- 1Centres for Antimicrobial Optimization Network (CAMO-Net), Infectious Diseases Institute, Makerere University College of Health Sciences, Kampala, Uganda
- 2Management Sciences for Health, Kampala, Uganda
- 3Global Health Security Department, Infectious Diseases Institute, Makerere University College of Health Sciences, Kampala, Uganda
- 4Department of Medical Microbiology, Makerere University College of Health Sciences, Kampala, Uganda
- 5Makerere University School of Public Health, Kampala, Uganda
Globally, Healthcare-associated infections (HCAIs) pose a significant threat to patient safety and healthcare systems. In low- and middle-income countries (LMICs), the lack of adequate resources to manage HCAIs, as well as the weak healthcare system, further exacerbate the burden of these infections. Traditional surveillance methods that rely on laboratory tests are cost-intensive and impractical in these settings, leading to ineffective monitoring and delayed management of HCAIs. The rates of HCAIs in resource-limited settings have not been well established for most LMICs, despite their negative consequences. This is partly due to costs associated with surveillance systems. Syndromic surveillance, a part of active surveillance, focuses on clinical observations and symptoms rather than laboratory confirmation for HCAI detection. Its cost-effectiveness and efficiency make it a beneficial approach for monitoring HCAIs in LMICs. It provides for early warning capabilities, enabling timely identification and response to potential HCAI outbreaks. Syndromic surveillance is highly sensitive and this helps balance the challenge of low sensitivity of laboratory-based surveillance systems. If syndromic surveillance is used hand-in-hand with laboratory-based surveillance systems, it will greatly contribute to establishing the true burden of HAIs in resource-limited settings. Additionally, its flexibility allows for adaptation to different healthcare settings and integration into existing health information systems, facilitating data-driven decision-making and resource allocation. Such a system would augment the event-based surveillance system that is based on alerts and rumours for early detection of events of outbreak potential. If well streamlined and targeted, to monitor priority HCAIs such as surgical site infections, hospital-acquired pneumonia, diarrheal illnesses, the cost and burden of the effects from these infections could be reduced. This approach would offer early detection capabilities and could be expanded into nationwide HCAI surveillance networks with standardised data collection, healthcare worker training, real-time reporting mechanisms, stakeholder collaboration, and continuous monitoring and evaluation. Syndromic surveillance offers a promising strategy for combating HCAIs in LMICs. It provides early warning capabilities, conserves resources, and enhances patient safety. Effective implementation depends on strategic interventions, stakeholder collaboration, and ongoing monitoring and evaluation to ensure sustained effectiveness in HCAI detection and response.
Introduction
Healthcare-associated infections (HCAIs) are of significant public health concern worldwide, impacting patient safety during hospitalisation and healthcare systems in high, middle, and low-income countries (Haque et al., 2018; Maki and Zervos, 2021). These infections result in longer hospital stays, long-term disability, increased microbial resistance to antimicrobials, higher healthcare costs, financial burdens for patients and families, and unnecessary deaths (Ling et al., 2015; Marchetti and Rossiter, 2013). The burden is particularly high in low- and middle-income countries (LMICs) due to limited resources, weak health systems that include deficiencies in trained personnel, and inconsistent surveillance (Maki and Zervos, 2021; Abubakar et al., 2022; Vandijck et al., 2013).
Like many LMICs in sub-Saharan Africa, Uganda faces a daunting challenge in monitoring and managing HCAIs. Research conducted over a decade ago in two hospitals reported a prevalence of HCAIs at 34%, with the majority being mixed infections, including bloodstream infections, surgical wound infections, urinary tract infections, lower respiratory tract infections, and gastrointestinal infections (Ankunda et al., 2010; Greco and Magombe, 2011). In most cases, these infections were associated with longer hospital stays, intravenous and urinary catheterization, emergency surgeries, inadequate hand hygiene, poor isolation practices, and inadequate supplies for disinfection. These factors compromise infection prevention and control practices on the wards (Greco and Magombe, 2011). Data providing a clear national picture of HCAI surveillance in Uganda and many LMICs is limited due to the inadequacy of the traditional surveillance methods which require sophisticated laboratory and diagnostic capacity to identify the specific pathogens responsible for these infections (Haque et al., 2018).
Public health surveillance
Public Health Surveillance is the epidemiological foundation of global health and involves ongoing systematic identification, collection, collation, analysis, interpretation, and dissemination of disease occurrence and public health event data for timely and robust action (Nsubuga et al., 2011; Berkelman et al., 2009; Ministry of Health in Uganda, 2021). It is an essential tool for measuring disease burden, including monitoring morbidity/mortality trends, to effectively guide the planning, implementation, monitoring, and evaluation of control programs and the corresponding allocation of resources (Nsubuga et al., 2011). Public health surveillance is hinged on two approaches, including indicator-based (passive) and event-based (active) surveillance as shown in Figure 1 (Ministry of Health in Uganda, 2021). Indicator-based surveillance is a system where a health jurisdiction or institution receives routine reports from hospitals, clinics, public health units, communities, or other sources. It is a relatively cost-effective approach for covering large areas, utilizing several critical indicators embedded in health management information systems to monitor diseases and outbreaks within the community (Ministry of Health in Uganda, 2021; Sharp et al., 2015). Event-based surveillance, on the other hand, involves proactive search for information about public health events, risks, or conditions from health providers, health facilities and the communities. This includes records review by health workers, screening for specific outbreak-prone health conditions such as Ebola virus disease, contact tracing during outbreaks, regular communication, and maintaining contact with key reporting sources (Ministry of Health in Uganda, 2021; Chung et al., 2015; McNamara, 2016). In both approaches, syndromic surveillance is typically applied, utilizing definitions based solely on clinical features without confirmatory laboratory diagnoses. For example, during a measles outbreak, any child below five with a rash would be considered a measles patient (May et al., 2009). This method aids in the early identification of illness clusters before diagnoses are confirmed, ensuring timely reporting to public health agencies for rapid response, thereby reducing morbidity and mortality (Henning, 2004). The two approaches complement each other. In many surveillance systems, they have been merged into integrated systems, utilizing the same infrastructure to gather information on multiple public health events of interest (Nsubuga et al., 2011). These integrated surveillance systems provide an early warning system for early detection of public health events for rapid detection, investigation, and response to public health events (World Health Organization, 2014).
