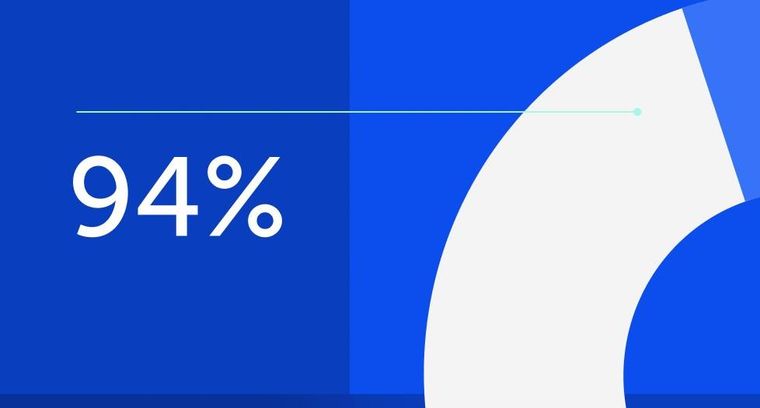
94% of researchers rate our articles as excellent or good
Learn more about the work of our research integrity team to safeguard the quality of each article we publish.
Find out more
ORIGINAL RESEARCH article
Front. Microbiol., 29 August 2024
Sec. Microbiological Chemistry and Geomicrobiology
Volume 15 - 2024 | https://doi.org/10.3389/fmicb.2024.1463665
This article is part of the Research TopicMicrobial Involvement in Biogeochemical Cycling and Contaminant Transformations at Land-Water EcotonesView all 11 articles
Mining disturbance has great impacts on soil physicochemical factors, causing notable differences between pre-mining and after-mining conditions, and between coal mining areas and non-mined areas. However, little is known about whether the fissure statuses induced by mining activities affect the edaphic factors and how soil microbial communities respond to these fissure development states. In this study, we systematically investigated the edaphic factors and microbial communities in a mining disturbance area exhibiting the full development status of soil fissures, where the sampling sites were divided into soil fissure development and closure zones. Microbial alpha-and beta-diversity, correlation coefficient matrix, non-metric multi-dimensional scaling, principal co-ordinates analysis, mantel test, and microbial co-occurrence network were employed to elucidate variations, correlations, and interactions between edaphic factors and microbial communities under the two different soil fissure states. Results suggested that soil physicochemical properties were significantly affected by fissure states, showing an increasing trend in soil moisture content and soil nutrients. The associations among edaphic factors have weakened during the soil fissure development process. Soil microbial communities showed different compositions and the underlying influential mechanisms between two soil fissure states. Soil moisture content, pH, particle compositions, organic matter, and heavy metals largely affected microbial communities. Rare species were vulnerable to mining disturbance and were keystone taxa that reinforced the overall interconnections of the soil microbial community (e.g., Nordella, Sphingomonas, Massilia, and Rubritepida). Our study revealed the impacts of distinct fissure states on the soil physicochemical properties and microbial communities, and the edaphic conditions showed key contributions to the soil microbial communities, particularly the abundance and ecological roles of rare species.
China’s energy supply heavily relies on coal resources, although vigorously advocating the “two-carbon policy” in recent years. The Ordos Plateau and its adjacent areas had been bearing tremendous shallow-buried coal deposits and became one of the most important coal-producing areas in China. However, the development of the coal mining industry has resulted in soil fissures, land subsidence, degradation, etc. in mining disturbance areas (Liu et al., 2015; Wang G. et al., 2017; Yang et al., 2019), and further causing countless losses, severe anthropogenic geological disasters. Mining fissures are categorized into three types: tensile fissures, collapse fissures, and sliding fissures. The dynamic characteristics of mining-induced fissures undergo a development cycle of fissure-expansion-closure (Liu et al., 2019; Kang et al., 2023). Ground fissures showed an inverse C-shape feature and developed in advance of the coal seam working face excavation, and then distributed in the “O” form on the boundary of the working face after subsidence stabilized (Hou et al., 2022).
The emergence of soil fissures has a profound influence on the ecological environment and soil physicochemical factors (Liu et al., 2019). Mining activities significantly altered soil organic matter (SOM), total nitrogen (TN), and available phosphorus (AP). Upon mining disturbances, SOM, TN, and AP typically decreased by 35.49, 23.56, and 38.06%, respectively, compared to the pre-mining topsoil (Wang et al., 2021). Other lowered indicators included soil moisture content, soil clay content, and available potassium (Luo et al., 2019; Ma et al., 2019). Moreover, in the vertical profile of the mining disturbance area, most soil parameters (e.g., SOM, AP) were lower than those in the nonmined area at all depths. In contrast, these indicators showed an opposite trend in the nonmined area with increasing depth, indicating that mining activities altered the vertical distribution of edaphic factors (Shi et al., 2017). However, in other cases, soil nutrients (e.g., TP, TN) showed an increasing trend with the development of soil fissures (Peng et al., 2020; Long et al., 2022).
Soil microorganisms are ubiquitously present in soil and have tight correlations with their environment and nutrient levels (Chapman et al., 2018). Therefore, soil microbial communities are highly susceptible to the changing environment in mining disturbance areas. Compared to non-mined sites, soil microbial community structure and diversity in the coal mining area would be impaired and significantly influenced by soil properties (Ezeokoli et al., 2019; Yuan et al., 2022). Under the mining disturbance conditions, soil pH was deemed as one of the most influential factors for shaping microbial diversity, taxonomic composition, and ecological distribution, especially along the mining disturbance gradient (Xiao et al., 2021a). Additionally, other factors influencing soil bacterial community included conductivity (EC), soil moisture content, soil depth, nutrient concentrations, etc. (Banning et al., 2012; Guo et al., 2021; Sheng et al., 2021b). Mining activities have been shown to reduce soil microbial abundance and diversity (Shi et al., 2017). There were also concerns regarding the recovery of the soil environment and microbial communities after mining activity ceased and the duration required for recovery. While soil pH, moisture content, nutrients, and heavy metals were stabilized and reconnected with microbial communities after soil restoration, no matter the positive or negative restoration processes, these attributes rarely return to their original levels, even after decades of restoration efforts (Banning et al., 2012; de Quadros et al., 2016).
In all, there remains a substantial knowledge gap regarding whether the different fissure states induced by mining activities have an impact on soil microbial communities, and how the edaphic factors and microbial communities co-varied during the different fissure development states. Therefore, based on field investigation findings, we selected a shallow-buried coal seam located in the Shaanxi Province, known for its extensively developed soil fissures. An integrated approach combining soil edaphic and microbial community analysis was employed to elucidate soil physicochemical variables and microbial community variation, as well as the correlation between the soil edaphic factors and microbial communities under different soil fissure states. Soil physicochemical properties, including size distribution of soil particles, inorganic variables, organic components, and heavy metals, were determined to decipher soil edaphic conditions in mining disturbance areas. Soil bacteria and archaea communities were analyzed using high-throughput sequencing of the 16S rRNA gene. This study aimed to uncover the variations and interconnections of the edaphic condition and microbial community across distinct soil fissure states.
The study area is located at the junction of Shaanxi and Inner Mongolia Provinces, northeast of the Yushenfu mining area, one of the most coal production fields in China. This area is characterized by a temperate semi-arid continental monsoon climate with dryness, low precipitations, and meteorological disasters. The annual temperature is approximately 6.6°C, while the annual precipitation ranges from 109 mm to 819 mm, and 76% of total precipitation is concentrated from June to September (Xiao et al., 2020). In contrast, the annual evaporation is seven times greater than the rainfall. This vast deficit between precipitation and evaporation has led to the scarcity of regional water resources and fragile ecological environments. Soil texture is dominated by aeolian sandy soil and loess, characterized by poor structure, low fertility, and low resistance to erosion (Hou et al., 2022). Therefore, surface soil is sensitive to mining disturbance, and fissures are extensively developed. The sampling site was chosen at one of the most productive coal mines, the Ningtiaota coalfield, with flat topography in the Yushenfu mining area (Huang et al., 2018). In this area, the active mining depth of the Ningtiaota coalfield is less than 200 m, causing surface fissures to reach the topsoil directly. Due to shallow-buried coal seams and large coal yields, two different types of soil fissures developed: fissure development state and fissure closure state (Hou et al., 2022).
