- 1China Animal Health and Epidemiology Center, Qingdao, China
- 2Key Laboratory of Animal Biosafety Risk Prevention and Control (South), Ministry of Agriculture and Rural Affairs, Qingdao, China
- 3Key Laboratory of Animal Biosafety, Qingdao, China
- 4Qingdao Yebio Biological Engineering Co., Ltd., Qingdao, China
Infectious bronchitis virus (IBV) can cause serious harm to poultry industry. It is belong to Coronaviridae which is highly variable. A kind of emerging recombinant IBV (ahysx-1) has been detected in chicken from China in 2016. To understand the epidemiology and characterization of the emerging recombinant IBV, 35,455 samples of chickens from the 15 provinces in China were collected and detected. One hundred and ninety-six out of the 537 flocks (positive rate, 36.49%), and 908 out of 35,455 samples (positive rate, 2.56%) were positive in the detection. The results showed that the emerging recombinant IBV was pandemic in China. Thirteen emerging recombinant IBV isolates were selected and continuous subcultured to the fourth generation and analyzed by Next-generation sequencing. Compared with the reported sequence of ahysx-1, the genomic analysis showed that multiple position insertions and deletions were in 1a gene, 3b gene, M gene and N gene. The identity of the S gene nucleotide sequence between all the 13 emerging recombinant IBV isolates and reference stain ahysx-1 were 98.1–99.1%, while the identity of amino acid sequence were 98.0–99.8%. To better understand the recombination mechanism of the emerging recombinant IBV, the genomic sequence of the 13 isolates were compared with turkey coronavirus or guinea fowl coronavirus. The results suggest that all the 13 emerging recombinant IBV isolates were likely to be the recombination of turkey coronavirus or guinea fowl coronavirus with IBV. Turkey coronavirus or guinea fowl coronavirus as minor parents are the donors of S gene. The major parents donors of the genome backone of these recombination events were lineages GI-19 or GVI-1 of IBV. One isolate (IBV/chicken/Henan/H1173/2021) was selected for pathogenicity analysis. The results showed that IBV/chicken/Henan/H1173/2021 was avirulent to SPF embryonated eggs, but could cause intestinal symptoms in of chicks. This study provides a foundation for understanding the epidemic situation and characterization of the emerging recombinant IBV. It is of great significance for the prevention and control of avian coronavirus infection.
1 Introduction
Infectious bronchitis (IB) is a highly contagious disease of poultry, which can cause high economic losses in poultry industry (Jackwood, 2012). IBV was first detected in North Dakota, USA, in the 1930s (Schalk and Hawn, 1931). In chickens, IBV damages not only the respiratory system but also the bursae of Fabricius, digestive tract tissues, and the urogenital system. It can cause poor egg quality and even death (Johnson and Marquardt, 1975; Jackwood, 2012).
IBV is a member of the Gammacoronavirus genus, the Coronaviridae family, and the Nidovirales order. The IBV genome consists of a single-stranded positive-senese RNA molecule of approximately 27.6 kb, which encodes four structural proteins, including the spike glycoprotein (S) and envelope (E), membrane (M), and nucleocapsid (N) proteins, as well as four non-structural accessory proteins (3a, 3b, 5a and 5b) (Johnson and Marquardt, 1975). Genetic diversity of IBV is caused by genetic mutations and recombination. The lack of proofreading capabilities of viral RNA-dependent RNA polymerase (RdRp) is responsible for the genetic mutations such as point mutations, insertions and deletions during viral replication (Shi et al., 2000; Liu et al., 2009). Therefore, new genotypes and serotypes of IBV are continually emerging worldwide, even among vaccinated flocks. Genetic analysis based on the S1 gene of IBV has become a common method for virus genotype classification. Until now, phylogenetic classification of IBV strains results in seven genotypes (GI-GVIII) consisting of 37 genetic lineages (Valastro et al., 2016; Jiang et al., 2017; Ma et al., 2019; Molenaar et al., 2020). Genotype GI has the largest number of genetic lineages (n: 29). Genotype GII has two genetic lineages. Genetic recombination, deletion, and insertion drive the evolution of IBV and new variants continue to emerge (Xiong et al., 2024). Several kinds of IBV variants are continuously discovered in recent years (Li et al., 2017; Jang et al., 2018; Domanska-Blicharz et al., 2020). Some recombination events were identified to be between field and vaccine strains (Ren et al., 2020; Gong et al., 2022).
In China, IBV was reported for the first time in the 1980s (Lian et al., 2021). At present, IBV is widespread in poultry. GI-19 (QX), GI-13 (4/91), GVI-1 (TC07-2), GI-7 (TW-I), GI-1 (Mass), and GI-28 (LDT3-A) genotypes are dominant in China (Ji et al., 2020; Lian et al., 2021; Yan et al., 2021). Variant IBVs emerged and have been isolated continuously in China (Xue et al., 2012; Jiang et al., 2020). In 2020, a kind of the emerging recombinant IBV (ahysx-1) with a S gene closely related to that of a turkey coronavirus was detected in a chicken using viral metagenomics (Wang et al., 2020). The S gene of the emerging recombinant IBV showed low similarity (58% identity) to the other IBVs, however higher similarity (>75% identity) to turkey coronaviruses. The skeleton sequences were highly similar (98% identity) to IBV isolates from chickens.
So far, only one genome sequence of the emerging recombinant IBV has been published. The prevalence and characteristics of the virus was unknown. To further investigate the epidemiology and characterization of the emerging recombinant IBV in China, the epidemiology and characterization of the emerging recombinant IBV was analyzed in this study.
2 Materials and methods
2.1 Ethics statement
This study was conducted according to the animal welfare guidelines of the World Organization for Animal Health (Edgar, 2004), and approved by the Animal Welfare Committee of Qingdao Yebio Biological Engineering Co., Ltd. The approval number was YBFL2022001. Swab and drinking-water samples were all collected with permission given by multiple relevant parties, including the Ministry of Agriculture of China, the China Animal Health and Epidemiology Center, and the relevant veterinary sections in the provincial and county government.
