- 1Department of Food Hygiene and Environmental Health, University of Helsinki, Helsinki, Finland
- 2Medical Services, Defence Command, The Finnish Defence Forces, Helsinki, Finland
- 3Microbiology Unit, Finnish Food Authority, Seinäjoki, Finland
- 4Retired, Turku, Finland
Clostridium perfringens is a causative agent of various human and animal enteric diseases including food poisoning. In this study, we describe an interesting case of a persistent food poisoning outbreak among Finnish peacekeepers in Eritrea, possibly caused by Clostridium perfringens carrying a new variant of the chromosomally encoded enterotoxin gene. C. perfringens strains causing food poisoning carry the enterotoxin gene, cpe, in its chromosome (c-cpe) or on a plasmid (p-cpe). PCR assays are widely used for toxinotype C. perfringens strains. The integration sites for the cpe gene are highly conserved, and PCR assays targeting the cpe gene and the adjacent IS elements (the IS1470 in c-cpe and the IS1470-like or IS1151 in p-cpe strains) are used to further determine the genetic location of the cpe gene. We sequenced nine enteropathogenic C. perfringens strains related to a persistent food poisoning outbreak among Finnish peacekeepers in Eritrea. Six of these strains produced non-typeable cpe results in the standard PCR assay due to divergence in the enterotoxin integration site. The gene order of the new variant of the chromosomal cpe insertion site with an additional IS1470 element impairing genotyping PCR assay for the location of cpe is described. In addition, variant c-cpe strains carried 58–81 copies of IS1470 in their genomes, compared to 9–23 copies found in previously described c-cpe strains. Thus, the present study represents an untraditional type of C. perfringens food poisoning caused by variant c-cpe strains, and the sequenced strains bring geographic variation to the existing strain collection of sequenced C. perfringens.
1 Introduction
Clostridium perfringens is an anaerobic, spore former causing gas gangrene, wound infections, and a variety of human and animal diseases involving the gastrointestinal (GI) system (Lindström et al., 2011; Kiu and Hall, 2018). Traditional C. perfringens food poisoning outbreaks are associated with temperature-abused food and typically affect many people at the same time. In addition, cases of antibiotic-associated diarrhea (AAD) by C. perfringens may be transmitted through food (Lindström et al., 2011).
Both food poisoning and AAD cases of C. perfringens diarrhea are primarily caused by type F strains (strains carrying enterotoxin gene cpe), which produce pore-forming C. perfringens enterotoxin (CPE). The prevailing understanding is that all C. perfringens strains may carry the cpe gene, but only approximately 5% do and produce CPE (Miyamoto et al., 2006). The cpe allele encoding a 319 aa end product is located either in pCPF5603 or pCPF4969 plasmid (plasmid-mediated cpe, p-cpe strains) or in a transposable element Tn5565 integrated into the chromosome (chromosomal cpe, c-cpe strains) (Cornillot et al., 1995; Brynestad et al., 1997; Miyamoto et al., 2006; Li et al., 2010). The Tn5565 includes insertion sequences (IS) IS1470 and IS1469 directly adjacent to the cpe gene (Brynestad et al., 1997). Known cpe-carrying plasmids are all conjugative and horizontally transferable (Miyamoto et al., 2006). The plasmid cpe gene is adjacent to IS1151- or IS1470-like elements (Daube et al., 1993; Miyamoto et al., 2002).
Foodborne outbreaks are caused by both p-cpe and c-cpe strains (Lahti et al., 2008), but multiple studies have shown that most outbreaks are caused by chromosomal cpe strains. In addition, a 325 aa variant of the cpe gene of unknown clinical relevance has been described in the pCPBB1 plasmid (Miyamoto et al., 2011). Persistent longitudinal outbreaks of C. perfringens have been suggested in some studies (Kiu et al., 2019), and these outbreaks have been associated with plasmidial cpe-carrying strains.
Six toxin genes detected by PCR have been widely used for toxinotype strains of types A–G (Rood et al., 2018). The only invariably chromosomal gene in the toxinotyping scheme is alpha (plc), which is present in all C. perfringens strains, while the other toxinotyping toxins are carried on transposable elements or a family of conjugative plasmids (Li et al., 2013). Toxinotyping, therefore, does not reflect the phylogenetic lineage of strains. To address this, the availability of genomic sequences has led to the establishment of genetic lineages (Kiu et al., 2017; Feng et al., 2020; Jaakkola et al., 2021), and a virulence gene profile scheme including chromosomal genes has been proposed (Abdelrahim et al., 2019).
PCR genotyping is also used to detect the location and type of enterotoxin gene in enteropathogenic C. perfringens. Despite the transmissible nature of these genetic structures, the gene order on both chromosomal and plasmidial integration sites is so highly conserved that IS elements are utilized as PCR probe targets (Brynestad, 1997), and reported variant strains have been related to the presence of a larger variant of the enterotoxin gene itself (325 aa) (Heikinheimo et al., 2006; Li et al., 2007).
In this study, we describe a persistent food poisoning outbreak putatively caused by Eritrean C. perfringens strains. In addition, we describe six outbreak strains with chromosomal cpe that exhibit an additional IS1470 insertion sequence near the cpe gene, which affects the ability of the PCR assay to detect the cpe location and genotyping results.
2 Materials and methods
2.1 Outbreak and stool samples
The occurrence of gastroenteritis among Finnish peacekeepers deployed in the United Nations peacekeeping operation in Eritrea in Africa (UNMEE) increased during the summer and autumn of 2004. In the autumn of 2004, an investigation team from Finland was sent to Eritrea to investigate the possible outbreak, take measures, and give instructions to address the situation. Inspections of the camp food premises, water distribution system, and local water suppliers were conducted. Water samples were collected and analyzed for the total bacterial count, total coliforms, E. coli, parasites (Giardia, Cryptosporidium, and Entamoeba histolytica), and noroviruses using the standard drinking water methods. No E. coli, parasites, or noroviruses were detected in any of the 13 samples.
Of the 184 peacekeepers, 163 (91.3%) answered the epidemiological questionnaire and 98 (60.1%) of them had suffered from symptoms such as diarrhea (93.9%), flatulence (69.4%), abdominal pain (58.2%), nausea without vomiting (51.5%), lack of appetite (43.9%), abdominal distention (33.7%), fever (31.6%), vomiting (22.4%), muscular pain (17.3%), abdominal cramps and other symptoms (16.3%), and bloody diarrhea (7.1%). The illnesses occurred consistently from June to October, with no distinct peak in their occurrence. The analysis of questionnaires and medical reports revealed that on average, approximately five peacekeepers were sick each day from June to October.
Stool specimens were collected from 184 Finnish peacekeepers (sample numbers 1–184) and 38 local Eritrean staff (cooks and cleaners) members (sample numbers 200–237) and transported to the Laboratory of Helsinki University Central Hospital. Salmonella, Shigella, Yersinia, and Campylobacter, parasites (Cryptosporidium, Cyclospora, Cystoisospora, and Dientamoeba), norovirus and astrovirus, and antigens for Giardia, Cryptosporidium, and Entamoeba histolytica were investigated from the stool samples using standardized methods of the laboratory. The epidemiological and laboratory investigations revealed no specific bacteria, virus, or parasite connected to the illnesses. However, the symptoms resembled those of Clostridium perfringens food poisoning, and the fecal samples were taken for further examination.
2.2 Detection and isolation of Clostridium perfringens
Each fecal swab sample was dissolved into a tube that contained sterilized water, and the hydrophobic grid membrane filter-colony hybridization (HGMF-CH) (Heikinheimo et al., 2004) was used to detect and isolate cpe-carrying C. perfringens from the samples. The samples giving a positive signal in the HGMF-CH assay were considered positive for cpe-carrying C. perfringens. The probe-positive colonies were isolated from each sample to obtain cpe-carrying C. perfringens from a single sample and to further study the genetic relatedness of these isolates.
2.3 PCR and PFGE typing
We detected major toxins and cpe in the C. perfringens isolates with PCR, as previously described by Heikinheimo and Korkeala (2005) in 2004–2005. The current nomenclature was used in this article (Rood et al., 2018). C. perfringens strains NCTC 8239, ATCC 3626, CCUG 2036, CCUG 2037, and CCUG 44727 were used as positive controls. C. perfringens type F isolates were further studied by PCR to determine the cpe genotype based on the cpe insertion site with IS elements. The total DNA was isolated by using Advamax beads (Edge Biosystems, Gaithersburg, MD, USA), according to the manufacturer’s instructions. IS elements downstream of cpe that determine the cpe genotype (IS1151, IS1470-like, or IS1470) of each isolate were characterized by using PCR with the previously described primers (Daube et al., 1993; Brynestad, 1997; Brynestad et al., 1997; Miyamoto et al., 2002; Miyamoto et al., 2004) and protocols (Heikinheimo et al., 2006).
