- 1The Collaboration Unit for State Key Laboratory of Infectious Disease Prevention and Control, Jiangxi Provincial Health Commission Key Laboratory of Pathogenic Diagnosis and Genomics of Emerging Infectious Diseases, Nanchang Center for Disease Control and Prevention, Nanchang, Jiangxi, China
- 2Department of Infectious Diseases, The First Affiliated Hospital of Nanchang University, Nanchang, Jiangxi, China
- 3Nanchang Police Dog Base of the Ministry of Public Security, Nanchang, Jiangxi, China
- 4Center for Disease Control and Prevention of Donghu District, Nanchang, Jiangxi, China
- 5NHC Key Laboratory of Tropical Disease Control, School of Tropical Medicine, Hainan Medical University, Haikou, Hainan, China
Introduction: Severe fever with thrombocytopenia syndrome (SFTS) is an emerging infectious disease discovered in China in 2009. SFTS monitoring has been carried out since 2010 in mainland China. In recent years, human infection with SFTS virus (SFTSV) has frequently been detected in Jiujiang of Jiangxi Province, Central China.
Methods: Sera of SFTS surveillance cases and samples collected from humans, animals and ticks surrounding the cases were used to detect SFTSV RNA by real-time RT-PCR. SFTSV-positive samples were further subjected to sequencing and analysis of the S, M, and L segments of SFTSV.
Results: Four patients were positive for SFTSV infection. However, the subjects like humans, animals and ticks around the cases were all detected as negative for the virus infection. Phylogenetic analysis of the partial segments revealed that SFTSVs in three patients from Jiujiang were clustered with genotype C4 and C5 on the L and M segment-established phylogenetic tree; however, phylogenetic analysis of the S segment showed that the strains were grouped into genotype J1. These results suggested that the S, M, and L segments of these strains underwent segmental reassortment, which was later supported by recombination signal detection. The reassortment event was detected by at least four methods with a significance level of p value <0.05. In addition, the RDP recombination consensus score (RDPRCS) was greater than 0.40. To avoid poor tree topology support with the partial S, M, and L segments, we further performed analysis of the complete genome of SFTSV. The full-length L, M, and S segment sequences of the strains were consistently clustered into two genotypes, namely the genotype C5 and the genotype C4. The strains belonging to genotype C5 were detected for recombination signal with moderate confidence by all the six methods, with a significance level of p value <0.05 and an RDPRCS of 0.41. The recombination event might have occurred between the minor parent (2011YPQ11, C3/C3/C3) and major parent (SPL053A, J1/ J1/ J1). However, there was no genetic recombination detected in the strains belonging to genotype C4. The event was detected by only two methods with a significance level of p value <0.05.
Discussion: Two genotypes of SFTSVs were identified in Jiujiang City, Jiangxi Province of Central China, and they were genotype C5 and genotype C4. The genotype C5 underwent genetic recombination in this region, with the minor parent of the strain 2011YPQ11 and the major parent of the strain SPL053A.
1 Background
Severe fever with thrombocytopenia syndrome (SFTS) is an acute tick-borne infectious disease with a high case mortality risk, ranging from 6.18 to 27% (Casel et al., 2021). The disease is caused by the SFTS virus (SFTSV), a novel Bunyavirus. In China, SFTS has been endemic in many locations since the first case was reported in 2009 (Yu et al., 2011), and the majority of cases were clustered with four geographical areas: the Changbai Mountain area (Cluster I), the Jiaodong Peninsula (Cluster II), the Taishan Mountain area (Cluster III), and the Huaiyangshan Mountain area (Cluster IV) (Miao et al., 2021). So far, the annual number of cases has been continually increasing, which raises significant public health awareness in China (Li et al., 2022). In recent years, patients with SFTSV infection have also been increasingly found in the Mufu mountains of Jiujiang City, Jiangxi Province. However, infections of SFTSV in Jiangxi Province have not been well investigated until now; furthermore, characterization of molecular features of SFTSV in Jiangxi remains elusive. Here, we phylogenetically analyzed the genomes of SFTSV strains from Jiangxi in combination with other strains from China and Japan available in GenBank. The results suggested potential recombinants of SFTSV appearing in Jiangxi strains.
2 Materials and methods
2.1 Sample collection from patients and ticks, animals and individuals in the affected areas at a high risk
Patients who were clinically diagnosed with symptoms as described previously (Hu et al., 2018), were considered suspected SFTS cases and thus were recruited in this study. Ten milliliter of blood samples were collected at the acute phase of suspected SFTS cases by the local CDC or hospital.
Ticks were directly plucked from infested animals or collected by flagging vegetation in the affected areas where patients were found (Figure 1). All collected ticks were maintained alive in cases at room temperature before the study. Tick species were identified morphologically (Chen and Yang, 2021) and confirmed by a molecular method (Lv et al., 2014).
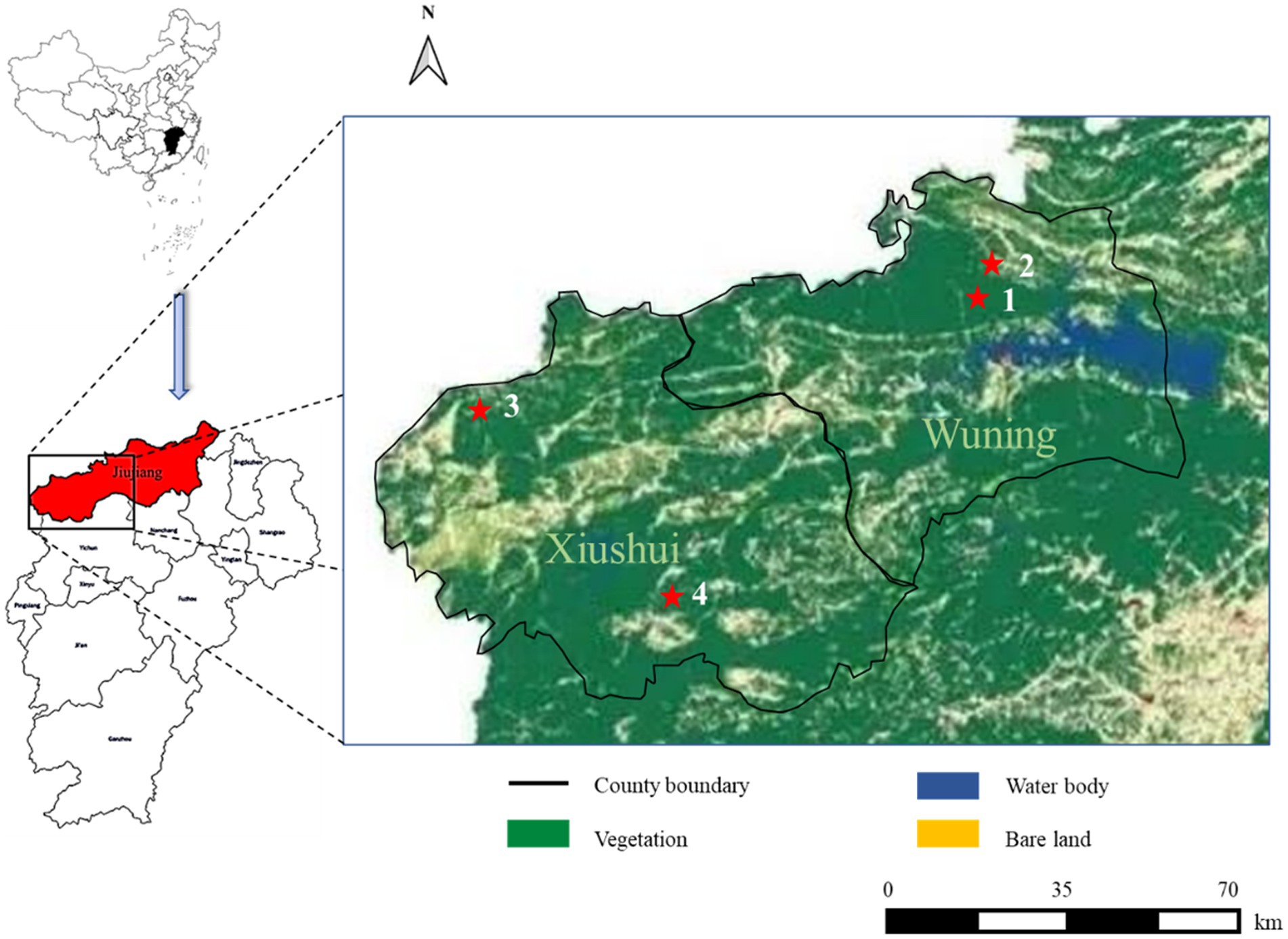
Figure 1. Severe fever with thrombocytopenia syndrome (SFTS) case distribution from this study in different land covers of Wuning and Xiushui County in Jiujiang City of Jiangxi Province from China. SFTS cases are shown with red filled pentagrams.
