- 1Guangdong Laboratory for Lingnan Modern Agriculture, National Risk Assessment Laboratory for Antimicrobial Resistance of Animal Original Bacteria, College of Veterinary Medicine, South China Agricultural University, Guangzhou, China
- 2State Key Laboratory for Animal Disease Control and Prevention, South China Agricultural University, Guangzhou, China
- 3Guangdong Wenshi Dahuanong Biotechnology Co. Ltd., Yunfu, Guangdong, China
- 4Animal Laboratory Center, Guangzhou University of Chinese Medicine, Guangzhou, China
- 5Guangdong Provincial Key Laboratory of Veterinary Pharmaceutics Development and Safety Evaluation, South China Agricultural University, Guangzhou, China
Introduction: The emergence of the wide variety of novel tigecycline resistance tet(X) variants, including tet(X3), tet(X4), tet(X5), and tet(X6), has raised a serious threat to global public health and posed a significant challenge to the clinical treatment of multidrug-resistant bacterial infections.
Methods: In this study, we evaluated the synergism of tigecycline combining with other antibiotics as a means of overcoming the tet(X)-mediated resistance in Acinetobacter spp. Antibiotic synergistic efficacy was evaluated through in vitro chequerboard experiments, time-kill assays and dose–response curves. The in vivo synergistic effect of the combination was confirmed in a mouse model of thigh with neutrophilic granulocyte reduction. Additionally, combinations were tested for their ability to prevent high-level tigecycline-resistant mutants.
Results: We found that the combinations of tigecycline with apramycin exhibited synergistic activity against tet(X)-harboring Acinetobacter spp. with FICI of 0.088. The MICTGC decreased more than 5 times in the presence of subinhibitory levels of apramycin. The combination showed in vitro synergism in time-kill assays and in vivo therapeutic effectiveness in the mouse thigh infection model.
Discussion: This study shed light on the synergism of tigecycline in combination with apramycin which offers a viable therapeutic alternative for infections caused by tet(X)-harboring Acinetobacter spp.
1 Introduction
The rapid emergence and global dissemination of Acinetobacter spp. as a major nosocomial pathogen is remarkable, particularly in intensive care units where it can cause illnesses such as pneumonia, tracheobronchitis, meningitis, endocarditis, peritonitis, skin and soft tissue infection and urinary tract and bloodstream infections (Glew et al., 1977; Malta et al., 2020). In recent decades, infections caused by Acinetobacter spp. have increased in frequency and severity due to the abuse of antibiotics and antimicrobial resistance (Zweier, 2014). Currently, the options for combatting multidrug-resistant Acinetobacter spp. using antibiotics are limited.
Universally, treatment regimens for Acinetobacter spp. infections rely on colistin and tigecycline, either alone or in combination with carbapenems (Cheng et al., 2021). Tragically, these last line agents for treating MDR-bacterial infections are increasingly challenged by the emergence and spread of antimicrobial resistance genes such as tet(X4)/(X5), mcr and blaNDM/blaKPC (Cui C. et al., 2020; Li et al., 2020). Tigecycline, approved for clinical use in 2005, can overcome most of the resistance mechanisms related to tetracyclines (Koomanachai et al., 2009; Rubinstein and Vaughan, 2005; Sun et al., 2019). It provides an opportunity for its use in nosocomial infections where resistance is more likely to occur, especially as the incidence of multidrug-resistant (MDR) Acinetobacter spp. is rising (Lee et al., 2017). However, this last-resort antibiotic may become ineffective due to new tigecycline resistance tet(X) variants that include tet(X3), tet(X4), tet(X5), and tet(X6). Recent studies have highlighted the urgent need for new treatment options (Tang et al., 2021; Cui Z. H. et al., 2020). In such situations, tigecycline-based regimens are alternative treatments against MDR bacteria (Hornsey and Wareham, 2011; Tamma et al., 2012; Nulsopapon et al., 2021) and it is crucial to explore effective drug combination to reduce the required tigecycline dosage.
Combination therapy is a method that involves the use of two or more active antibiotics in conjunction. This approach minimizes the dosage of toxic drugs and reduces the frequency of drug resistance, achieving more significant biochemical effects than monotherapy (Fitzgerald et al., 2006). For instance, a combination regimen of tigecycline and amikacin efficiently inhibited development of tigecycline resistance and successfully suppressed emergence of resistant populations (Moland et al., 2008). Tigecycline activity was also increased both in vitro and in vivo when used in combination with zidovudine against E. coli that carried tet(X) and mcr-1 (Zhou et al., 2020). Additionally, the use of apramycin in a mouse model of A. baumanii was effective when the AUC/MIC ratio was >50 and Cmax/MIC was 10 or higher (Meyer et al., 2014).
Here we explore the combination of tigecycline and apramycin. There are only a limited number of studies exploring antibiotic synergy and its mechanisms. Therefore, we sought to explore whether apramycin in combination with tigecycline can be effective against multidrug-resistant (MDR) Acinetobacter spp. strains harboring tet(X).
2 Methods and materials
2.1 Bacterial profiling and antimicrobial susceptibility
A total of nine Acinetobacter isolates carrying different tet(X) variants were separated from human, pig, migratory bird and environmental samples over the years and stored in a 30% glycerin broth at −80°C. The bacterial species were identified by matrix-assisted laser desorption/ionization time of flight mass spectrometry (MALDI-TOF, MS). The presence of tet(X) and co-harboring with blaNDM were identified by polymerase chain reaction (PCR) amplification and sequencing as previously reported (Cui C. et al., 2020). Antimicrobial susceptibility testing of tigecycline, meropenem, colistin, polymyxin B and the aminoglycosides amikacin, gentamicin, apramycin, kanamycin was performed by broth microdilution or disc diffusion methods according to EUCAST guidelines (EUCAST, 2023) And reference strain ATCC 25922 served as quality control.
2.2 Checkerboard assays and fractional inhibitory concentration index (FICI)
The checkerboard assay was used to test the joint antibacterial activity of tigecycline combined with seven antibiotics (see above). The reasons for these tests were as follows: (i) these drugs remained certain active against multidrug-resistant (MDR) Acinetobacter isolates (Fishbain and Peleg, 2010; Kang et al., 2017); (ii) some of them, like meropenem and colistin are still considered last resort treatments of infections caused by MDR Gram-negative bacteria (Hornsey and Wareham, 2011; Gordon and Wareham, 2010); (iii) few studies have evaluated the synergistic potential of tigecycline combinations against Acinetobacter spp. (Li et al., 2017).
In brief, isolate FS38-2 (MICTGC = 16 μg/mL and co-harboring tet(X3), tet(X6), and blaNDM-1 genes) was randomly selected for screening the synergic partners combining with tigecycline for the other seven antibiotics. The checkerboard assays of the potential drug combinations were performed against all nine isolates to further confirm the synergism. Bacterial cultures of 106 CFU/mL were exposed to 2-fold dilutions of antibiotics with concentrations ranging from 1/8× to 8 × MIC in 96-well plates and incubated for 18 h at 37°C. The FICI was analyzed by the following equation:
where FICI ≤0.5 represents “synergism”; 0.5 < FICI ≤1 means “addition”; “indifferent” was defined when 1 < FICI ≤2; and FICI >2 denotes “antagonism” (Yu et al., 2018).