In pulsed-field gel electrophoresis (PFGE) analysis, the DNA was digested with ApaI (New England Biolabs, Beverly, MA, USA), and the genetic relationships between isolates were assessed using the previously described assay (Ridell et al., 1998), which was modified by adding thiourea to the electrophoresis running buffer (Leclair et al., 2006). The digital images of PFGE patterns were analyzed using Bionumerics software (version 4.6, Applied Maths, Sint-Martens-Latem, Belgium), and the similarity analysis of PFGE patterns was performed using the Dice coefficient (optimized 2%, tolerance 1.2%). Clustering and construction of dendrograms were performed by using the unweighted pair-group method with arithmetic averages.
2.4 Sequencing of selected Clostridium perfringens isolates and annotation
Nine strains were sequenced in 2022 and have been deposited in the GenBank. These nine strains were selected for sequencing based on the genotyping results, and they represented both chromosomal and variant chromosomal cpe-carrying isolates in the genotyping assay.
Genomic DNA of C. perfringens isolates was extracted, as described by Keto-Timonen et al. (2006), and whole-genome sequencing was performed using PacBio RSII (Institute of Biotechnology, Helsinki, Finland). Sequenced genomes were assembled using HGAP3 and checked for circularity using Gap4 (Staden et al., 2003; Chin et al., 2013). To improve the draft assembly, Illumina MiSeq reads and the Pilon tool were used for genome polishing (Walker et al., 2014). Both sequenced and downloaded genomes were annotated using Prokka (Seemann, 2014).
Selected reference strains (ATCC 13124, SM101, and Str. 13) and previously sequenced lineage IV strains were included in the cgMLST analysis (Supplementary Table S1). Genomes were downloaded from the Bacterial and Viral Bioinformatics Resource Center.1
2.5 Comparative genome analysis
Bacterial and Viral Bioinformatics Resource Center (see text footnote 1) was used to perform comparative genome analysis and to combine results with previous studies (Jaakkola et al., 2021). To determine the cgMLST target gene set and create a genome-wide gene-by-gene comparison, ChewBBACA (3.0.0) was used (Silva et al., 2018). A schema created by Abdel-Glil et al. (2021) available at https://www.cgmlst.org was used. The core genome of 63 C. perfringens genomes consisted of 1,236 genes. For cgMLST results, a minimal spanning tree was calculated by GrapeTree (Zhou et al., 2018) using MSTreeV2. Strains (n = 63) included in the comparative genome analysis are listed in Supplementary Table S1.
Sequenced genomes were queried for the presence of IS elements (IS1470, IS1469, and IS1151) and selected genetic features (Supplementary Table S2). For IS elements, the hits with >80% identity over 80% of length were considered a match. For other genetic features, an identity threshold of 90% was used.
2.6 Phylogenetic analysis
Single-nucleotide polymorphisms between sequenced strains and selected reference strain genomes were identified using Snippy 4.6. (Seemann et al., 2015), with strain SM101 (289380.15) as a reference. A phylogeny based on core-SNP alignment was created by IQ-TREE 2.3.0. (Nguyen et al., 2015). Bootstrap values for branches were approximated using ultrafast bootstrapping (-B 1000), and FigTree v1.4.4. was used to visualize the trees (Rambaut and Drummond, 2008). Strains (n = 63) included in phylogenetic analysis are listed in Supplementary Table S1.
3 Results
3.1 cpe-positive Clostridium perfringens samples
Altogether, 50 of 222 (22.5%) human stool samples gave a positive signal in the HGMF-CH and were considered positive for the presence of cpe-carrying C. perfringens. The prevalence of type F C. perfringens differed between the samples from peacekeepers and local staff members. Overall, 32 of 184 (17.4%) of the samples of the peacekeepers and 18 of 38 (47.3%) of the samples of local staff members were positive. Altogether, we isolated 96 C. perfringens isolates from 12 samples (Table 1). The isolates were regarded as cpe-positive since they gave a signal in the HGMF-CH.
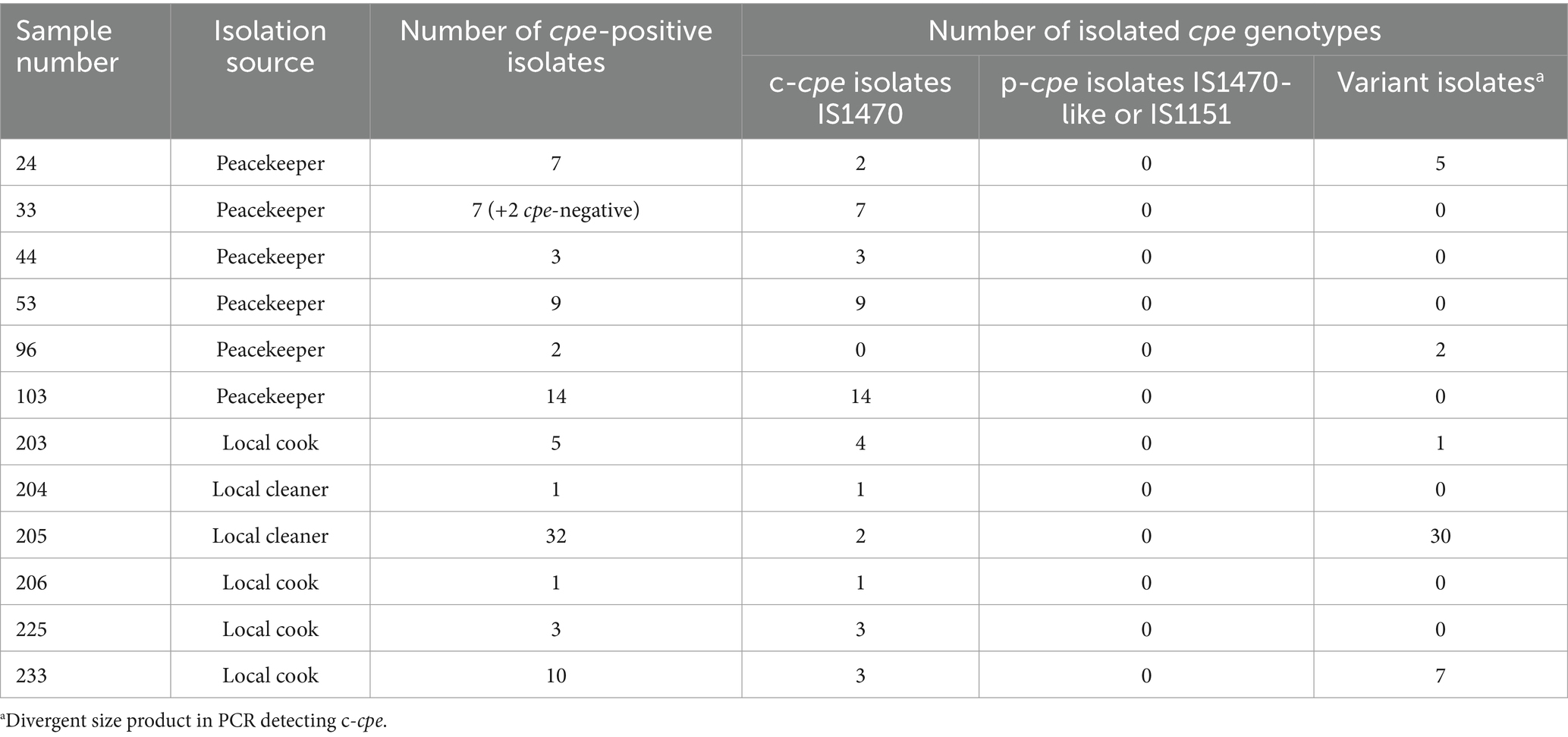
Table 1. cpe-positive samples yielded cpe-positive isolates, the isolation source of the samples, the number of cpe-positive isolates, and their cpe type (chromosomal/plasmidial).
Of the peacekeepers who gave cpe-positive or cpe-negative samples, 72 and 59%, respectively, had symptoms of intestinal disease between June and October 2004.