2.2 Sample collection and RNA extraction
From 2021 to 2023, a total of 35,455 samples from 20,859 chickens, 9,592 ducks, 2,818 pigeons, 1,973 goose and 193 other birds were collected from the 15 provinces in China, including 35,285 swab samples and 170 drinking-water samples. These samples were collected from 537 live poultry markets (LPMs), poultry farms or slaughter houses. The swab samples were smears taken from both the cloacal and oropharyngeal tracts of poultry (Wang et al., 2017). All the 35,455 samples were clarified by centrifugation at 10,000 rpm for 5 min, and then the supernatants were inoculated in 10-day-old specific-pathogen-free (SPF) chicken embryonated eggs (Shandong Healthtec Laboratory Animal Breeding Company, Jinan, China) via the allantoic sac route. The inoculated eggs were incubated at 37°C for 3 days. After the incubation period, the allantoic fluids of all the inoculated eggs were collected and centrifuged at 12,000 rpm, 4°C for 10 min. The genomic RNA was extracted from 200 μL supernatants of each sample with the QIAxtractor platform using a cador Pathogen 96 QIAcube HT kit (Qiagen, Hilden, Germany) according to manufacturer’s instructions. All the extracted RNA samples were stored at −80°C.
2.3 Emerging recombinant IBV detection
To detect and analyze the emerging recombinant IBVs with the genomic characterization that the S gene is higher homology with Turkey coronavirus, while other genes is higher homology with IBVs, a pair of primers was developed to amplify a 1,373-base fragment including partial S gene (1,187 bases). The forward primer was designed on the 1b gene, and the reverse primer was designed on the s1 gene of ahysx-1 (GenBank number: MK142676). The RT-PCR method could only detect the emerging recombinant strains but not the non-recombinant strains. The forward primer was rIBV-F: 5′-AAAACM(A/C)CTGCACGCAAATTA-3′, and the reverse primer was rIBV-R 5′-TCAAAACCTTCR(A/G)ACACTAACAATA-3′. RT-PCR was performed by Evo M-MLV One Step RT-PCR Kit (Accurate Biotechnology (Hunan) Co., Ltd., Hunan, China; AG11606). The reaction system included 12.5 μL of 2× One-step Master Mix, 1 μL each of the upstream and downstream primers (10 pM), 1 μL of Evo M-MLV RTase, 3 μL of nucleic acids, and 6.5 μL of RNase Free dH2O. RT-PCR was performed with incubation at 50°C for 30 min, followed by denaturation at 94°C for 2 min, and 30 cycles of 94°C for 30 s, 55°C for 30 s, 72°C for 2 min, and 72°C for 5 min. The RT-PCR products were purified with an agarose gel DNA extraction kit, and sequenced directly with the ABI 3730xl DNA Analyzer (Sangon, Shanghai, China). All the extracted RNA from the 35,455 samples were detected. The specific RT-PCR products were identified as emerging recombinant IBV by sequencing and comparison with the reference sequence of ahysx-1.
2.4 Subculture of the emerging recombinant IBVs
A total of 106 positive samples collected from different regions were selected for continuous subculture, and their pathogenicity to SPF chicken embryo was observed at the same time. Only 13 emerging recombinant IBV isolates could be continuous subcultured to the fourth generation and negative in the Avian influenza virus (AIV), Newcastle disease virus (NDV) and J subgroup avian leukemia virus (ALV-J) detection. AIV, NDV and ALV-J were detected by RT-qPCR using the Evo M-MLV One Step RT-qPCR Kit II (Accurate Biotechnology (Hunan) Co., Ltd., Changsha, China; AG11713). The primers and probes in each test reaction are shown in Supplementary Table S1. The reaction system included 10 μL of 2× One Step RT-qPCR Buffer II (Probe), 0.4 μL each of the upstream and downstream primers (10 pM), 0.4 μL of probe (10 pM), 0.4 μL of Evo M-MLV RTase Enzyme Mix II, 0.4 μL of Pro Taq HS DNA Polymerase, 2 μL of nucleic acids, and 6 μL of RNase Free dH2O. The reaction conditions were as follows: reverse transcription for 5 min at 42°C, followed by 30 s at 94°C, followed by 40 cycles of denaturation for 5 s at 94°C, annealing and extension for 30 s at 60°C. Nucleic acids of the related viruses were also detected as positive controls. The detection results were determined according to the judgment standards in published paper listed in Supplementary Table S1. The fourth generation allantoic fluid of the 13 isolates were collected and analyzed in NGS and animal experiments.
2.5 Genome sequencing of the emerging recombinant IBV
The sequencing libraries of the selected 13 emerging recombinant IBV isolates genome were constructed using the Ion Total RNA-Seq kit v2 (Qiu et al., 2019). The DNA library was pretreated using the Ion Personal Genome Machine (PGM) Template OT2 200 Kit (Life Technologies, USA). The libraries were loaded onto an Ion 318 chip and sequenced using the Ion Torrent PGM platform (Life Technologies) with the Ion PGM Sequencing 200 Kit (Life Technologies, USA) (Merriman and Rothberg, 2012). Quality control was autorun by the Ion Torrent PGM platform to delete some low quality reads. The sequencing adaptor of reads was automatically deleted using Torrent Server. The Ion Torrent PGM singleton reads of each library were assembled according to the reference sequence of ahysx-1 (GenBank accession number: MK142676) by CLC genomics workbench (version 12).
2.6 Phylogenetic analysis of the emerging recombinant IBV genome
The assembled emerging recombinant IBV sequences obtained in this study were analyzed using MEGA 6.0. The genome sequences and S gene, M gene, N gene of the 13 emerging recombinant IBV isolates were aligned with 84 genome reference sequences (list in Supplementary Table S2) of Alphacoronavirus, Betacoronavirus, Gammacoronavirus and Deltacoronavirus available in GenBank using the software MUSCLE (Edgar, 2004). Bayesian information criterion (BIC) scores of substitution models and phylogenetic relationships were calculated using the software package MEGA 6.0 (Hall, 2013; Tamura et al., 2013). Phylogenetic relationships were calculated using the model with the lowest BIC score which is assumed to describe the substitution pattern the best. Gaps were handled by partial deletion and bootstrap values were calculated out of 1,000 replicates (Hall, 2013).
2.7 Characterization analysis of S gene, M gene and N gene
Antigenic epitopes in the S1 and S2 subunits of S protein of the emerging recombinant IBVs were predicted and analyzed by the online prediction tool.1 It has been reported that the method has an accuracy of about 75%, which could predict the sequence of possible antigen fragments by simulating the antibody response (Kolaskar and Tongaonkar, 1990).
The M protein transmembrane region of the emerging recombinant IBVs were predicted and analyzed by transmembrane hidden Markov model (TMHMM) using the DTU Bioinformatics online prediction tool.2 TMHMM is one of the most complete and best-performing topological structure prediction methods for transmembrane proteins at present (Chen et al., 2003; Nugent and Jones, 2009). In this study, the original amino acid sequence is encoded through the pre-trained model, and then the topological structure is decoded through the prediction space model. The transmembrane region can be predicted with high accuracy. Seventeen kinases (ATM, CKI, CKII, CaM-II, DNAPK, EGFR, GSK3, INSR, PKA, PKB, PKC, PKG, RSK, SRC, cdc2, cdk5 和 p38MAPK) were predicted and the phosphorylation site of N protein in the emerging recombinant IBV isolates were analyzed by the online tool3 (Dinkel et al., 2011).
