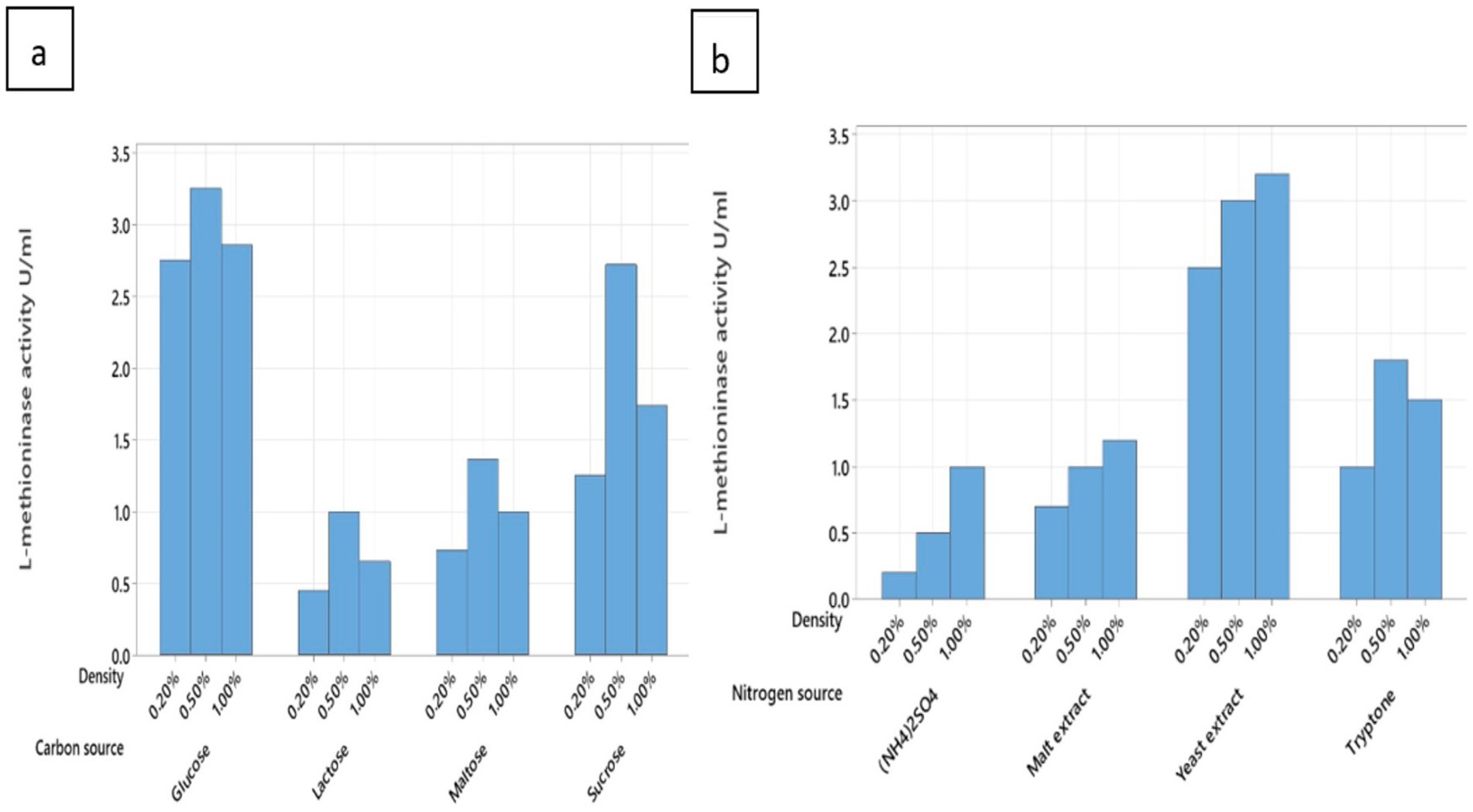
Figure 5. Effect of various factors carbon source (A) and nitrogen source (B) on L-methioninas production.
The influence of different carbon sources, glucose was the best carbon source for L-methioninase production (3.25 ± 0.1 U/mL), followed by 0.5% concentration (Figure 5B). Like other studies that have been done before, it shows that in lack of carbon source, L-methioninase production was inhibited. This is significant for bacteria like Alcaligenes and Acromobacter starkeyi. Despite our findings, some bacteria produce more L-methioninanase with lactose source, which is probably related to the β-galactosidase gene in those bacteria like Alcaligenes (Mohkam et al., 2020).