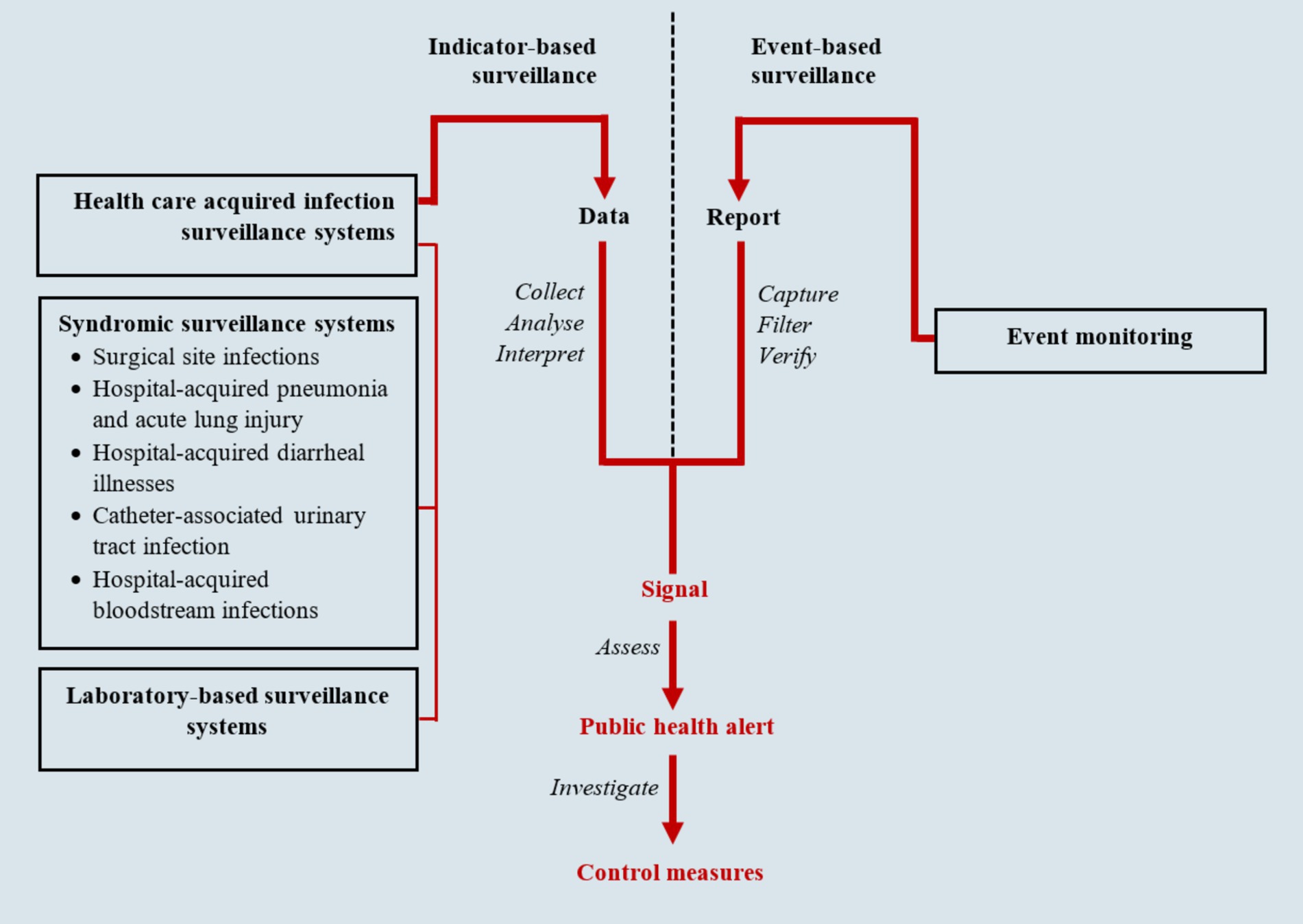
Figure 1. Infectious disease surveillance framework (adapted from National Academies of Sciences, Engineering, and Medicine) (Ministry of Health in Uganda, 2021; National Academies of Sciences, Engineering, and Medicine, 2007).
Surveillance of HCAIs
Surveillance of HAIs serves as a cornerstone for infection control programs, antimicrobial stewardship programs, patient safety and early warning systems for disease outbreak detection (Shenoy, 2023). Additionally, conducting surveillance for HCAIs allows for the detection of variations in the rate or distribution of HCAIs, which can subsequently lead to the identification of potentially contagious disease threats within the health facility (Shenoy, 2023; Surveillance CNI, 2023). For example, it could indicate hospital environmental contamination and lead to corrective action (Surveillance CNI, 2023).
Laboratory surveillance of HCAIs
Traditional surveillance for HCAIs typically involves laboratory confirmation, active case finding, and reviewing of patient medical records during hospitalisation (Magill et al., 2015; Monegro et al., 2017; Dramowski et al., 2017; Hearn et al., 2017). Laboratory confirmation involves culturing (bacterial growth and enumeration) and antimicrobial sensitivity testing of specific pathogens from priority clinical specimens, such as blood, urine, and pus, to track infections acquired during healthcare (Dramowski et al., 2017). This confirmation is usually complemented by active case finding, where healthcare personnel actively search for patients who may have been exposed to those with the given HCAI in the wards. In some instances, healthcare personnel also review patient medical records for any signs and symptoms suggestive of infection, alongside laboratory results, to support the HCAI diagnosis (Hearn et al., 2017). Traditional HCAI surveillance is a vital tool, especially in high-income countries with adequate healthcare infrastructure. However, it is resource-intensive and involves long turnaround times, posing significant challenges in resource-limited settings such as in Sub-Saharan Africa (Maki and Zervos, 2021). consequently, alternative approaches, such as syndromic surveillance, are necessary for monitoring HCAIs in these resource-limited settings. This paper explores the use of syndromic surveillance for monitoring HCAIs in resource-limited settings that lack advanced laboratory diagnostics and real-time surveillance capabilities, such as Uganda.