Approximately 1–2 mL of blood samples of animals were collected from jugular veins depending on body size and serum was obtained after blood clotting, then stored in boxes with ice packs and transported to the laboratory along with the ticks where all were stored at −80°C until further processing. Serum samples of individuals at a high risk were collected from close contacts and the surrounding population when the patients were epidemiologically, molecularly, and clinically confirmed.
2.2 Total nucleic acid extraction
The tick pools of each sample location with the same species at the same stage were homogenized in a Tissuelyzer II (Qiagen, Germany) at 4°C and centrifuged at 10,000 × g at 4°C for 10 min. Fifty microliter aliquots of each supernatant were transferred and pooled together in fresh tubes. Twenty microliter of each human or animal serum were pooled. All supernatants and pooled sera were sterilized by filtration through 0.22-μm syringe filters, digested with 5 μL DNase at 20–30°C for 15 min, and immediately subjected to total nucleic acid automatic extraction in a QIAcube (Qiagen, Germany) using the RNeasy mini kit (Qiagen, Germany).
2.3 Quantitative real-time PCR (qRT-PCR)
Total RNA was extracted from the sera of patients, individuals at high risk, animals, and ticks using the QIAamp Viral RNA Mini Kit (Qiagen, Germany) according to the manufacturer’s instructions. The eluate (5 μL) was tested for SFTSV RNA using a commercial kit (Shuoshi Biotech., Jiangsu, China). The cutoff cycle-threshold (Ct) value for positive samples was set as 37 cycles.
2.4 Sequencing and analysis of the S, M, L segments of SFTSV
The SFTSV RNA was extracted from different samples with the QIAamp Viral RNA Mini Kit (Qiagen, Germany). The nucleic acids were reverse transcribed with the Reverse-Transcription Kit (TaKaRa, Dalian, China). To detect SFTSV RNA, we performed nested PCRs with a kit (Toyobo, Japan) and the amplified primers were listed in Table 1. Fragments covering the full-length L, M, and S segments of the SFTSV genome were amplified using walking primers designed based on the full-genome sequence of the detected SFTSV strain (Table 2). All PCR products were examined by electrophoresis on 1.5% agarose gels and the products with the targeted band were sent to a company for sequencing (Sangon, Shanghai, China).
Sequences generated from this study and retrieved from GenBank were divided into the partial L, M, and S datasets, and the complete L, M, and S datasets for respective phylogenetic analysis. Comparative sequence analyses, including sequence alignments and best-fit substitution model selection, were performed using the ClustalW program of the Molecular Evolutionary Genetic Analysis (MEGA) software (version 7.0) (Tamura et al., 2013). Phylogenetic trees were constructed using the Maximum Likelihood method with a Kimura two-parameter model for analysis of the partial S, M, L, and the complete S segments of SFTSV. The Maximum Likelihood method with General Time Reversible model was employed for analysis of the complete M and L segments of SFTSV, which were provided in the MEGA software (version 7.0), and branch support was calculated based on 500 bootstrap replicates (Kimura, 1980; Saitou and Nei, 1987). Non-uniformity of evolutionary rates among sites were modeled using a discrete Gamma distribution (+G) with 5 rate categories.
2.5 Detecting recombination and reassortment events in SFTSV strains
Recombination and reassortment events were detected using the RDP software package, which integrates six recombination detection methods, including GENECONV, BootScan, MaxChi, Chimaera, SiScan, and 3Seq (Martin et al., 2015). To identify reassortment occurrences, the partial sequences of the L, M, and S segments from SFTSV strains in Supplementary Dataset 1 were sequentially concatenated and subsequently analyzed using the RDP software package. A reassortment event was characterized as a recombination breakpoint detected at the ends of segments (Nagarajan and Kingsford, 2011). For a recombination event to be confirmed, it had to fulfill two criteria outlined in the RDP manual: (i) the event must be validated by at least two methods with a significance level of p-value <0.05; and (ii) the RDP recombination consensus score (RDPRCS) must exceed 0.60. If an event met the first criterion but fell within the RDPRCS range of 0.4–0.6, it was considered a probable recombination; otherwise, it was disregarded. For recombination detection in the complete genome of SFTSV, the full-length L, M, and S segments from SFTSV strains stored in Supplementary Dataset 2 were sequentially concatenated and subsequently analyzed using the same methods as mentioned above.
2.6 GenBank data contribution
In the present study, 12 sequences were obtained and deposited in GenBank under accession numbers OR035637-OR035639, OR557464-OR557466, OR574960-OR574965, and OR915871-OR915873. We named the SFTSV strain from patient 1 SFTSV strain JX22-1 and successfully generated partial S segment (OR035639), M segment (OR035637), and L segment (OR035638). We termed the SFTSV strain from patient 2 SFTSV strain JX23WN and successfully generated partial S segment (OR557464), M segment (OR557465), and L segment (OR557466), and complete S segment (OR574963), M segment (OR574964), and L segment (OR574965). Patient 3 was infected by the SFTSV strain JX23XSH, and we successfully generated partial S segment (OR574960), M segment (OR574961), and L segment (OR574962), and complete S segment (OR915871), M segment (OR915872), and L segment (OR915873) from this strain.
3 Results
3.1 SFTSV detection in patients, humans living around the patients, and ticks and animals
A total of four patients were enrolled in this study, and their clinical symptoms were provided in Supplementary Table S1. From three of them, we successfully generated partial and/or complete segment sequences of SFTSV. From June 26 to 28, 2022, and from July 12 to 14, 2023, a total of 56 humans who live and work around the patients’ home environment were tested for SFTSV infection. Among these individuals, 43 reported tick bites and nine had multiple tick bites. The PCR results for SFTSV infection in all individuals tested were negative. A total of 91 ticks, 18 domestic animals (12 dogs, five goats, and one cat), and seven rodents found in the patients’ home environment were also sampled. Ticks were composed of three Haemaphysalis longicornis, seven Haemaphysalis hystricis and one Ixodes granulatus ticks. Eighty host-attached ticks were collected with tweezers and consisted of 45 H. longicornis, 32 H. hystricis, two Haemaphysalis kitaokai and one unidentified Haemaphysalis tick. Among all the collected ticks, only four were nymphs and the remaining were adults. SFTSV RNA was not detected in any of ticks and animals tested.
3.2 Genetic analysis of SFTSV in three patients
Previous studies indicated that SFTSVs were clustered into the Japanese clade (including J1, J2, and J3 genotypes) and the Chinese clade (including C1, C2, C3, and C4 genotypes) (Shi et al., 2017; Yoshikawa et al., 2015). The phylogenetic analyses based on 878-bp L segment sequences revealed that sequences of the JX22-1 and JX23WN strains were clustered into an unidentified genotype (here assigned as C5) of the Chinese clade, and the JX23XSH strain into genotype C4 (Figure 2A). Phylogenetic analysis of 560-bp M segment sequences placed the JX22-1 and JX23WN strains on the genotype C5 branch, and the JX23XSH strain on the genotype C4 branch (Figure 2B). However, the phylogenetic analysis based on 577-bp S segment sequences revealed that all three SFTSV strains obtained in this study clustered with genotype J1 (Figure 2C), although none of the patients in Jiangxi had a history of travel to Japan prior to the onset of their disease. From the tree topology of the partial L, M, and S sequences, the three strains presented different evolutionary positions and changed among different clades. These results suggested that the S, M, and L segments of these strains may have derived from different ancestral genotypes, and they underwent segmental reassortment. The JX22-1 and JX23WN strains were subjected to segmental reassortment between genotype C5 and genotype J1, and the JX23XSH strain between the genotype C4 and the genotype J1 (Figure 2).
