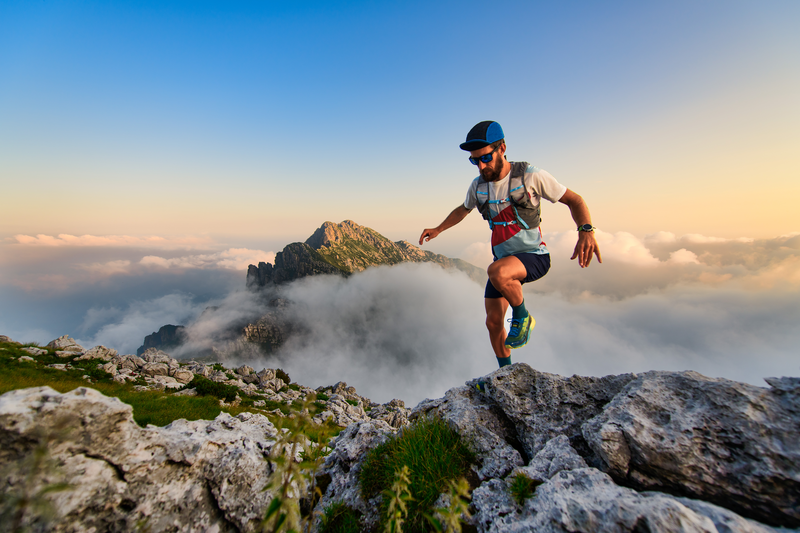
95% of researchers rate our articles as excellent or good
Learn more about the work of our research integrity team to safeguard the quality of each article we publish.
Find out more
ORIGINAL RESEARCH article
Front. Microbiol. , 04 December 2024
Sec. Terrestrial Microbiology
Volume 15 - 2024 | https://doi.org/10.3389/fmicb.2024.1487619
This article is part of the Research Topic Soil Microbial Communities to Promote Suppressiveness against Soil-Borne Pathogens and Diseases View all 9 articles
Background: Inter-cropping is a reasonable planting pattern between different plants. Inter-cropping of Torreya grandis with Polygonatum sibiricum is a relatively mature planting pattern in China, which has been applied to improve soil ecological environment and reduce the occurrence of pests and diseases in China. However, there is currently limited knowledge on the response of soil microbial communities to this practice.
Methods: In this study, we employed Illumina MiSeq sequencing coupled with Functional Annotation of Prokaryotic Taxa (FAPROTAX) and Fungi Functional Guild (FUNGuild) analyses to investigate the dynamic changes in soil microbial communities across seven treated groups [the bulk soil of the T. grandis inter-cropping with P. sibiricum (IB), the bulk soil for mono-cropping of P. sibiricum (PB), the bulk soil for mono-cropping of T. grandis (TB), the P. grandis rhizosphere soil of the T. grandis inter-cropping with P. sibiricum (IPR), the rhizosphere soil for mono-cropping of P. sibiricum (PR), the T. grandis rhizosphere soil of the T. grandis inter-cropping with P. sibiricum (ITR), and the rhizosphere soil for mono-cropping of T. grandis (TR)].
Results: The results showed that the rhizosphere soil of Torreya-Polygonatum inter-cropping exhibited higher microbial community richness, diversity and evenness than mono-cropping (ITR > TR, IPR > PR). Inter-cropping increased the abundance of Micrococcaceae, Xanthobacteraceae, Saitozyma, while decreased Bacillus, Burkholderia, Streptomyces, Cladosporium, and Gibberella significantly of the rhizosphere soil of T. grandis. Further, the abundance of pathogens, such as Fusarium and Neocosmospora, was higher in mono-cropping samples compared to inter-cropping. There existed distinct variations in bacterial and fungal communities among all groups except for IB and TB. The FAPROTAX and FUNGuild analyses results indicated that inter-cropping significantly enhanced soil microbial function associated with nutrient cycling and exhibited a consistent increase in the relative abundance of nitrogen-cycling and carbon-cycling bacteria, and decreased the abundance of plant pathogen guild in the inter-cropping sample ITR compared to the mono-cropping TR.
Conclusion: Our findings suggest that T. grandis inter-cropping with P. sibiricum not only enhance the diversity of soil microbial communities, but also improve the nitrogen and carbon cycling functions. In addition, the inter-cropping can effectively reduce the relative abundance of some soil-borne pathogens for T. grandis and P. sibiricum, indicating that this intercropping method may alleviate the impact of pathogens on crops, thus providing assistance for plant disease prevention and sustainable management.
Plant rhizosphere communicating with soil microorganisms represents the important site for the interaction between microbes and plant hosts (Hinsinger et al., 2009). The host can reshape the microbial community structure through root exudates and soil composition has effect on rhizosphere microbial communities (Haichar et al., 2008; Berg and Smalla, 2009). Higher microbial diversity enhances nutrient exchange and resource utilization, and increases plant productivity (Tedersoo et al., 2020). The reduction of soil biodiversity, however, significantly impairs the functioning of ecosystems (Wagg et al., 2014).
Plant cropping practice impacts plant growth, soil physicochemical properties and microbial community. Long-term cropping of the same plant species leads to the decrease of soil enzyme activity, deterioration of physiochemical properties, and change of the soil microbial structure and diversity (Machado et al., 2007; Du et al., 2017; Yu et al., 2017; Bai et al., 2019; Pervaiz et al., 2020). It thus reduces plant growth and yield, and promotes damage from pests, diseases and weeds (Zhang et al., 2017; Zeng et al., 2020). In contrast, inter-cropping of different plant species can improve soil micro-ecological environment by recruiting beneficial microbiota (Li et al., 2007; Wang et al., 2021). The mustard inter-cropping with cucumber increased the diversity of soil microbiota and the abundance of beneficial microorganisms (Li and Wu, 2018). The peanut-tobacco inter-cropping improved soil ecology and microbial structure, increasing the number of beneficial bacteria and decreasing the number of pathogenic bacteria (Gao et al., 2019). Plant inter-cropping can also inhibit the spread of soil-borne diseases such as Fusarium wilt and Phytophthora blight by changing the content and composition of root exudates and increasing the diversity of rhizosphere microbial community structure (Yu et al., 2019; Lv et al., 2020; Zhang et al., 2020; Song et al., 2022).
Torreya grandis cv Merrillii (Taxaceae, Torreya) is a precious economic tree cropped in subtropical mountains in China with various uses such as for fruit, medicine, oil, wood and ornamental purposes. The root system of T. grandis exhibits characteristics such as shallow depth, wide distribution, and strong adaptability to drought and nutrient-poor soil conditions. Furthermore, the afforestation process minimizes any potential damage to existing vegetation, rendering it highly suitable for cultivation in forested areas (Chen and Jin, 2019; Chen and Chen, 2021). The perennial herbaceous plant, Polygonatum sibiricum (Asparagaceae Polygonatum) is a perennial tuberous herb with shallow roots, which can be taken as food, and is rich in chemical components beneficial to human health (Kato et al., 1994; Xian et al., 2012; Luo et al., 2018). Inter-cropping of T. grandis with P. sibiricum has thus been applied in China for efficient land utilization and yield increase, thereby facilitates the rapid establishment of young Torreya forests, thereby expediting forest formation while also yielding short-term economic benefits, and effectively mitigating soil and water erosion resulting from excessive planting (Luo et al., 2023). However, its effect on soil microbial community remains unexplored.