3.2 Genotyping results
Based on multiplex PCR, 94 (98%) of the 96 isolates were cpe-carrying type F (former type A). None of the 94 cpe-carrying isolates carried plasmidial cpe. PCR genotyping identified 49 (52%) of the 94 isolates as typical c-cpe isolates. The remaining 45 (48%) of the 94 isolates yielded PCR products of divergent size compared to c-cpe; thus, the genetic location of the cpe gene of these 45 isolates was interpreted as a variant. The PCR results are shown in Table 1.
We typed 78 (83%) of 94 cpe-positive isolates with PFGE. Isolates from four peacekeepers (samples 33, 44, 53, and 103) displayed four distinct patterns (Figure 1). Isolates from a fifth peacekeeper (sample 24) and four locally employed workers (203, 205, 206, and 233) displayed closely related patterns and included both c-cpe and variant isolates. Among this cluster, a subcluster of indistinguishable isolates from the fifth peacekeeper and three local staff members was selected for further analysis.
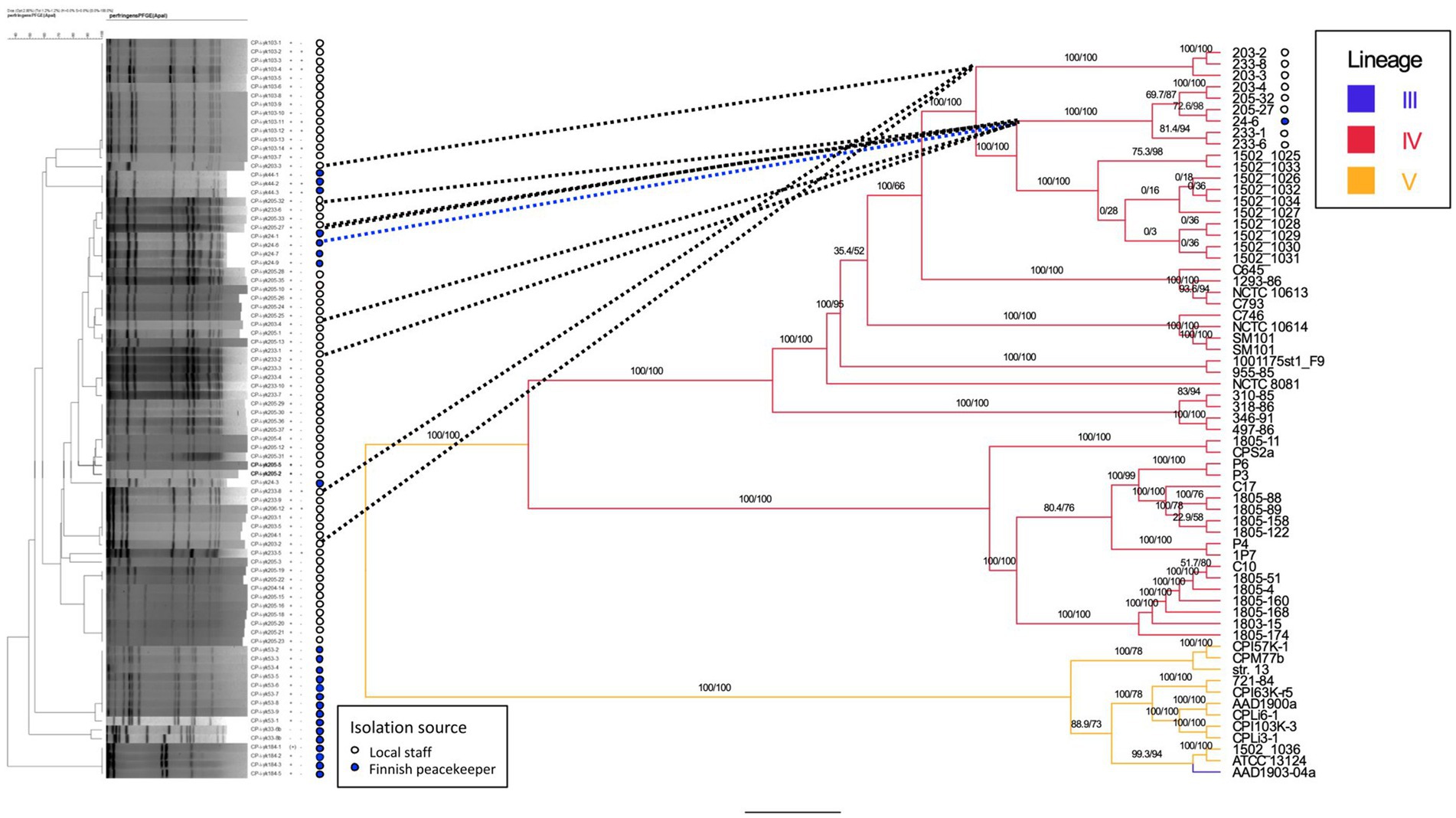
Figure 1. Phylogenetic tree of sequenced strains and previously published strains. Comparison is made between the dendrogram (Dice coefficient with 2%, UPGMA clustering, 84 isolates) of PFGE results (left) and the phylogenetic tree (IQ-TREE 2.3.0, visualized by FigTree v1.4.4., 63 isolates) based on whole-genome sequences (center, right). For the strains isolated in this study, the isolation source (local staff, peacekeeper) is annotated next to the PFGE tree, and bootstrapping values for tree nodes are given. For sequenced genomes and previously published genomes, the genetic lineages (III, IV, and V) of strains are indicated on the right. Nomenclature of strains: CP-i-yk was used as the study ID in the PFGE analysis. The numbers and hyphen (4–6 characters) following the study ID constitute the strain/genome ID. Reference strain = C. perfringens strain SM101.
3.3 Sequenced genomes
Nine isolates representing both c-cpe typed isolates and PCR variant cpe isolates were sequenced (Table 2). Sequencing revealed that all carried a chromosomally inserted cpe and they, despite the varied PCR results and the differences observed in PFGE, belonged to the same phylogenetic lineage IV and phylogenetically clustered together with c-cpe food poisoning isolates (Figure 2). Different c-cpe groups, 1 and 2, have been recently suggested (Jaakkola et al., 2021). C-cpe group 1 strains seem to be equipped for changing pH and acidic, high-temperature environments where iron uptake is competitive, and citrate utilization is beneficial, whereas strains of c-cpe group 2 lack these genes and operons. The isolates described here belonged to c-cpe group 1, among many well-researched food poisoning strains such as SM101, NCTC 8239, and NCTC 10613.
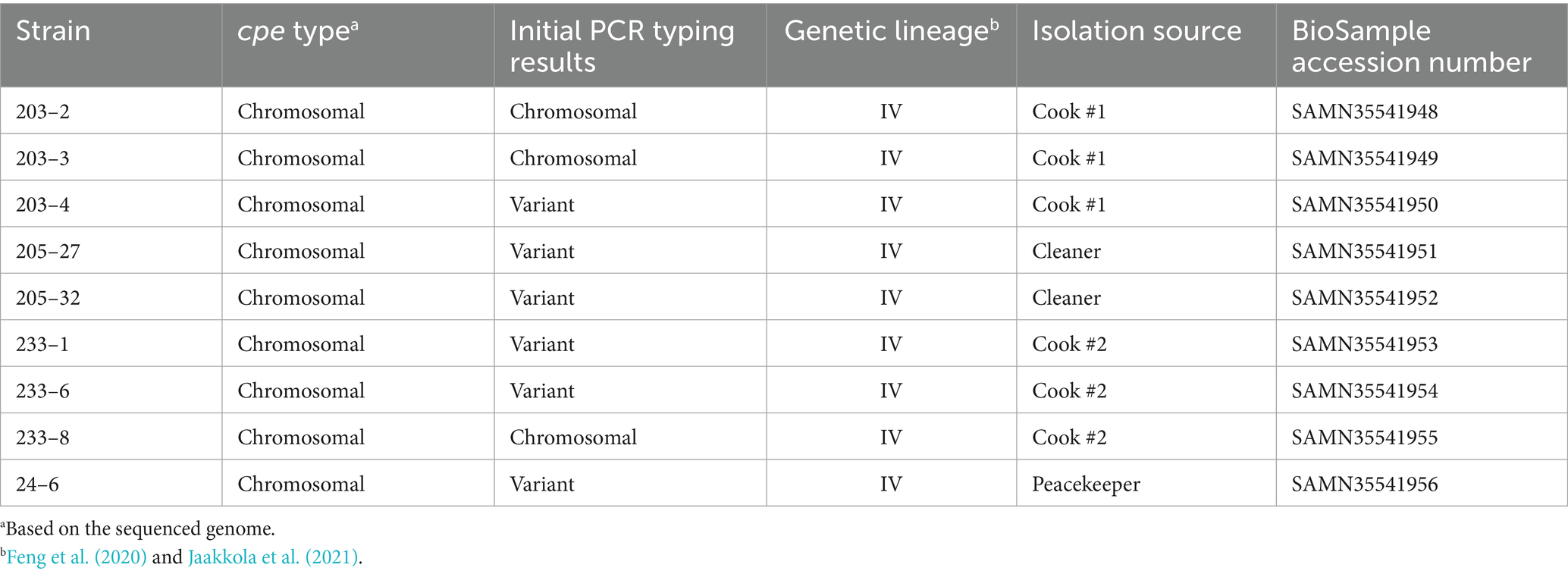
Table 2. Sequenced C. perfringens strains, their cpe type (chromosomal/plasmidial), initial PCR typing results, genetic lineage, isolation source, and BioSample accession number.