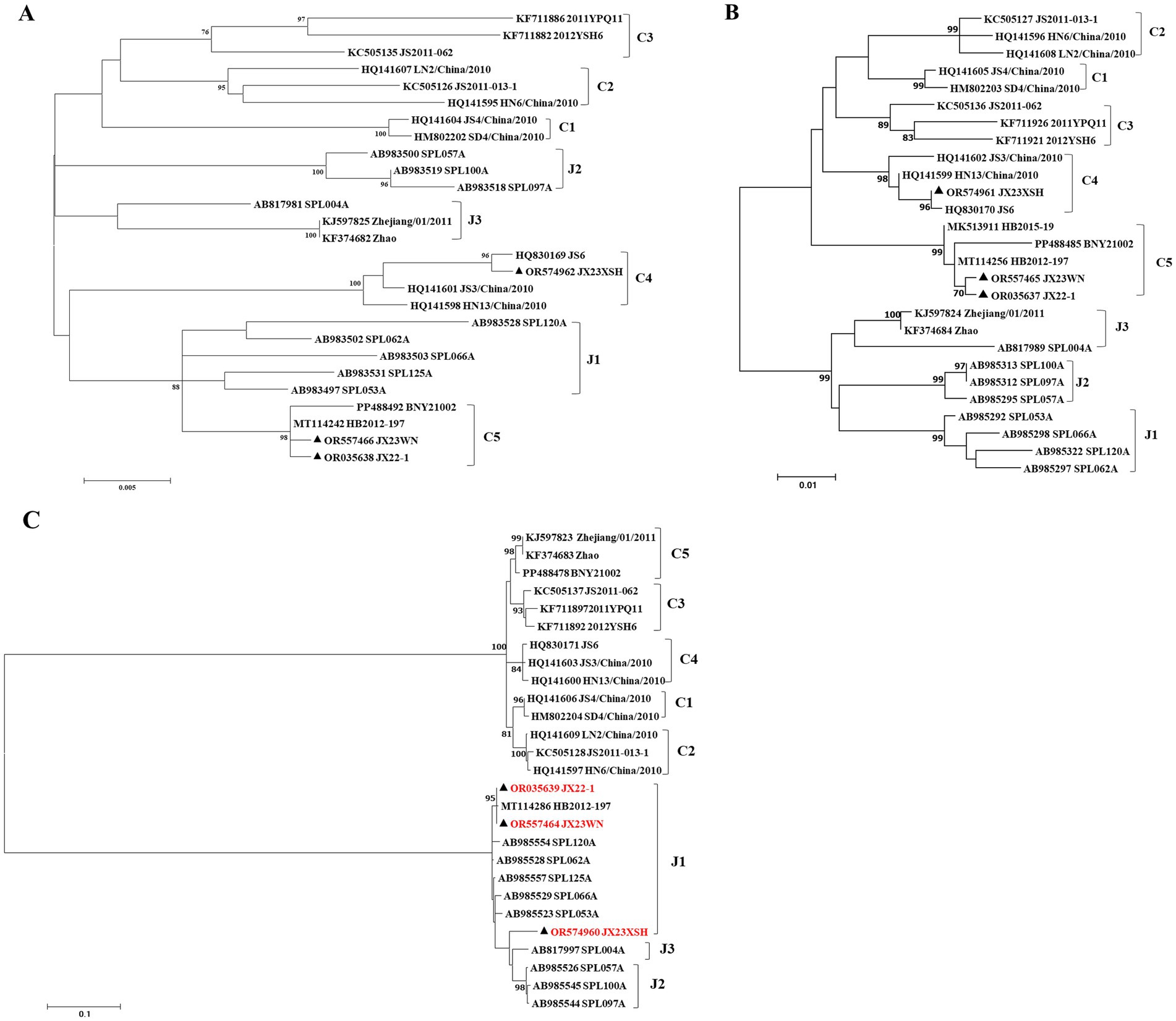
Figure 2. The maximum likelihood trees of the SFTSV genome in rectangular format for the partial L (A), M (B), and S (C) segments. The tree is drawn to scale, with branch lengths measured in the number of substitutions per site. Eight of the genotypes have been categorized, and the names of genotypes to the right side of trees (C1 to C5 and J1 to J3) are also shown. The values of the bootstrap percentage are shown next to the branches. The strains indicated by red-faced characters may have undergone segmental reassortment between genotypes. L, large; M, medium; S, small; SFTSV, severe fever with thrombocytopenia syndrome virus. The sequences with filled triangles are detected in this study.
To avoid poor tree topology support with the partial S, M, and L segments, we further performed sequencing and analysis of the complete genome of SFTSV. The whole genome sequencing was successful in the JX23WN and JX23XSH strains, while the JX22-1 strain failed to be fully sequenced due to low SFTSV load. Identical to the tree topology of the partial L and M segments, the full-length L and M segment sequences of the JX23WN strain were also clustered into genotype C5, and the JX23XSH into genotype C4. However, different from the tree topology of the partial S segments, the full-length S segment sequences of the JX23WN and JX23XSH strains were grouped on the genotype C5 and C4 branches, respectively, not on the genotype J1 branch of the partial S tree (Figure 3). In combination with the L, M, and S phylogenetic tree of the partial and complete segments, these findings indicated that three strains in this study might have undergone segmental recombination between genotypes.
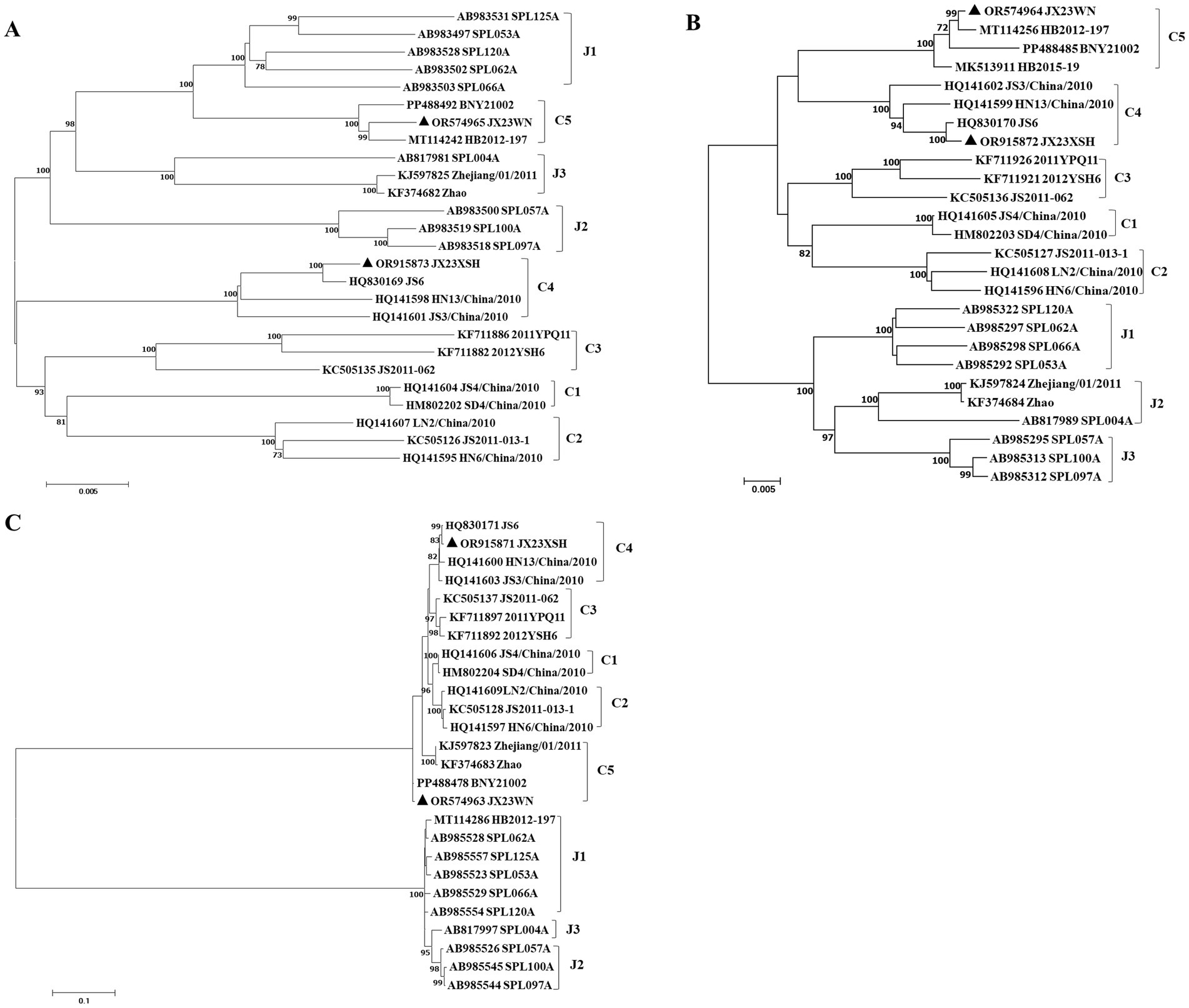
Figure 3. The maximum likelihood trees of the SFTSV genome in rectangular format for the complete L (A), M (B), and S (C) segments. The tree is drawn to scale, with branch lengths measured in the number of substitutions per site. Eight of the genotypes have been categorized, and the names of genotypes to the right side of trees (C1 to C5 and J1 to J3) are also shown. The values of the bootstrap percentage are shown next to the branches. L, large; M, medium; S, small; SFTSV, severe fever with thrombocytopenia syndrome virus. The sequences with filled triangles are detected in this study.
