- 1Department of Microbiology and Immunology, Faculty of Pharmacy (Boys), Al-Azhar University, Cairo, Egypt
- 2Department of Basic Medical and Dental Sciences, Faculty of Dentistry, Zarqa University, Zarqa, Jordan
- 3Department of Medical Microbiology and Immunology, Faculty of Medicine, Al-Azhar University (Assiut Branch), Assiut, Egypt
- 4Department Botany and Microbiology, Faculty of Science, Al-Azhar University, Cairo, Egypt
- 5Department of Botany and Microbiology, Faculty of Science, King Saud University, Riyadh, Saudi Arabia
- 6Integrated Molecular Plant Physiology Research, Department of Biology, University of Antwerp, Antwerp, Belgium
- 7Department of Microbiology and Immunology, Faculty of Pharmacy, Fayoum University, Al-Fayoum, Egypt
Commonly used antimicrobial agents are no longer effective due to their overuse or misuse. In addition, many medicinal plant extracts can combat infectious diseases due to their main active constituents or secondary metabolites. The current study aimed to assess the bioactivities of Launaea nudicaulis (LN) leaf extract (LE) against different multi-drug resistant (MDR) Pseudomonas aeruginosa (P. aeruginosa) isolates. The ethyl acetate extract of a Launaea nudicaulis (LN) leaf was analyzed using GC–MS, which identified 27 key bioactive compounds. The major constituents found were as follows: 7-acetyl-6-ethyl-1,1,4,4-tetramethyltetralin, isopropyl myristate, thiocarbamic acid, N,N-dimethyl, S-1,3-diphenyl-2-butenyl ester, hahnfett, cyclopentane acetic acid, 3-oxo-2-pentyl-, methyl ester, hexadecanoic acid, and dotriacontane. Our study demonstrated that the LN leaf was a rich source of other important phytochemicals, including phenolic acids, tannins, saponins, and steroids. The relative biosafety of the L. nudicaulis LE was determined from the elevated inhibitory concentration 50 (IC50) of 262 μg/mL, as calculated from the cytotoxicity assay against the Wi-38 normal cell line. Conversely, 12.7 and 24.5 μg/mL were the recorded low IC50 values for the tested extract against the MCF-7 and Hep-G2 cancerous cell lines, respectively, reflecting its potent activity against the tested cancerous cell lines. Microbiologically, the susceptible P. aeruginosa isolates to the tested extract showed a growth inhibition zone diameter, in the well diffusion assay, ranging from 11.34 ± 0.47 to 26.67 ± 0.47 mm, and a percent inhibition (PI) value of 50–106.2%, reflecting its acceptable activity. In addition, the broth microdilution assay recorded minimum inhibitory concentration (MIC) and minimum bactericidal concentration (MBC) in the ranges of 15.625–1,000 μg/mL and 125–1,000 μg/mL, respectively. In conclusion, the L. nudicaulis LE revealed showed promising activity and high selectivity against P. aeruginosa. Moreover, the extract exhibited natural anticancer activities with safe low concentrations, indicating its potential as a superior candidate for future studies of its active constituents.
1 Introduction
The overuse or misuse of antimicrobial agents in different sectors, particularly clinical healthcare, animal treatment, and agricultural practices, has resulted in the emergence of antimicrobial resistance. As a result, commonly used anti-infectious agents are no longer effective, posing a serious global health concern in the 21st century (Ahmed et al., 2024). Pseudomonas aeruginosa is a highly adaptable bacterium, with a potential risk of developing increased resistance to currently used antimicrobials. Consequently, this pathogen is among the top pathogens that develop a multi-drug resistant (MDR) phenotype in response to antimicrobial misuse or overuse (Coșeriu et al., 2023). Hence, the Infectious Diseases Society of America (IDSA) categorized P. aeruginosa as a causative agent of difficult-to-treat infections (Sathe et al., 2023). Although g/mL were classified common carbapenems are used as a last line of defense to control severe infections, the Centers for Disease Control (CDC) has announced the urgent need to monitor bacterial carbapenem resistance (Coșeriu et al., 2023). The WHO included carbapenem-resistant P. aeruginosa on its pathogens list, emphasizing the critical need for innovative therapeutic strategies against the listed pathogens (El-Sapagh et al., 2023).
Plant extracts have long been recognized as a source of antimicrobial compounds, with many species producing secondary metabolites such as phenolics, terpenoids, alkaloids, and flavonoids that exhibit broad-spectrum activity against bacteria, fungi, viruses, and even protozoa (Cowan, 1999; Vaou et al., 2021; Yetgin, 2024). These plant-derived antimicrobials can disrupt microbial cell membranes, inhibit enzymes, and interfere with other vital cellular processes, making them potentially useful in various applications, including food preservation, healthcare, and agriculture (Khare et al., 2021). The complex and diverse nature of plant-derived antimicrobials can also make it more challenging for microbes to develop resistance as they would need to overcome multiple mechanisms of action simultaneously (Abdallah et al., 2023). In addition, the evolving application of antibacterial phytochemicals against bacterial biofilms resulted from the increasing research on the activity of these natural products (Wu et al., 2024). The use of such products has several benefits, including enhanced patient tolerance, effortless biodegradability, renewability, relatively low cost, minimal side effects, and acceptability (Paneri et al., 2023).
The methanolic extract of Launaea mucronata showed remarkable antimicrobial activity against Candida albicans and Proteus vulgaris. The extract also exhibited high antioxidant and biosafety levels and high activity against hepatocellular cancerous cell lines (Abouzied et al., 2021).
Similarly, Launaea nudicaulis (LN) extract showed strong free radical scavenging activity and demonstrated selective antiproliferative effects against different cancer cell lines compared to normal cells. In addition, the LN methanolic extract demonstrated significant natural insecticide effects (Abouzied et al., 2021).
This study aimed to identify the key compounds in L. nudicaulis leaf extract (LE) using GC–MS and phytochemical analyses and aimed to examine its antibacterial and antibiofilm activities against various MDR P. aeruginosa clinical isolates. In addition, the study aimed to evaluate the extract’s cytotoxic activities toward both normal and cancerous cell lines.
2 Materials and methods
2.1 Identification of the clinical isolates
Primarily identified 24 MDR P. aeruginosa clinical isolates from patients with infected wounds were obtained from the microbiology laboratory at Sayed Galal University Hospital, Al–Azhar University, Cairo, Egypt. The Pseudomonas aeruginosa isolates were identified according to the conventional standard guidelines described by Procop et al. (2017). These standards include initial culturing of clinical specimens on nutrient agar, 5% blood agar, and MacConkey agar, followed by aerobic incubation for 24 h at 37°C. Subsequently, the colony morphology, motility, Gram-stain reaction, and bacterial cell shape and arrangement were examined. Furthermore, the pigment production, the aerobic growth at 40 and 42°C on MacConkey agar, and the growth behavior on cetrimide and TSI agars were evaluated. In addition, the ability of the tested isolates to produce catalase, oxidase, citratase, and tryptophanase enzymes was assessed. Finally, a phenotypic evaluation of the obtained P. aeruginosa clinical isolates’ susceptibility to imipenem was aseptically performed using the broth microdilution protocol described by the Clinical and Laboratory Standard Institute (2020) recommendations. The assayed isolates with an imipenem minimum inhibitory concentration (MIC) of ≥8 μg/mL were classified as carbapenem-resistant P. aeruginosa.
2.2 Plant material and preparation of the crude extract of L. nudicaulis leaves
Launaea nudicaulis (L.) Hook was collected from the arid region at Ain-Sokhna Road, Suez, Egypt. The identification of the plant used in this study was carried out by Prof. Dr. Abdou Marie Hamed at the Botany and Microbiology Department, Faculty of Science, Al-Azhar University, Cairo, Egypt. All experimental research and field studies on plants, including the collection of plant materials, comply with relevant institutional, national, and international guidelines and legislation.
Dried LN leaves (20 g) were extracted using 100 mL of ethyl acetate as a solvent. The mixture was allowed to stand for 72 h at room temperature, and then, it was filtered using a Whatman No. 2 filter paper. After the filtration stage, the filtrate was evaporated and left to dry at room temperature. Then, the crude extract was stored at 4°C until use (Gennaro, 2008).
2.3 GC–MS analysis
The leaf extract of LN was first solubilized in CH3OH, then dried using anhydrous Na2SO4, and finally passed through a 0.45 μm syringe filter before injection into a GC–MS system. The GC–MS system used was a Trace GC Ultra-ISQ system (Thermo Scientific, United States). The initial column temperature was set at 70°C, then increased to 280°C at a rate of 5°C per min and held for 2 min, followed by a further increase to 300°C at a rate of 10°C per min. The extracted components were identified and quantified by comparing their mass spectra and retention times to the databases of known compounds from the Wiley 09 and NIST 11 libraries (Rivero-Cruz et al., 2020).
2.4 Phytochemical analysis
The test samples were subjected to phytochemical analysis to determine qualitatively the presence of chemical constituents, as described in the works of Trease and Evans (1989), Harborne (1998), and Sofowara (1993).
2.4.1 Total phenolic acids content determination
The total phenolic content (TPC) was determined using the Folin–Ciocalteu (FC) method, as described by Makkar (2003). Fifty μL of the L. nudicaulis LE was aliquoted into test tubes, and the volume in each tube was adjusted to 1 mL using distilled water. To each tube, including the blank one, 0.5 mL of FC (1 N) was added and allowed to stand for 5 min at 25°C. Then, 2.5 mL of a 5% Na2CO3 solution was added to all the tubes, including the blank one. The samples were incubated in the dark for 40 min at 25°C. The absorbance of the samples was measured at 725 nm using a spectrophotometer.
