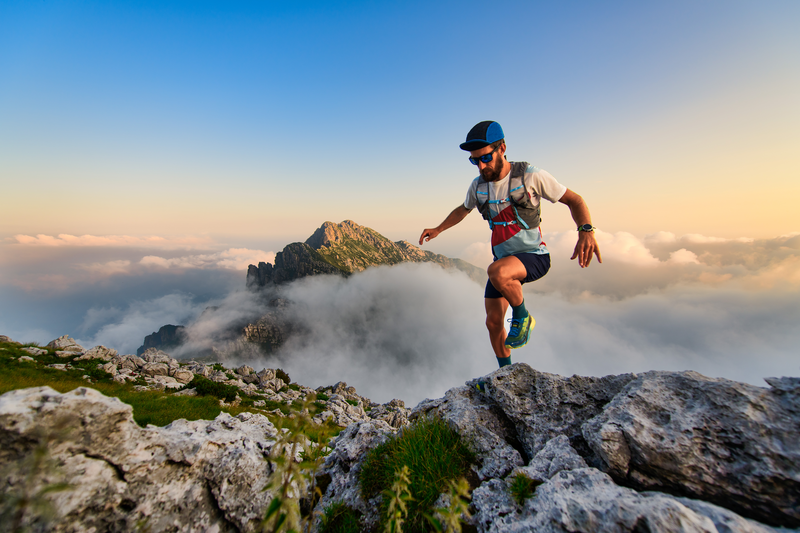
94% of researchers rate our articles as excellent or good
Learn more about the work of our research integrity team to safeguard the quality of each article we publish.
Find out more
ORIGINAL RESEARCH article
Front. Microbiol. , 11 September 2024
Sec. Microorganisms in Vertebrate Digestive Systems
Volume 15 - 2024 | https://doi.org/10.3389/fmicb.2024.1458754
This article is part of the Research Topic Fecal Microbiota Transplants: challenges in translating microbiome research to clinical applications View all 17 articles
Background: Research on the effects of intestinal microbiota transplantation (IMT) on chronic HBV infection (CHB) progression associated liver disease (HBV-CLD) and alterations in the microbiota post-IMT are quite limited for the moment.
Methods: By integrating microbiome with metabolome analyses, we aimed to the function of IMT and the alterations of gut microbiota in patients with HBV-CLD. First, this study included 20 patients with HBV-CLD and ten healthy controls. Then, 16 patients with CHB were given IMT with donor feces (heterologous) via oral capsule. Fecal samples from CHB patients were obtained before and after IMT, as well as healthy controls, for 16S rDNA sequencing and untargeted metabolomics analysis.
Results: The proalbuminemia were significantly increased after IMT, and the HBsAg and TBA showed a significant decrease after IMT in the HBV-CLD patients. There was statistical difference in the Chaol indexes between between CHB patients and healthy controls, suggesting a lower abundance of the gut microbiota in HBV-CLD patients. In addition, there was statistical difference in the Shannon and Simpson indexes between prior to IMT and post-IMT, indicating that the impaired abundance of the gut microbiota had been improved after IMT. The host-microbiota-metabolite interplay, amino acid metabolism, nicotinate and nicotinamide metabolism, starch and sucrose metabolism, steroid biosynthesis, and vitamins metabolism, were significantly lower in HBV-CLD patients than healthy controls.
Conclusion: IMT may improve the therapeutic effects on patients HBV-CLD. Furthermore, IMT appears to improve amino acid metabolism by impaired abundance of the gut microbiota and therefore improve liver prealbumin synthesis.
Hepatitis B virus infection-associated liver disease (HBV-CLD) places a significant economic burden on the state and society, despite ongoing good interventions in many countries and areas. In the normal course of HBV infection, an estimated 15–40% of individuals with chronic hepatitis B (CHB) infection develop to cirrhosis, liver failure, or hepatocellular carcinoma (Arora et al., 2018).
The ideal endpoint for patients with HBV (both HBeAg positive and HBeAg negative) infection is hepatitis B surface antigen (HBsAg) loss, with or without seroconversion to anti-HBs. This is associated with complete and definitive remission of the activity of HBV (Chotiyaputta and Lok, 2010; Chen et al., 2002). During the natural history of chronic HBV infection, the loss of serum HBeAg and the development of anti-HBe antibodies (HBeAg seroconversion) mark a transition from the immune-active phase of the disease to the inactive carrier state (Chen and Yang, 2011).
