Frontiers Corrigendum Template for Authors
- 1Sichuan Zoige Alpine Wetland Ecosystem National Observation and Research Station, Southwest Minzu University, Chengdu, China
- 2College of Grassland Resources, Southwest Minzu University, Chengdu, China
- 3College of Animal Science and Veterinary Medicine, Southwest Minzu University, Chengdu, China
- 4School of Mathematics and Statistics, Qinghai Normal University, Xining, China
- 5College of Agro-grassland Science, Nanjing Agricultural University, Nanjing, China
Introduction: Weak aerobic stability is a notable challenge for whole-plant corn silage, particularly in hot and humid regions. Acetobacter is commonly regarded as an indicator of aerobic deterioration in silage, yet its precise role in fermentation and during aerobic exposure, as well as the factors that promote its growth, remain insufficiently understood.
Methods: In this study, whole-plant corn silage was prepared using a bagged method with controlled dry matter (DM) content at 20%, 25%, and 30%, and initial concentrations of A. pasteurianus at 40%, 50%, and 60%. The silage was stored for 60 days under varying temperatures (20°C, 30°C, and 40°C). Following the anaerobic storage phase, the silage was exposed to air at room temperature (20-25°C) for 7 days, both with and without A. pasteurianus inoculation.
Results: The results demonstrated that A. pasteurianus did not impact the nutritional value of the silage during anaerobic fermentation, maintaining a low pH (< 3.80). However, during aerobic exposure, the presence of A. pasteurianus significantly reduced the aerobic stability of the silage. The microbial community shifted from primarily Klebsiella species initially to Lactobacillus and Acetobacter species post-ensiling. During the aerobic exposure phase, A. pasteurianus and A. fabarum became the dominant species. Response Surface Methodology (RSM) analysis identified optimal conditions for the proliferation of A. pasteurianus during the aerobic phase, which occurred at 28°C, 25% DM, and 52% initial concentration at 3 ml/kg.
Discussion: These findings confirm that A. pasteurianus plays a critical role in reducing the aerobic stability of whole-plant corn silage. Additionally, the study identifies the optimal conditions that favor the proliferation of A. pasteurianus, offering valuable insights for the development of strategies to prevent and control this bacterium, thereby improving the aerobic stability of silage in hot and humid regions.
1 Introduction
The defining characteristics of hot and humid climatic zones include elevated temperatures, high humidity, substantial precipitation, minimal daily temperature variations, and negligible wind speeds. These conditions are primarily associated with tropical rainforest climates. Currently, an increasing number of dairy farms are undergoing a transition from grazing to confinement systems, including free-stall barns and compost barns. The elevated demand for beef, lamb, and dairy products has resulted in a considerable deficit in the forage required by the livestock industry, thereby necessitating the year-round provision of preserved feed for dairy farms (Miguel et al., 2023). Silage has historically constituted a primary preserved feedstuff, particularly given the growth of forage during the humid, rainy season. It is therefore crucial for farmers to produce high-quality, long-lasting silage on farms during periods of high grain prices to ensure the success of their operations (Daniel et al., 2019).
The conversion of forages with high temperatures, low dry matter (DM) content, and low soluble carbohydrates into high-quality silage was a challenging process due to their high biochemical oxygen demand in tropical and subtropical regions (Loures et al., 2005). Furthermore, the fermentation quality of this type of silage was often unstable, frequently exhibiting high acetic acid fermentation with a noticeable acetic acid smell (Guan et al., 2020). The ensiling of tropical grass may result in the production of large amounts of acetic acid instead of lactic acid (Nishino et al., 2012), despite the initial detection of lactic acid during the ensiling process. However, in subsequent stages, there was often a decline in lactic acid levels and an increase in acetic acid levels (Hou and Nishino, 2021). The fermentation of high acetic acid silage, which was similar to lactic acid fermentation dominated by homofermentative lactic acid bacteria (LAB), often results in a low pH, which can lead to misinterpretation of the quality of the silage fermentation (Kung et al., 2018). It has been documented that silage with a low DM content (less than 20%) can exhibit acetic acid levels as high as 3–6% DM, excessive acetic acid fermentation (greater than 3% DM) has been observed to results in significant DM loss, accelerate aerobic spoilage, and markedly reduce animal intake (Gerlach et al., 2020). It is therefore recommended that high acetic acid fermentation be given particular attention in the production of silage in tropical and subtropical regions.
The process of acetic acid fermentation was primarily driven by heterofermentative LAB, enterobacteria, and Acetobacter bacteria (Yan et al., 2022). In particular, during the opening stage of silage in the hot and humid regions, a significant presence of Acetobacter bacteria has been observed (Guan et al., 2018). In a study conducted by Carvalho-Estrada et al. (2020), it was reported that after 120 days of fermentation, the abundance of Acetobacter bacteria in two varieties of corn silage in São Paulo, Brazil, exceeded 80%. Thi Minh Tu et al. (2014) documented the presence of Acetobacter bacteria in small-scale TMR silages in a laboratory setting in Vietnam. Acetobacter bacteria were frequently regarded as the primary initiators of aerobic spoilage in silage (Spoelstra et al., 1988). However, their metabolic byproducts were also acetic acid, analogous to the extensively utilized heterofermentative lactic acid bacterium Lactobacillus buchneri. The role and mechanism of Acetobacter bacteria during the entire silage fermentation and aerobic exposure stages remained unclear.
To investigate the impact of environmental temperature and DM content on the microbial community, fermentation quality, and aerobic stability of silage inoculated with A. pasteurianus, whole-plant corn was employed in this study. The application of response surface methodology (RSM) facilitated the identification of the critical points of key factors affecting A. pasteurianus proliferation, which will provide a scientific basis for the future prevention and control of A. pasteurianus to improve aerobic stability in hot and humid areas.
2 Materials and methods
2.1 Experimental materials and experimental design
The experiment was conducted at the National Grass Variety Regional Test Base of the Grassland Technology Research and Promotion Center in Sichuan Province, China (N30°76′, E103°76′) in August 2023. The mean maximum temperature was 31°C, with a minimum temperature of 23°C. Please refer to Supplementary Table S1 for further details.
Response Surface Methodology (RSM) is a statistical method employed for the optimization of biological processes (Wang et al., 2016). This method can be employed for the development of models, the evaluation of factors, and the identification of optimal conditions for optimal responses. In our study, RSM was used to examine the influence of environmental factors on the quality of silage: (1) storage temperature (T); (2) DM content (M); (3) initial A. pasteurianus concentration (A). The response was the concentration of A. pasteurianus produced during the process. The experimental design comprised three levels of each variable: initial A. pasteurianus concentration (40, 50, 60%), DM content (30, 25, 20%), and storage temperature (20°C, 30°C, 40°C). RSM was employed to optimize and evaluate the principal interactions and secondary effects of the variables. The variables were arranged according to the Box–Behnken design, which required 17 experiments, including 5 repetitions at the central point (Table 1).
Using Design Expert software (Version 11), the obtained data were analyzed through Analysis of Variance (ANOVA) to evaluate the significance of the model. A second-order polynomial model was used to analyze the experimental data as follows:
The materials used in this experiment were whole corn plants (Zea mays L.), of the variety AoYu 005, with a single field plot area of 15 m2 (3 m × 5 m), planted in 3 plots. The whole corn plants were harvested when it reaches the 1/2 milk-line stage on August 3, 2023, with a cutting height of 15 ~ 30 cm above the ground. The processed grain achieved a crushing degree of 80%. The A. pasteurianus (CGMCC No. 1.1810) strain was obtained from the China General Microbiological Culture Collection Center (CGMCC).
Whole-plant corn raw materials (RAW) from the 3 plots were harvested separately and chopped into 1–2 cm pieces using a forage cutter (Lingong Machinery, Shandong, China). The maize was ensiled following the same method for each plot, with each batch of silage material weighing 1 kg. The DM content of the silage was adjusted to 30% (M30), 25% (M25), and 20% (M20) as described by Zhu (2021). A. pasteurianus solutions at concentrations of 40% (A40), 50% (A50), and 60% (A60) were sprayed evenly onto the silage material at 3 mL/kg (Xu et al., 2019) (initial concentration of 106 cfu·ml−1, by mixing 40 mL of a 100% concentration A. pasteurianus solution with 60 mL of sterile distilled water to obtain 100 mL of a 40% concentration A. pasteurianus suspension. Similarly, prepare A. pasteurianus suspension with concentrations of 50 and 60%.). After thorough mixing, each batch of silage material was packed into polyethylene plastic bags (30 cm × 40 cm), vacuum-sealed using a vacuum sealer (DZQ-390, Anshengke, Fujian, China), and stored in temperature-controlled incubators at 20°C (T20), 30°C (T30), and 40°C (T40) for 60 days. Each treatment had 3 replicates. Subsequently, the 60-day silage was subjected to an aerobic stability test, including treatments with A. pasteurianus (ASA) and controls without A. pasteurianus (ASN), using the same methods as before ensiling. Each sample weighed 300 g, with 3 replicates for each treatment. For each experimental unit, 800 g of silage was packed in a 1 L container compacted, placed in an incubation room at 20°C, and covered with 2 layers of cheesecloth. A temperature probe was placed in the center of the silage and connected to a data logger (BCL3016P multipoint temperature recorder, A-BF). An additional temperature probe was placed in an empty bin to record the ambient temperature as a control. Spoiled samples were collected immediately and unspoiled samples were collected at the end of the 7-day trial period.
2.2 Fermentation quality
Samples were thoroughly mixed, and 20 g of fresh material (FM) was accurately weighed and placed in 15 × 20 cm polyethylene plastic bags. 180 mL of distilled water was added, and the samples were homogenized and sealed, then extracted overnight at 4°C (Jiang et al., 2023). The plant residue was filtered through four layers of cheesecloth, and the pH of the filtrate was measured immediately. The remaining filtrate was stored at −20°C for the determination of organic acids and ammonia nitrogen (NH3-N) content. All samples were stored at −80°C for further DNA extraction and sequencing analysis.
The pH was measured using a glass electrode pH meter (PHSJ-5; LEICI, Shanghai, China); the NH3-N content was determined using the phenol-hypochlorite colorimetric method (Broderick and Kang, 1980); the filtrate was filtered through a 0.22 μm filter and analyzed for lactic acid (LA), acetic acid (AA), propionic acid (PA), and butyric acid (BA) concentration using an ultra-high performance liquid chromatograph (Thermo Fisher UltiMate3000, United States). The chromatographic conditions were an RSpak KC-811 column, 0.1% H3PO4 as the mobile phase, a flow rate of 0.5 mL·min−1, a column temperature of 55°C, and a detection wavelength of 210 nm (Zhao et al., 2016).
2.3 Nutritional quality
Fresh material and silage samples were inactivated in a ventilated oven at 105°C for 15 min, then dried in a ventilated oven at 65°C for 48 h to a constant weight to partially dry the material prior to grinding (Zhao et al., 2021). Samples were pulverized into fine powder using a grinder (CT293 CyclotecTM, FOSS Analytical A/S, Hillerød, Denmark), passed through a 1 mm sieve for subsequent experiments.
