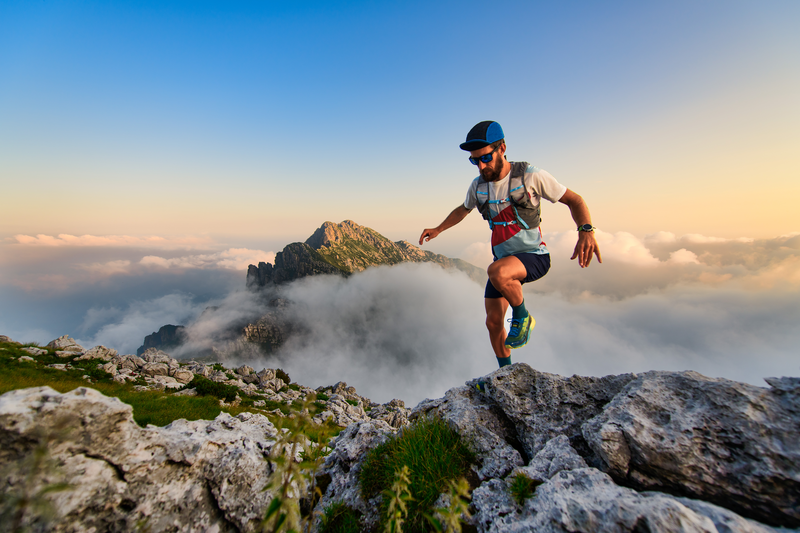
95% of researchers rate our articles as excellent or good
Learn more about the work of our research integrity team to safeguard the quality of each article we publish.
Find out more
ORIGINAL RESEARCH article
Front. Microbiol. , 20 November 2024
Sec. Microorganisms in Vertebrate Digestive Systems
Volume 15 - 2024 | https://doi.org/10.3389/fmicb.2024.1501211
This article is part of the Research Topic Harnessing Natural Plant Extracts and Probiotics to Enhance Host-Gut Microbiome Interactions View all 13 articles
Grape seed proanthocyanidin (GSP) is a kind of plant polyphenols with a wide variety of biological activities. In this study, we explored the effect of dietary GSP supplementation on growth performance, nutrient digestibility, and intestinal microbiota in growing pigs. A total of 180 growing pigs (30.37 ± 0.31 kg) were randomly assigned to five treatment groups, each consisting of six replicate pens with six pigs per pen. The pigs received either a basal diet (control) or a basal diet supplemented with GSP at 15, 30, 60, or 120 mg/kg. The trial lasted for 33 days, and blood and fecal samples were collected for biochemical measurements. GSP supplementation at a dose from 30 to 120 mg/kg decreased the ratio of feed intake to gain (F:G) (p < 0.05). GSP also increased the digestibility of dry matter, crude protein, ether extract, and gross energy (p < 0.05). GSP supplementation at 30 mg/kg increased the serum concentrations of immunoglobulin (Ig) A (p < 0.05). Interestingly, GSP supplementation at 60 mg/kg decreased the serum concentrations of urea and malondialdehyde (p < 0.05). However, the serum concentrations of glutathione peroxidase and total superoxide dismutase were significantly increased upon GSP supplementation (p < 0.05). Importantly, GSP supplementation at 120 mg/kg significantly increased the abundance of the phylum Firmicutes, but decreased the abundance of phylum Bacteroidetes and Epsilonbacteraeota in the feces (p < 0.05). Moreover, GSP supplementation significantly elevated the abundance of genus Lactobacillus, but decreased the abundance of genus Prevotellaceae NK3B31 (p < 0.05). Dietary GSP supplementation improves the growth performance and nutrient digestibility in growing pigs, which may be associated with enhancement of the antioxidative capacity, as well as improvement in gut microbiota. This study may promote the use of GSP in animal nutrition and the feed industry.
Oxidative stress is characterized by excessive production of reactive oxygen species (ROS) within the cell, which leads to disruption of the redox balance in the body (Bhor et al., 2004). Various factors contribute to oxidative stress in pigs, including weaning stress, mycotoxin contamination, social interactions, and the feeding environment (Novais et al., 2021; Zhao et al., 2013; Yang et al., 2020). Accumulating evidence showed that the modulation of the diet of pigs can also significantly reduce oxidative stress. For instance, dietary supplementation of probiotics during the weaning period was found to attenuate oxidative stress and improve the growth performance of piglets by improving the gut microbiota and inhibiting intestinal inflammation (Xiang et al., 2020). Moreover, dietary supplementation of an antioxidant compound can enhance feed conversion and increase oxidative defense in finishing pigs (Zhu et al., 2022).
Proanthocyanidin is a natural pigment that is abundant in numerous plant species and belongs to a kind of flavonoid compound. Grape seed proanthocyanidin (GSP), is extracted from grape seeds, which is a polymeric compound formed by the condensation of phenolic compounds with trihydroxyflavones. Their chemical structure comprises the core anthocyanin structure, wherein a benzene ring is attached to a tricyclic flavonoid scaffold, featuring hydroxyl and methoxy functional groups (Rodríguez et al., 2019). Prior research has demonstrated that GSP possesses a range of bioactive properties. For instance, GSP exhibits strong antioxidant properties, capable of scavenging free radicals and mitigating oxidative stress, thereby aiding in cellular protection against oxidative damage (Chedea et al., 2010). It also has anti-bacterial (Guo et al., 2020), anti-inflammatory (Tyagi et al., 2013), anti-viral (Ignea et al., 2013), immunomodulatory (Tong et al., 2011), etc. Recently, GSP has been utilized by a wide variety of animal species. For instance, GSP can improve the health status of tilapia and enhance its growth rate (Zhai et al., 2016). GSP has also been reported to enhance rumen fermentation function in cattle, thereby increasing nutrient digestibility (Ma et al., 2023). Moreover, GSP can protect the integrity of the goose intestinal barrier, increase the abundance and diversity of cecal microflora, and promote the growth of beneficial bacteria (Deng et al., 2023).
