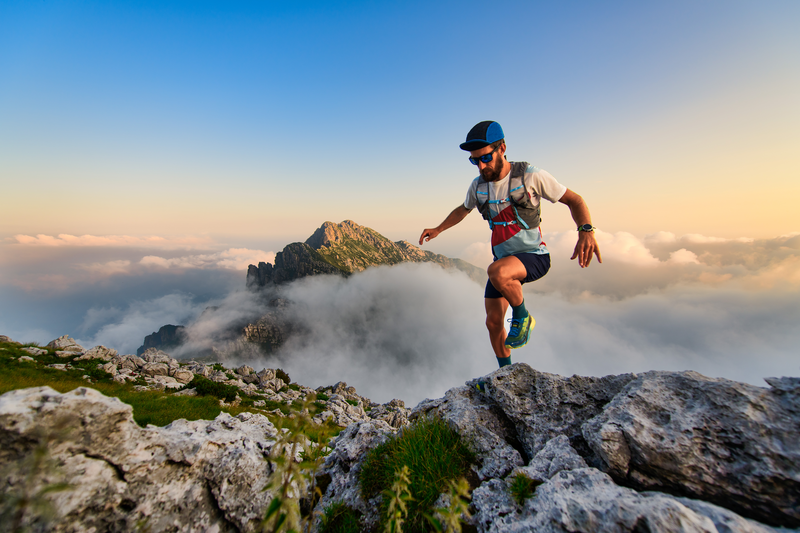
94% of researchers rate our articles as excellent or good
Learn more about the work of our research integrity team to safeguard the quality of each article we publish.
Find out more
ORIGINAL RESEARCH article
Front. Microbiol. , 13 January 2025
Sec. Terrestrial Microbiology
Volume 15 - 2024 | https://doi.org/10.3389/fmicb.2024.1482904
Continuous cropping (CC) profoundly impacts soil ecosystems, including changes in soil factors and the structure and stability of microbial communities. These factors are interrelated and together affect soil health and plant growth. In this research, metagenomic sequencing was used to explore the effects of CC on physicochemical properties, enzyme activities, microbial community composition, and functional genes of the rhizosphere soil of patchouli. We found that this can lead to changes in various soil factors, including the continuous reduction of pH and -N and the unstable changes of many factors. In addition, S-PPO enzyme activity increased significantly with the cropping years, but S-NAG increased in the first 2 years and decreased in the third cropping year. Metagenomic sequencing results showed that CC significantly changed the diversity and composition of rhizosphere microbial communities. The relative abundance of Pseudomonas and Bacteroides decreased substantially from the phylum level. At the genus level, the number of microbial genera specific to the zero-year cropping (CK) and first (T1), second (T2), and third (T3) years decreased significantly, to 1798, 172, 42, and 44, respectively. The abundance of many functional genes changed, among which COG0823, a gene with the cellular process and signaling functions, significantly increased after CC. In addition, -N, S-CAT, S-LAP, and SOC were the main environmental factors affecting rhizosphere-dominant microbial communities at the phylum level, while pH, SOC, and AK were the key environmental factors affecting rhizosphere functional genes of Pogostemon cablin. In summary, this study showed the dynamic changes of soil factors and rhizosphere microorganisms during CC, providing a theoretical basis for understanding the formation mechanism and prevention of CC obstacles and contributing to the formulation of scientific soil management and fertilization strategies.
Pogostemon cablin (Patchouli), a medicinal plant in the Lamiaceae family, is native to Southeast Asian countries, including Vietnam, the Philippines, and Malaysia (Maheshwari et al., 1993; Swamy et al., 2010; Wu et al., 2010). Patchouli's main crop-growing regions in China are in the southern localities, particularly in Guangdong and Hainan (Wu et al., 2010). Based on its growing areas, this plant is categorized into four different types (Nanxiang, Paixiang, Zhaoxiang, and Zhanxiang) in the market of traditional Chinese medicine (Liu et al., 2023). The dried aboveground parts of patchouli are often used to treat fever, nausea during pregnancy, headaches, influenza prevention, indigestion, and their anti-inflammatory and analgesic properties (Chakrapani et al., 2013). Furthermore, patchouli volatile oil (extracted from leaves) restrains ample amounts of ketone and alcohol, crucial for the perfume industry's fragrance durability (Zhang et al., 2018).
Continuous cropping (CC) obstacles refer to continuously planting the same crop or closely related species on the same land, resulting in crop growth, yield, and quality declines (Zeeshan Ul Haq et al., 2023). This issue arises from multiple factors, including soil degradation, nutrient depletion, and the accumulation of soil-borne pathogens, all collectively weaken plant health and productivity (Zeng et al., 2020). Numerous studies have documented the adverse effects of CC on agricultural systems. For instance, research indicates that CC leads to an average yield reduction of 22%, posing a significant threat to sustainable farming practices (Wang K. et al., 2024; Wang F. et al., 2024). Additionally, continuous soybean cropping negatively impacts soil microbial communities, with increased pathogenic fungi and decreased beneficial microorganisms contributing to yield declines (Liu et al., 2022). Similarly, leguminous plants such as soybeans and peanuts, while also experiencing changes in microbial communities, benefit from the symbiotic characteristics of rhizobia, which enhance their resistance to variations in mineral nutrition, pests, and diseases to a certain extent (Jaiswal et al., 2021). Long-term CC exacerbates nutrient loss and degrades soil quality, jeopardizing agricultural productivity (Kartini et al., 2024). Studies on various crops, including Glycine max (Tian et al., 2020; Liu et al., 2020), Arachis hypogaea (Li et al., 2014), Gossypium spp. (Xi et al., 2019), and Lpomoea batatas (Gao et al., 2019) have shown that CC disrupts soil microbial communities, leading to significant yield reductions. Due to limited arable land and improper agricultural practices, this issue is prevalent in many farming systems in China (Lei et al., 2020). The complexity behind CCOs stems from intricate interactions within the “plant-soil-microorganism” system (Zhao J. et al., 2024; Zhao X. et al., 2024).
Modern research suggested that CC systems may lead to increased release of metabolites and leachates from plants, potentially enhancing the diversity and abundance of harmful microorganisms and promoting pathogenic bacterial proliferation (Zeng et al., 2020). This phenomenon disrupts the balance of soil microbial communities, resulting in reduced populations and diversity of beneficial and harmful bacteria, ultimately damaging the structure of the microenvironment. As these changes occur, soil microbial diversity significantly alters, impacting soil ecological functions and limiting plant growth and development (Wu et al., 2015; Tan et al., 2016). Microorganisms, as the most dynamic components of the soil ecosystem, provide crucial feedback and responses to these environmental changes (Toledo et al., 2023; Zhao J. et al., 2024; Zhao X. et al., 2024).
Changes in microbial community composition, nutrient imbalances in soil, and autotoxicity from root exudates are three primary factors associated with the occurrence of CC obstacles (Garbeva et al., 2004; Zhu et al., 2018; Liu et al., 2019). The P. cablin faces severe CC obstacles; however, current research primarily focuses on its molecular response mechanisms to CC, while studies on its effects on rhizosphere microbial communities, soil enzyme activities, and physicochemical properties remain relatively scarce. This research aims to (1) examine the effects of CC of patchouli on soil physiochemical properties and enzyme activities, (2) the interaction of soil properties and enzyme activities with microorganisms, and the core environmental aspects affecting the rhizosphere microbial communities of patchouli were elucidated, and (3) evaluate the variations in the diversity and structure of microbial communities in the rhizosphere under CC. Ultimately, the study seeks to recognize the impact of CC on patchouli rhizosphere soil and microorganisms, providing a theoretical basis for overcoming CC obstacles and modernizing the production of traditional Chinese medicinal materials.