Soil sampling sites were strategically chosen based on the field investigations during September 2021, with sampling points distributed across the soil fissure development and closure areas of the coal mine (Figure 1; Supplementary Figure S1). A total of 12 sampling points were selected based on the soil fissure states, of which half were distributed at the soil fissure development zone (FDZ, including NTT02, NTT03, NTT06, NTT07, NTT10, and NTT12), and the other half were distributed at the soil fissure closure zone (FCZ, including NTT01, NTT04, NTT05, NTT08, NTT09, and NTT11). Soil samples were collected 10 cm below the surface to avoid interference of potential surface pollutants. For each sample, five replicates were uniformly collected within a 1 m radius and mixed to effectively form one soil sample based on the five-point sampling method (Deng et al., 2013). Each collected sample was split into two parts for physicochemical and biological analysis. For the edaphic factors determination, rocks and roots were removed from the soil samples, which were then air-dried and sieved through a 0.25 mm sieve before the measurements (Xin et al., 2016). Soil samples for microbial analysis were collected by sterile shovel, stored in dry ice immediately in the field, and transported into the laboratory within 48 days for further DNA extraction (Sheng et al., 2021b). All samples were preserved under 4°C before being transported to the laboratory within 48 h.
Soil samples were analyzed for a variety of geochemical parameters. Soil pH and electrical conductivity (EC) were tested using a glass electrode in water (1:2.5 and 1:5 soil/water ratio, respectively). Soil texture, soil moisture content (SMC), cation exchange capacity (CEC), total phosphorus (TP), available phosphorus (AP), total potassium (TK), and available potassium (AK) were determined by standard methods (Lu, 1999). The soil organic carbon (SOC) content was measured using the dichromate oxidation method (Kalembasa and Jenkinson, 1973). Total carbon (TC) and dissolved organic carbon (DOC) content were measured by the total organic carbon analyzer (TOC-V Series SSM-5000A). Soil microbial biomass carbon (MBC) and nitrogen (MBN) were determined by the chloroform fumigation-extraction method, which is the most common technique for measuring microbial biomass elements (Cleveland and Liptzin, 2007). Heavy metals, including cadmium (Cd), iron (Fe), copper (Cu), manganese (Mn), and chromium (Cr), were estimated by atomic absorption spectrometry (McLaughlin et al., 2000; Tüzen, 2003). Hydride generation atomic fluorescence detection measured total arsenic (As) in soil samples (Shi et al., 2003). Total nitrogen (TN) was measured by the Kjeldahl method, and ammonium nitrogen (NH4-N) and nitrate nitrogen (NO3-N) were determined by extracting fresh soil with 1 mol/L KCl for 30 min and were analyzed using an autoanalyzer. Alkali-hydrolyzable nitrogen (AHN) was regarded as soil-available nitrogen and determined by the diffusion-absorption method (Zhang et al., 2018; Xiao et al., 2019). Nitrite nitrogen (NO2-N) was analyzed colorimetrically by extraction method with potassium chloride (KCl, 1 mol/L) solution (Dai et al., 2020). Humus substance (Humus) and its fractions [humin (Hm), humic acid (HA), and fulvic acid (FA)] were determined based on the standard protocols after extraction with a mix of sodium hydroxide (0.1 mol/L) and sodium pyrophosphate (0.1 mol/L) (Sheng et al., 2021b).
Soil genomic DNA was extracted using the FastDNA Spin Kit (MP Biomedicals™, Fisher Scientific, United States) according to the manufacturer’s instructions. Amplification of partial hypervariable 16S rRNA gene was performed by using bacterial primer pairs 338F (5′-ACTCCTACGGGAGGCAGCAG-3′)/806R (5′-GGACTACHVGGGTWTCTAAT-3′) for V3–V4 region (Liu et al., 2020), and archaeal primer pairs 524F10extF (5′-TGYCAGCCGCCGCGGTAA-3′)/Arch958RmodR (5′-YCCGGCGTTGAVTCCAATT-3′) for V4–V5 region (Liu et al., 2016). Bacterial and archaeal soil genomic DNA was amplified by PCR which consisted of an initial denaturation step (94°C) for 5 min, followed by 30 cycles of 94°C (1 min), 64°C (1 min), and 72°C (1 min), and a final 10 min elongation (72°C) (Sheng et al., 2021b). After PCR, 16S rRNA amplicons were extracted from 2% agarose gels and purified using the AxyPrep DNA Gel Extraction Kit (Axygen Biosciences, United States) and quantified by a fluorescent quantitation system (QuantiFluor™-ST, Promega, United States) according to the manufacturer’s instructions. Purified amplicons were pooled in equimolar and paired-end sequenced on an Illumina MiSeq platform. The raw reads were deposited into the NCBI Sequence Read Archive (SRA) database (Accession Number: SRP503985).
Totally 542,868 bacterial raw reads and 684,944 archaeal raw reads were produced for all samples, and the rarefaction curve showed that the numbers of observed operational taxonomic units (OTU) were close to saturation, indicating a sufficient sequencing depth (Sheng et al., 2016). The sequencing quality controlling of raw reads was conducted by removing low-quality reads using the QIIME pipeline as previously described (Caporaso et al., 2010; Guo et al., 2022). Operational taxonomic units (OTUs) were clustered using UPARSE based on the 97% similarity criteria (Edgar, 2013). The taxonomy of each OTUs was analyzed according to the SILVA (SSU115) database (Wang et al., 2007). Representative OTUs were also blasted against the NCBI taxonomic database1 to acquire a more reliable taxonomic resolution for further analysis. Alpha-and beta-microbial diversity were calculated within QIIME, and microbial beta-diversity was conducted by Bray–Curtis metrics based on pairwise distance values (Guo et al., 2022).
The correlation between edaphic factors was measured by the Pearson correlation coefficient. Mantel test was used to evaluate the correlation between soil physicochemical properties and microbial communities. To avoid the bias induced by different units of edaphic factors, soil datasets were scaled before calculating. Pearson correlation analysis and Mantel test were performed and visualized by using “vegan,” and “LinkET” packages in R. Non-metric multi-dimensional scaling (NMDS) and principal co-ordinates analysis (PCoA) were conducted to evaluate the similarity of microbial communities in soil samples. Other statistical analyses, e.g., one-way analysis of variance (ANOVA), and analysis of similarity (ANOSIM) with 9,999 permutations were conducted by using SPSS 19.0 (IBM Company, 2010). Redundancy analysis (RDA, lengths of gradient ≤3.0) was implemented with the forward selection of variables in Canoco 5.0, and the contribution of each edaphic factor to microbial beta diversity and associated statistical tests were calculated. To estimate microbial species co-occurrence patterns, distance-based microbial networks were constructed with those microbial taxa (OTU level) with >50% presence at each sample by the Spearman correlation matrix of pairwise associations among the OTUs (Sheng et al., 2021c). The network topological indices [e.g., nodes, links, average degree (avgK), average clustering coefficient (avgCC), average path distance (GD), within-module connectivity (Zi), and among-module connectivity (Pi)] were calculated to describe the properties of the networks and the network was visualized by the Gephi 0.9.2 as previously described (Deng et al., 2012).
The size distribution of soil particles in the study area predominantly consisted of fine sand and silt, with relatively low proportions of clay and coarse sand (Table 1). The content of fine sand in the fissure closure zone (FCZ, 64.8 ± 3.0%) was slightly lower than that in the fissure development zone (FDZ, 67.9 ± 4.0%). In contrast, the contents of silt and clay in the FCZ group were higher than those in FDZ, with silt at 21.85 ± 2.24% and clay at 12.0 ± 0.7% % in FCZ, compared to 20.43 ± 2.9% and 10.2 ± 1.4% in FDZ. Coarse sand, the largest particle in soil, was less than 2% in both FCZ and FDZ groups. Soil pH exhibited minor variations, ranging from 7.95 to 9.05 with an average of 8.6, indicating an alkaline nature. EC and SMC exhibited significant variations. The FCZ group displayed lower EC (41.1 ± 5.6 μs/cm) and SMC (4.77 ± 0.26%) compared to the FDZ group (53.2 ± 3.6 μs/cm and 5.71 ± 0.22%, respectively).