2.4.2 Total flavonoid content determination
The total flavonoid (TF) content was determined using AlCl3, as described by Zhishen et al. (1999). The L. nudicaulis LE (0.5 mL) was pipetted into a series of test tubes, and the volume in each tube was adjusted to 1 mL with distilled water. Then, 150 μL of a 5% NaNO2 solution was added to all the tubes, and the tubes were allowed to incubate for 5 min at 25°C. Next, 150 μL of a 10% AlCl3 solution was added to all the test tubes, and the tubes were incubated for 6 min at 25°C. After this, 2 mL of a 4% NaOH solution was added to all the tubes, and the final volume was made up to 5 mL with distilled water. The test tubes were vortexed and allowed to stand in distilled water for 15 min. The absorbance of the samples was measured at 510 nm using a spectrophotometer.
2.4.3 Total tannins content determination
The tannin content (TC) was determined using the Folin–Denis (FD) method, as described by Makkar (2003). To measure the non-tannin phenolics (NTP), 0.5 mL of the LE and 0.5 mL of distilled water were added to 0.1 g of polyvinyl polypyrrolidone (PVPP) at 0°C. The mixture was then incubated at 4°C for 4 h and centrifuged for 10 min. The supernatant, which contained the NTP, was collected. For tannin determination, 0.5 mL of the FD reagent (1 N) and 100 μL of the NTP extract were combined, and the volume was made up to 1 mL with distilled water for each sample, including the blank. After mixing, the samples were allowed to stand at 25°C for 5 min. Then, 2.5 mL of a 5% Na2CO3 solution was added to all the test tubes, including the blank. The tubes were mixed again and incubated at 25°C for 40 min in the dark. The absorbance of the samples was measured at 725 nm using a spectrophotometer.
2.4.4 Total flavonol content determination
The total flavonol content in the L. nudicaulis LE was determined using AlCl3, as described by Miliauskas et al. (2004). To the sample, 2 mL of an AlCl3 solution and 6 mL of a sodium acetate (CH3COONa) solution were added. The mixture was then incubated at 20°C for 2 h. The absorbance of the samples was measured at 440 nm using a UV–Vis spectrophotometer.
2.4.5 Total steroid content determination
A 5 g sample of the dried L. nudicaulis extract was weighed and hydrolyzed by boiling it in 50 mL of a hydrochloric acid solution for 30 min. The solution was then filtered. An equal volume of ethyl acetate was added to the filtrate, and the mixture was mixed well and allowed to separate into layers. The ethyl acetate layer, containing the extracted compounds, was recovered, while the aqueous layer was discarded. The ethyl acetate layer was dried for 5 min at 100°C in a steam bath, and the steroids were then extracted by heating with concentrated amyl alcohol. This resulted in a turbid mixture. The mixture was weighed, and the steroid content was calculated and expressed as mg/100 g of dry weight of the plant sample, as described by Harborne and Harborne (1973).
2.4.6 Total alkaloid content determination
The total alkaloid content of the dried L. nudicaulis extract was measured quantitatively using the method described by Harborne and Harborne (1973). A 1 g sample of L. nudicaulis powder was mixed with a 4:1 ratio of 70% ethanol and glacial acetic acid. The mixture was allowed to stand for at least 6 h and then filtered. The alkaloids present in the supernatant were precipitated by the dropwise addition of a concentrated ammonia solution. The precipitated alkaloids were then filtered on a pre-weighed scale and dried in an oven at 70°C until they reached a constant weight. The alkaloids content was calculated and expressed as mg/100 g of dry weight of the plant sample.
2.4.7 Total saponin content determination
Approximately 50 mg of the dried L. nudicaulis extract was added to 25 mL of 20% ethanol. The sample was heated on a magnetic stirrer for 4 h at a temperature of 55°C, with continuous stirring. The mixture was then filtered, and the residue was re-extracted with an additional 50 mL of 20% ethanol. The filtrates were combined and concentrated at 90°C to a volume of 10 mL. The concentrated filtrate was then mixed with 5 mL of diethyl ether, and the diethyl ether layer was removed. The remaining aqueous layer was stored. This purification step was repeated. The remaining aqueous layer was then mixed with 15 mL of butanol, forming two layers. The butanol layer was separated, and the aqueous layer was filtered again with an additional 15 mL of butanol. The combined butanol layers were mixed with 5 mL of 5% NaCl. The solution was then evaporated in a water bath, and the sample was dried in an oven until a constant weight was reached. The saponin content was calculated and expressed as mg/100 g of dry weight of the plant sample, as described by Ajiboye et al. (2013).
2.5 Cytotoxicity and anticancer effects
The growth inhibitory activity of the L. nudicaulis LE was evaluated using 3-(4,5-dimethylthiazol-2-yl)-2,5-diphenyletetrazolium bromide (MTT) assay, as described by van de Loosdrecht et al. (1994). Normal human diploid (WI-38) cell lines were used for the assessment of the cytotoxic effect, while breast cancerous (MCF-7) cell lines and hepatocellular carcinoma (Hep-G2) cell lines were used to determine the anticancer activity of the L. nudicaulis LE. The optical density (OD) of each tested cell line at 560 nm was measured and used for cell viability or inhibitory percentage calculation, following Equations 1 or 2, respectively.
2.6 Antibacterial activity of the L. nudicaulis LE against the P. aeruginosa clinical isolates
For the preparation of the inoculum of each tested P. aeruginosa clinical isolate, several pure colonies of a fresh culture were aseptically suspended in sterile normal saline and adjusted to 0.5 McFarland standard turbidity (1 × 108 CFU/mL).
2.6.1 Primary screening for antibacterial activity
The primary in vitro activity of the L. nudicaulis LE against the MDR P. aeruginosa isolates was evaluated using agar well diffusion assay, which was conducted as three independent tests, according to Assefa et al. (2024). In brief, each sterile Mueller–Hinton agar (MHA) plate was aseptically inoculated with each tested bacterial inoculum using a sterile cotton swab to obtain a uniform lawn bacterial growth form. Then, on the agar surface, equidistance three wells were made using a sterile borer of a diameter of 6 mm. One well was filled with 80 μL of the 2000 μg/mL tested L. nudicaulis LE dissolved in dimethyl sulfoxide (DMSO), while the wells containing DMSO and the wells containing ceftriaxone (30 μg/mL) were included as negative controls and positive controls, respectively. Before aerobic incubation at 37°C for 24 h, the L. nudicaulis LE was allowed to pre-diffuse into the MHA by keeping the inoculated plates in a refrigerator for 30 min. Each inhibition zone diameter (IZD) was measured in millimeters (mm), calculated as the mean of triplicate results, and presented as the mean ± standard deviation (mean ± SD). Finally, the activity index (AI) for the L. nudicaulis LE against each tested isolate was calculated using Equation 3. In addition, the percent inhibition (PI) of the L. nudicaulis LE against the tested isolates was estimated using Equation 4, as described by Munazir et al. (2012).
2.6.2 Quantitative antibacterial activity
The minimum concentrations of the tested L. nudicaulis LE required to inhibit the growth or destroy the tested clinical isolates were inspected using the broth microdilution method (CLSI, 2020). In brief, a stock solution of the tested L. nudicaulis LE (1,000 μg/mL) in DMSO was prepared, and a 2-fold serial dilution was conducted in each row of sterile 96-well microtiter plates using sterile Mueller–Hinton broth (MHB). In each row, 10 μL of the tested clinical isolate inoculum was added to each tested well. In addition, wells containing MHB and DMSO inoculated with the tested isolate without the L. nudicaulis LE served as positive controls, while the negative control consisted of un-inoculated MHB containing the L. nudicaulis LE and DMSO. After incubation at 37°C for 24 h, 40 μL of a resazurin solution (0.015%) was added to each well, and the plates were incubated for 2 h at 37°C. In each row, the lowest concentrations of the L. nudicaulis LE that exhibited no observable change in the color of resazurin to red were designated as the MIC. A 10 μL suspension from the wells representing the MIC and the higher concentrations was inoculated onto MHA, followed by incubation at 37°C for 24 h. The lowest concentration causing no visible bacterial growth on the MHA was designated as the minimum bactericidal concentration (MBC) of the L. nudicaulis LE. The interpretation of the antibiosis effect of the tested L. nudicaulis LE, either bacteriostatic or bactericidal, was clarified after the calculation of the MIC index (MICi) using Equation 5. An MICi value of ≤2 indicated the extract’s bactericidal activity, while an MICi value of ≥4 indicated its bacteriostatic effect (Shehabeldine et al., 2023)
In addition, to illustrate the antibacterial activity of the L. nudicaulis LE caused either by its selective toxicity toward the tested microorganisms or due to its general toxic effect, the selectivity or therapeutic index (TI) of the L. nudicaulis LE was calculated, as described by Adamu et al. (2014) following Equation 6.