The liver secretes bile into the bile duct system, while a variety of gut-derived signals, such as bacterial products, environmental toxins, and dietary antigens, enter the gut and travel through the biliary tract to the liver (Albillos et al., 2020). Based on the “gut-liver axis, “an increasing number of research have discovered a link between hepatic damage and disturbance of gut microbial homeostasis. Growing data suggests that gut microbe-derived metabolites such as trimethylamine, bile acids, and short-chain fatty acids play a substantial role in the beginning and progression of liver diseases (Zhao et al., 2020; Jia et al., 2018). Furthermore, a large signaling network regulated by microbiota-derived metabolism continues to be studied, providing gut-liver targets for disease therapy (Li et al., 2023).
Intestinal microbiota transplantation (IMT) is a novel approach to restoring and reconstructing the intestinal microecological balance and diversity. IMT has been shown to be effective in the treatment of a variety of disorders, including Clostridium difficile infection, metabolic diseases, malignancies, autoimmune diseases, nonalcoholic fatty liver disease (NAFLD), and hepatic encephalopathy (Surawicz et al., 2013; de Groot et al., 2017; Vaughn et al., 2019; Meighani et al., 2020). Besides, IMT appears to be safe and potentially effective in terms of viral suppression and HBeAg clearance in patients with HBeAg-positive CHB (Chauhan et al., 2021).
Although some studies have been conducted on the diversity of gut microbiota in chronic hepatitis B, as well as the clinical effects of IMT on E antigen and liver cirrhosis, research on the effects of IMT on early stage cirrhosis or pre-cirrhosis patients and changes in the microbiota post-IMT is quite limited. Therefore, in the present study, we performed a clinical study to characterize gut microbiota in CHB progression, followed by an investigation into the role of IMT in HBeAg-negative CHB patients, and finally, we examined the impact of IMT on gut microbiota composition and metabolomics signature.
There were three study groups, a cross-sectional study was conducted to see if there was any alternation in gut microbiota and metabonomics in HBV-CLD patients with healthy controls. Afterward, a perspective study was performed in the cohort of patients before IMT and after IMT. The study was approved by The Research Ethics Committee at The Fifth Affiliated Hospital of Sun Yat-Sen University (no.·ZDWY [2023] Lunzi no.·K254-1). Written informed consent was obtained from each participant before the initiation of study procedures.
A total of 16 HBeAg-negative HBV-CLD patients were enrolled at The Fifth Affiliated Hospital of Sun Yat-Sen University from February 2022 to March 2024, including 3 early stage of cirrhosis and 13 pre-cirrhosis. HBV-CLD patients on oral antivirals, which included entecavir (ETV), tenofovir disoproxil fumarate (TDF) or tenofovir alafenamide fumarate (TAF) alone, for more than a year undetectable of serum levels of HBVDNA and those who consented for the IMT were included in the IMT arm. A total of 10 age-and BMI-matched Chinese healthy individuals were enrolled, The inclusion criteria were as follows: (a) alcohol free history; (b) smooth and soft stool that was sausage or snake shaped, and (c) voluntary participation in this study. Patients with co-infections with hepatitis C virus (HCV) or human immunodefciency virus (HIV), chronic kidney disease, hepatocellular carcinoma, underlying other comorbidities, and those unwilling to provide written informed consent for the IMT procedure were excluded. We also excluded patients with underlying cirrhosis.
Fecal microbiota capsules for the IMT group were obtained from healthy undergraduate donors (Guangdong Pharmaceutical University) and evaluated using comprehensive physical examinations. The preparation and the transplantation of fecal microbiota capsules were performed as previously described (Kao et al., 2017). Forty capsules were manufactured from 1 donation. All HBV-CLD patients received a total of 120 capsules, 6–10 capsules three times a day (according to the digestive reaction of patients, each dosage can be appropriately reduced), for a total course of about 6 days. Theoretically, there were four groups: Group A, HBV-CLD patients; group B, healthy controls; group C, pri-IMT; group D, post-IMT.
All patients who underwent IMT were evaluated clinically daily while taking the fecal microbiota capsules for the development of any new symptoms, assessment of side-effects post-procedure. Adverse events in the form of eight gastrointestinal symptom parameters were observed within 7 days following IMT. Liver function tests, blood routine examinations, HBsAg, and HBVDNA levels were measured at baseline and weeks 8, 24. All 16 patients completed blood tests, 13 patients completed pri-IMT stool 16S rRNA sequencing, and 7 patients completed post-IMT 16S rRNA sequencing.
