- 1Institute of Animal Science and Technology, Guangxi University, Nanning, China
- 2Key Laboratory of Veterinary Biotechnology, Guangxi Veterinary Research Institute, Nanning, China
- 3Key Laboratory of China (Guangxi)-ASEAN Cross-Border Animal Disease Prevention and Control, Ministry of Agriculture and Rural Affairs of China, Nanning, China
- 4Virginia Polytechnic Institute and State University, Blacksburg, VA, United States
- 5Guizhou Provincial Livestock and Poultry Genetic Resources Management Station, Guiyang, China
- 6Guilin Animal Epidemic Disease Prevention and Control Center, Guilin, China
Lactiplantibacillus plantarum strains are potentially rich sources of probiotics that could help avoid infections. In order to evaluate their efficacy in bolstering resistance to Salmonella typhimurium infection among chicks. In this study, L. plantarum and commercial probiotics were administered via the water supply at a dosage of 1×109 CFU per chicken from days 1 to 7 to establish a protective system for the chicks. On days 8 and 9, S. typhimurium was attacked to investigate the preventive effects and potential mechanisms of L. plantarum in comparison with commercial probiotics. Post-treatment, we took a broad range of measurements, including body weight, immune organ index changes, the viable count of S. typhimurium in the liver, spleen, and cecum, as well as pathological changes in the liver. Our findings demonstrated that both L. plantarum and the commercial probiotic could safeguard chicks from S. typhimurium infection. The data also suggested that probiotic medication could ease weight loss postinfection, lower the bacterial count in the liver, spleen, and cecum, and attenuate liver pathological damage among all treated participants. Subsequently, we did high-throughput sequencing of 16S rRNA to examine the fecal microbiota of the chicks 5 days post-infection. We discovered that both L. plantarum and the commercial probiotic could fend off the invasion of S. typhimurium by affecting the bacterial population of Anaerotruncus, Colidextribacter, and Lactobacillus. Generally speaking, the addition of L. plantarum as a feed additive protects yellow-feathered broilers from S. typhimurium illness, suggesting great potential for commercial uses in the poultry industry.
1 Introduction
In poultry production, chicks are reared in a distinct, isolated environment that minimally interacts with the external world. Contrary to other mammals, newly hatched chicks do not inherit maternal antibodies; however, they do acquire a beneficial microbial population from adult chickens through daily interactions. The intestinal microbiota of these newly hatched chicks is rudimentary, displaying low diversity and density (Apajalahti et al., 2004; Javadi et al., 2024; Jiangrang et al., 2003). Consequently, their intestinal colonization predominantly arises from environmental sources and is fragile when exposed to pathogens. Studies have reported that Salmonella can readily colonize and interact with the intestinal epithelium of newly hatched chicks (Dragana et al., 2013).
Salmonella, a Gram-negative facultative anaerobic bacterium from the Enterobacteriaceae family, is a primary contributor to various poultry diseases. It leads to substantial economic losses due to growth retardation and increased mortality rates within the poultry industry. As one of the major foodborne pathogens, it also poses a significant food safety risk globally (Eng et al., 2015; Ferrari et al., 2019; Norma and Santos, 2018). At present, over 2,600 serotypes of Salmonella exist, with numerous pathogens affecting both chickens and humans (Santos et al., 2018). It has been reported that a Salmonella infection can significantly hinder broiler growth (Abudabos et al., 2014), causing symptoms like mucosal damage, diarrhea, and reduced feed intake (Vandeplas et al., 2009). Due to the immature immune system of young chickens, Salmonella infections are frequent (Abudabos et al., 2018) and can be easily transmitted to humans through the consumption of poultry meat, leading to severe gastrointestinal diseases (Wilson et al., 2016).
With the global demand for poultry meat on the rise (Assem et al., 2021; Maeve et al., 2017), over 65 billion chickens are reared each year for protein production, with an anticipated increase of 16% by 2030 (OECD, Food and Agriculture Organization of the United Nations, 2016). Antibiotics have been employed in the poultry industry to thwart Salmonella infection among young chickens (Wenguang et al., 2018). Unfortunately, this preventive method has led to a grave food safety concern: antibiotic resistance. Both China and the European Union enacted bans on the use of growth-promoting antibiotics as feed additives in 2020 and 2006, respectively (Guo et al., 2020). Hence, the quest for alternative poultry feed additives has emerged as an urgent necessity for both food safety and global public health (Celi et al., 2017). In addition, the declining efficacy of antibiotics against bacterial pathogens such as Salmonella, combined with the rise of antibiotic-resistant bacteria, underscores the need for novel approaches to prevent or treat infections caused by intestinal pathogens.