2.7 Antibiofilm assay
The sterile 96-well microtiter plate was used for crystal violet (CV) assay to evaluate the biofilm formation level and the activity of the L. nudicaulis LE against various P. aeruginosa biofilm formations. In brief, several colonies of each tested bacterial isolate were inoculated into MHB and incubated at 37°C for 24 h. Each bacterial culture of the 0.5 McFarland standard (1.5 × 108 CFU/mL) was then diluted 1:100 using the sterile MHB. For the determination of the biofilm formation category, a triplicate assay for each tested isolate cultured on the MHB was incubated at 37°C for 24 h, where the wells containing bacterial untreated MHB is considered negative control (N). To determine the effect of the tested L. nudicaulis LE on the biofilm formation, the same assay was conducted in the presence of a 0.5 MIC of the plant extract. After discarding the planktonic bacterial suspension and the excess nutrient medium, the OD at 620 (OD620) of each CV–stained bacterial biofilm was determined using an ELISA reader. The optical density cut-off (ODc) of the negative control was calculated as the mean OD plus three times the SD (Kamali et al., 2020). Bacterial categorization into non- (OD < ODc), weak (OD < OD < 2ODc), moderate (2OD < OD < 4ODc), or strong (OD > 4ODc) biofilm forming was then recorded. In addition, the obtained OD was used to calculate the percentage change in the biofilm formation caused by the L. nudicaulis LE (Elkady et al., 2024) using the Equation 7.
2.8 Statistical analysis
The data obtained from all experiments, conducted in triplicate, were presented as the mean ± standard deviation (mean ± SD) and analyzed using SPSS for Windows version 20 (Statistical Package for Social Services, Chicago, IL, United States). The graphs were prepared using GraphPad Prism statistical software version 8. The activity index was determined to compare the antibacterial activity of the L. nudicaulis LE with standard antibacterials. In addition, the MIC index and therapeutic index were calculated to assess the antibiosis activity and biosafety of the L. nudicaulis LE, respectively.
3 Results and discussion
3.1 Confirmation and screening for carbapenem-resistant P. aeruginosa
Twenty-four P. aeruginosa clinical isolates, designated as P1–P24, were identified. These phenotypically confirmed non-lactose fermenting, non-hemolytic isolates were primarily characterized as motile, Gram-negative bacteria, with typical yellowish-blue pigmented, large, round, and opaque colonies with irregular margins and a distinctive fruity odor. In addition, these isolates were able to grow aerobically on cetrimide agar and MacConkey agar at either 40 or 42°C, as well as on TSI agar, producing a red slant and butt. Furthermore, phenotypic characterization were confirmed by the positive results for oxidase, catalase, and citratase enzyme production. According to the broth microdilution assay findings in our study, four isolates, P8, P21, P22, and P23, were categorized as carbapenem-resistant P. aeruginosa. Similarly, the P. aeruginosa clinical isolates were initially identified in the microbiology laboratory based on their motility and growth behavior on MacConkey and blood agar plates, and they were further identified according to their characteristic biochemical patterns in the oxidase, catalase, citrate, and TSI tests (Abdi et al., 2024). In addition, the P. aeruginosa isolates were selectively recovered using cetrimide agar. All isolates grew as a non-lactose fermenter on MacConkey agar and produced pigments on MHA, exhibiting a diagnostic yellowish-green to bluish-green color and a grape-like odor. Biochemically, these isolates were strongly positive for the production of oxidase, catalase, and citratase enzymes (Ezeador et al., 2020). These results underscored the importance of microscopic, cultural, and biochemical characteristics in the phenotypic identification of P. aeruginosa clinical isolates.
3.2 Phytochemicals screening of the L. nudicaulis LE
The results shown in Tables 1, 2 indicated the presence of compounds such as flavonoids, polyphenols, alkaloids, and quinones in LN. Furthermore, LN exhibited significant levels of various bioactive compounds such as flavonoids, phenolic acids, tannins, alkaloids, saponins, and steroids. Our findings align with those of Rani et al. (2023), who found that the methanol extract of LN contains a significant number of flavonoids. These compounds are often associated with antioxidant, anti-inflammatory, and other health-promoting properties (Hilal et al., 2024). The presence of glycosides, polyphenols, and other bioactive compounds suggests potential applications in pharmaceuticals, nutraceuticals, or functional foods. The results shown in Tables 1, 2 also indicated the absence of cardiac glycosides, anthraquinones, and anthocyanins. The phytochemicals in LN vary according to the type of solvents used: Aspidofractinin and tocopherol were detected in the chloroform extract of LN (Ramdan and Abdallah, 2017), while dihydro-isosteviol-methylester and flavone-4’-OH,5-OH,7-di-O-glucoside were found in the methanol extract of LN (Ramdan and Abdallah, 2017).
3.3 GC–MS of the L. nudicaulis LE
The ethyl acetate extract of the LN plant contained 27 compounds that were identified by the GC mass analysis as being critically important. The major compounds included 7-acetyl-6-ethyl-1,1,4,4-tetramethyltetralin (20.61%), isopropyl myristate (7.69%), thiocarbamic acid, N,N-dimethyl, S-1,3-diphenyl-2-butenyl ester (6.48%), hahnfett (3.56%), cyclopentane acetic acid, 3-oxo-2-pentyl-, methyl ester (2.74%), hexadecanoic acid (2.58%), and dotriacontane (2.06%) (Table 3 and Figure 1). The compound 7-acetyl-6-ethyl-1,1,4,4-tetramethyltetralin, characterized by its tetralin molecular structure, is notable for its potent antioxidant and antimicrobial activities (Bester, 2009). Isopropyl myristate, a fatty acid ester, is widely employed in cosmetics and pharmaceuticals owing to its ability to moisturize and reduce friction through anti-inflammatory effects (Purnamawati et al., 2017). The thiocarbamic acid functional group consists of a sulfur atom double-bonded to a carbon atom, with two organic substituents attached. Thiocarbamic acid derivatives have been investigated for potential uses as pesticides, fungicides, pharmaceuticals, and in industrial applications (Ajiboye et al., 2022). A cyclopentane ring provides a rigid, cyclic scaffold that can influence the three-dimensional conformation and intermolecular interactions of a molecule. This can be important for binding to biological targets. The presence of the 3-oxo and 2-pentyl substituents on the cyclopentane ring further diversifies the chemical structure and enhances the potential biological activities, including anti-inflammatory, analgesic, antimicrobial, and anticancer effects, among others (Asif, 2016). A 16-carbon chain length is typical for many naturally occurring fatty acids, providing a good balance of physicochemical properties for biological functions. Hexadecanoic acid exhibits inhibitory effects against certain bacteria, fungi, and viruses (Orhan et al., 2011). The ethyl acetate extract of Launaea nudicaulis has been found to have several medicinal uses, including antidiabetic activity, pharmacological effects, and nutraceutical relevance (Mashlawi et al., 2024).
3.4 Cytotoxicity and anticancer effects
Chemotherapeutic agents that kill or inhibit the proliferation of cancerous cells have the drawback of being unable to differentiate between normal cells and malignant cells. As a result, they affect both cell types, leading to elevated toxicity and adverse effects (Zhong et al., 2021). In the present study, the low cytotoxicity of the L. nudicaulis LE, at different concentrations in the range of 31.25–1,000 μg/mL, toward the Wi-38 normal cell lines was evident. Notably, the viability of the cells improved with exposure to the elevated concentrations of the L. nudicaulis LE, with a promising inhibitory concentration 50 (IC50) value of about 262 μg/mL. Furthermore, the treatment of the MCF-7 and Hep-G2 malignant cell lines with the serially diluted L. nudicaulis LE in the range of 7.81–250 μg/mL demonstrated a significant anticancer activity. The viability percentage in both cell lines decreased after treatment with increased L. nudicaulis LE concentrations. Relatively low and promising IC50 values of approximately 12.7 and 24.5 μg/mL for MCF-7 and Hep-G2, respectively, were recorded (Figure 2).