In this study, we collected the rhizosphere and bulk soil samples of inter-cropping and mono-cropping of T. grandis and P. sibiricum. The high-throughput Illumina MiSeq sequencing coupled with Functional Annotation of Prokaryotic Taxa (FAPROTAX) and Fungi Functional Guild (FUNGuild) analyses were used to address: (a) The effects of Torreya-Polygonatum inter-cropping on the composition of rhizosphere and bulk soil bacterial and fungal communities comparing to mono-cropping; (b) The response and function of Torreya-Polygonatum inter-cropping to bacterial and fungal communities.
The study areas were located in Chun'an County, Hangzhou, China (29°11′46″N, 118°42′10″E), from where there is subtropical monsoon climate, characterized by high temperature and rainy summer, and mild winter with little rain. The meteorological parameters there were obtained from the China Meteorological Data Service website (http://data.cma.cn/). The average annual sunshine and precipitation are 1,850.3 h and 1,515.1 mm with average temperature of 17.2°C ranging from −5.4°C in winter to 37.6°C in summer. The T. grandis plantation was established in 2011, and it has been inter-cropped with P. sibiricum since 2015 (Supplementary Table 1).
Seven different sets of samples including the rhizosphere and bulk soil were collected from inter-cropping and mono-cropping of T. grandis and P. sibiricum (Figure 1): the bulk soil of the T. grandis inter-cropping with P. sibiricum (IB), the bulk soil for mono-cropping of P. sibiricum (PB), the bulk soil for mono-cropping of T. grandis (TB), the P. grandis rhizosphere soil of the T. grandis inter-cropping with P. sibiricum (IPR), the rhizosphere soil for mono-cropping of P. sibiricum (PR), the T. grandis rhizosphere soil of the T. grandis inter-cropping with P. sibiricum (ITR), and the rhizosphere soil for mono-cropping of T. grandis (TR).
Figure 1. Schematic diagram showing different cropping practice. (a) Polygonatum sibiricum mono-cropping. (b) Torreya grandis mono-cropping. (c) Inter-cropping of P. sibiricum and T. grandis.
The soil samples were collected during the maturation season of T. grandis and P. sibiricum (September 22, 2022), and arranged in a randomized block design (3 replications). The bulk soil samples were collected using four-point sampling method to select four square points at a distance of 10 cm away from the plant roots, and passed through a 2-mm sieve to remove plant roots and debris. The rhizosphere soil samples were collected from the roots of T. grandis and P. sibiricum and rinsed with phosphate buffer solution (PBS; McPherson et al., 2018).
Soil DNA was extracted using the Soil DNA kit (OMEGA BIO TEK) following the manufacturer's instruction. DNA concentration and quality were detected by 1% agarose gel electrophoresis with NanoDrop 2000 spectrophotometer (Thermo Fisher Scientific Inc., USA).
The bacterial V3-V4 variable region was amplified with primers 338F (5′-ACTCCTACGGGAGGCAGCAG-3′) and 806R (5′-GGACTACHVGGGTWTCTAAT-3′; Xu et al., 2016). The fungal ITS region was amplified with primers ITS3F (5′-GCATCGATGAAGAACGCAGCGCATCGATGAAGAACGCAGC-3′) and ITS4R (5′-TCCTCCGCTTATTGATATGC-3′; Toju et al., 2012). The PCR reaction condition was as follows: initial denaturation at 95°C for 3 min, 27 cycles of denaturation at 95°C for 30 s, annealing at 55°C for 30 s, 72°C for 30 s, and then a final extension of 10 min at 72°C. The PCR amplification products were mixed and detected by 2% agarose gel electrophoresis. Amplicons were extracted using QuantiFluor™-ST (Promega) and quantified using the AxyPrep DNA Gel Extraction Kit (Axygen Biosciences, Union City, CA, U.S.) according to the manufacturer's protocol. The PCR product was sequenced with paired-end (PE = 300) Illumina MiSeq platform at Majorbio (Shanghai International Medical Zone). The raw reads were deposited in the Sequence Read Archive database maintained by the National Center for Biotechnology Information (NCBI, Accession Number: PRJNA1150097).
The raw sequences were denoised, quality controlled and clustered into Amplicon Sequence Variant (ASV). The optimized data were then processed using Sequence Denoising Methods (DADA2/Deblur, etc.) to obtain ASV representing sequence and abundance information. Based on the representative ASV sequence and abundance information, analyses including microbial taxa, community diversity, species difference, correlation, and functional prediction were performed.
The bacterial and fungal community richness (Chao1), α-diversity index (Shannon), evenness (Shannoneven), and Pd (Phylogenetic diversity) were calculated using QIIME (Version 1.7.0). The bacterial and fungal indicator taxa were identified using the linear discriminant analysis Effect Size (LEfSe). LEfSe uses non-parametric Kruskal-Wallis rank sum tests to detect significant features and performs LDA to estimate the effect size of each feature (P < 0.05, and LDA score > 3.5). Principal coordinate analysis (PCoA) based on Bray–Curtis similarities at ASV level were used to calculate the difference in soil microbial community composition (beta diversity) by R (R v.3.4.4) program. Microbial networks diagrams were drawn using the Gephi software. Ecological function prediction of microorganisms b was performed using the FAPROTAX database (Louca et al., 2016) for bacteria (16s gene) and the FUNGuild database (Nguyen et al., 2016) for fungi (ITS gene).
Results showed that there were significant differences among the samples (P < 0.05) for bacterial and fungal community richness (Chao1), community diversity (Shannon), community evenness (Shannoneven), and phylogenetic diversity (PD). The rhizosphere soil of T. grandis mono-cropping (TR) exhibited the lowest bacterial and fungal community richness (381.70 ± 36.70; 136.47 ± 6.45) as well as phylogenetic diversity (114.25 ± 9.07; 41.48 ± 3.05), along with a lower bacterial community diversity (3.30 ± 0.45) and bacterial community evenness (0.55 ± 0.066; Figure 2, Supplementary Table 2). In contrast, the rhizosphere soil of Torreya-Polygonatum inter-cropping samples had higher fungal Chao1, Shannon, Shannoneven, and PD indices than those of mono-cropping (IPR > PR, ITR > TR). These results were consistent with the findings observed in the ITR and TR samples for bacterial communities. However, inter-cropping had minimal impact on the composition of rhizosphere soil bacterial community. Further, the bulk soil of the T. grandis inter-cropping with P. sibiricum (IB) had the higher fungal community richness, diversity, evenness and Phylogenetic diversity than the bulk soil of T. grandis (TB) and P. sibiricum (PB), respectively. There was no significant difference in bacterial richness, diversity, evenness and phylogenetic diversity between the rhizosphere and bulk soil samples in the T. grandis inter-cropping with P. sibiricum and P. sibiricum (IB and IPR, ITR; PB and PR), while the rhizosphere soil for mono-cropping of T. grandis were lower than bulk soil (TB and TR). After inter-cropping, the richness and phylogenetic diversity of the inter-cropping rhizosphere soil of P. sibiricum were significantly higher than bulk soil (IB and IPR), while no significant difference for T. grandis (IB and ITR). Moreover, there was no significant difference in fungal richness and phylogenetic diversity between the bulk soil and the rhizosphere soil for mono-cropping of T. grandis and P. sibiricum (PB and PR; TB and TR; Figure 2, Supplementary Table 2).