2.3 MICsTGC in presence of apramycin
MIC values were determined using broth microdilution. Two-fold serial dilutions of tigecycline ranged from 0.25 to 128 μg/mL in 96-well microplates, respectively. The final concentration of apramycin was 0, 0.25, 0.5, 1, 2 μg/mL by adding 50 μL of different concentrations to each well. Furthermore, a purified single colony of isolated strains was picked up and emulsified to using MH broth medium. After that, 100 μL bacterial solution was added to make the final bacterial suspension contained an inoculum density of ~106 CFU/mL. MICsTGC in presence of apramycin were determined after incubation at 37°C for 18 h.
2.4 Time-killing assays
To further evaluate the synergistic effect of potential combinations, tet(X)-harboring Acinetobacter strains: A. lwoffi HZE30-1 and A. indicus WF106-1 (both harboring tet(X3), tet(X6), and blaNDM-1), Acinetobacter HNS1-2 carrying tet(X3) and A. indicus Q186-3 carrying tet(X4) and tet(X5) were randomly selected for in vitro time-killing assays. It was performed to evaluate the synergistic activity of sub-MICs of tigecycline and apramycin alone or in combination using LB broth medium with an initial inoculum of 106 CFU/mL Acinetobacter strains as previously described. The prepared system was then incubated at 37°C and 180 rpm, and 100 μL of the suspension was diluted at 0, 3, 6, 9, and 24 h for bacterial counting.
2.5 Dose-response assays
As described previously (Yu et al., 2018), OD values at 8 h was selected to calculate the correlation between antimicrobial effects and dosage of drugs with formula using GraphPad Prism Version 8. The OD values for bacterial growth in the absence of antibiotics was used as the normalization standard. Results are shown as the mean value of all 9 isolates at a 95% CI. The dose–response correlation of tigecycline or apramycin was calculated with the equation:
where E is the bacterial growth estimated as the OD600 normalized by that of control; Emax is the maximal antimicrobial efficacy; E0 is the bacterial growth in the absence of any antibiotics; C is the concentration of tigecycline or apramycin; IC50 is the drug concentration demonstrating 50% of Emax; Hillslope describes the curve gradient.
2.6 In vivo therapeutic experiment
As schematically illustrated in Figure 1A, the neutropenic mouse thigh model was employed for testing the in vivo synergistic efficacy of tigecycline plus apramycin. Specific-pathogen-free (SPF) female ICR mice weighing 25 ± 2 g at six-weeks-old (Hunan Silaikejingda Lab Animal, Hunan, China) were rendered temporarily neutropenic by immunosuppression with cyclophosphamide (Yuanye Biotechnology, Shanghai, China) at 150 mg/kg on the first four days and 100 mg/kg at the fifth day by intraperitoneal injection before bacterial inoculation (23). Four tet(X)-harboring strains used in the in vitro time-killing curve assays were employed here. The mid-log bacterial cultures were appropriately diluted with normal saline and the neutropenic mice (neutrophils ≤100/mm3) were then intramuscularly inoculated with 100 μL of bacterial suspension (106 CFU/mL) into each posterior thigh muscle. After 1 h, infected mice were administered in the following manner: phosphate buffer saline (PBS) as control (Group I), tigecycline or apramycin alone as single-drug groups (Group II and III); and tigecycline combining with apramycin (Group IV). Tigecycline was administrated at 5 mg/kg subcutaneously, apramycin as 20 mg/kg subcutaneously and the injection volume was 100 μL for all drugs. The tigecycline doses were selected to mimic the pharmacokinetic profiles of recommended human clinical doses (Koomanachai et al., 2009; Meagher et al., 2005). In the case of apramycin, the doses were selected based on previous reports of pharmacodynamic effects on different MIC and preliminary experimental results (Meyer et al., 2014; Kang et al., 2018). The group IV was simultaneously injected subcutaneously with tigecycline 5 mg/kg/d and apramycin 20 mg/kg/d. Following 24 h of treatment, mice were sacrificed and thigh homogenates were sampled for bacterial burden quantification. Differences between groups were estimated by one-way analysis of variance (ANOVA) with 95% CI and graphs were generated using GraphPad Prism Version 8.
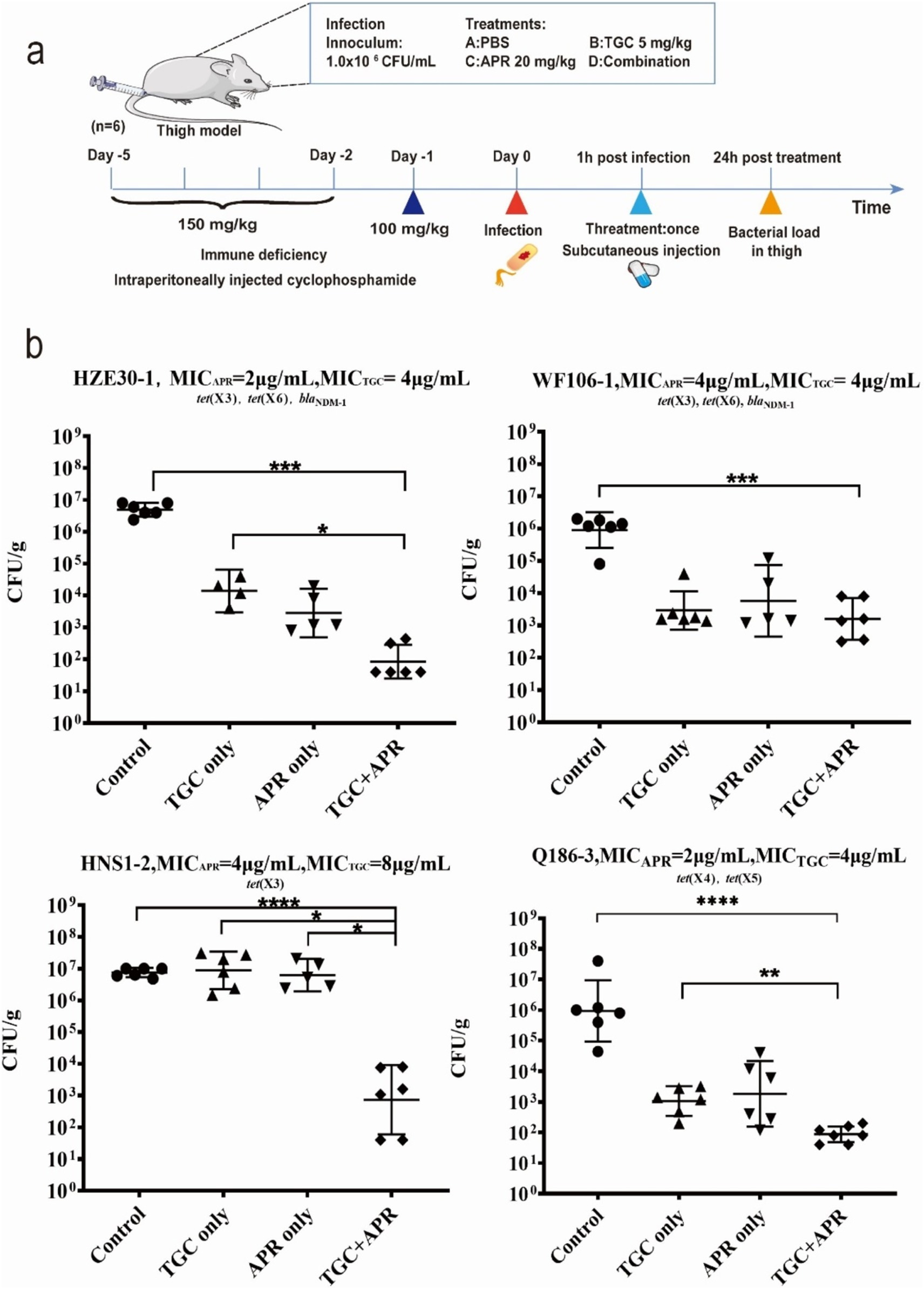
Figure 1. The in vivo synergy of tigecycline combining apramycin. (A) Scheme of the experimental protocol of animal trials. (B) Combination therapy of tigecycline plus apramycin in a mouse thigh model. *p < 0.05; **p < 0.01; ***p < 0.005; ****p < 0.001 by the one-way ANOVA.