3.4 Optimization condition for production of L-methioninase using response surface methodology
We tested four measure conditions such as pH, temperature, glucose, and yeast extract, and we tried to optimize these conditions to the optimum. We got help from Box–Behnken design and applied many conditions tabulated in Table 1. The variance analysis (ANOVA) result is tabulated in Table 2. The variable is considered significant when the p value is lower than 0.05. ANOVA showed the significance of all four factors (pH, temperature, glucose, and yeast extract) in producing L-methioninase. However, the interactive effects had no significant impact on L-methioninase production. We analyzed the data in multiple regression and found the model for predicting L-methioninase.
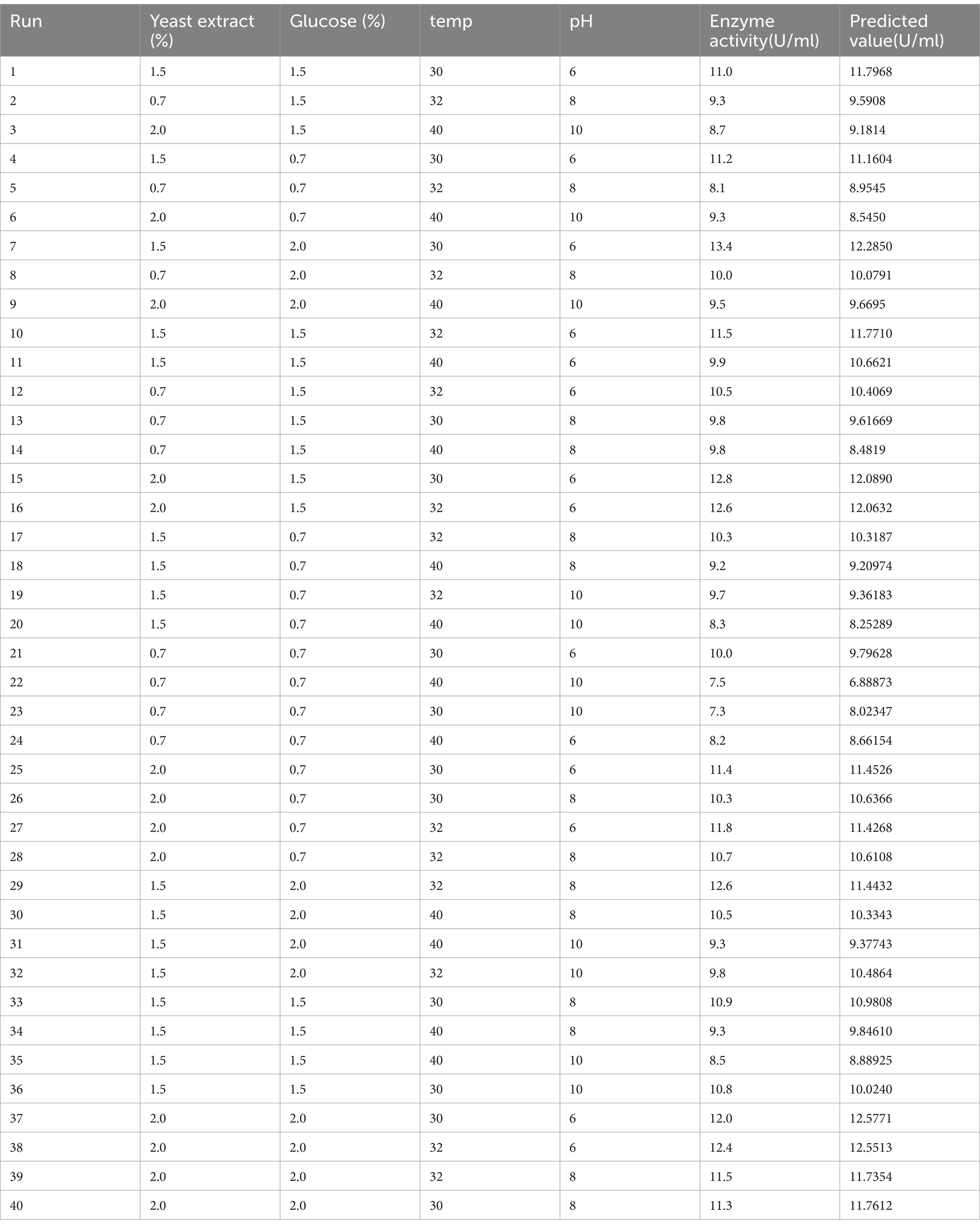
Table 1. The results of an experimental study and the predicted values of L-methioninase production using the Box–Behnken design.