Figure 2. Alpha diversity of bacterial and fungal in bulk soil. (a, b) Chao1 index in (a) bacteria and (b) fungi. (c, d) Shannon index in (c) bacteria and (d) fungi. (e, f) Shannoneven diversity index in (e) bacteria and (f) fungi. (g, h) Pd diversity index in (g) bacteria and (h) fungi. Different lowercase letters indicate significant differences among the seven sets of samples. IB: the bulk soil of the T. grandis inter-cropping with P. sibiricum, PB: the bulk soil for mono-cropping of P. sibiricum, TB: the bulk soil for mono-cropping of T. grandis, IPR: the P. grandis rhizosphere soil of the T. grandis inter-cropping with P. sibiricum, PR: the rhizosphere soil for mono-cropping of P. sibiricum, ITR: the T. grandis rhizosphere soil of the T. grandis inter-cropping with P. sibiricum, and TR: the rhizosphere soil for mono-cropping of T. grandis.
All obtained sequences were classified to bacterial and fungal domains. Among them, 74.7% were classified to 39 bacterial phyla and 1,003 genera, and 79.8% were classified to 13 fungal phyla and 545 genera. The bacterial and fungal community coverage is 99.96–100% (Supplementary Table 2), indicating that the sequence data reasonably reflect the species and basic structure.
In Venn diagrams, 19,458 bacterial ASVs (73.2% of the total ASV) and 1,658 fungal ASVs (65.2% of the total ASV) were used to calculate the distribution of soil bacterial and fungal ASVs in the cropping. The seven samples shared 147 ASVs (0.8%) and 83 ASVs (4.9%) of the bacterial and fungal ASVs. The bacterial and fungal ASVs in the rhizosphere soil of Torreya-Polygonatum inter-cropping were higher than those in mono-cropping [bacterial: 3,281 (IPR) > 2,804 (PR), 3,189 (ITR) > 1,743 (TR); fungal: 378 (IPR) > 169 (PR), 401 (ITR) > 88 (TR; Figure 3)].
Figure 3. Venn diagram showing the bacterial (a) and fungal (b) unique and shared ASVs among the seven treated groups based on Bray–Curtis distances at the ASV level. IB: the bulk soil of the T. grandis inter-cropping with P. sibiricum, PB: the bulk soil for mono-cropping of P. sibiricum, TB: the bulk soil for mono-cropping of T. grandis, IPR: the P. grandis rhizosphere soil of the T. grandis inter-cropping with P. sibiricum, PR: the rhizosphere soil for mono-cropping of P. sibiricum, ITR: the T. grandis rhizosphere soil of the T. grandis inter-cropping with P. sibiricum, and TR: the rhizosphere soil for mono-cropping of T. grandis.
PCoA based on the Bray–Curtis distance was performed to address the bacterial and fungal community structure of seven samples. The two extracted principal coordinates explained 45.38 and 50.7% of the total variation in bacteria and fungi, respectively (Figure 4). The rest five samples showed separation. The R-values of bacterial and fungal communities were 0.9894 and 0.8786, respectively.
Figure 4. Principal coordinate analysis (PCoA) showing the bacterial (a) and fungal (b) community structure among the seven sets of samples. IB: the bulk soil of the T. grandis inter-cropping with P. sibiricum, PB: the bulk soil for mono-cropping of P. sibiricum, TB: the bulk soil for mono-cropping of T. grandis, IPR: the P. grandis rhizosphere soil of the T. grandis inter-cropping with P. sibiricum, PR: the rhizosphere soil for mono-cropping of P. sibiricum, ITR: the T. grandis rhizosphere soil of the T. grandis inter-cropping with P. sibiricum, and TR: the rhizosphere soil for mono-cropping of T. grandis.
The dominant bacterial phyla were Actinobacteriota (33.3%), Proteobacteria (32%), Acidobacteriota (11.5%), Firmicutes (7.4%), and Chloroflexi (6.7%) accounted for most (90.9%) of the bacterial sequences (Figure 5a, Supplementary Figure 1). The dominant fungal phyla were Ascomycota (80.6%) and Basidiomycota (13.8%), Rozellomycota (1.4% and Mortierellomycota (1.4%) accounted for the rest (Figure 5b, Supplementary Figure 1). The abundance of the dominant phyla differed across the samples. Compared to TR sample, ITR increased the relative abundance of phyla Actinobacteriota, Acidobacteriota, Chloroflexi, Basidiomycota, Rozellomycota, Mortierellomycota, and Glomeromycota, while Proteobacteria, Firmicutes and Ascomycota significantly decreased (Figures 6a, b). However, except for the higher abundance of Chloroflexi within IPR, there was no significant difference in the abundance of other dominant bacterial and fungal phyla between IPR and PR (Figures 6a, b). For the bulk soil samples, the IB samples showed higher abundance of the Chloroflexi, Mortierellomycota, and Glomeromycota compared to TB sample (IB and PB). Each of the bacterial and fungal phyla in the rhizosphere soil (IPR, PR, ITR, and TR) showed similar abundance. The overall phyla composition remained consistent across the different samples, though the abundance was different (Figures 5a, b, 6).
Figure 5. The top 10 most abundant bacterial and fungal groups. (a, b) Phyla level at (a) bacteria and (b) fungi. (c, d) Genus level at (c) bacteria and (d) fungi. IB: the bulk soil of the T. grandis inter-cropping with P. sibiricum, PB: the bulk soil for mono-cropping of P. sibiricum, TB: the bulk soil for mono-cropping of T. grandis, IPR: the P. grandis rhizosphere soil of the T. grandis inter-cropping with P. sibiricum, PR: the rhizosphere soil for mono-cropping of P. sibiricum, ITR: the T. grandis rhizosphere soil of the T. grandis inter-cropping with P. sibiricum, and TR: the rhizosphere soil for mono-cropping of T. grandis.
Figure 6. Differences in the abundance of the top bacterial (a, c) and fungal (b, d). (a, b) Top five phyla level at (a) bacteria and (b) fungi. (c, d) Top 10 genus level at (c) bacteria and (d) fungi. *Indicate significant differences among the seven sets of samples. IB: the bulk soil of the T. grandis inter-cropping with P. sibiricum, PB: the bulk soil for mono-cropping of P. sibiricum, TB: the bulk soil for mono-cropping of T. grandis, IPR: the P. grandis rhizosphere soil of the T. grandis inter-cropping with P. sibiricum, PR: the rhizosphere soil for mono-cropping of P. sibiricum, ITR: the T. grandis rhizosphere soil of the T. grandis inter-cropping with P. sibiricum, and TR: the rhizosphere soil for mono-cropping of T. grandis.