Although a number of studies revealed a prominent health-promoting effect of GSP, clear evidence establishing direct links among GSP dosage, product origin, developmental stage, and animal species is lacking. Furthermore, the biological events influenced by GSP are still not fully understood in terms of their underlying mechanisms. This study investigated the impact of dietary GSP supplementation on growth performance and intestinal microbiota in growing pigs. We found that GSP significantly improved growth performance in growing pigs, which may be associated with improvement in nutrient digestibility, antioxidative capacity, and intestinal microbiota. This study not only sheds light on the mechanisms underlying GSP’s effects on growth in pigs, but may also facilitate its application in animal nutrition and the feed industry.
The research underwent submission and approval by the Committee on Animal Care Advisory of Sichuan Agricultural University, with the authorization number SICAU-2021-007. The experiment procedures were conducted in accordance with the Guidelines for the Care and Use of Laboratory Animals.
A total of 180 pigs (Duroc × Landrace × Yorkshire) with an initial BW of 30.37 ± 0.66 kg (90 barrows and 90 gilts) were allocated to five treatments based on Randomized Complete Block Design. Each treatment group comprised six replicate pens (6 pigs per pen). For each treatment, three replicate pens were utilized to house the barrows and another three pens were utilized to house the gilts (pigs of the same gender were housed in a pen). The treatments were a control diet formulated to meet the requirements from NRC (2012) nutrient requirements (Table 1) with no addition of GSP, and the control diet with four increasing levels of GSP (15, 30, 60, and 120 mg/kg). The GSP was supplied by Fengpeng Biotechnology Co., Ltd. (Guilin, China) and contains 86.81% proanthocyanidin oligomers, 1.52% catechin, 2.41% epicatechin, and 0.98% proanthocyanidin B2, with a total proanthocyanidin purity of 96.58%. The feeders were refilled three times a day, and drinking water ad libitum throughout the experimental period (33 days). The feed intake was recorded every day. The final body weight, average daily feed intake (ADFI), average daily gain (ADG), and the ratio of feed intake to gain (F:G) were measured at the end of the experiment.
At the outset of the experiment, feed samples were gathered and preserved for subsequent analysis. During days 25–28 of the experiment, fecal samples were collected daily for four consecutive days from each replicate to assess nutrient digestibility. Hydrochloric acid at a concentration of 10% was added to the fecal samples for nitrogen fixation, and then the samples were dried in an oven at 60°C. The dried samples were then pulverized in a high-speed pulverizer and passed through 0.45 pm filters for chemical assay. Additionally, a portion of fecal samples was collected and stored at −80°C for 16S rDNA sequencing. Approximately 15 mL of blood was drawn from the anterior vena cava of one randomly selected pig from each of the six pens per group on day 33 of the experiment. The blood samples were then centrifuged at 3,000 × g for 20 min at 4°C to isolate the serum.
Dried and ground feed and fecal samples were utilized for nutrient digestibility analysis, employing acid-insoluble ash (AIA) as an endogenous indicator. The AIA in diet and fecal samples was measured as described by National National Standard (2009). The diet and fecal samples were evaluated for dry matter (DM), crude protein (CP) (Method 976.05; AOAC, 2007), and ether extract (EE) (Method 922.06; AOAC, 2007). An automated oxygen bomb calorimeter (Model 6,400, Parr, United States) was used to evaluate the gross energy (GE). The apparent total tract nutrient digestibility for all parameters was calculated using the following formula:
in which A1: is the AIA content of the diet, A2: is the AIA content of fecal, F1: is the nutrient content of the diet, F2: is the nutrient content of fecal. The diet was analyzed for calcium and total phosphorus content by inductively coupled plasma spectroscopy (method 985.01 A, B, and C; AOAC, 2007). The calculated nutrient levels of the diet were obtained from the China Feed Database (2020).
Serum biochemical parameters were measured using an Olympus automatic analyzer (Shanghai, China). Utilizing ELISA kits (MEIMIAN, Yancheng, Jiangsu, China), the concentrations of immunoglobulins (Ig) A (MM-090502), G (MM-040302), and M (MM-040202) were measured. Commercial kits from Nanjing Jiancheng Bioengineering Institute (Nanjing, Jiangsu, China) were utilized to measure the concentrations of total antioxidative capacity (T-AOC) (Cat. No. A015-1-2), glutathione peroxidase (GSH-Px) (Cat. No. A005-1-2), catalase (CAT) (Cat. No. A007-1-1), total superoxide dismutase (T-SOD) (Cat. No. A001-1-1), and malondialdehyde (MDA) (Cat. No. A003-1-2).
From 0.5 g of fecal samples, nucleic acids were extracted with the Stool DNA kit (TIANGEN, China) in accordance with the manufacturer’s guidelines. The analysis of bacterial community diversity and composition was conducted using the Quantitative Insights Into Microbial Ecology (QIIME) software package. The PCR amplification focused on the V4 region of the 16S rRNA gene, employing primers 515F (5’-GTGYCAGCMGCCGCGGTAA-3′) and 806R (5’-GGACTACHVGGGTWTCTAAT-3′). The PCR conditions were as follows: initial denaturation at 94°C for 1 min, followed by 1 cycle; denaturation at 94°C for 20 s, annealing at 54°C for 30 s, and extension at 72°C for 30 s for 25–30 cycles; final extension at 72°C for 5 min; held at 4°C. Amplification was carried out using a Verity Thermocycler (Applied Biosystems). PCR products were purified through electrophoresis on a 2% agarose gel. Desired bands were excised from qualified samples and purified using the Zymoclean Gel Recovery Kit (D4008), quantified with a Qubit 2.0 Fluorometer (Thermo Scientific), and mixed in equimolar amounts. Sequencing was conducted using the PE250 method with the Illumina NovaSeq 6,000 SP Reagent Kit V1.5.
Paired-end reads were merged using FLASH. Sequences were demultiplexed based on barcodes with SABRE, followed by barcode trimming, and quality control was performed using QIIME2. Within QIIME2, sequences were denoised and chimeras were removed using the Deblur algorithm, generating an ASV feature table and feature sequences. A 97% similarity threshold was used to define operational taxonomic units (OTUs). The analysis of community composition was performed in R, employing various data transformations and utilizing the ggplot2 package for visualization. The alpha diversity was analyzed in R, with PD index calculations performed using the Picante package, while other indices were calculated using the Vegan package. Bray–Curtis distance was computed using the vegdits function in Vegan, and PCoA analysis was conducted with the ape package.