X1, X2, X3, and X4 represent the coded values of pH, temperature, glucose (%), and yeast extract (%), respectively.
According to analysis, the significant p value (<0.001) and high F value showed that the model equation was in excellent agreement with the experimental. The coefficient of determination (R2) was 0.852 for L-methioninase production, which describes a higher correlation between experimental results and their predictions. The closer R2 is to 1.0, the more robust the model is and the better its prediction.
After that, the surface plot was immersed in different conditions to determine the best conditions for L-methioninase production (Figures 6A–F). To identify the optimized values of the parameters, we created plots for the pairwise combination of parameters while keeping the other parameters constant at their middle level. Although the response surface plots helped evaluate the parameters, assessing them all simultaneously took work. Hence, we used Minitab’s Response Surface Optimizer function to determine the optimal levels of yeast extract (2.0%), glucose (2.0%), pH (Sahoo and Sahoo, 2021), and temperature (30°C). The model has predicted that L-methioninase will have an activity of 12.56 U/mL. The excellent correlation between the forecasted and examined values of the Box–Behnken design (as presented in Table 2) confirms the validity of the response model and the existence of an optimal point. In a similar study, Kharayat et al. used the RSM technique to optimize L-methioninase production in Bacillus subtilis, achieving 17.4 U/mL under optimized conditions. According to Sundar et al., the optimized conditions from Serratia marcescens resulted in an optimum activity of 13.7 U/mL, matching with Mokhom et al. from Alcaligenes (Mohkam et al., 2020).
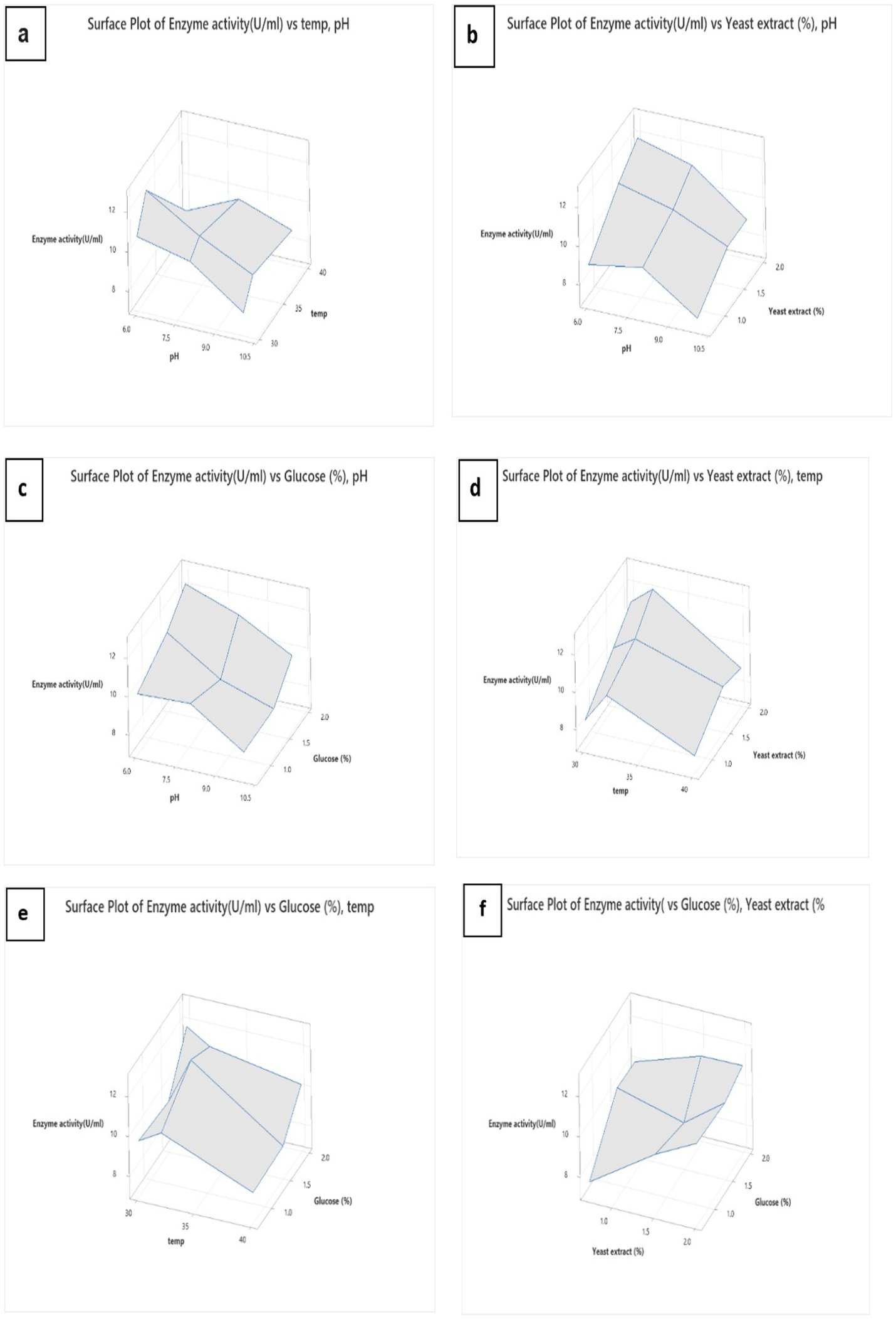
Figure 6. Surface plot of L-methioninase production, pH and temperature (A), pH and yeast extract (B), pH and glucose (C), temperature and yeast extract (D), temperature and glucose (E), and yeast extract and glucose (F).