At present, a range of methods have proven effective in preventing Salmonella infection, particularly the utilization of probiotics (Abudabos et al., 2018; Wilson et al., 2016; Khan and Naz, 2013). Probiotics are living microorganisms that, when consumed or applied, are intended to bestow health benefits. The earliest probiotics primarily consisted of a single microorganism, typically from the Saccharomyces or Lactobacillus genera. Benefits of probiotics include competing with pathogens for intestinal receptor sites, producing specific metabolites (such as short-chain fatty acids, hydrogen peroxide, and other antimicrobial substances), and eliciting immunostimulatory effects (Madsen et al., 2001). These protective mechanisms assist in inhibiting and eliminating potential pathogens such as Salmonella, improving the intestinal microenvironment, fortifying the intestinal barrier, reducing inflammation, and enhancing certain antigen-specific immune responses (Lin and Zhang, 2017; Markowiak and Śliżewska, 2017). Lactiplantibacillus plantarum (L. plantarum), a common strain of lactic acid bacteria, exhibits probiotic characteristics. In this study, L. plantarum was isolated from the intestine of a healthy chicken. This strain, functioning as a probiotic, demonstrated robust growth under simulated gastrointestinal conditions and exhibited strong antibacterial effects against both Gram-negative and Gram-positive bacteria. Additionally, it possessed the capability to degrade corn gibberellin and aflatoxin (Yin et al., 2023). Therefore, this study progresses to employ this specific strain of L. plantarum in investigating the resistance of chicks to Salmonella typhimurium (S. typhimurium) infection, while also exploring its probiotic properties in depth. Multiple studies have demonstrated that it benefits the growth performance of broilers (Jinfeng et al., 2018) and aids in the prevention and control of diseases (Baikui et al., 2021).
Over the past 70 years, researchers have primarily focused on improving poultry through genetic enhancement and innovative feed compositions. However, adequate attention might not have been directed towards controlling microbial infections such as Staphylococcus, Salmonella, Streptococcus, and Campylobacter (Agyare et al., 2019). Broiler chickens are especially susceptible to bacterial infections in the initial few weeks when their immune systems are still developing (Ohimain and Ofongo, 2012). Furthermore, it can take up to 8 weeks to establish a stable intestinal microbiota (Lutful, 2009). The longer it takes to achieve bacterial homeostasis, the higher the risk of pathogenic bacterial infection (Irshad, 2006). In this study, one-day-old broiler chickens were utilized to evaluate the protective effects against Salmonella when either L. plantarum or a commercial probiotic was added to their drinking water. Results indicated that the preventive addition of L. plantarum and the commercial probiotic could alleviate weight loss induced by Salmonella infection, reduce the Salmonella count in the liver, spleen, and cecum, and lessen liver pathological damage. Additionally, probiotics could further prevent Salmonella infection by modulating the intestinal microbiota in young chicks.
2 Materials and methods
2.1 Bacteria strains and testing animals
All animal procedures in this study adhered to the protocol approved by the Institutional Animal Ethics Examination Committee of the Guangxi Veterinary Research Institute in Nanning, China (Approval No. 8/2014/JU). The probiotics used in the study included L. plantarum GX17, obtained from the Key Laboratory of Biotechnology at the Guangxi Veterinary Research Institute. The strain was isolated from the intestinal contents of healthy chickens. The specific steps are as follows: the intestinal contents were gradiently diluted using PBS, 100 μL of the diluted liquid was spread on MRS solid culture medium for culture to obtain single colonies, and Gram staining was performed after multiple purifications to observe the staining results and morphological characteristics. The species of the isolated bacteria was preliminarily identified as Lactobacillus according to Bergey’s manual of systematic bacteriology, 2nd edition (Boone et al., 2001). The bacterial genomic DNA was extracted, and PCR amplification and sequencing of the 16S rDNA sequence were performed. For more accurate identification, we determined its whole genome sequence, and the result was the same as 16S rDNA sequencing, so the strain was determined to be L. plantarum. The whole genome information of L. plantarum GX17 is available in the NCBI Sequence-Read-Archive repository [CP159198, CP159199, CP159200, CP159201, CP159202]. The commercial probiotic product contains probiotic, composed of chicken-derived Lactobacillus, Streptococcus thermophilus, their metabolites, and glucose. It was sourced from Shandong Baolai Lilai Biotechnology Co., Ltd. The pathogenic bacterium used in the study was S. typhimurium (strain SM022), generously provided by Professor Alejandro Aballay from Duke University, USA. One-day-old healthy yellow-feathered broiler chickens were purchased from Guangxi Jinling Agriculture and Animal Husbandry Group Co., Ltd.