Soil carbon, nitrogen, and organic matter were significantly affected by variations in soil fissure status induced by mining disturbance (Table 1 and Supplementary Figure S2). In FCZ, TC, MBC, and DOC were significantly lower than those in FDZ (p < 0.05). Specifically, TC was 17.34 ± 1.44 g/kg in FCZ compared to 22.32 ± 1.13 g/kg in FDZ, MBC was 53.4 ± 4.84 mg/kg in FCZ versus 80.5 ± 9.76 mg/kg in FDZ, and DOC was 55.96 ± 2.81 mg/kg in FCZ compared to 67.51 ± 1.64 mg/kg in FDZ, indicating declines of 22.3, 33.7, and 17.1%, respectively. Furthermore, compared with the FDZ region, the contents of SOC (4.33 ± 0.59 g/kg and 4.46 ± 0.28 g/kg), Humus (1.01 ± 0.1 g/kg and 1.15 ± 0.06 g/kg), and HA (0.68 ± 0.08 g/kg and 0.85 ± 0.09 g/kg) decreased in FCZ, accounting for reductions of 2.9, 11.8, and 20.69%, respectively. Hm, the largest fraction of molecules remained stable in both sampling regions (3.31 ± 0.49 g/kg and 3.31 ± 0.24 g/kg). While most organic matter decreased in FCZ, FA increased from 0.29 ± 0.06 g/kg in FDZ to 0.33 ± 0.03 g/kg in FCZ, an increase of 14.27%. NO3-N, MBN, and AHN in FCZ also decreased significantly compared with those in FDZ. NO3-N content dropped from 4.65 ± 0.41 mg/kg in FDZ to 2.36 ± 0.28 mg/kg in FCZ, MBN from 8.76 ± 1.32 mg/kg to 5.55 ± 0.67 mg/kg, and AHN from 28.88 ± 1.33 mg/kg to 23.92 ± 1.84 mg/kg, representing reductions of 49.2, 36.6, and 17.3%, respectively. The contents of AK and AP in FCZ were 58.5 ± 5.0 mg/kg and 1.0 ± 0.2 mg/kg, whereas these were 72.7 ± 7.4 mg/kg and 1.3 ± 0.2 mg/kg in FDZ, representing decreases of 19.5 and 22.7%, respectively. Similarly, compared with FDZ, there was an overall reduction of 8.4% in TP and 0.4% in total potassium (TK) in FCZ. However, heavy metals had higher contents in FCZ compared to FDZ, including Cd (0.11 ± 0.02 mg/kg and 0.08 ± 0.02 mg/kg), Cr (20.62 ± 1.67 mg/kg and 17.35 ± 1.35 mg/kg), Fe (22.38 ± 1.51 g/kg and 20.12 ± 1.05 g/kg), and Mn (0.23 ± 0.02 g/kg and 0.23 ± 0.01 g/kg). These variables increased by 36.5% for Cd, 18.84% for Cr, 11.22 for Fe, and 6.18% for Mn. Conversely, only Cu (5.06 ± 0.55 mg/kg and 5.33 ± 0.45 mg/kg) and As (5.33 ± 0.67 mg/kg and 5.57 ± 0.38 mg/kg) in FCZ had lower contents than that of FDZ, the declines accounted for 5.06 and 4.33%, respectively. To sum up, soil nutrients decreased but metals generally increased from FDZ to FCZ.
In the study area, the correlations between the edaphic factors in FCZ differed significantly from those in FDZ (Figures 2A,B). The heatmap results revealed that edaphic factors had high-level associations in FCZ and the coefficient was generally greater than that in FDZ. Only CEC and TN had significant positive correlations with heavy metals (such as Cu, Mn, Cr, Fe, and As), and the correlation between heavy metals strengthened in FDZ. The results clearly showed that the relationship among soil physicochemical properties significantly deteriorated during the development process of soil fissures in the study area. Notably, the size distribution of soil particles was significantly correlated with soil physicochemical components in FCZ (Figure 2A). Clay and silt, which are smaller diameter particles, had a negative correlation with organic components (including SOC, Humus, HA, and Hm), AHN, CEC, and Mn, while fine sand particles with larger diameter showed a significant positive correlation with above-mentioned factors. Coarse sand was not significantly correlated with these variables but was negatively correlated with SMC, TC, MBC, and FA. This is a solid dataset indicating that the soil physicochemical properties were significantly affected by the different fissure states caused by mining disturbance, resulting in significant divergence in their co-appearance and correlations.
Figure 2. Pearson correlation matrix of the edaphic factors, and the correlation between microbial community composition and the edaphic factors using the Mantel test. (A) Pearson and Mantel results in the FCZ. (B) Pearson and Mantel results in the FDZ. *p < 0.05, **p < 0.01, and ***p < 0.001. The strength of the correlation is represented by the thickness of the lines, and the significance p-values are annotated with colors (yellow: p < 0.01; green: p < 0.05; gray: p > 0.05).
According to the results of microbial high-throughput sequencing, a total of 486,595 bacterial sequences and 607,615 archaeal sequences were obtained, with the number of valid archaeal sequences surpassing that of bacterial sequences, indicating a substantial and non-neglectable archaea community harbored in the soils. This study evaluated the microbial community’s richness, diversity, and evenness using the microbial alpha diversity index (Table 2). The Sobs and Chao indexes represent the observed and theoretical values of microbial richness, which had an average value of 2,563 and 3,170 for the bacteria community and 254 and 366 for the archaea community, indicating a higher microbial richness level of the bacteria community than the archaea community. The Shannon and the Simpson indexes represent the diversity of microbial communities. The bacterial community showed higher diversity levels than the archaea community, as the average values were 6.5 and 0.0035 for the bacteria community and 2.1 and 0.17 for the archaea community. The evenness of the bacterial community ranged from 0.25 to 0.29, while that of the archaeal community ranged from 0.02 to 0.04, suggesting that the archaea community was less evenly distributed.
The archaea communities’ composition was simple and homogeneous (Figure 3A and Supplementary Table S1). Nitrososphaeria class, the most broadly distributed chemolithoautotrophy of Archaea and known for its capacity of aerobic oxidation of ammonia (Kerou et al., 2016), had an overwhelming abundance of more than 95% in all soil samples. At the genus taxonomic level, 72.04% ± 1.6% archaea lineages belonged to the genus Nitrososphaera, while 17.52% ± 0.9% archaea were identified as Candidatus Nitrososphaera, genetically similar to those of the Nitrososphaera genus. Additionally, 8.17% ± 1.4% of archaea belonged to an unclassified genus, Candidatus Nitrocosmicus. The aforementioned archaea showed no significant difference between the FDZ and FCZ groups (p > 0.05), indicating that mining disturbance has a relatively minor impact on the distribution of dominant archaea. Correspondingly, Methanomassiliicoccus (0.9% ± 0.2%) and numerous rare species (classified as “others” in this study) with relative abundances less than 1% showed significant differences between the two distinct sampling regions (p < 0.05), indicating mining disturbance has a greater impact on rare species within the archaea community.
Figure 3. Microbial community compositions in the mining disturbance areas. (A) Archaea community at the genus taxonomic level. (B) Bacteria community at the class taxonomic level.
The bacteria community was more complex and divergent compared to the archaea community. Due to many bacterial sequences not matching to the database at the genus taxonomic level, the bacteria composition was analyzed at the class taxonomic level (Figure 3B and Supplementary Table S2). No single bacterial lineage exceeded 25% relative abundance within the bacterial community. The most abundant bacteria, Actinomycetes, had an average abundance of 20.0% ± 0.6% across all soil samples, with small variations among sampling points. Thermoleophilia, Alphaproteobacteria, and Vicinamibacteria maintained relative abundances of around 10%, with average values of 11.79% ± 0.5, 11.75% ± 0.6, and 9.46% ± 1.1%. Blastocatellia, Dehalococcoidia, Gemmatimonadetes, Chloroflexia, Gammaproteobacteria, and Bacilli were all top 10 abundant bacteria, with abundances of 5.46% ± 0.5, 4.77% ± 0.3, 3.92% ± 0.2, 3.84% ± 0.2, 3.67% ± 0.2, and 3.36% ± 0.5%, respectively. Furthermore, Rubrobacteria, Acidimicrobiia, Methylomirabilia, Bacteroidia, Bacillota sensu stricto incertae sedis, and Anaerolineae exceeded 1% of bacteria community. Similar to archaeal community, there was no significant difference (p > 0.05) in the relative abundances of bacteria above 1% between FDZ and FCZ, while some rare species (e.g., Rhodothermia, no-ranked Armatimonadota, and no-ranked Bacteroidota) exhibited significant differences (p < 0.05) between the two different regions.