The most abundant bacterial genus was Bacillus (belong to Firmicutes, 6.08% of the sequences), followed by Unidentified Micrococcaceae (Actinobacteriota, 5.24% of the sequences) and Burkholderia (Proteobacteria, 4.63% of the sequences), Streptomyces (Actinobacteriota, 3.32% of the sequences), and unidentified Vicinamibacterales (Actinobacteriota, 3.17% of the sequences; Figure 5c, Supplementary Figure 2). The top five fungal genera were Fusarium (Ascomycota, 23.18% of the sequences), Cladosporium (Ascomycota, 10.84% of the sequences), Gibberella (Ascomycota, 8.22% of the sequences), Neocosmospora (Ascomycota, 6.37% of the sequences), and Trichoderma (Ascomycota, 5.84% of the sequences; Figure 5d, Supplementary Figure 2).
Among the top 10 genera of bacteria and fungi with high relative abundance, compared to the TR, ITR increased the relative abundance of unidentified Micrococcaceae, unidentified Xanthobacteraceae, unidentified Gaiellales and Saitozyma, while the relative abundance of Bacillus, Burkholderia, Streptomyces, Cladosporium, Gibberella Apiotrichum, and Pseudopithomyces decreased significantly (Figures 6c, d, Supplementary Tables 3, 4). For two rhizosphere soil of P. sibiricum (IPR and PR), Fusarium and Neocosmospora had higher abundance in the PR sample (Figures 6c, d, Supplementary Tables 3, 4). For the bulk and rhizosphere soil samples, Streptomyces, Nocrdioides, and Trichoderma had lower abundance in the rhizosphere soil after inter-croping, while unidentified Gaiellales was higher (IB and IPR, ITR; Figures 6c, d, Supplementary Tables 3, 4). Compare to the TB samples, the TR samples increased the relative abundance of Bacillus, Burkholderia, Streptomyces, Bradyrhizobium, Gibberella, Neocosmospora, and Penicillium, while the unidentified vicinamibacterales, Nocardioides, Apiotrichum, and Pseudopithomyces were decreased (Figures 6c, d, Supplementary Tables 3, 4).
The FAPROTAX classifier facilitates the annotation and prediction of microorganism functions through the construction of a comprehensive database that correlates microbial classification with their respective functionalities. The total number of 2,517 ASVs (9.46% of the total ASV) was assigned to 46 functional bacterial groups based on 16S rDNA sequence classification using FAPROTAX classifier. The dominant functional groups were associated with biogeochemical cyclings, including chemoheterotrophy (33.6%), aerobic chemoheterotrophy (33.3%), and aromatic_compound_degradation (4.7%) involved with C cycle, followed by the groups in the N cycle associated with nitrogen_fixation (3.9%) and nitrate_reduction (1.8%; Supplementary Table 5).
ANOVA analysis disclosed that the functions with significant differences among samples were mainly concentrated in the C cycle (phototrophy, photoheterotrophy, photoautotrophy, and anoxygenic_photoautotrophy, Figures 7a–d) and N cycle (nitrate_respiration, nitrite_denitrification, nitrite_respiration, and nitrogen_respiration, Figures 7e–h) with the same trend. These functions (Figures 7a–h) had the lowest abundance in the TR, of which was significantly lower than that in the ITR. For the bulk and the rhizosphere soil samples, except for the groups of phototrophy, photoheterotrophy, nitrite_denitrification, and nitrite_respiration, there was no significant difference in the other function groups in between.
Figure 7. Bacterial ecological functions predicted by FAPROTAX under different cropping practice. Bar chart showing the significantly different functional groups involved in C cycle (a–d), N cycle (e, h). C cycle in phototrophy (a); photoheterotrophy (b); photoautotrophy (c); anoxygenic_photoautotrophy (d); N cycle in nitrate_respiration (e); nitrite_denitrification (f); nitrite_respiration (g); nitrogen_respiration (h). Different lowercase letters indicate significant differences among the seven sets of samples. IB: the bulk soil of the T. grandis inter-cropping with P. sibiricum, PB: the bulk soil for mono-cropping of P. sibiricum, TB: the bulk soil for mono-cropping of T. grandis, IPR: the P. grandis rhizosphere soil of the T. grandis inter-cropping with P. sibiricum, PR: the rhizosphere soil for mono-cropping of P. sibiricum, ITR: the T. grandis rhizosphere soil of the T. grandis inter-cropping with P. sibiricum, and TR: the rhizosphere soil for mono-cropping of T. grandis.
FUNGuild was used to predict the function of the fungal community structure of seven samples, incorporating current literature and authoritative website data for categorizing fungi into distinct functional groups based on their nutritional modes. In total, 3,846 ASVs were assigned to different functional groups according to the annotation of ITS sequence classification. According to the absorption and utilization of environmental resources of the fungal community, the fungi were divided into pathotroph, symbiotroph, and saprotroph. Undefined saprotroph was the most enriched functional group, followed by plant and animal pathogens. Fungi are classified into various guilds based on the three major nutritional methods, encompassing animal pathogens, arbuscular mycorrhizal fungi, ectomycorrhizal fungi, lichenicolous fungi, lichenized fungi, plant pathogens, undefined root endophytes, undefined saprotrophs, and wood saprotrophs as the main functional groups. The seven samples had different effects on the abundance of the functional groups. The results further revealed that inter-cropping significantly enhanced the abundance of plant pathogens and Animal Pathogen-Endophyte-Lichen Parasite-Plant Pathogen-Wood Saprotroph in the rhizosphere soil of P. sibiricum, conversely, it resulted in a decrease in the abundance of Animal Pathogen-Endophyte-Lichen Parasite-Plant Pathogen-Soil Saprotroph-Wood Saprotroph (Figure 8, Supplementary Table 6). Simultaneously Fungal Parasite-Undefined Saprotroph function groups exhibited an increase in the rhizosphere soil of T. grandis after inter-cropping, while plant pathogens and Animal Pathogen-Endophyte-Lichen Parasite-Plant Pathogen-Wood Saprotroph decreased (Figure 8, Supplementary Table 6).
Figure 8. Compositions and relative abundance of assigned fungal functional groups (guild) inferred by FUNGuild. IB: the bulk soil of the T. grandis inter-cropping with P. sibiricum, PB: the bulk soil for mono-cropping of P. sibiricum, TB: the bulk soil for mono-cropping of T. grandis, IPR: the P. grandis rhizosphere soil of the T. grandis inter-cropping with P. sibiricum, PR: the rhizosphere soil for mono-cropping of P. sibiricum, ITR: the T. grandis rhizosphere soil of the T. grandis inter-cropping with P. sibiricum, and TR: the rhizosphere soil for mono-cropping of T. grandis.