We conducted several experiments to examine various factors affecting the production of L-methioninase. Due to the complexity of the process and the large number of experiments, we performed half of the experiments under different conditions. Our experiments found that the highest production of L-methioninase occurred when yeast extract was used at a concentration of 1.5%, glucose at 2.0% at a temperature of 30°C, and a pH level of 6. We used a Multiple Regression software called MiniTab to determine a model for predicting enzyme activity under different conidiation conditions. The model was highly accurate (86.63%) in our experiments. We then utilized this model to identify the optimal conidiation conditions for producing enzymes from Pseudomonas mosselii. According to the model, the best conidiation conditions are pH = 6, temperature = 30°C, glucose = 2%, and yeast extract = 2%, which produced 12.5670 U/mL of L-methioninase.
4 Conclusion
The current study has presented new bacterial strains for producing L-methioninase. Three bacterial genera, namely Pseudomonas mosselii spp. MN02, Ralstonia solanacearum spp. MN02, and Cytobacillus kochii spp. MN02, were isolated and identified from soil samples. We used a combination of one-factor-at-a-time (OFAT) and Box–Behnken experimental approaches to optimize the production of L-methioninase from the best producer among these three bacterial species. We analyzed the components of the environment and modeled the data, resulting in a significant increase in L-methioninase production. The Box–Behnken approached proved particularly effective in developing a mathematical model to predict the optimal placement of materials. The high R2 value of 0.852 for L-methioninase activity indicated the effectiveness of this method, which was further validated by a model accuracy of 86.63%. Initially, the production of L-methioninase by *Pseudomonas mosselii* spp. MN02 from the primary culture medium was measured at 3.25 ± 0.1 U/mL. After optimizing the culture conditions—pH at 6, temperature at 30°C, glucose at 2%, and yeast extract at 2%—the production increased to 12.5670 U/mL. This substantial increase demonstrates that *Pseudomonas mosselii* spp. MN02 could be a promising source of L-methioninase for potential use in anticancer therapy. This study highlights a new strategy for optimizing L-methioninase production, which could have significant implications for cancer treatment research.
Data availability statement
The original contributions presented in the study are included in the article/supplementary material. Further inquiries can be directed to the corresponding author.
Author contributions
MN: Conceptualization, Data curation, Formal analysis, Funding acquisition, Investigation, Methodology, Project administration, Resources, Software, Supervision, Validation, Visualization, Writing – original draft, Writing – review & editing. MM-D: Conceptualization, Data curation, Formal analysis, Funding acquisition, Investigation, Methodology, Project administration, Resources, Software, Supervision, Validation, Visualization, Writing – review & editing. PK: Conceptualization, Data curation, Formal analysis, Funding acquisition, Investigation, Methodology, Project administration, Resources, Software, Supervision, Validation, Visualization, Writing – review & editing.
Funding
The author(s) declare that no financial support was received for the research, authorship, and/or publication of this article.
Conflict of interest
The authors declare that the research was conducted in the absence of any commercial or financial relationships that could be construed as a potential conflict of interest.
Publisher’s note
All claims expressed in this article are solely those of the authors and do not necessarily represent those of their affiliated organizations, or those of the publisher, the editors and the reviewers. Any product that may be evaluated in this article, or claim that may be made by its manufacturer, is not guaranteed or endorsed by the publisher.