Soil microorganisms play a vital role in maintaining the surface ecological environment and geochemical elements cycling, especially in ecologically vulnerable areas. Dry climate, scarce rainfall, and extensive coal mining activities in the study area destroyed the original structures and stresses of soil and rock and further exacerbated the vulnerability of the surface ecological environment. To quantitatively describe variations in microbial community compositions and how they respond to the mining activities, microbial beta-diversity analysis was employed. Microbial beta-diversity of the archaea and bacteria communities was analyzed by NMDS and PCoA methods, and both analyses were conducted at the same taxonomic level to ensure the consistency and comparability of the results.
Briefly, the stress values of NMDS results for the microbial communities were less than or close to 0.05, and the explanatory degree of the first two components (PC1 and PC2) in PCoA results exceeded 70%. This indicated high representativeness, allowing for a quantitative description of microbial composition characteristics (Figure 4). The distribution of the archaeal community was scattered with no obvious clustering between samples, primarily following the direction of the horizontal axis on the biplots (Figures 4A,B). Rare species could be the main attributions for the scattered pattern since significant variations observed in the rare species among the archaeal communities. Additionally, the archaeal communities collected from FDZ and FCZ overlapped on the biplot. The archaeal communities in FDZ showed more scattered distribution compared to that of FCZ on the plot, indicating more pronounced differences. Comparatively, the distribution of the bacterial community on the biplots appeared to be more clustered compared to the archaea community (Figures 4C,D), indicating a higher similarity in bacteria community compositions. In the biplot, samples from FDZ were generally distributed along the horizontal axis while those from FCZ were distributed along the vertical axis, hinting that the bacteria communities in FDZ and FCZ regions were influenced by distinct dominant factors.
Figure 4. NMDS and PCoA biplot of (A,B) archaea community and (C,D) bacteria community, respectively.
Mining activities lead to significant changes in soil physicochemical properties due to soil fissure development and the subsequent closure. From the results of microbial beta diversity, the impact of mining disturbances on bacteria communities was more profound than that of archaea communities. Although the distribution characteristics of the two bacteria community subgroups on the biplot were different, the overall beta diversity differences between FCZ and FDZ were not significantly greater than within-group differences (ANOSIM, p > 0.05), reflecting the complexity of soil microbial communities.
In the present study, under the two different fissure statuses, the interactions between various environmental indicators and soil bacteria and archaea communities were determined by the Mantel test (Figure 2). Results showed that the bacteria community was significantly correlated with SMC, Cr, Fe, and AP, while the archaea community was strongly correlated with soil particle compositions (including silt and fine sand particles), pH, SMC, TN, TC, DOC, Hm, HA, Cr, Fe, and AP in the FCZ subregion (Figure 2A). In contrast, three environmental variables including coarse sand composition, DOC, and AP were significantly associated with the bacteria community in the FDZ region. At the same time, TN, FA, Mn, Cd, Cr, Fe, and TP likely impacted the archaea community due to significant correlations (Figure 2B). Archaea communities showed stronger interactions with environmental variables than bacteria communities under both stages of soil fissures, in terms of the number of associated environmental variables. This result indicated that the impact on archaeal communities may be more significant and far-reaching, despite variations in soil physicochemical properties caused by mining disturbances. Furthermore, for both bacterial and archaeal communities, the number and strength of significant correlations with environmental variables were fewer and weaker in the FDZ region than in the FCZ region, suggesting that the associations between microorganisms and soil environments deteriorated during the development of soil fissure, consistent with the observed deterioration in correlations among edaphic factors.
In this study, a total of 32 soil physicochemical variables were measured. However, collinearity among these parameters could obstruct the accuracy of analyses regarding the relationship between environmental conditions and soil microbial communities. To address this, a collinearity test was conducted, and 10 parameters were selected, including SMC, EC, MBN, NO3-N, DOC, FA, Cr, Fe, TK, and AP. The two-dimensional ordination plot generated by RDA results showed that the top two components accounted for more than 50% of the overall community variance (Figure 5). Edaphic factors had different impacts on bacteria and archaea communities in FCZ and FDZ. Fe, SMC, and AP had significant impacts on the bacteria community (p < 0.05), explaining total variances of 17.8, 17, and 12%, respectively (Figure 5A and Supplementary Table S3). MBN also accounted for a high level of explanatory degree (12.2%). In addition, the explanations of EC and FA were greater than 5%. In the FCZ subgroup, the edaphic factors, including Fe, Cd, MBN, FA, DOC, and NO3-N, showed strong influences on bacterial communities. Among these factors, Fe, DOC, and MBN exhibited greater contributions. In the FDZ subregion, the direction of SMC, EC, AP, and TK were the main influential factors. The influence of the physicochemical factors on the archaea community was also depicted by the RDA biplot (Figure 5B and Supplementary Table S4). The impact of SMC, FA, and Cd on the archaea community was significant (p < 0.05) and these three environmental factors collectively explained 53.8% of the overall community variations, with individual contributions of 25.6, 19.1, and 13.6%, respectively. Additionally, NO3-N, Fe, DOC, and EC accounted for 9.3, 8.9, 7.9, and 5.5% of the overall community variance. In the FCZ subsampling area, SMC, DOC, NO3-N, EC, AP, and MBN exhibited strong influences on the soil archaea communities, and SMC had the greatest contribution. In contrast, in the FDZ, FA, Cd, and Fe presented a high correlation with the archaea community, and FA and Cd significantly correlated with archaea communities (p < 0.05).
Figure 5. Drivers of microbial community composition. Redundancy analysis (RDA) between (A) bacteria community and (B) archaea community indicating the relationship between the edaphic factors and microbial communities at the OTU taxonomic level.
Soil microbial co-occurrence networks were used to explore the interactions among soil microorganisms in the FCZ and FDZ subregions. No significant difference in the number of nodes, edges, and avgK of the bacterial community co-occurrence network was observed under the two different stages of soil fissure (Figures 6A–D and Table 3). Briefly, the number of microbial co-occurrence network nodes, edges and avgK of the bacteria communities in FCZ was 1,822, 6,307, and 6.923. Correspondingly, the number of nodes, edges, and avgK of the bacteria communities in FDZ was 1,841, 6,489, and 7.049. However, as for the archaeal communities, significant difference was observed. The microbial co-occurrence network of archaea communities in FDZ had more interactions than those in FCZ, in terms of the number of nodes (155 in FDZ, 132 in FCZ), edges (465 in FDZ, 324 in FCZ), and avgK (6 in FDZ, 4.909 in FCZ). In addition, soil microbial co-occurrence network in FDZ had closer interactions than FCZ based on the GD values (Bacteria: 14.053 in FDZ, 15.006 in FCZ; Archaea: 6.623 in FDZ, 7.394 in FCZ; Table 3). Stronger internal microbial interactions reflected the variations of the external soil environment. The correlation analysis and Mantel result both suggested that the associations among the edaphic factors deteriorated during the development of soil fissures, which may be the main reason for the changes in the microbial co-occurrence network.
Figure 6. Microbial co-occurrence networks of archaea community in (A) FCZ, (B) FDZ, and bacteria community in (C) FCZ, (D) FDZ, respectively. The corresponding results about the roles of nodes in co-occurrence networks as indicated by their values of within-module connectivity (Zi) and among-module connectivity (Pi) of archaea community in (E) FCZ, (F) FDZ, and bacteria community in (G) FCZ, (H) FDZ.
Table 3. The microbial co-occurrence network topological parameters between the two soil fissure states.
The positive edge ratios in the co-occurrence networks of both bacterial and archaeal communities were relatively low, ranging from 55.7 to 59.26% in both FCZ and FDZ groups. This indicated interspecific competitive (or antagonistic) interactions were notable under the situation of soil disturbed by mining disturbances, regardless of whether the fissures were in development or developed state. According to Zi and Pi values, most nodes in the co-occurrence networks were peripheral (Figures 6E–H), and the number of modules of the bacterial communities (145 in FCZ, 143 in FDZ) was much higher than that of the archaeal communities (14 in FCZ, 13 in FDZ), suggesting greater abundance and complexity in bacterial communities. Soil bacteria networks had only 1 connector hub and 6 module hubs in FCZ, but those from FDZ had up to 3 connector hubs and 9 module hubs. The co-occurrence network of archaeal communities showed similar characteristics: archaeal networks had zero connector hubs or module hubs in FCZ, while the FDZ region had 1 connector hub but zero module hubs. These results indicated that soil microbial communities had more complex and interactive compositions under the soil fissures development states. Rare species constituted module hubs and connectors (Figures 6E–H), highlighting their keystone roles in microbial co-occurrence networks.