The experiment material for this study was the “Nanxiang” cultivar of patchouli, sourced from the germplasm source orchard of patchouli plants at Hainan University. This research was conducted at the experimental center of Ducun, Yazhou District, Sanya City, Hainan province (18° 21′23.072” N, 109° 10′7.738” E) (Supplementary Figure S1). The area is specified by a tropical marine monsoon climate, with an average annual temperature ranging from 24.9°C to 26°C. It receives an average of 2,572.8 h of sunlight annually and has precipitation of 1,100–1,300 mm/year. Healthy and pest-free branches of patchouli were taken and propagated by cuttings on a disinfected sand substrate (Yan et al., 2021). After 45 days of propagation, carefully selected patchouli seedlings with consistent growth and robust root systems. The selected seedlings were transferred to plastic pots (22c m x 20 cm x 15 cm), and each pot was filled with soil and organic fertilizer mixture in a 1:6 ratio (organic fertilizer to soil). The experimental material consisted of 120 pots containing four different cropping year soil, with each treatment having three replications and each replication containing ten pots. The pots were filled with soil (sourced from the P. cablin germplasm resource garden of Hainan University; 20° 3′35″ N, 110° 19′8″ E) from four different cropping years, including the zero-year (CK) cropping soil, first-year (T1) cropping soil, second-year (T2) cropping soil, and third-year (T3) CC soil. The experiment was conducted on September 3, 2023. The plants received consistent watering and management practices throughout the experiment for 100 days.
Patchouli started a key phase of persistent cropping obstacles on January 13, 2024: a rapid growth period. Four main phases define the patchouli growth period: slow growth stage, rapid growth stage, mature stage, and fully mature stage (Yan et al., 2022). Soil samples were collected from four different treatments; each treatment contains three replicates, and three pots of patchouli seedlings were randomly selected from each replicate; the rhizosphere soil (2mm from the roots) was then taken and mixed as a biological replicate sample. First, remove the plants, humus, and dry surface soil while sampling soil; then, uproot the patchouli seedlings and gently shake the roots to remove bigger dirt blocks and loosely attached soil. We divided the soil into three sections for further analysis: (1) for immediately stored the rhizosphere soil at −80°C temperature for subsequent metagenomic analysis, (2) for air-dried and sieved (2 mm mesh) soil samples for basic soil physiochemical properties, and (3) for some were stored at 4°C for enzyme activities.
To extract microbial DNA from patchouli rhizosphere soil, follow the manufacturer's directions employing the E.Z.N.A. ® stool DNA Kit (Omega Bio-tek, Norcross, GA, USA). Metagenomic sequencing was conducted by BGI Genomics Co., Ltd., in Shenzhen, China. A Covaris M220 focused ultrasound (Woburn, MA, USA) was used to cleave genomic DNA (1 μg) for individual samples, preparing a sequencing library with a fragment length (450 bp) approximately. The BGI DNBSEQ-T7 instrument was used to sequence all samples in 150 bp paired-end (PE150) mode.
Raw sequence reads were processed using fine-tuning v0.36 (http://www.usadellab.org/cms/uploads/supplementary/Trimmomatic) for quality trimming to eliminate adapter impurities and inferior reads. Subsequently, the BWA mem algorithm (parameters: m-m-32-t16, http://bio-bwa.sourceforge.net/bwa.shtml) was operated to map quality-controlled reads to the genome of patchouli (version: hg38). Clean readings for further investigation were those devoid of low-quality data and host genome contamination.
The FASTP (version 0.18.0) was used to filter the original data, resulting in clean reads for assembly analysis. The filtering standards were as follows: (1) reads having unidentified nucleotides (N) at a proportion of 10% or higher were removed; (2) reads with phred quality scores of 20 or below in at least 50% of the bases were eliminated; and (3) Reads that included adapters were obliterated. The filtered clean reads were then analyzed for assembly (Chen et al., 2018). MEGAHIT (version 1.1.1) was used to assemble the clean reads of the sample, and the continuous long sequences obtained from the assembly were called contigs (Li et al., 2015). MetaGeneMark (version 3.38) was then used to predict genes for contigs longer than 500 bp (Zhu et al., 2010).
Select all gene sequences with a length of ≥300 bp, using CD-HIT (version 4.8.1) to merge the sequences with a sequence similarity of ≥95% and read coverage of >90% into a cluster, and select the best sequence in each cluster (Fu et al., 2012). Long sequences are used as representative sequences (Unigene). Use Bowtie (version 2.2.5) to re-align the reads to Unigene and count the number of reads (Langmead and Salzberg, 2012). Filter the genes whose read support number is <2. The final Unigene obtained is called a non-redundant gene set. The Unigene sequence was compared to the Nr library, and the species annotation of the gene was obtained based on the MEGAN software (version 6.19.9) (Huson et al., 2011) LCA (Lowest Common Ancestor) algorithm (Huson et al., 2007); based on the gene abundance, the species abundance was obtained.
A soil and water deferment (1:2.5, weight/volume) was made to assess the pH of the soil with a pH meter instrument (Model: SevenDirect SD20, Mettler Toledo, Zurich, Switzerland). Soil organic carbon (SOC) was determined using the potassium dichromate volumetric and external heating methods. Available potassium (AK), available phosphorus (AP), ammonium-nitrogen (-N), and nitrate-nitrogen (NO3-N) were determined using acetic acid ammonia extraction and flame photometry (Paul et al., 2024), the molybdenum antimony colorimetric method (Li J. et al., 2023; Li L. et al., 2023; Li Z. et al., 2023), indophenol blue colorimetric method and a flow analyzer, and hydrazine sulfate reduction method, colorimetric, and flow analyzer (Mo et al., 2021), respectively. Soil enzyme activities were measured following the protocol with the respective assay kits (Suzhou Geruisi Biotechnology Co., Ltd. China). For soil catalase (S-CAT) and polyphenol oxidase (S-PPO), 0.10 g of soil was used, while 0.05 g was used for soil ß-glucosidase (S-ß-GC), N-acetyl-β-D-glucosaminidase (S-NAG), and leucine aminopeptidase (S-LAP). Samples were centrifuged under specific conditions: S-CAT, S-ß-GC, and S-NAG at 9,711×g for 10 min at 25°C; S-PPO at 9,711×g for 5 min at 4°C; and S-NAG additionally at 4,316×g for 5 min at 25°C. The supernatant was subsequently collected for enzymatic activity determination and absorbance readings were taken at specific wavelengths: S-CAT (Cat. No. G0303F) at 510 nm, S-PPO (Cat. No. G0311F) at 475 nm, and S-ß-GC (Cat. No. G0312F), S-NAG (Cat. No. G0321F), and S-LAP (Cat. No. G0329F) at 405 nm.
Statistical software was applied to construct an Analysis of variance (ANOVA) based on the Waller-Duncan and Tukey tests to study the significance of alterations in soil properties and enzyme activities at different locations. GraphPad Prism (version 8.0.1) visualized the comprehensive microbial distribution. The stack diagram was created using Wekemo Bioincloud (https://www.bioincloud.tech). Other analyses were conducted using R (version 4.4.1). NMDS (non-metric multidimensional scaling) analysis based on Bray-Curtis distance and hierarchical clustering were employed to determine the variations in composition (microbial community) in CC of patchouli, using the “Vegan” package. The “pheatmap” program was employed to conduct the analysis, resulting in a heat map depicting the functional gene abundance and dominant microorganisms at the genus level in the sample microbial community. The “ggplots” package was employed to generate boxplots of the most prevalent microorganisms at the phylum level. The “igraph” package generated a phylum-level correlation network diagram of dominant microorganisms and COG genes. The “linkET” was utilized to conduct a mental-test analysis of the phylum-level dominant microorganisms and environmental factors. Referring to Lai Jiangshan's method, the impression of environmental aspects on microbial communities and functional genes was explored through redundancy analysis (Lai et al., 2022). To reduce the collinearity of independent variables, variables with the most significant VIF (variance inflation factor) were gradually eliminated until the VIF of all remaining variables was <20. Hierarchical partitioning was then performed to quantify the explanatory degree of different independent variables. Similarly, the “vegan” package drew RDA diagrams of microorganisms and functional genes at the phylum level. The VPA (variance partitioning analysis) was steered to determine the role of soil properties and enzyme activities in the diversity of dominant microbial communities, and “rdacca.hp” was used for variance decomposition. In addition, the “Hmisc” program was used to create a co-occurrence network established on the rank coefficient (r) of patchouli rhizosphere microorganisms at the phylum level. Gephi version 0.9.2 was used to visualize the network, with each node and edge representing a specific species and a substantial association between the two species. The degree of the distinctive topological characteristics indicated the number of edges that connected each node to the remainder of the network. Lastly, network stability was assessed by randomly deleting and repeating nodes to see how rapidly robustness declined. The natural connectedness of the nodes measured network resilience in a static network.