Stool samples were collected before IMT and 1 month after IMT. Study participants were given a stool sample kit at each in-person visit to collect the stool sample. Explicit instructions were given to the participants on how to collect the samples. Once collected (within seven days of an inperson visit), the samples were frozen at −80°C until sequencing. Libraries were generated by amplification of the V3–V4 region of the 16S ribosomal RNA gene of DNA extracted from gut mucosal biopsies and from stool samples, and sequenced on the Illumina MiSeq platform (Illumina, San Diego, California). For 16S analysis, QIIME (version 1.9.1) was used to demultiplex and quality-filter the raw FASTQ files. Next, operational taxonomic units (OTUs) were generated and clustered using a 97% threshold and Usearch (version 7.1). After aligning with the gold database (v20110519), UCHIME (4.2.40) was used to filter out chimaeric sequences, and use arch_global was used to quantify the OTU abundances for each sample. For taxonomic analysis of each representative OTU, the Greengenes database (v201305) was used based on the RDP classifier (Version 2.2) with a 0.8 confidence value. OTUs were then assigned to different hierarchical levels and taxonomic relative abundance profiles were summarized.
To understand the roles and interactions of proteins, metabolites, and genes in biological systems, the KEGG database was built. In this study, we used DIAMOND (v0.9.7) to annotate the representative sequences of gene sets with the KEGG database. To obtain the functional abundance at each level, we used the BLAST comparison parameters with an expectation of E < 1e−5. To find the metabolic pathway enrichment results for metabolites, enrichment analysis was carried out using their KEGG IDs. Pathway entries that were considerably enriched in significantly differently expressed metabolites relative to the background were identified using hypergeometric testing.
To analyze differences in continuous variables, we employed the paired-samples t-test or the Wilcoxon signed-rank test. Pearson correlation analysis for gut microbiota post IMT, FDR for multiple correction. Microbial features (species and metabolites) found in fewer than 20% of the samples were eliminated prior to statistical analysis of the relative abundance data. Spearman’s rank correlation was used to examine associations between gut microbiota and metabolites, which were then adjusted for significance using the Benjamini-Hochberg approach. Linear discriminant analysis effect size (LEfSe) was used to assess groups for statistically significant species and functional differences. The nonparametric factorial Kruskal-Wallis test, Wilcoxon rank sum test, and LDA were used to identify biological and functional markers that were enriched for differences between multiple metadata categories. We performed statistical analyses using SPSS 22.0 (IBM Corporation, Armonk, New York, United States) and GraphPad Prism 6.0 (GraphPad Software, Inc. La Jolla, CA, United States). Unless otherwise stated, all statistical tests are two-tailed, and p < 0.05 was deemed statistically significant.
Between December 2023 and March 2024, 30 participants were screened and 26 were enrolled in this study: 16 HBV-CLD patients accepted IMT, 10 healthy controls. Two HBV-CLD patients were excluded for they did not complete the treatment course of IMT. Fourteen HBV-CLD patients received full course of IMT, while two patients did not complete blood tests.
All of the patients had undetectable HBVDNA at baseline and continued on their previous antiviral medication while undergoing IMT. 78.5% of patients had hypothrombocytemia, with a median of 75 × 109/L. Plaque increased considerably after IMT at 8 weeks (p = 0.001) and 24 weeks (p<0.001) (Figure 1B). Additionally, 78.5% had hypoproalbuminemia, with a median of 145 g/L 8 weeks (p = 0.259), 24 weeks (p = 0.007) (Figure 1F), HBsAg showed a significant decrease after IMT: 8 weeks (p = 0.026), 24 weeks (p = 0.116) (Figure 1C), and total bile acid (TBA) showed a significant decrease after IMT: 8 weeks (p = 0.024), 24 weeks (p = 0.001) (Figure 1E). Some individuals with hypoleukocytoemia had an increase in white blood cells following transplantation (Figure 1A). Nonetheless, the alanine transaminase (ALT) (Table 1) and aspartate aminotransferase (AST) levels were mostly maintained in the normal range following IMT.
Figure 1. Changes curve in laboratory parameters over time post IMT. (A) the change curve of white blood cells (WBC); (B) the change curve of platelet (PLT); (C) the change curve of HBsAg; (D) the change curve of alanine aminotransferase (ALT); (E) the change curve of total bile acid (TBA); (F) the change curve of prealbumin. *p < 0.05, **p < 0.01.