2.7 Prevention of high-level tigecycline-resistant mutants
To further investigate whether antibiotic combinations prevent the occurrence of high-level tigecycline-resistant mutants, nine strains were challenged with prolonged and repeated exposure to tigecycline alone and tigecycline plus apramycin. Approximately 106 CFU/mL of tet(X)-carrying Acinetobacter isolates were challenged by tigecycline only and tigecycline plus 0.5 μg/mL apramycin. After incubating at 37°C for 18 h, cultures from each MIC well and the last well containing bacterial growth were mixed and challenged with the antibiotic groups described above. This protocol was repeated continuously for 14 days.
3 Results
3.1 Bacterial information
Table 1 showed the profiles of nine tet(X)-carrying Acinetobacter isolates, including Acinetobacter haemolyticus, Acinetobacter spp. and Acinetobacter beijerinckii isolates carrying tet(X3), Acinetobacter lwoffii and Acinetobacter indicus isolates co-harboring tet(X3), tet(X6) and blaNDM-1 and three A. indicus isolates harboring tet(X3), tet(X6)/blaNDM-3/tet(X4), and tet(X4)/tet(X5). All nine strains were resistant to tigecycline with MICs of 4–16 μg/mL but susceptible to colistin, polymyxin B, amikacin and apramycin. Four blaNDM-positive isolates exhibited resistance to meropenem and two A. indicus isolates were resistant to gentamicin.
3.2 In vitro checkerboard assays
In Figure 2A, strain FS38-2 (tet(X3), tet(X6), and blaNDM-1) was selected for screening the synergism of tigecycline combined with other 7 antibiotics. The combination of tigecycline plus apramycin produced a significant antibacterial effect (FICI = 0.088). The other antibiotics with tigecycline showed ether indifferent or antagonistic interactions (FICI >1). Additionally, further evaluation of tigecycline plus apramycin was repeated against nine Acinetobacter strains and FICIaverage of 0.32 was detected by checkerboard assays (Figure 2B; Supplementary Table S1).
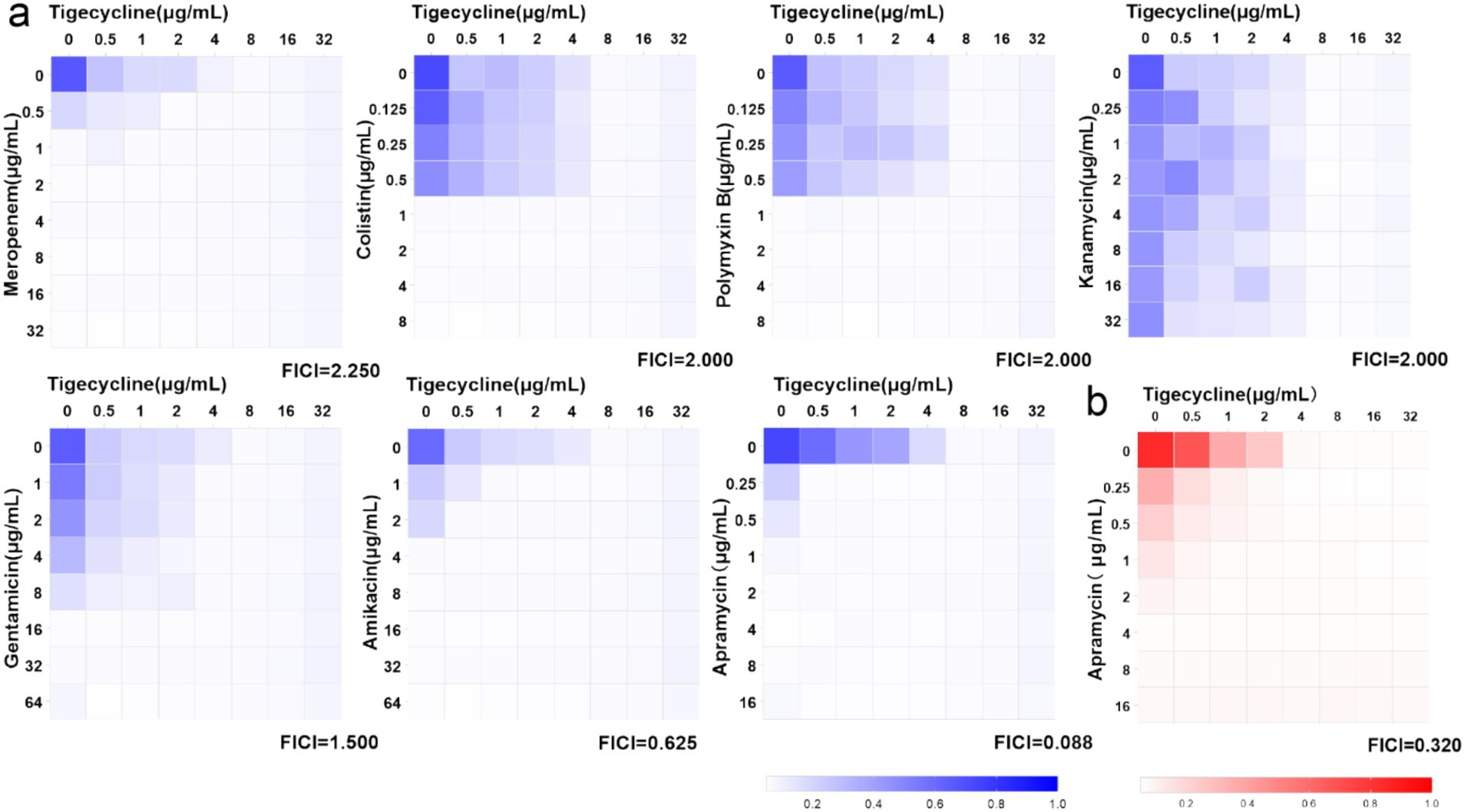
Figure 2. Heat-maps of checkerboard assays for tigecycline combined with the listed antibiotics. (A) Strain FS38-2 was used in the preliminary screen. (B) Synergistic effect of tigecycline plus apramycin against nine strains (FICIaverage = 0.32, n = 9 biological replicates).
3.3 MICsTGC in presence of apramycin
A combination of tigecycline and a series of sub-inhibitory concentrations of apramycin to determine whether the combination of the two is effective. As apramycin concentration increasing, the MICsTGC distribution shifted towards lower MIC values of 0.25–0.50 μg/mL (Figure 3A). Over 5 times reduction of tigecycline MIC was observed in the presence of subinhibitory levels of apramycin. Notably, tigecycline MICs dropped more than 20 times in presence of 2 μg/mL apramycin on average (n = 9), which was significantly lower than 0.25 μg/mL apramycin (p < 0.001) (Figure 3B).