Data were analyzed by IBM SPSS 27.0 software (Statistical Product and Service Solutions, Inc., United States). In the context of growth performance and nutrient digestibility, each pen is regarded as the experimental unit; however, for other experimental data, individual pigs are treated as the experimental unit. The growth performance data were analyzed utilizing Linear Mixed Model (LMM), considering dietary treatment and sex as fixed effects with an interaction term for dietary treatment × sex, while considering pen as a random effect. Other data were analyzed using a general linear model (GLM), with dietary treatment and sex as fixed effects with an interaction term for dietary treatment × sex. The effects of dietary treatment were evaluated using one-way ANOVA, followed by Duncan’s multiple comparison test to assess mean differences. Results are expressed as mean ± standard error of the mean (SEM), with statistical significance set at p < 0.05 and trends defined as 0.05 < p < 0.10.
As shown in Table 2, dietary GSP supplementation at a dose from 30 to 120 mg/kg significantly increased the ADG and decreased the F:G ratio of the pigs (p < 0.05). Moreover, with dietary GSP supplementation, the digestibility of dry matter, crude protein, ether extract, and gross energy increased quadratically (quadratic, p < 0.05).
As shown in Table 3, dietary GSP supplementation did not affect serum biochemical parameters such as the ALT, AST, CK, and TP (p > 0.05). Nevertheless, the serum concentration of urea decreased quadratically by GSP supplementation (quadratic, p < 0.05).
As shown in Table 4, GSP supplementation at 30 mg/kg significantly elevated serum IgA levels (p < 0.05). Additionally, serum MDA levels decreased quadratically with GSP supplementation (quadratic, p < 0.05), while serum levels of GSH-Px and T-SOD exhibited a quadratic increase (quadratic, p < 0.05).
Following OTU assignment and chimera checking, a total of 1,002,573 effective tags representing the 16S rRNA gene V4 region were selected from 1,027,009 tags, yielding an average of 33,419 sequences per fecal sample. The percentage of combined sequences for each sample ranged from 97.17 to 98.04% (Supplementary Table S1). The rarefaction curves indicated that the depth of sampling was adequate to assess the bacterial communities (Figure 1).
Figure 1. Rarefaction curves. Con, control group; GSPs15, 15 mg/kg GSP group; GSPs30, 30 mg/kg GSP group; GSPs60, 60 mg/kg GSP group; GSPs120, 120 mg/kg GSP group (n = 6).
The alpha diversity index is shown in Figure 2 and Supplementary Table S2. Dietary GSP supplementation has no significant differences in the alpha diversity index. Principal coordinate analysis (PCoA) based on Bray–Curtis distance metrics (Figure 3) indicated a distinct separation in microbiota composition between the 120 mg/kg GSP group and other treatment groups (p < 0.05).
Figure 2. The boxplot of differences on bacterial community diversity and richness. (a) Shannon index. (b) Simpson index. (c) Chao1 index. (d) ACE index. Con, control group; GSPs15, 15 mg/kg GSP group; GSPs30, 30 mg/kg GSP group; GSPs60, 60 mg/kg GSP group; GSPs120, 120 mg/kg GSP group (n = 6). *Means significant difference (p < 0.05) between groups.
Figure 3. The PCoA analysis based on Bray–Curtis distance. Con, control group; GSPs15, 15 mg/kg GSP group; GSPs30, 30 mg/kg GSP group; GSPs60, 60 mg/kg GSP group; GSPs120, 120 mg/kg GSP group (n = 6).
At the phylum level, all of the qualified sequences were assigned to 21 known phyla (Figure 4A and Supplementary Table S3). Among these predominant phyla, GSP supplementation at 120 mg/kg enhanced the abundance of Firmicutes compared to the CON group (p < 0.05), but decreased the abundance of the phylum Bacteroidetes in fecal samples (p < 0.05). At the genus level, the sequences derived from fecal samples were to 21 known genera (Figure 4B and Supplementary Table S4). GSP supplementation at 120 mg/kg increased the abundance of the Lactobacillus (p < 0.05). Moreover, GSP supplementation at 120 mg/kg decreased the Prevotellaceae NK3B31 group and Alloprevotella abundance at the genus level (p < 0.05). The heatmap displays the abundance of selected phyla and genera across samples, clearly highlighting significant differences in distribution between the treatment groups (Figure 5).
Figure 4. Bar graph shows the phylum (a) and genus (b) level composition of bacteria. Color coded bar plot shows the relative abundance of bacterial phyla and genus across the different samples. Con, control group; GSPs15, 15 mg/kg GSP group; GSPs30, 30 mg/kg GSP group; GSPs60, 60 mg/kg GSP group; GSPs120, 120 mg/kg GSP group (n = 6).
Figure 5. Heatmap distribution of OTUs at phylum (a) and genus (b) level. OTUs were arranged in rows and are clustered on the vertical axis. Samples are arranged vertically and are on the horizontal axis. Different colors indicate the relative abundance of taxons. Con, control group; GSPs15, 15 mg/kg GSP group; GSPs30, 30 mg/kg GSP group; GSPs60, 60 mg/kg GSP group; GSPs120, 120 mg/kg GSP group (n = 6).
