- ICAR-National Research Centre on Pomegranate, Solapur, India
Bacterial blight in pomegranate, caused by Xanthomonas citri pv. punicae (Xcp), is one of the most devastating diseases, leading to substantial economic losses in pomegranate production. Methods for blight management in pomegranate production are scarce and not well established. To date, the major control strategy is targeting the pathogen with antibiotics and copper-based compounds. However, excessive use of antibiotics has resulted in the development of antibiotic resistance in the field population of Xcp. Hence, as a means of eco-friendly and sustainable management of bacterial blight, the use of native endophytes was investigated under field conditions in the current study. Endophytic bacteria were isolated from micro-propagated nodal explants of pomegranate and were identified as Bacillus haynesii, B. tequilensis, and B. subtilis. They were found to produce volatiles that inhibited Xcp growth during in vitro antibiosis assay. GC–MS-based volatile profiling revealed the presence of several bioactive compounds with reported antimicrobial activities. These endophytes (CFU of 108/mL) were then spray-inoculated on leaves of 6-month-old pomegranate plants in the polyhouse. They were found to induce ROS-scavenging enzymes such as catalase and peroxidase. This alteration was a manifestation of host tissue colonization by the endophytes as ROS scavenging is one of the mechanisms by which endophytes colonize the host plants. Furthermore, two-season field trials with endophytes for blight control resulted in a reduction of disease index by 47–68%, which was considerably higher than the reduction due to the chemical immune modulator (2-bromo-2-nitro-1, 3-propanediol) currently being recommended for blight control. In addition, these endophytes also exhibited reduced sensitivity to this immune modulator; thus, the current study advocates the use of B. haynesii, B. subtilis, and B. tequilensis as biocontrol agents for bacterial blight of pomegranate either alone or as a part of integrated disease management.
1 Introduction
Pomegranate (Punica granatum L.), also known as the “Fruit of Paradise,” has edible red arils that are highly rich in anti-oxidants and have proven health benefits (Seeram et al., 2006; Viuda-Martos et al., 2013). These benefits include reduction of inflammation, muscle damage, and an increase of platelets blood levels to name a few. In addition to edible parts, non-edible parts of the tree can also be used commercially owing to their several beneficial properties, for example, peel powder can be used as a food preservative (Lacivita et al., 2021; Giri et al., 2024). Owing to its innumerable benefits and uses, the global pomegranate market is expected to expand to USD 33.86 (by 2028) from USD 24.8 billion (2021). Furthermore, due to its adaptability to a wide range of weather and soil conditions, fresh pomegranate fruit is available in India all throughout the year. Therefore, India has become one of the global leaders in pomegranate production. Currently, India ranks seventh in the production of pomegranate in the world with a total area under cultivation of approximately 275,500 hectares, producing 3,215,000 metric tons of pomegranate fruits (DA& FW, GoI, 2023). Notably, in 2022–2023, 62,280 metric tons of pomegranates worth USD 58.36 million were exported from India (APEDA, 2024). Consequently, pomegranate plantation has played a very significant role in improving the socio-economic scenario of small landholders (2.5 million), particularly in the Indian states of Maharashtra, Karnataka, Gujarat, Rajasthan, and Andhra Pradesh. However, as with any other crop, pomegranate production is threatened by several pests and diseases due to the unavailability of any resistant germplasm.
One of the most devastating diseases of pomegranate is bacterial blight caused by Xanthomonas axonopodis pv. punicae (Syn = Xanthomonas citri pv. punicae: Xcp), which was first reported in India in the 1950s (Hingorani and Mehta, 1952). The bacterial blight symptoms mostly appear on the fruits and leaves, sometimes the stem too, leading to damage to the whole plant and economic losses to the grower (Sharma et al., 2017). In India, bacterial blight, also called oily spot, can result in 60–80% and in some cases 100% losses in fruit yield (Sharma et al., 2022a). Until now, the main strategy for bacterial blight control in pomegranate production relied upon the application of antibiotics and copper-based chemical compounds (Benagi et al., 2012; Sharma et al., 2022b). Extensive use of antibiotics in agriculture may, however, cause a reduction in the efficacy of antibiotics by facilitating the emergence of antibiotic resistance among the pathogens. For instance, Erwinia amylovora has been reported to have developed antimicrobial resistance in a number of geographic locations where antibiotics have been used indiscriminately in apple and pear orchards (Tancos et al., 2016). The impact of streptomycin-resistant E. amylovora in orchards remains a subject of ongoing scientific debate (Förster et al., 2015). Recently, reduced sensitivity to antibiotics and copper compounds in the field populations of pomegranate bacterial blight pathogen has also been reported from India (Krishna et al., 2020; Sharma et al., 2022a). Moreover, the majority of antibiotics are non-specific, meaning they not only act against the target pathogen but may also affect other beneficial bacteria naturally present in or on the plants (Daisley et al., 2022). Consequently, the Government of India issued a draft gazette notification on “Prohibition of Streptocycline (Streptomycin sulfate 90% w/w + Tetracycline hydrochloride 10% w/w) in agriculture and horticulture” dated 17 December 2021, released by the Ministry of Agriculture and Farmer’s Welfare. The notification imposes a ban on the manufacture and import of this product, w.e.f: with effect from 1 February 2022, with the ban on its use set to take effect from 1 January 2024. This gazette was brought out by the government because of the growing concerns over the risk of the development of resistance to these antibiotics in human beings and animals. Thus, the development of efficient and eco-friendly technologies focused on the elimination or reduction of the application of antibiotics in agriculture is highly desirable. In addition, globally the export of residue-free fruits is highly promoted, therefore providing an impetus to find new alternatives for organic production of pomegranate.
One such promising approach is the utilization of microbes for the biological control of pathogens (Berg and Hallmann, 2006; Morales-Cedeño et al., 2021). Biocontrol agents (BCA) can mediate plant protection through direct mechanisms such as the production of secondary metabolites (Pretali et al., 2016) or hydrolytic enzymes that have antagonistic effects on the growth and survival of plant pathogens (Oukala et al., 2021); or indirect mechanism of defense involving the phenomenon of induced systemic resistance (ISR) (Wei et al., 1991), through jasmonic acid/ethylene pathway (Kannojia et al., 2019). ISR induction occurs when microbe-associated molecular patterns (MAMPs) of the BCAs are recognized by the plant/host and a defense response is mounted (Pieterse et al., 2014). This type of resistance is durable because the chances of the development of resistance in a pathogen against this type of resistance are very low as the pathogen does not directly interact with the BCAs or the resistance-stimulating agent (Romanazzi et al., 2016). Consequently, more recently attention has been focused on the beneficial aspects of endophytes and the application of these endophytes as BCAs (Backman and Sikora, 2008; Strobel, 2018; Collinge et al., 2022). Endophytes, derived from the Greek words “endo” meaning “inside” and “phyte” meaning “plant,” are symbiotic groups of microorganisms that colonize the internal tissues of plants asymptomatically (Nair and Padmavathy, 2014; Santoyo et al., 2016). These endophytes, apart from being effective BCAs, also have plant growth-promoting (PGP) activities through (i) production of enzyme 1-Aminocyclopropane-1-carboxylase (ACC) deaminase, which is the precursor to plant growth regulator ethylene, hence reducing the levels of ethylene; (ii) nutrient acquisition such as nitrogen, phosphate, potassium, and iron; and (iii) synthesis of phytohormones such as auxin, cytokinin, and GA (Santoyo et al., 2016). A significant range of endophytic bacteria and fungi have been isolated from a variety of plants and shown to have a number of positive effects on the host plant (Bacon and Hinton, 2007; Manjunatha et al., 2022; Salvi et al., 2022). Such benefits embrace the lessening of chemical fertilizer usage, the enhancement of soil quality, the conservation of the environment, and sustainable agriculture. The well-studied and most abundant organisms isolated from plant tissues belong to Actinobacteria, Proteobacteria, and Firmicutes and include members of the genera Streptomyces, Pseudomonas, Azoarcus, Enterobacter, Burkholderia, Stenotrophomonas, and Bacillus (Malfanova et al., 2013; López et al., 2018).
Horticultural crops, including vegetables and fruits, harbor endophytic bacteria (Pseudomonas and Bacillus), and many of them have been reported to possess antimicrobial properties against a wide range of pathogens (Ray et al., 2016; Pavithra et al., 2021). Despite adequate knowledge of the diversity of bacterial endophytes in fruit crops, studies on their biocontrol potential against pomegranate disease are scarce. In one of the studies, the antagonistic activity of endophytic bacteria or fungi isolated from different wild genotypes or cultivars of pomegranate was tested against Xcp (Karn et al., 2022), and in another study, the endophytes were isolated from pomegranate roots and tested against wilt causing pathogen (Maruti and Sriram, 2021). However, both these studies were preliminary as they focused only on the in vitro antagonism. More recently, in vitro and in planta antagonistic effects of bacterial endophytes against Xcp, the most dreaded bacterial pathogen of pomegranate, were reported (Singh et al., 2023). It was the first report of endophytes isolated from micro-propagated pomegranate plants, and the isolates could inhibit the pathogen in vitro and under polyhouse conditions. However, there are still no reports of utilization of endophytes for biocontrol of Xanthomonas citri pv. punicae under field conditions. Moreover, none of the abovementioned studies deciphered the probable mechanism by which the endophytes exert their antagonistic effect on the pathogen.
Therefore, the current study was undertaken to decipher the probable mechanisms behind the biocontrol action of endophytes and to evaluate their effectiveness against X. citri pv. punicae under field conditions. Specifically, the study aimed to: (i) isolate and identify endophytes with potential biocontrol activity against the bacterial blight pathogen; (ii) assess the antagonistic activity of volatile compounds produced by these endophytes against the pathogen using an in vitro approach, and profile the volatile organic compounds (VOCs) through GC–MS to identify the antimicrobial compounds; (iii) evaluate the alteration in the host’s biochemical response upon endophyte inoculation and confirm in vivo host colonization by the endophytes; (iv) conduct on-field trials to assess the effectiveness of endophytic bacteria against bacterial blight, as well as test the sensitivity of endophytic bacteria to bactericides and copper compounds (used in current practices for blight management) in vitro. Although the results of the present study advocate the use of potential bacterial endophytes for biocontrol of pomegranate bacterial blight under field conditions, it will be important to standardize the mode of application of these endophytes in the future for better results. In addition, there is a need to evaluate the scalability of this endophyte technology for commercial use and its long-term impact on pomegranate production.