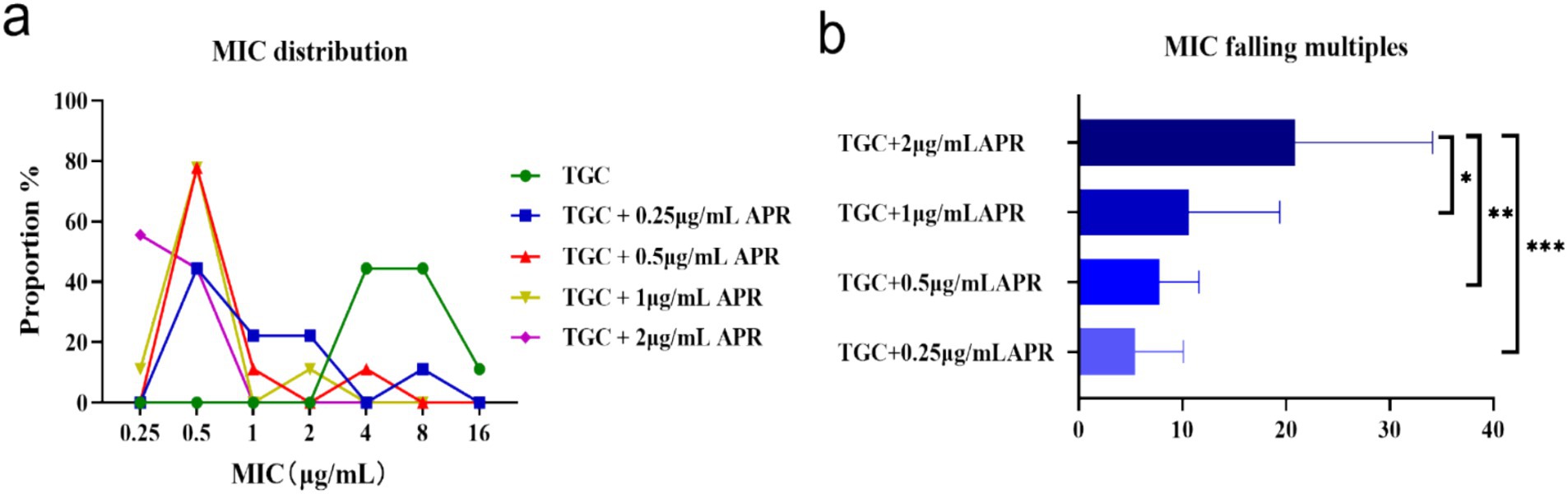
Figure 3. MIC changes of tigecycline against 9 tet(X)-harboring Acinetobacter strains when combined with apramycin at various concentrations. (A) MIC distributions of tigecycline alone or combined with different doses of apramycin. (B) MIC reductions of tigecycline in the presence of apramycin. *p < 0.05, **p < 0.01, and ***p < 0.001 by the one-way ANOVA. APR, apramycin; TGC, tigecycline; control, normal saline.
3.4 Time-kill assay
The time-killing curves of tet(X)-harboring Acinetobacter strains treated with tigecycline plus apramycin were shown in Figure 4, and the grow of 4 Acinetobacter isolates (HZE 30-1, WF106-1, HNS1-2, Q186-3) were all inhibited with the delayed logarithmic phase and stationary phase. Synergistic effects for the combinations were determined in isolates HZE 30–1, WF106-1, HNS1-2, and Q186-3 with concentrations of 1MIC TGC + 1/4MIC APR, 1MIC TGC + 1/8MIC APR, 1/2MIC TGC + 1/8MIC APR, and 1MIC TGC + 1/4MIC APR, respectively. In Figure 4, none of the drugs alone could completely inhibit bacterial growth during the nine hours of exposure. On the contrary, the cell growth and proliferation were severely inhibited using the combined regimen after 9 h of incubation showing an effective synergism. After 24 h, more than 5 log10 reductions in colony count were detected in all combination groups.
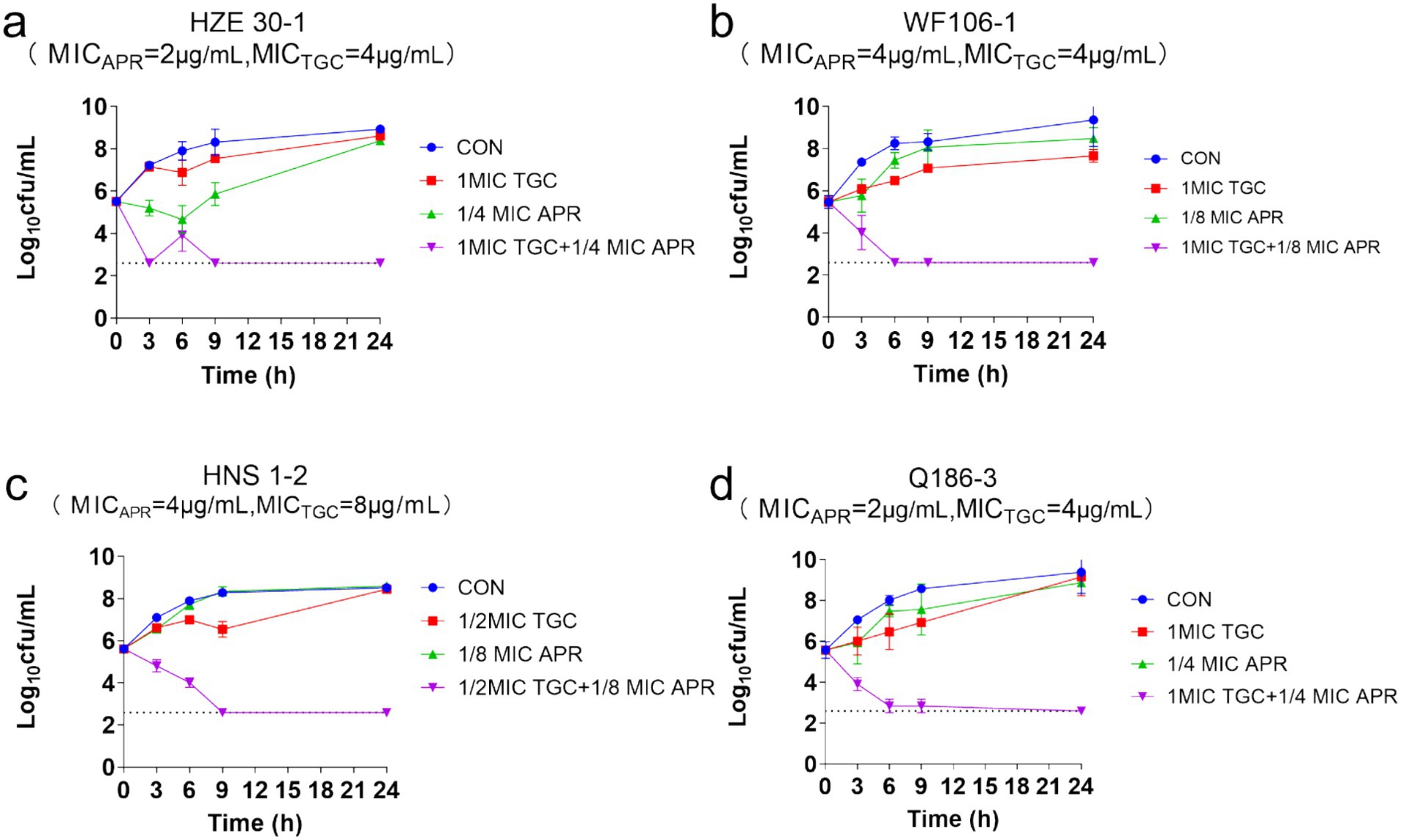
Figure 4. The time-killing curves of Acinetobacter strains treated with apramycin, tigecycline, and combined regimen.