Analysis using Spearman correlation (Figure 6) demonstrated significant links between the top 30 genera of intestinal microbiota and essential metrics such as growth performance, nutrient digestibility, and antioxidant capacity in growing pigs. A notable negative correlation was observed between average ADG and the relative abundance of Alloprevotella (p < 0.05). Additionally, dry matter digestibility showed an inverse relationship with the relative abundances of Clostridium sensu stricto 1, the Prevotellaceae NK3B31 group, and Ruminococcus 1 (p < 0.05). Moreover, the digestibility of GE was negatively associated with the relative abundance of Clostridium sensu stricto 1 (p < 0.05). A negative correlation was found between the digestibility of CP and the relative abundances of Clostridium sensu stricto 1, Prevotella 1, and Ruminococcus 1 (p < 0.05). Negative associations were observed between the digestibility of EE and the relative abundances of Clostridium sensu stricto 1 and Campylobacter (p < 0.05). Furthermore, serum MDA levels were negatively correlated with the Ruminococcaceae NK4A214 group (p < 0.05). A positive correlation was identified between serum GSH-Px levels and the relative abundances of Streptococcus, Ruminococcaceae UCG-014, Oscillospira, Eubacterium coprostanoligenes group, and Prevotellaceae UCG-003 (p < 0.05). Moreover, the serum T-SOD concentration was negatively correlated with the relative abundance of Alloprevotella, but was positively correlated with the relative abundances of Selenomonas and Ruminococcaceae NK4A214 group (p < 0.05).
Figure 6. The Spearman correlation analysis of fecal microbial composition with growth performance, nutrient digestibility, and antioxidant capacity in growing pigs. Spearman correlation coefficients of ADG, F/G, nutrient digestibility (DM, GE, CP, and EE), serum MDA concentration, serum GSH-Px concentration, and serum T-SOD concentration with fecal microbiota at genus level, are displayed. The heatmap with red indicated a positive correlation, while blue represented a negative correlation. ADG, average daily gain; F/G, feed to gain ratio; DM, dry matter; GE, gross energy; CP, crude protein; EE, ether extract. *p < 0.05, **p < 0.01.
In the present study, we explored the effect of dietary GSP supplementation on growth performance and nutrient digestibility in growing pigs. We found that GSP supplementation significantly increased the feed efficiency, as indicated by the decrease in the ratio of F:G and the increase in the digestibility of DM, CP, EE, and GE. This finding aligns with earlier research conducted on piglets and growing pigs (Fiesel et al., 2014; Park et al., 2014). Improved feed efficiency may be linked to enhanced immunity and antioxidative capacity resulting from GSP supplementation (Chedea et al., 2010; Hao et al., 2021; Tong et al., 2011). In this study, serum concentrations of IgA were significantly elevated by dietary supplementation of GSP at 30 mg/kg, which predominantly exists on mucosal surfaces, such as those of the respiratory and digestive tracts, where it serves as a crucial first line of defense by preventing pathogen adhesion and penetration of the mucosa (Pabst, 2012). Previous study also indicated that polyphenolic compounds, such as magnolol, can enhance amino acid absorption and protein synthesis in growing pigs, thereby maintaining immune homeostasis (Liu et al., 2023).
Oxidative stress can induce acute inflammatory response in the intestine, leading to structural and functional disruptions in the intestinal epithelium and increasing intestinal permeability (Assimakopoulos et al., 2004; John et al., 2011). Moreover, oxidative stress can also lead to oxidative modifications of digestive enzymes, resulting in decreased enzyme activity, which impairs the digestion and absorption of nutrients (Bhor et al., 2004). In recent years, various antioxidants have been utilized to enhance the antioxidant capacity of pigs. For instance, certain plant extracts such as resveratrol, β-glucans, daidzein, etc., have been reported to scavenge excessive free radicals in the body, effectively alleviate intestinal oxidative damage, promote villus growth and increase intestinal wall thickness, thereby enhancing feed efficiency (Goh et al., 2023; Li et al., 2021; Meng et al., 2018). The GSP has potent antioxidant properties, as the multiple hydroxyl groups in its polyphenolic structure can effectively neutralize free radicals and reduce their activity through resonance stabilization (Spranger et al., 2008). By activating the Nrf2 signaling pathway and enhancing the expression of downstream antioxidant enzymes, GSP can also mitigate oxidative stress (Xu et al., 2019). In this study, the antioxidative capacity of the pigs was significantly enhanced by GSP supplementation, as indicated by the decrease in serum concentration of MDA. The MDA is one of the end products of lipid peroxidation and can indirectly reflect the extent of oxidative damage in the body (Marnett, 1999). Moreover, GSP supplementation also enhanced the concentrations of T-SOD and GSH-Px. GSH-Px is a selenium-containing enzyme that catalyzes the reduction of hydrogen peroxide and organic peroxides to water and the corresponding alcohol using reduced glutathione as a substrate, and the T-SOD is a type of metal-containing enzyme that is the first line of defense in the detoxification of oxidative stress products (Ceballos-Picot et al., 1996; Fattman et al., 2003). These findings align with earlier reports indicating that dietary GSP supplementation can enhance serum antioxidant indices in finishing pigs through the activation of the Nrf2 signaling pathway (Feng et al., 2023).
The intestinal microbiota, which comprises all microorganisms residing in the digestive tract, is crucial for the normal functioning of digestive, immune, metabolic, and nervous systems through its balance and diversity (Álvarez et al., 2021). The microbial communities in different sections of the digestive tract exhibit significant differences in composition, whereas fecal microbiota provides a comprehensive reflection of the entire gut microbiome (Eckburg et al., 2005). Earlier research indicated that GSP significantly impacts the modulation of intestinal microbiota dysbiosis (Jin et al., 2018; Seo et al., 2017; Tao et al., 2019). In this study, PCoA analysis revealed distinct microbial community structures and compositions in the group receiving 120 mg/kg GSP compared to the other groups. This result aligns with earlier studies conducted on both mice and finishing pigs (Choy et al., 2014; Sheng et al., 2020). This change may reflect competitive displacement among microbial populations under GSP treatment, resulting in a new microbial equilibrium, which could affect nutrient absorption, immune response, and overall gut function (Biagi et al., 2016; Hertz et al., 2020).