The DM and ash content of the fresh material and silage were analyzed following the standard methods set forth by the Association of Official Analytical Chemists (AOAC). The crude protein (CP) content was determined utilizing a KjeltecTM8400 automatic Kjeldahl nitrogen analyzer (AOAC, 1990). The neutral detergent fiber (NDF, without heat-stable amylase and including residual ash) and acid detergent fiber (ADF, including residual ash) were measured using a fiber analyzer (Fibretherm FT12, C. Gerhardt, Germany) as described by Van Soest et al. (1991).
2.4 Aerobic stability evaluation
The aerobic stability of the silage was determined by measuring the temperature at the center of the bagged silage following the methodology proposed by Ranjit and Kung (2000). The temperature of the core area of the silage (at a depth of 10 cm) was measured by real-time temperature logging (MT-X, Shenhua Science and Technology Co., Ltd., Shenzhen, China) every 5 min for 7 days, with 3 replicates for each treatment.
2.5 DNA extraction
Guan et al. (2018) described the DNA extraction method. A total of 20 g of frozen sample was added to 180 mL of sterile distilled water and incubated at 4°C with agitation for 15 min, followed by centrifugation at 8000 rpm for 10 min at 4°C. The supernatant was removed, and the pellet was retained for DNA extraction using the bacterial DNA extraction kit (DP302-02, Tiangen, Beijing, China). DNA purification was performed using the purification kit (DP214-02, Tiangen, Beijing, China). The purity and concentration of the DNA were assessed with a NanoDrop 2000 before storing the DNA samples at −80°C. Qualified DNA samples were subsequently stored at −20°C.
2.6 Microbial analysis based on sequencing
The full-length 16S rRNA genes of the D60, ASA, and ASN groups were amplified by PCR using the primers (5′-AGRGTTTGATYNTGGCTCAG-3′) and (5′-TASGGHTACCTTGTTASGACTT-3′). The primers were supplied by Beijing Biomarker Technologies Co., Ltd. The amplified products were subjected to sequencing on the PacBio Sequel platform (Pacific Biosciences, Menlo Park, CA, United States). The raw subreads were corrected to obtain circular consensus sequencing (CCS) sequences using the SMRT (version 8.0) software. Lima software (version 1.7.0) was employed to identify the CCS sequences from different samples through barcode sequences and to remove chimeras, thereby obtaining high-quality CCS sequences. Operational taxonomic unit (OTU) clustering was conducted at a similarity level of 97% using USEARCH (version 10.0). A threshold of 0.005% of all sequences was used to filter OTUs by default. The DADA2 method in QIIME2 (version 2020.6) was used to denoise the quality-controlled data to obtain the optimal sequences.
2.7 Absolute quantification of Acetobacter pasteurianus
The method of Li et al. (2020) was employed to quantify Acetobacter bacteria DNA using real-time fluorescence quantitative polymerase chain reaction (RT-FQPCR). Universal primers for A. pasteurianus (5′-AAGGGGGCTAGCGTTGCTCG-3′ and 5′-ACCGCCTACACGCCCTTTACG-3′) were used. A standard curve of the target A. pasteurianus DNA concentration versus the cycle threshold (Ct) value was constructed through serial gradient dilutions, and a melting curve was generated. Subsequently, the DNA extraction products from the D60, ASA, and ASN groups underwent amplification by PCR, and the Ct values were compared with the standard curve to quantify A. pasteurianus. The RT-PCR reaction procedure was as follows: stage 1—initial denaturation at 95°C for 5 min; stage 2—95°C for 10 s, 60°C for 30 s, for a total of 40 cycles; stage 3—the instrument’s default melting curve.
2.8 Statistical analysis
SPSS software was employed for statistical analysis with one-way ANOVA and Duncan’s multiple-range test. The GraphPad Prism (version 10.0) software was employed for plotting. The absolute quantitative results for A. pasteurianus were subjected to analysis using Design-expert (version 11.0). All values are the mean of 3 replicates.
3 Results
3.1 Chemical compositions of forage and silage
As illustrated in Table 2, following the processing of fresh materials, the DM was 33.41% of the original weight, and the contents of CP, NDF, ADF, starch, and ash were 8.92% DM, 51.63% DM, 21.73% DM, 29.85% DM, and 4.01% DM, respectively. Following a 60-day ensiling period, the CP content ranged from 8.17% DM to 8.84% DM, which exhibited a significant reduction in comparison to the raw material (p < 0.05). The NDF content showed no significant differences among the groups, and it was significantly diminished in comparison to the raw material (p < 0.05). The ADF and starch contents showed no significant differences and no significant divergence from the raw material. The ash content varied between 4 and 5.5% DM.
3.2 Fermentation quality on silages inoculated with Acetobacter pasteurianus
The final fermentation products of the silage samples and aerobic exposure samples were shown in Table 3. The pH of the D60 group samples was below 3.80, with the lowest reading being 3.46 in group 16. During the transition from the fermentation phase to the aerobic exposure phase, a tendency for the pH and NH3-N content to increase. Group 6 exhibited the lowest NH3-N content (20.38 mg dL−1), with LA content ranging from 3.04% DM to 4.84% DM, AA content varying between 0.73% DM and 1.84% DM, and no PA and BA detected. In the ASA group samples, the pH exhibited a range of 4.12–6.21, while the NH3-N content ranged from 286.83 to 316.31 mg dL−1. Notably, LA was undetected in groups 2, 4, 6, 8, and 9, while in other groups, it varied between 0.43% DM and 3.14% DM. Similarly, AA content ranged from 0.25% DM to 1.39% DM across all groups. Additionally, PA was identified in groups 2, 4, 6, 7, 9, 10, 13, and 16, with concentrations ranging from 0.22% DM to 0.78% DM. No BA was detected in any of the samples. In the ASN group samples, the pH exhibited a range of 3.84 to 4.91, while the NH3-N content spanned from 217.98 to 254.07 mg dL−1. The LA content varied between 0.68% DM and 6.52% DM, and the AA content ranged from 0.54% DM to 2.07% DM. Notably, no PA or BA was detected in these samples.
3.3 Aerobic stability of silage
Figure 1 illustrates the duration of aerobic stability. With the prolongation of exposure time, the temperature of each treatment group exhibited a gradual increase. The ASN group demonstrated the greatest overall stability, with the center temperature of the majority of silage samples remaining below the ambient temperature by 2°C after 7 days. Group 2 and Group 8 exhibited signs of spoilage at 120 and 80 h of aerobic exposure, respectively. The ASA group exhibited a tendency towards rapid spoilage, with the majority of samples showing signs of deterioration within a period of 48–120 h. It is noteworthy that Group 7 in the ASA group exhibited the longest aerobic stability duration, exceeding 168 h.
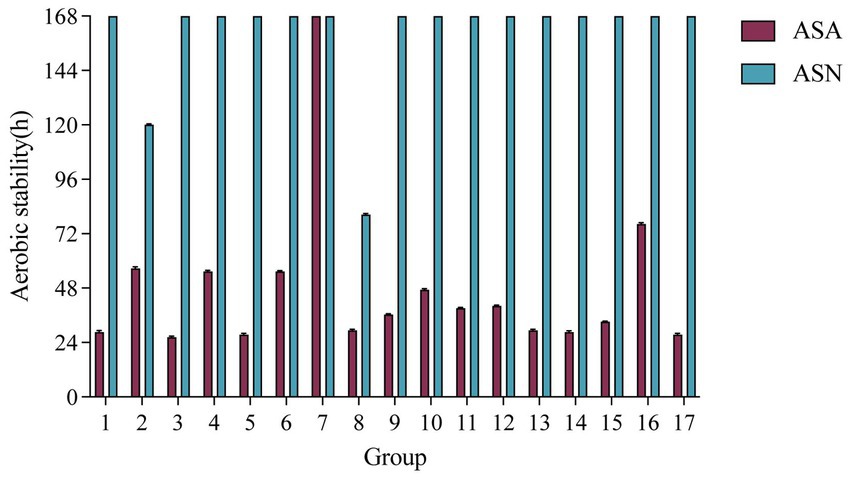
Figure 1. Effect of A. pasteurianus on the aerobic stability of whole-plant corn silage after 60 days of fermentation. ASA, silages inoculated with A. pasteurianus during aerobic exposure; ASN, silages without A. pasteurianus during aerobic exposure.
3.4 Multifactorial interaction of absolute quantification of Acetobacter pasteurianus under different environmental conditions
The three independent factors selected for RSM optimization were temperature, DM content, and initial concentration of A. pasteurianus. The Box–Behnken design (BBD) yielded a total of 17 sets of experiments, the results of which are presented in Table 4. The concentration of A. pasteurianus obtained from the ASN phase in these experiments ranged from 0.67 to 5.59 log cfu·ml−1.
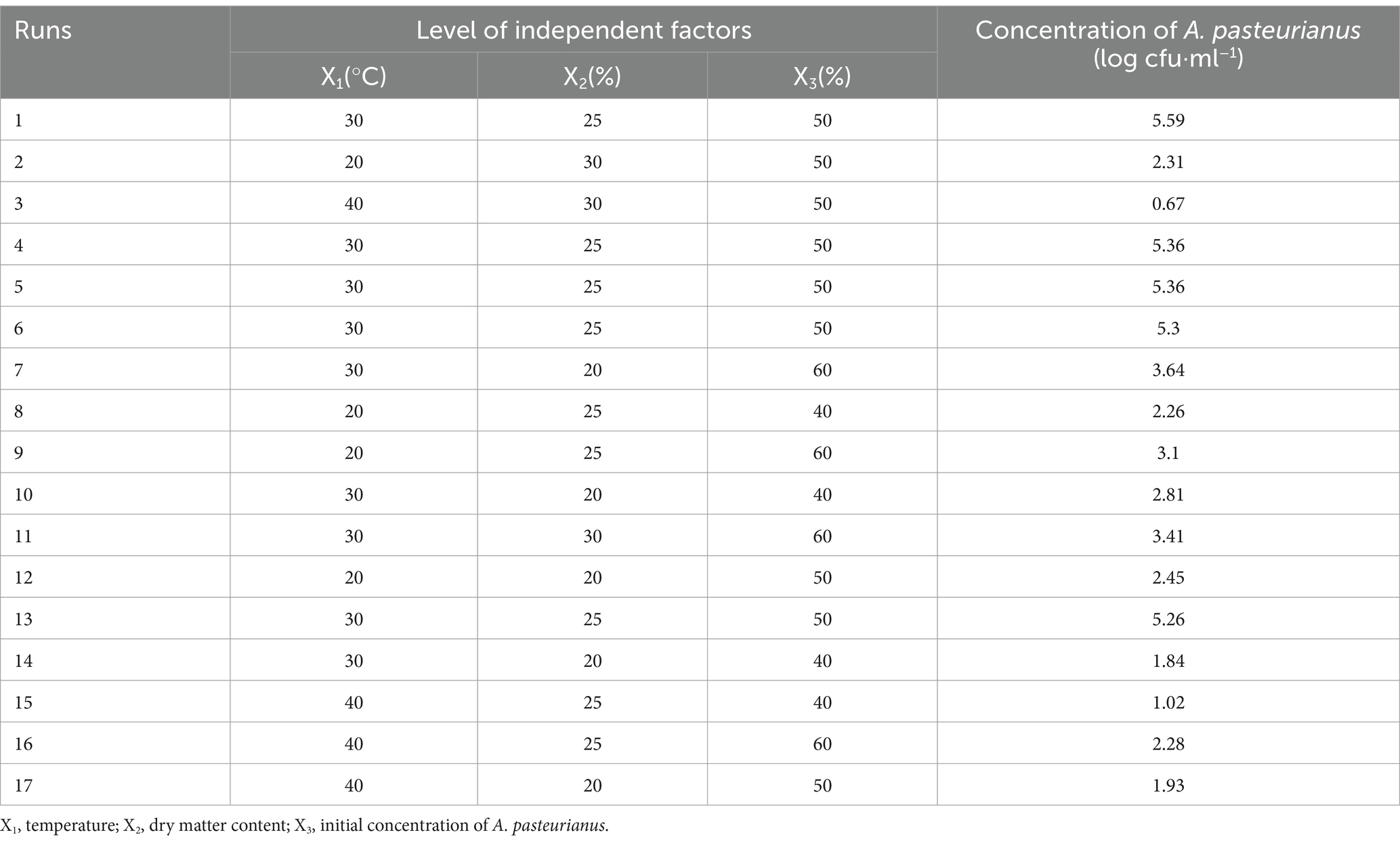
Table 4. The Box–Behnken design with independent factors and observed values for the absolute quantitative results of A. pasteurianus.