3.5 Dose-response curves
The concentration-effect relationship was fitted to a Hill-type equation in order to accurately determine the response to antibiotic challenge. Curves for tigecycline alone and tigecycline plus apramycin illustrated that greater inhibitory activity were obtained as the tigecycline concentration was increased (Figure 5A). Importantly, compared with the single-drug groups, the IC50 of two-drug groups (apramycin with 0.5 μg/mL tigecycline and tigecycline with 0.5 μg/mL apramycin) dropped to <0.0001 μg/mL (Table 2).
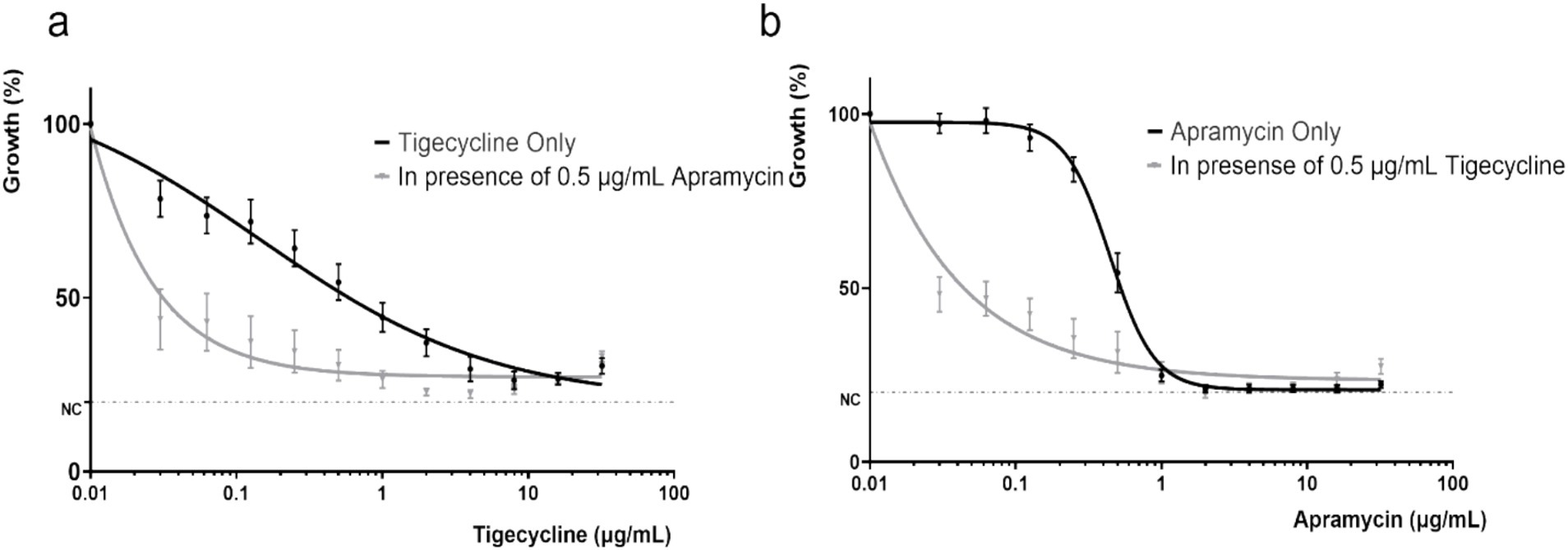
Figure 5. Dose response curves of different combinations of tigecycline and apramycin for tet(X)-harboring Acinetobacter strains. (A) Dose response curve of tigecycline alone and in combination with apramycin (0.5 μg/mL). (B) Dose response curve of apramycin alone and in combination with tigecycline (0.5 μg/mL). NC, negative control (minimum detection limit).

Table 2. Dose–response parameters of different combinations of tigecycline and apramycin against 9 tet(X)-harboring Acinetobacter.
3.6 In vivo synergistic efficacy
We next examined whether this synergism would also be apparent in vivo using a neutropenic mouse thigh model. The bacterial density in the control groups were maintained at 106–107 CFU/g after 24 h treatment with saline. In contrast, bacterial growth was reduced to 102–103 CFU/g using combination therapy against all four test strains (p < 0.001). Significant differences (p < 0.05) between groups of tigecycline alone and combined with apramycin were shown in Figure 1B against tested strains except WF106-1. Comparing with apramycin alone, the combined regimen showed a slight decrease in bacterial burden after 24 h treatment, but a significant bacterial reduction was only observed against strain HNS1-2 (p < 0.05). These data indicated that combination therapy of tigecycline and apramycin generated a synergistic antibacterial efficacy in vivo.
3.7 High-level tigecycline-resistance development
When tested strains were exposed to tigecycline alone, tigecycline MICs for most of the strains showed rapid fluctuating rising trend. This was especially apparent for strains MM119-1 and Z51-2 with tigecycline MICs reached 64 μg/mL on the third or fourth day. The addition of 0.5 μg/mL apramycin slowed down the booming of tigecycline MIC, however, it would reach to 16 to 32 μg/mL after 10 days exposure. The findings indicated that strains were losing sensitivity with continuous exposure to even the combined regimen. Notably, during the 14 days of passaging only strain JXZ5-1 was completely inhibited by the combined drugs (Figure 6).
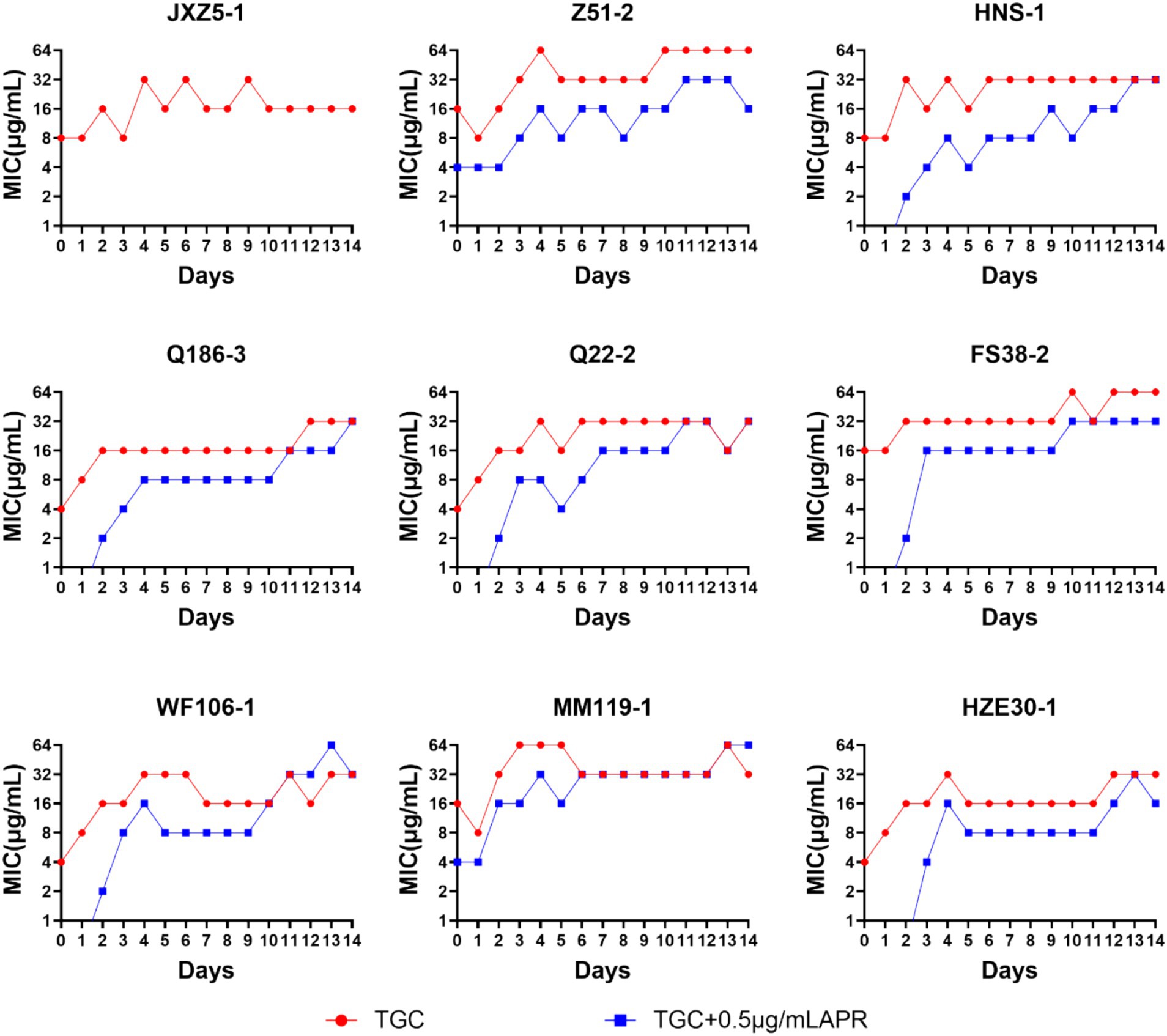
Figure 6. MICTGC changes of tet(X)-harboring Acinetobacter strains under drug selective pressure for 14 days. Red line, tigecycline alone; blue line, tigecycline plus 0.5 μg/mL apramycin.