The agricultural productivity and stability rely heavily on the richness and diversity of soil microbial communities (Van Der Heijden et al., 2008). They are often reshaped with different cropping practice. Of which, inter-cropping has been demonstrated to have the potential to enhance beneficial soil microbial communities and effectively mitigate pests and diseases (Zhang et al., 2019; Chai et al., 2021). In this study, we addressed the responses of soil microbial communities to T. grandis inter-cropping with P. sibiricum, and compared the microbial community disparities between bulk and rhizosphere soil samples.
Intercropping has the potential to modulate the diversity of soil microbial communities. Previous studies showed that maize inter-cropping with peanut leads to an increase of soil beneficial microbial abundance and diversity (Du et al., 2020; He et al., 2013). Li and Wu (2018) disclosed that wheat inter-cropping with cucumber or mustard with cucumber can enhance the richness of operational microbial taxonomic in soil. In this study, PCoA results revealed that there were distinct variations in bacterial and fungal communities (Figure 4). Compared to mono-cropping, indices of Chao1, Shannon, Shannoneven, and PD using α-diversity analysis were increased both for bulk and rhizosphere soil samples of T. grandis inter-cropping with P. sibiricum (Figure 2). The study conducted by Deng et al. (2022) found no statistically significant differences in bacterial community richness (Chao1) and diversity (Shannon) between T. grandis inter-cropping with P. sibiricum and mono-cropping of T. grandis (ITR and TR). However, this study revealed a significant variation in the richness and diversity of bacteria and fungi in the rhizosphere soil of T. grandis after inter-cropping, while having minimal impact on the composition of bacterial communities in the rhizosphere soil of P. sibiricum. Our findings differ from Deng's observations in T. grandis plantation, potentially attributed to variations in sampling points (Deng et al., 2022).
Bacterial phyla of Actinobacteriota, Proteobacteria, Acidobacteriota, Firmicutes, and Chloroflexi were predominant within all samples here (Figure 5). Our results align with previous studies conducted in pure T. grandis forest (Feng et al., 2019; Deng et al., 2022) with the exception of Firmicutes. The most predominant phylum Actinobacteriota (33.2%) had significantly higher abundance within T. grandis rhizosphere soil after inter-cropping, compared to mono-cropping. The Actinobacteria taxa mainly consist of copiotrophs that strategically thrive on carbon availability and are actively involved with organic matter decomposition and cycling (Liu et al., 2017). Further, T. grandis inter-cropping with P. sibiricum resulted in relatively elevated presence of Acidobacteriota and Chloroflexi. Taxa residing these two phyla are known to plays crucial roles in polysaccharide degradation as well as the decomposition of non-degradable organic matter (Moghimian et al., 2017; Li et al., 2020). However, the relative abundance of Proteobacteria (represented by Burkholderia) and Firmicutes (represented by Bacillus) applying inter-cropping was decreased (Figure 6), which is in agreement of previous studies (Gao et al., 2019; Deng et al., 2022).
Inter-cropping known for effectively modulating the soil microbial community can be applied for disease management through reducing pathogen abundance (Zhang et al., 2023). Cladosporium speices are crucial plant pathogens responsible for stem rot and leaf spot diseases (Mohamed and Ibrahim, 2021), and in this study, the abundance of Cladosporium in the rhizosphere soil of T. grandis was significantly decreased following T. grandis inter-cropping with P. sibiricum (Figure 6d). Fusarium is a significant causative agent of root rot in T. grandis and P. sibiricum (Zhang et al., 2016; Li and Guo, 2019). The similar trend was found on Fusarium pathogen within the P. sibiricum (Figure 6d). The abundance of the opportunistic pathogen Neocosmospora known to induce canker disease, stem and root rot, as well as cane wilt (Zeng and Zhuang, 2023) was also substantially decreased as the result of inter-cropping (Figure 6d). Therefore, it is hypothesized that T. grandis inter-cropping with P. sibiricum may reduce the abundance of certain pathogens.
Inter-cropping, on the other side, can enhance plant resistance to pathogens and promote plant growth by recruiting beneficial microbial communities (Mendes et al., 2011; Latz et al., 2012; Dhar Purkayastha et al., 2018; Humphrey et al., 2020; Shalev et al., 2022). Xanthobacteraceae comprises a cluster of bacteria closely associated with the carbon cycle, and some species within this group exhibit a facultatively chemolithoautotrophic lifestyle, enabling them to both assimilate and generate carbon dioxide (Kappler et al., 2012). Gemmatimonadaceae species are a group of nitrogen removal bacteria associated with the nitrogen cycle that play a key role in efficient phytic acid mineralization in different ecosystems (Jia et al., 2023). In this study, T. grandis inter-cropping with P. sibiricum significantly increased the abundance of Xanthobacteraceae and Gemmatimonadaceae in the rhizosphere soil of T. grandis (Figure 6c). Based on the analysis and prediction results from FAPROTAX, it can be inferred that intercropping has the potential to enhance the soil microbial communities' capacity for carbon and nitrogen cycling, thereby facilitating nutrient circulation within plants. The probiotic Saitozyma present in soil and plant rhizosphere exhibits the potential to enhance plant growth and disease management (Das et al., 2023). In this study, the rhizosphere soil of T. grandis was significantly improved after inter-cropping practice, suggesting its ability to recruit beneficial microorganisms for growth and disease control.
Changes in microbial community structure can result in microbial functional alterations (Preston-Mafham et al., 2002). FAPROTAX was employed to predict the functional profiles of soil bacteria under different samples. The results revealed that chemoheterotrophy (33.6%) and aerobic chemoheterotrophy (33.3%) were the predominant enriched functions, consistent with previous findings (Rocha et al., 2020; Wang et al., 2023). Previous studies disclosed that inter-cropping significantly enhanced soil microbial function associated with nutrient cycling and exhibited a consistent increase in the relative abundance of nitrogen-cycling and carbon-cycling bacteria (Zhong et al., 2022; Marcos-Pérez et al., 2023). In our study, we found that genes involved in carbon cycling (phototrophy, photoheterotrophy, photoautotrophy, and anoxygenic_photoautotrophy) and nitrogen cycling (nitrate_respiration, nitrite_denitrification, nitrite_respiration, and nitrogen_respiration) exhibited significantly higher abundance in the ITR sample compared to TR. These results suggest that T. grandis inter-cropping with P. sibiricum may enhance nutrient cycling in soil through its influence on bacterial functionality and alteration of soil bacterial community composition. This could be attributed to feedback cycles facilitated by C-cycle bacteria (Xanthobacteraceae) and N-cycle bacteria (Gemmatimonadaceae), which showed a significant increase under the inter-cropping samples.
FUNGuild was used to achieve fungal functional determination (Song et al., 2017; Nguyen et al., 2016). Undefined saprotrophs as the most abundant fungal functional group, may be attributed to the high prevalence of ascomycetes in each sample group, given that a significant portion of fungi within this phylum are associated with organic decomposition processes (Nguyen et al., 2016). Inter treatment comparisons reveal a lower prevalence of plant pathogen guild in the intercropped sample ITR compared to the TR, which aligns with the reduced abundance of pathogenic fungi such as Cladosporium, Gibberella, and Neocosmosporain in ITR, further contributes to mitigating soil-borne plant pathogens, thereby enhancing crop yield and disease resistance.