Alpha diversity indexes indicating community richness, diversity, evenness and coverage were assessed via Chaol, Shannon, Simpson, ACE and Coverage indexes. Therein, there was no difference in the Shannon index and Simpson index, but the Chao1 index was higher in the patient group. But, Chao1 places a strong emphasis on the presence of rare species within a community, with a primary focus on species richness or the total number of different species present. However, its drawback lies in the fact that it does not take into account the distribution abundance and evenness of species within the community. Both qualitative PCoA and PCA analysis were performed to evaluate β diversity. No significant changes in β diversity was observed in the comparison between HBV-CLD patients and healthy controls (Supplementary Figure S1).
There was significant difference in the Shannon and Simpson indexes between prior to IMT (pri-IMT) and post-IMT (Figures 2E,F; Shannon p = 0.034, Simpson p = 0.011), indicating that the impaired abundance of the gut microbiota had been improved after IMT in HBV-CLD patients. No significant changes in β diversity was observed in the comparison between pri-IMT and post-IMT (Supplementary Figure S2). In addition, we conducted pearson correlation analysis of individual microflora post-IMT. Although there was still a strong correlation between the microflora before and after transplantation, individual evolution occurred to different degrees (Table 2).
Figure 2. The α diversity of gut microbiota. The α diversity for HBV-CLD patients (group A) compared with healthy controls (group B) in Chaol indexes (A), Shannon indexes (B), Simpson indexes (C), the α diversity for pri-IMT (group C) compared with post-IMT (group D) in Chaol indexes (D), Shannon indexes (E), Simpson indexes (F). *p < 0.05.
To further explore the taxonomic features and composition differences of gut microbiota associated with HBV-CLD patients, the raw macrogenome data were checked by quality control, filtered, and assembled to construct abundance profiles at the corresponding taxonomic levels. And we compared bacteria abundance before IMT and after IMT to further demonstrate the microbiota shifts in HBV-CLD patients. In LEfSe analysis (p < 0.05, q < 0.1, LDA > 2.0), compared with the HCs, the c. Bacilli, o. Lactobacillales, f. Streptococcaceae, g. Streptococcus, g. Paeniclostridium, g. Veillonella, were further diminished in CHB patients (Figure 3). While f. Rikenellaceae, f. Lachnospiraceae, f. Barnesiellaceae, g. Megamonas, g. Alistipes, g. Erysipelatoclostridium, were more enriched in CHB patients (Figures 4A,B).
Figure 3. Gut microbiota profile for HBV-CLD patients. The relative abundance between group A and group B (A). LEfSe analysis shows species with significant differences in abundance between group A and group B (B). Group A: HBV-CLD patients, group B: healthy controls.
Figure 4. Alternation of microbiome and the predicted microbial functional composition with PICRUST analysis. The relative abundance of microbiome in genus level between group A and group B (A); the relative abundance of microbiome in species level between group C and group D (B). The predicted microbial functional composition with PICRUST analysis for HBV-CLD patients (level II) (C). The KEGG pathways (level III) with PICRUST analysis after IMT (D). Group A: HBV-CLD patients, group B: healthy controls, group C: pri-IMT, group D: post-IMT.
After IMT, compared with pri-IMT, the o. Coriobacteriales f. Enterobacteriaceae, g. Collinsella, g. Lachnoclostridium, g. Caproiciproducens, were significantly decreased in post-IMT patients (Figure 5).
Figure 5. Alternation of gut microbiota composition after IMT. The relative abundance between group C and group D (A). LEfSe analysis shows species with significant differences in abundance between group C and group D (B). Group C: pri-IMT, group D: post-IMT.
To determine if the functional pathways involved in gut metabolites and microbiota are consistently changed, we ran pathway enrichment analysis on the KEGG IDs of metabolites and obtained pathway enrichment findings. Representatively various metabolites would be examined.
Compared to the HCs, amino acid metabolism, including alanine, aspartate, glutamate and tryptophan metabolism, was significantly decreased in CHB patients. In addition, the nicotinate and nicotinamide metabolism, starch and sucrose metabolism, steroid biosynthesis, and vitamins metabolism, were significantly lower in CHB patients than HCs. It is worth noting that oxidative phosphorylation and nucleotide excision repair were further depleted in CHB patients. Whereas p53 signaling pathway was more exuberantly in CHB patients than HCs (Figure 4C).
Following IMT, PICRUST analysis showed that the relative abundance of t the metabolites listed above were partially restored. In addition, the PPAR signaling pathway and pathogenic Escherichia coli infection significantly lower in post-IMT than pri-IMT (Figure 4D).