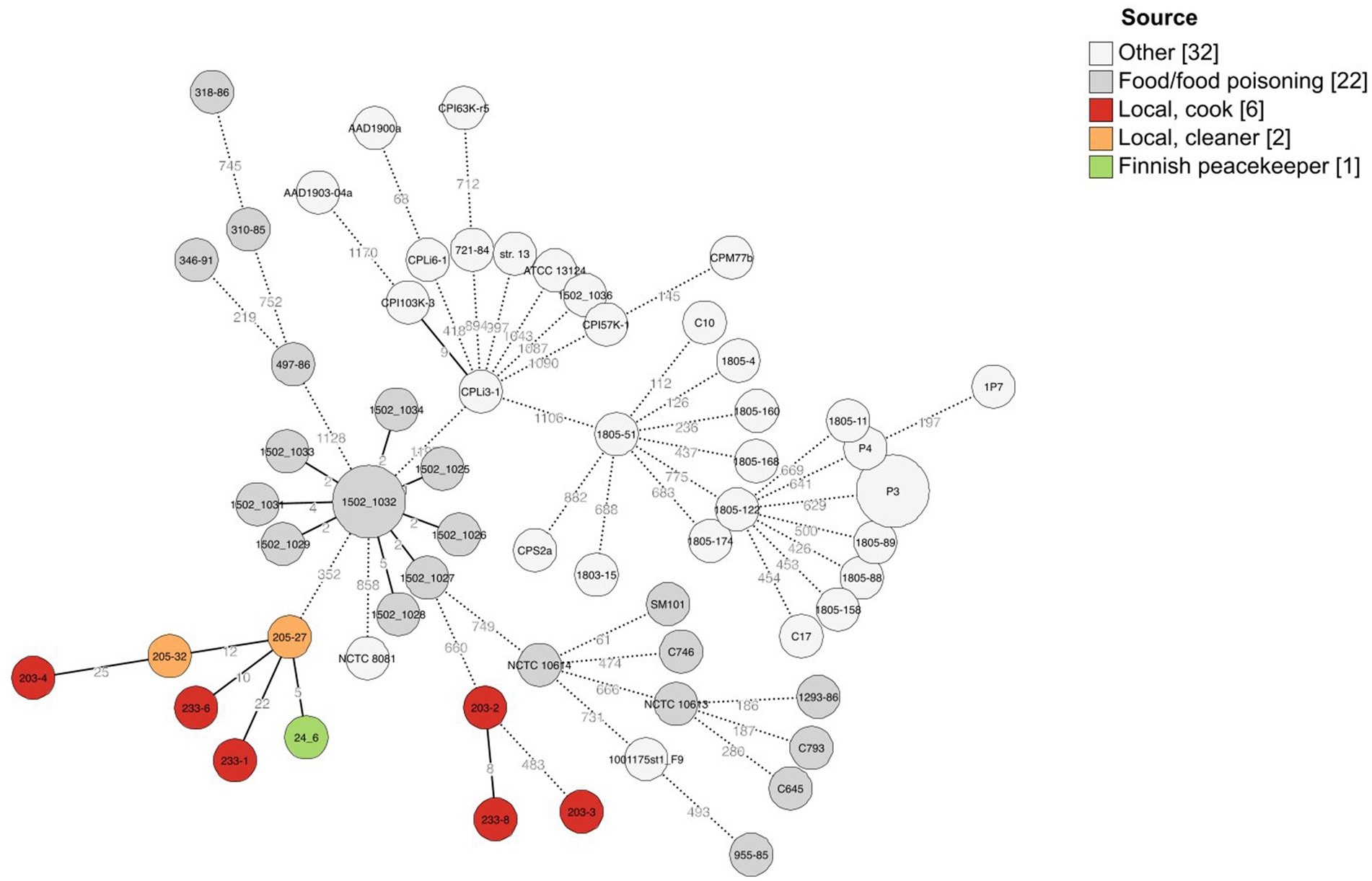
Figure 2. Minimal spanning tree of cgMLST results of 63 C. perfringens strains including here sequenced strains (n = 9) and previously published C. perfringens strains (n = 54). A scheme created by Abdel-Glil et al. (2021) was used for allele calling, and 1,236 loci belonging to the core genome were used to create the minimal spanning tree with GrapeTree (MSTreeV2). The isolation sources of strains have been added as a colored annotation, and branches below two allelic differences are collapsed.
All sequenced cpe genes were highly conserved and encoded a 319 aa enterotoxin sequence. Genome analysis revealed that the six strains in Table 2 with variant cpe PCR results (24–6, 203–4, 205–27, 205–32, 233–1, and 233–6) carried an additional IS1470 sequence directly downstream the cpe gene (Figure 3), while the genomes typed as chromosomal in PCR (Table 2) had a typical gene order and genetic composition, as described by Miyamoto et al. (2006) around their chromosomally inserted cpe gene. For the sake of brevity, these six genes with new gene order for their cpe insertion site are called “variant c-cpe strains” in this article.
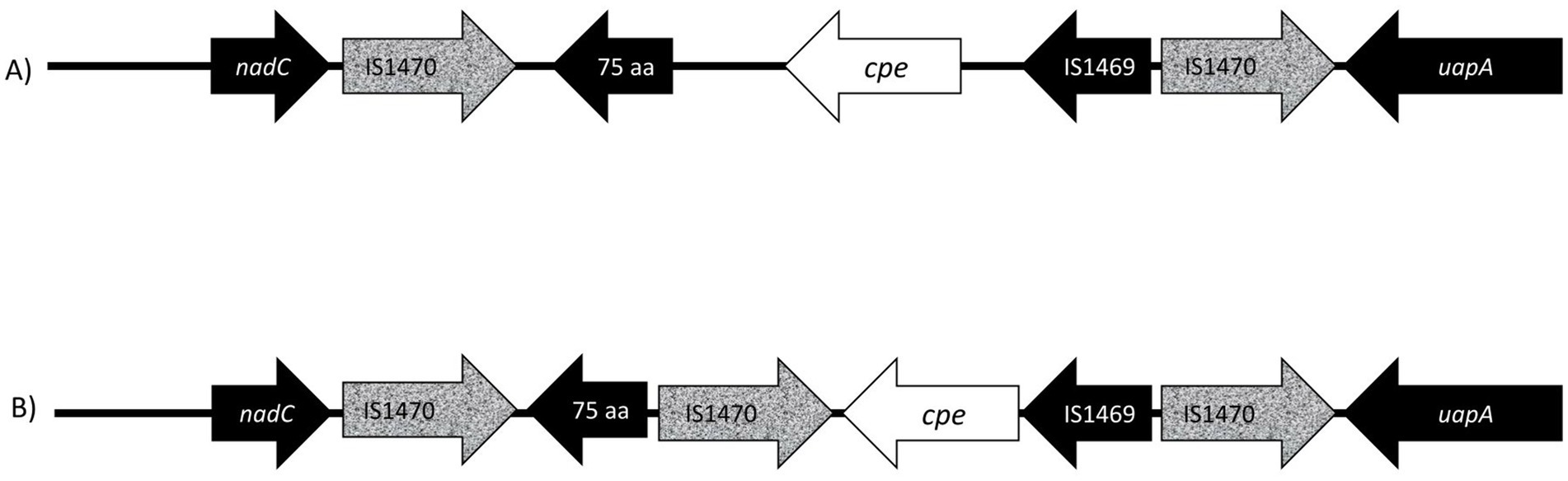
Figure 3. Presentation of gene order on chromosomal insertion sites of enterotoxin gene (cpe). (A) The well-described and conserved gene order (Brynestad et al., 1997) is commonly utilized for PCR genotyping, and probes target the IS1470 element downstream of the cpe gene and the cpe gene itself. In this study, six isolates (233–6, 233–1, 205–27, 203–4, 205–32, and 24–6) produced variant PCR results in PCR genotyping and had a new variant cpe loci (B) with a 1,053 aa IS1470 insertion element directly downstream of the cpe gene. The additional IS1470 element was 99% identical to the other, well-described IS1470 in the close vicinity of the cpe gene in chromosomal strains.