2.2 Experimental designs
A total of 375 one-day-old yellow-feathered chicks were randomly divided into 5 groups (with 5 replicates per group and 15 chicks per replicate): the L. plantarum group (LP group), the L. plantarum + S. typhimurium group (LP + Sty group), the commercial probiotic + S. typhimurium group (S + Sty group), the S. typhimurium group (Sty group), and the control group (no disposed). The average initial body weight did not significantly vary among the groups. In the LP group, during the first 7-day period, chicks were provided with drinking water supplemented with 1.5 × 109 colony-forming units (CFU) of live L. plantarum per chick. On days 8 and 9, they were orally administered 1 mL phosphate buffered saline (PBS) and had free access to food and water for the rest of the time. In the LP + Sty experimental group, during the first 7-day period, chicks drank water supplemented with 1.5 × 109 CFU of live L. plantarum per chick daily. On days 8 and 9, each chick was orally administered 1 mL of S. typhimurium at a concentration of 1.5 × 109 CFU/mL. In the S + Sty group, during the first 7-day period, chicks were provided with drinking water supplemented with 1.5 × 109 CFU of a commercial probiotic per chick daily. On days 8 and 9, each chick was orally administered 1 mL of S. typhimurium at a concentration of 1.5 × 109 CFU/mL. In the Sty group, on days 8 and 9 of the experiment, each chick was orally administered 1 mL of S. typhimurium at a concentration of 1.5 × 109 CFU/mL, while for the rest of the time, they had free access to feed and water. The control group orally administered 1 mL PBS during days 8 and 9 of the experiment, and for the rest of the time, they had free access to feed and water. Before feeding the probiotics, water was withheld for 1 h each day. The probiotic solution was consumed within 3 h, after which the drinking containers were completely washed and then refilled with regular drinking water. The control group received PBS during days 8–9 of the experiment, and for the rest of the time, they had free access to feed and water. The experimental design is shown in Figure 1.
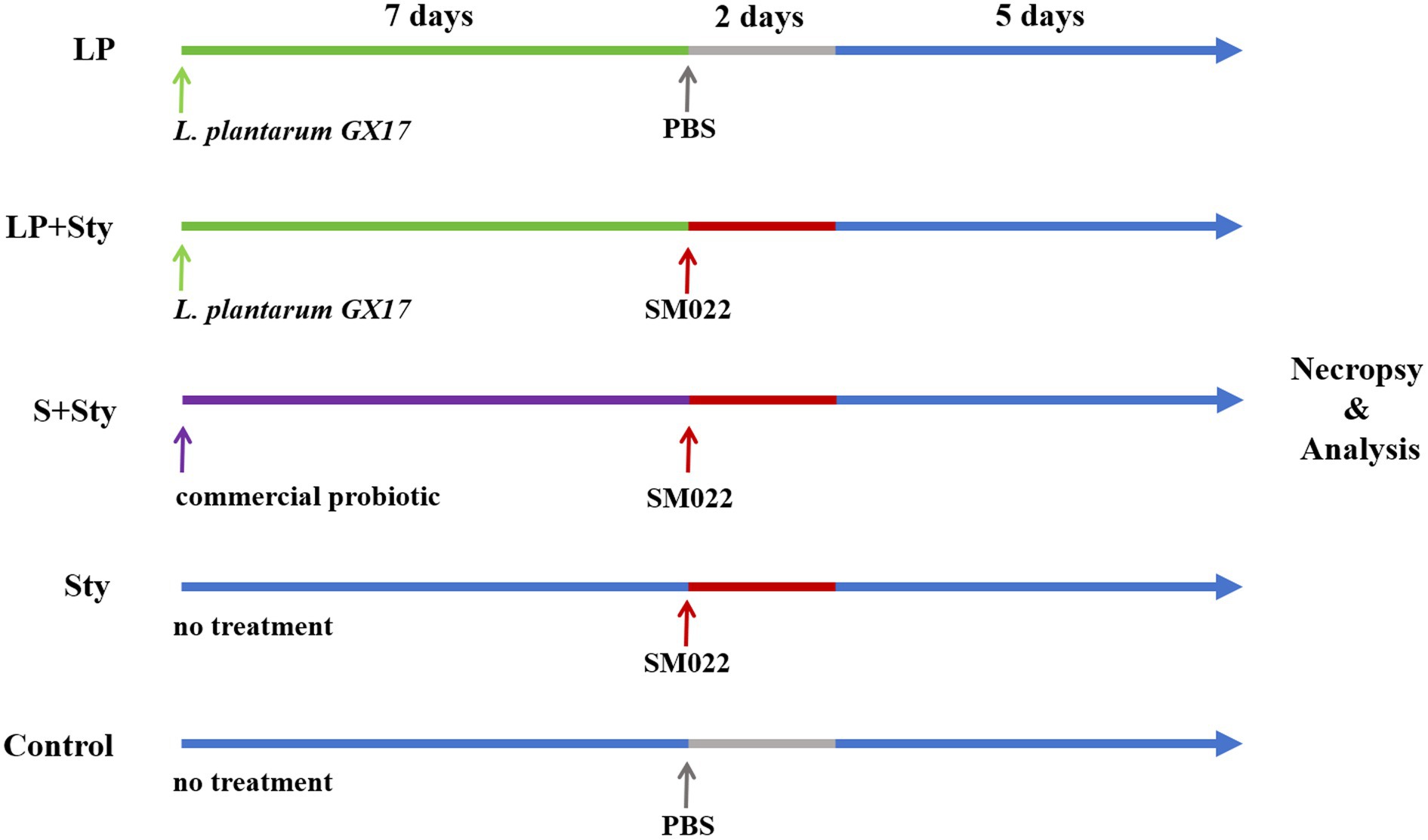