The different CC years altered patchouli plant rhizosphere soil's properties and enzyme activities (Table 1). These obstacles significantly altered the soil pH and ammonium nitrogen (-N), which decreased over the cropping years. In CC, soil pH decreased by 1.5%, 2.7%, and 3.9% in the first, second, and third years of CC, respectively, compared with CK. Meanwhile, soil polyphenol oxidase (S-PPO) and available phosphorus (AP) increased over the CC years. Moreover, soil organic carbon (S0C), available potassium (AK), soil catalase (S-CAT), soil-β-Glucosidase (S-β-GC), soil-Leucine aminopeptidase (S-LAP), and soil N-acetyl-β-D-glucosaminidase (S-NAG) showed a significant increase in first 2 years of CC. It decreased in the third year of cropping, while nitrate nitrogen (NO3-N) has vice versa.
After removing human and host genome sequences, the total soil microbial community of the 12 samples accounted for 91.38%, including bacterial communities were dominant (RAavg; average relative abundance, 88.8%), followed by Archaea (2.11%), viruses (0.27%), and eukaryotes (0.19%) (Figure 1A). Whereas among the four treatments, there was no significant difference in the relative abundance of the bacterial communities. The RA of Archaea was highest at T2 and lowest at T1. Meanwhile, the number of viruses gradually decreased with the years of CC. Moreover, the RA of eukaryotes was highest in CK, decreased in T1, and then increased slowly with CC years. In addition, the microbial structure of the four plots differed significantly at the species level (Figure 1B). Among the four different soil cropping years microbial communities, the main microorganisms at the phylum level are Acidobacteriota, Pseudomonadota, Chloroflexota, Actinomycetota, Bacteroidota, and Eisenbacteria (Figure 2B). Hierarchical clustering analysis of the RA of soil microbial communities based on Bray-Curtis distance showed significant differences in microbial abundance between CK and CC soils (Figure 2A). Compared with CK, the RA of Acidobacteriota, Chloroflexota, Actinomycetota, and Eisenbacteria in CC soil increased, while Pseudomonadota and Bacteroidota's RA decreased. In addition, the RA of other microorganisms in soils planted with patchouli continuously showed significant differences at the phylum level compared with CK. CC significantly increased the abundance of Acidobacterota (Figure 3A), demonstrating that the rhizosphere soil structure contributed to enhanced resilience. The abundance of Pseudomonadota gradually decreased with CC, reaching the lowest value at T3 (Figure 3B). The abundance of Chloroflexota gradually increased but decreased significantly at T3 (Figure 3C). The abundance of Actinomycetota increased substantially at T1 but progressively decreased with CC (Figure 3D). Similarly, the abundance of Bacteroidota was highest during CK and significantly reduced after CC (Figure 3E). The abundance of Eisenbacteria was lowest at CK but gradually increased with CC (Figure 3F). Metagenomic analysis identified 10,183, 8,808, 8,975, and 9,160 microbial genera in CK, T1, T2, and T3, respectively. Among them, 1898, 127, 42, and 44 microbial genera were unique to CK, T1, T2, and T3, respectively (Figure 4A). The heat map analysis provided a more detailed examination of the variations in the prevalence of microbial species belonging to the top 20 dominating genera in the presence of RA under CC circumstances (Figure 4B). Among them, the RA of Sediminibacterium, Sphingomicrobium, Mesorhizobium, Pseudolabrys, Afipia, Variovorax, and Bradyrhizobium in CK was significantly higher than in CC soils. On the contrary, PSRFO1, SCGC-AG-212-J23, 2-12-FULL-66-21, QHWTO1, UBA12294, JAIOPA01, Gp6-AA40, JACDCA01, OLB14, and CADEEDO1 have higher abundance in CC soil than CK.
Figure 1. CC impact on soil microbial community composition and classification. (A) The classification of bacteria, archaea, eukaryotes, and viruses at different CC years was analyzed (n = 4). (B) NMDS (non-metric multidimensional scaling) analysis based on Bray-Curtis differences showed significant species-level differences in soil microbial composition. The stress value indicates the model's applicability. The model is deemed a good fit (P < 0.001) when the stress value is <0.05.
Figure 2. Hierarchical clustering analysis of soil microbial communities based on Bray-Curtis distance (A), stacked plot of soil microbial abundance based on phylum level (B).
Figure 3. Soil microbial communities abundance at phylum level changes in CC of patchouli. Due to the long-term CC, the abundance of Acidobacteriota (A), Pseudomonadota (B), Chloroflexota (C), Actinomycetota (D), Bacteroidota (E), and Eisenbacteria (F) has changed. Different alphabets showed notable variations (p < 0.05, n = 4).
Figure 4. Soil microbial community domain genera affected by CC IN patchouli plant. (A) The Upset graph shows common and unique microbial community characteristics at the genus level. (B) The heat-map shows the differences between the Top 20 dominant microbial community at the genus level.
Variations in the RA of numerous functional genes in the soil microbial community are induced by CC. Sample clustering analysis of heat maps was used to group samples from the same consecutive planting years. There were significant differences between CK and other groups. The most enormous RA disparity in various functional genes was between the CK and T3 groups (Figure 5). Functional genes interact significantly with the community makeup of soil microbial communities. COG0823 was substantially more abundant than the other functional genes. It was favorably associated with many functional genes, with a stronger association with COG2010, COG1595, and COG0515. It showed a negative correlation with COG1028 and COG0477 (Figure 6A). Furthermore, COG0500, COG0515, COG0457, and COG2010 have intricate network connections and interact with several functional genes. However, the COG0745 network link is apparent and positively correlated with COG2205. Bacteroidota, the most prevalent phylum, had a negative correlation with Tectomicrobia but a positive correlation with Cyanobacteriota, Nitrospirota, and CSP1–3 (Figure 6B). Myxococcota's network interaction is quite essential and positively correlated to Verrucomicrobiota.
Figure 5. Heat map of functional gene abundance (eggNOG, top30) in microbial communities at 4 soil sampling points. COG ID, function, description, and categories are shown in Supplementary Table S1.
Figure 6. Top 15 functional genes (A) and top 20 soil microbial community at phylum level (B) correlation network diagram. This link is indicated by node connections (Spearman correlation coefficient, r = 0.6; p = 0.05). The abundance is proportional to the node size. Supplementary Table S1 displays each category's functional gene database ID, function, category, and description.