References
Abozeid, A. A. (2023). Short review on microbial L-methioninase and its applications. Bull. Facul. Sci. Zagazig Univ. 2022, 107–139. doi: 10.21608/bfszu.2022.148461.1155
Banihashemi, M., Safari, A., Mohkam, M., Mohammad, S. S., Rasoul-Amini, S., and Ghasemi, Y. (2016). Statistical medium optimization to increase rhamnolipid production by Pseudomonas aeruginosa sp. Minerv. Biotecnol. 28, 193–200.
Box, G., and Behnken, D. (1960). Simplex-sum designs: a class of second order rotatable designs derivable from those of first order. Ann. Math. Stat. 31, 838–864. doi: 10.1214/aoms/1177705661
Irajie, C., Mohkam, M., Vakili, B., and Nezafat, N. (2021). Computational elucidation of phylogenetic, functional and structural features of Methioninase from Pseudomonas, Escherichia, Clostridium and Citrobacter strains. Recent Pat. Biotechnol. 15, 286–301. doi: 10.2174/1872208315666210910091438
Mohkam, M., Taleban, Y., Golkar, N., Berenjian, A., Dehshahri, A., Mobasher, M. A., et al. (2020). Isolation and identification of novel L-methioninase producing bacteria and optimization of its production by experimental design method. Biocatal. Agric. Biotechnol. 26:101566. doi: 10.1016/j.bcab.2020.101566
Raboni, S., Faggiano, S., Bettati, S., and Mozzarelli, A. (2024). Methionine gamma lyase: structure-activity relationships and therapeutic applications. Biochim. Biophys. Acta Proteins Proteomics 1872:140991. doi: 10.1016/j.bbapap.2023.140991
Sahoo, S. M., and Sahoo, S. (2021). L-Asparaginase and Methioninase as prospective anticancer enzymes: current applications and production approaches. Healthcare Sustain. Environ., 349–362. doi: 10.1007/978-981-33-4195-1_16
Salim, N., Santhiagu, A., and Joji, K. (2019). Process modeling and optimization of high yielding L-methioninase from a newly isolated Trichoderma harzianum using response surface methodology and artificial neural network coupled genetic algorithm. Biocatal. Agric. Biotechnol. 17, 299–308. doi: 10.1016/j.bcab.2018.11.032
Selim, M., Elshikh, H., el-Hadedy, D. E., Saad, M., Eliwa, E., and Abdelraof, M. (2015). L-Methioninase from some Streptomyces isolates I: isolation, identification of best producers and some properties of the crude enzyme produced. J. Gen. Eng. Biotechnol. 13, 129–137. doi: 10.1016/j.jgeb.2015.08.001
Siblini, Y., Namour, F., Oussalah, A., Guéant, J.-L., and Chéry, C. (2022). Stemness of Normal and Cancer cells: the influence of methionine needs and SIRT1/PGC-1α/PPAR-α players. Cells 11:3607. doi: 10.3390/cells11223607
Singh, P., and Kharayat, B. (2014). Statistical optimization of reaction condition of L-methioninase from Bacillus subtilis. Research Reports 2.
Sneath, P. H., Mair, N. S., Sharpe, M. E., and Holt, J. G. (1986). Bergey\u0027s manual of systematic bacteriology 21986.
Keywords: L-Methioninase, cancer, native bacteria, optimization process, fermentation
Citation: Nasirian M, Mobini-Dehkordi M and Khosravian P (2024) The native Iranian soil bacteria with high potential to produce extracellular methionine gamma-lyase. Front. Microbiol. 15:1504742. doi: 10.3389/fmicb.2024.1504742
Edited by:
Mariusz Cycoń, Medical University of Silesia, PolandReviewed by:
Lilia Arely Prado Barragan, Metropolitan Autonomous University, MexicoShuchi Singh, University of Illinois at Urbana-Champaign, United States
Copyright © 2024 Nasirian, Mobini-Dehkordi and Khosravian. This is an open-access article distributed under the terms of the Creative Commons Attribution License (CC BY). The use, distribution or reproduction in other forums is permitted, provided the original author(s) and the copyright owner(s) are credited and that the original publication in this journal is cited, in accordance with accepted academic practice. No use, distribution or reproduction is permitted which does not comply with these terms.
*Correspondence: Mohsen Mobini-Dehkordi, bW1vYmluaWRAZ21haWwuY29t