2.8 Recombination analysis of the emerging recombinant IBV genome
The recombination of the emerging recombinant IBVs were analyzed by Recombination Detection Programme 4 (RDP4) with various genotypes of IBVs, turkey coronaviruses and guinea fowl coronaviruses, respectively, (Martin et al., 2015). In order to ensure the accuracy of composite analysis, seven calculation methods are used. The calculation result was judged to reliable when p-value ≤10−12 (p < 0.05). When at least five of the seven calculation results were credible, recombination event was considered to be occurred (Thor et al., 2011).
2.9 Pathogenicity assays in SPF chickens
To understand the pathogenicity of the emerging recombinant IBVs, one isolate (IBV/chicken/Henan/H1173/2021) was selected for SPF chickens infection experiments. SPF 1-day-old White Leghorn chickens provided by Qingdao Yebio Biological Engineering Co., Ltd. were randomly divided into two groups of 10 chickens each. One group of SPF chickens was infected with the selected emerging recombinant IBV isolate via intranasally and eye drop inoculation route using 200 μL inoculum (102.65 EID50/0.1 mL) (Callison et al., 2006). The other group was inoculated with sterile PBS as negative controls. All chickens were observed twice a day for clinical symptom including tracheal rales, nasal discharge, coughing, eye irritation and watery diarrhea. Morbidity and mortality were recorded every day.
3 Results
3.1 Epidemiology of emerging recombinant IBV
A total of 908 sequences were obtained and align with the reference sequence of ahysx-1. The similarity between 908 sequences to ahysx-1 stain ranged from 94.1 to 100%. Therefore, the corresponding 908 samples were considered positive for the emerging recombinant IBV. One hundred and ninety-five out of the 537 flocks (group positive rate, 36.49%) and 908 out of the 35,455 samples (individual positive rate, 2.56%) tested positive for emerging recombinant IBV. The 908 emerging recombinant IBV positive samples were obtained from 869 chickens, 22 ducks, 14 pigeons and three geese. The emerging recombinant IBVs have been detected in 14 out of 15 provinces. The detection results were listed in Table 1. The emerging recombinant IBV was widely distributed in flocks, suggesting that the virus was prevalent in China. Chickens are the main host of the emerging recombinant IBV.
3.2 Genome characterization and phylogenetic analysis
The 13 emerging recombinant IBV isolates were sequenced by next-generation sequencing. The genomes were all sequenced by PGM in a single run with the sequencing depth more than 500. The genomic information of the 13 isolates, such as sequence length and GenBank accession numbers were listed in Table 2.