4 Discussion
The majority of the tet(X)-harboring Acinetobacter strains are associated with livestock, raising concerns that the tigecycline-resistance tet(X) genes could be transmitted to humans through opportunistic pathogens like Acinetobacter (Cheng et al., 2021; Chen et al., 2020; Nulsopapon et al., 2021). Previously reported, infections caused by non-fermentative Gram-negative bacilli have been treated successfully with tigecycline plus aminoglycosides (Zhou et al., 2019). Our study proved that the combination of tigecycline and apramycin was effective against Acinetobacter strains possessing tet(X) as well (Figure 2B; Supplementary Table S1).
Combination therapy has become a commonly employed approach for the treatment of severe infections caused by Acinetobacter spp. (Nasr, 2020). In our study, when partnered with apramycin, certain strains reverted to the tigecycline sensitive phenotype especially with the addition of 2 μg/mL apramycin and resulted in a 20-times lower in tigecycline MIC (Figure 3). The FICI and the in vitro time-killing curves (Figure 4) indicated that apramycin is a candidate potentiating tigecycline against tet(X)-harboring Acinetobacter strains. Dose response models in preclinical trials can be predictors of drug interactions (Kong and Lee, 2006), we found the accentuated bacteriostatic effect of tigecycline in the presence apramycin (Figure 5; Table 2). Moreover, a recent report demonstrated the potential of combining colistin/amikacin and tigecycline to achieve synergistic effects against Acinetobacter strains further support our findings (Wu et al., 2021).
Although the initial response of patients to a combination therapy often appears promising, the emergence of resistance to continuous therapy is common (He et al., 2020). In our group of tet(X)-carrying isolates, one was significantly inhibited or killed by tigecycline plus 0.5 μg/mL apramycin during the 14 days of passaging and only 22.2% of isolates possessed a high tigecycline MIC (64 μg/mL) with continuous exposure of the combination (Figure 6). This indicated that apramycin may delay the MIC increase of tigecycline.
Further confirmation of the antimicrobial activity of the combination therapy was carried out using an in vivo mouse model. Four strains carrying different tet(X) variants were colonized in the mouse thigh as expected. During the treatment, differences of 3–5 log CFU/g were achieved between control groups and the combined treatment groups and differences of 1–5 log CFU/g were observed between monotherapies and the combination therapies. Drug combination demonstrated significant antibacterial efficacy when compared to the control group (Figure 1). Previous reports have indicated that elevated MICs of either apramycin or tigecycline would dampen their clinical efficacy when utilized as a monotherapy option (Abdul-Mutakabbir et al., 2021; Sader et al., 2005; Lee et al., 2016). We found that the combination displayed a highly significant synergistic response in vivo against strain HNS1-2 (MICTGC = 8 μg/mL, MICAPR = 4 μg/mL) carrying tet(X3), while tigecycline monotherapy failed to produce antibacterial outcomes. These preliminary results suggest that combinations of tigecycline with apramycin may exhibit activity against tigecycline-resistant strains.
Notably, apramycin has also been demonstrated to be of lower toxicity than other aminoglycosides (Ishikawa et al., 2019). Apramycin has completed a Phase 1 clinical trial assessing the safety, tolerability, and pharmacokinetics in healthy adults (ClinicalTrials.gov ID: NCT04105205). Given these promising findings, a combination of apramycin and tigecycline may offer a potential therapeutic option for the treatment of Acinetobacter infections in the future. While the present study provides promising results from both in vitro and in vivo experiments, the translation of these findings to clinical practice requires further validation. Future studies should focus on clinical trials and the use of clinical samples to assess the feasibility of applying our findings to patient populations.
Tigecycline, the first member of the glycylcycline class of antibiotics, has shown promising in vitro activity against multidrug-resistant strains. It binds with high affinity to bacterial ribosomes and is unaffected by the typical mechanisms that render bacteria resistant to the tetracycline class. Aminoglycosides inhibit protein synthesis by binding to the 16S rRNA and disrupt the integrity of bacterial cell membranes that may facilitate tigecycline passive accumulation (Vidaillac et al., 2012; Shakil et al., 2008). During combination therapy, tigecycline might lead to synergistic bactericidal effects by enhancing the aminoglycoside effect and inhibiting bacterial adaptive responses (Ma et al., 2022). Further research is needed to determine the exact mechanisms for the success of this combination therapy.
This study provides strong evidence that the combination of tigecycline and apramycin exhibited synergistic activity against tet(X)-harboring Acinetobacter strains. Both in vitro and in vivo results show that this combination reduces the MIC of tigecycline and enhances its bactericidal activity. Apramycin appears to delay the emergence of resistance and potentiates the efficacy of tigecycline, offering a promising strategy for treating multidrug-resistant Acinetobacter infections. The findings highlight the potential of this combination therapy to address the growing challenge of multidrug-resistant Acinetobacter spp., offering a valuable treatment option where other antibiotics may have failed.
Data availability statement
The data presented in the study are deposited in the NCBI SRA repository, under the BioProject accession number PRJNA1194363.
Ethics statement
The animal study was approved by the Animal Research Committee of South China Agricultural University and the Guangdong Association for Science and Technology [ID: SCXK (Guangdong) 2018-0002]. Guangdong Laboratory Animal Welfare and Ethics and the Institutional Animal Care and Use Committee of the South China Agricultural University [2021F138]. The study was conducted in accordance with the local legislation and institutional requirements.
Author contributions
JL: Conceptualization, Writing – original draft, Writing – review & editing, Investigation, Software. S-LZ: Conceptualization, Data curation, Investigation, Methodology, Software, Supervision, Writing – review & editing. J-JW: Data curation, Methodology, Supervision, Writing – original draft, Writing – review & editing. MZ: Data curation, Methodology, Supervision, Writing – review & editing. D-TC: Data curation, Methodology, Supervision, Writing – review & editing. YZ: Methodology, Supervision, Writing – review & editing, Data curation. JS: Formal analysis, Project administration, Validation, Writing – review & editing. X-PL: Formal analysis, Project administration, Validation, Writing – review & editing. YY: Formal analysis, Funding acquisition, Project administration, Resources, Visualization, Writing – review & editing, Validation.