2 Materials and methods
2.1 Isolation and selection of endophytic bacteria as biocontrol agent
Bacterial endophytes were isolated from tissue-cultured (TC series) pomegranate plants of Bhagwa variety in our previous study (Singh et al., 2023). Isolated pure colonies were then picked up and maintained as pure culture until further use. The isolates were tested for their antagonistic activity against the bacterial blight pathogen (Xanthomonas citri pv. punicae: Xcp) using in vitro dual culture assays (Supplementary Figure S1A) and under polyhouse conditions. On the basis of the screening results, three effective isolates were selected for further studies in the present investigation. These isolates exhibited equal to or more than 50% inhibition of pathogen growth in vitro, and under polyhouse conditions, they either completely checked the incidence of bacterial blight or reduced the severity of the symptoms. Pure cultures of these isolates have been deposited at a culture repository under the National Biodiversity Act, 2002 with accession numbers NAIMCC-B-03178 to NAIMCC-B-03180.
2.2 Molecular identification of potent endophytic bacteria
Effective bacterial endophytes were identified at the molecular level based on a partial 16S rRNA gene sequence. Cultures grown in nutrient glucose broth (NGB) for 24 h with shaking at 120 rpm at 28 ± 1°C were used for genomic DNA (gDNA) isolation using HiPurATM Bacterial Genomic DNA Purification kit (HiMedia) as per the manufacturer’s instructions. For each isolate, PCR was performed using good quality gDNA and amplified using universal primers for 16 s rRNA region (F-AGAGTTTGATCCTGGCTCAG and R-GGTTACCTTGTTACGACTT) (Patel, 2001) with initial denaturation at 94°C for 4 min, 35 cycles of denaturation at 94°C for 30s, annealing at 50°C for 30s, and extension at 72°C for 1 min, followed by final extension at 72°C for 8 min (Manjunatha et al., 2023).
2.3 Phylogenetic analysis of potent endophytic bacteria
Phylogenetic analysis was carried out to confirm the identity and understand the genetic relatedness of the effective isolates with other bacteria belonging to the same genus and species. The 16 s rRNA region amplified from bacterial endophytes and deposited at the GenBank NCBI with accession numbers ON629736, KY575578, and KY575582, along with reference sequences downloaded from the database was aligned using the MUSCLE algorithm. BLASTn search, limiting the search to type material, was performed, and the reference sequence with the maximum similarity was included in the phylogenetic analysis. Neighbor-joining trees were drawn, evolutionary distances were computed using the Tamura-Nei method, 1,000 bootstrap replications were performed, and the consensus tree was rooted to Paenibacillus polymyxa and reported. Since Paenibacillus polymyxa has been found to be phylogenetically distinct from Bacillus spp. (Ash et al., 1993), it was used as an out-group in the current study. This analysis involved 20 nucleotide sequences including three from the isolates examined in the current study. All ambiguous positions were removed for each sequence pair (pairwise deletion option) after which there were a total of 1,496 positions in the final dataset. Phylogenetic analyses were conducted in MEGA11 (Tamura et al., 2021).
2.4 Study of antimicrobial properties of the potent endophytic bacteria
2.4.1 Antibiosis test for production of inhibitory volatile compounds
Inhibitory effects of volatiles secreted by endophytic Bacillus spp. on the growth of Xcp were assayed using a method that prevented direct contact between the two microbes, thereby excluding any contact-dependent inhibitory effects of the endophytic bacteria on the pathogen. In this method, adopted from the study by Dennis and Webster (1971), two nutrient glucose agar (NGA) plates were used in which one of the plates was inoculated with the pathogen and kept on top of the other plate that was inoculated with the endophyte (Supplementary Figure S1B). Volatiles would be emitted by the endophytes in the upward direction; hence, the endophyte plate was kept below, and the pathogen plate was kept on top. For the control setup, one of the plates was inoculated with the pathogen, and the other plate contained un-inoculated NGA only such that if any effect is produced by the media components, it will be visible in the control plates. The plates were sealed with parafilm and incubated at 28 ± 1°C, making sure that the pathogen-containing plate was on top of the endophyte-containing plate and the growth of Xcp was monitored and recorded. The growth of Xcp was monitored in terms of (i) zone of inhibition in the area just above the endophyte-inoculated area, (ii) reduction in cells/colonies, and (iii) reduction in yellow pigment production.
2.4.2 Identification of antimicrobial compounds through gas chromatography–mass spectrometry
Solvent (methanol and ethyl acetate) extracts of Bacillus culture were subjected to GC–MS to identify their volatile organic compound profile. In brief, bacterial isolates were inoculated in nutrient glucose broth (NGB) and incubated at 28 ± 1°C for 24–48 h on a shaker incubator (120 rpm). When the OD600 was 1.5, then 1:105 dilution was performed to obtain an initial inoculum of 200–300 CFU per 10 μL, and 10 μL of the culture was added in 50 mL NGB and incubated again after which volatile collection was performed. In brief, the culture flask was placed with a volatile collection assembly customized to have two lateral openings in an aseptic chamber (Nagrale et al., 2022). Volatile collection was carried out for 4 h, and volatiles were consecutively eluted in the desired solvent (methanol or ethyl acetate) in a final volume of 250 μL (Nagrale et al., 2022). GC–MS-QP2020 (Shimadzu) equipped with a DB5-MS column was used for volatile separation and spectrum analysis. The GC–MS columns and settings were as detailed in Nagrale et al. (2022). Identification of metabolites was performed based on mass spectra similarity with library spectra available at the National Institute of Standards and Technology (NIST-2014 version).
2.5 In planta experiments for the evaluation of host biochemical response upon endophyte inoculation
The effective endophytes were exogenously sprayed onto pomegranate plants, under polyhouse conditions. In brief, endophytes were inoculated in NGB and kept on a shaker incubator at 28°C for 24–48 h. The bacterial suspension was then diluted to 108 cells per ml (OD600 nm = 0.4), of which approximately 25 mL solution was sprayed on each plant. Three biological replicates were used for each treatment, i.e., TC-4, TC-6, and TC-310 inoculated plants as well as the control (not inoculated with any endophyte). Host biochemical response was observed by measuring (i) the activity of enzymes involved in the anti-oxidative defense system, namely catalase, peroxidase (POD), and superoxide dismutase (SOD), and (ii) total phenolic content (TPC) in the host.
2.5.1 Total phenolic content estimation
Total phenols were extracted by crushing 0.5 g of leaf tissue in 5 mL of 30% ethanol. The sample was centrifuged at 10,000 rpm for 20 min, and the supernatant was collected. Five milliliters of 30% ethanol was added to 1 mL supernatant. The solvent was evaporated by incubating in a hot air oven at 60°C for 3 h. The sample was resuspended in water and used for TPC estimation (Waterhouse, 2002). TPC was measured against a standard curve of gallic acid with absorption at 765 nm, and gallic acid equivalents (GAEs)/g of dry plant material were calculated for control (un-inoculated) and treatment (endophyte-inoculated).
2.5.2 Enzyme extraction and activity estimation
Enzymes were extracted by crushing 0.5 g leaf tissue in 10 mL of 0.1% trichloroacetic acid (TCA). The sample was centrifuged at 10,000 rpm for 20 min, and the supernatant was collected as a source of enzymes.
Catalase activity was measured using a hydrogen peroxide (H2O2) standard curve with absorption at 240 nm (Aebi, 1984). One unit of enzyme activity was defined as the amount of enzyme required to decompose 1 μmole of H2O2 per min. Total peroxidase activity (POD) was measured using guaiacol as a standard with absorption at 470 nm (Chance and Maehly, 1955). One unit of enzyme activity was defined as the amount of enzyme required to produce 1 μmole of guaiacol per min.
Superoxide dismutase (SOD) activity was measured following the nitroblue tetrazolium (NBT) reduction method (Dhindsa et al., 1981). The reaction mixture (3 mL) contained 50 mM phosphate buffer, pH 7.8, 26 mM methionine, 20 μM riboflavin, 750 μM NBT, and 1 μM EDTA. After adding enzyme solution (0.1%) and distilled water, the reaction was allowed to run for 15 min under 4,000 lx light. The absorbance was recorded at 560 nm, and one unit of enzyme activity was defined as the amount of enzyme that reduced the absorbance by 50% of the absorbance of no enzyme control.
Fold change, in enzyme activity or accumulation of TPC, between endophyte-inoculated (treatment) and un-inoculated (control) plants was calculated and finally reported.
2.6 Host colonization by the endophytes
The host colonization pattern of the endophytes was checked in vivo. In brief, pomegranate plants were sprayed with endophyte culture [CFU of 108/mL (absorbance at 600 nm ≈ 0.4)] in a polyhouse. Then, 25 mL of endophyte culture was sprayed manually on each plant, and plants that were not inoculated with this endophyte served as control. Leaves from inoculated plants were excised at 24-h intervals, and periodic isolations were taken to check the colonization pattern of the endophytes. The leaf tissues were cut into small pieces and surface-sterilized with sodium hypochlorite (1%) for 30 s and ethanol (70%) for 30 s followed by three rinses with sterile distilled water (Anjum and Chandra, 2015). The sterile leaf tissues were then placed onto nutrient glucose agar media containing Petri dishes. Water after the last rinse was also plated to confirm the endophytic nature of the organism. Isolations in the same way were also taken from control plants. The bacterial colonies obtained in treated plants that were different from those obtained in control plants were only considered; then, these were checked for their morphological similarities with TC-310 that was sprayed onto plants. On these two bases, the frequency of endophyte colonies was measured, and percent recovery was calculated. Furthermore, 16S sequencing was performed to confirm the isolation of endophytes.
2.7 Bio-efficacy of potent endophytic bacteria under field conditions
The effective endophytes were utilized for field trials at research farms of the ICAR-National Research Centre on Pomegranate (Hiraj, Solapur, Maharashtra, India) and compared to the performance of the immune modulator (2-bromo-2-nitro-1, 3-propanediol) and water control. The research plot (H-22) was a 5-year-old (in 2022) pomegranate orchard cv. Bhagwa with a spacing of 4.5 m × 3.0 m. This plot was selected for the study and is dedicated to all ongoing bacterial blight-related studies at the center. No bacterial control sprays were applied throughout the trial period, up to harvesting. The season selected for the study was Mrig bahar (Rainy season), during which there is maximum incidence of bacterial blight naturally due to conducive weather conditions. The age of the plants in the research orchard was 5 years at the time of fruit regulation during the 2022 season trials.