Coal mining activities have changed the stability of geological structures, especially soil fissures that occurred in the topsoil layer (Liu et al., 2019; Yang et al., 2019). Generally speaking, soil fissures experience two distinct stages: fissure development and subsequent fissure closure (Kang et al., 2023). Previous studies have shown that mining activities disturbed soil physicochemical conditions and nutrient levels to varying degrees (Ma et al., 2019; Wang et al., 2021). Lots of effort paid attention to whether and to what extent mining disturbance changes soil conditions. However, little is known about whether the fissure statuses induced by mining activities affect the edaphic factors and how soil microbial communities respond to these fissure development states. Here, a shallow buried coal mining area was selected to investigate whether the fissure status impacts soil physicochemical characteristics. In this area, according to the accurate locations of active mining panels and surface soil conditions, two states of soil fissures were identified. Almost all organic matters (e.g., MBC, MBN, DOC, AHN, and HA), SMC, AK, AP, pH, and CEC, had lower contents in the soil fissure closure zone (FCZ). This result differed from some previous studies showing that the nutrients were depleted upon fissure development (Ma et al., 2019; Wang et al., 2021). In addition, heavy metals and fine soil particles including clay and silt were enriched in FCZ (Table 1). The increase of soil fissures altered the size distribution of soil particles during soil fissure development. Clay and silt, the smaller particles in soil compositions, had lower contents in FDZ (Table 1), which might be the result of the enhanced small-sized particle’s downward movement under the increase of soil fissure space and connectivity (Wang et al., 2021).
The expansion of soil fissures would reduce the tortuosity of the water flow path to promote vertical movement of the water and result in increasing infiltration (Karacan and Goodman, 2009; Bi et al., 2014), leading to an enhancement of the effective downward flow and increase in soil moisture content (Zang et al., 2012; Wang J. et al., 2017). The contents of soil physicochemical properties, except for heavy metals, increased to a certain extent with the increasing soil moisture content in FDZ (Table 1), due to the enhanced dissolution of soluble substances in topsoil and accumulated in the subsoil (Zhu et al., 2020). In addition, lower contents of heavy metals (e.g., Mn, Cd, Cr, and Fe) in FDZ were probably due to high adsorption or binding capacity with dissolved organic matter (Ponizovsky et al., 2006; Liu et al., 2021) and microbial weathering or reductive dissolution of metals into the mobilized forms (Sheng et al., 2021a, 2023a).
However, despite these increased nutrients in FDZ, the connections among soil physicochemical properties in this area were damaged by the process of the fissure development, weakening the relationships among factors (Wang et al., 2021; Zhang et al., 2022). Soil physicochemical variables in FCZ had high-level correlations, with greater coefficients than FDZ. The size distribution of soil particles strengthened in correlations with soil physicochemical components in FCZ, especially having a significant relationship with organic matters (such as AHN, HA, and Humus). This phenomenon hinted that the associations of soil physicochemical properties were recovered as the process of the soil fissures closed. This result was similar to the previous research illustrating that mining disturbance significantly reduced TN, NO3-N, and TC contents in the soil, and the correlations of soil variables were generally recovered after the mining activities ceased (Ngugi et al., 2018).
Soil nutrients, SMC, and inorganic properties were significantly altered in the mining disturbance area, leading to a large differentiation of soil microbial biomass, community structure, and their activities (Huang et al., 2015; Guo et al., 2021; Sheng et al., 2022; Sheng et al., 2023b). The dominant viewpoint held that surface subsidence reduced microbial richness and diversity of the soil microbial community. For instance, the relative abundance of predominant microbial lineages, such as Pseudomonas, Gemmatimonas, Arthrobacter, Aciditerrimonas, Gaiella, and Sphingomonas in the mining area decreased significantly (Shi et al., 2017; Wang et al., 2023). In addition, mining disturbance changed the soil microbial co-occurrence network, and the phylogenetic community assembly mechanism differed between mining-disturbed and non-disturbed areas (Ma et al., 2023; Wang et al., 2023). However, whether and how the soil microbial community’s diversity, composition, and interaction changed under the different soil fissure states induced by mining disturbance still lacked evidence and remained unclear.
In this research, we investigated soil microbial communities under the two states of soil fissure. The archaeal community was solely composed of Nitrososphaeria which had a predominant abundance exceeding 95% in FCZ and FDZ. For the bacterial community, Actinomycetes, Thermoleophilia, Alphaproteobacteria, and Vicinamibacteria were dominant bacteria members with an average abundance exceeding 10% across all soil samples, with some variations between FCZ and FDZ (Figure 3 and Supplementary Tables S1, S2). Although there were little variations from the perspective of dominant microbial community composition, this study reported a distinct composition of rare species (e.g., Methanomassiliicoccus, Rhodothermia, Armatimonadota) between FCZ and FDZ, as well as the microbial co-occurrence network were connected by rare species (Figure 6).
Microbial communities had close correlations with soil environment conditions, including different fissure states induced by mining disturbance (Poncelet et al., 2014; Shi et al., 2017). The distribution of microbial communities in FDZ and FCZ regions showed independent vertical characteristics, revealing that the edaphic factors had varying degrees of impact on bacterial and archaeal communities in soils at two different stages of soil fissures (Figures 4, 5). During the soil fissure development process in the present study, the original porosity and structure were destroyed and the relatively close correlations among soil edaphic factors were weakened, except for heavy metals. As a result, the archaeal community showed a high correlation with organic matters in the FCZ area, and transformed to the correlations with heavy metals in the FDZ region (Figure 5B). The bacteria community showed similar features and had much lower correlations with soil physicochemical conditions in the FDZ area (Figure 5A). When the external environment changes, microorganisms tend to strengthen internal connections in response to environmental variations (Lu et al., 2022). The soil microbial co-occurrence network depicted a more complex and interactive microbial network in the FDZ region, with the soil bacterial co-occurrence network having three connector hubs and nine module hubs in FDZ and lowered to one connector hub and six module hubs in FCZ. Similar characteristics were also observed in the archaeal network, which had one connector hub in FDZ but none in FCZ (Table 3). The results showed that the relationship between soil microorganisms and the environment is destroyed in the process of developing fissures.
Not only was the microbial community affected by mining activities, but the soil microbial diversity and biomass of the restored mining area also significantly differed from that in the undisturbed area (de Quadros et al., 2016). Over time, the bacterial communities on restored sites became progressively more similar to those of nonmined analog sites (Ngugi et al., 2018). However, some findings also argued that the microbial composition and abundance of the restoration site still had significant differences with the undisturbed area after a long period of restoration time (de Quadros et al., 2016; Du et al., 2021). In this research, the process of soil fissure closure was similar to the restoration sites (Ngugi et al., 2018). As the soil fissure closed, the disturbed correlations among soil physicochemical variables reconnected, showing higher and more significant correlations compared to those in FDZ (Figure 2). Correspondingly, the bacterial and archaeal communities in FCZ showed closer correlations with soil environmental parameters compared with the microbial communities in FDZ, both in terms of the number of significantly correlated variables and the value of their correlation coefficient (Figure 2). The compositions and variations of soil microbial community and microbial-soil interactions were largely attributed to the continuity of the soil environment, as well as the correlations among physicochemical variables (e.g., soil organic matter), which is conducive to the establishment of stable microbial communities (Guo et al., 2021; Luo et al., 2019).