Syndromic surveillance of HCAIs
Rather than relying on laboratory confirmation of specific pathogens, syndromic surveillance focuses on identifying groups/sets of clinical signs and symptoms (syndromes) suggestive of possible infections, frequency (such as the rate of the episodes) in which these syndromes occur in a given population (Reingold, 2003; Katz et al., 2011; Boyle and Sparks, 2022). Syndromic surveillance is a fundamental approach to detecting aberrations in health data and signalling the onset of public health threats (May et al., 2011). Its core principle is to provide an early warning system that monitors disease patterns and tracks the emergence of unusual signs and symptoms grouped into case definitions. This enables public health authorities to initiate timely and effective interventions (May et al., 2011; Sosin, 2003). For HCAIs, syndromic surveillance tracks groups of symptoms or clinical signs without needing aetiological or pathological confirmation. This approach effectively bridges precise case definitions with infection rate reporting and measurement uncertainty, helping to avoid systematic undercounting of cases (Furness, 2016a,b).
Role of syndromic surveillance of HCAIs in resource-limited settings
Unlike traditional surveillance approaches that rely on laboratory diagnostics as a starting point for HCAI surveillance, syndromic surveillance offers distinct advantages in resource-limited settings (Shenoy and Branch-Elliman, 2023).
In settings where laboratory diagnostic capacities are underdeveloped, syndromic surveillance reduces the need for extensive testing of each suspected case, thereby easing the burden on laboratories (May et al., 2011). This, in the long run, conserves resources and enables prioritized testing for confirming and characterizing outbreaks in health facilities. Additionally, monitoring syndromes rather than confirmed diagnoses allows for more efficient resource allocation based on observed syndromes, even before specific pathogens are identified (European Commission, 2012). By targeting surveillance efforts on patients at higher risk for developing HCAIs, syndromic surveillance may enhance the efficacy and cost-effectiveness of surveillance systems. Patients at increased risk may have specific risk factors such as type of disease, disease severity, medical interventions being utilised (e.g., indwelling catheters, central lines, ventilators) or because the patients are in specific places in the hospital (e.g., ICU, PICU, high-dependency units etc.) (Shenoy, 2023; Puro et al., 2022).
Syndromic surveillance offers a health facility-based early warning system for potential HCAI outbreaks by identifying suspect cases and unusual infection patterns through clusters of symptoms before laboratory confirmation is available (Nsubuga et al., 2011). This approach facilitates earlier identification, detection, and response to outbreaks, allowing for more timely interventions.
Syndromic surveillance systems’ flexibility and adaptability allow for tailoring to the specific needs and resources of a particular setting, from the highest to the lowest levels of the healthcare system where patients are admitted for care (Nsubuga et al., 2011). Thus, syndromic surveillance can be adapted and scaled to monitor various syndromes in health facilities, including those caused by yet-to-be-determined pathogens or those with non-specific symptoms.
Syndromic surveillance can be integrated into the existing healthcare systems, such as national health information systems like electronic medical records and other reporting systems such as the District Health Information System (DHIS-2) platform and the electronic Integrated Disease Surveillance and Response (e-IDSR) (Ministry of Health in Uganda, 2021). This integration can facilitate real-time monitoring of potential HCAIs based on syndromes, enabling prompt response to emerging threats.
The data generated from syndromic surveillance of HCAIs can be utilised for decision-making at various levels of the healthcare system, from individual point of care to public and global health policy (Haque et al., 2020). This data-driven approach enhances the generation of targeted interventions, including quality improvement projects and more informed resource allocation strategies.
Lastly, laboratory-based methods for hospital infection surveillance often lead to systematic underreporting (low sensitivity, high specificity). The low sensitivity, may lead to underreporting of the true burden of HCAIs and limit the ability to implement interventions aimed at reducing HCAIs effectively (Sim et al., 2024; Furness, 2016c). On the other hand, syndromic surveillance is highly sensitive helping to address this issue (Furness, 2016c). Hence, syndromic surveillance should complement laboratory surveillance, to support HCAIs control programs.
Priority syndromes for HCAI surveillance
Table 1 shows examples of syndromes for HCAI surveillance.
Surgical site infections
Surgical site infections (SSIs) are infections that occur within the first 30 days (one month) after surgery or within a year after surgery in patients receiving implants that affect either the skin, muscles, tissues or even organs at the operation site (Padilla et al., 2019; Owens and Stoessel, 2008; Smyth and Emmerson, 2000). Wound discharges (serous, purulent, and bloody), redness, inflammation or swelling around the wound, pain, fever, and separating or gaping wound edges are significant indicators of SSIs within the first 30 days post-surgery (Nasser et al., 2013). These commonly associated signs and symptoms should be prioritized in the syndromic surveillance of SSIs (Monahan et al., 2020; Costabella et al., 2023).
Hospital-acquired pneumonia and acute lung injury
These are usually suspected if a patient develops new signs and symptoms consistent with lower respiratory infections occurring 48 h after admission to the hospital (Russell et al., 2016). These signs and symptoms include productive cough with purulent sputum (off-white, yellow, green, or opaque sputum), fever, tachypnoea (abnormal rapid and difficult breathing), shortness of breath, changes on chest X-rays, and white blood cell count abnormalities (Russell et al., 2016). Ventilator-associated pneumonia (VAP) is the most prevalent type of hospital-acquired pneumonia, with a global incidence of 15.6% and occurring at a rate of 8–28% (10–41.5 per 1,000 ventilator days) in LMICs (Xie et al., 2018; Mazwi et al., 2023). VAP occurs in patients who have been on mechanical ventilation for more than 48 h with associated risk factors including patient characteristics such as being over 65 years old, male sex, smoking, and prolonged mechanical ventilation. Other factors include disorders of consciousness, head trauma, burns, and various comorbidities such as coronary heart disease, diabetes, pre-existing pulmonary disease or chronic obstructive pulmonary disease, HIV infection, and multiple organ system failure. Furthermore, the risk of developing VAP is heightened by prior antibiotic therapy, invasive procedures, and certain gene polymorphisms (Mazwi et al., 2023).