The genome sequences analysis were performed to further investigate the characterization and phylogenetic of the emerging recombinant IBVs. Sequence analysis results demonstrated that the genome structure of 13 emerging recombinant IBVs was 5′-1a,b-S(S1,S2)-3a,b,c(E)-M-5a,b-N-Poly(A)-3′, which was consistent with the reported isolate ahysx-1. Some insertions and deletions were discovered during the analysis of nucleotide sequences with ahysx-1 strain and common used IBV reference stains (Supplementary Figure S1). IBV/chicken/Jiangxi/E1283/2021 and IBV/chicken/Gungdong/G1225/2021 have deletions of 27 nucleotides and 30 nucleotides in the 1a gene which similar to H120 strain (GenBank accession number: NC001451), respectively. Three isolates have deletions of 27 nucleotides in the 3a and 3b genes which similar to ahysx-1strain but different from the common IBV reference strains. The 13 emerging recombinant IBV isolates have two specific deletions of 9 and 3 nucleotides in M gene respectively, similar to ahysx-1 strain but different from the common IBV reference strains. IBV/chicken/Gungdong/G1225/2021 have specific deletion of three nucleotides in the N gene different from other 12 emerging recombinant IBV isolates, ahysx-1 strain and the common IBV reference strains. The percentage of nucleotide sequence similarity of the 13 emerging recombinant IBV isolates is 97.61% on average. Phylogenetic analysis results showed that all the 13 emerging recombinant IBVs belong to Gammacoronavirus (Figure 1). The S gene, M gene and N gene of 13 emerging recombinant IBVs were all derived from Gammacoronavirus (Figure 2).
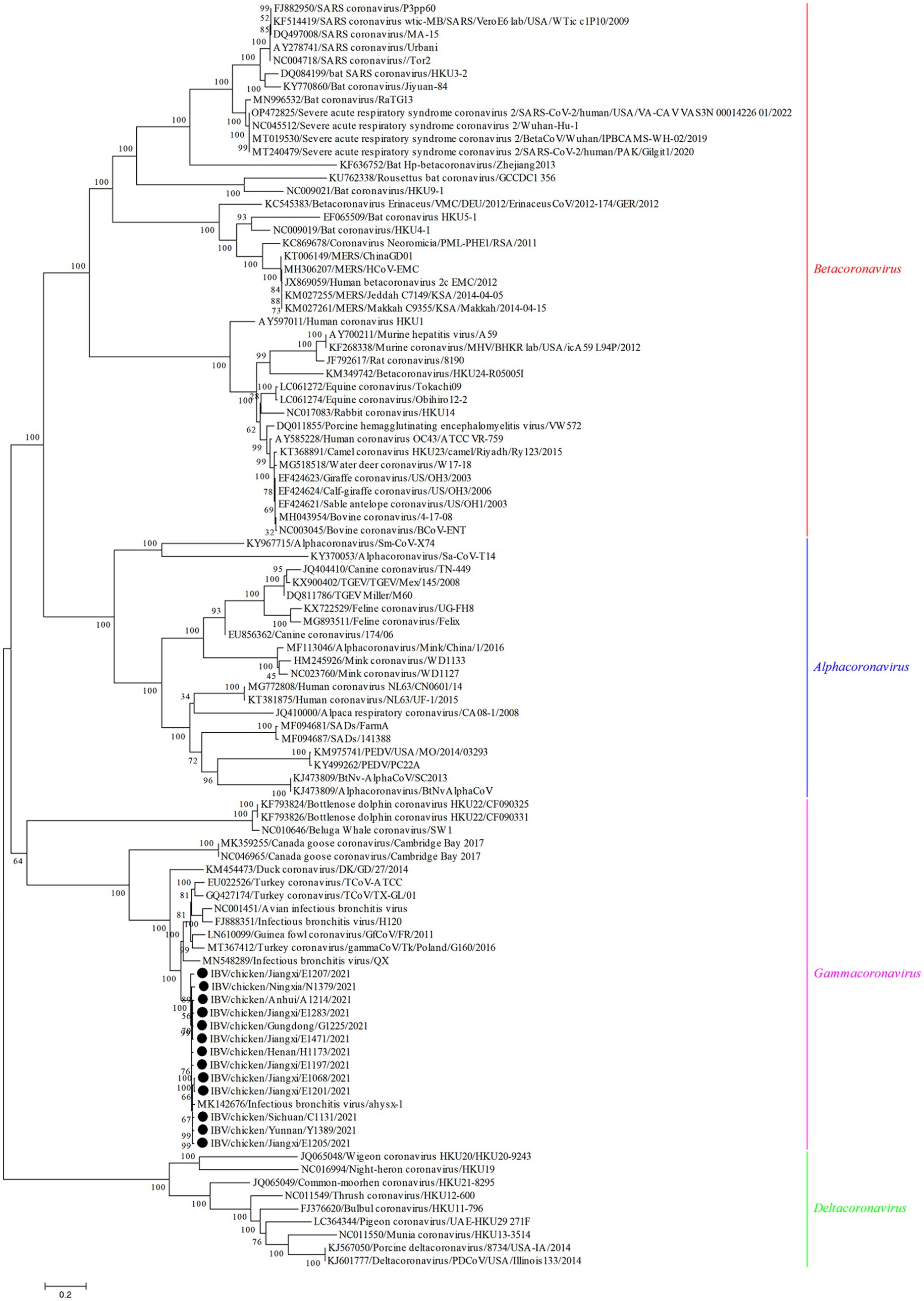
Figure 1. Phylogenetic analysis of genome sequences of 13 emerging recombinant IBVs. The sequences were aligned using Clustal W. The trees were constructed using the model with the maximum likelihood (ML) method, gaps were handled by partial deletion and bootstrap values were calculated out of 1,000 replicates. The 13 emerging recombinant IBV isolates detected in this study were labeled by black solid circles “●.” All the 13 emerging recombinant IBVs belong to Gammacoronavirus.
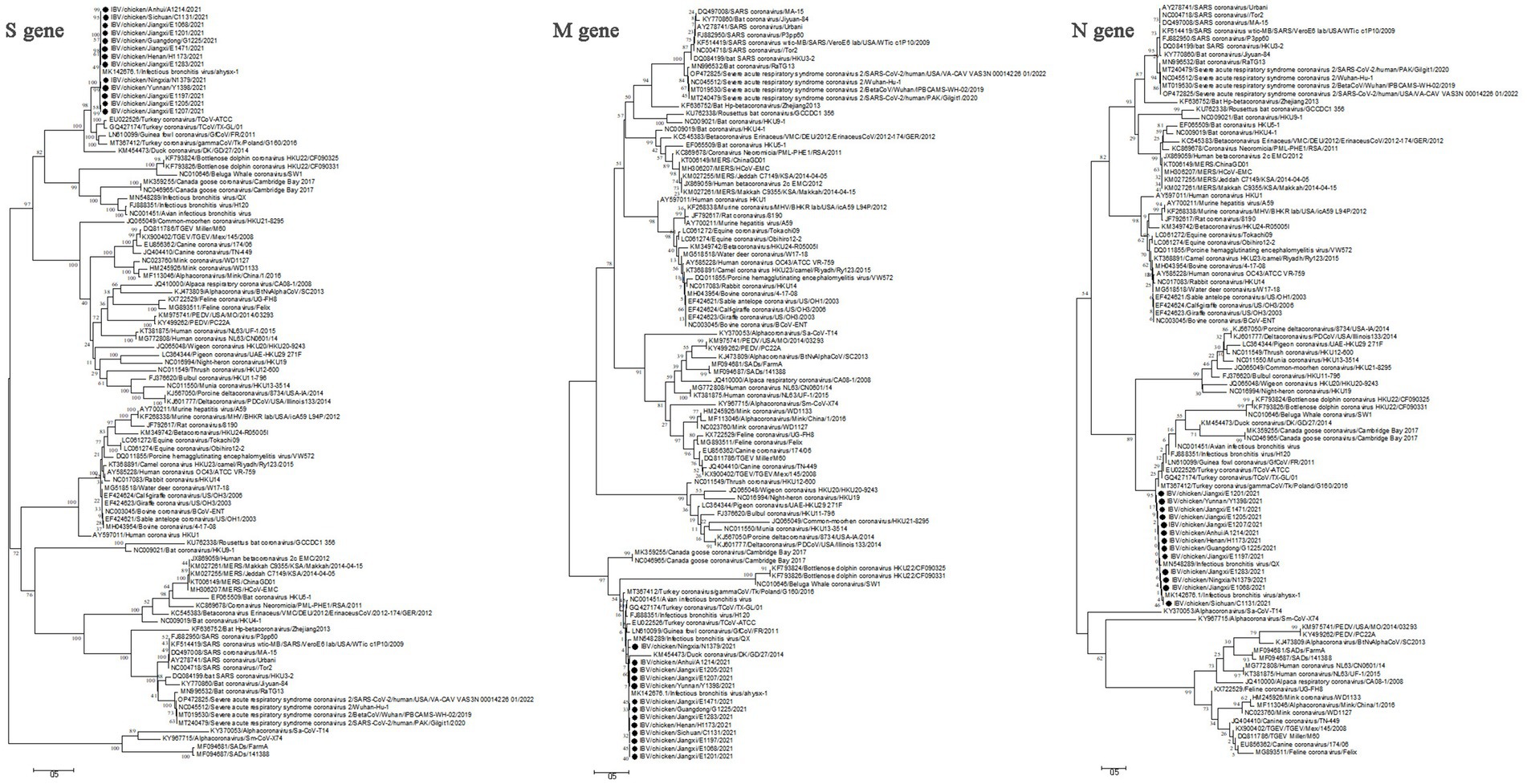
Figure 2. Phylogenetic analysis of S gene, M gene and N gene of 13 emerging recombinant IBVs. The sequences were aligned using Clustal W. The trees were constructed using the model with the maximum likelihood (ML) method, gaps were handled by partial deletion and bootstrap values were calculated out of 1,000 replicates. The 13 emerging recombinant IBV isolates detected in this study were labeled by black solid circles “●.” The S gene, M gene and N gene of 13 emerging recombinant IBVs were all derived from Gammacoronavirus.