A randomized block design experiment was carried out with five treatments, three replicates, and two plants per replicate. The treatments included T1: TC-4 (B. haynesii), T2: TC-6 (B. subtilis), T3: TC-310 (B. tequilensis), T4: immunomodulator (bactronol), and T5: control (water). Bacterial endophytes were grown in NGB overnight. The next day, the culture was used as a primary culture to inoculate larger volumes of NGB to obtain a final volume of the culture (6 L) with a total CFU of 108/mL or higher (absorbance at 600 nm ≈ 0.4). This culture was sprayed onto plants, with 1 L of the culture or chemical solution sprayed per plant, and 0.3 mL of sticker was added to all solutions. In the case of the control, 1 L of water with 0.3 mL of sticker was sprayed. A total of five sprays, at an interval of 5 days each, were performed after fruit setting and when blight Percent Disease Index (PDI) reached 5% in at least one of the treatments. For observation of disease incidence and severity, five branches with maximum fruits on each branch were labeled, and data were recorded for only these branches. Hence, for one plant, the data were mean of five branches. Observations for disease incidence and severity on leaves and fruits were recorded weekly, and the percent disease index (PDI) was calculated using the formula given below (Sharma et al., 2017). To confirm the pathogen, isolation of Xcp was performed in the laboratory from infected leaves. Such field trials were conducted for 2 consecutive years (2022 and 2023) during Mrig bahar (May–June crop; second or monsoon flowering)
2.8 Chemical sensitivity test
The selected endophytes were tested for their sensitivity to commercially available bactericides and other chemicals using a good diffusion assay (Holder and Boyce, 1994). These chemicals included the immune modulator: 2-bromo-2-nitro-1, 3-propanediol (0.5 g/L); and other copper-based compounds: copper hydroxide (2 g/L) and copper oxychloride (2 g/L). Their doses were chosen as per the recommendation of the center to pomegranate farmers for field application during bacterial blight management. In brief, culture suspension of bacterial colony with a CFU of 108/mL was made in sterile distilled water and spread on NGA plates. A well of 6 mm was dug in the middle of the plate using a sterile cork borer, and the required volume (100 μL) of the test chemical was added to the well. The clear zone around the well, which was devoid of any bacterial growth, i.e., the zone of inhibition (ZOI), was measured using zone scales (HiMedia) after 24 h of growth at 28 ± 1°C in a BOD incubator. Percent inhibition (PI) in the growth of endophytes on the chemical was calculated by the following formula and plotted.
2.9 Statistical analysis
All experiments were carried out with a minimum of three replicates with appropriate controls. For biochemical response, a t-test was carried out to determine whether the difference (fold change) between treatment and control was statistically significant at a p-value of < 0.05. For polyhouse experiments, five plants were taken per treatment considering each plant as one replicate, and the experiment design was completely randomized design (CRD). For field studies, three replicates per treatment were taken with two plants per replicate, and the experiment design was randomized block design (RBD). Statistical analysis was performed using WASP 2.01 (Jangam and Wadekar, 2004), and the critical difference was expressed at a p-value of ≤ 0.05.
3 Results
3.1 Isolation and selection of endophytic bacteria
Microbes isolated from plant endosphere, inhabiting the internal tissues in a symptomless manner, are known as endophytes. Such microbes can also be isolated from tissue-cultured plants and may have a diverse impact on the growth of hosts. In our previous study (Singh et al., 2023), we reported, for the first time, the isolation of endophytes from nodal segments of micro-propagated pomegranate plants. These endophytes (TC series) along with endophytes isolated from field-grown pomegranate plants (EB series) were tested for their antagonistic effects on the growth of bacterial pathogen Xanthomonas citri pv. punicae (Xcp) in vitro and in planta. We observed higher inhibitory effects were shown by TC series endophytes as compared to EB series endophytes both in vitro and in planta, for example, three endophytes, namely TC-4, TC-6, and TC-310 showed equal to or more than 50% inhibition of Xcp in vitro, while none of the EB series endophytes showed equal to or more than 50% inhibition. During in planta antagonistic assays, the endophytes were applied in prophylactic mode, i.e., sprayed prior to pathogen challenge inoculation at an interval of 8 days. Plants inoculated with TC-4 or TC-310 did not show any symptoms, indicating that these endophytes had controlled blight incidence completely, while plants inoculated with TC-6 exhibited lower disease incidence and severity. Based on these results, it was deduced that these three isolates showed varying mechanisms by which pathogen’s growth was inhibited, and therefore in the current study, these isolates and their inhibition mechanisms were investigated further.
3.2 Molecular characterization and identification of potent endophytic bacteria
To understand the nature of the antagonistic mechanism, it is important to first identify the bioagent. In the current study, bacterial endophytes were identified based on BLASTn homology search of 16S rDNA sequence followed by phylogenetic analysis. When we did BLASTn for TC-310 (KY575582), limiting the search to type material, the maximum similarity was obtained with B. tequilensis (NR104919) followed by another B. tequilensis (MN543830). Similarly, for the other isolate TC-4 (ON629736), the maximum similarity was obtained with B. haynesii, and for TC-6 (KY575578), it was obtained with B. subtilis. Although these results are more reliable as they are based on type material confirming the identity, we did not just rely on the BLASTn results. We further performed phylogenetic analysis and as shown in Figure 1, TC-4 grouped separately with B. haynesii isolates, away from the clade containing B. subtilis and B. tequilensis, with a high bootstrap support of 98–99%. Hence, the identity of the isolates was further confirmed based on the phylogenetic relationship between effective endophytes and type strains’ sequences retrieved from the NCBI database (Figure 1). All these sequences have been deposited at GenBank NCBI, and the pure cultures have been deposited at a designated microbial repository for agriculturally important microorganisms (AIMs) under the National Biodiversity Act, 2002 and a member of World Federation of Culture Collections (WFCC; Table 1).
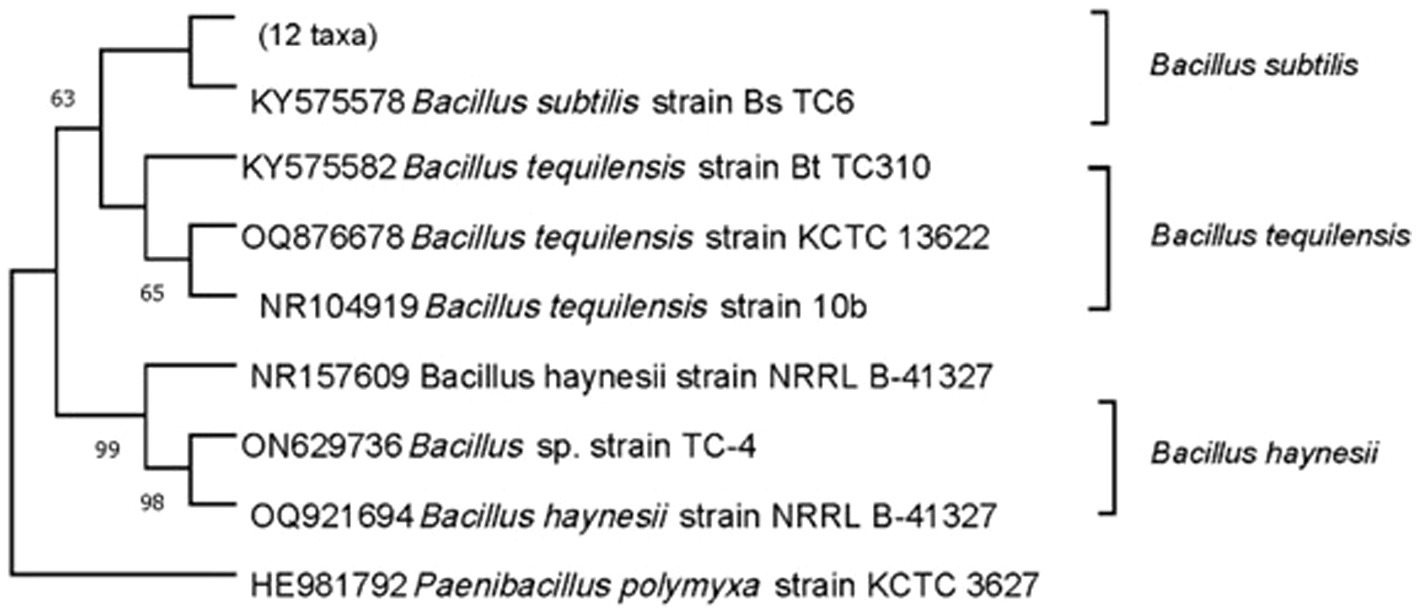
Figure 1. Molecular characterization and identification of endophytic bacteria used as biological agents in the study. Neighbor-joining tree depicting the phylogenetic relationship between effective endophytes (TC-4, TC-6, and TC-310) and type strains’ sequences retrieved from the NCBI database. The Tamura-Nei method was used as the best model for constructing the phylogenetic tree with 1,000 replicates. The tree shown represents the bootstrap consensus tree inferred from 1,000 replicates, and numeric values on the branches indicate bootstrap support values in percentage. Branches corresponding to partitions reproduced in less than 50% bootstrap replicates are collapsed. Paenibacillus polymyxa was used as an out-group.
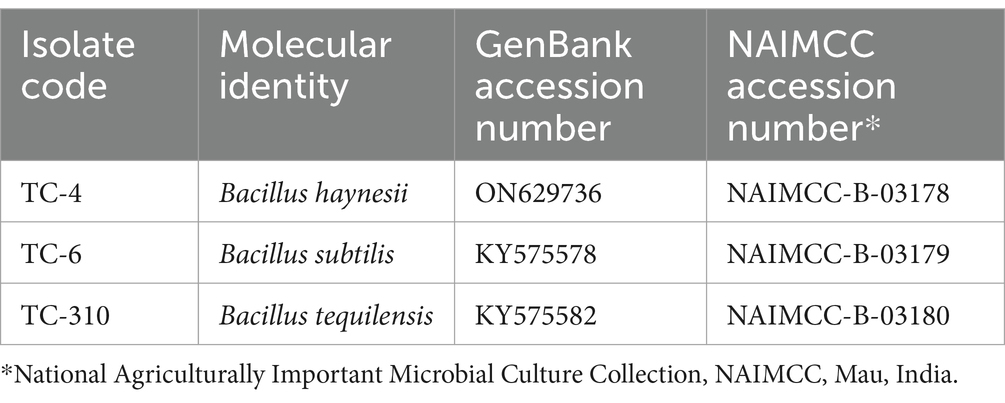
Table 1. Details of the most promising endophytic bacterial bioagents against Xanthomonas citri pv. punicae causing bacterial blight of pomegranate.