The statistical significance of the second-order response surface model was evaluated through one-way ANOVA, as illustrated in Table 5. The model exhibited a high F-value (391.28) and a low p-value (<0.001). In contrast, the F-value of the model’s lack of fit was 0.7884 (p > 0.05), which was not statistically significant. Furthermore, the model exhibited a low coefficient of variation (C.V.% = 3.39), R2 = 0.9980, and an adjusted R2 = 0.9955, indicating that this model can explain 99.80% of the variability in the three factors, and 99.55% of A. pasteurianus content under environmental conditions can be explained by this model.
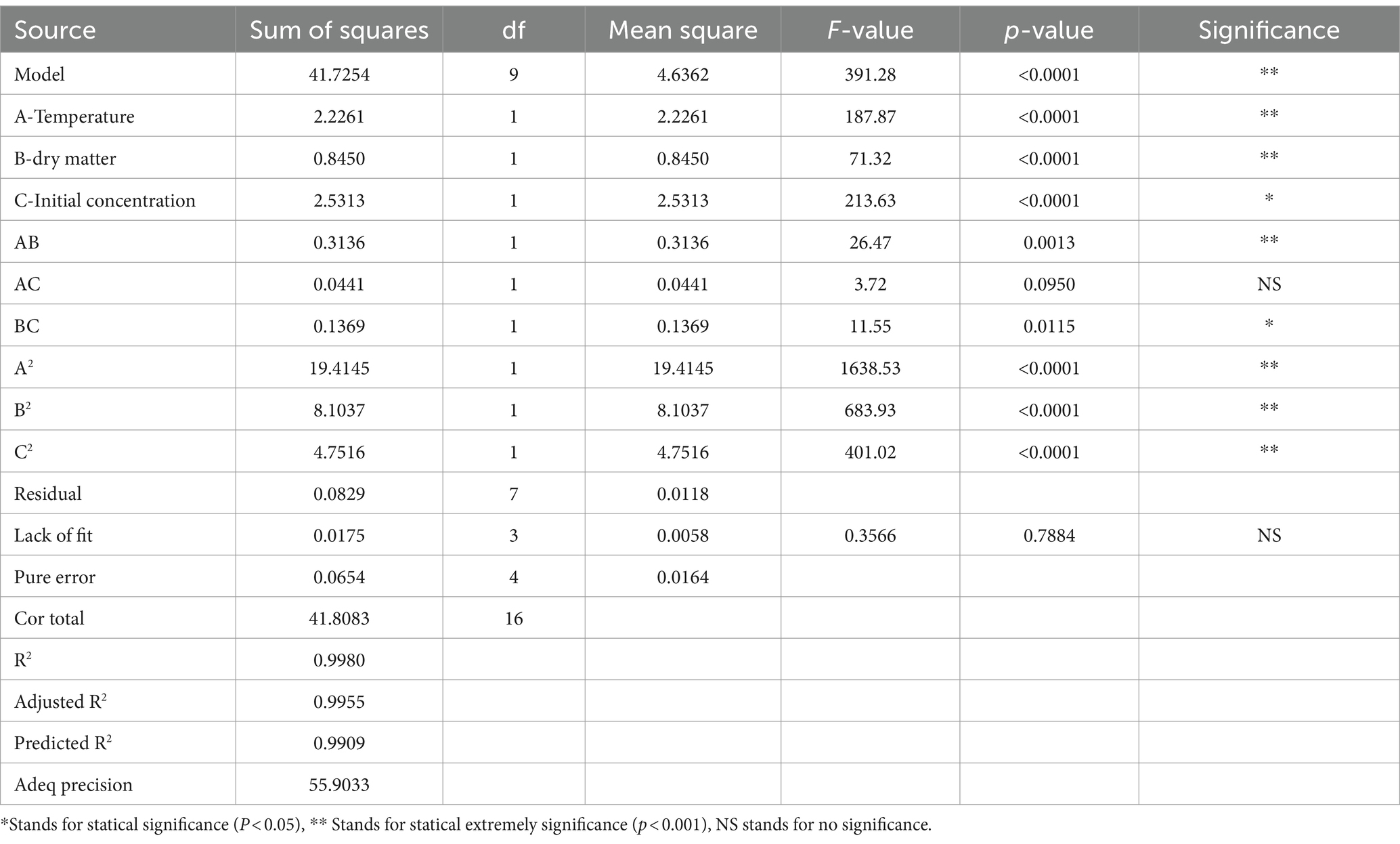
Table 5. Analysis of variance for absolute quantitative response surface modelling of A. pasteurianus.
The impact of each factor on the total concentration of A. pasteurianus is inversely proportional to the square of the factor’s F-value. Therefore, the larger the F value, the greater the impact of the single factor on the total concentration of A. pasteurianus. The order of influence of the individual factors was as follows: C (initial A. pasteurianus concentration) > A (temperature) > B (DM content). The linear, interaction, and quadratic parameters were all found to be statistically significant. A multiple regression analysis was conducted on the experimental data to derive a second-order polynomial equation that describes the relationship between the variables and the response. The fitting equation parameters are as follows:
Three-dimensional (3D) and two-dimensional (2D) response surface and contour plots were employed to predict the interactive effects of temperature, pH, and initial A. pasteurianus concentration on the growth of A. pasteurianus (Figure 2). As illustrated in the figure, the initial concentration of A. pasteurianus in the silage exhibited a more positive impact on the total concentration of A. pasteurianus in the ASN group. The results of the ANOVA and the response surface plots indicated that the interactions between storage temperature and DM content (AB) and DM content and initial A. pasteurianus concentration (AC) were significant.
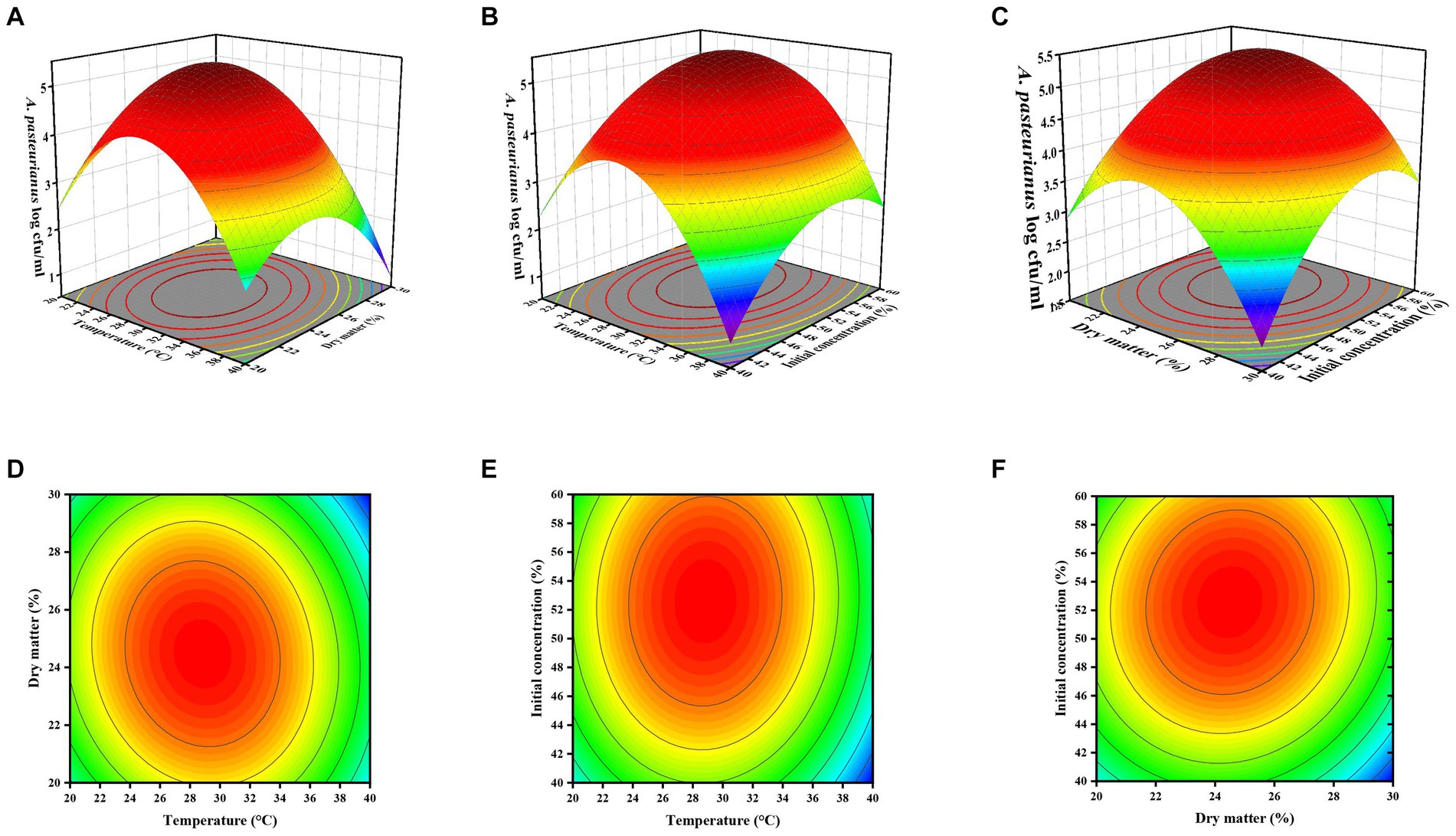
Figure 2. Three-dimensional response surface plots (A,C,E) and two-dimensional contour plots (B,D,F) of ASN A. pasteurianus content (A–F), respectively, represent the interactions between temperature, dry matter content, and initial A. pasteurianus concentration.