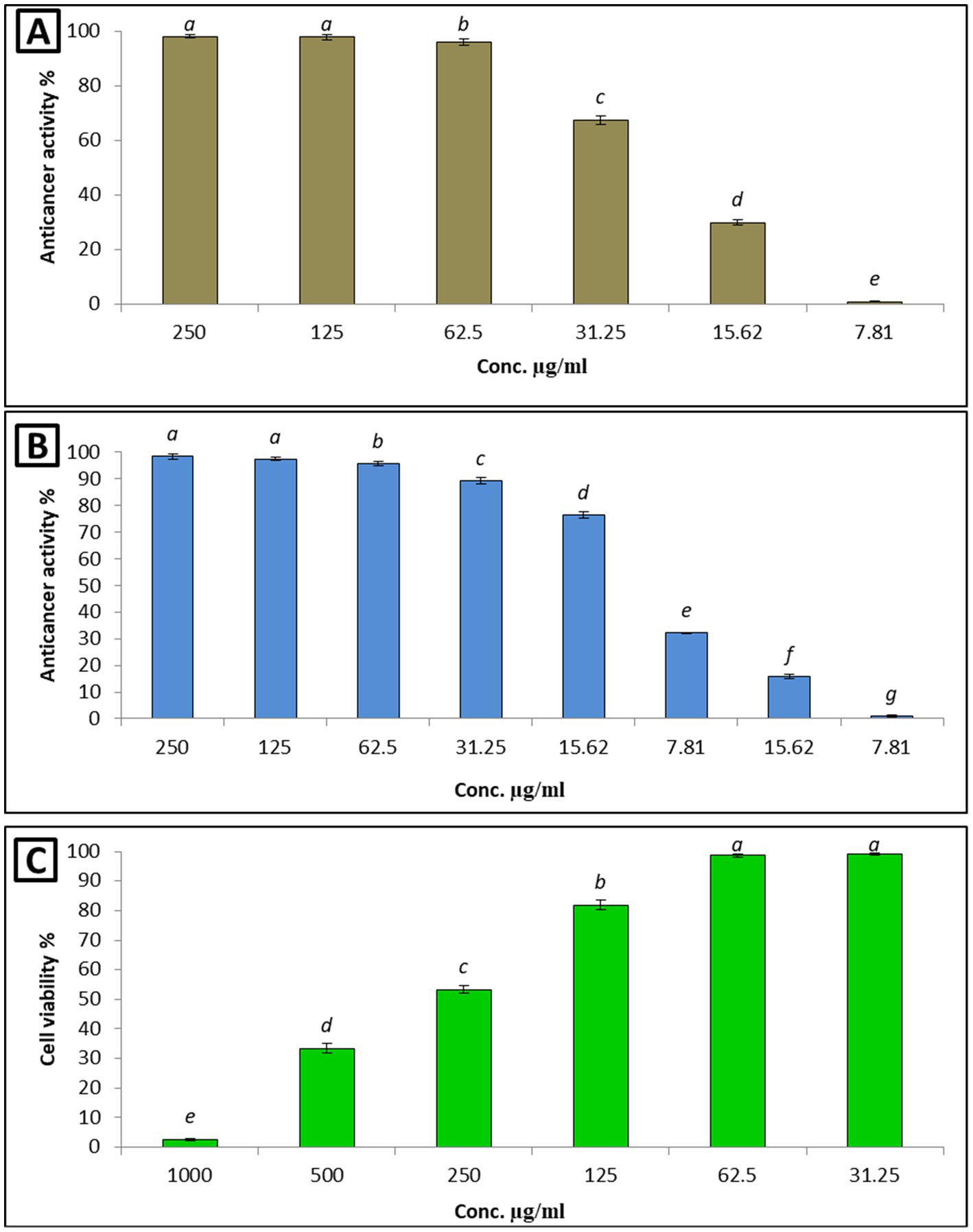
Figure 2. Anticancer effects of the different L. nudicaulis LE concentrations against Hep-G (A) and MCF-7 (B) cancerous cell lines, and its cytotoxicity on Wi-38 as a normal cell line (C).
Our findings align with those of (Mashlawi et al., 2024) a similar study conducted by Mashlawi et al. (2024), in which the in vitro cytotoxicity of the methanolic extract of L. nudicaulis against Wi-38, MCF-7, and HPG-2 cell lines was evaluated. Their results showed a concentration-dependent cytotoxic activity of the L. nudicaulis methanolic extract, with a relatively higher IC50 value of 46.30 and 38.56 μg/mL against MCF-7 and HPG-2 cell lines, respectively. However, their methanolic extract exhibited a simple inhibition (14.06%) on WI-38 normal cells. In addition, the ethyl acetate L. nudicaulis LE exhibited a respectable activity against another two cancerous cell lines, human colon carcinoma (HT-29) and human promyelocytic leukemia (HL-60), with IC50 values of approximately 10.7 and 12.40 μg/mL, respectively (Elhawary et al., 2023). These findings suggest that our L. nudicaulis LE had a specific ability to inhibit the proliferation of different cancerous cell lines with minimal harm to normal cells. This was likely due to the presence of several anticancer compounds, such as 9-octadecenoic acid, methyl jasmonate, quinindoline, mangostine, cyclohexane, 1,3,5-triphenyl, and 13-docosenamide (Table 3). Therefore, the L. nudicaulis LE could serve as a source of valuable, naturally derived, and efficient antitumor compounds.
3.5 Antibacterial activity
The obtained behavior of the L. nudicaulis LE against the tested MDR P. aeruginosa clinical isolates from the well diffusion and broth microdilution assays is presented in Table 4. The initial antibacterial activity of the L. nudicaulis LE, represented in terms of the zone of inhibition, AI, and PI from the agar well diffusion assay findings (Figure 3), clarified the initial in vitro activity of the 2000 μg/mL ethyl acetate L. nudicaulis LE against 50% (12/24) of the P. aeruginosa clinical isolates. The susceptible isolates exhibited a mean IZD ranging from 11.34 ± 0.47 to 26.67 ± 0.47 mm. Interestingly, the obtained IZD of the ceftriaxone (30 μg/mL) positive control for these isolates was in the range of 15–29 mm. Accordingly, the calculated percentage inhibition of the L. nudicaulis LE against the susceptible isolates was in the range of 50–106.2%, indicating acceptable activity of our extract against the tested isolates. The highest antimicrobial activity, an IZD of 17 ± 0.82 mm and an activity index of 106.2%, with subsequent significant antibacterial effects was observed against the clinical isolate P8. Our results were in accordance with those of Khatri and Chhillar (2015) a relatively related study conducted by Khatri and Chhillar (2015). Their disc diffusion assay findings were dependent on the extraction solvent. Maximum IZDs of 14.95, 14.76, and 13.54 mm were recorded for a 25 mg/mL acetone extract against Serratia marcescens, Staphylococcus aureus (S. aureus), and Salmonella enterica ser. Typhi with PIs of 68, 59, and 74%, respectively. In another study, the evaluation of the antimicrobial activity of a 400 mg/mL methanolic extract of L. nudicaulis revealed an inhibitory effect in the form of variable IZDs of (6.5 ± 0.5 mm), (6.7 ± 0.3 mm), and (10.5 ± 0.5 mm) against Escherichia coli ATCC 35218, S. aureus ATCC 25923, and S. epidermidis ATCC 12228, respectively. In addition, when comparing these findings with the IZD of a standard gentamicin disc (10 μg), PIs of 29, 23, and 46% for E. coli, S. aureus, and S. epidermidis, respectively, were found (Ramdan and Abdallah, 2017). Furthermore, the maximum antibacterial activity of a 1 mg/well L. nudicaulis methanol extract was demonstrated in well diffusion assay against Klebsiella pneumoniae. The measured IZD was 22.5 mm for the methanol extract compared to an IZD of 25.5 mm for the positive control, 10 μg/well of ofloxacin, and the calculated PI was 88% (Rashid et al., 2000). Specifically, the agar diffusion assay clarified the antimicrobial activity of the essential oils from LN against Klebsiella sp., Proteus sp., E. coli, and S. aureus (Fadjkhi et al., 2020). The activity indices could be valuable for assessing the comparative antimicrobial effect of the tested plant extract and could help in deciding the importance of active constituent extraction (Assefa et al., 2024). Our PI findings highlight the promising effects of the evaluated LN extracts or their active constituents against Gram-negative bacteria. The activity of the L. nudicaulis LE against the P. aeruginosa clinical isolates could be correlated with the observed 7-acetyl-6-ethyl-1,1,4,4-tetramethyltetralin compound that possess potent antimicrobial activity and other active constituents shown in Table 3. In addition, the enhanced antibacterial activity of the L. nudicaulis extract could be mainly attributed to different biologically active phytochemical constituents, including terpenoids, flavonoids, tannins, alkaloids, glycosides, saponins, and anthraquinones, which vary depending on the extraction solvent. Synergistic interactions between these constituents could also contribute to the improved activity index of the tested extract. However, there is no evidence for the presence of a direct correlation between the anticancer and antibacterial activities of L. nudicaulis LE.
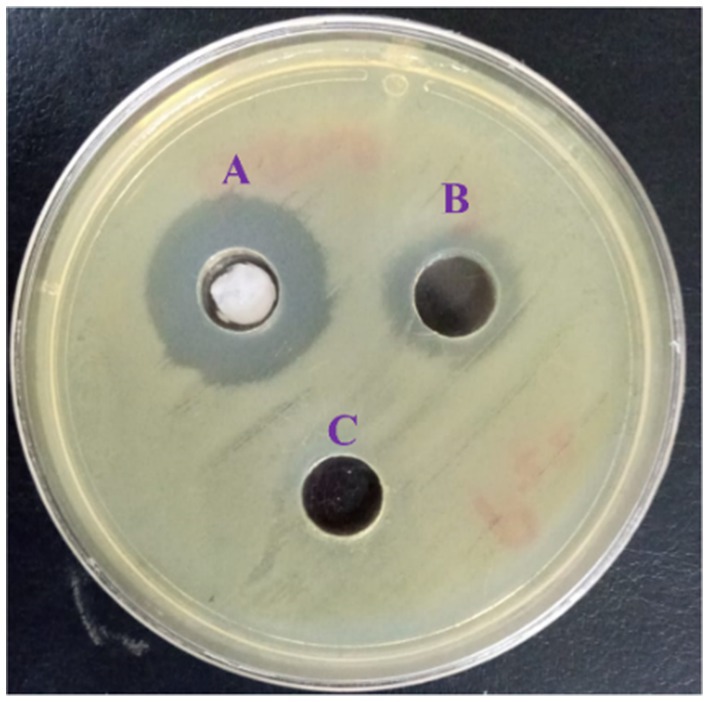
Figure 3. Agar well diffusion assay of the L. nudicaulis LE showing the P. zones of growth inhibition for the aeruginosa clinical isolate (P22). Wells (A) 30 μg/mL ceftriaxone (positive control), (B) 2000 μg/mL L. nudicaulis LE, and (C) DMSO (negative control).