3.3 Antimicrobial properties of the potent endophytic bacteria
An antibiosis test was performed using a method that prevented direct contact between the pathogen and endophyte. This means that whatever inhibition was observed in the growth of the pathogen could be due to the volatiles released by the endophytic bacteria. The antibiosis results revealed that the endophytes could inhibit the growth of the pathogen and reduce the production of yellow pigment (Supplementary Figure S1C). Thus, volatile organic compound (VOC) profiling of the isolates was performed using GC–MS. Indeed, GC–MS-based VOC profiles of the isolates (B. subtilis and B. tequilensis) were found to be composed of some really useful bioactive compounds, such as fatty acids and benzimidazole derivatives, which have reported antimicrobial activities. Some of these compounds also exhibit their anti-bacterial activity by inhibiting biofilm production by pathogens and interfering with quorum sensing of the bacteria (Table 2; Supplementary Figure S2). Hexadecanoic acid methyl ester and Pyrrolo[1,2-a] pyrazine-1,4-dione hexahydro-3-(phenylmethyl) were identified in B. subtilis and B. tequilensis. N-acetyl-3-methyl-1,4-diazabicyclo [4.3.0] nonan-2,5-dione was identified in B. tequilensis only, while 2-(p-(Dimethyl amino) phenyl) benzimidazole and 9,9-dimethyl-Xanthene were identified in B. subtilis only.
3.4 Evaluation of host biochemical response upon endophyte inoculation
In our previous study, the effect of endophytes on host physiological parameters was studied. Therefore, in the current study, we further evaluated the effect of the exogenous application of endophytes on the anti-oxidant machinery of the host plants (Figure 2). It was found that the effect of endophytes on different enzyme activities varied. B. haynesii and B. subtilis upregulated catalase and peroxidase activity and downregulated SOD activity, while the trend was reversed in the case of B. tequilensis. The accumulation of total phenolics also differed under the influence of these endophytes. B. haynesii-treated plants accumulated more phenolics as compared to the control, while B. subtilis and B. tequilensis treatment caused a reduction in the levels of total phenolics as compared to the control. This reaffirmed that the endophytes exhibited their antagonistic effects via different mechanisms. Hence, the endophytes can be used to form a consortium to be used as a biocontrol agent against Xcp.
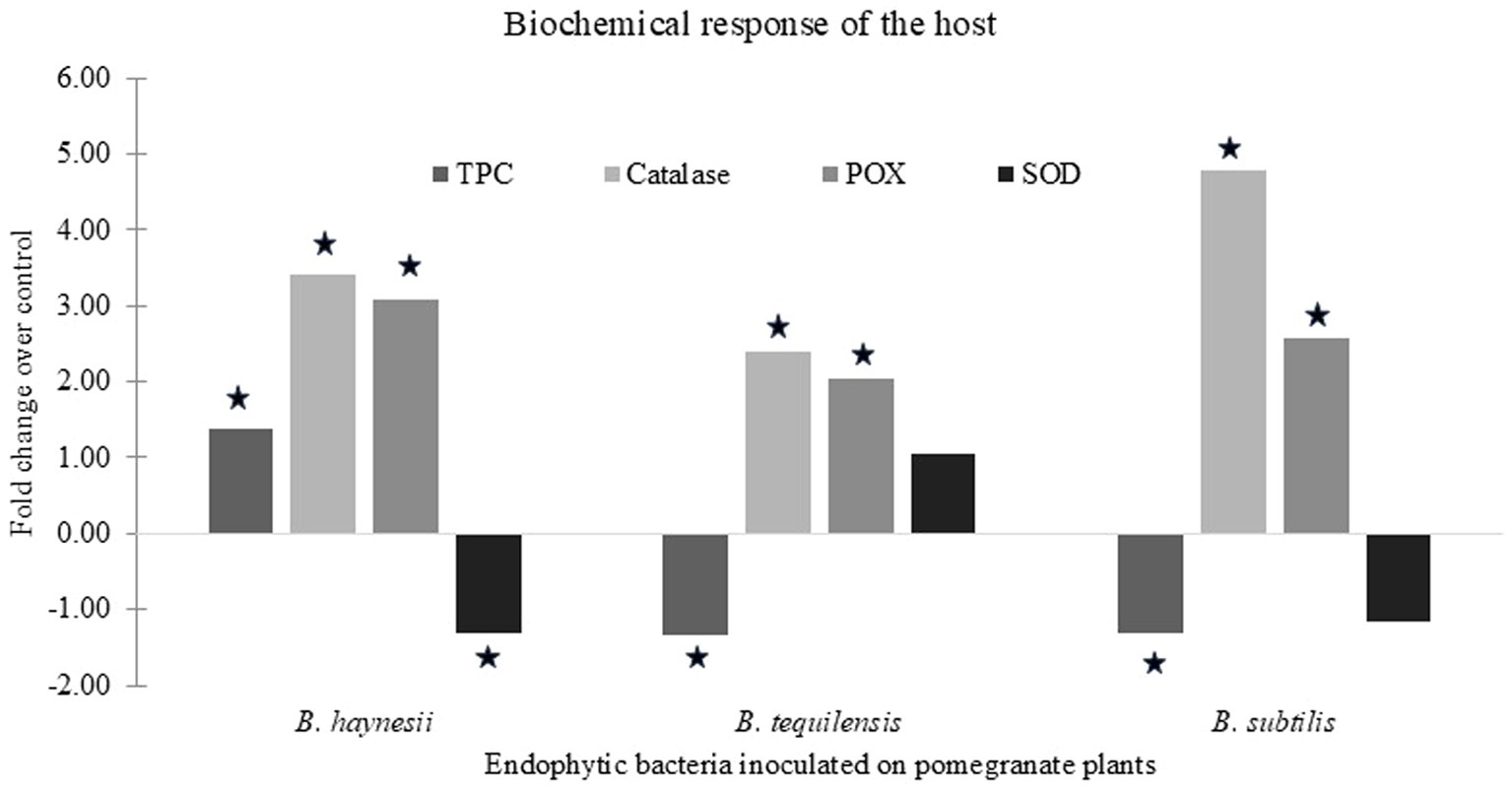
Figure 2. Biochemical response of host upon endophyte inoculation. Fold change in levels of total phenolic content (TPC) and activities of anti-oxidative enzymes such as catalase, peroxidase (POX), and superoxide dismutase (SOD) in pomegranate plants inoculated with endophytes over un-inoculated control plants. Values that are statistically significant (p < 0.05) have been indicated by a (star) sign on the bars.
3.5 Host colonization by the endophytes
To validate any endophyte-mediated response, it is important to understand the colonization pattern. Since all the endophytes altered the host’s biochemical response and host–pathogen interaction, we further validated the colonization of host leaf tissue by the endophytes following artificial inoculation. One of the most effective endophyte B. tequilensis (TC-310) was sprayed onto plants and recovered from leaves after periodic isolations. The endophyte could be recovered up to 7 days post-artificial inoculation of endophytes on host plants indicating their successful colonization. Hence, the observed changes in host biochemical response or reduction in blight incidence mediated by the endophytes can be attributed to the successful colonization by the endophytes (Supplementary Figure S3). However, more elaborate studies are required to illustrate colonization traits of the endophytic bacteria.
3.6 Bio-efficacy of potent endophytic bacteria under field conditions
The current study reports, for the first time, the successful application of endophytes for biocontrol of bacterial blight in pomegranate under field conditions (Supplementary Figure S4). Endophytic bacterial isolates (B. haynesii, B. subtilis, and B. tequilensis) were used for field trials against blight, which were conducted during Mrig bahar (May–June crop; second or monsoon flowering) for 2 consecutive years (2022 and 2023). The percent disease index (PDI) on pomegranate fruits ranged from 2.2 to 36.4% during season I (2022) and 5.6 to 17.7% during season II (2023) in the control (Figure 3). All the endophytes could reduce the disease in the range of 47 to 68% over the control. Interestingly, this reduction of disease over control was higher than the reduction due to chemical immune modulators in both seasons (Supplementary Table S1). PDI on leaves and stems remained below 1% throughout the trials during both seasons.
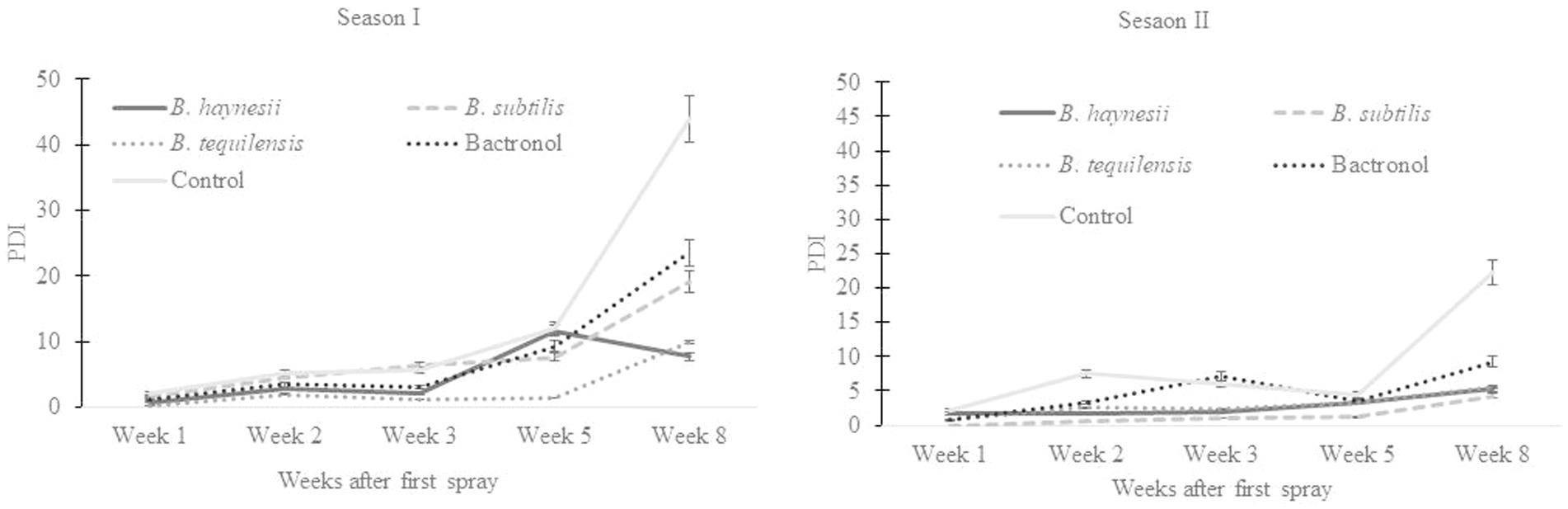
Figure 3. Biocontrol of bacterial blight of pomegranate under field conditions using endophytic bacteria indicating a reduction in disease (PDI: percent disease index) as compared to chemical check [2-bromo-2-nitro-1, 3-propanediol (bactronol)] and un-treated control. (A) Trials during the 2022 rainy season crop. (B) Trials during the 2023 rainy season crop. Values presented are mean of three replicates, and error bars are based on standard deviation.