Furthermore, under the predicted optimal conditions—temperature of 28.889°C, DM content of 24.557%, and initial A. pasteurianus concentration of 52.517%—the ASN stage had the highest A. pasteurianus content. At a temperature of 39.853°C, DM content of 29.909%, and initial A. pasteurianus concentration of 56.690%, the ASN stage exhibited the lowest A. pasteurianus content (Table 6). For the purposes of practical processing, it is necessary to verify the aforementioned conditions to be whole numbers. The data presented in figures a-f illustrate that as temperature and DM content, as well as temperature and initial concentration of A. pasteurianus, and DM content and initial concentration of A. pasteurianus increase, the A. pasteurianus content in the ASN group initially rises before declining. The data presented above demonstrate that environmental temperature and the initial concentration of A. pasteurianus are key factors affecting the increase in A. pasteurianus concentration during aerobic exposure, which is related to the DM content of the raw materials.
3.5 Dynamics of silage microbial diversity at different stages
Based on SMRT sequencing of the full-length 16S rRNA genes of silage bacteria, an average of 12,814 CCS sequences were obtained from each sample. Alpha diversity, reflecting bacterial richness and diversity, was characterized using the ACE, Chao1, Shannon, and Simpson indices (Figure 3). In this study, the Chao1 index of all samples significantly decreased after silage (p < 0.05), while the ACE index decreased but not significantly. The Chao1, ACE, Simpson, and Shannon indices of the ASA and ASN groups were significantly lower than the D60 group (p < 0.05). The bacterial richness and community diversity in the ASN group were found to be significantly higher than in the ASA group (p < 0.05).
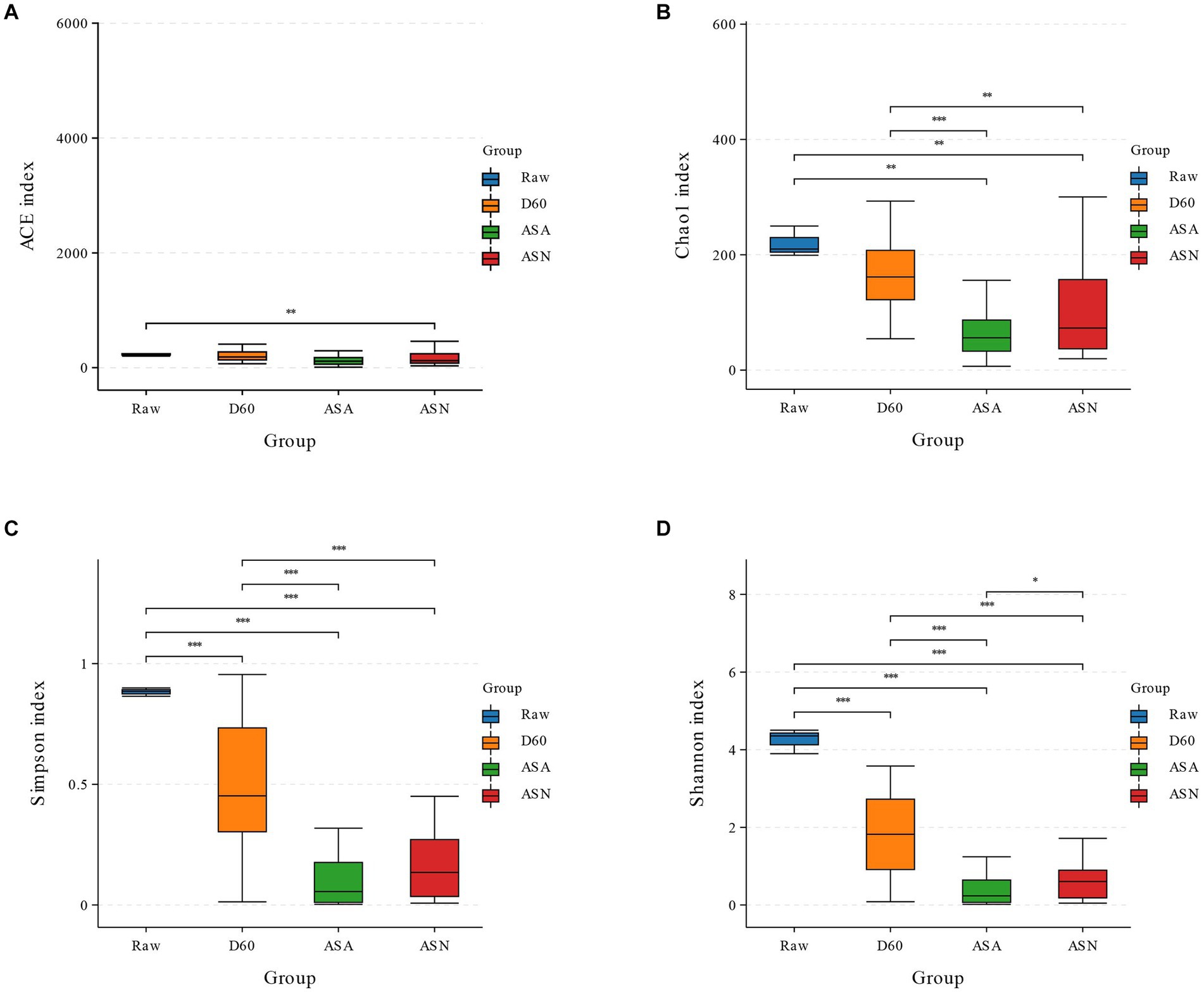
Figure 3. Bacterial alpha diversity of silage (A, ACE index; B, Chao1 index; C, Shannon index; D, Simpson index). RAW, pre-ensiled silage; D60, whole-plant corn ensiled for 60 days; ASA, silages inoculated with A. pasteurianus during aerobic exposure; ASN, silages without A. pasteurianus during aerobic exposure.
Principal coordinate analysis (PCoA) of beta diversity highlighted differences in bacterial communities across various treatments during the raw material, fermentation, and aerobic exposure stages (Figure 4). The data presented in the figure reveal a significant degree of dispersion between the D60 group and the ASA group, as well as between the D60 group and the ASN group.
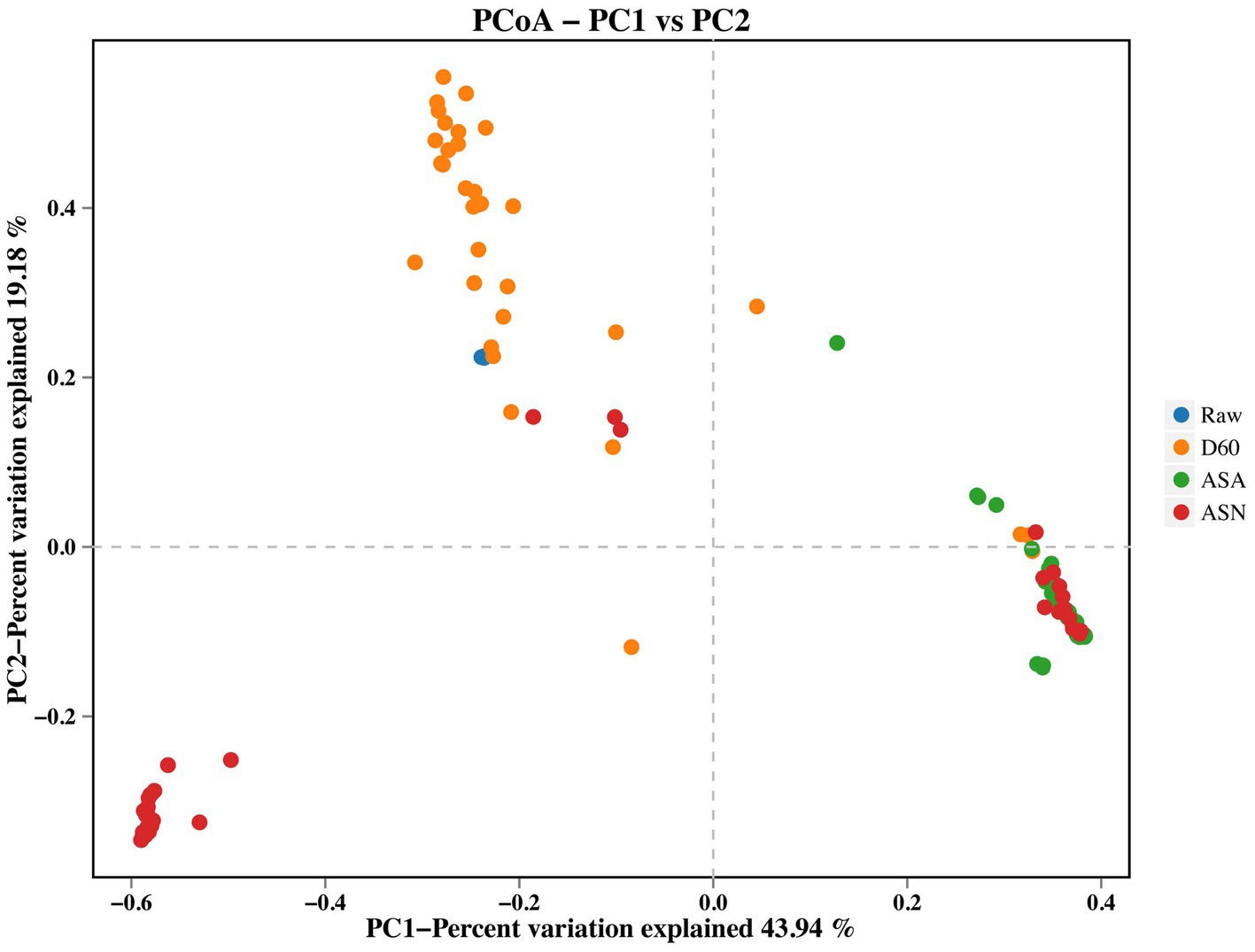
Figure 4. Bacterial beta diversity of silage. RAW, pre-ensiled silage; D60, whole-plant corn ensiled for 60 days; ASA, silages inoculated with A. pasteurianus during aerobic exposure; ASN, silages without A. pasteurianus during aerobic exposure.