Quantitatively, the broth microdilution assay (Table 4 and Figure 4) showed the inhibitory activities of the L. nudicaulis LE against the MDR P. aeruginosa clinical isolates at different concentrations. The recorded low MIC values, ranging from 15.625 to 1000 μg/mL, demonstrated a high sensitivity of the tested isolates toward the L. nudicaulis LE. In addition, the tested isolates showed low MBC values, ranging from 125 to 1000 μg/mL, along with MIC indices ranging from 1 to 8. This indicated that both bacteriostatic and bactericidal antibiosis activities of the L. nudicaulis LE against the tested isolates depended on the concentration applied. At a high concentration (1000 g/mL), the L. nudicaulis LE exhibited bactericidal activity, while the bacteriostatic effect was observed at a lower concentration. Our findings align with (Khan et al., 2023) those of Khan et al. (2023), which reported the potent antibacterial activity of L. nudicaulis leaf, stem, and root methanolic extracts against S. aureus. In related research, methanol extracts of L. nudicaulis, rich in alkaloids, saponins, and flavonoids, exhibited antibacterial activity against Streptococcus pneumoniae, with an MIC value of 248 μg/mL (Nivas and Boominathan, 2015). In addition, the study conducted by Khatri and Chhillar (2015) reported high antibacterial activity of an LN leaves acetone extract against Serratia marcescens, S. aureus, and Salmonella enterica ser. Typhi, with MIC values of 190, 390, and 190 μg/mL, respectively, and MBC values of 190, 1560, and 190 μg/mL, respectively. The calculated MIC index of 1 for Serratia marcescens and Salmonella enterica ser. Typhi and 4 for of S. aureus indicated that the antibiosis mechanism of the extract was due to its bactericidal activity against the tested Gram-negative bacteria and its bacteriostatic activity against the tested Gram-positive bacteria.
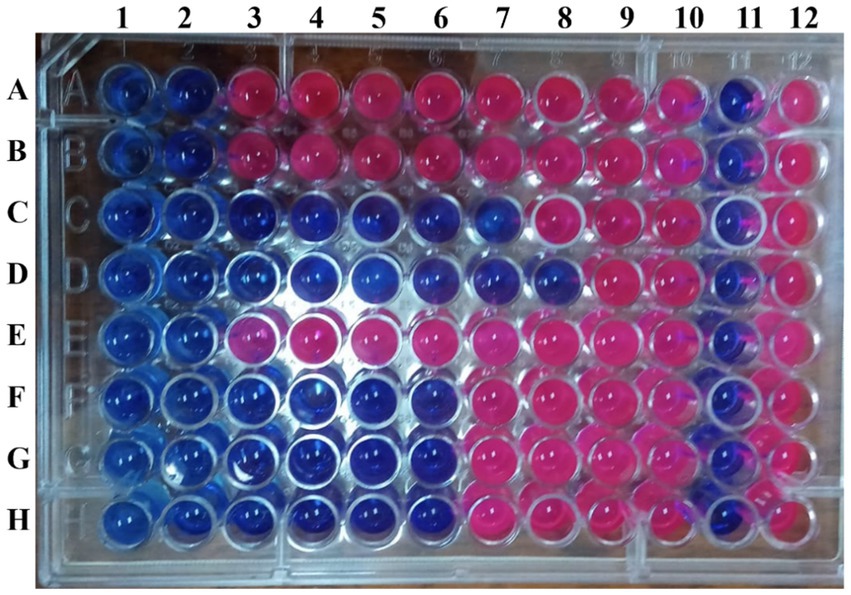
Figure 4. Resazurin-based broth microdilution assay illustrating the inhibitory activities of the L. nudicaulis LE. Rows A-D inoculated with P. aeruginosa P1-P8, respectively. Columns 1–10 containing the 2-fold serially diluted L. nudicaulis LE at the concentrations ranging from 2000 to 3.906 μg/mL. Columns 11 and 12 represent the negative and positive controls, respectively.
Furthermore, the therapeutic indices of the tested L. nudicaulis LE calculated from its effect on a normal human cell line were in the range of 0.262–16.768. The higher therapeutic indices reflect its relative antibacterial selectivity toward the P. aeruginosa clinical isolates. In a relatively related study, the biosafety or low toxicity evaluation of newly tested antimicrobial agents was correlated with their recorded therapeutic indices, which ranged from 2 to 109 (Scaccaglia et al., 2024).
3.6 Antibiofilm activity
Regarding the biofilm formation side, the calculated ODc from the CV assay classified the tested 24 isolates into non (3/24; 12.5%), weak (2/24; 8.3%), moderate (11/24; 45.9%), or strong (8/24; 33.3%) biofilm-forming P. aeruginosa (Table 5). In addition, the majority (P8, P22, and P23) of the carbapenem-resistant isolates (3/4; 75%) were biofilm forming. In the research carried out by Lordelo et al. (2024), the tested P. aeruginosa strains were categorized according to their biofilm-forming capability into non-biofilm producers (3%), weak biofilm producers (9.1%), moderate biofilm producers (21.2%), and strong biofilm formers (66.7%). Another similar study reported a relatively comparable prevalence rate of 18.3, 18.3, 53.0, and 10.4% for strong, moderate, weak, and non-biofilm-forming P. aeruginosa isolates, and 95% of their tested carbapenem-resistant isolates were identified as biofilm formers (Heidari et al., 2022). The variation in biofilm-forming capacities among P. aeruginosa clinical isolates could be attributed to the types of collected specimens, the number of tested isolates, and the specific capabilities of each isolate for biofilm formation. Understanding the growth behavior and biofilm formation capacity of P. aeruginosa isolates could help in overcoming their possible medical device colonization and in ensuring proper eradication of this superbug.
Furthermore, this assay demonstrated the inhibitory activity of the L. nudicaulis LE against biofilm-forming P. aeruginosa in the range of 3.542 ± 0.07–74.403 ± 0.42%. In a related study, Alam et al. (2020) reported that extracts from Clematis grata and Bergenia ciliata, which are rich in catechin flavonoids, efficiently inhibited P. aeruginosa biofilm formation by 80 and 81%, respectively, without affecting viable growth. In addition, the catechin bioactive compound can reduce 72% of P. aeruginosa biofilms’ extracellular DNA content, an essential structural component of bacterial biofilm formation. Similarly, Song et al. (2018) found that negletein flavone, isolated from the leave extract of Scutellaria oblonga, exhibited a 72.3% P. aeruginosa biofilm-reducing ability at a concentration of 64 μg/mL. These findings underscore the potential application of plant extracts as antibacterial agents against biofilms, highlighting the role of bioactive constituents, such as flavonoids and other phenolic compounds, in minimizing bacterial adherence, which is the initial step in bacterial biofilm formation.
4 Conclusion
The development of various MDR bacterial species necessitates the development of potential antimicrobial alternatives. Accordingly, extensive research should be directed to uncover, isolate, and identify various bioactive constituents from widely distributed medicinal plants. The ethyl acetate extract of the L. nudicaulis LE was analyzed using GC–MS, identifying 27 key bioactive compounds, including 7-acetyl-6-ethyl-1,1,4,4-tetramethyltetralin, isopropyl myristate, and others. The LN was also found to be a rich source of various important phytochemicals, such as phenolic acids, tannins, saponins, and steroids. Our study reveals the anticancer activities of L. nudicaulis leaf extract, demonstrating low toxic outcomes in normal cells. On the microbiological side, the extract exhibited promising antibacterial activity, with high selectivity against P. aeruginosa clinical isolates, which could be help control antimicrobial resistance. The results also suggest that L. nudicaulis leaf extract is a promising candidate for future studies that plan to separate, purify, and identify its various active constituents and that plan to independently evaluate its possible therapeutic bioactivity for selecting the most potent therapeutic constituent. In addition, the findings highlight the need for further investigations to clarify the microbial targets and identify the destructive mechanism of the most active constituents.
Data availability statement
The original contributions presented in the study are included in the article/supplementary material, further inquiries can be directed to the corresponding authors.
Author contributions
FE: Formal analysis, Methodology, Resources, Writing – original draft, Writing – review & editing. BB: Methodology, Writing – original draft, Writing – review & editing. AH: Conceptualization, Data curation, Formal analysis, Investigation, Methodology, Resources, Validation, Writing – original draft, Writing – review & editing. MA: Methodology, Writing – original draft, Writing – review & editing. AA: Formal analysis, Methodology, Writing – original draft, Writing – review & editing. AA-A: Funding acquisition, Methodology, Writing – original draft, Writing – review & editing. GA: Data curation, Formal analysis, Writing – original draft, Writing – review & editing. HH: Investigation, Methodology, Validation, Writing – original draft, Writing – review & editing.
Funding
The author(s) declare that financial support was received for the research, authorship, and/or publication of this article. The authors extend their gratitude to the researchers of King Saud University, Riyadh, Saudi Arabia.
Acknowledgments
The authors extend their gratitude to the researchers of King Saud University, Riyadh, Saudi Arabia (supporting project number RSP2024R505). The authors sincerely acknowledge the Faculty of Pharmacy and Faculty of Science, Al-Azhar University, Cairo, Egypt, for providing essential research facilities. The authors also extend their gratitude to Zarqa University, Jordan, for the partial funding of this study.
Conflict of interest
The authors declare that the research was conducted in the absence of any commercial or financial relationships that could be construed as a potential conflict of interest.