In this study, we investigated the effectiveness of IMT on HBV-CLD patients and characterized gut microbiota in HBV-CLD progression by combining microbiome with metabolome pri-IMT and post-IMT. HBV-CLD patients in our study presented with hypoproalbuminemia, hypersplenism such as leukopenia and thrombocytopenia, but no sequelae from decompensated cirrhosis. Long-term antiviral medication did not ameliorate these problems.
In this study, a decrease in HBsAg was observed in E antigen-negative patients post-IMT 8 weeks. There were reported that IMT appeared to be safe and potentially effective in terms of viral suppression and HBeAg clearance in patients with HBeAg-positive CHB, but achieved no HBsAg clearance (Chauhan et al., 2021; Ren et al., 2017). IMT has the potential to modulate the host’s immune response and alter its susceptibility to HBV infection (Wang et al., 2022).
Oral IMT capsules were safe and well-tolerated in patient with cirrhosis and recurrent HE; besides, gut microbial function in cirrhosis is beneficially affected by capsular FMT, with improved inflammation and cognition (Wang et al., 2022; Bajaj et al., 2019). For patients with HBV-CLD in this study, increase in platelets and white blood cells could be observed after 24 weeks. It has been proven that gut flora (including Bacteroidetes, Parabacteroides, and Proteobacteria) were restored to levels comparable to those reported in healthy persons following splenectomy, which was followed by an increase in WBC and PLT counts in liver cirrhosis patients (Liu et al., 2018). Furthermore, significant improvements in proalbumin could be observed post-IMT. PICRUST analysis revealed that IMT restored the relative abundance of KEGG pathways involved in amino acid metabolism. That might be contribute by the improved composition of the gut microbiota by IMT. Furthermore, IMT might contribute largely to slow the progress of liver impairment.
The α diversity of gut microbiota was significantly decreased in the HBV-CLD arm, but showed considerable rebuilding following IMT. The hepatic portal and bile secretion networks allow the gut to connect directly with the liver (Cesaro et al., 2011). Improved gut microbiota and metabolomics may help to minimize liver damage.
In this study, the HBV-CLD arm exhibited a decrease in families from the Lactobacillales and Bacillus orders. Bacteroides, Lactobacillus, and Clostridiumsedis were linked to survival in cirrhosis patients with hepatic encephalopathy during a one-year period (Sung et al., 2019). Lactobacillus GG and prebiotics/synbiotics could improve microbial composition and outcomes in patients with cirrhosis (Dhiman et al., 2014). Lactobacillus play a role in the conversion of primary bile acids through the production of bile salt hydrolase (Li et al., 2023).
In accordance with previous studies, the abundance of Clostridia was downregulated in HBV-CLD patients (Ren et al., 2019; Zeng et al., 2020). The main producers of butyrate are Clostridia, Eubacteria, and Roseburia microbes (Nicholson et al., 2012). Short-chain fatty acids (SCFAs) are saturated fatty acids containing one to six carbon atoms, including acetate, propionate and butyrate. SCFAs regulate liver immune homeostasis and lipid metabolism by activating G-protein-coupled receptors or inhibiting the activity of histone deacetylase (HDAC) (Koh et al., 2016). Butyrate plays an important role in the regulation of intestinal barrier function, intestinal immunity, and inflammation response. Butyrate can bind to and activate peroxisome proliferator-activated receptorγ (PPARγ), thereby exerting an antiinflammatory effect through the antagonism of nuclear factor-κB transcription (Cani et al., 2019). The recovery observed in the gut microenvironment following IMT reflects changes in not only the abundances of bacteria and metabolites but also in the correlations between them.
The abundance of Megamonas was up-regulated in HBV-CLD patients in this study. It has been reported that Macroomonas was more abundant in Asian populations with colorectal cancer (Yachida et al., 2019). In addition, the attention-deficit/hyperactivity disorder (ADHD) group showed higher levels of Dialister and Megamonas. IMT modified the abundance of the Megamonas. Notably, the abundance of E. coli was down-regulated, and PICRUST analysis showed that the pathogenic Escherichia coli infection significantly lower in post-IMT than pri-IMT. E. coli and Streptococcus are the main causes of bacterial infection in patients with cirrhosis (Riordan and Williams, 2010). Bacteria from Enterobacteriaceae family (including Escherichia coli, Klebsiella, Proteus) are all regarded as PAMPs-producing bacteria (Chen et al., 2011), and the intestinal microflora is the main source of portal LPS and represents an important prerequisite for the development of liver fibrosis in chronic liver injuries (Paik et al., 2003).