3.3 Characterization and phylogenetic analysis of the S gene, M gene, N gene
The gene sequences of the major structural proteins of the 13 emerging recombinant IBV isolates were highly homologous. In this study, all the S gene of 13 isolates contained 3,618 nucleotides which could encode 1,206 amino acids. All the M gene of 13 isolates contained 672 nucleotides which could encode 224 amino acids. Furthermore, all the N gene of 13 isolates contained 1,230 nucleotides which could encode 410 amino acids The percent similarity of S gene, M gene, N gene between the 13 emerging recombinant IBVs and ahysx-1 were listed in Table 2.
The cleavage site of S protein in the 13 emerging recombinant IBV isolates was located at the same amino acid position 537–542. The nucleotide sequence of S1 gene is being widely used to analyze the genetic evolution of IBV. The results showed that the number of peptide in the S1 subunit of 13 isolates was different, while in the S2 subunit was identical. The average propensities of S protein, S1 subunit and S2 subunit were all greater than 1.0, indicating that all of them had potentially antigenic (Supplementary Table S3). It was speculated that the 13 isolates have one potential site for amino acid glycosylation at the same amino acid position. Three transmembrane regions were predicted at the N-terminal of M protein of 13 isolates (Supplementary Figure S2 and Supplementary Table S4). The structural characteristics of M protein were consistent with 13 isolates. Phosphorylated modification was found to be require for the processing of N protein. The phosphorylation sites of N protein in the 13 isolates ranged from 41 to 44.
3.4 Recombination analysis of the 13 emerging recombinant IBV isolates
The recombination of the 13 emerging recombinant IBVs were analyzed by RDP4 with a total of 68 various genotypes of IBVs, turkey coronaviruses and guinea fowl coronaviruses from GenBank, respectively. The results showed that the S gene of the 13 isolates may have undergone recombination events. It suggested that turkey coronavirus (GenBank accession number: EU022526, GQ427174, GQ427176) and guinea fowl coronavirus (GenBank accession number: LN610099) might be the main donors of S gene. The major parents donors of the genome backone of these recombination events were genotype GI-19 or GVI-1 of IBV (Supplementary Figure S3 and Table 3). The results of recombination analysis were similar to the previously reported finding (Wang et al., 2020).
3.5 Pathogenicity analysis of the emerging recombinant IBVs
There were no classical symptoms such as enbryo stunting, curling, urate deposits in the mesonephros of the kidney or death in the continuous subculture of the selected IBV/chicken/Henan/H1173/2021 isolate. The results revealed that the IBV/chicken/Henan/H1173/2021 isolate was avirulent to SPF embryonated eggs. Pathogenicty analysis results in the SPF chickens indicated that all the 10 chickens in the infected group brought about mild diarrhea, but did not die. All chicks appeared paste anus on day 4 (Supplementary Figure S4). At necropsy the intestine revealed congestion but no necrotic foci (Supplementary Figure S4). In control group, all the SPF chickens had no clinical symptoms and no death. All oropharyngeal and cloacal swab samples detected positive with the Ct value ranging from 16.31 to 27.83. In conclusion, IBV/chicken/Henan/H1173/2021 isolate continuous to be excreted through oropharynx or /and cloaca after infected the SPF chickens.
4 Discussion
In this study, the epidemiology and characterization of the emerging recombinant IBVs were analyzed for the first time. The results showed that 196 out of the 537 flocks (positive rate, 36.49%), and 908 out of the 35,455 samples (positive rate, 2.56%) were detected to be positive for emerging recombinant IBV. According to the published paper, the positive rate of IBV was 15.35% in the healthy flocks, and 33.1% in the examined flocks showing respiratory symptoms from China (Li et al., 2021; Lian et al., 2021). Although the individual positive rate of the emerging recombinant IBV was significantly lower than that of IBV, the emerging recombinant IBV has been detected in 14 out of 15 provinces, suggesting that the virus was prevalent in poultry flocks in China. Similar to IBVs, the main host of emerging recombinant IBVs is chicken. Therefore, surveillance should be focused on chicken flocks to understand the prevalence and variation of emerging recombinant IBVs.
Thirteen emerging recombinant IBV isolates were selected for sequencing by NGS to analyze the genetic evolution and biological characteristics. Genome phylogenetic analysis showed that all the 13 isolates belonged to Gammacoronavirus. Although some insertions and deletions were discovered during the analysis of nucleotide sequences, the 13 isolates with high homology. The data also indicate the S gene of the 13 isolates had higher homology with turkey coronavirus than IBV. Therefore, the recombination analysis of 13 isolates was carried out by RDP4. The results showed that 13 isolates may have occurred recombination events with the turkey coronaviruses (11/13) or guinea fowl coronaviruses (2/13) as the minor parent and IBVs as the major parent. Turkey coronavirus or guinea fowl coronavirus were donors of S gene. Interestingly, 10 out of 13 parental IBV strains as the donor of genome backbone belonged to lineage GI-19, which is similar to the previously reported (Wang et al., 2020). The other three IBV strains belonged to lineage GVI-1 which has not been reported previously.
According to the reports, the average substitution rate for coronavirus was about 10−4 substitutions per site 1 year which was high compared to other single-stranded RNA viruses (Pyrc et al., 2006). The cross-host transmission of coronavirus may lead to an increased incidence of recombination events (Pyrc et al., 2006; Woo et al., 2006). In addition, the mutations and recombinations of the large RNA genome in coronavirus can increase the probability for the emergence of novel coronavirus under the right conditions (Pyrc et al., 2006; Su et al., 2016). Furthermore, SARS-CoV-2 is fueled by the emergence of more infectious Omicron variants. Ongoing concerns of emergent variants include possible recombination, as genome recombination is an important evolutionary mechanism for the emergence and re-emergence of human viral pathogens. Therefore, monitoring the evolving coronavirus, including IBV and SARS-CoV-2, genomes especially for recombination is critically important for recognition of abrupt changes to viral attributes including its epitopes which may call for vaccine modifications.
High gene mutation rate and recombination are the important factor to the genetic diversity of IBV (Jackwood et al., 2012). Recombination may create greater genetic variation and lead to the emergence of new strains (Worobey and Holmes, 1999). Potential recombination sites were found in the S proteins of IBV strains, and point mutations in the S1 gene were common, which promotes the evolution of IBV. In recent years, recombination of IBV isolates occurred frequently, with some recombination occurred between vaccine and field isolates (Lee and Jackwood, 2000, 2001; Feng et al., 2017; Li et al., 2017; Zhang et al., 2020).