The author(s) declared that they were an editorial board member of Frontiers, at the time of submission. This had no impact on the peer review process and the final decision.
Publisher’s note
All claims expressed in this article are solely those of the authors and do not necessarily represent those of their affiliated organizations, or those of the publisher, the editors and the reviewers. Any product that may be evaluated in this article, or claim that may be made by its manufacturer, is not guaranteed or endorsed by the publisher.
References
Abdallah, E. M., Alhatlani, B. Y., De Paula Menezes, R., and Martins, C. H. G. (2023). Back to nature: medicinal plants as promising sources for antibacterial drugs in the post-antibiotic era. Plan. Theory 12:3077. doi: 10.3390/plants12173077
Abdelaziz, A. M., El-Wakil, D. A., Hashem, A. H., Al-Askar, A. A., Abdelgawad, H., and Attia, M. S. (2023a). Efficient role of endophytic aspergillus terreus in biocontrol of Rhizoctonia solani causing damping-off disease of Phaseolus vulgaris and Vicia faba. Microorganisms 11:1487. doi: 10.3390/microorganisms11061487
Abdelaziz, A. M., Sharaf, M. H., Hashem, A. H., Al-Askar, A. A., Marey, S. A., Mohamed, F. A., et al. (2023b). Biocontrol of fusarium wilt disease in pepper plant by plant growth promoting Penicillium expansum and Trichoderma harzianum. Notulae Botanicae Horti Agrobotanici Cluj-Napoca 51, 13302–13323. doi: 10.15835/nbha51313302
Abdi, F. A., Motumma, A. N., Kalayu, A. A., and Abegaz, W. E. (2024). Prevalence and antimicrobial-resistant patterns of Pseudomonas aeruginosa among burn patients attending Yekatit 12 hospital medical College in Addis Ababa, Ethiopia. Plos One 19:e0289586. doi: 10.1371/journal.pone.0289586
Abouzied, A. S., Break, M., Younes, K., Essam, A., Unissa, R., Alafnan, D., et al. (2021). In vitro antimicrobial, anticancer, and apoptosis-inducing effects of the methanolic extract of Launaea mucronata. Notulae Botanicae Horti Agrobotanici Cluj-Napoca 49:12556. doi: 10.15835/nbha49412556
Adamu, M., Naidoo, V., and Eloff, J. N. (2014). The antibacterial activity, antioxidant activity and selectivity index of leaf extracts of thirteen south African tree species used in ethnoveterinary medicine to treat helminth infections. BMC Vet. Res. 10:52. doi: 10.1186/1746-6148-10-52
Ahmed, S. K., Hussein, S., Qurbani, K., Ibrahim, R. H., Fareeq, A., Mahmood, K. A., et al. (2024). Antimicrobial resistance: impacts, challenges, and future prospects. J. Med. Surg. Pub.Health 2:100081. doi: 10.1016/j.glmedi.2024.100081
Ajiboye, T. O., Ajiboye, T. T., Marzouki, R., and Onwudiwe, D. C. (2022). The versatility in the applications of dithiocarbamates. Int. J. Mol. Sci. 23:1317. doi: 10.3390/ijms23031317
Ajiboye, B., Ibukun, E., Edobor, G., Ojo, A., and Onikanni, S. (2013). Qualitative and quantitative analysis of phytochemicals in Senecio biafrae leaf. Int. J. Inv. Pharm. Sci. 1, 428–432.
Alabi, K., Lajide, L., and Owolabi, B. (2018). Biological activity of oleic acid and its primary amide: experimental and computational studies. J. Chem. Soc. 43, 9–18.
Alam, K., Farraj, D. A. A., Mah-E-Fatima, S., Yameen, M. A., Elshikh, M. S., Alkufeidy, R. M., et al. (2020). Anti-biofilm activity of plant derived extracts against infectious pathogen-Pseudomonas aeruginosa PAO1. J. Infect. Public Health 13, 1734–1741. doi: 10.1016/j.jiph.2020.07.007
Alhasan, D. A., Dawood, A. Q., and Alfartossy, H. H. (2019). GC-MS analysis of fungal filtrate crude extracts produced by two species of Aspergillus. J. Educ. Pure Sci. 9, 271–283.
Asif, M. (2016). Pharmacological potential of benzamide analogues and their uses in medicinal chemistry. Modern Chem. Appl. 4:1000194. doi: 10.4172/2329-6798.1000194
Assefa, A., Belay, S., and Kloos, H. (2024). Evaluation of in-vitro antibacterial activity of extracts of Calpurina aurea, Vernonia amygdalina and Rumex nepalensis in Goba district, southeastern Ethiopia. Egyptian J. Basic Appl. Sci. 11, 69–83. doi: 10.1080/2314808X.2024.2312785
Attia, M. S., Sharaf, M. H., Hashem, A. H., Mahfouz, A. Y., Daigham, G. E., Al-Askar, A. A., et al. (2023). Application of Rhizopus microsporus and aspergillus oryzae to enhance the defense capacity of eggplant seedlings against Meloidogyne incognita. Notulae Botanicae Horti Agrobotanici Cluj-Napoca 51, 13300–13323. doi: 10.15835/nbha51313300
Bester, K. (2009). Analysis of musk fragrances in environmental samples. J. Chromatogr. A 1216, 470–480. doi: 10.1016/j.chroma.2008.08.093
Brigelius-Flohé, R., and Traber, M. G. (1999). Vitamin E: function and metabolism. FASEB J. 13, 1145–1155. doi: 10.1096/fasebj.13.10.1145
Calder, P. C. (2010). Omega-3 fatty acids and inflammatory processes. Nutrients 2, 355–374. doi: 10.3390/nu2030355
Cavalcanti, B. C., Bezerra, D. P., Magalhaes, H. I., Moraes, M. O., Lima, M. A. S., Silveira, E. R., et al. (2009). Kauren-19-oic acid induces DNA damage followed by apoptosis in human leukemia cells. J. Appl. Toxicol. 29, 560–568. doi: 10.1002/jat.1439
Chandrasekaran, M., Kannathasan, K., and Venkatesalu, V. (2008). Antimicrobial activity of fatty acid methyl esters of some members of Chenopodiaceae. Zeitschrift für Naturforschung C 63, 331–336. doi: 10.1515/znc-2008-5-604
Clinical and Laboratory Standards Institute. Performance standards for antimicrobial susceptibility testing. 30th ed. CLSI supplement M100. (2020). Wayne PA.
Coșeriu, R. L., Vintilă, C., Pribac, M., Mare, A. D., Ciurea, C. N., Togănel, R. O., et al. (2023). Antibacterial effect of 16 essential oils and modulation of mex efflux pumps gene expression on multidrug-resistant Pseudomonas aeruginosa clinical isolates: is cinnamon a good fighter? Antibiotics 12:163. doi: 10.3390/antibiotics12010163
Cowan, M. M. (1999). Plant products as antimicrobial agents. Clin. Microbiol. Rev. 12, 564–582. doi: 10.1128/CMR.12.4.564
El-Fayoumy, E. A., Shanab, S. M., Gaballa, H. S., Tantawy, M. A., and Shalaby, E. A. (2021). Evaluation of antioxidant and anticancer activity of crude extract and different fractions of Chlorella vulgaris axenic culture grown under various concentrations of copper ions. BMC Compl. Medi. Ther. 21, 1–16. doi: 10.1186/s12906-020-03194-x
El-Sapagh, S., El-Shenody, R., Pereira, L., and Elshobary, M. (2023). Unveiling the potential of algal extracts as promising antibacterial and antibiofilm agents against multidrug-resistant Pseudomonas aeruginosa: in vitro and in silico studies including molecular docking. Plan. Theory 12:3324. doi: 10.3390/plants12183324
Elhawary, S., Hassan, M. H. A., El-Desoucky, S. I., Elwekeel, A., Mohamed, E. I. A., Abdelmohsen, U. R., et al. (2023). Metabolomic profiling and cytotoxic activity of Launaea nudicaulis: molecular docking with topoisomerases. Rev. Bras 33, 432–437. doi: 10.1007/s43450-023-00371-0
Elkady, F. M., Hashem, A. H., Salem, S. S., El-Sayyad, G. S., Tawab, A. A., Alkherkhisy, M. M., et al. (2024). Unveiling biological activities of biosynthesized starch/silver-selenium nanocomposite using Cladosporium cladosporioides CBS 174.62. BMC Microbiol. 24:78. doi: 10.1186/s12866-024-03228-1
Ezeador, C., Ejikeugwu, P., Ushie, S., and Agbakoba, N. (2020). Isolation, identification and prevalence of Pseudomonas aeruginosa isolates from clinical and environmental sources in Onitsha Metropolis, Anambra state. Eur. J. Med. Health Sci. 2:188. doi: 10.24018/ejmed.2020.2.2.188
Fadjkhi, N., Hameurlaine, S., Fellah, O., Djermane, M., Zellagui, A., and Abidi, A. (2020). Chemical composition and antibacterial activity of Algerian Launaea nudicaulis essential oils. Int. J. Chem. Biochem. Sci. 17.
Gennaro, L. (2008). Extraction technology for medicinal and aromatic plant. Trieste: United Nations Industrial Development Organization and the International Centre for Science and High Technology, 6–8.
Harborne, A. (1998). Phytochemical methods a guide to modern techniques of plant analysis. Cham: Springer Science & Business Media.