Changes in soil properties and enzyme activities are substantially correlated with the diversity of the dominant Phyla of microorganisms due to CC. Among them, AK is associated with Acidobacteriota and Bacteroidota, -N is associated with Gemmatimonadota, and CAT is associated with Planctomycetota, and their relative abundances are remarkably significantly correlated (p < 0.01); SOC is associated with Gemmatimonadota and Planctomycetota, NO3-N is associated with Gemmatimonadota, AK is associated with Actinomycetota, and LAP and Gemmatimonadota, CAT and Gemmatimonadota, whose relative abundances were significantly correlated (p < 0.05) (Figure 7A). RDA study revealed that soil physiochemical variables influence the number of functional genes in microbial communities. We implemented redundancy analysis to investigate the influence of environmental assays on functional genes. In order to reduce the collinearity of independent variables, we gradually eliminated variables with the most significant variance inflation factors (VIF) until the VIF of all remaining variable values was <20. Subsequently, we performed hierarchical segmentation of these factors to quantify the explanatory degree of different independent variables. Five major environmental factors (pH, SOC, -N, NO3-N, AK) affecting functional genes were screened. The first two axes accounted for 94.84% and 1.13% of the total change in the model, while the explanatory variables accounted for 99.97% (Figure 7B). AK, SOC, and NO3-N correlate positively with the first ranking axis, and pH and -N negatively correlate with the first ranking axis. pH, SOC, and AK are environmental factors with a large contribution rate, and the functional genes of each treatment are mainly affected by these three soil factors. pH is negatively correlated with the gene abundance of TOP10; SOC is highly positively correlated with the gene abundance of COG0577 genes involved in cellular processes and signal transduction and COG4771 genes involved in metabolic processes; AK is positively correlated with the abundance of multiple functional genes, the most relevant one is the COG0823 gene involved in cellular processes and signal transduction. While, VPA analysis based on Hierarchical Partitionin determined the degree of influence of various soil factor variables on microbial community diversity at the phylum level. SOC, pH, and AK had the most significant impact on microbial community diversity at the phylum level, accounting for 50.84%, 25.25%, and 12.92%, respectively (Figure 7C).
Figure 7. Soil chemical assays and enzyme activities influence microbial composition and functional genes. (A) Mantel-test analysis demonstrates the relationship between dominating microbial diversity at the phylum level and soil chemical characteristics and enzyme activities. (B) RDA (Redundancy analysis) analysis reveals the effects of soil chemical assays and enzyme activities on functional genes. (C) VPA analysis shows the contribution of soil chemistry and enzyme activities to the diversity of dominant microbial communities. Supplementary Table S1 displays each category's functional gene database ID, function, category, description, and meaning.
The Spearman rank correlation method generated a symbiotic network to identify the interactions between microorganisms in CC patchouli at the modular level. Every point and line in the network relates to a species of patchouli and interactions among species. The network structure's colors reflect module-level clustering products of microbial interactions. According to co-occurrence network analysis, nodes are necessary for establishing a modular structure since the probability of coexistence of microorganisms within the same module is increased. The microbial network in the patchouli rhizosphere showed different patterns in different CC years (Figure 8). This study divided the rhizosphere soil microorganisms of CC patchouli into four main modules: CK, T1, T2, and T3. The stability of the microbial communities of T1 and T2 was higher than CK and T3, respectively (Figure 9).
Figure 8. The co-occurrence network is constructed based on patchouli rhizosphere microorganisms' grade coefficient (r) at the species level under CC. Through network analysis, the co-occurrence pattern of patchouli rhizosphere microbial communities under different CC years is revealed. Nodes are colored according to different modularities. The size of each node is proportional to the degrees. A join indicates a strong (SparCC |r| > 0.5) and significant (P < 0.05) correlation.
Figure 9. Average degree and network robustness during network invulnerability test in the CC of patchouli.
CC obstacles not only affect plant growth but also retard root growth and development over the years (Zeeshan Ul Haq et al., 2023). Similarly, in patchouli plants, CC changed the soil composition and enzyme activities, which usually led to an imbalance of soil nutrients and increased pathogenic microorganisms (Zeng et al., 2020). It also stimulated the plant's roots to exude allelopathic substances, making the soil more susceptible to damage and invasion by infectious diseases (Liu et al., 2022; Zeeshan Ul Haq et al., 2023). The environmental factors in rhizosphere soil changed under the influence of CC, in which pH and -N decreased year after year in our results. At the same time, the concentration of AP increased with the CC years, but NO3-N content observed fluctuations with the passing years (Table 1). This decrease in soil pH and -N can negatively impact nutrient availability, alter microbial communities, and potentially increase metal toxicity, nitrogen deficiency in plants, disrupt the overall nitrogen cycle, and influence soil microbial composition in the soil (Barth et al., 2019; Gu et al., 2022; Wang F. et al., 2024; Wang K. et al., 2024). NO3-N and AP fluctuations can lead to inconsistent nitrogen availability for plants, affecting growth and yield stability across cropping years (Ferretti et al., 2021). The enzyme activities of the rhizosphere soil are also affected by CC. Among them, the four enzyme activities of LAP, NAG, CAT, and GC showed a significant increase compared with CK at the first 2 cropping years (T1 and T2), then decreased at the third (T3), while PPO increased year after year in CC. The CC might alter soil microbial community diversity and composition, raising the changes in pathways (microbial metabolic) and altering the soil enzyme activities (Liu et al., 2022). This suggested that while initial years of cropping may stimulate specific soil biological processes, prolonged CC can lead to alterations in soil biochemical functions (Qu et al., 2022).
The total microbial communities in patchouli rhizosphere soil in CC accounted for 91.38%, of which the bacterial community was dominant (RAavg, 88.8%), and the RA difference between the four treatments was minimal. The RA of eukaryotes and viruses decreased significantly in CC (Figure 1). This suggests that eukaryotes and viruses are more susceptible to variations in the soil environment than bacteria in the soil. A study on the change of environmental factors on eukaryotic communities in soil found that eukaryotes are more sensitive to temperature and humidity alterations than bacteria in the soil (Zhao et al., 2018). Similarly, another investigation discovered that land use patterns significantly influence the structure and functionality of the soil virus communities (Liao et al., 2022). These findings suggest that eukaryotes and viruses were more susceptible to changes in the soil environment than bacteria. Environmental factors play a crucial role in shaping their communities and functions. After CC, the patchouli rhizosphere microbial community has undergone significant changes. From the perspective of dominant microorganisms at the phylum level, compared with CK, the RA of Acidobacteriota, Chloroflexota, Actinomycetota, and Eisenbacteria in CC soil increased, and Pseudomonadota and the RA of Bacteroidota decreased (Figure 2). The increase in these phyla suggests an adaptation of the soil microbial community to the specific conditions, including Acidobacteriota might induced by acidic conditions (Zeng et al., 2020), Chloroflexota increased might be due to the accumulation of specific organic substrates from patchouli root exudates (Wang F. et al., 2024; Wang K. et al., 2024), Actinomycetota induced due to decomposition activities in the rhizosphere (Yao et al., 2020), and Eisenbacteria associated with the organic matter decomposition which aligns with the continuous input of plant-derived materials in monoculture systems (Li et al., 2024) created by CC of patchouli. Similarly, RA of Pseudomonadota is often associated with plant growth promotion and nutrient cycling (Yao et al., 2020; Zeng et al., 2020). Bacteroidota is known for degrading complex organic compounds, and its reduction could affect soil organic matter dynamics (Zeng et al., 2020; Sui et al., 2023). These changes are consistent with observations in other CC systems, such as the similar shifts in bacterial communities reported by Liu et al. in continuously cropped cucumber rhizosphere soil (Yao et al., 2020). There are 1898, 127, 42, and 44 unique microbial genera in CK, T1, T2, and T3, respectively (Figure 4). At the genus level, the number of unique microbial genera in the soil significantly decreased across CC years. Studies have shown that as the duration of CC increased, the number of unique microbial genera decreased prominently, leading to a loss of overall microbial diversity and soil functional capacity (Huang et al., 2023). This observation is consistent with other research, indicating that CC reduces microbial diversity in Cucumis sativus and A. hypogaea (Zhou et al., 2020; Yao et al., 2020).