The IBV/chicken/Henan/H1173/2021 isolate was selected for pathogenicity assay. The results showed that this isolate had no virulence to SPF embryonated eggs. However, the IBV/chicken/Henan/H1173/2021 isolate could cause intestinal symptoms which similar to the clinical symptoms of nephrotropic IBV isolates. The above results showed that the phenotype and pathogenicity of the emerging recombinant IBV isolates may be inconsistent. Because IBV often co-infection with other avian respiratory pathogens in poultry, although the emerging recombinant IBV showed no virulence or low virulence to SPF enbryonated eggs, the further verification is needed to determine whether synergistic effects occur when the emerging recombinant IBV co-infection with other pathogens. IBV and H9N2 AIV are frequently identified in chickens with respiratory symptoms (Kong et al., 2021). Studies have shown that co-infection of IBV and AIV-H9N2 is the most prevalent combination in broiler flocks, can lead to severe clinical symptoms and high mortality (Hassan et al., 2017; Abdelaziz et al., 2019). Therefore, the joint surveillance of IBV and AIV H9N2 will propose effective prevention and control of respiratory disease in poultry.
At present, the prevention and control of IBV mainly rely on the vaccine immunization in China (Feng et al., 2015). Live attenuated vaccines produced by the lineages GI-1 (H120, H52, W93, 28/86, Ma5 and LDT3-A strains), GVI-1 (793B strain) and GI-19 (QXL87 strain) are widely used. The emerging recombinant IBV was detected for the first time from healthy poultry feces in China in 2016 (Wang et al., 2020). In this study, the flocks positive rate of emerging recombinant IBV in poultry has reached 36.49% in China; the emerging recombinant IBVs were detected in almost all the 15 provinces (14/15). The genomic characterization of emerging recombinant IBV is very different from the IBV vaccines used in China. Whether the existing IBV vaccines can produce immune protection against the emerging recombinant IBV was unknown. The pathogenic mechanism of the emerging recombinant IBV should be researched in the further. Further research is needed to develop the new vaccines and antiviral agents should for the prevention and control of the emerging recombinant IBV.
5 Conclusion
In this study, the epidemiological surveillance of emerging recombinant IBV were conducted, and the results indicated that the virus was pandemic in China. The genome sequences of 13 emerging recombinant IBV isolates were obtained by Next-generation sequencing. The analysis results of genome sequence showed that the S gene of 13 emerging recombinant IBV isolates were highly homologous to the reported ahysx-1 isolate. These 13 emerging recombinant IBV isolates may be formed by recombination of turkey coronaviruses or guinea fowl coronaviruses with IBVs, and could lead to intestinal symptoms in chicks. The spread of the emerging recombinant IBV may increase the difficulty of prevention and control of IB. This study provides a foundation for further research the epidemiology, genetic variation, vaccination and molecular evolutionary relationships of IBV.
Data availability statement
All relevant data are within the manuscript and Supplementary material.
Ethics statement
The animal study was approved by Animal Welfare Committee of Qingdao Yebio Biological Engineering Co., Ltd. The study was conducted in accordance with the local legislation and institutional requirements.
Author contributions
SWa: Data curation, Methodology, Software, Validation, Visualization, Writing – original draft, Writing – review & editing. JP: Data curation, Formal analysis, Writing – review & editing. KZ: Data curation, Formal analysis, Writing – review & editing. DC: Resources, Writing – review & editing. JJL: Resources, Writing – review & editing. YC: Resources, Writing – review & editing. QQ: Resources, Writing – review & editing. SWe: Resources, Writing – review & editing. CL: Resources, Writing – review & editing. JS: Resources, Writing – review & editing. FW: Resources, Writing – review & editing. JPL: Investigation, Writing – review & editing. GH: Investigation, Writing – review & editing. HL: Conceptualization, Validation, Writing – review & editing. KW: Conceptualization, Data curation, Formal analysis, Funding acquisition, Methodology, Writing – original draft, Writing – review & editing.
Funding
The author(s) declare that financial support was received for the research, authorship, and/or publication of this article. This project was funded by the National Key Research and Development Program of China (2023YFD1800604).
Conflict of interest
DC and JJL were employed by Qingdao Yebio Biological Engineering Co., Ltd.
The remaining authors declare that the research was conducted in the absence of any commercial or financial relationships that could be construed as a potential conflict of interest.
Publisher’s note
All claims expressed in this article are solely those of the authors and do not necessarily represent those of their affiliated organizations, or those of the publisher, the editors and the reviewers. Any product that may be evaluated in this article, or claim that may be made by its manufacturer, is not guaranteed or endorsed by the publisher.
Supplementary material
The Supplementary material for this article can be found online at: https://www.frontiersin.org/articles/10.3389/fmicb.2024.1456415/full#supplementary-material
Information on primers and probes of RT-qPCR assay for AIV, NDV and ALV-J were shown in Supplementary Table S1. Genome information of the 13 recombinant IBVs isolated in this study were shown in Supplementary Table S2. Antigenic epitope prediction analysis of S protein of 13 emerging recombinant IBVs were shown in Supplementary Table S3. The M protein transmembrane region prediction of 13 emerging recombinant IBVs were shown in Supplementary Table S4 and Supplementary Figure S2. The bootscan analysis of 13 recombination events were shown in Supplementary Figure S3. The insertions and deletions of the 13 emerging recombinant IBV strains with reference sequences were shown in Supplementary Figure S1. The clinical symptoms of chicks after inoculation were shown in Supplementary Figure S4.