3.3 Recombination events have been detected on SFTSV in Jiangxi Province
Based on the phylogenetic data, there were likely some recombination occurrences in SFTSVs from three patients residing in Jiangxi Province. To detect and further characterize recombination events, recombination signals and possible breakpoints were identified using six methods in the RDP package. The concatenated 878-bp L, 560-bp M, and 577-bp S sequences of SFTSV strains from Supplementary Dataset 1 were uploaded into the RDP package and run with default values. Consequently, two reassortment events appeared to occur between different clades (Table 3), and were found in the three strains with segments of inconsistent ancestral genotypes identified in the S, M, and L phylogenetic trees (Figure 2 and Table 3). The JX23XSH reassortment event was detected with high confidence (Table 3 and Figure 4). The event was detected by all the six methods with a significance level of p-value <0.05. RDPRCS was 0.71, which is greater than the threshold value 0.60 (Table 3 and Figure 4). The JX23XSH strain, from Xiushui County, Jiujiang City, Jiangxi Province, was designated as one reassortment event with the major parent (SPL097A, J2/J2/J2) and the minor parent (JS6, C4/C4/C4) (Table 3 and Figures 2, 4, 5). The GENECONV method identified the JX23XSH strain with two breakpoint positions at 1386 and 1897 nucleotide sites (Figures 4, 5). In comparison, the JX23WN reassortment event was detected with lower confidence. The event was detected by four methods with a significance level of p-value <0.05. RDPRCS was 0.50, falling within the range of 0.4 and 0.6 (Table 3 and Figure 6). Therefore, the event was considered a probable recombination. The JX22-1strain had the same recombination even as JX23WN strain. The JX23WN strain from Wuning County, Jiujiang City, Jiangxi Province, was designated as one reassortment event with the major parent (SPL097A, J2/J2/J2) and the minor parent (JS2011-062, C3/C3/C3) (Table 3 and Figures 2, 6, 7). The GENECONV method detected the strain with two breakpoint positions at 1,342 and 1,901 nucleotide sites (Figures 6, 7).
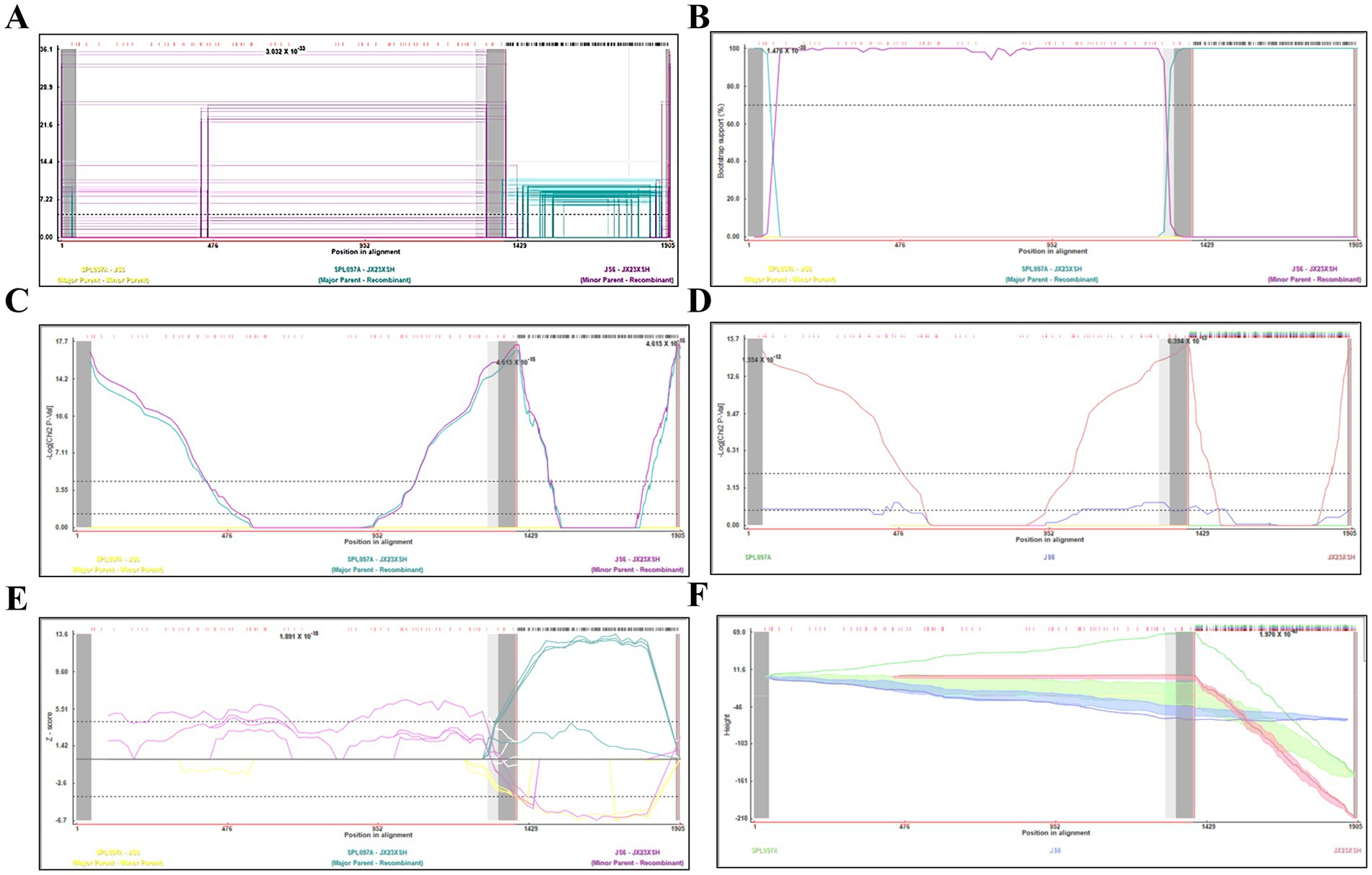
Figure 4. Plot of high scoring fragments for JX23XSH recombination signal based on the concatenation of the partial sequences of the L, M, and S segments. (A) GENECONV; (B) BootScan; (C) MaxChi; (D) Chimaera; (E) SiScan; (F) 3Seq. The left and right bounds of the red region indicate breakpoint positions suggested by the GENECONV method. Numbers in the panels are approximate p-values.
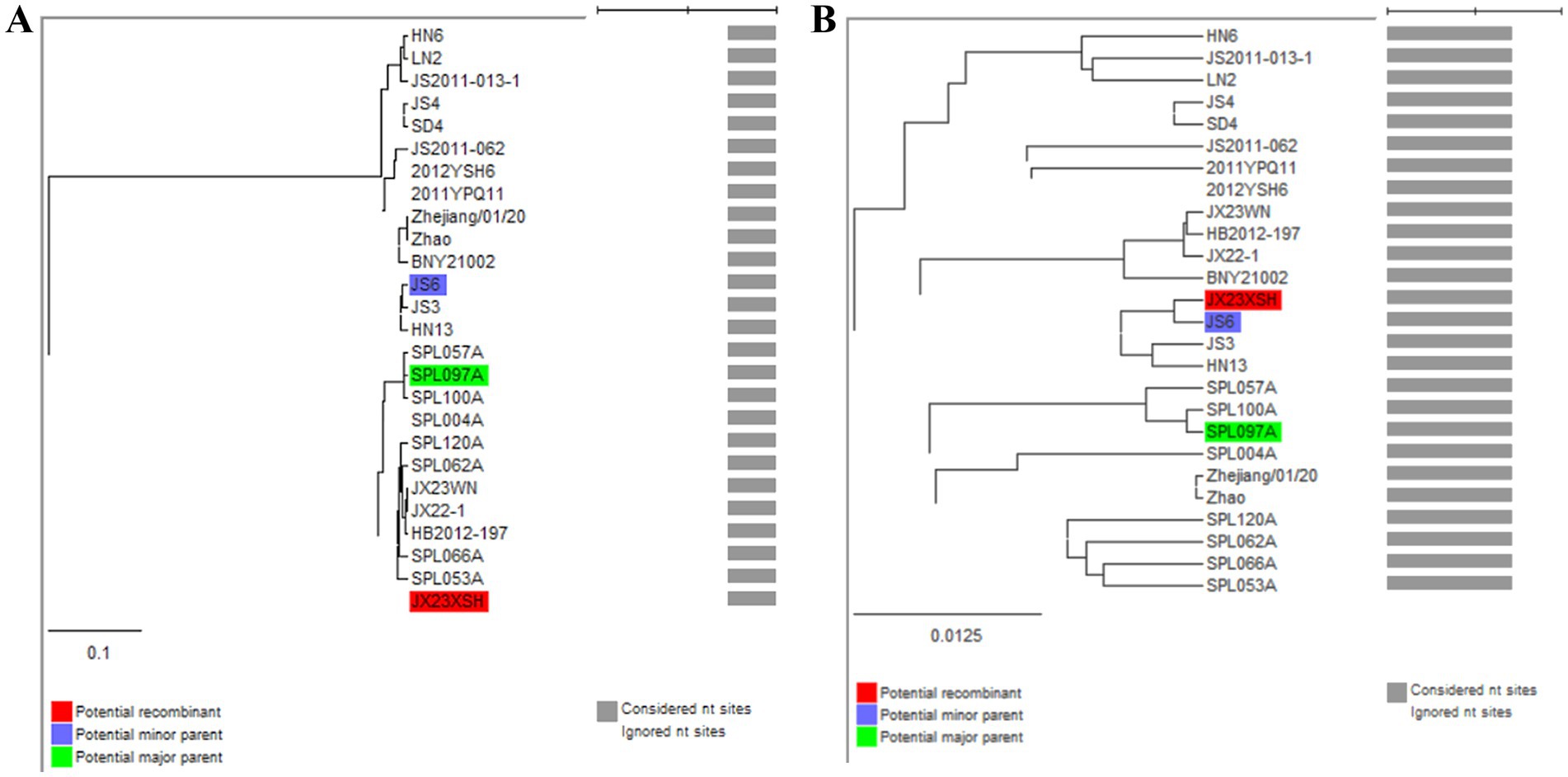
Figure 5. Unweighted pair-group method with arithmetic means (UPGMA) tree constructed with regions derived from major parent (1387–1897) (A) and minor parent (1–1,386 and 1,898–1,905) (B) of JX23XSH based on the concatenation of the partial sequences of the L, M, and S segments.