The additional IS1470 element in strains with variant gene order was 99% identical with the other, well-described IS1470 in the close vicinity of the cpe gene in chromosomal strains. Further analysis of IS1470 revealed that the variant c-cpe strains such as 24–6, 203–4, 205–27, 205–32, 233–6, and 233–1 had also accumulated other additional copies of IS1470 elements (Table 3). Variant c-cpe strains carried 58 to 81 copies of IS1470 in their genomes, while the majority of studied chromosomal strains carried 9 to 23 copies of IS1470. P-cpe strains carried under 10 copies of IS1470 (0 to 7) (Supplementary Table S2). All c-cpe strains carried 1 copy of IS1469 (Supplementary Table S2).
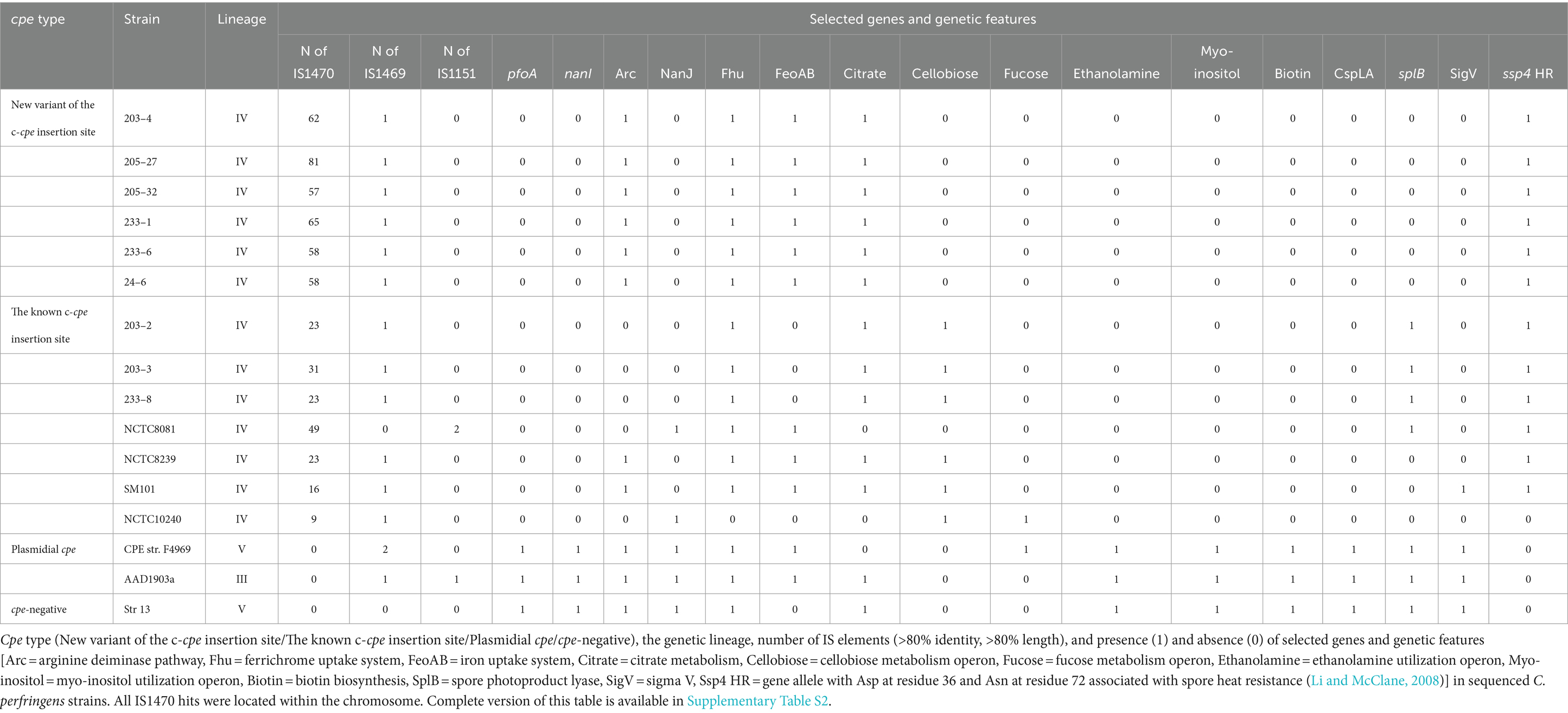
Table 3. Comparison of selected genetic features of the c-cpe strains sequenced in this study and some well-known C. perfringens strains.
The traditional and variant c-cpe strains sequenced in this study had slight differences when selected genetic features were compared (Table 3). The traditional c-cpe strains lacked the arginine deiminase pathway Arc and the iron uptake system FeoAB, which were present in variant c-cpe strains. The traditional c-cpe strains sequenced in this study carried cellobiose metabolism operon and spore photoproduct lyase SplB, which were absent in the variant c-cpe strains. Sequenced strains were subjected to cgMLST analysis to characterize the strains and shed light on their epidemiological context. Analyzed C. perfringens strains (Supplementary Table S1) shared 1,236 genes in their core genome. Strains 24–6, 203–4, 205–27, 205–32, 233–1, and 233–6 formed a clade and shared highly similar cgMLST gene profiles (5 to 25 differences), suggesting genetic relatedness and a recent shared origin (Figure 1). These strains had been isolated from a peacekeeper (24–6), two local kitchen staff members (Cook #1203: 203–4 and Cook #2233: 233–1 and 233–6), and a local cleaner (205–27 and 205–32). Strains 203–2, 203–3, and 233–8 shared another clade with a genetic difference of 8 between 203–2 and 233–8 (Figure 2).
4 Discussion
In the present study, we found C. perfringens type F strains in the stool samples of peacekeepers and locally employed workers. Among the samples from locally employed workers and peacekeepers, 47 and 17%, respectively, tested positive for cpe-carrying C. perfringens. Altogether, 94 c-cpe strains were isolated from 12 people, including clinically healthy individuals (the locally employed workers and some peacekeepers) and those who were ill or recovering from intestinal disease (some peacekeepers). The isolation of c-cpe strains from multiple people suggests that there may have been one or more C. perfringens food poisoning outbreaks among peacekeepers with symptoms of disease as c-cpe strains are known to be a common cause of food poisoning (Lindström et al., 2011). However, since the sampling was conducted in October and the symptoms of the peacekeepers had occurred between June and October, CPE or large numbers of type F isolates were not found in the feces of affected people, and we could not definitively identify these symptoms being caused by C. perfringens type F. The local workers were not included in the epidemiological questionnaire or systematically interviewed, so it is unclear whether they had some symptoms of intestinal disease at the same time period as the peacekeepers. Interestingly, we obtained only c-cpe isolates, especially among locally employed workers. P-cpe strains are often present in the normal intestinal microbiota of many healthy people (Heikinheimo et al., 2006; Carman et al., 2008). Noteworthy, the isolation of C. perfringens strains was not successful from all cpe-positive samples, and whether p-cpe or c-cpe strains were present in these samples remains unknown.
In the present study, four peacekeepers had individual c-cpe strains according to the PFGE results. Those c-cpe strains were not found in anyone else. Based on the questionnaire, at least two of these peacekeepers had the symptoms of intestinal disease 1–2 months earlier, but not at the time of sampling. Moreover, one peacekeeper shared the same c-cpe strain with two local cooks and one local cleaner. According to the questionnaire, this peacekeeper had symptoms of intestinal disease 3 weeks earlier but not at the time of sampling. This may indicate that the peacekeepers had become carriers of the c-cpe strains after food poisoning.