Figure 5A illustrated that at the species level, the epiphytic microbial flora of fresh corn mainly consisted of Klebsiella pneumoniae (26.58%), Leuconostoc citreum (13.93%), Serratia marcescens (7.61%), Lactiplantibacillus plantarum (1.06%), and A. pasteurianus (0.35%). After 60 days of ensiling, the following microbial species were identified: A. pasteurianus (30.31%), Limosilactobacillus panis (22.25%), Lactiplantibacillus plantarum (9.05%), Levilactobacillus brevis (6.94%), Clostridium guangxiense (6.75%), and A. fabarum (1.52%). The D60 group exhibited an increased relative abundance of A. pasteurianus, Limosilactobacillus panis, L. plantarum, Levilactobacillus brevis, Clostridium guangxiense, and A. fabarum, while the relative abundances of Serratia marcescens and Klebsiella pneumoniae were reduced. During the aerobic exposure stage, irrespective of the treatment, the A. pasteurianus genus was the most prevalent. In the ASN group, the genus A. fabarum exhibited the highest relative abundance (58.51%), followed by A. pasteurianus (29.94%) and L. plantarum (1.22%). In the ASA group, A. pasteurianus (92.61%) had the highest relative abundance, accompanied by a minor presence of L. plantarum (3.15%). Figure 5B showed that the abundance of A. pasteurianus in the ASA group was significantly higher than in the RAW, D60, and ASN groups (p < 0.05). Additionally, the abundance of A. fabarum in the ASN group is significantly higher compared to the RAW, D60, and ASA groups (p < 0.05).
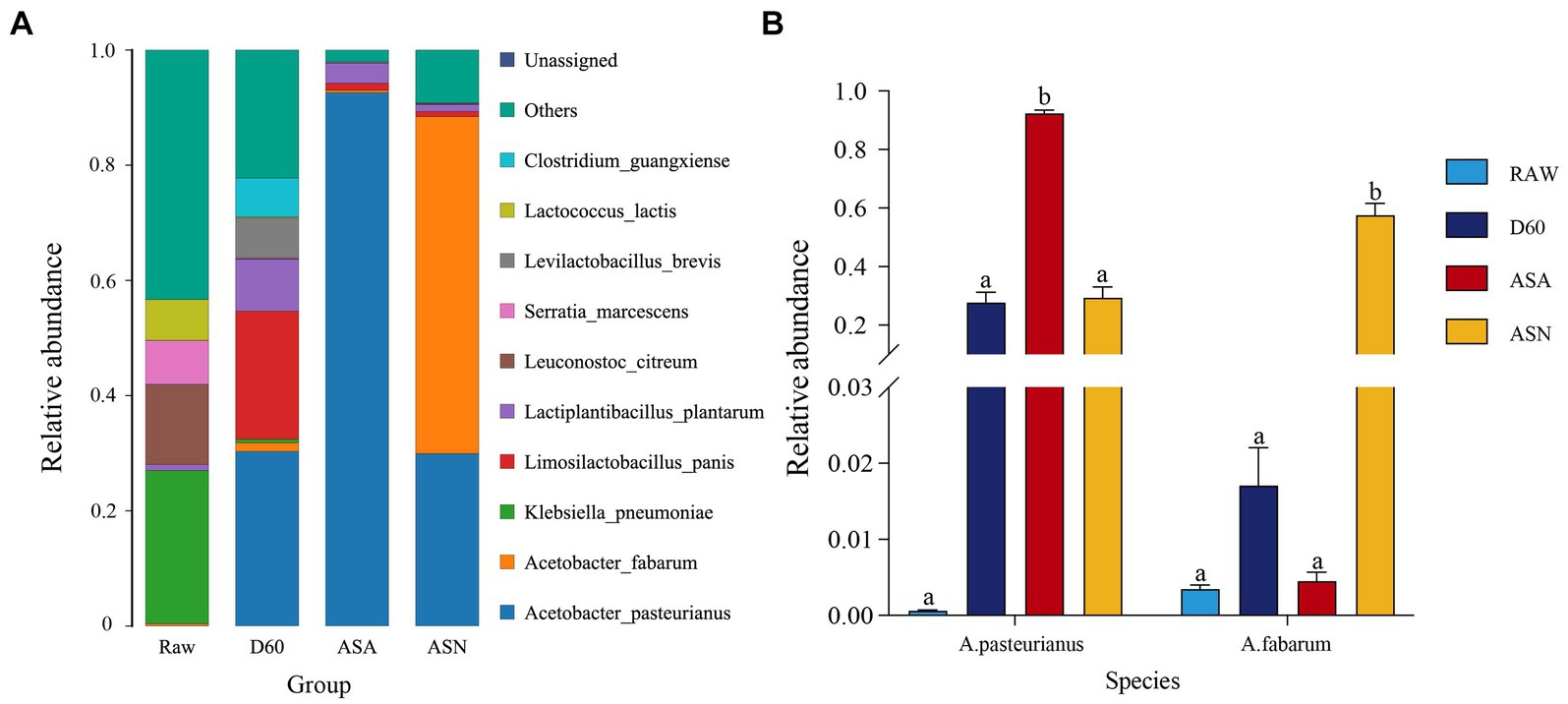
Figure 5. Bacterial community structure at the species level (A) and relative abundance of A. pasteurianus and A. fabarum at the species level (B) in pre-ensiled, ensiled, and aerobically exposed silage. RAW, pre-ensiled silage; D60, whole-plant corn ensiled for 60 days; ASA, silages inoculated with A. pasteurianus during aerobic exposure; ASN, silages without A. pasteurianus during aerobic exposure.
Linear discriminant analysis effect size (LEfSe, LDA = 4) was used to identify differences in bacterial community structure among the groups (Figure 6). This analysis calculates the relative abundance of bacterial genera in RAW, D60, ASA, and ASN groups. LEfSe identifies the taxa that are most likely to explain the differences in bacterial community structure among the groups. The results indicated that the genera Klebsiella, Leuconostoc, Acinetobacter, Serratia, Lactococcus, Enterobacter, Pantoea, Chryseobacterium, and Pluralibacter are enriched in the RAW group. The genera Limosilactobacillus, Lactiplantibacillus, Lactobacillus, Clostridium sensu stricto 11, Levilactobacillus, and Aeromonas were enriched in the D60 group. The ASA group was characterized by an enrichment of A. pasteurianus, while the ASN group was characterized by an enrichment of A. fabarum.
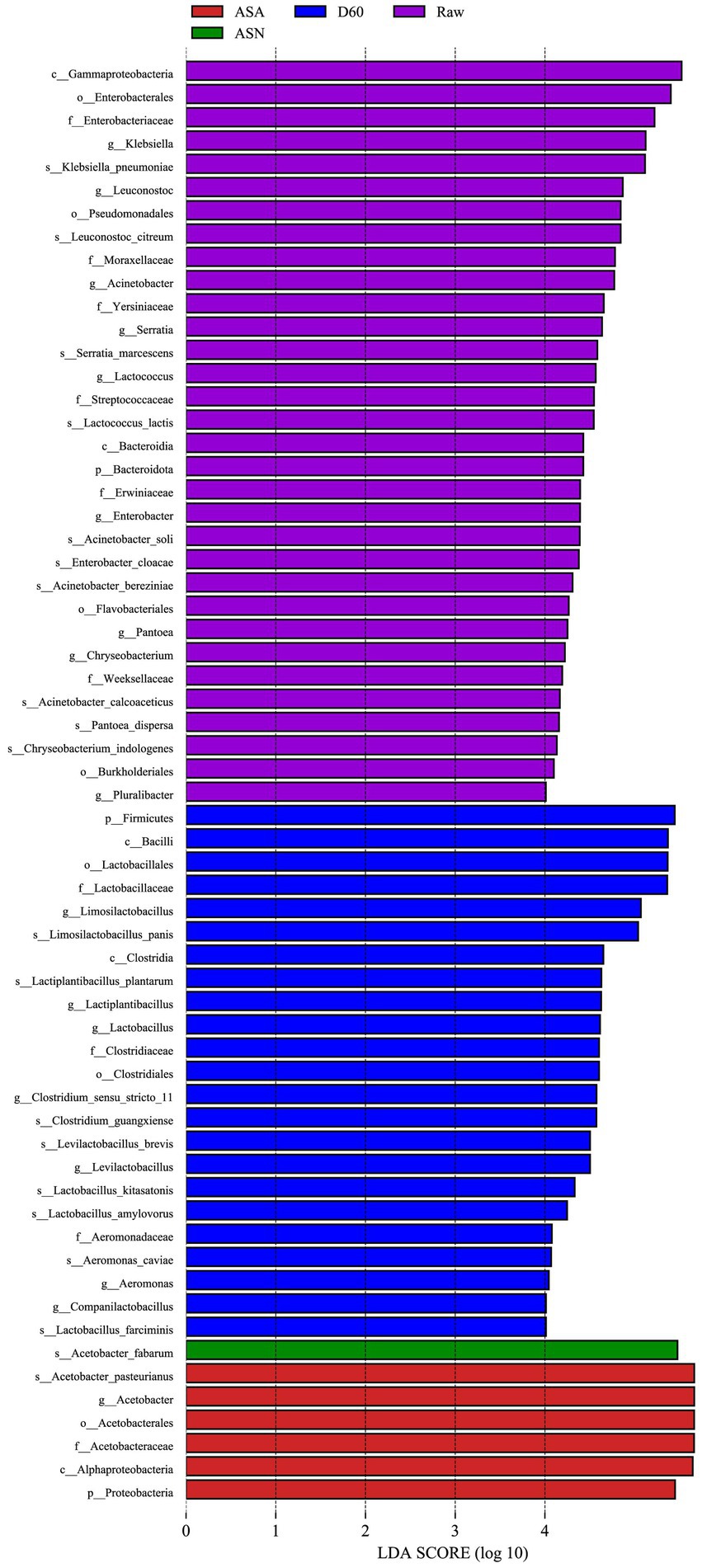
Figure 6. LEfSe analysis of pre-ensiled forage, ensiled forage, and aerobic-exposed ensiled forage. RAW, pre-ensiled silage; D60, whole-plant corn ensiled for 60 days; ASA, silages inoculated with A. pasteurianus during aerobic exposure; ASN, silages without A. pasteurianus during aerobic exposure.
4 Discussion
4.1 The nutritional and fermentation quality of whole-plant corn silage
To achieve suitable nutritional digestibility for animals, the optimal DM content of whole corn silage is 33–35%, corresponding to the 1/3–2/3 milk line stage of harvest (Lin et al., 1992; Liu et al., 2014). In this study, the DM content of the corn material prior to ensiling satisfied the aforementioned criteria. Following a 60-day ensiling period, no significant differences were observed in the DM, NDF, and ADF content between different treatments (p < 0.05), this suggests that under well-sealed conditions, different environmental conditions and the inoculation of A. pasteurianus did not result in any alterations to the fiber quality of the silage. In the D60 group, the final pH of all treatments was below 3.80, indicating that the corn silage was well-preserved (Cui et al., 2020). This finding is consistent with the observation made by McDonald et al. (1991) that the final pH of corn silage is typically between 3.7 and 4.0, this is likely due to the lower buffering capacity of corn silage, which is in the range of 200–250 mE·kg−1 DM. This pH level is recommended for the inhibition of bacterial growth, particularly that of harmful bacteria. The microbial counts indicated that the number of LAB was consistent with the epiphytic microbiota of whole corn silage. The addition of Acetobacter bacteria under anaerobic conditions had a negligible effect on the fermentation quality of the silage, as the organic acids produced by the epiphytic LAB primarily reduce the pH (Jatkauskas et al., 2018). The NH3-N content of the feed after 60 days of fermentation was significantly lower than that of the feed exposed to aerobic conditions, this may be attributed to the low acidity (pH), which inhibits protein degradation (Basso et al., 2014). It was observed that no yeast growth was evident when the silage was inoculated with acetic bacteria, which may be a principal factor contributing to aerobic spoilage (Spoelstra et al., 1988). Additionally, the study did not find a significant increase in AA content during the fermentation phase, possibly because the conversion of ethanol to acetic acid is often unobservable under practical or laboratory conditions (Honig and Woolford, 1980). The LA and AA content in this study is similar to other corn silages (Jiang et al., 2020).