3.7 Chemical sensitivity of the bacterial endophytes
All three endophytes showed reduced sensitivity with the chemicals such as immune modulator (2-bromo-2-nitro-1, 3-propanediol) and copper-based compounds (copper hydroxide and copper oxychloride) at their recommended doses (Supplementary Figure S5). Bacillus subtilis was found to be the least sensitive to all three chemicals with a maximum inhibition of 23% in 2-bromo-2-nitro-1, 3-propanediol. Similarly, 2-bromo-2-nitro-1, 3-propanediol inhibited the growth of B. tequilensis by 24%, and maximum inhibition was found in B. haynesii (33%). However, none of the chemicals exhibited growth inhibition of 50%, and hence, the endophytes can be called less sensitive to these chemicals (Figure 4).
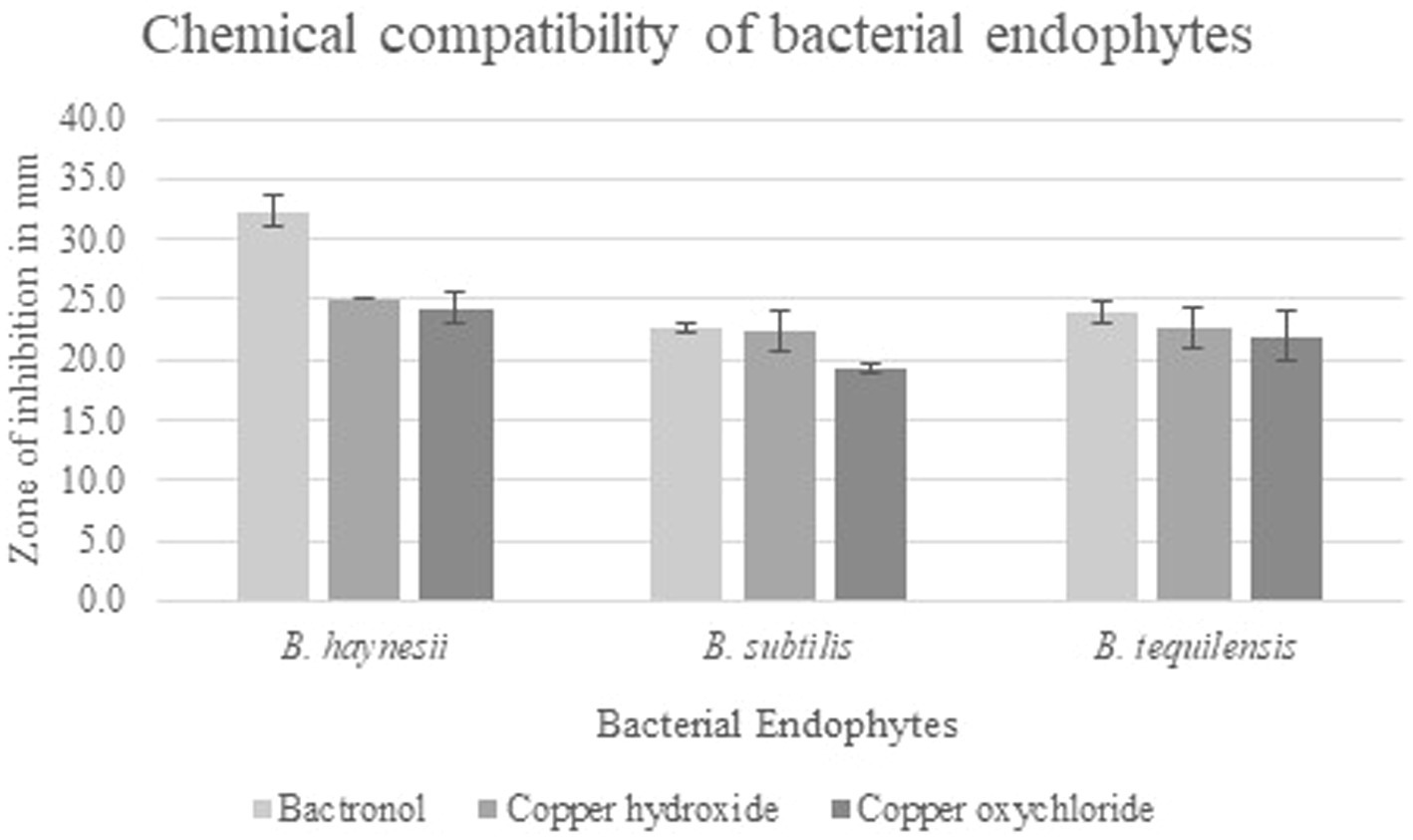
Figure 4. Chemical sensitivity of bacterial endophytes. Growth inhibition in terms of zone of inhibition of three bacterial endophytes B. haynesii, B. subtilis, and B. tequilensis on chemicals such as 2-bromo-2-nitro-1, 3-propanediol (bactronol), copper hydroxide, and copper oxychloride. Values presented are mean of three replicates, and error bars are based on standard deviation.
4 Discussion
Xanthomonas genus containing 27 species can infect more than 400 plant hosts and hence is notorious for causing huge economic losses to agriculture and horticulture, worldwide (Ryan et al., 2011). The use of microbial biological control agents (BCAs) for biocontrol of such phytopathogens is one of the eco-friendly and sustainable approaches (Marin et al., 2019). However, in several examples of biocontrol of Xanthomonas spp., field studies have not been carried out. Therefore, the current study provides the first-ever successful on-field application of endophytic Bacillus spp. to control bacterial blight causing X. citri pv. punicae (Xcp), a deadly pathogen of pomegranate. The study also provides novel insight into the antimicrobial activity of these endophytic Bacillus spp.
Endophytes isolated from micro-propagated pomegranate plants were able to successfully reduce the bacterial blight incidence and severity in pomegranate under polyhouse conditions (Singh et al., 2023). In the current study, the mode of action of these isolates (TC-4, TC-6, and TC-310) that showed maximum inhibition of Xcp was further validated. Based on their genetic relatedness to the type material sequences, these endophytes were identified as Bacillus haynesii (TC-4), B. tequilensis (TC-310), and B. subtilis (TC-6) (Figure 1). While the majority of the studies have reported B. subtilis as a successful biocontrol agent, only a few studies have reported B. tequilensis as a biocontrol agent and that too mostly against fungal pathogens (Li et al., 2018; Xu et al., 2019; Baard et al., 2023). Very recently, Eltokhy et al. (2024) reported the capability of B. haynesii to produce antimicrobial metabolites, which were antagonistic to several multi-drug-resistant pathogens. Nevertheless, to the best of our knowledge, this is the first study where endophytic B. haynesii has been reported as a BCA against any bacterial pathogen in plants.
In the current study, the results of the dual plate antibiosis assays suggested that the endophytic Bacillus spp. produced volatiles that not only inhibited the growth of the pathogen but also reduced the production of the yellow pigment by the pathogen. The yellow xanthomonadin pigments produced by phytopathogenic Xanthomonas spp. are crucial for the epiphytic survival of the pathogen during pathogen–host interaction (Basu and Wallen, 1967; Poplawsky and Chun, 1998; He et al., 2020). Several studies report epiphytic colonization as the first step in Xanthomonas infection cycle. While the pathogen remains on the leaf surface epiphytically, it has to protect itself from UV rays (sunlight) and other oxidative stressors. These yellow pigments also provide protection to the bacteria against such oxidative stress (He et al., 2020). Hence, a reduction in pigment production by Xcp, under the influence of Bacillus spp. as observed in the current study, indicates reduced survival fitness of the pathogen and compromised anti-oxidative activity.
The endophytic Bacillus spp. can have several ways of inhibiting the growth of the target pathogen, including the emission of volatiles (Bruisson et al., 2019) or induction of host defense response (Chandrasekaran and Chun, 2016; Oukala et al., 2021). In the current study, GC–MS-based VOC profiling of the endophytes confirmed the production of several bioactive compounds, by the isolates (Supplementary Figure S2). Additionally, an induction in anti-oxidant enzyme activities along with an increase in phenolic levels (Figure 2) in host cells suggested the possible defense priming of the host by the endophytes. These volatile compounds with known antimicrobial functions included hexadecanoic acid, which has been reported to be synthesized by different species of Bacillus and implicated to be an important component of their anti-fungal activity (Rajaofera et al., 2019); however, in the current study, it could be an important component of their anti-bacterial activity against Xanthomonas. Another compound found in the profiles of the effective endophytes was N-acetyl-3-methyl-1,4-diazabicyclo [4.3.0] nonan-2,5-dione, which belongs to the piperazine group of compounds and has recently been reported in profiles of a strain of B. subtilis that showed anti-fungal activity (Awan et al., 2023). This compound has also been reported as a major component of bioactive profiles of actinobacteria isolated from forest soil (Sharma and Thakur, 2020); however, its direct antimicrobial effects have never been reported earlier. While the antimicrobial activities of other piperazine compounds are known (Jadhav et al., 2017), there is a need to discern the specific antimicrobial effects of N-acetyl-3-methyl-1,4-diazabicyclo [4.3.0] nonan-2,5-dione. A pyrrole derivative, Pyrrolo [1,2-a] pyrazine-1,4-dione hexahydro-3-(phenylmethyl), was observed in the current VOC profiles. This compound has been reported in Bacillus spp. (Gopi et al., 2014) and has proven antimicrobial activities mediated by inhibition of quorum sensing of the pathogen (Kannabiran, 2016; Kiran et al., 2018). Similarly, xanthene derivatives also inhibit the growth of the pathogen by disrupting quorum sensing and biofilm synthesis by the pathogen (Maia et al., 2022); however, specific antimicrobial activity for 9,9-dimethyl xanthene (observed in VOC profiles in the current study) has not been reported yet and calls for further research. Bacillus VOCs can have other biocidal actions by disturbing the cell physiology and cell membrane integrity as they try to enter the aqueous phase of the cell membrane (Massawe et al., 2018). However, more elaborate studies are required to ascertain the mode of action of these inhibitory volatiles observed in the current study.