Footnotes
1. ^http://imed.med.ucm.es/Tools/antigenic.pl
2. ^https://services.healthtech.dtu.dk/service.php?TMHMM-2.0
References
Abdelaziz, A. M., Mohamed, M. H. A., Fayez, M. M., Al-Marri, T., Qasim, I., and Al-Amer, A. A. (2019). Molecular survey and interaction of common respiratory pathogens in chicken flocks (field perspective). Vet. World 12, 1975–1986. doi: 10.14202/vetworld.2019.1975-1986
Callison, S. A., Hilt, D. A., Boynton, T. O., Sample, B. F., Robison, R., Swayne, D. E., et al. (2006). Development and evaluation of a real-time Taqman RT-PCR assay for the detection of infectious bronchitis virus from infected chickens. J. Virol. Methods 138, 60–65. doi: 10.1016/j.jviromet.2006.07.018
Chen, Y., Yu, P., Luo, J., and Jiang, Y. (2003). Secreted protein prediction system combining CJ-SPHMM, TMHMM, and PSORT. Mamm. Genome 14, 859–865. doi: 10.1007/s00335-003-2296-6
Dinkel, H., Chica, C., Via, A., Gould, C. M., Jensen, L. J., Gibson, T. J., et al. (2011). Phospho.ELM: a database of phosphorylation sites--update 2011. Nucleic Acids Res. 39, D261–D267. doi: 10.1093/nar/gkq1104
Domanska-Blicharz, K., Sajewicz-Krukowska, J., and Lisowska, A. (2020). New PA/1220/98-like variant of infectious bronchitis virus in Poland. Avian Pathol. 49, 380–388. doi: 10.1080/03079457.2020.1754332
Edgar, R. C. (2004). MUSCLE: multiple sequence alignment with high accuracy and high throughput. Nucleic Acids Res. 32, 1792–1797. doi: 10.1093/nar/gkh340
Feng, K., Wang, F., Xue, Y., Zhou, Q., Chen, F., Bi, Y., et al. (2017). Epidemiology and characterization of avian infectious bronchitis virus strains circulating in southern China during the period from 2013-2015. Sci. Rep. 7:6576. doi: 10.1038/s41598-017-06987-2
Feng, K., Xue, Y., Wang, J., Chen, W., Chen, F., Bi, Y., et al. (2015). Development and efficacy of a novel live-attenuated QX-like nephropathogenic infectious bronchitis virus vaccine in China. Vaccine 33, 1113–1120. doi: 10.1016/j.vaccine.2015.01.036
Gong, H., Ni, R., Qiu, R., Wang, F., Yan, W., Wang, K., et al. (2022). Evaluation of a novel recombinant strain of infectious bronchitis virus emerged from three attenuated live vaccine strains. Microb. Pathog. 164:105437. doi: 10.1016/j.micpath.2022.105437
Hall, B. G. (2013). Building phylogenetic trees from molecular data with MEGA. Mol. Biol. Evol. 30, 1229–1235. doi: 10.1093/molbev/mst012
Hassan, K. E., Ali, A., Shany, S. A. S., and El-Kady, M. F. (2017). Experimental co-infection of infectious bronchitis and low pathogenic avian influenza H9N2 viruses in commercial broiler chickens. Res. Vet. Sci. 115, 356–362. doi: 10.1016/j.rvsc.2017.06.024
Jackwood, M. W. (2012). Review of infectious bronchitis virus around the world. Avian Dis. 56, 634–641. doi: 10.1637/10227-043012-Review.1
Jackwood, M. W., Hall, D., and Handel, A. (2012). Molecular evolution and emergence of avian gammacoronaviruses. Infect. Genet. Evol. 12, 1305–1311. doi: 10.1016/j.meegid.2012.05.003
Jang, I., Lee, H. J., Bae, Y. C., Park, S. C., Lee, H. S., and Choi, K. S. (2018). Genetic and pathologic characterization of a novel recombinant TC07-2-type avian infectious bronchitis virus. Avian Dis. 62, 109–113. doi: 10.1637/11764-103017-ResNote.1
Ji, J., Gao, Y., Chen, Q., Wu, Q., Xu, X., Kan, Y., et al. (2020). Epidemiological investigation of avian infectious bronchitis and locally determined genotype diversity in Central China: a 2016-2018 study. Poult. Sci. 99, 3001–3008. doi: 10.1016/j.psj.2020.03.023
Jiang, Y., Cheng, X., Zhao, X., Yu, Y., Gao, M., and Zhou, S. (2020). Recombinant infectious bronchitis coronavirus H120 with the spike protein S1 gene of the nephropathogenic IBYZ strain remains attenuated but induces protective immunity. Vaccine 38, 3157–3168. doi: 10.1016/j.vaccine.2020.01.001
Jiang, L., Zhao, W., Han, Z., Chen, Y., Zhao, Y., Sun, J., et al. (2017). Genome characterization, antigenicity and pathogenicity of a novel infectious bronchitis virus type isolated from South China. Infect. Genet. Evol. 54, 437–446. doi: 10.1016/j.meegid.2017.08.006
Johnson, R. B., and Marquardt, W. W. (1975). The neutralizing characteristics of strains of infectious bronchitis virus as measured by the constant-virus variable-serum method in chicken tracheal cultures. Avian Dis. 19, 82–90. doi: 10.2307/1588958
Kolaskar, A. S., and Tongaonkar, P. C. (1990). A semi-empirical method for prediction of antigenic determinants on protein antigens. FEBS Lett. 276, 172, 172–174. doi: 10.1016/0014-5793(90)80535-q
Kong, L., You, R., Zhang, D., Yuan, Q., Xiang, B., Liang, J., et al. (2021). Infectious bronchitis virus infection increases pathogenicity of H9N2 avian influenza virus by inducing severe inflammatory response. Front. Vet. Sci. 8:824179. doi: 10.3389/fvets.2021.824179
Lee, C. W., and Jackwood, M. W. (2000). Evidence of genetic diversity generated by recombination among avian coronavirus IBV. Arch. Virol. 145, 2135–2148. doi: 10.1007/s007050070044
Lee, C. W., and Jackwood, M. W. (2001). Spike gene analysis of the DE072 strain of infectious bronchitis virus: origin and evolution. Virus Genes 22, 85–91. doi: 10.1023/a:1008138520451
Li, T. T., Li, J. Y., Huang, T., and Ge, X. Y. (2017). Complete genome sequence of a novel strain of infectious bronchitis virus, isolated from chickens in China in 2016. Genome Announc. 5:e01277-17. doi: 10.1128/genomeA.01277-17
Li, Y., Zhuang, Q., Jiang, L., Jiang, W., Peng, C., Jiang, N., et al. (2021). Traceable surveillance and genetic diversity analysis of coronaviruses in poultry from China in 2019. Virus Res. 306:198566. doi: 10.1016/j.virusres.2021.198566
Lian, J., Wang, Z., Xu, Z., Chen, T., Shao, G., Zhang, X., et al. (2021). Distribution and molecular characterization of avian infectious bronchitis virus in southern China. Poult. Sci. 100:101169. doi: 10.1016/j.psj.2021.101169