Hospital-acquired diarrheal illnesses
Hospital-acquired diarrheal infections are a significant concern, affecting patients after 48 h of hospitalisation or admission. These infections manifest through several signs and symptoms, such as abdominal cramps, increased stool frequency and consistency (watery stool with mucus), nausea or vomiting, bloody stool, and even fever in some instances (Bhuiyan et al., 2014). The leading cause of hospital-acquired diarrheal illnesses worldwide is Clostridium difficile (CD), with its incidence and severity having risen in recent years (European Centre for Disease Prevention and Control, 2017). It typically affects patients who have been treated with antibiotics, which can disrupt the normal balance of bacteria in the gut, allowing C. difficile to proliferate and cause infection. This infection can lead to symptoms ranging from mild diarrhoea to severe, life-threatening colitis. The onset of CD in hospitals is facilitated by factors such as advanced age, female sex, admission from a long-term acute care facility, immunosuppression, length of hospital stay, and exposure to certain classes of antibiotics (Watson et al., 2018).
Catheter-associated urinary tract infection
Catheter-associated urinary tract infection (CAUTI) is the most prevalent HCAI and a leading cause of secondary bloodstream infections globally (Werneburg, 2022). CAUTI typically occurs in a patient whose urinary bladder is currently catheterized or has been catheterized within the past 48 h. Approximately 12 to 16% of adult hospital inpatients will have an indwelling urinary catheter (IUC) at some point during their hospital stay. Each day the IUC remains in place, the patient’s risk of developing a catheter-associated urinary tract infection increases by 3 to 7% (Magill et al., 2018). The burden of CAUTI in Africa remains significant and is on the rise in sub-Saharan Africa (Asmare et al., 2024a,b; Musinguzi et al., 2019). Symptoms and signs of CAUTI include increased bladder sensation, urgency, frequency, dysuria, pain in the urinary tract, suprapubic tenderness, fever, rigors, altered mental status, malaise, lethargy with no other identified cause, flank pain, and/or costovertebral angle tenderness and risk factors include age, female gender, diabetes, and prolonged catheterization time (Werneburg, 2022).
Hospital-acquired bloodstream infections
Hospital-acquired bloodstream infections (HA-BSI) occur when pathogens enter the bloodstream during a hospital stay, often due to invasive procedures such as central and peripheral vascular line insertions and the associated inadequate infection prevention and control practices. The majority of HA-BSI cases are vascular catheter-related, including central line-associated bloodstream infections and peripheral vascular catheter-related bloodstream infections (Gahlot et al., 2014). However, primary HA-BSIs can also occur in the absence of identifiable sources such as indwelling catheters, requiring comprehensive clinical algorithms that integrate detailed medical history, thorough physical examination, and continuous evaluation and monitoring of clinical signs of infection for accurate identification and diagnosis (Long et al., 2022). With a high mortality rate of up to 42%, HA-BSI pose a significant global burden, particularly affecting patients in intensive care units (ICUs) (Tabah et al., 2023). Signs and symptoms of hospital-acquired bloodstream infections include fever, chills, rapid heart rate, low blood pressure, fatigue, and confusion. Patients may also experience localized symptoms such as redness, swelling, or pain at the catheter insertion site (Gahlot et al., 2014). Sepsis is a life-threatening outcome of HA-BSI, commonly known as hospital-acquired sepsis, and is frequently encountered in high-risk patients in ICUs (Tabah et al., 2023). Sepsis develops when the body’s response to an infection spirals out of control, damaging its own tissues and organs. Several signs and symptoms have been found to be commonly associated with sepsis, including rapid breathing, high fever, rapid heart rate, confusion (altered mental state), and, in some instances, low blood pressure (Page et al., 2015; Drewry et al., 2013).
The additional role of emergency department syndromic surveillance systems for HCAIs in resource-limited settings
HCAIs have been found to be a major threat to patient safety globally, particularly those in emergency departments (Haque et al., 2020; Domer et al., 2021; Nabi et al., 2020), the first point of contact for patients requiring acute care or with injuries in most health facilities (Baugh et al., 2011). Traditional HCAI surveillance methods, which rely on laboratory confirmation and have significant turnaround times, face major limitations in the emergency departments of facilities in resource-limited settings. Early detection of potential HCAI and outbreaks, broad coverage over a wide range of syndromes, and timely collection and analysis of patient syndrome data for quicker response are critical advantages that make emergency department-based syndromic surveillance a preferable approach for HCAI surveillance in resource-limited settings (Hope et al., 2008; King et al., 2004; Hughes et al., 2020; Wu et al., 2008).
If well streamlined, the emergency department-based syndromic surveillance can be scaled up to health facility networks and eventually nationwide networks, standardizing HCAI data collection for facilitating comparison and national-level analysis (Wu et al., 2008). These networks would facilitate resource sharing and exchange of expertise and best practices for implementing and maintaining syndromic surveillance systems. They would also allow real-time analysis of syndromic data, enabling faster identification and response to potential outbreaks across facilities and regions. In the long run, the data would provide valuable insights into HCAI trends, informing public health policy and resource allocation for prevention and control. However, establishing these networks requires careful consideration of factors such as data accuracy, completeness of patient information, capacity for data interpretation, non-specificity, distinguishing between HCAIs and other infections, development of standardised data collection tools, and ensuring the necessary information technology infrastructure is in place (Government of Saskatchewan, 2016).
Recommendations
Maximisation of emergency department-based syndromic surveillance systems for HCAIs in resource-limited settings requires strategic, tailored interventions and approaches. Standardisation of data collection forms to simplify syndromic data collection is crucial, as it alleviates the burden on already strained healthcare resources (Hughes et al., 2020). These standardised forms, whether paper-based or electronic, should be designed to require minimal time, capacity, and effort from healthcare workers within emergency departments. Additionally, they should also be interoperable with existing infrastructure, such as health facility information and record systems, to ensure streamlined data collection, accuracy and timeliness (Mandl et al., 2004).
The development and rolling out of guidelines and a comprehensive curriculum for emergency department-based syndromic surveillance of HCAIs are critical steps in fostering a culture of proactive identification, detection, and response among healthcare workers. The guidelines should include simplified case definitions for specific priority syndromes relevant to the setting or health facility. The curriculum should be straightforward yet comprehensive, to equip healthcare professionals with the skills to recognize and appropriately report potential HCAI syndromes. Enhancing capacity for emergency department-based syndromic surveillance will also secure leadership and governance support, ensuring the sustainability of the program or system.