Non-phytopathogenic bacteria, such as endophytes, trigger plant immunity by recognizing microbe-associated molecular patterns (MAMPs) through the host cells. This results in a burst of reactive oxygen species (ROS) as a part of the host’s first line of defense (Newman et al., 2013). To overcome this burst and gain entry into plant cells, endophytes induce ROS-scavenging mechanisms (Liu et al., 2017). ROS scavenging could be the mechanism by which the endophyte B. tequilensis colonized pomegranate leaves. This is because the activities of all three enzymes, which are crucial in ROS scavenging, were induced upon inoculation with B. tequilensis.
Since there are only a few reports on successful on-field biological control of bacterial blight in pomegranate, the final objective of the study was to ascertain the applicability of endophytes and test their efficacy under field conditions. Many studies report antagonistic activities of biocontrol agents against pathogens in vitro or under polyhouse conditions but fail to extend their results to the field. Earlier researchers have used lactic acid bacteria (LAB) for successful integrated management of pomegranate bacterial blight where they reported the effect of LAB to be at par with the antibiotic streptocycline (Gajbhiye et al., 2023). In the current study, the performance of endophytes was better than or at par with the performance of the immune modulator (2-bromo-2-nitro-1, 3-propanediol) under field conditions where a reduction (in disease) of 47–61% during season I and 53 to 68% in season II was observed. Hence, for the first time, the biocontrol of bacterial blight, with the application of endophytes, is reported under field conditions. Under field conditions, it is difficult to say conclusively whether the sprays (5 sprays at 5-day intervals) acted in the prophylactic mode or curative mode, but the observed reduction in disease index could be attributed to the various underlying antagonistic mechanisms employed by Bacillus spp. The regular sprays could have primed the defense response of the host plants against Xcp, or the emission of the volatiles by the endophytes could have inhibited the growth and epiphytic survival of the pathogen itself by disrupting biofilm formation. Biofilm formation is an important component in Xanthomonas virulence, and strains lacking the ability to form biofilm lose their pathogenicity over the host (Mina et al., 2019).
Moreover, the tested endophytes were less sensitive to chemicals such as 2-bromo-2-nitro-1, 3-propanediol, copper hydroxide, and copper oxychloride at the tested doses. This is noteworthy because once the ban on the antibiotic streptocycline is imposed, these chemicals are the only effective measures to manage bacterial blight in pomegranate. Copper nanoparticles have also been found to be effective in controlling bacterial blight disease in pomegranate (Chikte et al., 2019). Hence, given the lower sensitivity of the bacterial endophytes with these chemicals, the results of the current study support and advocate the use of endophytes, namely, B. haynesii, B. subtilis, and B. tequilensis for bacterial blight management either alone or as a part of integrated disease management practices. Such an integrated management approach, utilizing biocontrol agents and copper, has been recently reported to manage center rot in onion (Koirala et al., 2024). However, the strain of B. subtilis used in that study was sensitive to copper at 250 ppm, while the isolates reported in the current study were compatible with 2,000 ppm of copper compounds. Similarly, the application of fungicide-compatible endophytic Trichoderma spp. has also been recommended for the management of rubber leaf fall disease caused by Corynespora (Seekham et al., 2024). Hence, it will be worth investigating in the future whether the integration of these biocontrol agents with other chemicals results in better bacterial blight management in pomegranate.
Based on the results of the current study, we conclude that endophytic B. haynesii, B. subtilis, and B. tequilensis control the bacterial blight incidence and severity in the following two ways (i) by priming the plant defense response and (ii) by hampering the infection mechanism of the pathogen. The host plant (pomegranate) behaves in a similar way against any alien microorganisms trying to enter its tissues, whether they are pathogens or useful endophytes. When endophytes are inoculated on the plants, the plants recognize their MAMPs and mount the first line of defense, which is manifested as a burst of ROS. To overcome this ROS burst and gain entry into host cells, endophytes induce the activity of ROS-scavenging enzymes such as catalase, peroxidase, and superoxide dismutase. This induction of the anti-oxidative machinery of the host against beneficial endophytes also facilitates defense priming against a subsequent pathogen attack. On the other hand, the beneficial endophytes also secrete volatiles that reduce the yellow pigments produced by Xanthomonas. These pigments are crucial for the epiphytic survival of the pathogen during its infection cycle on the host and also provide protection against oxidative stress; hence, reduction of pigment indicates reduced pathogen survival ability. Some of the volatiles also possess antimicrobial activities, which can directly kill the cells of the pathogen or hamper the infection process via the disruption of biofilm production. However, more elaborate studies are required to evaluate the molecular mechanism behind the induction of host defense response or the endophyte-mediated induced systemic resistance and understand the role of volatiles in inhibiting biofilm production during Xcp–pomegranate interaction.
Data availability statement
The datasets presented in this study can be found in online repositories. The names of the repository/repositories and accession number(s) can be found in the article/Supplementary material.
Author contributions
NM: Conceptualization, Data curation, Formal analysis, Writing – review & editing. SP: Conceptualization, Formal analysis, Writing – review & editing. RA: Formal analysis, Investigation, Methodology, Validation, Writing – original draft. NS: Data curation, Formal analysis, Resources, Writing – review & editing. JS: Conceptualization, Formal analysis, Project administration, Writing – review & editing. MH: Resources, Writing – review & editing. RM: Project administration, Resources, Writing – review & editing.
Funding
The author(s) declare financial support was received for the research, authorship, and/or publication of this article. The authors acknowledge the funding provided by the Indian Council of Agricultural Research (ICAR), New Delhi, as Institution grants to the ICAR-National Research Centre on Pomegranate.
Acknowledgments
The authors acknowledge the GC–MS facility at ICAR-CICR, Nagpur, for carrying out VOC profiling.
Conflict of interest
The authors declare that the research was conducted in the absence of any commercial or financial relationships that could be construed as a potential conflict of interest.
Publisher’s note
All claims expressed in this article are solely those of the authors and do not necessarily represent those of their affiliated organizations, or those of the publisher, the editors and the reviewers. Any product that may be evaluated in this article, or claim that may be made by its manufacturer, is not guaranteed or endorsed by the publisher.
Supplementary material
The Supplementary material for this article can be found online at: https://www.frontiersin.org/articles/10.3389/fmicb.2024.1491124/full#supplementary-material
Footnotes
References
Aebi, H. (1984). “Catalase in vitro” in Methods in enzymology, vol. 105 (Cambridge: Academic Press), 121–126.
Anjum, N., and Chandra, R. (2015). Endophytic bacteria: optimization of isolation procedure from various medicinal plants and their preliminary characterization. Asian J. Pharm. Clin. Res. 8:233.
APEDA. (2024). Available at: https://www.pib.gov.in/PressReleasePage.aspx?PRID=1946623 (Accessed November 2, 2024).
Ash, C., Priest, F. G., and Collins, M. D. (1993). Molecular identification of rRNA group 3 bacilli (Ash, farrow, Wallbanks and Collins) using a PCR probe test. Antonie Van Leeuwenhoek 64, 253–260. doi: 10.1007/BF00873085
Awan, Z. A., Shoaib, A., Schenk, P. M., Ahmad, A., Alansi, S., and Paray, B. A. (2023). Antifungal potential of volatiles produced by Bacillus subtilis BS-01 against Alternaria solani in Solanum lycopersicum. Front. Plant Sci. 13:1089562. doi: 10.3389/fpls.2022.1089562
Baard, V., Bakare, O. O., Daniel, A. I., Nkomo, M., Gokul, A., Keyster, M., et al. (2023). Biocontrol potential of Bacillus subtilis and Bacillus tequilensis against four Fusarium species. Pathogens 12:254. doi: 10.3390/pathogens12020254
Backman, P. A., and Sikora, R. A. (2008). Endophytes: an emerging tool for biological control. Biol. Control 46, 1–3. doi: 10.1016/j.biocontrol.2008.03.009
Bacon, C. W., and Hinton, D. M. (2007). “Bacterial endophytes: the endophytic niche, its occupants, and its utility” in Plant-associated Bacteria. ed. S. S. Gnanamanickam (Dordrecht: Springer).
Basu, P. K., and Wallen, V. R. (1967). Factors affecting virulence and pigment production of Xanthomonas phaseoli var. fuscans. Can. J. Bot. 45, 2367–2374. doi: 10.1139/b67-250
Beč, A., Cindrić, M., Persoons, L., Banjanac, M., Radovanović, V., Daelemans, D., et al. (2023). Novel biologically active N-substituted benzimidazole derived Schiff bases: design, synthesis, and biological evaluation. Molecules 28:3720. doi: 10.3390/molecules28093720
Benagi, V. L., RaviKumar, M. R., and Nargund, V. B. (2012). Threat of bacterial blight on pomegranate in India—mitigation by an integrated approach. In: II International Symposium on the Pomegranate, 113–116. Available at: http://om.ciheam.org/article.php?IDPDF=6917.
Berg, G., and Hallmann, J. (2006). “Control of plant pathogenic fungi with bacterial endophytes” in Microbial root endophytes. Soil biology. eds. B. J. E. Schulz, C. J. C. Boyle, and T. N. Sieber, vol. 9 (Berlin, Heidelberg: Springer).