Changes in the fecal microbiota have been shown in a previous study to be closely associated with intestinal health (Hopkins, 2001). In the present study, the phyla Firmicutes and Bacteroidetes were found to be the most predominant phyla in the fecal samples, which is consistent with a previous study in pigs (Verschuren et al., 2019). The bacteria of phylum Firmicutes can efficiently degrade plant fibers and other complex carbohydrates, producing short-chain fatty acid (Sun et al., 2022). In this study, we found that supplementation with GSP at 120 mg/kg significantly enhanced the abundance of the phylum Firmicutes, deepening our understanding of how dietary GSP can improve the bioavailability of nutrients. In animals, a major phylum present in the intestine is Bacteroidetes, which is involved in food digestion, nutrient absorption, and immune system regulation, but its overgrowth can lead to immune system imbalance (Ferolla et al., 2014; Pittayanon et al., 2019). In this study, we observed that GSP supplementation at 120 mg/kg reduced the ratio of the phylum Bacteroidetes in feces. This finding is consistent with earlier research indicating that plant polyphenols can inhibit Bacteroidetes growth, thereby affecting the host’s energy metabolism in the intestine (Xue et al., 2016). Moreover, Firmicutes and Bacteroidetes are crucial members of the gut microbiota, and they may engage in resource competition (Ley et al., 2006). The substantial increase in members of Firmicutes such as Lactobacillus may occupy more ecological niches and nutrient resources, thereby restricting the growth and proliferation of Bacteroidetes (Chen M. L. et al., 2016; Chen S. et al., 2016; Wang et al., 2024).
The Lactobacillus genus is a group of Gram-positive bacteria that can regulate the intestinal environment by producing beneficial metabolic products such as lactic acid, maintaining the acid–base balance, and inhibiting the growth of harmful bacterial populations (Dempsey and Corr, 2022). In this study, fecal samples from pigs receiving a diet supplemented with 120 mg/kg GSP showed a higher abundance of Lactobacillus compared to the CON group. This finding aligns with results from a previous study on another polyphenolic compound, resveratrol, which was shown to increase the levels of Lactobacillus in the gut, ultimately contributing to the regulation of bile acid metabolism (Chen M. L. et al., 2016; Chen S. et al., 2016). The Prevotellaceae is one of the major bacterial families in the large intestine of pigs, due to its ability to promote the production of short-chain fatty acids, is suggested to play an important role in intestinal metabolism (Amat et al., 2020; Zhang et al., 2021). Nevertheless, certain members of the Prevotellaceae family have been found to potentially exert negative effects on health by promoting inflammatory responses and contributing to disease progression. For instance, the mucin-degrading activity of the Prevotellaceae NK3B31 group may compromise intestinal epithelial integrity, fostering endotoxemia, inflammation, and insulin resistance (Hasain et al., 2021). Furthermore, previous research indicated that Prevotella copri (a member of Alloprevotella) exacerbates DSS-induced colitis in mice by downregulating ATF4 expression and altering the composition of intestinal microbiota (Wang and Cao, 2024). In the present study, GSP supplementation at 120 mg/kg decrease the genera abundances of the Prevotellaceae NK3B31 group and Alloprevotella. A previous study found that pigs with a low feed conversion ratio had higher abundances of taxa from the Prevotellaceae family in the ileum compared to those with a high feed conversion ratio (Quan et al., 2018). Consistently, the Spearman correlation analysis showed that the digestibility of DM was negatively correlated with the genus abundance of the Prevotellaceae NK3B31 group. Additionally, the abundance of Alloprevotella was found to be negatively correlated with both ADG and T-SOD concentration. Consequently, the observed dynamic changes in the intestinal microbiota community may partially account for the improvements in growth performance and nutrient digestibility noted in this study. Further studies, including fecal microbiota transplantation, are necessary to clarify the precise role and potential mechanisms underlying the biological events modulated by the intestinal microbiota.
In summary, dietary GSP supplementation not only increases the growth performance, but also improves the nutrient digestibility of growing pigs. The improved nutrient digestibility by GSP is likely linked to the enhancements in antioxidative capacity and improvement in the intestinal microbiota. Based on our findings, dietary supplementation of 30 and 60 mg/kg GSP may be optimal for growing pigs. This study may enhance the application of GSP in animal nutrition and the feed industry.
The raw sequence data for the 16S rRNA presented in this study can be found in online repositories. The names of the repository/repositories and accession number(s) can be found at: NCBI, PRJNA1176302.
The animal study was approved by Committee on Animal Care Advisory of Sichuan Agricultural University. The study was conducted in accordance with the local legislation and institutional requirements.
YZ: Data curation, Investigation, Software, Validation, Visualization, Writing – original draft. YLi: Investigation, Methodology, Resources, Software, Visualization, Writing – review & editing. BY: Software, Visualization, Writing – review & editing. ZH: Supervision, Visualization, Writing – review & editing. YLu: Software, Visualization, Writing – review & editing. PZ: Formal analysis, Supervision, Writing – review & editing. XM: Supervision, Visualization, Writing – review & editing. JY: Software, Supervision, Writing – review & editing. HT: Data curation, Investigation, Supervision, Validation, Writing – review & editing. JL: Supervision, Visualization, Writing – review & editing. HY: Software, Visualization, Writing – review & editing. JH: Conceptualization, Funding acquisition, Project administration, Resources, Supervision, Writing – review & editing.
The author(s) declare that financial support was received for the research, authorship, and/or publication of this article. This study was supported by the National Key R&D Program of China (2023YFD1301200) and the Porcine Innovation Team of Sichuan Province (SCCXTD-2024-8).
The support that Huifen Wang and Fali Wu provided throughout the animal testing and the gathering of samples is something for which we gratefully express our appreciation.
Author HT was employed by the company Wens Foodstuff Group Co., Ltd.
The remaining authors declare that the research was conducted in the absence of any commercial or financial relationships that could be construed as a potential conflict of interest.
All claims expressed in this article are solely those of the authors and do not necessarily represent those of their affiliated organizations, or those of the publisher, the editors and the reviewers. Any product that may be evaluated in this article, or claim that may be made by its manufacturer, is not guaranteed or endorsed by the publisher.