Several limitations should be acknowledged in our study. Firstly, the limited sample size of microbiome and metabolomics may restrict significance and stability of the results. A long-term follow-up of prospective cohorts is required to further validate potential taxa/metabolites. Secondly, it’s important to note that 16 s rRNA sequencing has limitations in providing gut microbiota annotations with accuracy at the ‘species’ level. Vague annotations may lead to contentious outcomes. Thus, short-gun sequencing of metagenomics and metatranscriptomics has the potential to disclose more accurate information about the microbial community’s makeup and function.
In conclusion, HBV-CLD patients who underwent IMT showed improvements in liver function as well as a recovery of the composition and metabolism of the gut microbiota in this study. IMT results in decreased relative abundance of pathogenic bacteria such as Enterobacteriaceae and Megamonas. Furthermore, a increase in prealbumin and metabolomics of amino acid (including Eaa, tryptophan). The underlying mechanism still needs further study. Therefore, the regulation of gut microbiota by IMT may provide a new clinical approach for the treatment of HBV-CLD.
The original contributions presented in the study are included in the article/Supplementary material, further inquiries can be directed to the corresponding authors.
The studies involving humans were approved by the Research Ethics Committee at The Fifth Affiliated Hospital of Sun Yat-Sen University. The studies were conducted in accordance with the local legislation and institutional requirements. The participants provided their written informed consent to participate in this study.
LD: Formal analysis, Writing – original draft, Writing – review & editing, Conceptualization, Investigation, Supervision, Methodology, Project administration, Resources, Software, Visualization. XG: Data curation, Resources, Writing – original draft, Investigation, Supervision. JC: Investigation, Project administration, Resources, Writing – original draft. BL: Project administration, Writing – original draft. NL: Investigation, Validation, Writing – original draft. JX: Funding acquisition, Supervision, Writing – original draft. MO: Funding acquisition, Investigation, Supervision, Writing – review & editing. ZH: Conceptualization, Funding acquisition, Supervision, Writing – review & editing.
The author(s) declare that financial support was received for the research, authorship, and/or publication of this article. Funding for this study was provided by Start-up funds for scientific research of high-level talents, the Fifth Affiliated Hospital, Sun Yat-sen University.
We acknowledge all health-care workers involved in the diagnosis and treatment of patients in the Fifth Affiliated Hospital, Sun Yat-sen University and thank all patients and their families involved in the study.
The authors declare that the research was conducted in the absence of any commercial or financial relationships that could be construed as a potential conflict of interest.
All claims expressed in this article are solely those of the authors and do not necessarily represent those of their affiliated organizations, or those of the publisher, the editors and the reviewers. Any product that may be evaluated in this article, or claim that may be made by its manufacturer, is not guaranteed or endorsed by the publisher.
The Supplementary material for this article can be found online at: https://www.frontiersin.org/articles/10.3389/fmicb.2024.1458754/full#supplementary-material
Albillos, A., de Gottardi, A., and Rescigno, M. (2020). The gut-liver axis in liver disease: pathophysiological basis for therapy. J. Hepatol. 72, 558–577. doi: 10.1016/j.jhep.2019.10.003
Arora, A., Singh, S. P., Kumar, A., Saraswat, V. A., Aggarwal, R., Bangar, M., et al. (2018). INASL position statements on prevention, diagnosis and Management of Hepatitis B Virus Infection in India: the Andaman statements. J. Clin. Exp. Hepatol. 8, 58–80. doi: 10.1016/j.jceh.2017.12.001