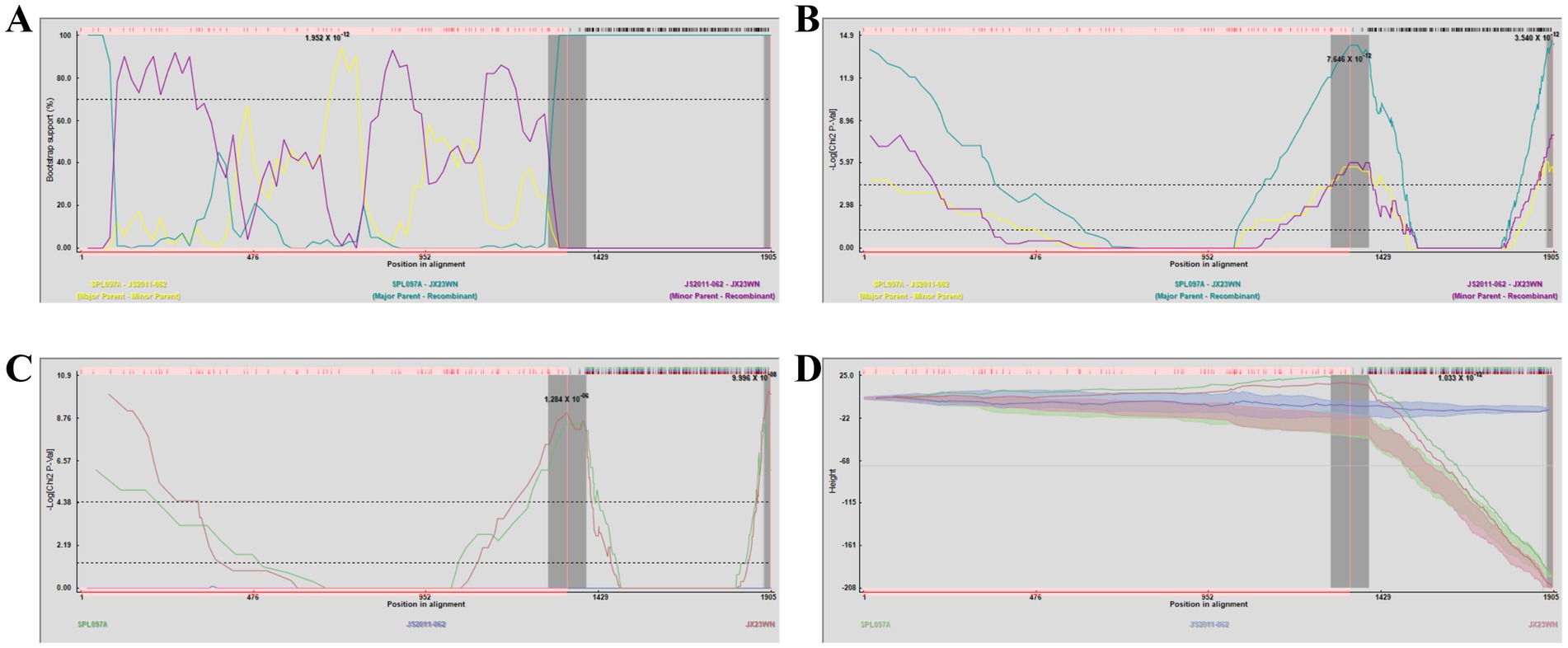
Figure 6. Plot of high scoring fragments for JX23WN recombination signal based on the concatenation of the partial sequences of the L, M, and S segments. (A) BootScan; (B) MaxChi; (C) Chimaera; (D) 3Seq. The left and right bounds of the red region indicate breakpoint positions suggested by the GENECONV method. Numbers in the panels are approximate p-values.
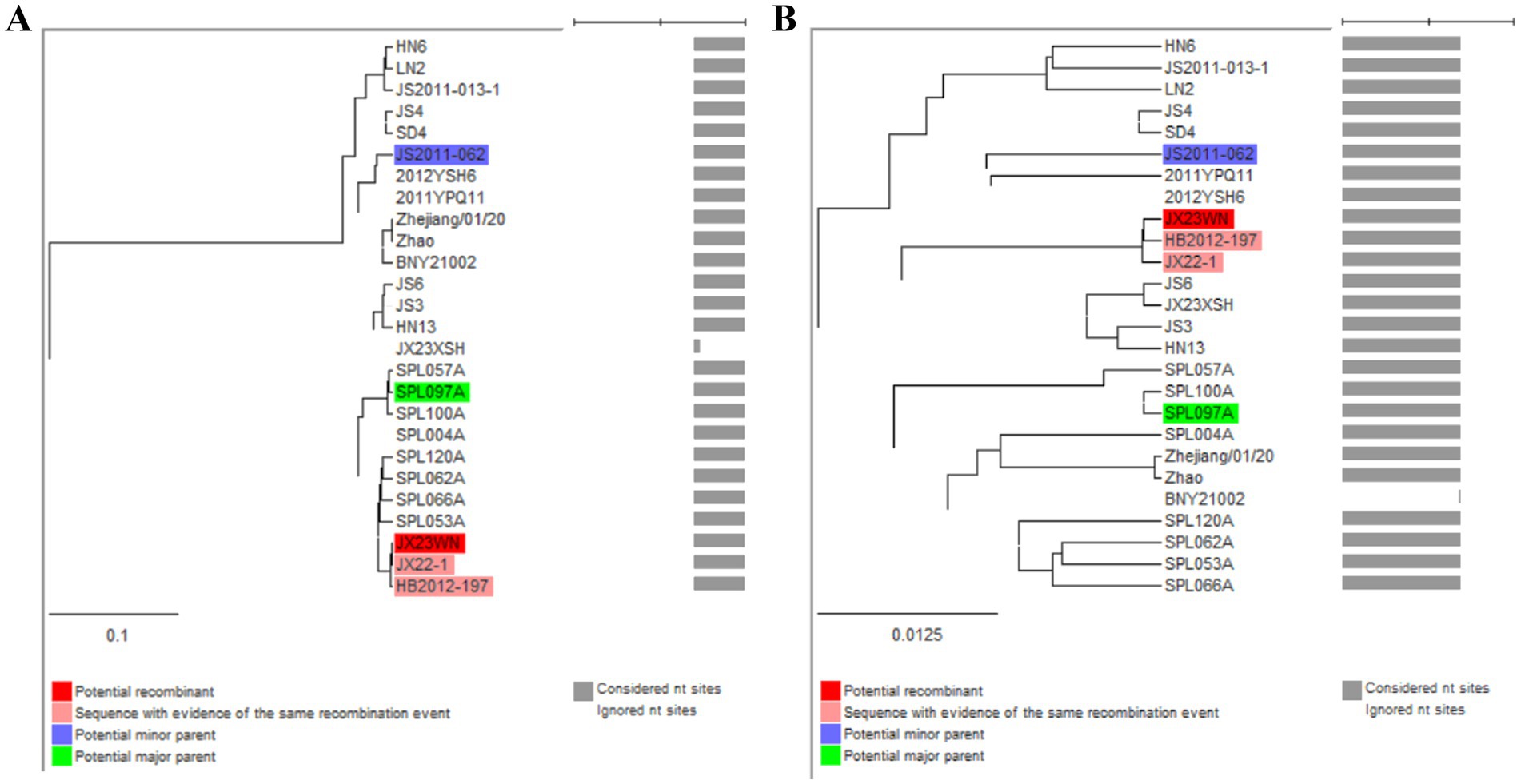
Figure 7. Unweighted pair-group method with arithmetic means tree constructed with regions derived from major parent (1,343–1,901) (A) and minor (1–1,342 and 1,902–1,905) (B) of JX23WN based on the concatenation of the partial sequences of the L, M, and S segments.