After CC, the soil's RA of various functional genes changed significantly. Among these changes, the difference in RA between the CK and T3 was the most pronounced. The data revealed a significant interaction between functional genes and the composition of soil microbial communities (Figure 5). This interaction is crucial as it implies that changes in the abundance of certain functional genes can influence the overall structure and function of the microbial community. For instance, research has demonstrated that the diversity and abundance of genes substantially affect the structure and function of microbial communities (Jiang et al., 2019). This dynamic relationship highlighted the importance of understanding how different functional genes interact within the soil composition (Cong et al., 2015; Waldrop et al., 2023). The functional gene COG0823, associated with cell signal transduction (Supplementary Table S1), exhibits significantly higher abundance than other functional genes (Figure 6A), highlighting its core role in soil microbial networks. Previous research suggested this gene is involved in bacterial pathogenicity (Szczepaniak et al., 2020). Furthermore, COG0823 is crucial in nutrient cycling, decomposition, and other essential microbial processes (Ge et al., 2018). Studies showed that soils with a high abundance of COG0823 host-specific microbial groups associated with nutrient cycling exhibit increased populations. This suggests a synergistic relationship that enhances soil fertility (Ding et al., 2024). In contrast, COG0745 demonstrated a relatively simple network relationship, being positively correlated only with COG2205 (Figure 6A). The limited interactions of COG0745 suggest it may play a specialized role within microbial communities (Ma et al., 2022). The positive correlation between COG0745 and COG2205 indicates a potential cooperative relationship between these two genes, which could be critical for specific ecological functions, such as facilitating symbiotic interactions or supporting shared metabolic pathways (Wu et al., 2005, 2020). For instance, the metabolic pathways involving COG0745 may rely on the material transport facilitated by COG2205. This positive correlation underscores their potential functional connections and highlights their significant roles in soil ecosystems. Future research could further investigate the specific molecular mechanisms underlying this relationship and its implications for soil health and ecological balance.
In continuous patchouli cropping systems, microbial communities exhibit notable interactions and correlations at the phylum level. Specifically, Bacteroidota, which had the highest abundance, was negatively associated with Tectomicrobia and positively associated with Cyanobacteriota, Nitrospirota, and CSP1-3. Additionally, the network relationship of Myxococcota was comparatively easy, being only positively associated with Verrucomicrobiota (Figure 6B). Bacteroidota, a dominant phylum in the patchouli rhizosphere, is crucial in soil health and nutrient cycling. The negative correlation with Tectomicrobia suggests competitive or antagonistic interactions, possibly due to competition for similar ecological niches or resources. Conversely, the positive correlations with Cyanobacteriota, Nitrospirota, and CSP1-3 indicate potential synergistic relationships. Cyanobacteria are known for their nitrogen-fixing capabilities, which could benefit Bacteroidota by enhancing soil fertility. Similarly, Nitrospirota, involved in nitrogen cycling, might create a favorable environment for Bacteroidota through their metabolic activities (Zeng et al., 2020; Yang et al., 2022; Kamada et al., 2023). The Myxococcota positive association with Verrucomicrobiota could be indicative of a mutualistic relationship where both phyla benefit from each other's presence, possibly through nutrient exchange or habitat modification (Kamada et al., 2023; Li J. et al., 2023; Li L. et al., 2023; Li Z. et al., 2023). The CC altered soil physiochemical properties and enzyme activities, significantly impacting the diversity and relative abundances of dominant microbial phyla, including AK and Acidobacteriota and Bacteroidota, -N and Gemmatimonadota, and CAT and Planctomycetota, with a significant correlation (p < 0.01) (Figure 7A), verified by the previous studies (Alami et al., 2021; Zhang et al., 2020; Yang et al., 2023; Song et al., 2024; Wang F. et al., 2024; Wang K. et al., 2024). RDA analysis between functional genes and environmental factors revealed that pH, SOC, and AK are key factors influencing the function of microbial communities (Figure 7B). Research has demonstrated that soil pH influences the bacterial communities in agricultural soils more significantly than nutrient content. Changes in pH can alter the microbial mechanisms of carbon accumulation and decomposition, impacting SOC dynamics (Malik et al., 2018; Wang et al., 2019; Kang et al., 2021). The microbial network in the patchouli rhizosphere exhibited different patterns across various CC years (Figure 8). CC decreased the stability of the patchouli rhizosphere microbial community, with the stability of T1 and T2 being higher than that of T3 and CK (Figure 9). The reduced stability of long-term CC suggests that extended CC may hinder key soil functions (disease suppression, nutrient cycling, and decomposition of organic matter) to lower soil fertility and crop production (Liu et al., 2020; Fox et al., 2022; Li J. et al., 2023; Li L. et al., 2023; Li Z. et al., 2023). Research showed that in CC systems, the reduction of microbial diversity leads to a weakened ability of soil to suppress diseases, consequently increasing the incidence of crop diseases (Olanrewaju and Babalola, 2022; Cao et al., 2024). The CC shortens microbial community structures, reduces the efficiency of organic matter decomposition, and ultimately results in decreased soil fertility (Yuan et al., 2021). The potential mechanisms underlying these phenomena include decreased nutrient availability. The CC can deplete essential nutrients in the soil or alter their availability, which may reduce the populations of beneficial microorganisms responsible for disease suppression and nutrient cycling. Studies have reported a correlation between improved soil nutrient status and enhanced disease suppression capabilities (Cao et al., 2024). In stable ecosystems with high microbial diversity, beneficial microorganisms effectively compete with pathogens for resources. However, pathogens may proliferate unchecked in systems where unique microbial genera are lost due to CC (Zhou et al., 2020). This shift increases disease pressure on crops and disrupts nutrient cycling processes mediated by beneficial microbes. Based on this study, we suggest that future efforts can focus on developing bio-fertilizers and monitoring and controlling environmental factors that significantly increase or decrease after CC that can alleviate CC obstacles.
In summary, CC is an unavoidable farming method in patchouli cultivation and production. On the other hand, prolonged CC alters the rhizosphere microorganisms while negatively affecting the physiochemical properties of the soil, enzyme activities, and microbial communities structure and their functions; after that, the microbial community is reduced. The microbial community structure of the patchouli rhizosphere soil was significantly altered by CC, as bacteria and archaea are more susceptible to adapting to changes in soil conditions than eukaryotes and viruses. The primary environmental factors influencing the makeup of the abundance of microbial community function genes are soil pH, SOC, and AK. Each successive year of CC diminishes microbial community stability in the patchouli rhizosphere. These findings showed that CC alters the structure and functions of the microbial communities and the soil's physiochemical and enzyme activities. Additionally, as CC increases, the stability of the microbial community declines. This work established a theoretical foundation for the obstacles faced in continuously cultivating patchouli. It comprehensively assessed the impacts of CC on the soil assays and microbial community structure in the patchouli rhizosphere.
The datasets presented in this study can be found in online repositories. The names of the repository/repositories and accession number(s) can be found below: https://www.ncbi.nlm.nih.gov/, PRJNA1191635.
GG: Conceptualization, Data curation, Formal analysis, Investigation, Methodology, Resources, Software, Validation, Visualization, Writing – original draft, Writing – review & editing. MZUH: Conceptualization, Data curation, Formal analysis, Investigation, Methodology, Resources, Software, Validation, Visualization, Writing – original draft, Writing – review & editing. XS: Formal analysis, Methodology, Resources, Software, Writing – review & editing. JZ: Formal analysis, Methodology, Resources, Visualization, Writing – review & editing. JY: Methodology, Resources, Software, Validation, Visualization, Writing – review & editing. YL: Formal analysis, Investigation, Methodology, Resources, Software, Writing – review & editing. DY: Formal analysis, Investigation, Methodology, Resources, Writing – review & editing. HY: Formal analysis, Investigation, Methodology, Resources, Writing – review & editing. YW: Conceptualization, Data curation, Formal analysis, Funding acquisition, Investigation, Methodology, Project administration, Resources, Software, Supervision, Validation, Visualization, Writing – original draft, Writing – review & editing.
The author(s) declare financial support was received for the research, authorship, and/or publication of this article. This study was financially supported by the National Natural Science Foundation of China (No. 82260737) and the Hainan Province Graduate Student Innovation Program (Qhys2023-244).