Harborne, J., and Harborne, J. (1973). The terpenoids. Phytochem. Methods Guide Modern Tech. Plant Anal. 22, 89–131. doi: 10.1007/978-94-009-5921-7_3
Hashem, A. H., Al-Askar, A. A., Abd Elgawad, H., and Abdelaziz, A. M. (2023). Bacterial endophytes from Moringa oleifera leaves as a promising source for bioactive compounds. Separations 10:395. doi: 10.3390/separations10070395
Hashem, A. H., Shehabeldine, A. M., Abdelaziz, A. M., Amin, B. H., and Sharaf, M. H. (2022). Antifungal activity of endophytic aspergillus terreus extract against some fungi causing mucormycosis: ultrastructural study. Appl. Biochem. Biotechnol. 194, 3468–3482. doi: 10.1007/s12010-022-03876-x
Heidari, R., Farajzadeh Sheikh, A., Hashemzadeh, M., Farshadzadeh, Z., Salmanzadeh, S., and Saki, M. (2022). Antibiotic resistance, biofilm production ability and genetic diversity of carbapenem-resistant Pseudomonas aeruginosa strains isolated from nosocomial infections in southwestern Iran. Mol. Biol. Rep. 49, 3811–3822. doi: 10.1007/s11033-022-07225-3
Hilal, B., Khan, M. M., and Fariduddin, Q. (2024). Recent advancements in deciphering the therapeutic properties of plant secondary metabolites: phenolics, terpenes, and alkaloids. Plant Physiol. Biochem. 211:108674. doi: 10.1016/j.plaphy.2024.108674
Hillard Iii, R., and Vollhardt, K. P. C. (1977). Substituted benzocyclobutenes, indans, and tetralins via cobalt-catalyzed cooligomerization of Alpha Omega-diynes with substituted acetylenes. Formation and synthetic utility of trimethylsilylated benzocycloalkenes. J. Am. Chem. Soc. 99, 4058–4069. doi: 10.1021/ja00454a026
Huidobro-Toro, J. P., and Harris, R. A. (1996). Brain lipids that induce sleep are novel modulators of 5-hydroxytrypamine receptors. Proc. Natl. Acad. Sci. 93, 8078–8082. doi: 10.1073/pnas.93.15.8078
Ibrahim, N. A., Musa, S. K., Yassin, S. M., Abuniama, N. H., Awadalkareem, S., Osama, A., et al. (2017). Chemical composition and antimicrobial activity of essential oil of Belpharis linariifolia. Int. J. Sci. Technol. Soc 5, 62–66. doi: 10.11648/j.ijsts.20170504.12
Javaid, A., Ferdosi, M. F., Khan, I. H., Shoaib, A., Hafiz, M. S., and Hassan, M. A. U. (2021). Biochemical analysis of flowers of Vinca major, a medicinal weed plant of hilly areas of Pakistan. J. Weed Sci. Res. 27, 537–546. doi: 10.28941/pjwsr.v27i4.1014
Jijie, R., Barras, A., Boukherroub, R., and Szunerits, S. (2017). Nanomaterials for transdermal drug delivery: beyond the state of the art of liposomal structures. J. Mater. Chem. B 5, 8653–8675. doi: 10.1039/C7TB02529G
Kamali, E., Jamali, A., Ardebili, A., Ezadi, F., and Mohebbi, A. (2020). Evaluation of antimicrobial resistance, biofilm forming potential, and the presence of biofilm-related genes among clinical isolates of Pseudomonas aeruginosa. BMC. Res. Notes 13:27. doi: 10.1186/s13104-020-4890-z
Khalil, M. N., Choucry, M. A., El Senousy, A. S., Hassan, A., El-Marasy, S. A., El Awdan, S. A., et al. (2019). Ambrosin, a potent NF-κβ inhibitor, ameliorates lipopolysaccharide induced memory impairment, comparison to curcumin. PLoS One 14:e0219378. doi: 10.1371/journal.pone.0219378
Khan, M., Zahid, H., Khan, M., Ahmed Khan, A., and Haneef, M. (2023). Antimicrobial and antioxidant activity on Pakistan origin Launaea nudicaulis (L.) hook f.: a promising halophyte. Pak. J. Pharm. Sci. 36, 649–652.
Khare, T., Anand, U., Dey, A., Assaraf, Y. G., Chen, Z. S., Liu, Z., et al. (2021). Exploring phytochemicals for combating antibiotic resistance in microbial pathogens. Front. Pharmacol. 12:720726. doi: 10.3389/fphar.2021.720726
Khatri, S., and Chhillar, A. (2015). Phytochemical screening and antibacterial, analysis of Launaea nudicaulis. Int. J. Basic Appl. Biol. 2, 399–403.
Kitaoka, N., Sano, Y., Fujikawa, S., Nabeta, K., and Matsuura, H. (2012). Biological activity and tissue specific accumulation of fluorescently labeled methyl jasmonate. Nat. Prod. Commun. 7:1934578X1200700. doi: 10.1177/1934578X1200700225
Lordelo, R., Branco, R., Gama, F., and Morais, P. V. (2024). Assessment of antimicrobial resistance, biofilm formation, and surface modification potential in hospital strains of Pseudomonas aeruginosa and Klebsiella pneumoniae. Heliyon 10:e30464. doi: 10.1016/j.heliyon.2024.e30464
Lotfy, R. A., El-Moaty, A., and Heba, I. (2016). Anti-bacterial activities of some active constituents isolated from Phlomis floccosa D. Don. J. Desert Res. 66, 69–78. doi: 10.21608/ejdr.2016.5768
Makkar, H. P. (2003). Quantification of tannins in tree and shrub foliage: a laboratory manual. Cham: Springer Science & Business Media.
Malathi, K., Anbarasu, A., and Ramaiah, S. (2017). Ethyl iso-allocholate from a medicinal rice Karungkavuni inhibits dihydropteroate synthase in Escherichia coli: a molecular docking and dynamics study. Indian J. Pharm. Sci. 78, 780–788.
Mashlawi, A. M., Alanazi, N. A. H., Mohamed, G., Alfattah, M. A., Mohamed, D. A., Fayad, E., et al. (2024). Exploring the phytochemical composition of Methanolic extract from Launaea nudicaulis: investigating its antioxidant, anticancer, and anti-dengue activities against Aedes aegypti. J. Biosci. Appl. Res. 10, 30–41. doi: 10.21608/jbaar.2024.343721
Matsumoto, K., Akao, Y., Kobayashi, E., Ohguchi, K., Ito, T., Tanaka, T., et al. (2003). Induction of apoptosis by xanthones from mangosteen in human leukemia cell lines. J. Nat. Prod. 66, 1124–1127. doi: 10.1021/np020546u
Mendez, M. V., Bracca, A. B., and Kaufman, T. S. (2018). Isolation, synthesis, and biological activity of Quindoline, a valuable Indoloquinoline natural product and useful key intermediate. Synthesis 50, 1417–1429. doi: 10.1055/s-0036-1591947
Miliauskas, G., Venskutonis, P., and Van Beek, T. (2004). Screening of radical scavenging activity of some medicinal and aromatic plant extracts. Food Chem. 85, 231–237. doi: 10.1016/j.foodchem.2003.05.007
Munazir, M., Qureshi, R., Arshad, M., and Gulfraz, M. (2012). Antibacterial activity of root and fruit extracts of Leptadenia pyrotechnica (Asclepiadaceae) from Pakistan. Pak. J. Bot. 44, 1209–1213.
Nascimento, J. M. D., Sousa, A. H. D., Lopes, L. M., Mendonça, J. F., and Santos, A. C. V. D. (2023). Bioactivity of Piper aduncum L. essential oil for insect pests of stored products 1. Pesquisa Agropecuária Trop. 53:e77482. doi: 10.1590/1983-40632023v5377482
Nivas, R. K., and Boominathan, M. (2015). Antimicrobial evaluation of selected south indian medicinal plants against Streptococcus pneumoniae. Int. J. Curr. Microbiol. Appl. Sci 4, 835–840.
Orhan, İ. E., Özçelik, B., and Şener, B. (2011). Evaluation of antibacterial, antifungal, antiviral, and antioxidant potentials of some edible oils and their fatty acid profiles. Turk. J. Biol. 35, 251–258. doi: 10.3906/biy-0907-107
Pacheco, Y. M., López, S., Bermúdez, B., Abia, R., Villar, J., and Muriana, F. J. (2008). A meal rich in oleic acid beneficially modulates postprandial sICAM-1 and sVCAM-1 in normotensive and hypertensive hypertriglyceridemic subjects. J. Nutr. Biochem. 19, 200–205. doi: 10.1016/j.jnutbio.2007.03.002
Pan, P.-H., Lin, S.-Y., Ou, Y.-C., Chen, W.-Y., Chuang, Y.-H., Yen, Y.-J., et al. (2010). Stearic acid attenuates cholestasis-induced liver injury. Biochem. Biophys. Res. Commun. 391, 1537–1542. doi: 10.1016/j.bbrc.2009.12.119
Paneri, M., Sevta, P., and Yagnik, V. D. (2023). Efficacy of plant extracts against Carbapenem resistant Acinetobacter baumannii: a notorious pathogen in the intensive care unit. Global J. Med. Pharm. Biomed.Update 18:24. doi: 10.25259/GJMPBU_154_2022
Procop, G., Church, D., Hall, G., Janda, W., Koneman, E., Schreckenberger, P., et al. (2010). Koneman’s Color Atlas and textbook of Diagnostic Microbiology. 7th Ed. ed. L. Williams Philadelphia, USA: Wilkins Company.