The Supplementary material for this article can be found online at: https://www.frontiersin.org/articles/10.3389/fmicb.2024.1501211/full#supplementary-material
Álvarez, J., Fernández Real, J. M., Guarner, F., Gueimonde, M., Rodríguez, J. M., Saenz de Pipaon, M., et al. (2021). Gut microbes and health. Gastroenterol. Hepatol. 44, 519–535. doi: 10.1016/j.gastre.2021.01.002
Amat, S., Lantz, H., Munyaka, P. M., and Willing, B. P. (2020). Prevotella in pigs: the positive and negative associations with production and health. Microorganisms 8:1584. doi: 10.3390/microorganisms8101584
Assimakopoulos, S. F., Vagianos, C. E., Patsoukis, N., Georgiou, C., Nikolopoulou, V., and Scopa, C. D. (2004). Evidence for intestinal oxidative stress in obstructive jaundice-induced gut barrier dysfunction in rats. Acta Physiol. Scand. 180, 177–185. doi: 10.1046/j.0001-6772.2003.01229.x
Bhor, V. M., Raghuram, N., and Sivakami, S. (2004). Oxidative damage and altered antioxidant enzyme activities in the small intestine of streptozotocin-induced diabetic rats. Int. J. Biochem. Cell Biol. 36, 89–97. doi: 10.1016/s1357-2725(03)00142-0
Biagi, E., Franceschi, C., Rampelli, S., Severgnini, M., Ostan, R., Turroni, S., et al. (2016). Gut microbiota and extreme longevity. Curr. Biol. 26, 1480–1485. doi: 10.1016/j.cub.2016.04.016
Ceballos-Picot, I., Witko-Sarsat, V., Merad-Boudia, M., Nguyen, A. T., Thévenin, M., Jaudon, M. C., et al. (1996). Glutathione antioxidant system as a marker of oxidative stress in chronic renal failure. Free Radic. Biol. Med. 21, 845–853. doi: 10.1016/0891-5849(96)00233-x
Chedea, V. S., Braicu, C., and Socaciu, C. (2010). Antioxidant/prooxidant activity of a polyphenolic grape seed extract. Food Chem. 121, 132–139. doi: 10.1016/j.foodchem.2009.12.020
Chen, S., Cheng, H., Wyckoff, K. N., and He, Q. (2016). Linkages of Firmicutes and Bacteroidetes populations to methanogenic process performance. J. Ind. Microbiol. Biotechnol. 43, 771–781. doi: 10.1007/s10295-016-1760-8
Chen, M. L., Yi, L., Zhang, Y., Zhou, X., Ran, L., Yang, J., et al. (2016). Resveratrol attenuates trimethylamine-N-oxide (TMAO)-induced atherosclerosis by regulating TMAO synthesis and bile acid metabolism via remodeling of the gut microbiota. MBio 7, 2210–2215. doi: 10.1128/mBio.02210-15
China Feed Database. (2020). Tables of feed composition and nutritive values in China. Beijing, China: Institute of Feed Research, Chinese Academy of Agricultural Sciences.
Choy, Y. Y., Quifer-Rada, P., Holstege, D. M., Frese, S. A., Calvert, C. C., Mills, D. A., et al. (2014). Phenolic metabolites and substantial microbiome changes in pig feces by ingesting grape seed proanthocyanidins. Food Funct. 5, 2298–2308. doi: 10.1039/c4fo00325j
Dempsey, E., and Corr, S. C. (2022). Lactobacillus spp. for gastrointestinal health: current and future perspectives. Front. Immunol. 13:840245. doi: 10.3389/fimmu.2022.840245
Deng, C., Zhai, Y., Yang, X., Chen, Z., Li, Q., and Hao, R. (2023). Effects of grape seed procyanidins on antioxidant function, barrier function, microbial community, and metabolites of cecum in geese. Poult. Sci. 102:102878. doi: 10.1016/j.psj.2023.102878
Eckburg, P. B., Bik, E. M., Bernstein, C. N., Purdom, E., Dethlefsen, L., Sargent, M., et al. (2005). Diversity of the human intestinal microbial flora. Science 308, 1635–1638. doi: 10.1126/science.1110591
Fattman, C. L., Schaefer, L. M., and Oury, T. D. (2003). Extracellular superoxide dismutase in biology and medicine. Free Radic. Biol. Med. 35, 236–256. doi: 10.1016/s0891-5849(03)00275-2
Feng, Y., Chen, X., Chen, D., He, J., Zheng, P., Luo, Y., et al. (2023). Dietary grape seed proanthocyanidin extract supplementation improves antioxidant capacity and lipid metabolism in finishing pigs. Anim. Biotechnol. 34, 1–11. doi: 10.1080/10495398.2023.2252012
Ferolla, S., Armiliato, G., Couto, C., and Ferrari, T. (2014). The role of intestinal bacteria overgrowth in obesity-related non-alcoholic fatty liver disease. Nutrients 6, 5583–5599. doi: 10.3390/nu6125583
Fiesel, A., Gessner, D. K., Most, E., and Eder, K. (2014). Effects of dietary polyphenol-rich plant products from grape or hop on pro-inflammatory gene expression in the intestine, nutrient digestibility and faecal microbiota of weaned pigs. BMC Vet. Res. 10:196. doi: 10.1186/s12917-014-0196-5
Goh, T. W., Kim, H. J., Moon, K., and Kim, Y. Y. (2023). Effects of beta-glucan with vitamin E supplementation on the growth performance, blood profiles, immune response, pork quality, pork flavor, and economic benefit in growing and finishing pigs. Anim Biosci 36, 929–942. doi: 10.5713/ab.22.0433
Guo, L., Yang, Z.-Y., Tang, R.-C., and Yuan, H.-B. (2020). Grape seed proanthocyanidins: novel coloring, flame-retardant, and antibacterial agents for silk. ACS Sustain. Chem. Eng. 8, 5966–5974. doi: 10.1021/acssuschemeng.0c00367
Hao, Y., Xing, M., and Gu, X. (2021). Research progress on oxidative stress and its nutritional regulation strategies in pigs. Animals 11:1384. doi: 10.3390/ani11051384
Hasain, Z., Raja Ali, R. A., Abdul Razak, S., Azizan, K. A., El-Omar, E., Razalli, N. H., et al. (2021). Gut microbiota signature among asian post-gestational diabetes women linked to macronutrient intakes and metabolic phenotypes. Front. Microbiol. 12:680622. doi: 10.3389/fmicb.2021.680622