The authors declare that the research was conducted in the absence of any commercial or financial relationships that could be construed as a potential conflict of interest.
All claims expressed in this article are solely those of the authors and do not necessarily represent those of their affiliated organizations, or those of the publisher, the editors and the reviewers. Any product that may be evaluated in this article, or claim that may be made by its manufacturer, is not guaranteed or endorsed by the publisher.
The Supplementary Material for this article can be found online at: https://www.frontiersin.org/articles/10.3389/fmicb.2024.1482904/full#supplementary-material
Alami, M. M., Pang, Q., Gong, Z., Yang, T., Tu, D., Zhen, O., et al. (2021). Continuous cropping changes the composition and diversity of bacterial communities: A meta-analysis in nine different fields with different plant cultivation. Agriculture 11:1224. doi: 10.3390/agriculture11121224
Barth, G., Otto, R., Almeida, R. F., Cardoso, E. J. B. N., Cantarella, H., and Vitti, G. C. (2019). Conversion of ammonium to nitrate and abundance of ammonium-oxidizing-microorganism in Tropical soils with nitrification inhibitor. Sci. Agric. 77:e20180370. doi: 10.1590/1678-992x-2018-0370
Cao, Y., Ou, Y., Lv, N., and Zhang, H. (2024). Soil nutrient levels associated with suppression of banana Fusarium wilt disease. Soil Ecol. Lett. 6, 1–6. doi: 10.1007/s42832-024-0247-1
Chakrapani, P., Venkatesh, K., Singh, B. C. S., Jyothi, B. A., Kumar, P., Amareshwari, P., et al. (2013). Phytochemical, pharmacological importance of Patchouli (Pogostemon cablin (Blanco) Benth) an aromatic medicinal plant. Int. J. Pharm. Sci. Rev. Res. 21, 7−15.
Chen, S., Zhou, Y., Chen, Y., and Gu, J. (2018). Fastp: an ultra-fast all-in-one FASTQ preprocessor. Bioinf. 34, i884–i890. doi: 10.1093/bioinformatics/bty560
Cong, J., Yang, Y., Liu, X., Lu, H., Liu, X., Zhou, J., et al. (2015). Analyses of soil microbial community compositions and functional genes reveal potential consequences of natural forest succession. Sci. Rep. 5:10007. doi: 10.1038/srep10007
Ding, M., Dai, H., He, Y., Liang, T., Zhai, Z., Zhang, S., et al. (2024). Continuous cropping system altered soil microbial communities and nutrient cycles. Front. Microbiol. 15:1374550. doi: 10.3389/fmicb.2024.1374550
Ferretti, G., Galamini, G., Deltedesco, E., Gorfer, M., Fritz, J., Faccini, B., et al. (2021). Gross ammonification and nitrification rates in soil amended with natural and NH4-enriched chabazite zeolite and nitrification inhibitor DMPP. Appl. Sci. 11:2605. doi: 10.3390/app11062605
Fox, A., Widmer, F., and Lüscher, A. (2022). Soil microbial community structures are shaped by agricultural systems revealing little temporal variation. Environ. Res. 214:113915. doi: 10.1016/j.envres.2022.113915
Fu, L., Niu, B., Zhu, Z., Wu, S., and Li, W. (2012). CD-HIT: accelerated for clustering the next-generation sequencing data. Bioinf. 28, 3150–3152. doi: 10.1093/bioinformatics/bts565
Gao, Z., Han, M., Hu, Y., Li, Z., Liu, C., Wang, X. T., et al. (2019). Effects of continuous cropping of sweet potato on the fungal community structure in rhizospheric soil. Front. Microbiol. 10:2269. doi: 10.3389/fmicb.2019.02269
Garbeva, P., Van Veen, J. A., and Van Elsas, J. D. (2004). Microbial diversity in soil: selection of microbial populations by plant and soil type and implications for disease suppressiveness. Ann. Rev. Phytopathol. 42, 243–270. doi: 10.1146/annurev.phyto.42.012604.135455
Ge, C. H., Sun, N., Kang, Q., Ren, L. F., Ahmad, H. A., Ni, S. Q., et al. (2018). Bacterial community evolutions driven by organic matter and powder activated carbon in simultaneous anammox and denitrification (SAD) process. Biores. Tech. 251, 13–21. doi: 10.1016/j.biortech.2017.12.017
Gu, X., Yang, N., Zhao, Y., Liu, W., and Li, T. (2022). Long-term watermelon continuous cropping leads to drastic shifts in soil bacterial and fungal community composition across gravel mulch fields. BMC. Microbiol. 22:189. doi: 10.1186/s12866-022-02601-2
Huang, S., Yu, J., Hou, D., Yue, H., Zhang, D., Li, Y., et al. (2023). Response of soil microbial community diversity to continuous cucumber cropping in facilities along the Yellow River irrigation area. PLoS ONE 18:e0289772. doi: 10.1371/journal.pone.0289772
Huson, D. H., Auch, A. F., Qi, J., and Schuster, S. C. (2007). MEGAN analysis of metagenomic data. Genome. Res. 17, 377–386. doi: 10.1101/gr.5969107
Huson, D. H., Mitra, S., Ruscheweyh, H. J., Weber, N., and Schuster, S. C. (2011). Integrative analysis of environmental sequences using MEGAN4. Genome. Res. 21, 1552–1560. doi: 10.1101/gr.120618.111
Jaiswal, S. K., Mohammed, M., Ibny, F. Y., and Dakora, F. D. (2021). Rhizobia as a source of plant growth-promoting molecules: potential applications and possible operational mechanisms. Front. Sustain. Food Syst. 4:619676. doi: 10.3389/fsufs.2020.619676
Jiang, X., Li, X., Yang, L., Liu, C., Wang, Q., Chi, W., et al. (2019). How microbes shape their communities? A microbial community model based on functional genes. Genom. Proteom. Bioinf. 17, 91–105. doi: 10.1016/j.gpb.2018.09.003
Kamada, S., Wakabayashi, R., and Naganuma, T. (2023). Phylogenetic revisit to a review on predatory bacteria. Microorganisms 11:1673. doi: 10.3390/microorganisms11071673
Kang, E., Li, Y., Zhang, X., Yan, Z., Wu, H., Li, M., et al. (2021). Soil pH and nutrients shape the vertical distribution of microbial communities in an alpine wetland. Sci. Total. Environ. 774:145780. doi: 10.1016/j.scitotenv.2021.145780
Kartini, N. L., Saifulloh, M., Trigunasih, N. M., Sukmawati, N. M. S., and Mega, I. (2024). Impact of long-term continuous cropping on soil nutrient depletion. Ecol. Eng. Environ. Tech. 25:191953. doi: 10.12912/27197050/191953
Lai, J. S., Zou, Y., Zhang, J. L., and Peres-Neto, P. R. (2022). Generalizing hierarchical and variation partitioning in multiple regression and canonical analyses using the rdacca.hp R package. Methods. Ecol. Evol. 13, 782–788. doi: 10.1111/2041-210X.13800
Langmead, B., and Salzberg, S. L. (2012). Fast gapped-read alignment with Bowtie 2. Nat. Methods. 9:357. doi: 10.1038/nmeth.1923
Lei, H., Liu, A., Hou, Q., Zhao, Q., Guo, J., and Wang, Z. (2020). Diversity patterns of soil microbial communities in the Sophora flavescens rhizosphere in response to continuous monocropping. BMC Microbiol. 20, 1–12. doi: 10.1186/s12866-020-01956-8
Li, D., Liu, C. M., Luo, R., Sadakane, K., and Lam, T. W. (2015). MEGAHIT: an ultra-fast single-node solution for large and complex metagenomics assembly via succinct de Bruijn graph. Bioinform. 31, 1674–1676. doi: 10.1093/bioinformatics/btv033
Li, G., Gong, P., Zhou, J., Wang, L., Song, X., Ding, P., et al. (2024). The succession of rhizosphere microbial community in the continuous cropping soil of tobacco. Front. Environ. Sci. 11:1251938. doi: 10.3389/fenvs.2023.1251938