Figure 1. Experimental design for chick treatment groups. n = 75 each group. Control group: chicks were orally administered PBS on the 8th and 9th days. LP group: chicks were orally administered L. plantarum from the 1st to the 7th day and given PBS on the 8th and 9th days. LP + Sty group: chicks were orally administered L. plantarum from the 1st to the 7th day and were given S. typhimurium on the 8th and 9th days. S + Sty group: chicks were orally administered a commercial probiotic from the 1st to the 7th day and given PBS on the 8th and 9th days. Sty group: chicks were orally administered S. typhimurium on the 8th and 9th days.
2.3 The duration of testing cycles
The study was conducted at the experimental base of the Guangxi Veterinary Research Institute. Prior to initiating the experiment, the chicken house was meticulously cleaned and disinfected. The chickens were housed in cages under natural lighting conditions, with unrestricted access to feed and water. Regular observations were made and records kept of the chicken’s feeding behavior and overall health status. The experiment spanned 14 days, with relevant measurements taken on the 14th (final) day.
The initial ambient temperature for the chicks’ cultivation was maintained at 31°C, with a gradual reduction of 2°C per week. The feed utilized in this experiment was formulated based on the “Chicken Feeding Standards” (NY/T 33-2004), taking into consideration the growth cycle and specific traits of the yellow-feathered broilers. The composition and nutritional content of the basal diet are presented in Table 1.
2.4 Chicken weight changes
The weight of each group of chicks was measured on the morning of the 7th and 14th days at 9:00 a.m. on an empty stomach. The weight changes before and after the infection were recorded for each group.
2.5 Immune organ index
On the 5th day after infection (5 dpi), three chicks from each group were euthanized. Their thymus, spleen, and bursa of Fabricius were collected aseptically. Adhering fat tissues were dissected, and the weight of each organ was measured to calculate the organ index. Immune organ index = immune organ weight (g)/chicken weight (kg).
2.6 Impact on the quantity of Salmonella typhimurium in chicken liver/spleen/ceca
On the 5 dpi, aseptic collection of chicken liver, spleen, and cecum was performed. Each organ was placed in sterilized Eppendorf tubes separately, weighed, and then suspended in sterile PBS buffer at a ratio of 1 g/mL. After thorough grinding and homogenization, the suspensions were serially diluted with PBS buffer. A volume of 100 μL of the diluted liquid was plated onto Xylose Lysine Desoxycholate agar plates and incubated at 37°C for 16 to 24 h. Colonies were counted to calculate the number of viable bacteria per gram of tissue.