This study focused the impact of T. grandis inter-cropping with P. sibiricum and mono-cropping on soil microbial diversity and function. The results demonstrate that T. grandis inter-cropping with P. sibiricum significantly increases the abundance and diversity of soil fungal and bacterial communities comparing to mono-cropping. Moreover, this agricultural practice also enhanced the microbial functionality pertaining to nitrogen and carbon cycles, thereby potentially facilitating nutrient uptake by plants while concurrently reducing the prevalence of specific pathogens. The findings arisen from the study are helpful for agroforestry sustainable development and effective management.
The datasets presented in this study can be found in online repositories. The names of the repository/repositories and accession number(s) can be found at: https://www.ncbi.nlm.nih.gov/, PRJNA1150097.
QW: Data curation, Formal analysis, Writing – original draft, Writing – review & editing, Conceptualization, Methodology. XP: Conceptualization, Data curation, Formal analysis, Investigation, Methodology, Writing – original draft. YY: Data curation, Formal analysis, Investigation, Methodology, Writing – review & editing. XZ: Conceptualization, Funding acquisition, Writing – original draft, Writing – review & editing. JH: Formal analysis, Funding acquisition, Project administration, Validation, Writing – review & editing. HW: Data curation, Project administration, Writing – original draft, Writing – review & editing.
The author(s) declare financial support was received for the research, authorship, and/or publication of this article. This work was supported by Department of Science and Technology of Zhejiang Province, China (2022C02009) and the Launching Funds for Talents of Zhejiang A & F University (2020FR036), China.
The authors declare that the research was conducted in the absence of any commercial or financial relationships that could be construed as a potential conflict of interest.
All claims expressed in this article are solely those of the authors and do not necessarily represent those of their affiliated organizations, or those of the publisher, the editors and the reviewers. Any product that may be evaluated in this article, or claim that may be made by its manufacturer, is not guaranteed or endorsed by the publisher.
The Supplementary Material for this article can be found online at: https://www.frontiersin.org/articles/10.3389/fmicb.2024.1487619/full#supplementary-material
Bai, Y. X., Wang, G., Cheng, Y. D., Shi, P. Y., Yang, C. C., Yang, H. W., et al. (2019). Soil acidification in continuously cropped tobacco alters bacterial community structure and diversity via the accumulation of phenolic acids. Sci. Rep. 9:12499. doi: 10.1038/s41598-019-48611-5
Berg, G., and Smalla, K. (2009). Plant species and soil type cooperatively shape the structure and function of microbial communities in the rhizosphere. FEMS Microbiol. Ecol. 68, 1–13. doi: 10.1111/j.1574-6941.2009.00654.x
Chai, Q., Nemecek, T., Liang, C., Zhao, C., Yu, A., Coulter, J. A., et al. (2021). Integrated farming with intercropping increases food production while reducing environmental footprint. Proc. Natl. Aca. Sci. U. S. A. 118:e2106382118. doi: 10.1073/pnas.2106382118
Chen, X., and Chen, H. (2021). Comparing environmental impacts of Chinese torreya plantations and regular forests using remote sensing. Environ. Dev. Sustainabil. 23, 133–150. doi: 10.1007/s10668-019-00570-7
Chen, X., and Jin, H. (2019). Review of cultivation and development of Chinese torreya in China. For. Trees Livelih. 28, 68–78. doi: 10.1080/14728028.2018.1553690
Das, S., Rabha, J., and Narzary, D. (2023). Assessment of soil yeasts Papiliotrema laurentii S-08 and Saitozyma podzolica S-77 for plant growth promotion and biocontrol of Fusarium wilt of brinjal. J. Appl. Microbiol. 134:lxad252. doi: 10.1093/jambio/lxad252
Deng, P., Fan, W., Wang, H., Ti, J., and Xu, X. (2022). Chinese Torreya agroforestry alters the rhizosphere soil bacterial communities and potential functions. Appl. Soil Ecol. 177:104504. doi: 10.1016/j.apsoil.2022.104504
Dhar Purkayastha, G., Mangar, P., Saha, A., and Saha, D. (2018). Evaluation of the biocontrol efficacy of a Serratia marcescens strain indigenous to tea rhizosphere for the management of root rot disease in tea. PLoS ONE 13:e0191761. doi: 10.1371/journal.pone.0191761
Du, L. T., Huang, B. J., Du, N. S., Guo, S. R., Shu, S., and Sun, J. (2017). Effects of garlic/cucumber relay intercropping on soil enzyme activities and the microbial environment in continuous cropping. HortScience 52, 78–84. doi: 10.21273/HORTSCI11442-16
Du, Q., Zhou, L., Chen, P., Liu, X. M., Song, C., Yang, F., et al. (2020). Relay-intercropping soybean with maize maintains soil fertility and increases nitrogen recovery efficiency by reducing nitrogen input. Crop J. 8, 140–152. doi: 10.1016/j.cj.2019.06.010
Feng, Y., Hu, Y., Wu, J., Chen, J., Yrjälä, K., and Yu, W. (2019). Change in microbial communities, soil enzyme and metabolic activity in a Torreya grandis plantation in response to root rot disease. For. Ecol. Manag. 432, 932–941. doi: 10.1016/j.foreco.2018.10.028
Gao, L., Liu, X. M., Du, Y. M., Zong, H., and Shen, G. M. (2019). Effects of tobacco-peanut relay intercropping on soil bacteria community structure. Ann. Microbiol. 69, 1531–1536. doi: 10.1007/s13213-019-01537-9
Haichar, F. E. Z., Marol, C., Berge, O., Rangel-Castro, J. I., Prosser, J. I., Balesdent, J., et al. (2008). Plant host habitat and root exudates shape soil bacterial community structure. ISME J. 2, 1221–1230. doi: 10.1038/ismej.2008.80
He, Y., Ding, N., Shi, J., Wu, M., Liao, H., and Xu, J. (2013). Profiling of microbial PLFAs: implications for interspecific interactions due to intercropping which increase phosphorus uptake in phosphorus limited acidic soils. Soil Biol. Biochem. 57, 625–634. doi: 10.1016/j.soilbio.2012.07.027
Hinsinger, P., Bengough, A. G., Vetterlein, D., and Young, I. M. (2009). Rhizosphere: biophysics, biogeochemistry and ecological relevance. Plant Soil 321, 117–152. doi: 10.1007/s11104-008-9885-9