Li, J., Cheng, X., Chu, G., Hu, B., and Tao, R. (2023). Continuous cropping of cut chrysanthemum reduces rhizospheric soil bacterial community diversity and co-occurrence network complexity. Appl. Soil. Ecol. 185:104801. doi: 10.1016/j.apsoil.2022.104801
Li, L., Huang, D., Hu, Y., Rudling, N. M., Canniffe, D. P., Wang, F., et al. (2023). Globally distributed Myxococcota with photosynthesis gene clusters illuminate the origin and evolution of a potentially chimeric lifestyle. Nat. Commun. 14:6450. doi: 10.1038/s41467-023-42193-7
Li, X. G., Ding, C. F., Zhang, T. L., and Wang, X. X. (2014). Fungal pathogen accumulation at the expense of plant-beneficial fungi as a consequence of consecutive peanut monoculturing. Soil. Biol. Biochem. 72, 11–18. doi: 10.1016/j.soilbio.2014.01.019
Li, Z., Wang, Y., Liu, Z., Han, F., Chen, S., and Zhou, W. (2023). Integrated application of phosphorus-accumulating bacteria and phosphorus-solubilizing bacteria to achieve sustainable phosphorus management in saline soils. Sci. Total Environ. 885:163971. doi: 10.1016/j.scitotenv.2023.163971
Liao, H., Li, H., Duan, C. S., Zhou, X. Y., Luo, Q. P., An, X. L., et al. (2022). Response of soil viral communities to land use changes. Nat. Commun. 13:6027. doi: 10.1038/s41467-022-33771-2
Liu, H., Pan, F., Han, X., Song, F., Zhang, Z., Yan, J., et al. (2019). Response of soil fungal community structure to long-term continuous soybean cropping. Front. Microbiol. 9:3316. doi: 10.3389/fmicb.2018.03316
Liu, L., Cao, H., Geng, Y., Zhang, Q., Bu, X., and Gao, D. (2022). Response of soil microecology to different cropping practice under Bupleurum chinense cultivation. BMC Microbiol. 22:223. doi: 10.1186/s12866-022-02638-3
Liu, X., Zeeshan Ul Haq, M., Yu, J., Liu, Y., Yang, H., Cui, H., et al. (2023). Identification of the CDPK gene family in patchouli and functional analysis in response to continuous cropping stress. Front. Plant Sci. 14:1300073. doi: 10.3389/fpls.2023.1300073
Liu, Z., Liu, J., Yu, Z., Yao, Q., Li, Y., Liang, A., et al. (2020). Long-term continuous cropping of soybean is comparable to crop rotation in mediating microbial abundance, diversity and community composition. Soil Till. Res. 197:104503. doi: 10.1016/j.still.2019.104503
Ma, X., Wang, T., Shi, Z., Chiariello, N. R., Docherty, K., Field, C. B., et al. (2022). Long-term nitrogen deposition enhances microbial capacities in soil carbon stabilization but reduces network complexity. Microbiome 10:112. doi: 10.1186/s40168-022-01349-1
Maheshwari, M. L., Vasantha, K. T., Sharma, N., and Chandel, K. P. S. (1993). Patchouli-An Indian perspective. Indian Perfumer 37, 9–11.
Malik, A. A., Puissant, J., Buckeridge, K. M., Goodall, T., Jehmlich, N., Chowdhury, S., et al. (2018). Land use driven change in soil pH affects microbial carbon cycling processes. Nat. Commun. 9:3591. doi: 10.1038/s41467-018-05980-1
Mo, Y., Peng, F., Gao, X., Xiao, P., Logares, R., Jeppesen, E., et al. (2021). Low shifts in salinity determined assembly processes and network stability of microeukaryotic plankton communities in a subtropical urban reservoir. Microbiome 9:128. doi: 10.1186/s40168-021-01079-w
Olanrewaju, O. S., and Babalola, O. O. (2022). The rhizosphere microbial complex in plant health: a review of interaction dynamics. J. Integr. Agric. 21, 2168–2182. doi: 10.1016/S2095-3119(21)63817-0
Paul, S., Das, D., Barman, M., Verma, B. C., Sinha, A. K., and Datta, A. (2024). Selection of a suitable extractant for sequential leaching of soil to evaluate medium-term potassium availability to plants. J. Soil. Sci. Plant. Nutr. 24, 1489–1506. doi: 10.1007/s42729-024-01654-8
Qu, Y., Tang, J., Liu, B., Lyu, H., Duan, Y., Yang, Y., et al. (2022). Rhizosphere enzyme activities and microorganisms drive the transformation of organic and inorganic carbon in saline–alkali soil region. Sci. Rep. 12:1314. doi: 10.1038/s41598-022-05218-7
Song, R., Zhu, W. Z., Li, H., and Wang, H. (2024). Impact of wine-grape continuous cropping on soil enzyme activity and the composition and function of the soil microbial community in arid areas. Front. Microbiol. 15:1348259. doi: 10.3389/fmicb.2024.1348259
Sui, J., Yang, J., Li, C., Zhang, L., and Hua, X. (2023). Effects of a microbial restoration substrate on plant growth and rhizosphere microbial community in a continuous cropping poplar. Microorganisms 11:486. doi: 10.3390/microorganisms11020486
Swamy, M. K., Balasubramanya, S., and Anuradha, M. (2010). In vitro multiplication of Pogostemon cablin Benth. through direct regeneration. Afr. J. Biotechnol. 9, 2069–2075.
Szczepaniak, J., Press, C., and Kleanthous, C. (2020). The multifarious roles of Tol-Pal in Gram-negative bacteria. FEMS Microbiol. Rev. 44, 490–506. doi: 10.1093/femsre/fuaa018
Tan, Y., Cui, Y., Li, H., Kuang, A., and Ji, X. (2016). Rhizospheric soil and root endogenous fungal diversity and composition in response to continuous Panax notoginseng cropping practices. Microbiol. Res. 194, 10–19. doi: 10.1016/j.micres.2016.09.009
Tian, L., Shi, S., Ma, L., Tran, L. P., and Tian, C. (2020). Community structures of the rhizomicrobiomes of cultivated and wild soybeans in their continuous cropping. Microbiol. Res. 232:126390. doi: 10.1016/j.micres.2019.126390
Toledo, S., Bondaruk, V. F., Yahdjian, L., Oñatibia, G. R., Loydi, A., and Alberti, J. (2023). Environmental factors regulate soil microbial attributes and their response to drought in rangeland ecosystems. Sci. Total Environ. 892:164406. doi: 10.1016/j.scitotenv.2023.164406
Waldrop, M. P., Chabot, C. L., Liebner, S., Holm, S., Snyder, M. W., Dillon, M., et al. (2023). Permafrost microbial communities and functional genes are structured by latitudinal and soil geochemical gradients. ISME J. 17, 1224–1235. doi: 10.1038/s41396-023-01429-6
Wang, C. Y., Zhou, X., Guo, D., Zhao, J. H., Yan, L., Feng, G. Z., et al. (2019). Soil pH is the primary factor driving the distribution and function of microorganisms in farmland soils in northeastern China. Ann. Microbiol. 69, 1461–1473. doi: 10.1007/s13213-019-01529-9
Wang, F., Wang, X., Zhao, R., Zhang, L., Li, S., Zhang, N., et al. (2024). Differences in the soil prokaryotic microbiome during continuous and single cultivation of three varieties of chinese cabbage. Eur. Soil. Sci. 2024, 1–14. doi: 10.1134/S1064229323603566
Wang, K., Lu, Q., Dou, Z., Chi, Z., Cui, D., Ma, J., et al. (2024). A review of research progress on continuous cropping obstacles. Front. Agric. Sci. Eng. 11, 253–270. doi: 10.15302/J-FASE-2024543
Wu, H., Mao, F., Olman, V., and Xu, Y. (2005). “Accurate prediction of orthologous gene groups in microbes,” in 2005 IEEE Computational Systems Bioinformatics Conference (CSB'05) (Stanford, CA: IEEE), 73–79.