It is critical to establish real-time reporting of syndromic data from emergency departments of health facilities to public health authorities. This involves developing clear reporting guidelines, communication channels, and automated systems to streamline data transmission. Enhancing data analysis systems based on these automated systems will enable quick analysis of HCAI syndrome data, facilitating the detection of abnormal patterns or spikes indicative of potential outbreaks. Clear and concise algorithms, along with visualisation tools, should be developed to support this process.
Fostering collaboration and data sharing among health facilities, public health agencies, and relevant stakeholders is fundamental for effective syndromic surveillance. Enhanced data and information sharing and coordination of response efforts strengthen the surveillance system through networks and partnerships and improve access to technical expertise and other shared resources (Nsubuga et al., 2011).
As the syndromic surveillance system and networks are established, continuous monitoring and evaluation of the activities—including data quality, timeliness, and system impact—are crucial for refining and adapting the system (Hughes et al., 2020). This continuous monitoring ensures that the unique challenges and characteristics of the system and local context, like healthcare infrastructural limitations, cultural practices and prevalent HCAIs, are identified early to tailor syndromic surveillance guidelines and response strategies accordingly.
Limitations and challenges of syndromic surveillance systems for HCAIs in resource-limited settings
Syndromic surveillance, while a promising approach for the early detection of HCAIs in resource-limited settings, it faces various challenges and limitations. The systems rely on the detection and interpretation of symptoms, which can lead to misclassification, false positives, and missed diagnoses. Symptoms of HCAIs, such as fever or respiratory distress, often overlap with many infectious and other non-infectious conditions. This is significant in resource-limited settings, where the prevalence of comorbidities, such as non-communicable diseases, may be underestimated or overlooked (Kamvura et al., 2022), complicating accurate diagnosis and reducing the system’s effectiveness. In such cases, the inclusion of laboratory findings becomes essential for accurate diagnosis. Effective detection and interpretation of symptoms of HCAIs requires thorough clinical skills. However, healthcare professionals in resource-limited settings often face heavy workload and time constraints, lack of specialized training, and limited access to diagnostic tools (AbdulRaheem, 2023), increasing the risk of misdiagnosis or delayed identification of HCAIs. Enhancing training for healthcare workers to recognize a wider range of conditions, alongside improving diagnostic support, can help mitigate these challenges and improve the accuracy of syndromic surveillance systems.
Another significant limitation is the common practice of self-medication and delayed presentation to healthcare facilities. In many LMICs, patients often seek over-the-counter treatments for symptoms such as fever or cough, which can mask or alter the clinical presentation of HCAIs. This makes it more difficult for healthcare professionals to accurately diagnose infections based solely on symptoms. Public health education campaigns to raise awareness about the risks of self-medication and the importance of seeking timely medical care, and strengthening community-based health services, including outreach programs and early symptom screening can help mitigate this.
Syndromic surveillance systems for HCAIs, though potentially cost-effective, still require resources for implementation and maintenance. In resource-limited settings, competing health priorities and limited funding may restrict the development and sustainability of such systems. Without adequate financial support, the necessary infrastructure—such as digital tools for real-time data collection or analysis platforms—remains insufficient.
Additional limitations and challenges include the lack of specificity in syndrome definition, data quality concerns (Yoon et al., 2017), time lag between symptom onset and diagnosis, and constraints in the granularity of the data (Todkill et al., 2016). Reporting and selection biases also affect data quality, and it may not be sensitive enough to detect low-incidence infections (Todkill et al., 2016).
Conclusion
Healthcare-associated infections are a significant public health threat, particularly in resource-limited settings. This narrative review advocates for the use of syndromic surveillance to identify potential HCAIs, highlighting its advantages, such as early warnings of possible outbreaks, reduced costs, higher sensitivity leading to better estimation of the burden of HAIs and reduced strain on laboratory resources. Effective emergency department-based HCAI syndromic surveillance relies on the consistent collection of quality data, comprehensive healthcare worker training, real-time reporting, stakeholder collaboration, and ongoing monitoring and evaluation for continuous improvement. Implementing this approach can significantly enhance the capacity to combat HCAIs and improve patient safety in resource-limited settings.
Author contributions
HeM: Conceptualization, Writing – original draft, Writing – review & editing. JW: Conceptualization, Writing – original draft, Writing – review & editing. RK: Conceptualization, Writing – review & editing. HoM: Conceptualization, Writing – review & editing. DanB: Writing – review & editing. DatB: Writing – review & editing. AK: Conceptualization, Writing – review & editing. FK: Conceptualization, Writing – review & editing.
Funding
The author(s) declare that financial support was received for the research, authorship, and/or publication of this article. Several researchers (HM, RK, HM, DMB, AK, and FK) are affiliated with the Wellcome Trust CAMO-Net program [grant ref.: 226692/Z/22/Z].
Conflict of interest
The authors declare that the research was conducted in the absence of any commercial or financial relationships that could be construed as a potential conflict of interest.
Publisher’s note
All claims expressed in this article are solely those of the authors and do not necessarily represent those of their affiliated organizations, or those of the publisher, the editors and the reviewers. Any product that may be evaluated in this article, or claim that may be made by its manufacturer, is not guaranteed or endorsed by the publisher.
References
AbdulRaheem, Y. (2023). Unveiling the significance and challenges of integrating prevention levels in healthcare practice. J. Prim. Care Community Health 14:21501319231186500. doi: 10.1177/21501319231186500
Abubakar, U., Amir, O., and Rodríguez-Baño, J. (2022). Healthcare-associated infections in Africa: a systematic review and meta-analysis of point prevalence studies. J. Pharm. Policy Pract. 15:99. doi: 10.1186/s40545-022-00500-5
Ankunda, R., Musisi, D., Tweheyo, R., and Namusisi, O. (2010). Prevalence and factors associated with hospital acquired infections in Kayunga district hospital, Central Uganda. Int. J. Infect. Dis. 14:e267. doi: 10.1016/j.ijid.2010.02.2080
Asmare, Z., Awoke, T., Genet, C., Admas, A., Melese, A., and Mulu, W. (2024a). Incidence of catheter-associated urinary tract infections by gram-negative bacilli and their ESBL and carbapenemase production in specialized hospitals of Bahir Dar, Northwest Ethiopia. Antimicrob. Resist. Infect. Control 13:10. doi: 10.1186/s13756-024-01368-7
Asmare, Z., Erkihun, M., Abebe, W., Ashagre, A., Misganaw, T., and Feleke, S. F. (2024b). Catheter-associated urinary tract infections in Africa: systematic review and meta-analysis. Infect. Dis. Health 29, 172–179. doi: 10.1016/j.idh.2024.02.005
Australian Commission on Safety and Quality in Health Care (2017). Approaches to surgical site infection surveillance for acute care settings in Australia. Sydney New York: Australian Commission, Editor.