Funding
The author(s) declare that financial support was received for the research, authorship, and/or publication of this article. This study was jointly supported by the Foundation for Innovative Research Groups of the National Natural Science Foundation of China (32121004); National key research and development program of China (2023YFD1800100); the Local Innovative and Research Teams Project of Guangdong Pearl River Talents Program (2019BT02N054); the National Key Research & Development Program of China (grant no. 2022YFE0103200); the Natural Science Foundation of Guangdong Province, China (General Program: 2022A1515011194); Double first-class discipline promotion project (2023B10564003); the 111 Center (D20008); the National Natural Science Foundation of China (grant no. 32002337) and the Guangdong provincial key research and development program (2023B0202150001).
Conflict of interest
JL was employed by Guangdong Wenshi Dahuanong Biotechnology Co. Ltd.
The remaining authors declare that the research was conducted in the absence of any commercial or financial relationships that could be construed as a potential conflict of interest.
Generative AI statement
The author(s) declare that no Gen AI was used in the creation of this manuscript.
Publisher’s note
All claims expressed in this article are solely those of the authors and do not necessarily represent those of their affiliated organizations, or those of the publisher, the editors and the reviewers. Any product that may be evaluated in this article, or claim that may be made by its manufacturer, is not guaranteed or endorsed by the publisher.
Supplementary material
The Supplementary material for this article can be found online at: https://www.frontiersin.org/articles/10.3389/fmicb.2024.1502558/full#supplementary-material
References
Abdul-Mutakabbir, J. C., Yim, J., Nguyen, L., Maassen, P. T., Stamper, K., Shiekh, Z., et al. (2021). In vitro synergy of colistin in combination with meropenem or tigecycline against carbapenem-resistant Acinetobacter baumannii. Antibiotics 10:880. doi: 10.3390/antibiotics10070880
Chen, C., Cui, C. Y., Yu, J. J., He, Q., Wu, X. T., He, Y. Z., et al. (2020). Genetic diversity and characteristics of high-level tigecycline resistance Tet(X) in Acinetobacter species. Genome Med. 12, 111–113. doi: 10.1186/s13073-020-00807-5
Cheng, Y.-Y., Liu, Y., Chen, Y., Huang, F.-M., Chen, R.-C., Xiao, Y.-H., et al. (2021). Sporadic dissemination of tet (X3) and tet (X6) mediated by highly diverse Plasmidomes among livestock-associated Acinetobacter. Microbiol. Spectr. 9:e0114121. doi: 10.1128/Spectrum.01141-21
Cui, C., Chen, C., Liu, B., He, Q., Wu, X., Sun, R., et al. (2020). Co-occurrence of plasmid-mediated Tigecycline and Carbapenem resistance in Acinetobacter spp. from waterfowls and their neighboring environment. Antimicrob. Agents Chemother. 64:2502. doi: 10.1128/AAC.02502-19
Cui, Z. H., Ni, W. N., Tang, T., He, B., Zhong, Z. X., Fang, L. X., et al. (2020). Rapid detection of plasmid-mediated high-level tigecycline resistance in Escherichia coli and Acinetobacter spp. J. Antimicrob. Chemother. 75, 1479–1483. doi: 10.1093/jac/dkaa029
EUCAST (2023). The European committee on antimicrobial susceptibility testing. Breakpoint tables for interpretation of MICs and zone diameters. Version 14.0, 2023. Available online at: http://www.eucast.org/fileadmin/src/media/PDFs/EUCAST_files/Breakpoint_tables/v_5.0_Breakpoint_Table_01.pdf
Fishbain, J., and Peleg, A. Y. (2010). Treatment of Acinetobacter infections. Clin. Infect. Dis. 51, 79–84. doi: 10.1086/653120
Fitzgerald, J. B., Schoeberl, B., Nielsen, U. B., and Sorger, P. K. (2006). Systems biology and combination therapy in the quest for clinical efficacy. Nat. Chem. Biol. 2, 458–466. doi: 10.1038/nchembio817
Glew, R. H., Moellering, M., and Kunz, L. J. (1977). Infections with Acinetobacter calcoaceticus (herellea vaginicola):clinical and laboratory studies. Medicine (Baltimore) 56, 79–97. doi: 10.1097/00005792-197703000-00001
Gordon, N. C., and Wareham, D. W. (2010). Multidrug-resistant Acinetobacter baumannii: mechanisms of virulence and resistance. Int. J. Antimicrob. Agents 35, 219–226. doi: 10.1016/j.ijantimicag.2009.10.024
He, W., Demas, D. M., Conde, I. P., Shajahan-Haq, A. N., and Baumann, W. T. (2020). Mathematical modelling of breast cancer cells in response to endocrine therapy and Cdk4/6 inhibition. J. R. Soc. Interface 17:339. doi: 10.1098/rsif.2020.0339
Hornsey, M., and Wareham, D. W. (2011). In vivo efficacy of glycopeptide-colistin combination therapies in a galleria mellonella model of Acinetobacter baumannii infection. Antimicrob. Agents Chemother. 55, 3534–3537. doi: 10.1128/AAC.00230-11
Ishikawa, M., García-Mateo, N., Čusak, A., López-Hernández, I., Fernández-Martínez, M., Müller, M., et al. (2019). Lower ototoxicity and absence of hidden hearing loss point to gentamicin C1a and apramycin as promising antibiotics for clinical use. Sci. Rep. 9, 2410–2415. doi: 10.1038/s41598-019-38634-3
Kang, A. D., Smith, K. P., Berg, A. H., Truelson, K. A., Eliopoulos, G. M., McCoy, C., et al. (2018). Efficacy of apramycin against multidrug-resistant Acinetobacter baumannii in the murine neutropenic thigh model. Antimicrob. Agents Chemother. 62:2585. doi: 10.1128/AAC.02585-17
Kang, A. D., Smith, K. P., Eliopoulos, G. M., Berg, A. H., McCoy, C., and Kirby, J. E. (2017). In vitro Apramycin activity against multidrug-resistant Acinetobacter baumannii and Pseudomonas aeruginosa. Diagn. Microbiol. Infect. Dis. 88, 188–191. doi: 10.1016/j.diagmicrobio.2017.03.006
Kong, M., and Lee, J. J. (2006). A generalized response surface model with varying relative potency for assessing drug interaction. Biometrics 62, 986–995. doi: 10.1111/j.1541-0420.2006.00579.x
Koomanachai, P., Kim, A., and Nicolau, D. P. (2009). Pharmacodynamic evaluation of tigecycline against Acinetobacter baumannii in a murine pneumonia model. J. Antimicrob. Chemother. 63, 982–987. doi: 10.1093/jac/dkp056
Lee, Y. T., Chiang, M. C., Kuo, S. C., Wang, Y. C., Lee, I. H., Chen, T. L., et al. (2016). Carbapenem breakpoints for Acinetobacter baumannii group: supporting clinical outcome data from patients with bacteremia. PLoS One 11, 1–13. doi: 10.1371/journal.pone.0163271