Bajaj, J. S., Salzman, N., Acharya, C., Takei, H., Kakiyama, G., Fagan, A., et al. (2019). Microbial functional change is linked with clinical outcomes after capsular fecal transplant in cirrhosis. JCI Insight 4:e133410. doi: 10.1172/jci.insight.133410
Cani, P. D., Van Hul, M., Lefort, C., Depommier, C., Rastelli, M., and Everard, A. (2019). Microbial regulation of organismal energy homeostasis. Nat. Metab. 1, 34–46. doi: 10.1038/s42255-018-0017-4
Cesaro, C., Tiso, A., Del Prete, A., Cariello, R., Tuccillo, C., Cotticelli, G, et al. (2011). Gut microbiota and probiotics in chronic liver diseases. Dig. Liver Dis. 43, 431–438. doi: 10.1016/j.dld.2010.10.015
Chauhan, A., Kumar, R., Sharma, S., Mahanta, M., Vayuuru, S. K., Nayak, B., et al. (2021). Fecal microbiota transplantation in hepatitis B e antigen-positive chronic hepatitis B patients: a pilot study. Dig. Dis. Sci. 66, 873–880. doi: 10.1007/s10620-020-06246-x
Chen, Y. C., Sheen, I. S., Chu, C. M., and Liaw, Y. F. (2002). Prognosis following spontaneous HBsAg seroclearance in chronic hepatitis B patients with or without concurrent infection. Gastroenterology 123, 1084–1089. doi: 10.1053/gast.2002.36026
Chen, C. J., and Yang, H. I. (2011). Natural history of chronic hepatitis B REVEALed. J. Gastroenterol. Hepatol. 26, 628–638. doi: 10.1111/j.1440-1746.2011.06695.x
Chen, Y., Yang, F., Lu, H., Wang, B., Chen, Y., Lei, D., et al. (2011). Characterization of fecal microbial communities in patients with liver cirrhosis. Hepatology 54, 562–572. doi: 10.1002/hep.24423
Chotiyaputta, W., and Lok, A. S. (2010). Endpoints of hepatitis B treatment. J. Viral Hepat. 17, 675–684. doi: 10.1111/j.1365-2893.2010.01369.x
de Groot, P. F., Frissen, M. N., de Clercq, N. C., and Nieuwdorp, M. (2017). Fecal microbiota transplantation in metabolic syndrome: history, present and future. Gut Microbes 8, 253–267. doi: 10.1080/19490976.2017.1293224
Dhiman, R. K., Rana, B., Agrawal, S., Garg, A., Chopra, M., Thumburu, K. K., et al. (2014). Probiotic VSL#3 reduces liver disease severity and hospitalization in patients with cirrhosis: a randomized, controlled trial. Gastroenterology 147, 1327–1337.e3. doi: 10.1053/j.gastro.2014.08.031
Jia, W., Xie, G., and Jia, W. (2018). Bile acid-microbiota crosstalk in gastrointestinal inflammation and carcinogenesis. Nat. Rev. Gastroenterol. Hepatol. 15, 111–128. doi: 10.1038/nrgastro.2017.119
Kao, D., Roach, B., Silva, M., Beck, P., Rioux, K., Kaplan, G. G., et al. (2017). Effect of Oral capsule-vs colonoscopy-delivered fecal microbiota transplantation on recurrent Clostridium difficile infection: a randomized clinical trial. JAMA 318, 1985–1993. doi: 10.1001/jama.2017.17077
Koh, A., De Vadder, F., Kovatcheva-Datchary, P., and Bäckhed, F. (2016). From dietary Fiber to host physiology: short-chain fatty acids as key bacterial metabolites. Cell 165, 1332–1345. doi: 10.1016/j.cell.2016.05.041
Li, S. Q., Shen, Y., Zhang, J., Weng, C. Z., Wu, S. D., and Jiang, W. (2023). Immune modulation of gut microbiota and its metabolites in chronic hepatitis B. Front. Microbiol. 14:1285556. doi: 10.3389/fmicb.2023.1285556
Liu, Y., Li, J., Jin, Y., Zhao, L., Zhao, F., Feng, J., et al. (2018). Splenectomy leads to amelioration of altered gut microbiota and metabolome in liver cirrhosis patients. Front. Microbiol. 9:963. doi: 10.3389/fmicb.2018.00963
Meighani, A., Alimirah, M., Ramesh, M., and Salgia, R. (2020). Fecal microbiota transplantation for Clostridioides difficile infection in patients with chronic liver disease. Int. J. Hepatol. 2020:1874570. doi: 10.1155/2020/1874570
Nicholson, J. K., Holmes, E., Kinross, J., Burcelin, R., Gibson, G., Jia, W., et al. (2012). Host-gut microbiota metabolic interactions. Science 336, 1262–1267. doi: 10.1126/science.1223813
Paik, Y. H., Schwabe, R. F., Bataller, R., Russo, M. P., Jobin, C., and Brenner, D. A. (2003). Toll-like receptor 4 mediates inflammatory signaling by bacterial lipopolysaccharide in human hepatic stellate cells. Hepatology 37, 1043–1055. doi: 10.1053/jhep.2003.50182
Ren, Z., Li, A., Jiang, J., Zhou, L., Yu, Z., Lu, H., et al. (2019). Gut microbiome analysis as a tool towards targeted non-invasive biomarkers for early hepatocellular carcinoma. Gut 68, 1014–1023. doi: 10.1136/gutjnl-2017-315084