Baugh, C. W., Venkatesh, A. K., and Bohan, J. S. (2011). Emergency department observation units: a clinical and financial benefit for hospitals. Health Care Manag. Rev. 36, 28–37. doi: 10.1097/HMR.0b013e3181f3c035
Berkelman, R. L., Sullivan, P., and Buehler, J. W. (2009). Public health surveillance. Oxford textbook of public health, Volume 2: the methods of public health. Ed. 5 Edn, Oxford University Press. 699–715.
Bhuiyan, M. U., Luby, S. P., Zaman, R. U., Rahman, M. W., Sharker, M. A. Y., Hossain, M. J., et al. (2014). Incidence of and risk factors for hospital-acquired diarrhea in three tertiary care public hospitals in Bangladesh. Am. J. Trop. Med. Hyg. 91, 165–172. doi: 10.4269/ajtmh.13-0484
Boyle, J., and Sparks, R. (2022). Syndromic surveillance to detect disease outbreaks using time between emergency department presentations. Emerg. Med. Australas. 34, 92–98. doi: 10.1111/1742-6723.13907
Chung, W. M., Smith, J. C., Weil, L. M., Hughes, S. M., Joyner, S. N., Hall, E. M., et al. (2015). Active tracing and monitoring of contacts associated with the first cluster of Ebola in the United States. Ann. Intern. Med. 163, 164–173. doi: 10.7326/M15-0968
Costabella, F., Patel, K. B., Adepoju, A. V., Singh, P., Attia Hussein Mahmoud, H., Zafar, A., et al. (2023). Healthcare cost and outcomes associated with surgical site infection and patient outcomes in low-and middle-income countries. Cureus 15:e42493. doi: 10.7759/cureus.42493
Domer, G., Gallagher, T. M., Shahabzada, S., Sotherland, J., Paul, E. N., Kumar, K. N., et al. (2021). “Patient safety: preventing patient harm and building capacity for patient safety” in Contemporary topics in patient safety-volume 1 Ed. Stanislaw P. Stawicki and Michael S. Firstenberg (IntechOpen).
Dramowski, A., Cotton, M., and Whitelaw, A. (2017). Surveillance of healthcare-associated infection in hospitalised south African children: which method performs best? S. Afr. Med. J. 107, 56–63. doi: 10.7196/samj.2016.v107.i1.11431
Drewry, A. M., Fuller, B. M., Bailey, T. C., and Hotchkiss, R. S. (2013). Body temperature patterns as a predictor of hospital-acquired sepsis in afebrile adult intensive care unit patients: a case-control study. Crit. Care 17, 1–11. doi: 10.1186/cc12894
European Centre for Disease Prevention and Control (2017). Surveillance of healthcare-associated infections and prevention indicators in European intensive care units: HAI-Net ICU protocol, version 2.2. Stockholm, Sweden: ECDC.
European Commission (2012). Commission Implementing Decision of 8 August 2012 amending Decision 2002/253/EC laying down case definitions for reporting communicable diseases to the Community network under Decision No. 2119/98/EC of the European Parliament and of the Council (notified under document C (2012) 5538). Off. J. Eur. Union 262:21.
Furness, C. (2016a). Syndromic Surveillance for healthcare-associated infections : JMIR Medical Informatics.
Furness, C. (2016b). “Syndromic Surveillance for healthcare-associated infections” in InfectionControl.tips. ed. A. Duong . (Accessed July 17, 2024).
Furness, C. Syndromic Surveillance for healthcare-associated infections. (2016c). Available at: https://infectioncontrol.tips/2016/02/15/syndromic-surveillance-for-healthcare-associated-infections/.
Gahlot, R., Nigam, C., Kumar, V., Yadav, G., Anupurba, S., Gahlot, R., et al. (2014). Catheter-related bloodstream infections. Int. J. Crit. Illn. Inj. Sci. 4, 162–167. doi: 10.4103/2229-5151.134184
Government of Saskatchewan (2016). Clostridium difficile Infection (CDI) Surveillance Protocol: Saskatchewan. Regina, Canada: Government of Saskatchewan.
Greco, D., and Magombe, I. (2011). Hospital acquired infections in a large north Ugandan hospital. J. Prev. Med. Hyg. 52, 55–58.
Haque, M., McKimm, J., Sartelli, M., Dhingra, S., Labricciosa, F. M., Islam, S., et al. (2020). Strategies to prevent healthcare-associated infections: a narrative overview. Risk Manag. Healthc. Policy 13, 1765–1780. doi: 10.2147/RMHP.S269315
Haque, M., Sartelli, M., McKimm, J., and Abu Bakar, M. B. (2018). Health care-associated infections–an overview. Infect. Drug. Resist. 11, 2321–2333. doi: 10.2147/IDR.S177247
Hearn, P., Miliya, T., Seng, S., Ngoun, C., Day, N. P. J., Lubell, Y., et al. (2017). Prospective surveillance of healthcare associated infections in a Cambodian pediatric hospital. Antimicrob. Resist. Infect. Control 6, 1–9. doi: 10.1186/s13756-017-0172-5
Hope, K., Merritt, T., Eastwood, K., Main, K., Durrheim, D. N., Muscatello, D., et al. (2008). The public health value of emergency department syndromic surveillance following a natural disaster. Commun. Dis. Intell. 32, 92–94.