It is generally believed that when the environment changes, the main manifestations of microbial community variation are changes in dominant species or functional genes, especially in environments such as soil and groundwater. Soil edaphic factors, such as pH, salinity, SMC, organic matter, etc., showed high attributes in shaping microbial diversity, taxonomic composition, and functional groups (Ezeokoli et al., 2019; Guo et al., 2021; Xiao et al., 2021a). In consideration of soil fissure stages in mining disturbance areas, in the present study, we observed a significant variation occurring in rare species, instead of dominant microorganisms as the previous research reported (Xiao et al., 2021a). Archaeal lineage (e.g., Methanomassiliicoccus) and bacterial members (e.g., Rhodothermia, Armatimonadota) were significantly different between FCZ and FDZ (p < 0.05). Rare species with an abundance of less than 1% accounted for the main inter-group differences, indicating that mining disturbances have a greater impact on rare species. Previous studies have illustrated that rare species play an over-proportional role and might be a hidden driver in biogeochemical cycles (Jousset et al., 2017). Both common and rare species could contribute to soil ecological function. Dominant species make persistent, unique contributions to functional diversity, while rare species play key roles in changing environments (Chapman et al., 2018; Liang et al., 2020). Thus, it is vital to account for the abundance and diversity of rare species when interpreting their contributions to the entire microbial community diversity and variation in different soil fissure states.
The microbial co-occurrence network proved that rare species occupied an important soil ecological position in the study area, as the module hubs and connectors were composed of rare species rather than common species. In addition, rare species reinforced the overall interconnections of the soil microbial community. According to the compositions of connector hubs or module hubs, rare species such as Nordella, unclassified species of Chloroflexia, and Acidimicrobiia were connector hubs in microbial co-occurrence networks. As for module hubs, they were largely composed of rare species Sphingomonas, Massilia, Candidatus Alysiosphaera, Rubritepida, and other unclassified rare lineages (Figures 6E–H). These rare species connected microbial symbionts and provided habitat complexity in the view of co-occurrence relationships. Abundant taxa have been reported more sensitive to ecological disturbance situations, showing significant variation between non-disturbed and disturbed areas (Yuan et al., 2022). Two different soil fissure states induced by mining activities significantly impacted the edaphic factors and the microbial community (Figure 7). Compared with abundant species, rare species of soil microbial community were vulnerable to mining disturbance, as illustrated by microbial composition and co-occurrence network. Soil fissure states had a great impact on the soil edaphic factors and deteriorated the correlation between these variables in the process of soil fissure development, except for the correlation between heavy metals (Figure 6). Among these factors, soil moisture content was the most influential edaphic factor attributed to microbial variations (Figure 5). Controlled by the distinct states of soil fissures, the edaphic conditions showed key attributions to microbial communities, particularly affecting the abundance and ecological roles of rare species (Xiao et al., 2021b).
Figure 7. Conceptual map of the impacts of mining disturbance on soil edaphic factors and microbial community. Two different soil fissure states induced by mining activities significantly impacted the edaphic factors and the microbial community, especially in rare species.
The effects of soil fissure status on the microbial community have been thoroughly investigated in a representative mining disturbance area. Here, we presented a novel insight into soil microbial community characteristics and variations under distinct soil fissure states through multiple statistical and bioinformatic approaches. Distinct soil fissure states significantly impacted the edaphic factors, especially in soil moisture content and organic matter. Soil microbial communities showed different structures under the two different fissure states, and the soil moisture content, pH, particle compositions, organic matter, and heavy metals contributed to the variation of microbial communities. Rare species of soil microbial community were vulnerable to mining disturbance and played keystone roles in the soil microbial community (e.g., Nordella, Sphingomonas, Massilia, and Rubritepida). Our work highlighted the impacts of soil fissure states on soil physicochemical properties and microbial communities, and expanded our knowledge of the effects of mining activities on the edaphic factors and microbial communities. In addition, future works should pay more attention to microbial function and metabolism (e.g., metagenomics, metatranscriptomics) under mining-disturbed conditions.
The original contributions presented in the study are included in the article/Supplementary material, further inquiries can be directed to the corresponding authors.
LG: Formal analysis, Funding acquisition, Investigation, Methodology, Software, Visualization, Writing – original draft, Writing – review & editing. XC: Investigation, Software, Visualization, Writing – review & editing. YS: Conceptualization, Data curation, Methodology, Writing – review & editing. NY: Funding acquisition, Investigation, Methodology, Resources, Writing – review & editing. EH: Conceptualization, Funding acquisition, Methodology, Project administration, Writing – review & editing. HF: Software, Visualization, Writing – review & editing.
The author(s) declare that financial support was received for the research, authorship, and/or publication of this article. This work was financially supported by grants from the National Natural Science Foundation of China (42107101), the China Postdoctoral Science Foundation (2022MD723822), the Natural Science Basic Research Program of Shaanxi Province (2023-JC-QN-0317), and the Outstanding Youth Science Fund of Xi’an University of Science and Technology.
The authors sincerely thank Yizhi Sheng for the valuable discussion and Fu Liao, Shen Qu, Chenyu Wang, and Fengxia Liu for their assistance during the field investigation and sampling collection.
The authors declare that the research was conducted in the absence of any commercial or financial relationships that could be construed as a potential conflict of interest.
The author(s) declared that they were an editorial board member of Frontiers, at the time of submission. This had no impact on the peer review process and the final decision.
All claims expressed in this article are solely those of the authors and do not necessarily represent those of their affiliated organizations, or those of the publisher, the editors and the reviewers. Any product that may be evaluated in this article, or claim that may be made by its manufacturer, is not guaranteed or endorsed by the publisher.
The Supplementary material for this article can be found online at: https://www.frontiersin.org/articles/10.3389/fmicb.2024.1463665/full#supplementary-material
Banning, N. C., Lalor, B. M., Cookson, W. R., Grigg, A. H., and Murphy, D. V. (2012). Analysis of soil microbial community level physiological profiles in native and post-mining rehabilitation forest: which substrates discriminate? Appl. Soil Ecol. 56, 27–34. doi: 10.1016/j.apsoil.2012.01.009
Bi, Y., Zou, H., and Zhu, C. (2014). Dynamic monitoring of soil bulk density and infiltration rate during coal mining in sandy land with different vegetation. Int. J. Coal Sci. Technol. 1, 198–206. doi: 10.1007/s40789-014-0025-2
Caporaso, J. G., Kuczynski, J., Stombaugh, J., Bittinger, K., Bushman, F. D., Costello, E. K., et al. (2010). QIIME allows analysis of high-throughput community sequencing data. Nat. Methods 7, 335–336. doi: 10.1038/nmeth.f.303