Liu, S., Zhang, X., Wang, Y., Li, C., Han, Z., Shao, Y., et al. (2009). Molecular characterization and pathogenicity of infectious bronchitis coronaviruses: complicated evolution and epidemiology in China caused by cocirculation of multiple types of infectious bronchitis coronaviruses. Intervirology 52, 223–234. doi: 10.1159/000227134
Ma, T., Xu, L., Ren, M., Shen, J., Han, Z., Sun, J., et al. (2019). Novel genotype of infectious bronchitis virus isolated in China. Vet. Microbiol. 230, 178–186. doi: 10.1016/j.vetmic.2019.01.020
Martin, D. P., Murrell, B., Golden, M., Khoosal, A., and Muhire, B. (2015). RDP4: detection and analysis of recombination patterns in virus genomes. Virus Evol. 1:vev003. doi: 10.1093/ve/vev003
Merriman, B., and Rothberg, J. M. (2012). Progress in ion torrent semiconductor chip based sequencing. Electrophoresis 33, 3397–3417. doi: 10.1002/elps.201200424
Molenaar, R. J., Dijkman, R., and de Wit, J. J. (2020). Characterization of infectious bronchitis virus D181, a new serotype (GII-2). Avian Pathol. 49, 243–250. doi: 10.1080/03079457.2020.1713987
Nugent, T., and Jones, D. T. (2009). Transmembrane protein topology prediction using support vector machines. BMC Bioinformatics 10:159. doi: 10.1186/1471-2105-10-159
Pyrc, K., Dijkman, R., Deng, L., Jebbink, M. F., Ross, H. A., Berkhout, B., et al. (2006). Mosaic structure of human coronavirus NL63, one thousand years of evolution. J. Mol. Biol. 364, 964–973. doi: 10.1016/j.jmb.2006.09.074
Qiu, Y., Wang, S., Huang, B., Zhong, H., Pan, Z., Zhuang, Q., et al. (2019). Viral infection detection using metagenomics technology in six poultry farms of eastern China. PLoS One 14:e0211553. doi: 10.1371/journal.pone.0211553
Ren, M., Han, Z., Zhao, Y., Sun, J., Liu, S., and Ma, D. (2020). Multiple recombination events between field and vaccine strains resulted in the emergence of a novel infectious bronchitis virus with decreased pathogenicity and altered replication capacity. Poult. Sci. 99, 1928–1938. doi: 10.1016/j.psj.2019.11.056
Schalk, A. F., and Hawn, M. C. (1931). An apparently new respiratory disease of baby chicks. J. Am. Vet. Med. Assoc. 78, 413–423.
Shi, Q., Wang, C., and Keirs, R. W. (2000). Genetic relationships of infectious bronchitis virus isolates from Mississippi broilers. Avian Dis. 44, 66–73. doi: 10.2307/1592509
Su, S., Wong, G., Shi, W., Liu, J., Lai, A. C. K., Zhou, J., et al. (2016). Epidemiology, genetic recombination, and pathogenesis of coronaviruses. Trends Microbiol. 24, 490–502. doi: 10.1016/j.tim.2016.03.003
Tamura, K., Stecher, G., Peterson, D., Filipski, A., and Kumar, S. (2013). MEGA6: molecular evolutionary genetics analysis version 6.0. Mol. Biol. Evol. 30, 2725–2729. doi: 10.1093/molbev/mst197
Thor, S. W., Hilt, D. A., Kissinger, J. C., Paterson, A. H., and Jackwood, M. W. (2011). Recombination in avian gamma-coronavirus infectious bronchitis virus. Viruses 3, 1777–1799. doi: 10.3390/v3091777
Valastro, V., Holmes, E. C., Britton, P., Fusaro, A., Jackwood, M. W., Cattoli, G., et al. (2016). S1 gene-based phylogeny of infectious bronchitis virus: an attempt to harmonize virus classification. Infect. Genet. Evol. 39, 349–364. doi: 10.1016/j.meegid.2016.02.015
Wang, Y., Cui, X., Chen, X., Yang, S., Ling, Y., Song, Q., et al. (2020). A recombinant infectious bronchitis virus from a chicken with a spike gene closely related to that of a Turkey coronavirus. Arch. Virol. 165, 703–707. doi: 10.1007/s00705-019-04488-3
Wang, K. C., Zhuang, Q. Y., Qiu, Y., Wang, T., and Chen, J. M. (2017). Genome sequence characterization of pigeon circoviruses in China. Virus Res. 233, 1–7. doi: 10.1016/j.virusres.2017.03.007
Woo, P. C., Lau, S. K., and Yuen, K. Y. (2006). Infectious diseases emerging from Chinese wet-markets: zoonotic origins of severe respiratory viral infections. Curr. Opin. Infect. Dis. 19, 401–407. doi: 10.1097/01.qco.0000244043.08264.fc
Worobey, M., and Holmes, E. C. (1999). Evolutionary aspects of recombination in RNA viruses. J. Gen. Virol. 80, 2535–2543. doi: 10.1099/0022-1317-80-10-2535
Xiong, T., Xie, H., Li, L., Liang, S., Huang, M., Yu, C., et al. (2024). Prevalence, genotype diversity, and distinct pathogenicity of 205 Gammacoronavirus infectious bronchitis virus isolates in China during 2019–2023. Viruses 16:930. doi: 10.3390/v16060930
Xue, Y., Xie, Q., Yan, Z., Ji, J., Chen, F., Qin, J., et al. (2012). Complete genome sequence of a recombinant nephropathogenic infectious bronchitis virus strain in China. J. Virol. 86, 13812–13813. doi: 10.1128/jvi.02575-12
Yan, W., Qiu, R., Wang, F., Fu, X., Li, H., Cui, P., et al. (2021). Genetic and pathogenic characterization of a novel recombinant avian infectious bronchitis virus derived from GI-1, GI-13, GI-28, and GI-19 strains in southwestern China. Poult. Sci. 100:101210. doi: 10.1016/j.psj.2021.101210
Keywords: emerging recombinant IBV, epidemiology, characterization, recombination, pathogenicity
Citation: Wang S, Pan J, Zhou K, Chu D, Li J, Chen Y, Qi Q, Wei S, Li C, Sui J, Wu F, Li J, Hou G, Liu H and Wang K (2024) Characterization of the emerging recombinant infectious bronchitis virus in China. Front. Microbiol. 15:1456415. doi: 10.3389/fmicb.2024.1456415
Edited by:
Lei Tan, Chinese Academy of Agricultural Sciences, ChinaCopyright © 2024 Wang, Pan, Zhou, Chu, Li, Chen, Qi, Wei, Li, Sui, Wu, Li, Hou, Liu and Wang. This is an open-access article distributed under the terms of the Creative Commons Attribution License (CC BY). The use, distribution or reproduction in other forums is permitted, provided the original author(s) and the copyright owner(s) are credited and that the original publication in this journal is cited, in accordance with accepted academic practice. No use, distribution or reproduction is permitted which does not comply with these terms.
*Correspondence: Kaicheng Wang, d2FuZ2thaWNoZW5nQGNhaGVjLmNu