Hughes, H. E., Edeghere, O., O’Brien, S. J., Vivancos, R., and Elliot, A. J. (2020). Emergency department syndromic surveillance systems: a systematic review. BMC Public Health 20, 1–15. doi: 10.1186/s12889-020-09949-y
Kamvura, T. T., Dambi, J. M., Chiriseri, E., Turner, J., Verhey, R., and Chibanda, D. (2022). Barriers to the provision of non-communicable disease care in Zimbabwe: a qualitative study of primary health care nurses. BMC Nurs. 21:64. doi: 10.1186/s12912-022-00841-1
Katz, R., May, L., Baker, J., and Test, E. (2011). Redefining syndromic surveillance. J. Epidemiol. Glob. Health 1, 21–31. doi: 10.1016/j.jegh.2011.06.003
Kawana, A., Teruya, K., Kirikae, T., Sekiguchi, J. I., Kato, Y., Kuroda, E., et al. (2006). “Syndromic surveillance within a hospital” for the early detection of a nosocomial outbreak of acute respiratory infection. Jpn. J. Infect. Dis. 59, 377–379. doi: 10.7883/yoken.JJID.2006.377
King, C.-C., Shih, F. Y., Yen, M. Y., Hu, F. C., Wu, J. S., Chang, F. K., et al. (2004). Syndromic surveillance of infectious diseases in Taiwan—before and after the challenges of severe acute respiratory syndrome (SARS). MMWR Morb. Mortal Wkly. Rep. 53:245.
Ling, M. L., Apisarnthanarak, A., and Madriaga, G. (2015). The burden of healthcare-associated infections in Southeast Asia: a systematic literature review and meta-analysis. Clin. Infect. Dis. 60, 1690–1699. doi: 10.1093/cid/civ095
Long, S. S., Prober, C. G., Fischer, M., and Kimberlin, D. (2022). Principles and practice of pediatric infectious diseases : Elsevier Health Sciences.
Magill, S. S., Dumyati, G., Ray, S. M., and Fridkin, S. K. (2015). Evaluating epidemiology and improving surveillance of infections associated with health care, United States. Emerg. Infect. Dis. 21, 1537–1542. doi: 10.3201/eid2109.150508
Magill, S. S., O'Leary, E., Janelle, S. J., Thompson, D. L., Dumyati, G., Nadle, J., et al. (2018). Changes in prevalence of health care–associated infections in U.S. hospitals. N. Engl. J. Med. 379, 1732–1744. doi: 10.1056/NEJMoa1801550
Maki, G., and Zervos, M. (2021). Health care–acquired infections in low-and middle-income countries and the role of infection prevention and control. Infect. Dis. Clin. 35, 827–839. doi: 10.1016/j.idc.2021.04.014
Mandl, K. D., Overhage, J. M., Wagner, M. M., Lober, W. B., Sebastiani, P., Mostashari, F., et al. (2004). Implementing syndromic surveillance: a practical guide informed by the early experience. J. Am. Med. Inform. Assoc. 11, 141–150. doi: 10.1197/jamia.M1356
Marchetti, A., and Rossiter, R. (2013). Economic burden of healthcare-associated infection in US acute care hospitals: societal perspective. J. Med. Econ. 16, 1399–1404. doi: 10.3111/13696998.2013.842922
May, L., Chretien, J. P., and Pavlin, J. A. (2009). Beyond traditional surveillance: applying syndromic surveillance to developing settings-opportunities and challenges. BMC Public Health 9, 1–11. doi: 10.1186/1471-2458-9-242
May, L., Katz, R. L., Test, E., and Baker, J. (2011). Applications of syndromic surveillance in resource poor settings. World Med. Health Policy 3, 1–29. doi: 10.2202/1948-4682.1206
Mazwi, S., van Blydenstein, S. A., and Mukansi, M. (2023). Ventilator-associated pneumonia in an academic intensive care unit in Johannesburg, South Africa. Afr. J. Thorac. Crit. Care Med. 29, 158–164. doi: 10.7196/AJTCCM.2023.v29i4.154
McNamara, L. A. (2016). Ebola Surveillance—Guinea, Liberia, and Sierra Leone. MMWR Suppl. 65, 35–43. doi: 10.15585/mmwr.su6503a6
Ministry of Health in Uganda (2021). National technical guidelines for integrated disease Surveillance and response. Third Edn. World Health Organisation AFRO.
Monahan, M., Jowett, S., Pinkney, T., Brocklehurst, P., Morton, D. G., Abdali, Z., et al. (2020). Surgical site infection and costs in low-and middle-income countries: a systematic review of the economic burden. PLoS One 15:e0232960. doi: 10.1371/journal.pone.0232960
Monegro, A. F., Muppidi, V., and Regunath, H. (2017). Hospital-acquired infections, StatPearls Publishing LLC, 29.
Musinguzi, B., Kabajulizi, I., Mpeirwe, M., Turugurwa, J., and Kabanda, T. (2019). Incidence and etiology of catheter associated urinary tract infection among admitted patients at Kabale regional referral hospital, South Western Uganda. Adv. Infect. Dis. 9, 183–196. doi: 10.4236/aid.2019.93014
Nabi, J., Akhter, Y., and Tabassum, N. (2020). Health care associated infections: a global threat. Epidemiol. Transm. Infect. Dis. 137–145.
Nasser, A., Zhang, X., Yang, L., Sawafta, F. J., and Salah, B. (2013). Assessment of surgical site infections from signs & symptoms of the wound and associated factors in public hospitals of Hodeidah City, Yemen. Int. J. Appl. 3, 101–110.
National Academies of Sciences, Engineering, and Medicine (2007). Global infectious disease surveillance and detection: assessing the challenges–finding solutions: workshop summary. Washington, DC: The National Academies Press.
Nsubuga, P., White, M. E., Thacker, S. B., Anderson, M. A., Blount, S. B., Broome, C. V., et al. (2011). Public health surveillance: a tool for targeting and monitoring interventions. The International Bank for Reconstruction and Development / The World Bank, Washington (DC).
Owens, C., and Stoessel, K. (2008). Surgical site infections: epidemiology, microbiology and prevention. J. Hosp. Infect. 70, 3–10. doi: 10.1016/S0195-6701(08)60017-1
Padilla, J. D. V., Reyes, J. D. S., Pineda, D. R., Muñoz, E. A. B., Rodríguez, F. A. O., and Yepes, R. M. B. (2019). Surgical site infection. Gen. Surg. 2, 1–3.