4.2 The aerobic stability of whole-plant corn silage
The utilization of corn silage subsequent to the opening of the bag represented the most critical aspect of the production process. The aerobic and acid-tolerant microorganisms that were activated by exposure to air oxidize different substrates, thereby promoting their growth and reproduction. This process, known as aerobic spoilage, reduced the palatability and feed value of the silage (Weiss et al., 2016). It is commonly accepted that silage exposed to air will undergo an increase in temperature and pH. The occurrence of spoilage was typically defined as a temperature increase in the center of the silage exceeding the ambient temperature by 2°C. The term “aerobic stability” was used to describe the ability of silage to resist spoilage during the transition from anaerobic to aerobic conditions (Drouin et al., 2021). A lack of aerobic stability in silage results in the oxidation and degradation of nutrients, which in turn lead to a reduction in feed intake and digestibility by animals. This, in turn, affected animal growth, reproduction, and milk quality (Oliveira et al., 2017). It is therefore imperative to ensure that corn silage exhibited optimal aerobic stability to guarantee that livestock receive nutrients with minimal loss, in conjunction with an absence of toxins and spores (Kung et al., 2003).
The capacity of silage to withstand deterioration was contingent upon the prevailing environmental conditions and the initial concentration of A. pasteurianus present. The application of A. pasteurianus exhibited a response to the treatments that was notably temperature-dependent. In addition to exogenously added Acetobacter bacteria, there was also the growth of endogenous Acetobacter bacteria. Dolci et al. (2011) posited that the proliferation of Acetobacter bacteria may result from the presence of excessive yeast or damage caused by propionic acid accumulation. This genus was capable of converting ethanol to acetic acid in the presence of oxygen and was also able to oxidize lactate and acetate to carbon dioxide and water. Consequently, it plays a pivotal role in the triggering of aerobic spoilage during the opening stage (Pahlow and Muck, 2009). Except for Group 7, the aerobic stability of ASN was significantly higher than that of ASA. The addition of aerobic bacteria accelerated the oxidation of acetate, disrupting the strong acidic environment at the end of silage fermentation. This sped up the growth of yeast and mold and hindered the lipophilic action of undissociated acetic acid on saprophytic bacteria, thereby accelerating the aerobic deterioration of silage upon contact with air and shortening the aerobic storage time (Ávila and Carvalho, 2020). The longest aerobic stability duration was observed under the conditions of T20M70A50 in Group 7, indicating that low temperature (20°C), high DM content (30%), and inoculation with a 50% concentration of A. pasteurianus bacteria effectively prevent the temperature rise during aerobic storage of corn silage. This result was at odds with the findings of Wilkinson and Davies (2013), which indicated that silage with a high DM content was more susceptible to aerobic spoilage than that with a low DM content. This discrepancy may be attributed to the fact that the preceding study did not take environmental temperature into account. In general, whole-corn silage was more susceptible to aerobic spoilage in hot and humid climates. In a study conducted by Santos et al. (2013), the optimal pH range for silage was identified as 3.80–4.20. In this experiment, Groups 2, 4, 6, 7, 9, 10, 11, 12, 15, and 16 were found to meet the requisite criteria for the production of good silage. In the current experiment, the aerobic stability time of Group 8 in the ASN group was found to be significantly lower than that of Group 2. This discrepancy may be attributed to the higher pH and significantly lower LA and AA content observed in Group 8 (p < 0.05). It has been demonstrated that the AA content of silage not only facilitates a reduction in pH but also effectively inhibits the growth of yeast and mold, thereby enhancing the aerobic stability of the silage (Kleinschmit and Kung, 2006).
The application of response surface methodology (RSM) enabled the identification of the factors that contribute to the proliferation of A. pasteurianus. The elevated high F-value and significantly low p-value verified the efficacy of the proposed model in anticipating and responding to temperature, DM content, and initial concentration of A. pasteurianus (Oroian et al., 2020). Furthermore, the insignificant lack of fit demonstrates that the model was an accurate representation and can predict the response values with precision (Wu et al., 2023). The low coefficient of variation indicates that the RSM regression model has good predictive accuracy, thereby ensuring that the model was comprehensive, accurate, and reliable.
4.3 The microbial community dynamics of whole-plant corn silage
The results demonstrated that the sequence depth in this study is sufficient to yield reliable conclusions. It is widely accepted that the gradual replacement of the complex microbial community of the raw material by LAB following anaerobic fermentation is a key indicator of successful silage fermentation. Consequently, the microbial richness undergoes a marked decline following the completion of the fermentation process. A reduction in bacterial community diversity was observed in the silage material following the fermentation process, in comparison to the original raw material. One potential explanation was that despite the attachment of a limited quantity of L. plantarum (1.06%), the production of sufficient lactic acid was unable to reduce the pH and impede the proliferation of other deleterious microorganisms. The ASN group exhibited greater bacterial richness and diversity than the ASA group, potentially due to the proliferation of acid-sensitive spoilage microorganisms during aerobic exposure of the silage feed (Wilkinson and Davies, 2013). Gharechahi et al. (2017) observed that an increase in the abundance of dominant bacteria resulted in a reduction in microbial community diversity. Following aerobic exposure, the relative abundance of Acetobacter was observed to dominate all treatment groups, which in turn led to a reduction in bacterial diversity and richness.
A substantial body of research has identified a range of microbial communities and successions in forages subjected to ensiling, both prior to and following the process. It can be concluded that the composition of the microbial community plays a pivotal role in the fermentation of ensiled forage. To gain a comprehensive understanding of the complex process of silage, it is essential to have an in-depth knowledge of the microbial community composition. Leuconostoc and Klebsiella were common bacteria that were naturally attached to corn in hot and humid regions (Cai, 1999). The results of studies on silage microorganisms indicated that Proteobacteria initially dominate in RAW, but were subsequently replaced by Firmicutes following the ensilage process. This finding was consistent with the results of the present study (Yang et al., 2019). The relative abundances of Klebsiella pneumoniae and Serratia marcescens were found to be elevated in RAW, both belonging to the family Enterobacteriaceae. Additionally, trace quantities of Clostridium guangxiense, a member of the Clostridiaceae family, were identified in the D60 group. The presence of Enterobacteriaceae and Clostridiaceae was undesirable as these bacteria are capable of metabolizing WSC to produce organic acids, thereby competing with LAB for nutrients. This can result in the fermentation of WSC to produce acetic and butyric acids (Lv et al., 2020), which can lead to high DM loss and poor silage quality (Wang et al., 2019). Following a 60-day ensiling period, the abundance of Firmicutes was observed to be higher in the D60 group than in the RAW group, with Lactobacillus becoming the second dominant genus in D60. Lactobacillillus were of great importance in the ensiling process, producing LA, lowering pH, and inhibiting the growth of undesirable bacteria, dominating high-quality silage, which can explain the good fermentation quality of whole-corn silage.
The composition of A. pasteurianus exhibited both some similarities and differences between silage samples subjected to disparate treatments at varying stages. It is currently the most prevalent bacterium following aerobic spoilage in the study, potentially due to its extensive distribution (Johnson et al., 1999). The research indicated that Acetobacter was hard to find in North America, but was more frequently identified in Asia and Europe, particularly in silage originating from the hot and humid regions of Asia (Oliveira et al., 2017). Previous studies have revealed that the information provided at the genus level was either limited or erroneous. For instance, there was a discrepancy between the roles assigned to homofermentive and heterofermentive LAB in silage, as evidenced by conflicting reports in the literature. Consequently, based on the bacterial abundance at the species level, this experiment identified two species of Acetobacter present during the silage and aerobic exposure stages: A. pasteurianus and A. fabarum. In the absence of inoculated A. pasteurianus, a small quantity of A. fabarum (0.35%) was also identified in the RAW group. The Acetobacter genus comprises a diverse range of species, each with distinct characteristics and functions. For instance, some oxidize ethanol to acetic acid under aerobic conditions (Nanda et al., 2021), while others engage in nitrogen fixation (Fuentes-Ramírez et al., 2001), pigment production (Malimas et al., 2009), or exopolysaccharide production (Gullo et al., 2017). However, A. fabarum has never been identified in silage and its role remained unclear. In the D60 group, A. pasteurianus emerged as a dominant species, with a relative abundance of 30.31%. This dominance may be attributed to the anaerobic fermentation of A. pasteurianus introduced over a short period. During the aerobic exposure stage, the dominance of L. plantarum was superseded by that of A. pasteurianus as a consequence of the alteration in the environment from anaerobic to aerobic. The abundance of A. pasteurianus in ASA was significantly higher than in other groups, which may be attributed to the rapid proliferation of A. pasteurianus under aerobic conditions. It is noteworthy that a high abundance of A. fabarum was observed in the uninoculated ASN group, in contrast to the ASA treatment group where A. pasteurianus was inoculated after 60 days of ensiling. The combined data on aerobic stability revealed that the ASN group exhibited significantly enhanced aerobic stability compared to the ASA group. This indicated that, despite both A. pasteurianus and A. fabarum belonging to the Acetobacter genus, their impact on the aerobic stabilization of silage may be different. The introduction of A. pasteurianus resulted in a rapid onset of aerobic destabilization, whereas the presence of A. fabarum did not appear to exert a detrimental impact on aerobic stability. The current research literature indicated that A. fabarum was commonly found during the fermentation of cocoa beans and moist maize dough (Moens et al., 2014), and was also a main species found in fig vinegar (Sommer and Newell, 2019), A. fabarum exhibited a slower rate of ethanol oxidation, with lactic acid undergoing oxidation primarily to acetonitrile and acetic acid. Following the depletion of ethanol, the oxidation of acetic acid commenced (Moens et al., 2014). Additionally, A. fabarum has been observed to engage in a mutualistic interaction with L. brevis (Sommer and Newell, 2019). However, there was a paucity of research on its role in silage, necessitating further investigation to ascertain its precise function within the silage process.