Hertz, F. B., Budding, A. E., van der Lugt-Degen, M., Savelkoul, P. H., Løbner-Olesen, A., and Frimodt-Møller, N. (2020). Effects of antibiotics on the intestinal microbiota of mice. Antibiotics 9:191. doi: 10.3390/antibiotics9040191
Hopkins, M. J. (2001). Age and disease related changes in intestinal bacterial populations assessed by cell culture, 16S rRNA abundance, and community cellular fatty acid profiles. Gut 48, 198–205. doi: 10.1136/gut.48.2.198
Ignea, C., Dorobanţu, C. M., Mintoff, C. P., Branza-Nichita, N., Ladomery, M. R., Kefalas, P., et al. (2013). Modulation of the antioxidant/pro-oxidant balance, cytotoxicity and antiviral actions of grape seed extracts. Food Chem. 141, 3967–3976. doi: 10.1016/j.foodchem.2013.06.094
Jin, G., Asou, Y., Ishiyama, K., Okawa, A., Kanno, T., and Niwano, Y. (2018). Proanthocyanidin-rich grape seed extract modulates intestinal microbiota in ovariectomized mice. J. Food Sci. 83, 1149–1152. doi: 10.1111/1750-3841.14098
John, L. J., Fromm, M., and Schulzke, J.-D. (2011). Epithelial barriers in intestinal inflammation. Antioxid. Redox Signal. 15, 1255–1270. doi: 10.1089/ars.2011.3892
Ley, R. E., Turnbaugh, P. J., Klein, S., and Gordon, J. I. (2006). Microbial ecology: human gut microbes associated with obesity. Nature 444, 1022–1023. doi: 10.1038/4441022a
Li, Y., He, G., Chen, D., Yu, B., Yu, J., Zheng, P., et al. (2021). Supplementing daidzein in diets improves the reproductive performance, endocrine hormones and antioxidant capacity of multiparous sows. Anim Nutr. 7, 1052–1060. doi: 10.1016/j.aninu.2021.09.002
Liu, Y., Li, Y., Yu, M., Tian, Z., Deng, J., Ma, X., et al. (2023). Magnolol supplementation alters serum parameters, immune homeostasis, amino acid profiles, and gene expression of amino acid transporters in growing pigs. Int. J. Mol. Sci. 24:13952. doi: 10.3390/ijms241813952
Ma, J., Fan, X., Zhang, W., Zhou, G., Yin, F., Zhao, Z., et al. (2023). Grape seed extract as a feed additive improves the growth performance, ruminal fermentation and immunity of weaned beef calves. Animals 13:1876. doi: 10.3390/ani13111876
Marnett, L. J. (1999). Lipid peroxidation—DNA damage by malondialdehyde. Mutat Res 424, 83–95. doi: 10.1016/s0027-5107(99)00010-x
Meng, Q., Guo, T., Li, G., Sun, S., He, S., Cheng, B., et al. (2018). Dietary resveratrol improves antioxidant status of sows and piglets and regulates antioxidant gene expression in placenta by Keap 1-Nrf2 pathway and Sirt1. J Anim Sci Biotechnol. 9:34. doi: 10.1186/s40104-018-0248-y
National Standard. (2009). National Standard of the People’s republic of China animal feeding stuffs-determination of ash insoluble in hydrochloric acid. Beijing, China: Standardization Administration of China.
Novais, A. K., Deschene, K., Martel-Kennes, Y., Roy, C., Laforest, J. P., Lessard, M., et al. (2021). Weaning differentially affects mitochondrial function, oxidative stress, inflammation and apoptosis in normal and low birth weight piglets. PLoS One 16:e0247188. doi: 10.1371/journal.pone.0247188
Pabst, O. (2012). New concepts in the generation and functions of IgA. Nat. Rev. Immunol. 12, 821–832. doi: 10.1038/nri3322
Park, J. C., Lee, S. H., Hong, J. K., Cho, J. H., Kim, I. H., and Park, S. K. (2014). Effect of dietary supplementation of procyanidin on growth performance and immune response in pigs. Asian Australas. J. Anim. Sci. 27, 131–139. doi: 10.5713/ajas.2013.13359
Pittayanon, R., Lau, J. T., Yuan, Y., Leontiadis, G. I., Tse, F., Surette, M., et al. (2019). Gut microbiota in patients with irritable bowel syndrome—a systematic review. Gastroenterology 157, 97–108. doi: 10.1053/j.gastro.2019.03.049
Quan, J., Cai, G., Ye, J., Yang, M., Ding, R., Wang, X., et al. (2018). A global comparison of the microbiome compositions of three gut locations in commercial pigs with extreme feed conversion ratios. Sci. Rep. 8:4536. doi: 10.1038/s41598-018-22692-0
Rodríguez, P., García, V., Guerra, H., and Verardo, V. (2019). Grape seeds proanthocyanidins: an overview of in vivo bioactivity in animal models. Nutrients 11:2435. doi: 10.3390/nu11102435
Seo, K.-H., Kim, D.-H., Jeong, D., Yokoyama, W., and Kim, H. (2017). Chardonnay grape seed flour supplemented diets alter intestinal microbiota in diet-induced obese mice. J. Food Biochem. 41:2396. doi: 10.1111/jfbc.12396
Sheng, K., Zhang, G., Sun, M., He, S., Kong, X., Wang, J., et al. (2020). Grape seed proanthocyanidin extract ameliorates dextran sulfate sodium-induced colitis through intestinal barrier improvement, oxidative stress reduction, and inflammatory cytokines and gut microbiota modulation. Food Funct. 11, 7817–7829. doi: 10.1039/d0fo01418d
Spranger, I., Sun, B., Mateus, A. M., Freitas, V. D., and Ricardo-da-Silva, J. M. (2008). Chemical characterization and antioxidant activities of oligomeric and polymeric procyanidin fractions from grape seeds. Food Chem. 108, 519–532. doi: 10.1016/j.foodchem.2007.11.004
Sun, Y., Zhang, S., Nie, Q., He, H., Tan, H., Geng, F., et al. (2022). Gut firmicutes: relationship with dietary fiber and role in host homeostasis. Crit. Rev. Food Sci. Nutr. 63, 12073–12088. doi: 10.1080/10408398.2022.2098249