Based on the concatenated full-length L, M, and S sequences of SFTSV strains from dataset 2, two predicted recombination events in SFTSV strains from Jiangxi province, including details of the recombinants and parents, are summarized in Table 4. The JX23WN recombination event was detected with moderate confidence (Table 4 and Figure 8). The event was detected by all the six methods with a significance level of p-value <0.05. However, RDPRCS was 0.41, falling within the range of 0.4 and 0.6 (Table 4 and Figure 8). Therefore, the event was considered a probable recombination. The JX23WN strain was designated as one probable recombination event with the minor parent (2011YPQ11, C3/C3/C3) and the major parent (SPL053A, J1/J1/J1) (Table 4 and Figures 8, 9). The recombination event contained parental sequences from different geographic areas, indicating the potential involvement of virus migration between different areas. The GENECONV method detected the strain with two breakpoint positions at 84 and 9,714 nucleotide sites (Figures 8, 9). The JX23XSH recombination event was detected with low confidence (Table 4). The event was detected by only two methods with a significance level of p-value <0.05 and RDPRCS was 0.42, falling within the range of 0.4 and 0.6 (Table 4). Therefore, the event was disregarded as a recombination.
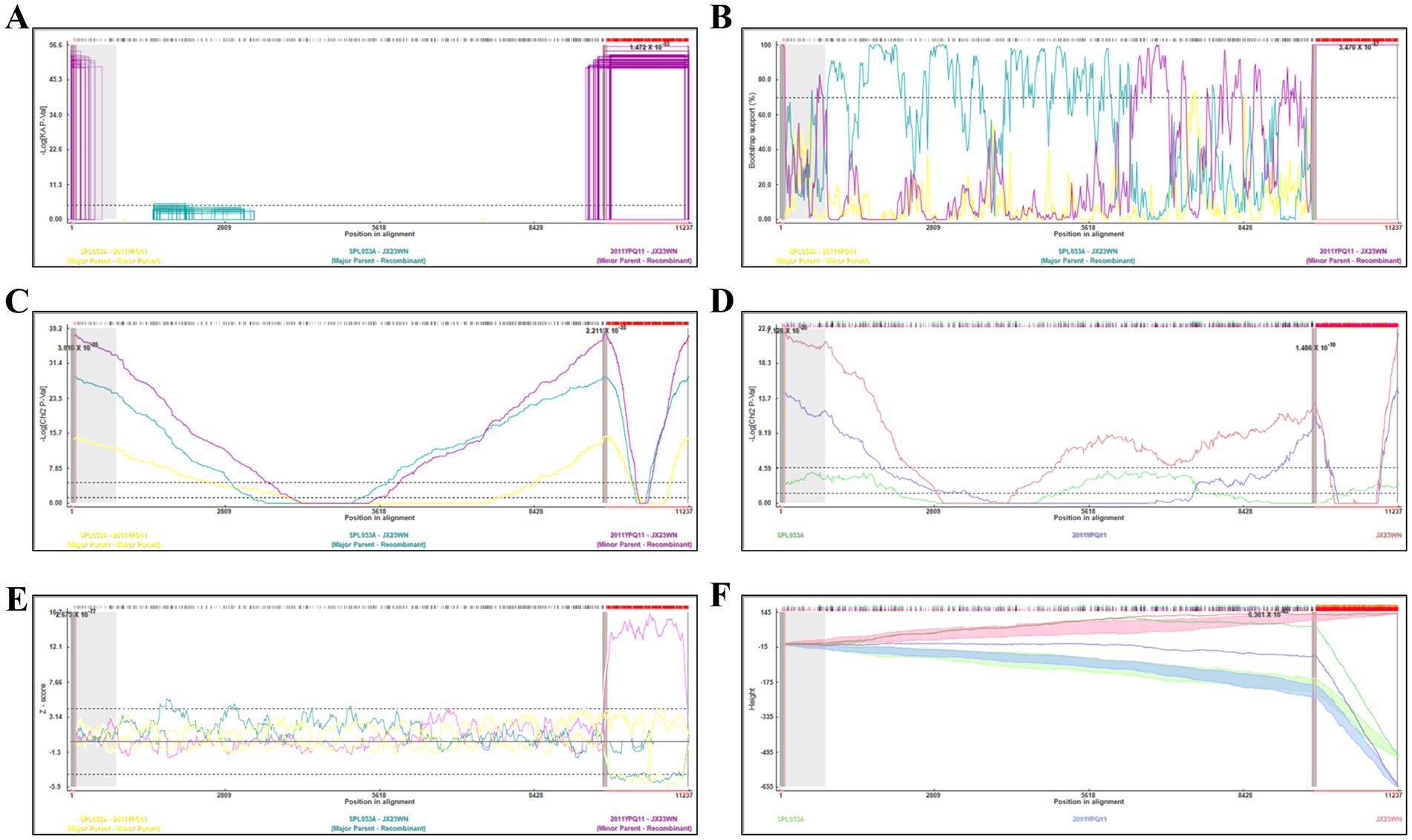
Figure 8. Plot of high scoring fragments for JX23WN recombination signal based on the concatenation of the complete sequences of the L, M, and S segments. (A), GENECONV; (B) BootScan; (C) MaxChi; (D) Chimaera; (E) SiScan; (F) 3Seq. The left and right bounds of the red region indicate breakpoint positions suggested by the GENECONV method. Numbers in the panels are approximate p-values.
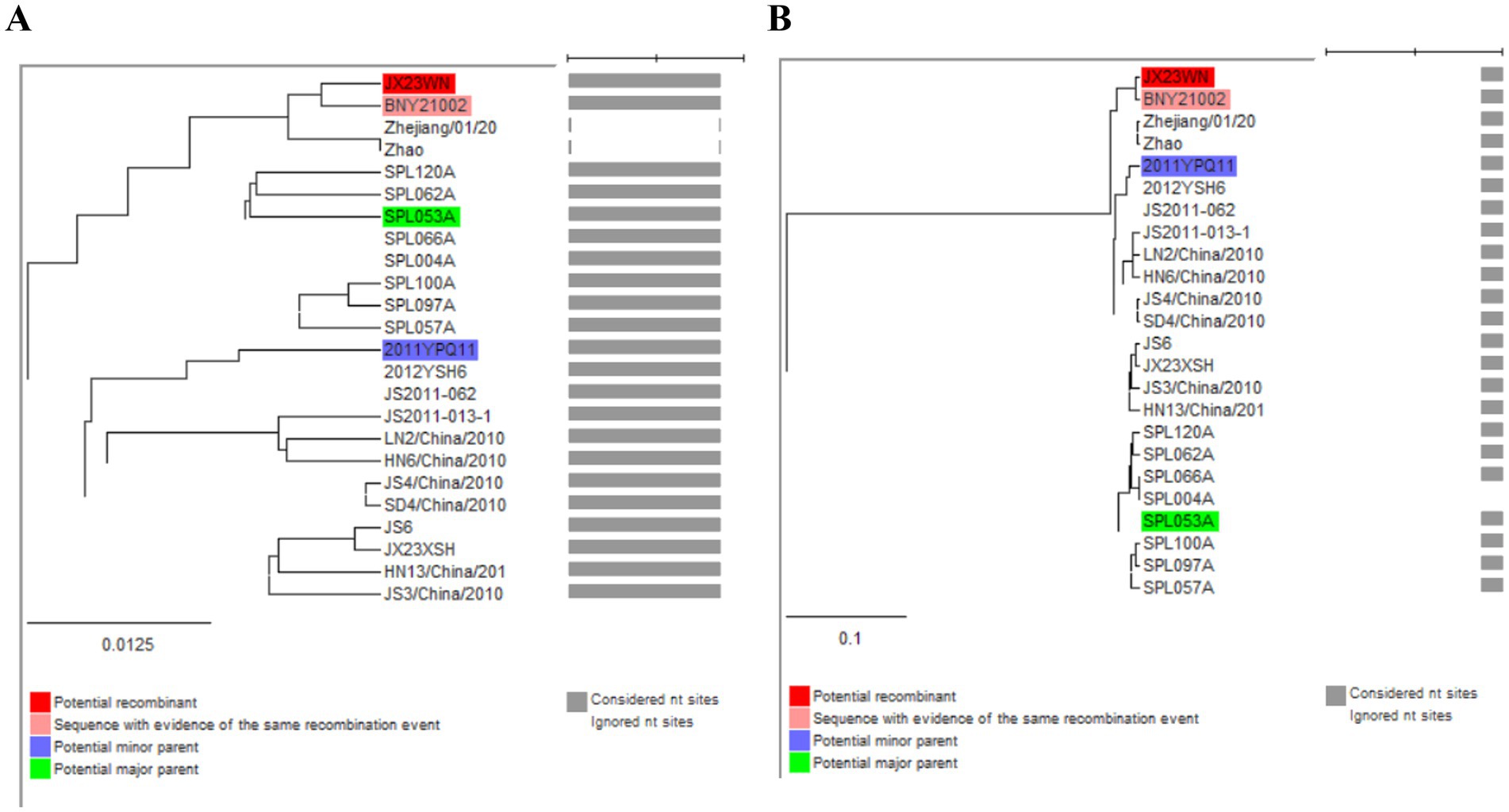
Figure 9. Unweighted pair-group method with arithmetic means tree constructed with regions derived from major parent (85–9714) (A) and minor (1–84 and 9,715–11,237) (B) of JX23WN based on the concatenation of the complete sequences of the L, M, and S segments.