The LEfSe results demonstrated a notable decline in the epiphytic pathogens on the corn raw material, from the initial stages of processing through to the anaerobic fermentation phase. This was accompanied by a surge in the prevalence of acid-producing bacteria. Lactobacillus bacteria play an indispensable role in the conversion of nutrients into volatile fatty acids, thereby improving the quality and aerobic stability of silage (Tahir et al., 2023). Furthermore, research has demonstrated that L. plantarum, a species within the L. genus, is associated with an elevated acetic acid content in long-term silage (Nishino et al., 2012). This is because the bacterium is capable of metabolizing lactic acid into acetic acid under conditions of low sugar availability (Lindgren et al., 1990). The metabolism of lactic acid may be a contributing factor to the observed enhancement of acetic acid fermentation, as the increase in acetic acid content is significantly greater than the decrease in lactic acid content (Li and Nishino, 2013), Clostridium guangxiense, which was isolated from the D60 group, is a high-caproic acid producer, and its primary function is the synthesis of ethyl caproate. It is a strictly anaerobic spore-forming bacterium that can grow in the absence of ethanol or with an ethanol content below 7%, utilizing a variety of substrates (Tracy et al., 2012). Moderate quantities of ethanol can serve as a carbon source for caproic acid bacteria, and at a pH of 6, the acetic acid content is also elevated (Xie and Chen, 2018). The source of volatile fatty acids was inconsequential, they all resulted in a reduction in the pH of silage, thereby extending its shelf life (Larissa et al., 2021).
5 Conclusion
The presence of A. pasteurianus and A. fabarum in whole-corn silage was a natural occurrence in hot and humid areas. During the conventional fermentation stage, A. pasteurianus did not negatively impact the quality of whole-corn silage when properly sealed. However, a transition from anaerobic to aerobic conditions resulted in a rapid decline in the aerobic stability of the silage inoculated with A. pasteurianus. The presence of A. fabarum did not appear to exert a detrimental impact on the aerobic stability of the silage. RSM analysis demonstrated that at a temperature of 28°C, a DM content of 25%, and an initial A. pasteurianus concentration of 5.2 × 105 cfu·ml−1, the highest concentration of A. pasteurianus was observed during the aerobic exposure stage. Future efforts should be focused on the prevention and control of A. pasteurianus in whole-plant corn silage to prolong aerobic stability in hot and humid regions.
Data availability statement
The original contributions presented in the study are publicly available. This data can be found here: https://www.ncbi.nlm.nih.gov/, SRA: SRP530196, BioProject: PRJNA1155248.
Author contributions
RB: Conceptualization, Methodology, Software, Data curation, Formal analysis, Investigation, Validation, Writing – original draft. HL: Formal analysis, Investigation, Writing – original draft. SC: Supervision, Writing – review & editing. XY: Writing – review & editing, Data curation, Methodology. YC: Writing – review & editing, Funding acquisition, Resources. YH: Resources, Writing – review & editing, Supervision. QZ: Resources, Writing – review & editing, Funding acquisition, Validation. HG: Writing – review & editing, Conceptualization, Methodology, Software, Supervision.
Funding
The author(s) declare that financial support was received for the research, authorship, and/or publication of this article. This research was funded by the National Natural Science Foundation of China (32301507), Southwest Minzu University Double World-Class Project (CX2023014), Sichuan Provincial Natural Science Foundation (2024NSFSC1198), and National Modern Agricultural Industry Technology System Sichuan Beef Cattle Innovation Team (SCCXTD-2024-13).
Acknowledgments
The authors express their gratitude to the Southwest Minzu University Laboratory for providing the necessary equipment for this study.
Conflict of interest
The authors declare that the research was conducted in the absence of any commercial or financial relationships that could be construed as a potential conflict of interest.
Publisher’s note
All claims expressed in this article are solely those of the authors and do not necessarily represent those of their affiliated organizations, or those of the publisher, the editors and the reviewers. Any product that may be evaluated in this article, or claim that may be made by its manufacturer, is not guaranteed or endorsed by the publisher.
Supplementary material
The Supplementary material for this article can be found online at: https://www.frontiersin.org/articles/10.3389/fmicb.2024.1473238/full#supplementary-material
References
Ávila, C. L. S., and Carvalho, B. F. (2020). Silage fermentation-updates focusing on the performance of micro-organisms. J. Appl. Microbiol. 128, 966–984. doi: 10.1111/jam.14450
Basso, F. C., Adesogan, A. T., Lara, E. C., Rabelo, C. H., Berchielli, T. T., Teixeira, I. A., et al. (2014). Effects of feeding corn silage inoculated with microbial additives on the ruminal fermentation, microbial protein yield, and growth performance of lambs. J. Anim. Sci. 92, 5640–5650. doi: 10.2527/jas.2014-8258
Broderick, G. A., and Kang, J. H. (1980). Determination of ammonia and total amino acids in ruminal fluid and in vitro media. J. Dairy Sci. 63, 64–75. doi: 10.3168/jds.S0022-0302(80)82888-8
Cai, Y. (1999). Identification and characterization of Enterococcus species isolated from forage crops and their influence on silage fermentation. J. Dairy Sci. 82, 2466–2471. doi: 10.3168/jds.S0022-0302(99)75498-6
Carvalho-Estrada, P. A., Fernandes, J., da Silva, É. B., Tizioto, P., Paziani, S. F., Duarte, A. P., et al. (2020). Effects of hybrid, kernel maturity, and storage period on the bacterial community in high-moisture and rehydrated corn grain silages. Syst. Appl. Microbiol. 43:126131. doi: 10.1016/j.syapm.2020.126131
Cui, X., Sun, H., Sobhi, M., Ju, X., Guo, J., and Dong, R. (2020). Butyric acid fermentation during ensiling of wilted maize Stover for efficient methane production. ACS Sustain. Chem. Eng. 8, 6713–6721. doi: 10.1021/acssuschemeng.0c00633
Daniel, J. L. P., Bernardes, T. F., Jobim, C. C., Schmidt, P., and Nussio, L. G. (2019). Production and utilization of silages in tropical areas with focus on Brazil. Grass Forage Sci. 74, 188–200. doi: 10.1111/gfs.12417
Dolci, P., Tabacco, E., and Borreani, G. (2011). Microbial dynamics during aerobic exposure of corn silage stored under oxygen barrier or polyethylene films. Appl. Environ. Microb. 77, 7499–7507. doi: 10.1128/AEM.05050-11
Drouin, P., Tremblay, J., Renaud, J., and Apper, E. (2021). Microbiota succession during aerobic stability of maize silage inoculated with Lentilactobacillus buchneri NCIMB 40788 and Lentilactobacillus hilgardii CNCM-I-4785. Microbiol. Open. 10:e1153. doi: 10.1002/mbo3.1153
Fuentes-Ramírez, L. E., Bustillos-Cristales, R., Tapia-Hernández, A., Jiménez-Salgado, T., Wang, E. T., and Martínez-Romero, E. (2001). Novel nitrogen-fixing acetic acid bacteria, Gluconacetobacter johannae sp. nov. and Gluconacetobacter azotocaptans sp. nov., associated with coffee plants. Int. J. Syst. Evol. Microbiol. 51, 1305–1314. doi: 10.1099/00207713-51-4-1305
Gerlach, K., Daniel, J., Jobim, C. C., and Nussio, L. G. (2020). A data analysis on the effect of acetic acid on dry matter intake in dairy cattle. Anim. Feed Sci. Tech. 272:114782. doi: 10.1016/j.anifeedsci.2020.114782
Gharechahi, J., Kharazian, Z. A., Sarikhan, S., Jouzani, G. S., Aghdasi, M., and Salekdeh, G. H. (2017). The dynamics of the bacterial communities developed in maize silage. Microb. Biotechnol. 10, 1663–1676. doi: 10.1111/1751-7915.12751
Guan, H., Yan, Y., Li, X., Li, X., Shuai, Y., Feng, G., et al. (2018). Microbial communities and natural fermentation of corn silages prepared with farm bunker-silo in Southwest China. Bioresour. Technol. 265, 282–290. doi: 10.1016/j.biortech.2018.06.018
Guan, H., Yang, S., Ran, Q. F., Yan, Y. H., Wang, X., Li, D. D., et al. (2020). The microbiome and metabolome of napier grass silages prepared with screened lactic acid bacteria during ensiling and aerobic exposure. Anim. Feed Sci. Tech. 269, 114673–118401. doi: 10.1016/j.anifeedsci.2020.114673
Gullo, M., Sola, A., Zanichelli, G., Montorsi, M., Messori, M., and Giudici, P. (2017). Increased production of bacterial cellulose as starting point for scaled-up applications. Appl. Microbiol. Biot. 101, 8115–8127. doi: 10.1007/s00253-017-8539-3
Honig, H., and Woolford, M. K. (1980). Changes in silage on exposure to air. Occas. Symp. Br. Grassl. Soc. 11, 76–87.
Hou, J., and Nishino, N. (2021). Bacterial and fungal microbiota of guinea grass silage shows various levels of acetic acid fermentation. Fermentation 8:10. doi: 10.3390/fermentation8010010
Jatkauskas, J., Vrotniakienė, V., and Stoškus, R. (2018). Variations in fermentation, bacterial population and aerobic stability in maize silage. Zemdirbyste 105, 377–382. doi: 10.13080/z-a.2018.105.048
Jiang, F., Cheng, H., Liu, D., Wei, C., An, W., Wang, Y., et al. (2020). Treatment of whole-plant corn silage with lactic acid bacteria and organic acid enhances quality by elevating acid content, reducing ph, and inhibiting undesirable microorganisms. Front. Microbiol. 11:593088. doi: 10.3389/fmicb.2020.593088
Jiang, Y. M., Guan, H., Li, H. P., Wang, H., Chen, Y. J., Jia, Z. F., et al. (2023). Effect of compound Lactobacillus and cutting height on silage quality of lodging oats. Acta Agrest. Sin. 31, 3858–3866. doi: 10.11733/j.issn.1007-0435.2023.12.032
Johnson, L., Harrison, J. H., Hunt, C., Shinners, K., Doggett, C. G., and Sapienza, D. (1999). Nutritive value of corn silage as affected by maturity and mechanical processing: a contemporary review. J. Dairy Sci. 82, 2813–2825. doi: 10.3168/jds.S0022-0302(99)75540-2
Kleinschmit, D., and Kung, J. L. (2006). A meta-analysis of the effects of Lactobacillus buchneri on the fermentation and aerobic stability of corn and grass and small-grain silages. J. Dairy Sci. 89, 4005–4013. doi: 10.3168/jds.S0022-0302(06)72444-4
Kung, L. J., Shaver, R. D., Grant, R. J., and Schmidt, R. J. (2018). Silage review: interpretation of chemical, microbial, and organoleptic components of silages. J. Dairy Sci. 101, 4020–4033. doi: 10.3168/jds.2017-13909
Kung, L., Taylor, C. C., Lynch, M. P., and Neylon, J. M. (2003). The effect of treating alfalfa with Lactobacillus buchneri 40788 on silage fermentation, aerobic stability, and nutritive value for lactating dairy cows. J. Dairy Sci. 86, 336–343. doi: 10.3168/jds.S0022-0302(03)73611-X
Larissa, S. G., Lucas, G. G., Caio, S. T., Nathália, T. S. G., Tássia, B. P. S., Júlia, A. M., et al. (2021). Different organic acid preparations on fermentation and microbiological profile, chemical composition, and aerobic stability of whole-plant corn silage. Anim. Feed Sci Tech. 281, 0377–8401. doi: 10.1016/j.anifeedsci.2021.115083
Li, Y. B., and Nishino, N. (2013). Effects of ensiling fermentation and aerobic deterioration on the bacterial community in italian ryegrass, guinea grass, and whole-crop maize silages stored at high moisture content. Asian Austr. J. Anim. 26, 1304–1312. doi: 10.5713/ajas.2013.13175
Li, B., Qian, Y. K., Xiao, Y. X., Shan, C., Liu, J. Q., Gao, F., et al. (2020). Rapid detection of acetic acid bacteria flora in wine by RT-FQPCR. China Brew. 39, 198–201. doi: 10.11882/j.issn.0254-5017.2020.08.037
Lin, C., Bolsen, K., Brent, B., and Fung, D. (1992). Epiphytic lactic acid bacteria succession during the pre-ensiling and ensiling periods of alfalfa and maize. J. Appl. Bacteriol. 73, 375–387. doi: 10.1111/j.1365-2672.1992.tb04992.x
Lindgren, S. E., Axelsson, L. T., and Mcfeeters, R. F. (1990). Anaerobic l-lactate degradation by Lactobacillus plantarum. FEMS Microbiol. Lett. 66, 209–213. doi: 10.1016/0378-1097(90)90284-W
Liu, Q., Yang, F., Zhang, J., and Shao, T. (2014). Characteristics of Lactobacillus parafarraginis ZH1 and its role in improving the aerobic stability of silages. J. Appl. Bacteriol. 117, 405–416. doi: 10.1111/jam.12530
Loures, D. R. S., Nussio, L. G., Paziani, S. F., Pedroso, A. F., Mari, L. J., and Ribeiro, J. L. (2005). Chemical composition and effluent yield of Tanzania grass silages affected by wilting, particle size and enzymatic/microbial additive. R. Bras. Zootec 34, 726–735. doi: 10.1590/S1516-35982005000300003
Lv, H., Pian, R., Xing, Y., Zhou, W., Yang, F., Chen, X., et al. (2020). Effects of citric acid on fermentation characteristics and bacterial diversity of Amomum villosum silage. Bioresour. Technol. 307:123290. doi: 10.1016/j.biortech.2020.123290
Malimas, T., Yukphan, P., Takahashi, M., Muramatsu, Y., Kaneyasu, M., and Potacharoen, W. (2009). Gluconobacter japonicus sp. nov., an acetic acid bacterium in the Alphaproteobacteria. Int. J. Syst. Evol. Microbiol. 59, 466–471. doi: 10.1099/ijs.0.65740-0
McDonald, P., Henderson, A. R., and Heron, S. J. E. (1991). The biochemistry of silage. 2nd Edn. Marlow, UK: Chalcombe.