While there is no clear evidence that c-cpe strains have been the cause of symptoms observed among the peacekeepers, the fact that we found genetically similar C. perfringens strains in samples from the locally employed workers and peacekeepers suggests that transmission of these strains has occurred among camp crew. The transmission has most likely been foodborne as this is the most common route of transmission for intestinal bacteria (Browne et al., 2017). This is further supported by the presence of the same strains in the kitchen staff. The high number of c-cpe C. perfringens among the local workers may even suggest humans as a reservoir of the c-cpe strains, and the persistence of c-cpe in peacekeepers several months after symptoms may even suggest that humans might become at least a transient reservoir of c-cpe strains in some cases. This subject needs to be further elucidated for better prevention of epidemics in the future. Reservoirs of the c-cpe strains are unclear, but humans as reservoirs have also been suggested previously (Heikinheimo et al., 2006).
In a previous study (Kiu et al., 2019), genetically highly similar p-cpe C. perfringens strains were associated with nine distinct care-home-associated outbreaks throughout a 5-year interval. Kiu et al. (2019) suggested that there was a common source linked to these outbreaks or transmission over time and space. Previous studies (Lahti et al., 2012; Jaakkola et al., 2021) have suggested that p-cpe strains and some c-cpe strains inhabit human intestines, so humans as carriers of food poisoning strains over time and space are possible. Since peacekeepers had symptoms evenly distributed during summer and autumn in 2004, it is possible that there had been a persistent food poisoning outbreak caused by the c-cpe strains carried by the kitchen workers.
An alternative explanation is that contaminated food ingredients have introduced the C. perfringens strains to the camp, where camp conditions may have facilitated the transmission of c-cpe strains among food consumers. C-cpe strains are known to enter the food chain and have been identified as the sole or predominant cpe-positive strains in retail meats from both the United States (Wen and McClane, 2004) and Turkey (Yibar et al., 2018). However, it is unclear how the c-cpe strains end up in the food chain, from food handlers or other sources. In the present study, the camp’s food premises were adequate when inspected, and the UN procured food from international operators known for their high standards of food safety.
In the present study, the higher proportion of the c-cpe C. perfringens among the local workers than among the peacekeepers may also indicate a specific local source of c-cpe C. perfringens. The high numbers of these pathogenic isolates in the stool of one local cleaner (32 isolates) and a local cook (10 isolates) support this. The exposure to local foods outside the camp may have increased the risk of C. perfringens food poisoning.
The cgMLST scheme for C. perfringens has been used to type strains in several studies, and allele differences between these variable species are usually relatively large, with differences ranging from 200 to 1,000 alleles between strains (Abdel-Glil et al., 2021; Jaakkola et al., 2021). The allele differences between these sequenced strains varied between 5 and 25, and they formed a distinct cluster, supported by a separate clade in phylogenetic analysis (Figure 2). The differences between epidemiologically linked isolates are usually below 10 alleles in bacterial species (Schürch et al., 2018), but the cluster thresholds are dependent on the sequencing method and bacterial species, and larger cluster thresholds have been suggested for C. perfringens and its highly variable genome (Abdel-Glil et al., 2021). We suggested that the isolation of strains with less than 50 allele differences from a diverse group of people working on the same campsite suggests recent genetic relatedness, and considering the associated symptoms of gastrointestinal disease, there is a possible persistent outbreak among the residents at the camp. It is also possible that the chosen sequencing method has introduced some observed differences between the isolates.
The cpe insertion sites in the C. perfringens are well-described and conserved; therefore, they are widely used for strain typing. In our PCR typing, 45 of 94 c-cpe isolates were variants, and the sequencing revealed that the sequenced strains with variant results had an additional IS1470 element inserted directly downstream of the cpe gene. Variation in cpe loci arrangements has been previously reported by Abdelrahim et al. (2019), but this is the first time a cpe loci arrangement has been reported to affect PCR typing. Furthermore, the additional IS1470 element within cpe loci observed here has not been previously described.
The PCR genotyping probes in the C. perfringens assay target the IS1470 element downstream of the cpe gene and the cpe gene itself, and the presence of an additional IS1470 element downstream of the cpe gene impaired the standardized genotyping PCR assay. These variant c-cpe strains could be misidentified as strains with unknown cpe locations. Due to the variant c-cpe, the genotyping PCR primers need to be redesigned to detect reliably the variant c-cpe as well.
The traditional and variant c-cpe strains sequenced in this study all belonged to c-cpe group 1, which tolerate changing pH and acidic environments (Jaakkola et al., 2021). Interestingly, the variant c-cpe strains and traditional c-cpe strains had slight differences when selected genetic features were compared (Table 3). The variant c-cpe strains carried the arginine deiminase pathway Arc and the iron uptake system FeoAB, which were absent in traditional c-cpe strains and lacked the cellobiose metabolism operon, which was present in the traditional c-cpe strains. These possible differences in the metabolism between the c-cpe types may indicate that the variant c-cpe strains are better equipped for harsh conditions.
The variant c-cpe strains with an additional IS1470 element downstream of the cpe gene had a higher number of IS1470 elements in their entire genome compared to other C. perfringens strains. IS1470 element has been reported on chromosomes, never plasmids, and generally in moderate numbers (0–10 copies) (Brynestad et al., 1994). However, IS1470-like sequences are common on C. perfringens plasmids (Miyamoto et al., 2004). IS elements together with other transposable elements are important mutagenic agents enabling the host to adapt to new environmental challenges and colonize new niches. IS expansion has been linked to genome rearrangements, genome size reduction, and gene inactivation characteristic of the emergence of pathogenic strains (Parkhill et al., 2003; Siguier et al., 2006; Vandecraen et al., 2017), with famous examples of Yersinia pseudotuberculosis and Yersinia pestis (Parkhill et al., 2001; Chain et al., 2004), Bordetella bronchiseptica, and Bordetella pertussis (Parkhill et al., 2003).
In C. perfringens, the expansion of IS1470 elements has previously been reported in Darmbrand strain NCTC 8081 (Ma et al., 2012), and in other bacteria, the expansion of IS elements has been associated with an increase in virulence (Parkhill et al., 2003; Siguier et al., 2006). The relevance of IS1470 expansion for these Eritrean strains remains unknown, but the impact of IS1470 expansion on C. perfringens gene expression and host adaptation would be interesting topics for further research.
5 Conclusion
Our results suggest that persistent food poisoning outbreaks caused by C. perfringens type F strains can occur and that humans are a likely reservoir and carrier for enteropathogenic C. perfringens. We also conclude that the occurrence of additional IS1470 elements in chromosomal cpe-carrying C. perfringens strains can impair the PCR typing, resulting in false-negative typing of these strains.
We present six new c-cpe C. perfringens genomes featuring an additional IS1470 element at the cpe insertion site and describe the organization of this new variant of the cpe locus. In addition, these variant c-cpe strains carry 58–81 copies of IS1470 in their genomes instead of 9–23 copies in previously described chromosomal cpe strains.
Our study contributes to the expansion of the pool of c-cpe strains by introducing Eritrean strains marking the first reported instances of c-cpe strains originating from Eastern Africa.
Data availability statement
The datasets presented in this study can be found in online repositories. The names of the repository/repositories and accession number(s) can be found in the article/Supplementary material.
Ethics statement
Ethical approval was not required for the studies involving humans because in our study, the epidemiological investigation, including the literary questionnaire, sampling of the fecal specimens, and environmental samples, was done based on the Finnish legal obligations of food safety and health protection regulations. The epidemiologic investigation was carried out in 2004 by order of the Health Protection Authority of the Finnish Defence Forces. The order included all parts of the investigation (questionnaire, sampling and reporting). For the quick solving of the epidemics, there was a continuous right and an obligation to carry out the investigation ordered by the authorities of the Defence Forces. The research did not involve intervening in the physical integrity of research participants. The persons participated as study objects were volunteers who took the samples themselves and delivered them to the investigation group. No ethical concerns were identified, and no specific ethical approval was required. The studies were conducted in accordance with the local legislation and institutional requirements. Written informed consent for participation was not required from the participants or the participants’ legal guardians/next of kin in accordance with the national legislation and institutional requirements because persons participating as study objects in the investigation were beforehand informed volunteers, and they agreed that the investigation results could be reported anonymized. An informed consent procedure was used, but not a written informed consent procedure since the results were anonymized.