4 Discussion and conclusion
The SFTS is a vector-borne disease transmitted primarily through the bite of infected ticks, particularly H. longicornis (Yu et al., 2011; Zhuang et al., 2018). The virus can also spread through direct contact with body fluids or blood from infected individuals (Tang et al., 2013). Symptoms of SFTS include the sudden onset of fever, gastrointestinal distress, bleeding, and low platelet count, which can lead to life-threatening complications if not promptly treated (Liu et al., 2014; Yu et al., 2011). Currently, the epidemic status of SFTSV in China is characterized by widespread presence across various provinces, with significant numbers of cases reported in region such as Henan, Hubei, Jiangsu, Zhejiang, Anhui, and Shandong (Li et al., 2022). Despite bordering with the epidemic Hubei, Anhui, and Zhejiang, Jiangxi has only a few cases, ranging from zero to two patients during 2011 and 2018 (Qian, 2020). However, in recent years patients with SFTSV infection have been increasingly found in the Mufu mountains of Jiujiang, Jiangxi. Here, we found four SFTS patients in Jiujiang, Jiangxi though we failed to sequence the viral genome from the fourth patient. We inferred the failure from low virus load. The first, second and third patients were detected positive for SFTSV with Ct value of 32, 26, and 30, respectively. They had at least 1,000-fold higher virus loads than that of the fourth patient, whose serum was positive in qRT-PCRs for SFTSV with Ct value of 35 (unpublished data).
The PCR tests for SFTSV infection in high-risk humans, animals and ticks surrounding these patients yielded negative results. However, two out of the 56 serum samples tested strongly positive for immunoglobulin G (IgG) antibody against SFTSV using ELISA, with one of samples belonging to the wife of patient 2. In addition, a serum sample from one of the 12 dogs tested was also positive for anti-SFTSV IgG (unpublished data). Our results indicate that Jiujiang may have natural conditions for SFTSV circulation.
The SFTSV strains have highly conservative L segment (95.81–99.64%), moderately conservative M segment (93.66–99.70%), and yet variable S segment (26.95–99.48%). Furthermore, this virus also exchanges genes between different strains. Therefore, SFTSV is reported as a rapidly evolutionary virus, and it currently has at least 10 genotypes at present. These genotypes consist of genotype C1–C4, primarily from China, and J1–J3, mainly from Japan (Ding and He, 2012; Lv et al., 2017; Wu et al., 2021). The evolutionary forces shaping these viruses are complex and multifaceted. SFTSV has high mutation rates (~10–4 substitutions/site/year) during its replication due to the lack of proofreading capabilities in their RNA-dependent RNA polymerases (RdRps) (Lam et al., 2013). Although recombination is uncommon in SFTSV, it can occur through the co-infection of a host cell by two or more genetically distinct viruses. Recombination can result in the exchange of genetic information and scientists have reported a recombination event between two strains JF837594/JS2007-01 and HM802203/SD4/China/2010, at the 503 and 1,410 nucleotide sites of M segment, leading to the generation of a novel genotype HQ642767/BX-2010/Henan/CHN with unique combinations of traits (Ding and He, 2012). SFTSV is one of segmented-genome viruses, with its genome divided into three segments. Reassortment can occur when two or more genetically distinct virus strains infect the same host cell simultaneously. During this process, viral segments from different strains can be shuffled and reassorted, leading to the generation of novel virus genotypes. For instance, the strain YSC3 has L and M segments from genotype C2, and exchanges its S segment from C2 to C4 (Lv et al., 2017). There are two reassortment patterns in SFTSV: single reassortment and multiple reassortment. The single reassortment occurs when a strain has one segment belonging to one genotype and the other two segments belonging to a distinct genotype. Wu et al. (2021) found single reassortment in 13 strains, with 6 reassortment events involving the S segment, 3 events involving the M segment, and 4 events involving the L segment. Multiple reassortment occurs when all the three segments belong to a different genotype. The strains AHL, NB-32, KADGH4, and ZJ2014-01 were involved in multiple reassortment events (Wu et al., 2021). Although some cases had been found in Jiangxi, there are only six strains of SFTSV genomes from Jiangxi Province searched in National Center for Biotechnology information (NCBI). These strains include three strains analyzed in this study. Up to now no evolutionary characteristics have been exposed on the virus from Jiangxi. Here, based on the phylogenetic analysis of the complete L, M, and S segments, two SFTSV genotypes were detected in Jiujiang city, Jiangxi Province: C4 and C5 genotypes, respectively. Further recombination analysis, as well as phylogenetic tree of the partial S segment, suggested that the strain JX23WN underwent genetic recombination. It evolved from the strain 2011YPQ11 as the minor parent and the strain SPL053A as the major parent. However, coinfection experiments need to be conducted to confirm whether recombination can occur between the strain 2011YPQ11 and the strain SPL053A to generate the strain JX23WN.
Although we sampled various specimens related to SFTSV infection, including patients, high risk humans, ticks and animals, and conducted a phylogenetic study to investigate the molecular characteristics of SFTSVs, we continue to recognize the significance of expanding our sample collection and acquiring additional SFTSV strains. Ticks collected by flagging vegetation and from domestic animals were negative for SFTSV sequences in the present study, and this might be attributed to the limited number of vector tick samples that were collected. Conducting surveying SFTSV infection in ticks, animals, and high-risk individuals only after case reporting could have potentially introduced bias into the determination of SFTSV distribution. Moreover, all of the ticks were extracted from domestic animals (goats, dogs, and cats), raising the likelihood of excluding ticks associated with wild animals within the surveyed locations. The SFTS virus is primarily transmitted by certain species of ticks, such as H. longicornis which is the dominant tick species in the survey areas (Zhuang et al., 2018). However, the prevalence of the virus in tick species can vary depending on various factors, including geographic location and seasonal variations. For example, the SFTSV was detected in 5.4% of H. longicornis specimens from Shandong, Henan and Hubei provinces in China (Yu et al., 2011). However, only 0.5% of tested specimens from Korea showed the presence of the SFTSV (Park et al., 2014). To accurately assess the presence of the SFTS virus in ticks, in the future we will collect an adequate number of samples including wild animals, tick vectors, and domestic animals from different locations and time periods in the Mufu mountains. This will ensure a representative sample size that can provide reliable data on the virus’s prevalence and molecular characteristics. In recent years, there have been many annual SFTS cases in the Mufu mountains of Jiangxi, and the morbidity is increasing. More effort should be made to systematically survey the epidemic of SFTS, its vectors and hosts, to elucidate the mechanism of the natural focal disease.
Although our study evaluated the recombination signals of SFTSVs in the Mufu mountains of Jiangxi, we could additionally obtain genetic sequences from Jiujiang and other areas of Jiangxi Province to better understand the evolutionary profiles in this province. A comprehensive understanding of evolutionary force and pathway will provide significant benefits in combating local SFTSV pandemics.
Taken together, SFTSV presence was found in Jiangxi. Furthermore, the sequence results demonstrate that C4 and C5 genotypes are present, and genotype C5 undergoes genetic recombination in Jiangxi Province of Central China.
Data availability statement
The datasets presented in this study can be found in online repositories. The names of the repository/repositories and accession number(s) can be found in the article/Supplementary material.
Ethics statement
The studies involving humans were approved by the ethics committees of the Nanchang Center for Disease Control and Prevention (NCCDCH-2022–15) and The First Affiliated Hospital of Nanchang University (FAHNU-2020-01). The studies were conducted in accordance with the local legislation and institutional requirements. Written informed consent for participation in this study was provided by the participants’ legal guardians/next of kin. The animal studies were approved by Animal Ethics Review Board of the Nanchang Center for Disease Control and Prevention (xyll-2022-03). The studies were conducted in accordance with the local legislation and institutional requirements. Written informed consent was obtained from the owners for the participation of their animals in this study.