Purnamawati, S., Indrastuti, N., Danarti, R., and Saefudin, T. (2017). The role of moisturizers in addressing various kinds of dermatitis: a review. Clin. Med. Res. 15, 75–87. doi: 10.3121/cmr.2017.1363
Qiao, L., Zhang, M., and Zhang, C. (2022). Vascular macrophages sense octanal and drive athero-inflammation. Cell. Mol. Immunol. 19, 1077–1078. doi: 10.1038/s41423-022-00896-9
Quranayati, Q., Iqhrammullah, M., Saidi, N., Nurliana, N., Idroes, R., and Nasution, R. (2022). Cytotoxicity and phytochemical profiles of Phyllanthus emblica stem barks with in silico drug-likeliness: focusing on antidiabetic potentials. J. Adv. Pharm. Technol. Res. 13, 281–285. doi: 10.4103/japtr.japtr_319_22
Ramdan, E.-S. E., and Abdallah, E. M. (2017). Phytochemical, antimicrobial and antioxidant properties of Launaea nudicaulis and Farsetia hamiltonii. J. Biol. Control. 31, 102–109. doi: 10.18311/jbc/2017/15910
Rani, J., Dhanda, N., Rani, P., and Sangwan, P. (2023). Phytochemical screening and antioxidant activities in different solvent extracts of Launeaea procumbens. Plant Archives 23, 318–322. doi: 10.51470/PLANTARCHIVES.2023.v23.no1.052
Rashid, S., Ashraf, M., Bibi, S., and Anjum, R. (2000). Antibacterial and antifungal activities of Launaea nudicaulis (Roxb.) and Launaea resedifolia (Linn.). Pak. J. Biol. Sci. 3, 630–632. doi: 10.3923/pjbs.2000.630.632
Rivero-Cruz, J. F., Granados-Pineda, J., Pedraza-Chaverri, J., Pérez-Rojas, J. M., Kumar-Passari, A., Diaz-Ruiz, G., et al. (2020). Phytochemical constituents, antioxidant, cytotoxic, and antimicrobial activities of the ethanolic extract of Mexican brown propolis. Antioxidants 9:70. doi: 10.3390/antiox9010070
Sathe, N., Beech, P., Croft, L., Suphioglu, C., Kapat, A., and Athan, E. (2023). Pseudomonas aeruginosa: infections and novel approaches to treatment “knowing the enemy” the threat of Pseudomonas aeruginosa and exploring novel approaches to treatment. Inf. Med. 2, 178–194. doi: 10.1016/j.imj.2023.05.003
Scaccaglia, M., Birbaumer, M. P., Pinelli, S., Pelosi, G., and Frei, A. (2024). Discovery of antibacterial manganese(i) tricarbonyl complexes through combinatorial chemistry. Chem. Sci. 15, 3907–3919. doi: 10.1039/D3SC05326A
Shan, T., Ma, Q., Guo, K., Liu, J., Li, W., Wang, F., et al. (2011). Xanthones from mangosteen extracts as natural chemo preventive agents: potential anticancer drugs. Curr. Mol. Med. 11, 666–677. doi: 10.2174/156652411797536679
Sharaf, M. H., Abdelaziz, A. M., Kalaba, M. H., Radwan, A. A., and Hashem, A. H. (2022). Antimicrobial, antioxidant, cytotoxic activities and phytochemical analysis of fungal endophytes isolated from Ocimum basilicum. Appl. Biochem. Biotechnol. 194, 1271–1289. doi: 10.1007/s12010-021-03702-w
Shehabeldine, A. M., Amin, B. H., Hagras, F. A., Ramadan, A. A., Kamel, M. R., Ahmed, M. A., et al. (2023). Potential antimicrobial and antibiofilm properties of copper oxide nanoparticles: time-kill kinetic essay and ultrastructure of pathogenic bacterial cells. Appl. Biochem. Biotechnol. 195, 467–485. doi: 10.1007/s12010-022-04120-2
Sianglum, W., Srimanote, P., Wonglumsom, W., Kittiniyom, K., and Voravuthikunchai, S. P. (2011). Proteome analyses of cellular proteins in methicillin-resistant Staphylococcus aureus treated with rhodomyrtone, a novel antibiotic candidate. PLoS One 6:e16628. doi: 10.1371/journal.pone.0016628
Sofowara, A. (1993). Medicinal plants and traditional medicine in Africa. Ibadan: Spectrum Books Ltd, 289.
Song, X., Xia, Y.-X., He, Z.-D., and Zhang, H.-J. (2018). A review of natural products with anti-biofilm activity. Curr. Org. Chem. 22, 789–817. doi: 10.2174/1385272821666170620110041
Soukup, R., Scarpellino, R., and Danielczik, E. (1964). Gas chromatographic separation of 2, 4-Dinitrophenylhydrazone derivatives of carbonyl compounds. Anal. Chem. 36, 2255–2256. doi: 10.1021/ac60218a010
Sugden, J., and Razdan, B. (1972). Some biological aspects of cyclopentane chemistry. Pharm. Acta Helv. 47, 257–264
Tamilmani, E., Radhakrishnan, R., and Sankaran, K. (2018). 13-Docosenamide release by bacteria in response to glucose during growth—fluorescein quenching and clinical application. Appl. Microbiol. Biotechnol. 102, 6673–6685. doi: 10.1007/s00253-018-9127-x
Ugolini, L., Della Noce, I., Trincia, P., Borzatta, V., and Palmieri, S. (2005). Benzodioxole derivatives as negative effectors of plant proteases. J. Agric. Food Chem. 53, 7494–7501. doi: 10.1021/jf0580418
Vadgama, R. N., Odaneth, A. A., and Lali, A. M. (2015). Green synthesis of isopropyl myristate in novel single phase medium part I: batch optimization studies. Biotechnol. Rep. 8, 133–137. doi: 10.1016/j.btre.2015.10.006
Van De Loosdrecht, A. A., Beelen, R. H. J., Ossenkoppele, G. J., Broekhoven, M. G., and Langenhuijsen, M. M. (1994). A tetrazolium-based colorimetric MTT assay to quantitate human monocyte mediated cytotoxicity against leukemic cells from cell lines and patients with acute myeloid leukemia. J. Immunol. Methods 174, 311–320. doi: 10.1016/0022-1759(94)90034-5
Vaou, N., Stavropoulou, E., Voidarou, C., and Tsigalou, C. (2021). Towards advances in medicinal plant antimicrobial activity: a review study on challenges and future. Perspectives 9:2041. doi: 10.3390/microorganisms9102041
Vengadeshkumar, L., and Sharmila, A. M. (2020). Antifungal activity of rhizophora apiculata against alternaria solani. Plant Archives 9725210:20.
Verme, J. L., Fu, J., Astarita, G., La Rana, G., Russo, R., Calignano, A., et al. (2005). The nuclear receptor peroxisome proliferator-activated receptor-α mediates the anti-inflammatory actions of palmitoylethanolamide. Mol. Pharmacol. 67, 15–19. doi: 10.1124/mol.104.006353
Wu, X., Wang, H., Xiong, J., Yang, G.-X., Hu, J.-F., Zhu, Q., et al. (2024). Staphylococcus aureus biofilm: formulation, regulatory, and emerging natural products-derived therapeutics. Biofilms 7:100175. doi: 10.1016/j.bioflm.2023.100175
Yetgin, A. (2024). Investigating medicinal plants for antimicrobial benefits in a changing climate. Int. J. Sec. Metab. 11, 364–377. doi: 10.21448/ijsm.1279531
Zhishen, J., Mengcheng, T., and Jianming, W. (1999). The determination of flavonoid contents in mulberry and their scavenging effects on superoxide radicals. Food Chem. 64, 555–559. doi: 10.1016/S0308-8146(98)00102-2
Keywords: Launaea nudicaulis , plant extract, P. aeruginosa , MIC, MBC, antibiofilm activity, anticancer activity
Citation: Elkady FM, Badr BM, Hashem AH, Abdulra hman MS, Abdelaziz AM, Al-Askar AA, AbdElgayed G and Hashem HR (2024) Unveiling the Launaea nudicaulis (L.) Hook medicinal bioactivities: phytochemical analysis, antibacterial, antibiofilm, and anticancer activities. Front. Microbiol. 15:1454623. doi: 10.3389/fmicb.2024.1454623
Edited by:
Matthew Gavino Donadu, University of Sassari, ItalyReviewed by:
Saptami Kanekar, Yenepoya University, IndiaMahmoud Yassien, Ain Shams University, Egypt
Copyright © 2024 Elkady, Badr, Hashem, Abdulrahman, Abdelaziz, Al-Askar, AbdElgayed and Hashem. This is an open-access article distributed under the terms of the Creative Commons Attribution License (CC BY). The use, distribution or reproduction in other forums is permitted, provided the original author(s) and the copyright owner(s) are credited and that the original publication in this journal is cited, in accordance with accepted academic practice. No use, distribution or reproduction is permitted which does not comply with these terms.
*Correspondence: Amr H. Hashem, amr.hosny86@azhar.edu.eg; Hany R. Hashem, hra11@fayoum.edu.eg