Bruisson, S., Zufferey, M., L’Haridon, F., Trutmann, E., Anand, A., Dutartre, A., et al. (2019). Endophytes and epiphytes from the grapevine leaf microbiome as potential biocontrol agents against phytopathogens. Front. Microbiol. 10:2726. doi: 10.3389/fmicb.2019.02726
Chance, B., and Maehly, A. C. (1955). Assay of catalase and peroxidases. Methods Enzymol. 2, 764–775. doi: 10.1016/S0076-6879(55)02300-8
Chandrasekaran, M., and Chun, S. C. (2016). Induction of defence-related enzymes in tomato (Solanum lycopersicum) plants treated with Bacillus subtilis CBR05 against Xanthomonas campestris pv. Vesicatoria. Biocontrol. Sci. Technol. 26, 1366–1378. doi: 10.1080/09583157.2016.1205181
Chikte, R. G., Paknikar, K. M., Rajwade, J. M., and Sharma, J. (2019). Nanomaterials for the control of bacterial blight disease in pomegranate: quo vadis? Appl. Microbiol. Biotechnol. 103, 4605–4621. doi: 10.1007/s00253-019-09740-z
Collinge, D. B., Jensen, D. F., Rabiey, M., Sarrocco, S., Shaw, M. W., and Shaw, R. H. (2022). Biological control of plant diseases–what has been achieved and what is the direction? Plant Pathol. 71, 1024–1047. doi: 10.1111/ppa.13555
DA& FW, GoI. (2023). DA& FW, GoI report. Available at: https://desagri.gov.in/wp-content/uploads/2024/09/Agricultural-Statistics-at-a-Glance-2023.pdf (Accessed November 2, 2024).
Daisley, B. A., Chernyshova, A. M., Thompson, G. J., and Allen-Vercoe, E. (2022). Deteriorating microbiomes in agriculture-the unintended effects of pesticides on microbial life. Microbiome Res. Rep. 1:6. doi: 10.20517/mrr.2021.08
Dennis, C., and Webster, J. (1971). Antagonistic properties of species-groups of Trichoderma: II. Production of volatile antibiotics. Trans. Br. Mycol. Soc. 57:41–IN4. doi: 10.1016/S0007-1536(71)80078-5
Dhindsa, R. S., Plumb-Dhindsa, P. A. M. E. L. A., and Thorpe, T. A. (1981). Leaf senescence: correlated with increased levels of membrane permeability and lipid peroxidation, and decreased levels of superoxide dismutase and catalase. J. Exp. Bot. 32, 93–101. doi: 10.1093/jxb/32.1.93
Eltokhy, M. A., Saad, B. T., Eltayeb, W. N., Alshahrani, M. Y., Radwan, S. M., Aboshanab, K. M., et al. (2024). Metagenomic nanopore sequencing for exploring the nature of antimicrobial metabolites of Bacillus haynesii. AMB Express 14:52. doi: 10.1186/s13568-024-01701-8
Förster, H., McGhee, G. C., Sundin, G. W., and Adaskaveg, J. E. (2015). Characterization of streptomycin resistance in isolates of Erwinia amylovora in California. Phytopathology 105, 1302–1310. doi: 10.1094/PHYTO-03-15-0078-R
Gajbhiye, M. H., Bankar, A. V., and Kapadnis, B. P. (2023). Lactic acid bacteria in the management of oily spot disease of pomegranate. Curr. Microbiol. 80:19. doi: 10.1007/s00284-022-03113-y
Giri, N. A., Gaikwad, P., Gaikwad, N. N., Manjunatha, N., Krishnakumar, T., Kad, V., et al. (2024). Development of fiber-enriched muffins using pomegranate peel powder and its effect on physico-chemical properties and shelf life of the muffins. J. Sci. Food Agric. 104, 2346–2358. doi: 10.1002/jsfa.13138
Gopi, M., Dhayanithi, N. B., Devi, K. N., and Kumar, T. T. A. (2014). Marine natural product, Pyrrolo [−a] pyrazine–dione, hexahydro-(C7H10N2O2) of antioxidant properties from Bacillus species at Lakshadweep archipelago. J. Coast Life Med. 2, 632–637. doi: 10.12980/JCLM.2.201414J40
He, Y. W., Cao, X. Q., and Poplawsky, A. R. (2020). Chemical structure, biological roles, biosynthesis and regulation of the yellow xanthomonadin pigments in the phytopathogenic genus Xanthomonas. Mol. Plant-Microbe Interact. 33, 705–714. doi: 10.1094/MPMI-11-19-0326-CR
Hingorani, M. K., and Mehta, P. P. (1952). Bacterial leaf spot of pomegranate. Indian Phytopathol. 5, 55–56.
Holder, I. A., and Boyce, S. T. (1994). Agar well diffusion assay testing of bacterial susceptibility to various antimicrobials in concentrations non-toxic for human cells in culture. Burns 20, 426–429. doi: 10.1016/0305-4179(94)90035-3
Jadhav, R. P., Raundal, H. N., Patil, A. A., and Bobade, V. D. (2017). Synthesis and biological evaluation of a series of 1, 4-disubstituted 1, 2, 3-triazole derivatives as possible antimicrobial agents. J. Saudi Chem. Soc. 21, 152–159. doi: 10.1016/j.jscs.2015.03.003
Jangam, A. K., and Wadekar, P. N. (2004). WASP-web Agri stat package 2.0 (2004). ICAR Research Complex for Goa, Ela, Old Goa, Goa. 403 402, India.
Kannabiran, K. (2016). Bioactivity of Pyrrolo [1, 2-a] pyrazine-1, 4-dione, hexahydro-3-(phenylmethyl)-extracted from Streptomyces sp. VITPK9 isolated from the salt spring habitat of Manipur, India. Asian J. Pharm. 10, 265–270. doi: 10.22377/ajp.v10i04.865
Kannojia, P., Choudhary, K. K., Srivastava, A. K., and Singh, A. K. (2019). “PGPR bioelicitors: induced systemic resistance (ISR) and proteomic perspective on biocontrol” in PGPR amelioration in sustainable agriculture (Sawston, Cambridge: Woodhead Publishing), 67–84.
Karn, M., Sharma, S. K., Sharma, A., and Pal, J. (2022). Isolation and characterization of endophytes against bacterial blight of pomegranate. Int. J. Bio-resource Stress Manag. 13, 683–692. doi: 10.23910/1.2022.2680
Kiran, G. S., Priyadharsini, S., Sajayan, A., Ravindran, A., and Selvin, J. (2018). An antibiotic agent pyrrolo [1, 2-a] pyrazine-1, 4-dione, hexahydro isolated from a marine bacteria Bacillus tequilensis MSI45 effectively controls multi-drug-resistant Staphylococcus aureus. RSC Adv. 8, 17837–17846. doi: 10.1039/C8RA00820E
Koirala, S., Shin, G. Y., Kvitko, B., and Dutta, B. (2024). Integrating biocontrol agents with copper for center rot management in onion. Crop Prot. 184:106785. doi: 10.1016/j.cropro.2024.106785
Krishna, P., Kumar, M. P., Channappa, M., Devanna, P., Singh, K., Eeregowda, P. M., et al. (2020). Antibiotic resilience in Xanthomonas axonopodis pv. Punicae causing bacterial blight of pomegranate. Curr. Sci. 119, 1564–1569. doi: 10.18520/cs/v119/i9/1564-1569
Lacivita, V., Incoronato, A. L., Conte, A., and Del Nobile, M. A. (2021). Pomegranate peel powder as a food preservative in fruit salad: a sustainable approach. Food Secur. 10:1359. doi: 10.3390/foods10061359
Li, H., Guan, Y., Dong, Y., Zhao, L., Rong, S., Chen, W., et al. (2018). Isolation and evaluation of endophytic Bacillus tequilensis GYLH001 with potential application for biological control of Magnaporthe oryzae. PLoS One 13:e0203505. doi: 10.1371/journal.pone.0203505
Liu, H., Carvalhais, L. C., Crawford, M., Singh, E., Dennis, P. G., Pieterse, C. M., et al. (2017). Inner plant values: diversity, colonization and benefits from endophytic bacteria. Front. Microbiol. 8:2552. doi: 10.3389/fmicb.2017.02552
López, S. M. Y., Pastorino, G. N., Franco, M. E. E., Medina, R., Lucentini, C. G., Saparrat, M. C. N., et al. (2018). Microbial endophytes that live within the seeds of two tomato hybrids cultivated in Argentina. Agronomy 8:136. doi: 10.3390/agronomy8080136
Maia, M., Durães, F., Resende, D. I., Szemerédi, N., Gales, L., Martins-da-Costa, P., et al. (2022). Xanthene derivatives targeting bacterial efflux pumps, quorum-sensing, and biofilm formation. Drugs Drug Candidat. 1, 29–42. doi: 10.3390/ddc1010004
Malfanova, N., Lugtenberg, B. J., and Berg, G. (2013). Bacterial endophytes: who and where, and what are they doing there? In: F. J. BrujinDe (ed) Molecular microbial ecology of the rhizosphere. John Wiley & Sons, Inc., Hoboken, New Jersey, pp. 391–403.
Manjunatha, N., Manjunatha, N., Li, H., Sivasithamparam, K., Jones, M. G., Edwards, I., et al. (2022). Fungal endophytes from salt-adapted plants confer salt tolerance and promote growth in wheat (Triticum aestivum L.) at early seedling stage. Microbiology 168:001225. doi: 10.1099/mic.0.001225
Manjunatha, N., Sharma, J., Pokhare, S. S., Chakranarayan, M. G., Agarrwal, R., Gavande, B. S., et al. (2023). Development of simple and quick DNA release protocol for conventional PCR based detection of Xanthomonas axonopodis pv. Punicae causing bacterial blight in pomegranate. Arch. Phytopathol. Plant Protect. 56, 889–902. doi: 10.1080/03235408.2023.2228004
Marin, V. R., Ferrarezi, J. H., Vieira, G., and Sass, D. C. (2019). Recent advances in the biocontrol of Xanthomonas spp. World J. Microbiol. Biotechnol. 35:72. doi: 10.1007/s11274-019-2646-5
Maruti, B., and Sriram, S. (2021). Isolation and in vitro screening of endophytes against wilt pathogen in pomegranate and guava. Mysore J. Agricult. Sci. 55, 181–188.