Author contributions
PL: Writing – review & editing, Writing – original draft, Investigation. KJ: Validation, Investigation, Writing – review & editing, Writing – original draft, Visualization, Software, Methodology, Formal analysis, Data curation. AHö: Resources, Writing – review & editing, Investigation, Conceptualization. AHe: Writing – review & editing, Methodology, Investigation. AS: Writing – review & editing, Resources, Investigation. HK: Writing – original draft, Supervision, Writing – review & editing, Resources, Project administration, Funding acquisition.
Funding
The author(s) declare that no financial support was received for the research, authorship, and/or publication of this article.
Acknowledgments
We thank Miia Lindström and Kirsi Ristkari from the University of Helsinki and Pia Laine from the Institute of Biotechnology for technical assistance and advice.
Conflict of interest
The authors declare that the research was conducted in the absence of any commercial or financial relationships that could be construed as a potential conflict of interest.
Publisher’s note
All claims expressed in this article are solely those of the authors and do not necessarily represent those of their affiliated organizations, or those of the publisher, the editors and the reviewers. Any product that may be evaluated in this article, or claim that may be made by its manufacturer, is not guaranteed or endorsed by the publisher.
Supplementary material
The Supplementary material for this article can be found online at: https://www.frontiersin.org/articles/10.3389/fmicb.2024.1459840/full#supplementary-material
Footnotes
References
Abdel-Glil, M. Y. P., Thomas, J. L., Busch, A., Wieler, L. H., Neubauer, H., and Seyboldt, C. (2021). Comparative in Silico genome analysis of Clostridium perfringens unravels stable Phylogroups with different genome characteristics and pathogenic potential. Sci. Rep. 11, 1–15. doi: 10.1038/s41598-021-86148-8
Abdelrahim, M., Abakabir, N. R., Delannoy, S., Djellal, S., Le Négrate, M., Hadjab, K., et al. (2019). Large-scale genomic analyses and Toxinotyping of Clostridium perfringens implicated in foodborne outbreaks in France. Front. Microbiol. 10:777. doi: 10.3389/fmicb.2019.00777
Browne, H. P., Anne Neville, B., Forster, S. C., and Lawley, T. D. (2017). Transmission of the gut microbiota: spreading of health. Nat. Rev. Microbiol. 2017, 531–543. doi: 10.1038/nrmicro.2017.50
Brynestad, S. (1997). Genetic studies on Clostridium perfringens enterotoxin. Oslo, Norway: Norwegian College of Veterinary Medicine.
Brynestad, S., Iwanejko, L. A., Stewart, G. S. A. B., and Granum, P. E. (1994). A complex Array of Hpr consensus DNA recognition sequences proximal to the enterotoxin gene in Clostridium perfringens type a. Microbiology 140, 97–104. doi: 10.1099/13500872-140-1-97
Brynestad, S., Synstad, B., and Granum, P. E. (1997). The Clostridium perfringens enterotoxin gene is on a transposable element in type a human food poisoning strains. Microbiology 143, 2109–2115. doi: 10.1099/00221287-143-7-2109
Carman, R. J., Sayeed, S., Li, J., Genheimer, C. W., Hiltonsmith, M. F., Wilkins, T. D., et al. (2008). Clostridium perfringens toxin genotypes in the feces of healthy north Americans. Anaerobe 14, 102–108. doi: 10.1016/J.ANAEROBE.2008.01.003
Chain, P. S. G., Carniel, E., Larimer, F. W., Lamerdin, J., Stoutland, P. O., Regala, W. M., et al. (2004). Insights into the evolution of Yersinia Pestis through whole-genome comparison with Yersinia Pseudotuberculosis. Proc. Natl. Acad. Sci. U. S. A. 101, 13826–13831. doi: 10.1073/pnas.0404012101
Chin, C.-S., Alexander, D. H., Marks, P., Klammer, A. A., Drake, J., Heiner, C., et al. (2013). Nonhybrid, finished microbial genome assemblies from long-read SMRT sequencing data. Nat. Methods 10, 563–569. doi: 10.1038/nmeth.2474
Cornillot, E., Saint-Joanis, B., Daube, G., Katayama, S.-i.-i., Granum, P. E., Canard, B., et al. (1995). The enterotoxin gene (Cpe) of Clostridium perfringens can be chromosomal or plasmid-borne. Mol. Microbiol. 15, 639–647. doi: 10.1111/j.1365-2958.1995.tb02373.x
Daube, G., Simon, P., and Kaeckenbeeck, A. (1993). IS1151, an IS-like element of Clostridium perfringens. Nucleic Acids Res. 21:352. doi: 10.1093/NAR/21.2.352
Feng, Y., Fan, X., Zhu, L., Yang, X., Liu, Y., Gao, S., et al. (2020). Phylogenetic and genomic analysis reveals high genomic openness and genetic diversity of Clostridium perfringens. Microbial Genomics 6:e000441. doi: 10.1099/mgen.0.000441
Heikinheimo, A., and Korkeala, H. (2005). Multiplex PCR assay for Toxinotyping Clostridium perfringens isolates obtained from Finnish broiler chickens. Lett. Appl. Microbiol. 40, 407–411. doi: 10.1111/J.1472-765X.2005.01702.X
Heikinheimo, A., Lindström, M., Granum, P. E., and Korkeala, H. (2006). Humans as reservoir for enterotoxin gene-carrying Clostridium perfringens type A. Emerg. Infect. Dis. 12, 1724–1729. doi: 10.3201/eid1211.060478
Heikinheimo, A., Lindström, M., and Korkeala, H. (2004). Enumeration and isolation of Cpe-positive Clostridium perfringens spores from feces. J. Clin. Microbiol. 42, 3992–3997. doi: 10.1128/JCM.42.9.3992-3997.2004
Jaakkola, K., Virtanen, K., Lahti, P., Keto-Timonen, R., Lindström, M., and Korkeala, H. (2021). Comparative genome analysis and spore heat resistance assay reveal a new component to population structure and genome epidemiology within Clostridium perfringens enterotoxin-carrying isolates. Front. Microbiol. 12:2530. doi: 10.3389/FMICB.2021.717176/BIBTEX
Keto-Timonen, R., Heikinheimo, A., Eerola, E., and Korkeala, H. (2006). Identification of Clostridium species and DNA fingerprinting of Clostridium perfringens by amplified fragment length polymorphism analysis. J. Clin. Microbiol. 44, 4057–4065. doi: 10.1128/JCM.01275-06
Kiu, R., Caim, S., Alexander, S., Pachori, P., and Hall, L. J. (2017). Probing genomic aspects of the multi-host pathogen Clostridium perfringens reveals significant Pangenome diversity, and a diverse Array of virulence factors. Front. Microbiol. 8:2485. doi: 10.3389/fmicb.2017.02485
Kiu, R., Caim, S., Painset, A., Pickard, D., Swift, C., Dougan, G., et al. (2019). Phylogenomic analysis of gastroenteritis-associated Clostridium perfringens in England and Wales over a 7-year period indicates distribution of clonal toxigenic strains in multiple outbreaks and extensive involvement of enterotoxin-encoding (CPE) plasmids. Microb. Genomics 5:e000297. doi: 10.1099/mgen.0.000297
Kiu, R., and Hall, L. J. (2018). An update on the human and animal enteric pathogen Clostridium perfringens. Emerg. Microbes Infect. 7. doi: 10.1038/s41426-018-0144-8
Lahti, P., Heikinheimo, A., Johansson, T., and Korkeala, H. (2008). Clostridium perfringens type a strains carrying a plasmid-borne enterotoxin gene (genotype IS1151-Cpe or IS1470-like-Cpe) as a common cause of food poisoning. J. Clin. Microbiol. 46, 371–373. doi: 10.1128/JCM.01650-07
Lahti, P., Lindström, M., Somervuo, P., Heikinheimo, A., and Korkeala, H. (2012). Comparative genomic hybridization analysis shows different epidemiology of chromosomal and plasmid-borne Cpe-carrying Clostridium perfringens type a. PLoS One 7:e46162. doi: 10.1371/journal.pone.0046162
Leclair, D., Pagotto, F., Farber, J. M., Cadieux, B., and Austin, J. W. (2006). Comparison of DNA fingerprinting methods for use in investigation of type E botulism outbreaks in the Canadian Arctic. J. Clin. Microbiol. 44, 1635–1644. doi: 10.1128/JCM.44.5.1635-1644.2006