Tao, W., Zhang, Y., Shen, X., Cao, Y., Shi, J., Ye, X., et al. (2019). Rethinking the mechanism of the health benefits of proanthocyanidins: absorption, metabolism, and interaction with gut microbiota. Compreh Rev Food Sci Food Saf. 18, 971–985. doi: 10.1111/1541-4337.12444
Tong, H., Song, X., Sun, X., Sun, G., and Du, F. (2011). Immunomodulatory and antitumor activities of grape seed proanthocyanidins. J. Agric. Food Chem. 59, 11543–11547. doi: 10.1021/jf203170k
Tyagi, A., Raina, K., Gangar, S., Kaur, M., Agarwal, R., and Agarwal, C. (2013). Differential effect of grape seed extract against human non-small-cell lung cancer cells: the role of reactive oxygen species and apoptosis induction. Nutr. Cancer 65, 44–53. doi: 10.1080/01635581.2013.785003
Verschuren, L. M. G., Schokker, D., Bergsma, R., Jansman, A. J. M., Molist, F., and Calus, M. P. L. (2019). Prediction of nutrient digestibility in grower-finisher pigs based on faecal microbiota composition. J. Anim. Breed. Genet. 137, 23–35. doi: 10.1111/jbg.12433
Wang, X., and Cao, H. (2024). P090 Prevotella copri promotes colitis in mice by reducing expression of ATF4 and disturbing the gut microbiota. J. Crohns Colitis 18:i368. doi: 10.1093/ecco-jcc/jjad212.0220
Wang, S., Mu, L., Yu, C., He, Y., Hu, X., Jiao, Y., et al. (2024). Microbial collaborations and conflicts: unraveling interactions in the gut ecosystem. Gut Microbes 16:2296603. doi: 10.1080/19490976.2023.2296603
Xiang, Q., Wu, X., Pan, Y., Wang, L., Cui, C., Guo, Y., et al. (2020). Early-life intervention using fecal microbiota combined with probiotics promotes gut microbiota maturation, regulates immune system development, and alleviates weaning stress in piglets. Int. J. Mol. Sci. 21:503. doi: 10.3390/ijms21020503
Xu, M., Niu, Q., Hu, Y., Feng, G., Wang, H., and Li, S. (2019). Proanthocyanidins antagonize arsenic-induced oxidative damage and promote arsenic methylation through activation of the Nrf2 signaling pathway. Oxidative Med. Cell. Longev. 2019, 1–19. doi: 10.1155/2019/8549035
Xue, B., Xie, J., Huang, J., Chen, L., Gao, L., Ou, S., et al. (2016). Plant polyphenols alter a pathway of energy metabolism by inhibiting fecal Bacteroidetes and Firmicutes in vitro. Food Funct. 7, 1501–1507. doi: 10.1039/c5fo01438g
Yang, C., Song, G., and Lim, W. (2020). Effects of mycotoxin-contaminated feed on farm animals. J. Hazard. Mater. 389:122087. doi: 10.1016/j.jhazmat.2020.122087
Zhai, S.-W., Lu, J.-J., and Chen, X.-H. (2016). Effects of dietary grape seed proanthocyanidins on growth performance, some serum biochemical parameters and body composition of tilapia (Oreochromis niloticus) fingerlings. Ital. J. Anim. Sci. 13:3357. doi: 10.4081/ijas.2014.3357
Zhang, Y., Yin, C., Schroyen, M., Everaert, N., Ma, T., and Zhang, H. (2021). Effects of the inclusion of fermented mulberry leaves and branches in the gestational diet on the performance and gut microbiota of sows and their offspring. Microorganisms 9:604. doi: 10.3390/microorganisms9030604
Zhao, Y., Flowers, W. L., Saraiva, A., Yeum, K. J., and Kim, S. W. (2013). Effect of social ranks and gestation housing systems on oxidative stress status, reproductive performance, and immune status of sows. J. Anim. Sci. 91, 5848–5858. doi: 10.2527/jas.2013-6388
Zhu, C., Yang, J., Nie, X., Wu, Q., Wang, L., and Jiang, Z. (2022). Influences of dietary vitamin E, selenium-enriched yeast, and soy isoflavone supplementation on growth performance, antioxidant capacity, carcass traits, meat quality and gut microbiota in finishing pigs. Antioxidants 11:510. doi: 10.3390/antiox11081510
Keywords: GSP, growth performance, nutrient digestibility, antioxidant capacity, intestinal microbiota, growing pigs
Citation: Zheng Y, Li Y, Yu B, Huang Z, Luo Y, Zheng P, Mao X, Yu J, Tan H, Luo J, Yan H and He J (2024) Grape seed proanthocyanidins improves growth performance, antioxidative capacity, and intestinal microbiota in growing pigs. Front. Microbiol. 15:1501211. doi: 10.3389/fmicb.2024.1501211
Received: 24 September 2024; Accepted: 29 October 2024;
Published: 20 November 2024.
Edited by:
Kang Xu, Chinese Academy of Sciences (CAS), ChinaReviewed by:
Yu Bai, Tianjin University of Science and Technology, ChinaCopyright © 2024 Zheng, Li, Yu, Huang, Luo, Zheng, Mao, Yu, Tan, Luo, Yan and He. This is an open-access article distributed under the terms of the Creative Commons Attribution License (CC BY). The use, distribution or reproduction in other forums is permitted, provided the original author(s) and the copyright owner(s) are credited and that the original publication in this journal is cited, in accordance with accepted academic practice. No use, distribution or reproduction is permitted which does not comply with these terms.
*Correspondence: Jun He, aGVqdW44MDY3QDE2My5jb20=
†These authors have contributed equally to this work and share first authorship
Disclaimer: All claims expressed in this article are solely those of the authors and do not necessarily represent those of their affiliated organizations, or those of the publisher, the editors and the reviewers. Any product that may be evaluated in this article or claim that may be made by its manufacturer is not guaranteed or endorsed by the publisher.
Research integrity at Frontiers
Learn more about the work of our research integrity team to safeguard the quality of each article we publish.