Wu, L., Yang, B., Li, M., Chen, J., Xiao, Z., Wu, H., et al. (2020). Modification of rhizosphere bacterial community structure and functional potentials to control Pseudostellaria heterophylla replant disease. Plant Dis. 104, 25–34. doi: 10.1094/PDIS-04-19-0833-RE
Wu, X., Zhao, Q., Zhao, J., Xun, W., Li, R., Zhang, R., et al. (2015). Different continuous cropping spans significantly affect microbial community membership and structure in a vanilla-grown soil as revealed by deep pyrosequencing. Microb. Ecol. 70, 209–218. doi: 10.1007/s00248-014-0516-0
Wu, Y., Guo, Q., He, J., Lin, Y., Luo, L., and Liu, G. (2010). Genetic diversity analysis among and within populations of Pogostemon cablin from China with ISSR and SRAP markers. Biochem. Syst. Ecol. 38, 63–72. doi: 10.1016/j.bse.2009.12.006
Xi, H., Shen, J., Qu, Z., Yang, D., Liu, S., Nie, X., and Zhu, L. (2019). Effects of Long-term cotton continuous cropping on soil microbiome. Sci. Rep. 9:18297. doi: 10.1038/s41598-019-54771-1
Yan, W., Cao, S., Liu, X., Yao, G., Yu, J., Zhang, J., et al. (2022). Combined physiological and transcriptome analysis revealed the response mechanism of Pogostemon cablin roots to p-hydroxybenzoic acid. Front. Plant Sci. 13:980745. doi: 10.3389/fpls.2022.980745
Yan, W., Yang, Y., Wu, Y., Yu, J., Zhang, J., Yang, D., et al. (2021). Isopentenyl diphosphate isomerase (IPI) gene silencing negatively affects patchouli alcohol biosynthesis. in Pogostemon cablin. Plant Mol. Biol. Rep. 1–9. doi: 10.1007/s11105-020-01269-0
Yang, X., Zhang, K., Shaghaleh, H., Qi, Z., Gao, C., Chang, T., et al. (2023). Continuous cropping alters soil hydraulic and physicochemical properties in the karst region of southwestern China. Agronomy 13:1416. doi: 10.3390/agronomy13051416
Yang, Z. N., Liu, Z. S., Wang, K. H., Liang, Z. L., Abdugheni, R., Huang, Y., et al. (2022). Soil microbiomes divergently respond to heavy metals and polycyclic aromatic hydrocarbons in contaminated industrial sites. Environ. Sci. Ecotech. 10:100169. doi: 10.1016/j.ese.2022.100169
Yao, Y., Yao, X., An, L., Bai, Y., Xie, D., and Wu, K. (2020). Rhizosphere bacterial community response to continuous cropping of Tibetan barley. Front. Microbiol. 11:551444. doi: 10.3389/fmicb.2020.551444
Yuan, M., Yu, T., Shi, Q., Han, D., Yu, K., Wang, L., et al. (2021). Rhizosphere soil bacterial communities of continuous cropping-tolerant and sensitive soybean genotypes respond differently to long-term continuous cropping in mollisols. Front. Microbiol. 12:729047. doi: 10.3389/fmicb.2021.729047
Zeeshan Ul Haq, M., Yu, J., Yao, G., Yang, H., Iqbal, H. A., Tahir, H., et al. (2023). A systematic review on the continuous cropping obstacles and control strategies in medicinal plants. Int. J. Mol. Sci. 24:12470. doi: 10.3390/ijms241512470
Zeng, J., Liu, J., Lu, C., Ou, X., Luo, K., Li, C., et al. (2020). Intercropping with turmeric or ginger reduce the continuous cropping obstacles that affect Pogostemon cablin (patchouli). Front. Microbiol. 11:579719. doi: 10.3389/fmicb.2020.579719
Zhang, J. F., He, L. P., Wu, Y. G., Ma, W. T., Chen, H., and Ye, Z. C. (2018). Comparative proteomic analysis of Pogostemon cablin leaves after continuous cropping. Protein. Expr Purif. 152, 13–22. doi: 10.1016/j.pep.2018.07.004
Zhang, S., Jiang, Q., Liu, X., Liu, L., and Ding, W. (2020). Plant growth promoting rhizobacteria alleviate aluminum toxicity and ginger bacterial wilt in acidic continuous cropping soil. Front. Microbiol. 11:569512. doi: 10.3389/fmicb.2020.569512
Zhao, H., Li, X., Zhang, Z., Zhao, Y., Chen, P., and Zhu, Y. (2018). Drivers and assemblies of soil eukaryotic microbes among different soil habitat types in a semi-arid mountain in China. PeerJ 6:e6042. doi: 10.7717/peerj.6042
Zhao, J., Xie, X., Jiang, Y., Li, J., Fu, Q., Qiu, Y., et al. (2024). Effects of simulated warming on soil microbial community diversity and composition across diverse ecosystems. Sci. Total Environ. 911:168793. doi: 10.1016/j.scitotenv.2023.168793
Zhao, X., Elcin, E., He, L., Vithanage, M., Zhang, X., Wang, J., et al. (2024). Using biochar for the treatment of continuous cropping obstacle of herbal remedies: a review. Appl. Soil. Ecol. 2024:105127. doi: 10.1016/j.apsoil.2023.105127
Zhou, Z., Wang, C., and Luo, Y. (2020). Meta-analysis of the impacts of global change factors on soil microbial diversity and functionality. Nat. Commun. 11:3072. doi: 10.1038/s41467-020-16881-7
Zhu, S., Wang, Y., Xu, X., Liu, T., Wu, D., Zheng, X., et al. (2018). Potential use of high-throughput sequencing of soil microbial communities for estimating the adverse effects of continuous cropping on ramie (Boehmeria nivea L. Gaud). PLoS ONE 13:e0197095. doi: 10.1371/journal.pone.0197095
Keywords: Pogostemon cablin, continuous cropping, metagenomic, rhizosphere soil, microbial community diversity
Citation: Gu G, Zeeshan Ul Haq M, Sun X, Zhou J, Liu Y, Yu J, Yang D, Yang H and Wu Y (2025) Continuous cropping of Patchouli alters soil physiochemical properties and rhizosphere microecology revealed by metagenomic sequencing. Front. Microbiol. 15:1482904. doi: 10.3389/fmicb.2024.1482904
Received: 19 August 2024; Accepted: 27 December 2024;
Published: 13 January 2025.
Edited by:
Yuguang Wang, Heilongjiang University, ChinaCopyright © 2025 Gu, Zeeshan Ul Haq, Sun, Zhou, Liu, Yu, Yang, Yang and Wu. This is an open-access article distributed under the terms of the Creative Commons Attribution License (CC BY). The use, distribution or reproduction in other forums is permitted, provided the original author(s) and the copyright owner(s) are credited and that the original publication in this journal is cited, in accordance with accepted academic practice. No use, distribution or reproduction is permitted which does not comply with these terms.
*Correspondence: Yougen Wu, OTkwOTM0QGhhaW5hbnUuZWR1LmNu
†These authors have contributed equally to this work
Disclaimer: All claims expressed in this article are solely those of the authors and do not necessarily represent those of their affiliated organizations, or those of the publisher, the editors and the reviewers. Any product that may be evaluated in this article or claim that may be made by its manufacturer is not guaranteed or endorsed by the publisher.
Research integrity at Frontiers
Learn more about the work of our research integrity team to safeguard the quality of each article we publish.