Humphrey, P. T., Satterlee, T. T., and Whiteman, N. K. (2020). Competitive hierarchies, antibiosis, and the distribution of bacterial life history traits in a microbiome. BioRxiv 2020–06. doi: 10.1101/2020.06.15.152272
Jia, L., Cheng, X., Fang, L., and Huang, X. (2023). Nitrogen removal in improved subsurface wastewater infiltration system: mechanism, microbial indicators and the limitation of phosphorus. J. Environ. Manag. 335:117456. doi: 10.1016/j.jenvman.2023.117456
Kappler, U., Davenport, K., Beatson, S., Lucas, S., Lapidus, A., Copeland, A., et al. (2012). Complete genome sequence of the facultatively chemolithoautotrophic and methylotrophic alpha proteobacterium Starkeya novella type strain (ATCC 8093T). Stand. Genom. Sci. 7, 44–58. doi: 10.4056/sigs.3006378
Kato, A., Miura, T., Yano, H., Masuda, K., Ishida, H., and Seino, Y. (1994). Suppressive effects of polygonati rhizoma on hepatic glucose output, GLUT2 mRNA expression and its protein content in rat liver. Endocr. J. 41, 139–144. doi: 10.1507/endocrj.41.139
Latz, E., Eisenhauer, N., Rall, B. C., Allan, E., Roscher, C., Scheu, S., et al. (2012). Plant diversity improves protection against soil-borne pathogens by fostering antagonistic bacterial communities. J. Ecol. 100, 597–604. doi: 10.1111/j.1365-2745.2011.01940.x
Li, D. L., and Guo, M. (2019). First report of Fusarium oxysporum causing root rot on Polygonatum sibiricum in Chizhou, China. Plant Dis. 103, 2956–2956. doi: 10.1094/PDIS-05-19-0922-PDN
Li, L., Li, S. M., Sun, J. H., Zhou, L. L., Bao, X. G., Zhang, H. G., et al. (2007). Diversity enhances agricultural productivity via rhizosphere phosphorus facilitation on phosphorus-deficient soils. Proc. Natl. Acad. Sci. U. S. A. 104, 11192–11196. doi: 10.1073/pnas.0704591104
Li, S., and Wu, F. (2018). Diversity and co-occurrence patterns of soil bacterial and fungal communities in seven intercropping systems. Front. Microbiol. 9:1521. doi: 10.3389/fmicb.2018.01521
Li, Y. Y., Feng, J., Zheng, L., Huang, J. B., Yang, Y., and Li, X. H. (2020). Intercropping with marigold promotes soil health and microbial structure to assist in mitigating tobacco bacterial wilt. J. Plant Pathol. 102:731. doi: 10.1007/s42161-020-00490-w
Liu, X., Cong, J., Lu, H., Xue, Y., Wang, X., Li, D., et al. (2017). Community structure and elevational distribution pattern of soil Actinobacteria in alpine grasslands. Acta Ecol. Sin. 37, 213–218. doi: 10.1016/j.chnaes.2017.02.010
Louca, S., Parfrey, L. W., and Doebeli, M. (2016). Decoupling function and taxonomy in the global ocean microbiome. Science 353:1272. doi: 10.1126/science.aaf4507
Luo, C., Li, W., Chun, Z., and Wu, Y. (2018). Discriminating five Polygonatum medical materials and monitoring their chemical changes associated with traditional process by FT-IR spectroscopy coupled with multivariate analysis. Vibrat. Spectrosc. 99, 104–112. doi: 10.1016/j.vibspec.2018.08.016
Luo, Y., Zhang, X., She, X. S., Zhuang, M., Su, M., Hu, C. Y., et al. (2023). Effects of compounds plantations of Torreya grandis on soil nutrient conservation in mountainous areas of Southern Anhui Province. Chin. Agricult. Sci. Bullet. 39, 51–59. doi: 10.11924/j.issn.1000-6850.casb2022-0595
Lv, J., Dong, Y., Dong, K., Zhao, Q., Yang, Z., and Chen, L. (2020). Intercropping with wheat suppressed Fusarium wilt in faba bean and modulated the composition of root exudates. Plant Soil 448, 153–164. doi: 10.1007/s11104-019-04413-2
Machado, S., Petrie, S., Rhinhart, K., and Qu, A. (2007). Long-term continuous cropping in the Pacific Northwest: tillage and fertilizer effects on winter wheat, spring wheat, and spring barley production. Soil Till. Res. 94, 473–481. doi: 10.1016/j.still.2006.09.007
Marcos-Pérez, M., Sánchez-Navarro, V., and Zornoza, R. (2023). Intercropping systems between broccoli and fava bean can enhance overall crop production and improve soil fertility. Scient. Horticult. 312:111834. doi: 10.1016/j.scienta.2023.111834
McPherson, M. R., Wang, P., Marsh, E. L., Mitchell, R. B., and Schachtman, D. P. (2018). Isolation and analysis of microbial communities in soil, rhizosphere, and roots in perennial grass experiments. J. Visual. Exp. 2018:137. doi: 10.3791/57932-v
Mendes, R., Kruijt, M., de Bruijn, I., Dekkers, E., van der Voort, M., Schneider, J. H. M., et al. (2011). Deciphering the rhizosphere microbiome for disease-suppressive bacteria. Science 332, 1097–1100. doi: 10.1126/science.1203980
Moghimian, N., Hosseini, S. M., Kooch, Y., and Darki, B. Z. (2017). Impacts of changes in land use/cover on soil microbial and enzyme activities. Catena 157, 407–414. doi: 10.1016/j.catena.2017.06.003
Mohamed, G. A., and Ibrahim, S. R. (2021). Untapped potential of marine-associated Cladosporium species: an overview on secondary metabolites, biotechnological relevance, and biological activities. Mar. Drugs 19:645. doi: 10.3390/md19110645
Nguyen, N. H., Song, Z., Bates, S. T., Branco, S., Tedersoo, L., Menke, J., et al. (2016). FUNGuild: an open annotation tool for parsing fungal community datasets by ecological guild. Fung. Ecol. 20, 241–248. doi: 10.1016/j.funeco.2015.06.006
Pervaiz, Z. H., Iqbal, J., Zhang, Q., Chen, D., Wei, H., and Saleem, M. (2020). Continuous cropping alters multiple biotic and abiotic indicators of soil health. Soil Syst. 4:59. doi: 10.3390/soilsystems4040059
Preston-Mafham, J., Boddy, L., and Randerson, P. F. (2002). Analysis of microbial community functional diversity using sole-carbon-source utilisation profiles-a critique. FEMS Microbiol. Ecol. 42, 1–14. doi: 10.1111/j.1574-6941.2002.tb00990.x
Rocha, S. M. B., Mendes, L. W., de Souza Oliveira, L. M., Melo, V. M. M., Antunes, J. E. L., Araujo, F. F., et al. (2020). Nodule microbiome from cowpea and lima bean grown in composted tannery sludge-treated soil. Appl. Soil Ecol. 151:103542. doi: 10.1016/j.apsoil.2020.103542
Shalev, O., Ashkenazy, H., Neumann, M., and Weigel, D. (2022). Commensal Pseudomonas protect Arabidopsis thaliana from a coexisting pathogen via multiple lineage-dependent mechanisms. ISME J. 16, 1235–1244. doi: 10.1038/s41396-021-01168-6