Miguel, M. F., Ribeiro-Filho, H. M. N., and Delagarde, R. (2023). Effects of corn silage supplementation strategy and grazing intensity on herbage intake, milk production, and behavior of dairy cows. J. Dairy Sci. 106, 1013–1025. doi: 10.3168/jds.2021-21649
Moens, F., Lefeber, T., and De, V. L. (2014). Oxidation of metabolites highlights the microbial interactions and role of Acetobacter pasteurianus during cocoa bean fermentation. Appl. Environ. Microb. 80, 1848–1857. doi: 10.1128/AEM.03344-13
Nanda, K., Taniguchi, M., Ujike, S., Ishihara, N., Mori, H., and Ono, H. (2021). Characterization of acetic acid bacteria in traditional acetic acid fermentation of rice vinegar (komesu) and unpolished rice vinegar (kurosu) produced in Japan. Appl. Environ. Microb. 67, 986–990. doi: 10.1128/AEM.67.2.986-990.2001
Nishino, N., Li, Y., Wang, C., and Parvin, S. (2012). Effects of wilting and molasses addition on fermentation and bacterial community in guinea grass silage. Lett. Appl. Microbiol. 54, 175–181. doi: 10.1111/j.1472-765X.2011.03191.x
Oliveira, A. S., Weinberg, Z. G., Ogunade, I. M., Cervantes, A. A. P., Arriola, K. G., and Jiang, Y. (2017). Meta-analysis of effects of inoculation with homofermentative and facultative heterofermentative lactic acid bacteria on silage fermentation, aerobic stability, and the performance of dairy cows. J. Dairy Sci. 100, 4587–4603. doi: 10.3168/jds.2016-11815
Oroian, M., Ursachi, F., and Dranca, F. (2020). Ultrasound-assisted extraction of polyphenols from crude pollen. Antioxidants 9:322. doi: 10.3390/antiox9040322
Pahlow, G., and Muck, R. E. (2009). Managing for improved aerobic stability. Proceedings of the 15th International Silage Conference. Madison, 77–90.
Ranjit, N. K., and Kung, L. J. (2000). The effect of Lactobacillus buchneri, Lactobacillus plantarum, or a chemical preservative on the fermentation and aerobic stability of corn silage. J. Dairy Sci. 83, 526–535. doi: 10.3168/jds.S0022-0302(00)74912-5
Santos, A. O., Ávila, C. L. S., and Schwan, R. F. (2013). Selection of tropical lactic acid bacteria for enhancing the quality of maize silage. J. Dairy Sci. 96, 7777–7789. doi: 10.3168/jds.2013-6782
Sommer, A. J., and Newell, P. D. (2019). Metabolic basis for mutualism between gut Bacteria and its impact on the Drosophila melanogaster host. Appl. Environ. Microb. 85, e01882–e01818. doi: 10.1128/AEM.01882-18
Spoelstra, S. F., Courtin, M. G., and Beers, J. A. C. V. (1988). Acetic acid bacteria can initiate aerobic deterioration of whole crop maize silage. J. Agric. Sci. 111, 127–132. doi: 10.1017/S0021859600082915
Tahir, M., Li, J. Y., and Xin, Y. F. (2023). Response of fermentation quality and microbial community of oat silage to homofermentative lactic acid bacteria inoculation. Front. Microbiol. 13:1091394. doi: 10.3389/fmicb.2022.1091394
Thi Minh Tu, T., Van, N., and Nishino, N. (2014). A pilot examination of the fermentation products, aerobic stability and bacterial community of total mixed ration silage produced in Vietnam. Grassl. Sci. 60, 63–68. doi: 10.1111/grs.12041
Tracy, B. P., Jones, S. W., Fast, A. G., Indurthi, D. C., and Papoutsakis, E. T. (2012). Clostridia: the importance of their exceptional substrate and metabolite diversity for biofuel and biorefinery applications. Curr. Opin. Biotech. 23, 364–381. doi: 10.1016/j.copbio.2011.10.008
Van Soest, P. J., Robertson, J. B., and Lewis, B. A. (1991). Methods for dietary fiber, neutral detergent fiber, and nonstarch polysaccharides in relation to animal nutrtion. J. Dairy Sci. 74, 3583–3597. doi: 10.3168/jds.S0022-0302(91)78551-2
Wang, C., He, L., Xing, Y., Zhou, W., Yang, F., Chen, X., et al. (2019). Fermentation quality and microbial community of alfalfa and stylo silage mixed with Moringa oleifera leaves. Bioresour. Technol. 284, 240–247. doi: 10.1016/j.biortech.2019.03.129
Wang, C., Wang, Z., and Wang, P. (2016). Multiple effects of environmental factors on algal growth and nutrient thresholds for harmful algal blooms: application of response surface methodology. Environ. Model. Assess. 21, 247–259. doi: 10.1007/s10666-015-9481-3
Weiss, K., Kroschewski, B., and Auerbach, H. (2016). Effects of air exposure, temperature and additives on fermentation characteristics, yeast count, aerobic stability and volatile organic compounds in corn silage. J. Dairy Sci. 99, 8053–8069. doi: 10.3168/jds.2015-10323
Wilkinson, J. M., and Davies, D. R. (2013). The aerobic stability of silage: key findings and recent developments. Grass Forage Sci. 68, 1–19. doi: 10.1111/j.1365-2494.2012.00891.x
Wu, D. T., Deng, W., Li, J., Geng, J. L., Hu, Y. C., Zou, L., et al. (2023). Ultrasound-assisted deep eutectic solvent extraction of phenolic compounds from thinned young kiwifruits and their beneficial effects. Antioxidants 12:1475. doi: 10.3390/antiox12071475
Xie, S. K., and Chen, J. X. (2018). Isolation and application of caproic acid bacteria strains from pit mud. Liquor Mak. Sci/ Technol. 9, 44–51. doi: 10.13746/j.njkj.2018089
Xu, D., Ding, W., Ke, W., Li, F., Zhang, P., and Guo, X. (2019). Modulation of metabolome and bacterial community in whole crop corn silage by inoculating homofermentative Lactobacillus plantarum and heterofermentative Lactobacillus buchneri. Front. Microbiol. 9:3299. doi: 10.3389/fmicb.2018.03299
Yan, J., Sun, Y. B., Kang, Y. H., Meng, X. Y., Zhang, H., Cai, Y. F., et al. (2022). An innovative strategy to enhance the ensiling quality and methane production of excessively wilted wheat straw: using acetic acid or hetero-fermentative lactic acid bacterial community as additives. Waste Manag. 149, 11–20. doi: 10.1016/j.wasman.2022.06.001
Yang, L., Yuan, X., Li, J., Dong, Z., and Shao, T. (2019). Dynamics of microbial community and fermentation quality during ensiling of sterile and nonsterile alfalfa with or without Lactobacillus plantarum inoculant. Bioresour. Technol. 275, 280–287. doi: 10.1016/j.biortech.2018.12.067
Zhao, S., Yang, F., Wang, Y., Fan, X., Feng, C., and Wang, Y. (2021). Dynamics of fermentation parameters and bacterial community in high-moisture alfalfa silage with or without lactic acid bacteria. Microorganisms 9:1225.
Zhao, Y. B., Yu, J. D., Liu, J. J., Yang, H. Y., Gao, L. J., Yuan, X. F., et al. (2016). Material and microbial changes during corn stalk silage and their effects on methane fermentation. Bioresour. Technol. 222, 89–99. doi: 10.1016/j.biortech.2016.09.113
Keywords: Acetobacter pasteurianus , whole-plant corn silage, aerobic stability, bacterial community, response surface methodology
Citation: Bai R, Li H, Chen S, Yuan X, Chen Y, Huang Y, Zhou Q and Guan H (2024) Microbiome and response surface methodology analyses reveal Acetobacter pasteurianus as the core bacteria responsible for aerobic spoilage of corn silage (Zea mays) in hot and humid areas. Front. Microbiol. 15:1473238. doi: 10.3389/fmicb.2024.1473238
Edited by:
Ilana Kolodkin-Gal, Reichman University, IsraelCopyright © 2024 Bai, Li, Chen, Yuan, Chen, Huang, Zhou and Guan. This is an open-access article distributed under the terms of the Creative Commons Attribution License (CC BY). The use, distribution or reproduction in other forums is permitted, provided the original author(s) and the copyright owner(s) are credited and that the original publication in this journal is cited, in accordance with accepted academic practice. No use, distribution or reproduction is permitted which does not comply with these terms.
*Correspondence: Hao Guan, guanh0427@outlook.com