Chapman, A. S. A., Tunnicliffe, V., and Bates, A. E. (2018). Both rare and common species make unique contributions to functional diversity in an ecosystem unaffected by human activities. Divers. Distrib. 24, 568–578. doi: 10.1111/ddi.12712
Cleveland, C. C., and Liptzin, D. (2007). C:N:P stoichiometry in soil: is there a “Redfield ratio” for the microbial biomass? Biogeochemistry 85, 235–252. doi: 10.1007/s10533-007-9132-0
Dai, S., Wen, T., Cai, Z., and Zhang, J. (2020). Dynamics of nitrite in acidic soil during extraction with potassium chloride studied using 15N tracing. Rapid Commun. Mass Spectrom. 34:e8746. doi: 10.1002/rcm.8746
de Quadros, P. D., Zhalnina, K., Davis-Richardson, A. G., Drew, J. C., Menezes, F. B., de Oliveira Camargo, F. A., et al. (2016). Coal mining practices reduce the microbial biomass, richness and diversity of soil. Appl. Soil Ecol. 98, 195–203. doi: 10.1016/j.apsoil.2015.10.016
Deng, Y., Jiang, Y. H., Yang, Y., He, Z., Luo, F., and Zhou, J. (2012). Molecular ecological network analyses. BMC Bioinformatics 13:113. doi: 10.1186/1471-2105-13-113
Deng, L., Wang, K. B., Chen, M. L., Shangguan, Z. P., and Sweeney, S. (2013). Soil organic carbon storage capacity positively related to forest succession on the Loess Plateau, China. Catena 110, 1–7. doi: 10.1016/j.catena.2013.06.016
Du, H., Wang, S., Nie, W., and Song, S. (2021). Soil properties and bacterial community dynamics in a coal mining subsidence area: active versus passive revegetation. J. Soil Sci. Plant Nutr. 21, 2573–2585. doi: 10.1007/s42729-021-00548-3
Edgar, R. C. (2013). UPARSE: highly accurate OTU sequences from microbial amplicon reads. Nat. Methods 10, 996–998. doi: 10.1038/nmeth.2604
Ezeokoli, O. T., Mashigo, S. K., Paterson, D. G., Bezuidenhout, C. C., and Adeleke, R. A. (2019). Microbial community structure and relationship with physicochemical properties of soil stockpiles in selected South African opencast coal mines. Soil Sci. Plant Nutr. 65, 332–341. doi: 10.1080/00380768.2019.1621667
Guo, L., Xie, Q., Sheng, Y., Wang, G., Jiang, W., Tong, X., et al. (2022). Co-variation of hydrochemistry, inorganic nitrogen, and microbial community composition along groundwater flowpath: a case study in Linzhou-Anyang area, Southern North China plain. Appl. Geochem. 140:105296. doi: 10.1016/j.apgeochem.2022.105296
Guo, J., Zhang, Y., Huang, H., and Yang, F. (2021). Deciphering soil bacterial community structure in subsidence area caused by underground coal mining in arid and semiarid area. Appl. Soil Ecol. 163:103916. doi: 10.1016/j.apsoil.2021.103916
Hou, E., Xie, X., Feng, D., Chen, Q., Che, X., and Hou, P. (2022). Laws and prevention methods of ground cracks in shallow coal seam mining. Coal Geol. Explor. 50, 30–40. doi: 10.12363/issn.1001-1986.22.05.0427 (in Chinese)
Huang, Y., Tian, F., Wang, Y., Wang, M., and Hu, Z. (2015). Effect of coal mining on vegetation disturbance and associated carbon loss. Environ. Earth Sci. 73, 2329–2342. doi: 10.1007/s12665-014-3584-z
Huang, X., Wang, G., Liang, X., Cui, L., Ma, L., and Xu, Q. (2018). Hydrochemical and stable isotope (δD and δ18O) characteristics of groundwater and hydrogeochemical processes in the Ningtiaota coalfield, Northwest China. Mine Water Environ. 37, 119–136. doi: 10.1007/s10230-017-0477-x
Jousset, A., Bienhold, C., Chatzinotas, A., Gallien, L., Gobet, A., Kurm, V., et al. (2017). Where less may be more: how the rare biosphere pulls ecosystems strings. ISME J. 11, 853–862. doi: 10.1038/ismej.2016.174
Kalembasa, S. J., and Jenkinson, D. S. (1973). A comparative study of titrimetric and gravimetric methods for the determination of organic carbon in soil. J. Sci. Food Agric. 24, 1085–1090. doi: 10.1002/jsfa.2740240910
Kang, H., Gao, F., Xu, G., and Ren, H. (2023). Mechanical behaviors of coal measures and ground control technologies for China’s deep coal mines—a review. J. Rock Mech. Geotech. Eng. 15, 37–65. doi: 10.1016/j.jrmge.2022.11.004
Karacan, C. Ö., and Goodman, G. (2009). Hydraulic conductivity changes and influencing factors in longwall overburden determined by slug tests in gob gas ventholes. Int. J. Rock Mech. Min. Sci. 46, 1162–1174. doi: 10.1016/j.ijrmms.2009.02.005
Kerou, M., Eloy Alves, R. J., and Schleper, C. (2016). “Nitrososphaeria” in Bergey’s manual of systematics of archaea and bacteria. eds. M. E. Trujillo, S. Dedysh, P. DeVos, B. Hedlund, P. Kämpfer, and F. A. Rainey, et al. (John Wiley & Sons, Inc.), 1–8.
Liang, Y., Xiao, X., Nuccio, E. E., Yuan, M., Zhang, N., Xue, K., et al. (2020). Differentiation strategies of soil rare and abundant microbial taxa in response to changing climatic regimes. Environ. Microbiol. 22, 1327–1340. doi: 10.1111/1462-2920.14945
Liu, H., Deng, K., Lei, S., and Bian, Z. (2015). Mechanism of formation of sliding ground fissure in loess hilly areas caused by underground mining. Int. J. Min. Sci. Technol. 25, 553–558. doi: 10.1016/j.ijmst.2015.05.006
Liu, H., Deng, K., Zhu, X., and Jiang, C. (2019). Effects of mining speed on the developmental features of mining-induced ground fissures. Bull. Eng. Geol. Environ. 78, 6297–6309. doi: 10.1007/s10064-019-01532-z
Liu, M., Han, X., Liu, C., Guo, L., Ding, H., and Lang, Y. (2021). Differences in the spectroscopic characteristics of wetland dissolved organic matter binding with Fe3+, Cu2+, Cd2+, Cr3+ and Zn2+. Sci. Total Environ. 800:149476. doi: 10.1016/j.scitotenv.2021.149476
Liu, C., Li, H., Zhang, Y., Si, D., and Chen, Q. (2016). Evolution of microbial community along with increasing solid concentration during high-solids anaerobic digestion of sewage sludge. Bioresour. Technol. 216, 87–94. doi: 10.1016/j.biortech.2016.05.048
Liu, Y., Sheng, Y., Feng, C., Chen, N., and Liu, T. (2020). Distinct functional microbial communities mediating the heterotrophic denitrification in response to the excessive Fe(II) stress in groundwater under wheat-rice stone and rock phosphate amendments. Environ. Res. 185:109391. doi: 10.1016/j.envres.2020.109391
Long, L., Liu, Y., Chen, X., Guo, J., Li, X., Guo, Y., et al. (2022). Analysis of spatial variability and influencing factors of soil nutrients in western China: a case study of the Daliuta mining area. Sustainability 14:2793. doi: 10.3390/su14052793
Lu, R. (1999). Soil agricultural chemical analysis. Nanjing, China: China Agricultural Science and Technology Press in Chinese.
Lu, M., Wang, X., Li, H., Jiao, J. J., Luo, X., Luo, M., et al. (2022). Microbial community assembly and co-occurrence relationship in sediments of the river-dominated estuary and the adjacent shelf in the wet season. Environ. Pollut. 308:119572. doi: 10.1016/j.envpol.2022.119572
Luo, Z., Ma, J., Chen, F., Li, X., Hou, H., and Zhang, S. (2019). Cracks reinforce the interactions among soil bacterial communities in the coal mining area of Loess Plateau, China. Int. J. Environ. Res. Public Health 16:4892. doi: 10.3390/ijerph16244892
Ma, X., Qu, H., Liao, S., Yuan, D., Yu, J., Li, J., et al. (2023). Changes in assembly processes and differential responses of soil microbial communities during mining disturbance in mining reclamation and surrounding grassland. Catena 231:107332. doi: 10.1016/j.catena.2023.107332
Ma, K., Zhang, Y., Ruan, M., Guo, J., and Chai, T. (2019). Land subsidence in a coal mining area reduced soil fertility and led to soil degradation in arid and semi-arid regions. Int. J. Environ. Res. Public Health 16:3929. doi: 10.3390/ijerph16203929
McLaughlin, M. J., Zarcinas, B. A., Stevens, D. P., and Cook, N. (2000). Soil testing for heavy metals. Commun. Soil Sci. Plant Anal. 31, 1661–1700. doi: 10.1080/00103620009370531
Ngugi, M. R., Dennis, P. G., Neldner, V. J., Doley, D., Fechner, N., and McElnea, A. (2018). Open-cut mining impacts on soil abiotic and bacterial community properties as shown by restoration chronosequence. Restor. Ecol. 26, 839–850. doi: 10.1111/rec.12631
Peng, X., Wang, X., Dai, Q., Ding, G., and Li, C. (2020). Soil structure and nutrient contents in underground fissures in a rock-mantled slope in the karst rocky desertification area. Environ. Earth Sci. 79:3. doi: 10.1007/s12665-019-8708-z