Page, D. B., Donnelly, J. P., and Wang, H. E. (2015). Community-, healthcare-, and hospital-acquired severe Sepsis hospitalizations in the university HealthSystem consortium. Crit. Care Med. 43, 1945–1951. doi: 10.1097/CCM.0000000000001164
Puro, V., Coppola, N., Frasca, A., Gentile, I., Luzzaro, F., Peghetti, A., et al. (2022). Pillars for prevention and control of healthcare-associated infections: an Italian expert opinion statement. Antimicrob. Resist. Infect. Control 11:87. doi: 10.1186/s13756-022-01125-8
Reingold, A. (2003). If syndromic surveillance is the answer, what is the question? Biosecur. Bioterror. 1, 77–81. doi: 10.1089/153871303766275745
Russell, C., Koch, O., Laurenson, I. F., O'Shea, D. T., Sutherland, R., and Mackintosh, C. L. (2016). Diagnosis and features of hospital-acquired pneumonia: a retrospective cohort study. J. Hosp. Infect. 92, 273–279. doi: 10.1016/j.jhin.2015.11.013
Sharp, T. M., Roth, N. M., Torres, J., Ryff, K. R., Rodriguez, N. P., Mercado, C., et al. (2015). Chikungunya cases identified through passive Surveillance and household investigations—Puerto Rico, May 5-august 12, 2014. Pediatr. Infect. Dis. J. 34:375.
Shenoy, E. S., and Branch-Elliman, W. (2023). Automating surveillance for healthcare-associated infections: rationale and current realities (part I/III). Antimicrob. Steward. Healthc. Epidemiol. 3:e25. doi: 10.1017/ash.2022.312
Sim, J. X. Y., Pinto, S., and van Mourik, M. S. M. (2024). Comparing automated surveillance systems for detection of pathogen-related clusters in healthcare settings. Antimicrob. Resist. Infect. Control 13:69. doi: 10.1186/s13756-024-01413-5
Smyth, E., and Emmerson, A. (2000). Surgical site infection surveillance. J. Hosp. Infect. 45, 173–184. doi: 10.1053/jhin.2000.0736
Sosin, D. M. (2003). Syndromic surveillance: the case for skillful investment. Biosecur. Bioterror. 1, 247–253. doi: 10.1089/153871303771861441
Surveillance CNI . (2023). Healthcare-associated infections and antimicrobial resistance in Canadian acute care hospitals, −.C.C.D.R.M.-d.
Tabah, A., Buetti, N., Staiquly, Q., Ruckly, S., Akova, M., Aslan, A. T., et al. (2023). Epidemiology and outcomes of hospital-acquired bloodstream infections in intensive care unit patients: the EUROBACT-2 international cohort study. Intensive Care Med. 49, 178–190. doi: 10.1007/s00134-022-06944-2
Todkill, D., Elliot, A. J., Morbey, R., Harris, J., Hawker, J., Edeghere, O., et al. (2016). What is the utility of using syndromic surveillance systems during large subnational infectious gastrointestinal disease outbreaks? An observational study using case studies from the past 5 years in England. J. Hyg. 144, 2241–2250. doi: 10.1017/S0950268816000480
Vandijck, D., Cleemput, I., Hellings, J., and Vogelaers, D. (2013). Infection prevention and control strategies in the era of limited resources and quality improvement: a perspective paper. Aust. Crit. Care 26, 154–157. doi: 10.1016/j.aucc.2013.07.005
Watson, T., Hickok, J., Fraker, S., Korwek, K., Poland, R. E., and Septimus, E. (2018). Evaluating the risk factors for hospital-onset Clostridium difficile infections in a large healthcare system. Clin. Infect. Dis. 66, 1957–1959. doi: 10.1093/cid/cix1112
Werneburg, G. T. (2022). Catheter-associated urinary tract infections: current challenges and future prospects. Res. Rep. Urol. 14, 109–133. doi: 10.2147/RRU.S273663
World Health Organization (2014). Early detection, assessment and response to acute public health events: Implementation of early warning and response with a focus on event-based surveillance: Interim version : World Health Organization.
Wu, T.-S. J., Shih, F. Y. F., Yen, M. Y., Wu, J. S. J., Lu, S. W., Chang, K. C. M., et al. (2008). Establishing a nationwide emergency department-based syndromic surveillance system for better public health responses in Taiwan. BMC Public Health 8, 1–13. doi: 10.1186/1471-2458-8-18
Xie, J., Yang, Y., Huang, Y., Kang, Y., Xu, Y., Ma, X., et al. (2018). The current epidemiological landscape of ventilator-associated pneumonia in the intensive care unit: a multicenter prospective observational study in China. Clin. Infect. Dis. 67, S153–S161. doi: 10.1093/cid/ciy692
Keywords: healthcare-associated infections (HCAIs), syndromic surveillance, resource-limited settings, emergency department, public health surveillance
Citation: Mwanja H, Waswa JP, Kiggundu R, Mackline H, Bulwadda D, Byonanebye DM, Kambugu A and Kakooza F (2024) Utility of syndromic surveillance for the surveillance of healthcare-associated infections in resource-limited settings: a narrative review. Front. Microbiol. 15:1493511. doi: 10.3389/fmicb.2024.1493511
Edited by:
Eleonora Cella, University of Central Florida, United StatesReviewed by:
Okon Okwong Kenneth, Federal Medical Centre Makurdi, NigeriaShaheen Mehtar, Infection Control Africa Network, South Africa
Copyright © 2024 Mwanja, Waswa, Kiggundu, Mackline, Bulwadda, Byonanebye, Kambugu and Kakooza. This is an open-access article distributed under the terms of the Creative Commons Attribution License (CC BY). The use, distribution or reproduction in other forums is permitted, provided the original author(s) and the copyright owner(s) are credited and that the original publication in this journal is cited, in accordance with accepted academic practice. No use, distribution or reproduction is permitted which does not comply with these terms.
*Correspondence: Herman Mwanja, aGVybWFud2FuamFAZ21haWwuY29t
†These authors have contributed equally to this work and share first authorship