Ren, Y. D., Ye, Z. S., Yang, L. Z., Jin, L. X., Wei, W. J., Deng, Y. Y., et al. (2017). Fecal microbiota transplantation induces hepatitis B virus e-antigen (HBeAg) clearance in patients with positive HBeAg after long-term antiviral therapy. Hepatology 65, 1765–1768. doi: 10.1002/hep.29008
Riordan, S. M., and Williams, R. (2010). Gut flora and hepatic encephalopathy in patients with cirrhosis. N. Engl. J. Med. 362, 1140–1142. doi: 10.1056/NEJMe1000850
Sung, C. M., Lin, Y. F., Chen, K. F., Ke, H. M., Huang, H. Y., Gong, Y. N., et al. (2019). Predicting clinical outcomes of cirrhosis patients with hepatic encephalopathy from the fecal microbiome. Cell. Mol. Gastroenterol. Hepatol. 8, 301–318.e2. doi: 10.1016/j.jcmgh.2019.04.008
Surawicz, C. M., Brandt, L. J., Binion, D. G., Ananthakrishnan, A. N., Curry, S. R., Gilligan, P. H., et al. (2013). Guidelines for diagnosis, treatment, and prevention of Clostridium difficile infections. Am. J. Gastroenterol. 108:499. doi: 10.1038/ajg.2013.4
Vaughn, B. P., Rank, K. M., and Khoruts, A. (2019). Fecal microbiota transplantation: current status in treatment of GI and liver disease. Clin. Gastroenterol. Hepatol. 17, 353–361. doi: 10.1016/j.cgh.2018.07.026
Wang, J., Zhou, X., Li, X., Guo, W., Zhu, Q., Zhu, B., et al. (2022). Fecal microbiota transplantation alters the outcome of hepatitis B virus infection in mice. Front. Cell. Infect. Microbiol. 12:844132. doi: 10.3389/fcimb.2022.844132
Yachida, S., Mizutani, S., Shiroma, H., Shiba, S., Nakajima, T., Sakamoto, T., et al. (2019). Metagenomic and metabolomic analyses reveal distinct stage-specific phenotypes of the gut microbiota in colorectal cancer. Nat. Med. 25, 968–976. doi: 10.1038/s41591-019-0458-7
Zeng, Y., Chen, S., Fu, Y., Wu, W., Chen, T., Chen, J., et al. (2020). Gut microbiota dysbiosis in patients with hepatitis B virus-induced chronic liver disease covering chronic hepatitis, liver cirrhosis and hepatocellular carcinoma. J. Viral Hepat. 27, 143–155. doi: 10.1111/jvh.13216
Zhao, M., Zhao, L., Xiong, X., He, Y., Huang, W., Liu, Z., et al. (2020). TMAVA, a metabolite of intestinal microbes, is increased in plasma from patients with liver steatosis, inhibits gamma-Butyrobetaine hydroxylase, and exacerbates fatty liver in mice. Gastroenterology 158, 2266–2281.e27. doi: 10.1053/j.gastro.2020.02.033
Keywords: chronic hepatitis B virus infection, microbiome, metabolome, intestinal microbiota transplantation, alterations
Citation: Deng L, Guo X, Chen J, Li B, Liu N, Xia J, Ou M and Hong Z (2024) Effect of intestinal microbiota transplantation on chronic hepatitis B virus infection associated liver disease. Front. Microbiol. 15:1458754. doi: 10.3389/fmicb.2024.1458754
Received: 11 July 2024; Accepted: 20 August 2024;
Published: 11 September 2024.
Edited by:
Ludovico Abenavoli, Magna Græcia University, ItalyReviewed by:
Bon-Sang Koo, Korea Research Institute of Bioscience and Biotechnology, Republic of KoreaCopyright © 2024 Deng, Guo, Chen, Li, Liu, Xia, Ou and Hong. This is an open-access article distributed under the terms of the Creative Commons Attribution License (CC BY). The use, distribution or reproduction in other forums is permitted, provided the original author(s) and the copyright owner(s) are credited and that the original publication in this journal is cited, in accordance with accepted academic practice. No use, distribution or reproduction is permitted which does not comply with these terms.
*Correspondence: Zhongsi Hong, aG9uZ3poc0BtYWlsLnN5c3UuZWR1LmNu; Mengdang Ou, b3VtZEBtYWlsLnN5c3UuZWR1LmNu
†These authors have contributed equally to this work
Disclaimer: All claims expressed in this article are solely those of the authors and do not necessarily represent those of their affiliated organizations, or those of the publisher, the editors and the reviewers. Any product that may be evaluated in this article or claim that may be made by its manufacturer is not guaranteed or endorsed by the publisher.
Research integrity at Frontiers
Learn more about the work of our research integrity team to safeguard the quality of each article we publish.