Song, C., Wang, W., Gan, Y., Wang, L., Chang, X., Wang, Y., et al. (2022). Growth promotion ability of phosphate-solubilizing bacteria from the soybean rhizosphere under maize-soybean intercropping systems. J. Sci. Food Agricult. 102, 1430–1442. doi: 10.1002/jsfa.11477
Song, Z., Kennedy, P. G., Liew, F. J., and Schilling, J. S. (2017). Fungal endophytes as priority colonizers initiating wood decomposition. Funct. Ecol. 31, 407–418. doi: 10.1111/1365-2435.12735
Tedersoo, L., Bahram, M., and Zobel, M. (2020). How mycorrhizal associations drive plant population and community biology. Science 367:eaba1223. doi: 10.1126/science.aba1223
Toju, H., Tanabe, A. S., Yamamoto, S., and Sato, H. (2012). High-coverage ITS primers for the DNA-based identification of ascomycetes and basidiomycetes in environmental samples. PLoS ONE 7:e40863. doi: 10.1371/journal.pone.0040863
Van Der Heijden, M. G., Bardgett, R. D., and Van Straalen, N. M. (2008). The unseen majority: soil microbes as drivers of plant diversity and productivity in terrestrial ecosystems. Ecol. Lett. 11, 296–310. doi: 10.1111/j.1461-0248.2007.01139.x
Wagg, C., Bender, S. F., Widmer, F., and Van Der Heijden, M. G. (2014). Soil biodiversity and soil community composition determine ecosystem multifunctionality. Proc. Natl. Acad. Sci. U. S. A. 111, 5266–5270. doi: 10.1073/pnas.1320054111
Wang, G., Bei, S., Li, J., Bao, X., Zhang, J., Schultz, P. A., et al. (2021). Soil microbial legacy drives crop diversity advantage: linking ecological plant-soil feedback with agricultural intercropping. J. Appl. Ecol. 58, 496–506. doi: 10.1111/1365-2664.13802
Wang, Z. H., Xu, M. Z., Li, F., Bai, Y., Hou, J. F., Li, X. Q., et al. (2023). Changes in soil bacterial communities and functional groups beneath coarse woody debris across a subalpine forest successional series. Glob. Ecol. Conserv. 43:e02436. doi: 10.1016/j.gecco.2023.e02436
Xian, Y. F., Lin, Z. X., Xu, X. Y., Su, Z. R., Chen, J. N., Lai, X. P., et al. (2012). Effect of Rhizoma Polygonati on 12-O-tetradecanoylphorbol-acetate-induced ear edema in mice. J. Ethnopharmacol. 142, 851–856. doi: 10.1016/j.jep.2012.06.013
Xu, N., Tan, G., Wang, H., and Gai, X. (2016). Effect of biochar additions to soil on nitrogen leaching, microbial biomass and bacterial community structure. Eur. J. Soil Biol. 74, 1–8. doi: 10.1016/j.ejsobi.2016.02.004
Yu, H., Chen, S., Zhang, X., Zhou, X., and Wu, F. (2019). Rhizosphere bacterial community in watermelon-wheat intercropping was more stable than in watermelon monoculture system under Fusarium oxysporum f. sp. niveum invasion. Plant Soil 445, 369–381. doi: 10.1007/s11104-019-04321-5
Yu, Y., Yang, J., Zeng, S., Wu, D., Jacobs, D. F., and Sloan, J. L. (2017). Soil pH, organic matter, and nutrient content change with the continuous cropping of Cunninghamia lanceolata plantations in South China. J. Soils Sediment. 17, 2230–2238. doi: 10.1007/s11368-016-1472-8
Zeng, J. R., Liu, J. Z., Lu, C. H., Ou, X. H., Luo, K. K., Li, C. M., et al. (2020). Intercropping with turmeric or ginger reduce the continuous cropping obstacles that affect Pogostemon cablin (patchouli). Front. Microbiol. 11:579719. doi: 10.3389/fmicb.2020.579719
Zeng, Z. Q., and Zhuang, W. Y. (2023). New species of Neocosmospora (Ascomycota) from China as evidenced by morphological and molecular data. Life 13:1515. doi: 10.3390/life13071515
Zhang, C. C., Dong, Y., Tang, L., Zheng, Y., Makowski, D., Yu, Y., et al. (2019). Intercropping cereals with faba bean reduces plant disease incidence regardless of fertilizer input; a meta-analysis. Eur. J. Plant Pathol. 154, 931–942. doi: 10.1007/s10658-019-01711-4
Zhang, C. Q., Zhang, S. Y., Chen, X. L., Qi, Q. Q., and Lou, H. Z. (2016). First report of Fusarium crown and root rot on Torreya grandis caused by Fusarium oxysporum species complex in China. Plant Dis. 100, 1500–1500. doi: 10.1094/PDIS-12-15-1514-PDN
Zhang, H., Yang, Y., Mei, X., Li, Y., Wu, J., Li, Y., et al. (2020). Phenolic acids released in maize rhizosphere during maize-soybean intercropping inhibit Phytophthora blight of soybean. Front. Plant Sci. 11:886. doi: 10.3389/fpls.2020.00886
Zhang, H. C., Wang, R., Chen, S., Qi, G. F., He, Z. L., and Zhao, X. Y. (2017). Microbial taxa and functional genes shift in degraded soil with bacterial wilt. Sci. Rep. 7:39911. doi: 10.1038/srep39911
Zhang, Y., Yang, Y., Lu, X., Wang, A., Xue, C., Zhao, M., et al. (2023). The effects and interrelationships of intercropping on Cotton Verticillium wilt and soil microbial communities. BMC Microbiol. 23, 1–15. doi: 10.1186/s12866-023-02780-6
Keywords: plant health, soil microbiota, agroforestry, sustainable development, soil microbial function
Citation: Wang Q, Peng X, Yuan Y, Zhou X, Huang J and Wang H (2024) The effect of Torreya grandis inter-cropping with Polygonatum sibiricum on soil microbial community. Front. Microbiol. 15:1487619. doi: 10.3389/fmicb.2024.1487619
Received: 28 August 2024; Accepted: 04 November 2024;
Published: 04 December 2024.
Edited by:
Jesus Fernandez Bayo, University of Granada, SpainReviewed by:
Yigal Achmon, Guangdong Technion-Israel Institute of Technology (GTIIT), ChinaCopyright © 2024 Wang, Peng, Yuan, Zhou, Huang and Wang. This is an open-access article distributed under the terms of the Creative Commons Attribution License (CC BY). The use, distribution or reproduction in other forums is permitted, provided the original author(s) and the copyright owner(s) are credited and that the original publication in this journal is cited, in accordance with accepted academic practice. No use, distribution or reproduction is permitted which does not comply with these terms.
*Correspondence: Haonan Wang, aGFvbmFud2FuZ0B6YWZ1LmVkdS5jbg==; Jianqin Huang, aHVhbmdqcUB6YWZ1LmVkdS5jbg==
Disclaimer: All claims expressed in this article are solely those of the authors and do not necessarily represent those of their affiliated organizations, or those of the publisher, the editors and the reviewers. Any product that may be evaluated in this article or claim that may be made by its manufacturer is not guaranteed or endorsed by the publisher.
Research integrity at Frontiers
Learn more about the work of our research integrity team to safeguard the quality of each article we publish.