Li, J., Adams, V., Bannam, T. L., Miyamoto, K., Garcia, J. P., Uzal, F. A., et al. (2013). Toxin plasmids of Clostridium perfringens. Microbiol. Mol. Biol. Rev. 77, 208–233. doi: 10.1128/mmbr.00062-12
Li, J., and McClane, B. A. (2008). A novel small acid soluble protein variant is important for spore resistance of most Clostridium perfringens food poisoning isolates. PLoS Pathog. 4:e1000056. doi: 10.1371/journal.ppat.1000056
Li, J., Miyamoto, K., and McClane, B. A. (2007). Comparison of virulence plasmids among Clostridium perfringens type E isolates. Infect. Immun. 75, 1811–1819. doi: 10.1128/IAI.01981-06
Li, J., Miyamoto, K., Sayeed, S., and McClane, B. A. (2010). Organization of the Cpe Locus in CPE-positive Clostridium perfringens Type C and D isolates. PLoS One 5:e10932. doi: 10.1371/journal.pone.0010932
Lindström, M., Heikinheimo, A., Lahti, P., and Korkeala, H. (2011). Novel insights into the epidemiology of Clostridium perfringens type a food poisoning. Food Microbiol. 28, 192–198. doi: 10.1016/j.fm.2010.03.020
Ma, M., Li, J., and Mcclane, B. A. (2012). Genotypic and phenotypic characterization of Clostridium perfringens isolates from Darmbrand cases in post-world war ii Germany. Infect. Immun. 80, 4354–4363. doi: 10.1128/IAI.00818-12
Miyamoto, K., Chakrabarti, G., Morino, Y., and McClane, B. A. (2002). Organization of the Plasmid Cpe Locus in Clostridium perfringens type a isolates. Infect. Immun. 70, 4261–4272. doi: 10.1128/IAI.70.8.4261-4272.2002
Miyamoto, K., Fisher, D. J., Li, J., Sayeed, S., Akimoto, S., and McClane, B. A. (2006). Complete sequencing and diversity analysis of the enterotoxin-encoding plasmids in Clostridium perfringens type a non-food-borne human gastrointestinal disease isolates. J. Bacteriol. 188, 1585–1598. doi: 10.1128/JB.188.4.1585-1598.2006
Miyamoto, K., Wen, Q., and McClane, B. A. (2004). Multiplex PCR genotyping assay that distinguishes between isolates of Clostridium perfringens type a carrying a chromosomal enterotoxin gene (Cpe) locus, a plasmid Cpe locus with an IS1470-like sequence, or a plasmid Cpe locus with an IS1151 sequence. J. Clin. Microbiol. 42, 1552–1558. doi: 10.1128/JCM.42.4.1552-1558.2004
Miyamoto, K., Yumine, N., Mimura, K., Nagahama, M., Li, J., McClane, B. A., et al. (2011). Identification of novel Clostridium perfringens type E strains that carry an iota toxin plasmid with a functional enterotoxin gene. PLoS One 6:e20376. doi: 10.1371/journal.pone.0020376
Nguyen, L. T., Schmidt, H. A., von Haeseler, A., and Minh, B. Q. (2015). IQ-TREE: a fast and effective stochastic algorithm for estimating maximum-likelihood phylogenies. Mol. Biol. Evol. 32, 268–274. doi: 10.1093/molbev/msu300
Parkhill, J., Sebaihia, M., Preston, A., Murphy, L. D., Thomson, N., Harris, D. E., et al. (2003). Comparative analysis of the genome sequences of Bordetella Pertussis, Bordetella Parapertussis and Bordetella Bronchiseptica. Nat. Genet. 35, 32–40. doi: 10.1038/NG1227
Parkhill, J., Wren, B. W., Thomson, N. R., Titball, R. W., Holden, M. T., Prentice, M. B., et al. (2001). Genome sequence of Yersinia Pestis, the causative agent of plague. Nature 413, 523–527. doi: 10.1038/35097083
Rambaut, A., and Drummond, A. (2008). FigTree: tree figure drawing tool, version 1.4. 4. Available at: http://tree.bio.ed.ac.uk/software/figtree/ (Accessed January 12, 2021).
Ridell, J., Björkroth, J., Eisgrüberg, H., Schalch, B., Stolle, A., and Korkeala, H. (1998). Prevalence of the enterotoxin gene and clonality of Clostridium perfringens strains associated with food-poisoning outbreaks. J. Food Prot. 61, 240–243. doi: 10.4315/0362-028X-61.2.240
Rood, J. I., Adams, V., Lacey, J., Lyras, D., McClane, B. A., Melville, S. B., et al. (2018). Expansion of the Clostridium perfringens toxin-based typing scheme. Anaerobe 53, 5–10. doi: 10.1016/j.anaerobe.2018.04.011
Schürch, A. C., Arredondo-Alonso, S., Willems, R. J. L., and Goering, R. V. (2018). Whole genome sequencing options for bacterial strain typing and epidemiologic analysis based on single nucleotide polymorphism versus gene-by-gene–based approaches. Clin. Microbiol. Infect. 24, 350–354. doi: 10.1016/j.cmi.2017.12.016
Seemann, T. (2014). Prokka: rapid prokaryotic genome annotation. Bioinformatics 30, 2068–2069. doi: 10.1093/bioinformatics/btu153
Seemann, T. (2015). Snippy: Fast bacterial variant calling from NGS reads. Available at: https://github.com/tseemann/snippy
Siguier, P., Filée, J., and Chandler, M. (2006). Insertion sequences in prokaryotic genomes. Curr. Opin. Microbiol. 9, 526–531. doi: 10.1016/J.MIB.2006.08.005
Silva, M., Machado, M. P., Silva, D. N., Rossi, M., Moran-Gilad, J., Santos, S., et al. (2018). Microb. Genomics 4:e000166. doi: 10.1099/mgen.0.000166
Staden, R., Judge, D. P., and Bonfield, J. K. (2003). “Managing sequencing projects in the GAP4 environment” in Introduction to bioinformatics. A Theoretical and Practical Approach. eds. S. A. Krawetz and D. D. Womble (Totawa, NJ 07512: Humana Press), 327–344.
Vandecraen, J., Chandler, M., Aertsen, A., and Van Houdt, R. (2017). The impact of insertion sequences on bacterial genome plasticity and adaptability. Crit. Rev. Microbiol. 43, 709–730. doi: 10.1080/1040841X.2017.1303661
Walker, B. J., Abeel, T., Shea, T., Priest, M., Abouelliel, A., Sakthikumar, S., et al. (2014). Pilon: an integrated tool for comprehensive microbial variant detection and genome assembly improvement. PLoS One 9:e112963. doi: 10.1371/journal.pone.0112963
Wen, Q., and McClane, B. A. (2004). Detection of Enterotoxigenic Clostridium perfringens type a isolates in American retail foods. Appl. Environ. Microbiol. 70, 2685–2691. doi: 10.1128/AEM.70.5.2685-2691.2004
Yibar, A., Cetin, E., Ata, Z., Erkose, E., and Tayar, M. (2018). Clostridium perfringens contamination in retail meat and meat-based products in Bursa, Turkey. Foodborne Pathog Dis. 15, 239–245. doi: 10.1089/FPD.2017.2350
Keywords: Clostridium perfringens, genotyping, variant strains, enterotoxin gene, food poisoning, persistent outbreak, IS element
Citation: Lahti P, Jaakkola K, Hörman A, Heikinheimo A, Sovijärvi A and Korkeala H (2024) Identification of a new Clostridium perfringens variant with a chromosomally encoded enterotoxin gene in a suspected persistent food poisoning outbreak in Eritrea. Front. Microbiol. 15:1459840. doi: 10.3389/fmicb.2024.1459840
Edited by:
Xuanyu Tao, University of Oklahoma, United StatesReviewed by:
Hui Li, University of Oklahoma, United StatesRicardo Oliveira, National Institute for Agrarian and Veterinary Research (INIAV), Portugal
Jihong Li, University of Pittsburgh, United States
Copyright © 2024 Lahti, Jaakkola, Hörman, Heikinheimo, Sovijärvi and Korkeala. This is an open-access article distributed under the terms of the Creative Commons Attribution License (CC BY). The use, distribution or reproduction in other forums is permitted, provided the original author(s) and the copyright owner(s) are credited and that the original publication in this journal is cited, in accordance with accepted academic practice. No use, distribution or reproduction is permitted which does not comply with these terms.
*Correspondence: Päivi Lahti, cGFpdmkubGFodGlAaGVsc2lua2kuZmk=
†These authors have contributed equally to this work
‡ORCID: Hannu Korkeala, https://orcid.org/0000-0003-0699-1290