Lee, C. R., Lee, J. H., Park, M., Park, K. S., Bae, I. K., Kim, Y. B., et al. (2017). Biology of Acinetobacter baumannii: pathogenesis, antibiotic resistance mechanisms, and prospective treatment options. Front. Cell. Infect. Microbiol. 7:55. doi: 10.3389/fcimb.2017.00055
Li, R., Peng, K., Li, Y., Liu, Y., and Wang, Z. (2020). Exploring tet(X)-bearing tigecycline-resistant bacteria of swine farming environments. Sci. Total Environ. 733:139306:139306. doi: 10.1016/j.scitotenv.2020.139306
Li, J., Yang, X., Chen, L., Duan, X., and Jiang, Z. (2017). In vitro activity of various antibiotics in combination with Tigecycline against Acinetobacter baumannii: a systematic review and Meta-analysis. Microb. Drug Resist. 23, 982–993. doi: 10.1089/mdr.2016.0279
Ma, X., Fu, S., Wang, Y., Zhao, L., Yu, W., He, Y., et al. (2022). Proteomics study of the synergistic killing of Tigecycline in combination with aminoglycosides against Carbapenem-resistant Klebsiella pneumoniae. Front. Cell. Infect. Microbiol. 12, 1–11. doi: 10.3389/fcimb.2022.920761
Malta, R. C. R., Ramos, G. J. D. S., Nascimento, P. A., Nascimento, J., and dos, S. (2020). From food to hospital: we need to talk about Acinetobacter spp. Germs 10, 210–217. doi: 10.18683/germs.2020.1207
Meagher, A. K., Ambrose, P. G., Grasela, T. H., and Ellis-Grosse, E. J. (2005). The pharmacokinetic and pharmacodynamic profile of tigecycline. Clin. Infect. Dis. 41, S333–S340. doi: 10.1086/431674
Meyer, M., Freihofer, P., Scherman, M., Teague, J., Lenaerts, A., and Böttger, E. C. (2014). In vivo efficacy of apramycin in murine infection models. Antimicrob. Agents Chemother. 58, 6938–6941. doi: 10.1128/AAC.03239-14
Moland, E. S., Craft, D. W., Hong, S. G., Kim, S. Y., Hachmeister, L., Sayed, S. D., et al. (2008). In vitro activity of tigecycline against multidrug-resistant Acinetobacter baumannii and selection of tigecycline-amikacin synergy. Antimicrob. Agents Chemother. 52, 2940–2942. doi: 10.1128/AAC.01581-07
Nasr, P. (2020). Genetics, epidemiology, and clinical manifestations of multidrug-resistant Acinetobacter baumannii. J. Hosp. Infect. 104, 4–11. doi: 10.1016/j.jhin.2019.09.021
Nulsopapon, P., Nasomsong, W., Pongchaidecha, M., Changpradub, D., Juntanawiwat, P., and Santimaleeworagun, W. (2021). The synergistic activity and optimizing doses of Tigecycline in combination with aminoglycosides against clinical Carbapenem-resistant Klebsiella pneumoniae isolates. Antibiotics 10:736. doi: 10.3390/antibiotics10060736
Rubinstein, E., and Vaughan, D. (2005). Tigecycline. Drugs 65, 1317–1336. doi: 10.2165/00003495-200565100-00002
Sader, H. S., Jones, R. N., Dowzicky, M. J., and Fritsche, T. R. (2005). Antimicrobial activity of tigecycline tested against nosocomial bacterial pathogens from patients hospitalized in the intensive care unit. Diagn. Microbiol. Infect. Dis. 52, 203–208. doi: 10.1016/j.diagmicrobio.2005.05.002
Shakil, S., Khan, R., Zarrilli, R., and Khan, A. U. (2008). Aminoglycosides versus bacteria – a description of the action, resistance mechanism, and nosocomial battleground. J. Biomed. Sci. 15, 5–14. doi: 10.1007/s11373-007-9194-y
Sun, J., Chen, C., Cui, C. Y., Zhang, Y., Liu, X., Cui, Z. H., et al. (2019). Plasmid-encoded tet(X) genes that confer high-level tigecycline resistance in Escherichia coli. Nat. Microbiol. 4, 1457–1464. doi: 10.1038/s41564-019-0496-4
Tamma, P. D., Cosgrove, S. E., and Maragakis, L. L. (2012). Combination therapy for treatment of infections with gram-negative bacteria. Clin. Microbiol. Rev. 25, 450–470. doi: 10.1128/CMR.05041-11
Tang, B., Yang, H., Jia, X., and Feng, Y. (2021). Coexistence and characterization of Tet(X5) and NDM-3 in the MDR-Acinetobacter indicus of duck origin. Microb. Pathog. 150:104697. doi: 10.1016/j.micpath.2020.104697
Vidaillac, C., Benichou, L., and Duval, R. E. (2012). In vitro synergy of colistin combinations against colistin-resistant Acinetobacter baumannii, Pseudomonas aeruginosa, and Klebsiella pneumoniae isolates. Antimicrob. Agents Chemother. 56, 4856–4861. doi: 10.1128/AAC.05996-11
Wu, H., Feng, H., He, L., Zhang, H., and Xu, P. (2021). In vitro activities of Tigecycline in combination with amikacin or Colistin against Carbapenem-resistant Acinetobacter baumannii. Appl. Biochem. Biotechnol. 193, 3867–3876. doi: 10.1007/s12010-021-03664-z
Yu, Y., Fang, J. T., Zheng, M., Zhang, Q., Walsh, T. R., Liao, X. P., et al. (2018). Combination therapy strategies against multiple-resistant Streptococcus suis. Front. Pharmacol. 9, 1–10. doi: 10.3389/fphar.2018.00489
Yu, Y., Walsh, T. R., Yang, R. S., Zheng, M., Wei, M. C., Tyrrell, J. M., et al. (2018). Novel partners with colistin to increase its in vivo therapeutic effectiveness and prevent the occurrence of colistin resistance in NDM- and MCR-co-producing Escherichia coli in a murine infection model. J. Antimicrob. Chemother. 74, 87–95. doi: 10.1093/jac/dky413
Zhou, L., Feng, S., Sun, G., Tang, B., Zhu, X., Song, K., et al. (2019). Extensively drug-resistant gram-negative bacterial bloodstream infection in hematological disease. Infect. Drug Resist. 12, 481–491. doi: 10.2147/IDR.S191462
Zhou, Y., Liu, P., Dai, S., Sun, J., Liu, Y., and Liao, X. (2020). Activity of Tigecycline or Colistin in combination with zidovudine against Escherichia coli harboring tet(X) and mcr-1. ASM J. 65:1172. doi: 10.1128/aac.01172-20
Keywords: tet(X)-harboring Acinetobacter spp., tigecycline, apramycin, synergistic combination therapy, mouse thigh infection model
Citation: Liu J, Zheng S-L, Wu J-J, Zheng M, Cai D-T, Zhang Y, Sun J, Liao X-P and Yu Y (2024) Overcoming tet(X)-harboring tigecycline resistance: a study on the efficacy of tigecycline-apramycin combinations. Front. Microbiol. 15:1502558. doi: 10.3389/fmicb.2024.1502558
Edited by:
Amanda Claire Brown, Tarleton State University, United StatesReviewed by:
Damir Gavric, University of Novi Sad, SerbiaFengxia Yang, Ministry of Agriculture and Rural Affairs, China
Copyright © 2024 Liu, Zheng, Wu, Zheng, Cai, Zhang, Sun, Liao and Yu. This is an open-access article distributed under the terms of the Creative Commons Attribution License (CC BY). The use, distribution or reproduction in other forums is permitted, provided the original author(s) and the copyright owner(s) are credited and that the original publication in this journal is cited, in accordance with accepted academic practice. No use, distribution or reproduction is permitted which does not comply with these terms.
*Correspondence: Yang Yu, R3JhY2V5eXVAc2NhdS5lZHUuY24=