Poncelet, D. M., Cavender, N., Cutright, T. J., and Senko, J. M. (2014). An assessment of microbial communities associated with surface mining-disturbed overburden. Environ. Monit. Assess. 186, 1917–1929. doi: 10.1007/s10661-013-3505-8
Ponizovsky, A. A., Metzler, D. M., Allen, H. E., and Ackerman, A. J. (2006). The effect of moisture content on the release of organic matter and copper to soil solutions. Geoderma 135, 204–215. doi: 10.1016/j.geoderma.2005.12.004
Sheng, Y., Baars, O., Guo, D., Whitham, J., Srivastava, S., and Dong, H. (2023a). Mineral-bound trace metals as cofactors for anaerobic biological nitrogen fixation. Environ. Sci. Technol. 57, 7206–7216. doi: 10.1021/acs.est.3c01371
Sheng, Y., Bibby, K., Grettenberger, C., Kaley, B., Macalady, J. L., Wang, G., et al. (2016). Geochemical and temporal influences on the enrichment of acidophilic iron-oxidizing bacterial communities. Appl. Environ. Microbiol. 82, 3611–3621. doi: 10.1128/aem.00917-16
Sheng, Y., Dong, H., Coffin, E., Myrold, D., and Kleber, M. (2022). The important role of enzyme adsorbing capacity of soil minerals in regulating β-glucosidase activity. Geophys. Res. Lett. 49:e2021GL097556. doi: 10.1029/2021GL097556
Sheng, Y., Dong, H., Kukkadapu, R. K., Ni, S., Zeng, Q., Hu, J., et al. (2021a). Lignin-enhanced reduction of structural Fe(III) in nontronite: dual roles of lignin as electron shuttle and donor. Geochim. Cosmochim. Acta 307, 1–21. doi: 10.1016/j.gca.2021.05.037
Sheng, Y., Hu, J., Kukkadapu, R., Guo, D., Zeng, Q., and Dong, H. (2023b). Inhibition of extracellular enzyme activity by reactive oxygen species upon oxygenation of reduced iron-bearing minerals. Environ. Sci. Technol. 57, 3425–3433. doi: 10.1021/acs.est.2c09634
Sheng, Y., Li, G., Dong, H., Liu, Y., Ma, L., Yang, M., et al. (2021b). Distinct assembly processes shape bacterial communities along unsaturated, groundwater fluctuated, and saturated zones. Sci. Total Environ. 761:143303. doi: 10.1016/j.scitotenv.2020.143303
Sheng, Y., Liu, Y., Yang, J., Dong, H., Liu, B., Zhang, H., et al. (2021c). History of petroleum disturbance triggering the depth-resolved assembly process of microbial communities in the vadose zone. J. Hazard. Mater. 402:124060. doi: 10.1016/j.jhazmat.2020.124060
Shi, J., Tang, Z., Jin, Z., Chi, Q., He, B., and Jiang, G. (2003). Determination of As(III) and As(V) in soils using sequential extraction combined with flow injection hydride generation atomic fluorescence detection. Anal. Chim. Acta 477, 139–147. doi: 10.1016/S0003-2670(02)01402-2
Shi, P., Zhang, Y., Hu, Z., Ma, K., Wang, H., and Chai, T. (2017). The response of soil bacterial communities to mining subsidence in the west China aeolian sand area. Appl. Soil Ecol. 121, 1–10. doi: 10.1016/j.apsoil.2017.09.020
Tüzen, M. (2003). Determination of heavy metals in soil, mushroom and plant samples by atomic absorption spectrometry. Microchem. J. 74, 289–297. doi: 10.1016/S0026-265X(03)00035-3
Wang, Q., Garrity, G. M., Tiedje, J. M., and Cole, J. R. (2007). Naïve Bayesian classifier for rapid assignment of rRNA sequences into the new bacterial taxonomy. Appl. Environ. Microbiol. 73, 5261–5267. doi: 10.1128/AEM.00062-07
Wang, H., Liu, H., Yang, T., Lv, G., Li, W., Chen, Y., et al. (2023). Mechanisms underlying the succession of plant rhizosphere microbial community structure and function in an alpine open-pit coal mining disturbance zone. J. Environ. Manag. 325:116571. doi: 10.1016/j.jenvman.2022.116571
Wang, J., Wang, P., Qin, Q., and Wang, H. (2017). The effects of land subsidence and rehabilitation on soil hydraulic properties in a mining area in the Loess Plateau of China. Catena 159, 51–59. doi: 10.1016/j.catena.2017.08.001
Wang, Z., Wang, G., Wang, C., Wang, X., Li, M., and Ren, T. (2021). Effect of environmental factors on soil nutrient loss under conditions of mining disturbance in a coalfield. Forest 12:1370. doi: 10.3390/f12101370
Wang, G., Wu, M., Wang, R., Xu, H., and Song, X. (2017). Height of the mining-induced fractured zone above a coal face. Eng. Geol. 216, 140–152. doi: 10.1016/j.enggeo.2016.11.024
Xiao, L., Bi, Y., Du, S., Wang, Y., and Guo, C. (2019). Effects of re-vegetation type and arbuscular mycorrhizal fungal inoculation on soil enzyme activities and microbial biomass in coal mining subsidence areas of Northern China. Catena 177, 202–209. doi: 10.1016/j.catena.2019.02.019
Xiao, E., Ning, Z., Xiao, T., Sun, W., and Jiang, S. (2021a). Soil bacterial community functions and distribution after mining disturbance. Soil Biol. Biochem. 157:108232. doi: 10.1016/j.soilbio.2021.108232
Xiao, E., Wang, Y., Xiao, T., Sun, W., Deng, J., Jiang, S., et al. (2021b). Microbial community responses to land-use types and its ecological roles in mining area. Sci. Total Environ. 775:145753. doi: 10.1016/j.scitotenv.2021.145753
Xiao, W., Zhang, W., Ye, Y., Lv, X., and Yang, W. (2020). Is underground coal mining causing land degradation and significantly damaging ecosystems in semi-arid areas? A study from an ecological capital perspective. Land Degrad. Dev. 31, 1969–1989. doi: 10.1002/ldr.3570
Xin, Z., Qin, Y., and Yu, X. (2016). Spatial variability in soil organic carbon and its influencing factors in a hilly watershed of the Loess Plateau, China. Catena 137, 660–669. doi: 10.1016/j.catena.2015.01.028
Yang, X., Wen, G., Dai, L., Sun, H., and Li, X. (2019). Ground subsidence and surface cracks evolution from shallow-buried close-distance multi-seam mining: a case study in Bulianta coal mine. Rock Mech. Rock. Eng. 52, 2835–2852. doi: 10.1007/s00603-018-1726-4
Yuan, Q., Wang, P., Wang, X., Hu, B., Liu, S., and Ma, J. (2022). Abundant microbial communities act as more sensitive bio-indicators for ecological evaluation of copper mine contamination than rare taxa in river sediments. Environ. Pollut. 305:119310. doi: 10.1016/j.envpol.2022.119310
Zang, Y., Ding, G., and Gao, Y. (2012). Effects of coal mining subsidence on infiltration into unsaturated soils in sand drift area. Adv. Water Sci. 23, 757–768. in Chinese. doi: 10.14042/j.cnki.32.1309.2012.06.001
Zhang, K., Yang, K., Wu, X., Bai, L., Zhao, J., and Zheng, X. (2022). Effects of underground coal mining on soil spatial water content distribution and plant growth type in Northwest China. ACS Omega 7, 18688–18698. doi: 10.1021/acsomega.2c01369
Zhang, X., Zhu, A., Xin, X., Yang, W., Zhang, J., and Ding, S. (2018). Tillage and residue management for long-term wheat-maize cropping in the North China Plain: I. Crop yield and integrated soil fertility index. Field Crop Res. 221, 157–165. doi: 10.1016/j.fcr.2018.02.025
Keywords: microbial community, soil biodiversity, mining disturbance, soil fissure, co-occurrence network
Citation: Guo L, Chen X, Sheng Y, Yang N, Hou E and Fang H (2024) Impact of soil fissure status on microbial community in mining-disturbed area, the northern Shaanxi province. Front. Microbiol. 15:1463665. doi: 10.3389/fmicb.2024.1463665
Received: 12 July 2024; Accepted: 19 August 2024;
Published: 29 August 2024.
Edited by:
Anhuai Lu, Peking University, ChinaReviewed by:
Moushumi Hazra, University of Nebraska-Lincoln, United StatesCopyright © 2024 Guo, Chen, Sheng, Yang, Hou and Fang. This is an open-access article distributed under the terms of the Creative Commons Attribution License (CC BY). The use, distribution or reproduction in other forums is permitted, provided the original author(s) and the copyright owner(s) are credited and that the original publication in this journal is cited, in accordance with accepted academic practice. No use, distribution or reproduction is permitted which does not comply with these terms.
*Correspondence: Nuan Yang, eWFuZ251YW5AeHVzdC5lZHUuY24=; Enke Hou, aG91ZWtAeHVzdC5lZHUuY24=
Disclaimer: All claims expressed in this article are solely those of the authors and do not necessarily represent those of their affiliated organizations, or those of the publisher, the editors and the reviewers. Any product that may be evaluated in this article or claim that may be made by its manufacturer is not guaranteed or endorsed by the publisher.
Research integrity at Frontiers
Learn more about the work of our research integrity team to safeguard the quality of each article we publish.