Author contributions
HH: Funding acquisition, Investigation, Methodology, Writing – original draft, Writing – review & editing. YH: Investigation, Resources, Writing – review & editing. FC: Data curation, Formal analysis, Investigation, Writing – review & editing. ZL: Investigation, Resources, Writing – review & editing. WW: Investigation, Resources, Writing – review & editing. SY: Writing – review & editing. KQ: Investigation, Methodology, Writing – review & editing. ZZ: Investigation, Resources, Writing – review & editing. YG: Investigation, Resources, Writing – review & editing. HL: Conceptualization, Supervision, Validation, Writing – review & editing. WZ: Conceptualization, Project administration, Supervision, Validation, Writing – review & editing.
Funding
The author(s) declare that financial support was received for the research, authorship, and/or publication of this article. This study was financially supported by the Nanchang Municipal Science and Technology Bureau (Grant numbers 2022-KJZC-024). WZ and HH received funding from the Nanchang Municipal Science and Technology Bureau with grant number 2022-KJZC-024.
Acknowledgments
We thank our colleagues from the Donghu Center for Disease Control and Prevention in Nanchang city, Jiangxi Province, for their assistance in epidemiological surveillance and patient serum collection.
Conflict of interest
The authors declare that the research was conducted in the absence of any commercial or financial relationships that could be construed as a potential conflict of interest.
Publisher’s note
All claims expressed in this article are solely those of the authors and do not necessarily represent those of their affiliated organizations, or those of the publisher, the editors and the reviewers. Any product that may be evaluated in this article, or claim that may be made by its manufacturer, is not guaranteed or endorsed by the publisher.
Supplementary material
The Supplementary material for this article can be found online at: https://www.frontiersin.org/articles/10.3389/fmicb.2024.1500146/full#supplementary-material
References
Casel, M. A., Park, S. J., and Choi, Y. K. (2021). Severe fever with thrombocytopenia syndrome virus: emerging novel phlebovirus and their control strategy. Exp. Mol. Med. 53, 713–722. doi: 10.1038/s12276-021-00610-1
Ding, N. Z., and He, C. Q. (2012). Discovery of severe fever with thrombocytopenia syndrome bunyavirus strains originating from intragenic recombination. J. Virol. 86, 12426–12430. doi: 10.1128/JVI.01317-12
Hu, B., Cai, K., Liu, M., Li, W., Xu, J., Qiu, F., et al. (2018). Laboratory detection and molecular phylogenetic analysis of severe fever with thrombocytopenia syndrome virus in Hubei Province, Central China. Arch. Virol. 163, 3243–3254. doi: 10.1007/s00705-018-3993-5
Kimura, M. (1980). A simple method for estimating evolutionary rates of base substitutions through comparative studies of nucleotide sequences. J. Mol. Evol. 16, 111–120. doi: 10.1007/BF01731581
Lam, T. T. Y., Liu, W., Bowden, T. A., Cui, N., Zhuang, L., Liu, K., et al. (2013). Evolutionary and molecular analysis of the emergent severe fever with thrombocytopenia syndrome virus. Epidemics 5, 1–10. doi: 10.1016/j.epidem.2012.09.002
Li, J. C., Zhao, J., Li, H., Fang, L. Q., and Liu, W. (2022). Epidemiology, clinical characteristics, and treatment of severe fever with thrombocytopenia syndrome. Inf. Med. 1, 40–49. doi: 10.1016/j.imj.2021.10.001
Liu, Q., He, B., Huang, S. Y., Wei, F., and Zhu, X. Q. (2014). Severe fever with thrombocytopenia syndrome, an emerging tick-borne zoonosis. Lancet Infect. Dis. 14, 763–772. doi: 10.1016/S1473-3099(14)70718-2
Lv, J., Wu, S., Zhang, Y., Chen, Y., Feng, C., Yuan, X., et al. (2014). Assessment of four DNA fragments (COI, 16S rDNA, ITS2, 12S rDNA) for species identification of the Ixodida (Acari: Ixodida). Parasit. Vectors 7:93. doi: 10.1186/1756-3305-7-93
Lv, J., Zhang, H., Tian, L., Zhang, R. L., Zhang, Z. J., Li, J., et al. (2017). Novel sub-lineages, recombinants and reassortants of severe fever with thrombocytopenia syndrome virus. Ticks Tick Borne Dis 8, 385–390. doi: 10.1016/j.ttbdis.2016.12.015
Martin, D. P., Murrell, B., Golden, M., Khoosal, A., and Muhire, B. (2015). RDP4: detection and analysis of recombination patterns in virus genomes. Virus Ecol 1:vev003. doi: 10.1093/ve/vev003
Miao, D., Liu, M. J., Wang, Y. X., Ren, X., Lu, Q. B., Zhao, G. P., et al. (2021). Epidemiology and ecology of severe fever with thrombocytopenia syndrome in China, 2010–2018. Clin. Infect. Dis. 73, e3851–e3858. doi: 10.1093/cid/ciaa1561
Nagarajan, N., and Kingsford, C. (2011). GiRaF: robust, computational identification of influenza reassortments via graph mining. Nucleic Acids Res. 39:e34. doi: 10.1093/nar/gkq1232
Park, S. W., Song, B. G., Shin, E. H., Yun, S. M., Han, M. G., Park, M. Y., et al. (2014). Prevalence of severe fever with thrombocytopenia syndrome virus in Haemaphysalis longicornis ticks in South Korea. Ticks Tick Borne Dis 5, 975–977. doi: 10.1016/j.ttbdis.2014.07.020
Qian, J. (2020). Studies on significant epidemiological characteristics of severe fever with thrombocytopenia syndrome and coronavirus disease 2019 in mainland China. Beijing: Peking Union Medical College.
Saitou, N., and Nei, M. (1987). The neighbor-joining method: a new method for reconstructing phylogenetic trees. Mol. Biol. Evol. 4, 406–425. doi: 10.1093/oxfordjournals.molbev.a040454
Shi, J., Hu, S., Liu, X., Yang, J., Liu, D., Wu, L., et al. (2017). Migration, recombination, and reassortment are involved in the evolution of severe fever with thrombocytopenia syndrome bunyavirus. Infect. Genet. Evol. 47, 109–117. doi: 10.1016/j.meegid.2016.11.015
Tamura, K., Stecher, G., Peterson, D., Filipski, A., and Kumar, S. (2013). MEGA6: molecular evolutionary genetics analysis version 6.0. Mol. Biol. Evol. 30, 2725–2729. doi: 10.1093/molbev/mst197
Tang, X. Y., Wu, W. L., Wang, H. F., Du, Y. H., Liu, L. C., Kang, K., et al. (2013). Human-to-human transmission of severe fever with thrombocytopenia syndrome Bunyavirus through contact with infectious blood. J. Infect. Dis. 207, 736–739. doi: 10.1093/infdis/jis748
Wu, X. L., Li, M. Y., Zhang, Y. F., Liang, B. Y., Shi, J. M., Fang, Y. H., et al. (2021). Novel SFTSV phylogeny reveals new reassortment events and migration routes. Virol. Sin. 36, 300–310. doi: 10.1007/s12250-020-00289-0
Yoshikawa, T., Shimojima, M., Fukushi, S., Tani, H., Fukuma, A., Taniguchi, S., et al. (2015). Phylogenetic and geographic relationships of severe fever with thrombocytopenia syndrome virus in China, South Korea, and Japan. J. Infect. Dis. 212, 889–898. doi: 10.1093/infdis/jiv144
Yu, X. J., Liang, M. F., Zhang, S. Y., Liu, Y., Li, J. D., Sun, Y. L., et al. (2011). Fever with thrombocytopenia associated with a novel bunyavirus in China. N. Engl. J. Med. 364, 1523–1532. doi: 10.1056/NEJMoa1010095
Keywords: severe fever with thrombocytopenia syndrome, genotype, C4 and C5, genetic recombination, Jiangxi
Citation: Hu H, He Y, Chen F, Liu Z, Wang W, Yang S, Qian K, Zhan Z, Guo Y, Li H and Zheng W (2024) Severe fever with thrombocytopenia syndrome virus was found in Northern Jiangxi Province, China. Front. Microbiol. 15:1500146. doi: 10.3389/fmicb.2024.1500146
Edited by:
Angela Stufano, University of Bari Aldo Moro, ItalyReviewed by:
Wentao Zhu, Capital Medical University, ChinaShikha Joon, National Cancer Institute at Frederick (NIH), United States
Copyright © 2024 Hu, He, Chen, Liu, Wang, Yang, Qian, Zhan, Guo, Li and Zheng. This is an open-access article distributed under the terms of the Creative Commons Attribution License (CC BY). The use, distribution or reproduction in other forums is permitted, provided the original author(s) and the copyright owner(s) are credited and that the original publication in this journal is cited, in accordance with accepted academic practice. No use, distribution or reproduction is permitted which does not comply with these terms.
*Correspondence: Hui Li, bmNjZGN5amJAMTYzLmNvbQ==; Weiqing Zheng, emhlbmd3ZWlxaW5nMjAwMUAxNjMuY29t