Massawe, V. C., Hanif, A., Farzand, A., Mburu, D. K., Ochola, S. O., Wu, L., et al. (2018). Volatile compounds of endophytic Bacillus spp. have biocontrol activity against Sclerotinia sclerotiorum. Phytopathology 108, 1373–1385. doi: 10.1094/PHYTO-04-18-0118-R
Mina, I. R., Jara, N. P., Criollo, J. E., and Castillo, J. A. (2019). The critical role of biofilms in bacterial vascular plant pathogenesis. Plant Pathol. 68, 1439–1447. doi: 10.1111/ppa.13073
Morales-Cedeño, L. R., del Carmen Orozco-Mosqueda, M., Loeza-Lara, P. D., Parra-Cota, F. I., de Los Santos-Villalobos, S., and Santoyo, G. (2021). Plant growth-promoting bacterial endophytes as biocontrol agents of pre-and post-harvest diseases: fundamentals, methods of application and future perspectives. Microbiol. Res. 242:126612. doi: 10.1016/j.micres.2020.126612
Nagrale, D. T., Gawande, S. P., Shah, V., Verma, P., Hiremani, N. S., Prabhulinga, T., et al. (2022). Biocontrol potential of volatile organic compounds (VOCs) produced by cotton endophytic rhizobacteria against Macrophomina phaseolina. Eur. J. Plant Pathol. 163, 467–482. doi: 10.1007/s10658-022-02490-1
Nair, D. N., and Padmavathy, S. J. T. S. W. J. (2014). Impact of endophytic microorganisms on plants, environment and humans. Sci. World J. 2014:250693. doi: 10.1155/2014/250693
Newman, M. A., Sundelin, T., Nielsen, J. T., and Erbs, G. (2013). MAMP (microbe-associated molecular pattern) triggered immunity in plants. Front. Plant Sci. 4:139. doi: 10.3389/fpls.2013.00139
Oukala, N., Aissat, K., and Pastor, V. (2021). Bacterial endophytes: the hidden actor in plant immune responses against biotic stress. Plan. Theory 10:1012. doi: 10.3390/plants10051012
Patel, J. B. (2001). 16S rRNA gene sequencing for bacterial pathogen identification in the clinical laboratory. Mol. Diagn. 6, 313–321. doi: 10.1054/modi.2001.29158
Pavithra, G., Manda, R. R., Addanki, V. A., and Srivastava, S. (2021). Evaluation of isolated endophytes, bio-agents and fungicides against anthracnose of chilli. Biopestic. Int. 17, 143–149.
Pieterse, C. M., Zamioudis, C., Berendsen, R. L., Weller, D. M., Van Wees, S. C., and Bakker, P. A. (2014). Induced systemic resistance by beneficial microbes. Annu. Rev. Phytopathol. 52, 347–375. doi: 10.1146/annurev-phyto-082712-102340
Poplawsky, A. R., and Chun, W. (1998). Xanthomonas campestris pv. Campestris requires a functional pigB for epiphytic survival and host infection. Mol. Plant-Microbe Interact. 11, 466–475. doi: 10.1094/MPMI.1998.11.6.466
Pretali, L., Bernardo, L., Butterfield, T. S., Trevisan, M., and Lucini, L. (2016). Botanical and biological pesticides elicit a similar induced systemic response in tomato (Solanum lycopersicum) secondary metabolism. Phytochemistry 130, 56–63. doi: 10.1016/j.phytochem.2016.04.002
Rajaofera, M. J. N., Wang, Y., Dahar, G. Y., Jin, P., Fan, L., Xu, L., et al. (2019). Volatile organic compounds of Bacillus atrophaeus HAB-5 inhibit the growth of Colletotrichum gloeosporioides. Pesticide Biochem. Phys. 156, 170–176. doi: 10.1016/j.pestbp.2019.02.019
Ray, S., Singh, S., Sarma, B. K., and Singh, H. B. (2016). Endophytic Alcaligenes isolated from horticultural and medicinal crops promotes growth in okra (Abelmoschus esculentus). J. Plant Growth Regul. 35, 401–412. doi: 10.1007/s00344-015-9548-z
Romanazzi, G., Sanzani, S. M., Bi, Y., Tian, S., Martínez, P. G., and Alkan, N. (2016). Induced resistance to control postharvest decay of fruit and vegetables. Postharvest Biol. Technol. 122, 82–94. doi: 10.1016/j.postharvbio.2016.08.003
Ryan, R. P., Vorhölter, F. J., Potnis, N., Jones, J. B., Van Sluys, M. A., Bogdanove, A. J., et al. (2011). Pathogenomics of Xanthomonas: understanding bacterium–plant interactions. Nat. Rev. Microbiol. 9, 344–355. doi: 10.1038/nrmicro2558
Salvi, P., Mahawar, H., Agarrwal, R., Kajal,, Gautam, V., and Deshmukh, R. (2022). Advancement in the molecular perspective of plant-endophytic interaction to mitigate drought stress in plants. Front. Microbiol. 13:981355. doi: 10.3389/fmicb.2022.981355
Santoyo, G., Moreno-Hagelsieb, G., del Carmen Orozco-Mosqueda, M., and Glick, B. R. (2016). Plant growth-promoting bacterial endophytes. Microbiol. Res. 183, 92–99. doi: 10.1016/j.micres.2015.11.008
Seekham, N., Kaewsalong, N., Jantasorn, A., and Dethoup, T. (2024). Biological control of Corynespora leaf fall disease in rubber by endophytic Trichoderma spp. under field conditions. Eur. J. Plant Pathol. 170, 207–218. doi: 10.1007/s10658-024-02875-4
Seeram, N. P., Zhang, Y., Reed, J. D., Krueger, C. G., and Vaya, J. (2006). “Pomegranate phytochemicals” in Pomegranates. (Boca Raton: CRC Press), 21–48.
Sharma, J., Maithy, A., Singh, N. V., Mallikarjun,, Manjunatha, N., and Pokhare, S. (2022b). Bimonthly pomegranate advisory for bearing orchards. Solapur: NRC-P, 2022.
Sharma, J., Manjunatha, N., Pokhare, S. S., Patil, P. G., Agarrwal, R., Chakranarayan, M. G., et al. (2022a). Genetic diversity and streptomycin sensitivity in Xanthomonas axonopodis pv. Punicae causing oily spot disease in pomegranates. Horticulturae 8:441. doi: 10.3390/horticulturae8050441
Sharma, J., Sharma, K. K., Kumar, A., Mondal, K. K., Thalor, S., Maity, A., et al. (2017). Pomegranate bacterial blight: symptomatology and rapid inoculation technique for Xanthomonas axonopodis pv. Punicae. J. Plant Pathol. 99, 109–119. doi: 10.4454/JPP.V99I1.3825.S9
Sharma, P., and Thakur, D. (2020). Antimicrobial biosynthetic potential and diversity of culturable soil actinobacteria from forest ecosystems of Northeast India. Sci. Rep. 10:4104. doi: 10.1038/s41598-020-60968-6
Singh, N. V., Sharma, J., Dongare, M. D., Gharate, R., Chinchure, S., Nanjundappa, M., et al. (2023). In vitro and in planta antagonistic effect of endophytic bacteria on blight causing Xanthomonas axonopodis pv. Punicae: a destructive pathogen of pomegranate. Microorganisms 11:5. doi: 10.3390/microorganisms11010005
Strobel, G. (2018). The emergence of endophytic microbes and their biological promise. J. Fungi 4:57. doi: 10.3390/jof4020057
Tamura, K., Stecher, G., and Kumar, S. (2021). MEGA 11: molecular evolutionary genetics analysis version 11. Mol. Biol. Evol. 38, 3022–3027. doi: 10.1093/molbev/msab120
Tancos, K. A., Villani, S., Kuehne, S., Borejsza-Wysocka, E., Breth, D., Carol, J., et al. (2016). Prevalence of streptomycin-resistant Erwinia amylovora in New York apple orchards. Plant Dis. 100, 802–809. doi: 10.1094/PDIS-09-15-0960-RE
Viuda-Martos, M., Pérez-Álvarez, J. A., Sendra, E., and Fernández-López, J. (2013). In vitro antioxidant properties of pomegranate (Punica granatum) peel powder extract obtained as coproduct in the juice extraction process. J. Food Process. Preserv. 37, 772–776. doi: 10.1111/j.1745-4549.2012.00715.x
Waterhouse, A. L. (2002). Determination of total phenolics. Curr. Protocol Food Anal. Chem. 6:I1. doi: 10.1002/0471142913.fai0101s06
Wei, G., Kloepper, J. W., and Tuzun, S. (1991). Induction of systemic resistance of cucumber to Colletotrichum orbiculare by select strains of plant growth-promoting rhizobacteria. Phytopathology 81, 1508–1512. doi: 10.1094/Phyto-81-1508
Wright, J. B. (1949). Histamine antagonists. V. Some 1-(β-Dimethylaminoethyl)-benzimidazole derivatives. J. Am. Chem. Soc. 71, 2035–2037. doi: 10.1021/ja01174a038
Keywords: Bacillus spp., biological control agents, integrated disease management, Punica granatum L., volatile organic compounds, Xanthomonas citri pv. punicae
Citation: Manjunatha N, Pokhare SS, Agarrwal R, Singh NV, Sharma J, Harsur MM and Marathe RA (2024) Possible biocontrol of bacterial blight in pomegranate using native endophytic Bacillus spp. under field conditions. Front. Microbiol. 15:1491124. doi: 10.3389/fmicb.2024.1491124
Edited by:
Debasis Mitra, Graphic Era University, IndiaReviewed by:
Rajeshwari Negi, Eternal University, IndiaSakshi Tewari, YMCA University of Science and Technology, India
Copyright © 2024 Manjunatha, Pokhare, Agarrwal, Singh, Sharma, Harsur and Marathe. This is an open-access article distributed under the terms of the Creative Commons Attribution License (CC BY). The use, distribution or reproduction in other forums is permitted, provided the original author(s) and the copyright owner(s) are credited and that the original publication in this journal is cited, in accordance with accepted academic practice. No use, distribution or reproduction is permitted which does not comply with these terms.
*Correspondence: N. Manjunatha, bWFuanVpZ2ZyaTE3QGdtYWlsLmNvbQ==
†Present address: Nripendra V. Singh, ICAR-Indian Agricultural Research Institute, New Delhi, India