2.7 Tissue pathological examination
On the 5 dpi, three chickens from each group were euthanized for tissue collection. Chicken liver, spleen, duodenum, and ceca were collected aseptically. The tissue was fixed in a 4% formaldehyde solution for 48 h, then sent to the Guangxi University of Chinese Medicine for further preparation.
2.8 Intestinal microbiota analysis
On the 5 dpi, respectively, three chickens from each testing group were randomly selected and sacrificed after overnight fasting to collect cecal contents. Total genomic DNA was extracted using the Bacterial Genome DNA Extraction Kit (Kangwei Century Biotechnology Co., Ltd., Beijing, China) following the manufacturer’s instructions. DNA concentration and integrity were measured with an Implen NanoPhotometer and agarose gel electrophoresis. The extracted DNA was stored at −20°C until further processing. The extracted DNA was used as a template for polymerase chain reaction (PCR) amplification of bacterial 16S ribosomal RNA (rRNA) genes with barcoded primers and Takara Ex Taq (Takara Bio Inc., Kyoto, Japan). For bacterial diversity analysis, V3–V4 variable regions of 16S rRNA genes were amplified with universal primers 343F (5′-TACGGRAGGCAGCAG-3′) and 798R (5′-AGGGTATCTAATCCT-3′) for V3–V4 regions (Herlemann et al., 2011).
The amplicon quality was visualized using agarose gel electrophoresis. The PCR products were purified with AMPure XP beads (Beckman Coulter, Inc., Pasadena, CA, United States) and amplified for another round of PCR. After being purified with the AMPure XP beads again, the final amplicon was quantified using the Qubit dsDNA Assay Kit (Thermo Fisher Scientific, Waltham, MA, United States). The concentrations were then adjusted for sequencing. Sequencing was performed on an Illumina NovaSeq 6000 with 250 bp paired-end reads (Illumina Inc., San Diego, CA).
The library sequencing and data processing were conducted by OE Biotech Co., Ltd. (Shanghai, China). Raw data was collected and stored in FASTQ format. The Cutadapt software was used to trim off the primer sequences. For the qualified paired-end raw data, an open-source software package DADA2 (version 2020.11) (Callahan et al., 2016) with the default settings of QIIME 2 (version 2020.11) (Bolyen et al., 2019) was employed to de-noise, removing sequencing errors, and merge and remove chimera sequences. The representative sequences and amplicon sequence variants (ASV) were then generated. After selecting representative sequences for each ASV using the QIIME 2 package, all representative sequences were annotated against the Silva (version 138) database. The species annotation was performed using the q2-feature-classifier software (version 2020.11) with default parameters. The α and β diversity analyses were then carried out using QIIME 2 software. The alpha diversity of samples was assessed using indices including Chao1 (Chao and Bunge, 2015) and Shannon (Hill et al., 2002). Unweighted UniFrac distance matrices were calculated by R software (version 4.2.2) and then used for unweighted UniFrac principal coordinates analysis (PCoA) to evaluate the β diversity of samples. ANOVA for differential gene expression analysis was running in R software.
2.9 Statistical analysis
The experimental data were statistically analyzed using SPSS 25.0. Data were presented as means and pooled as the standard error of the mean (SEM). The effects of dietary treatment on the measured variables were analyzed by one-way ANOVA, followed by Duncan’s multiple comparison test to compare among groups. Differences were considered significant at p < 0.05.
3 Results
3.1 The body weight changes among groups of treatments
As indicated in Figure 2, by the day of infection, animals had been receiving probiotics in their drinking water for 7 days, except for Sty and control group. We observed no significant differences in body weight among the experimental groups (p > 0.05). On the 5 dpi, compared to the control group, the average body weight of the Sty group significantly decreased (p < 0.05). Meanwhile, the LP, LP + Sty, and S + Sty groups exhibited no notable variation (p > 0.05). When compared to the LP + Sty and S + Sty groups, the Sty group exhibited a significant decrease in body weight (p < 0.05). Specifically, the average body weight reduction in the Sty group was 24.49 g, 24.85 g, and 23.92 g relative to the control, LP + Sty, and S + Sty groups, respectively. These results indicate that probiotics can mitigate weight loss after the infection of S. typhimurium.
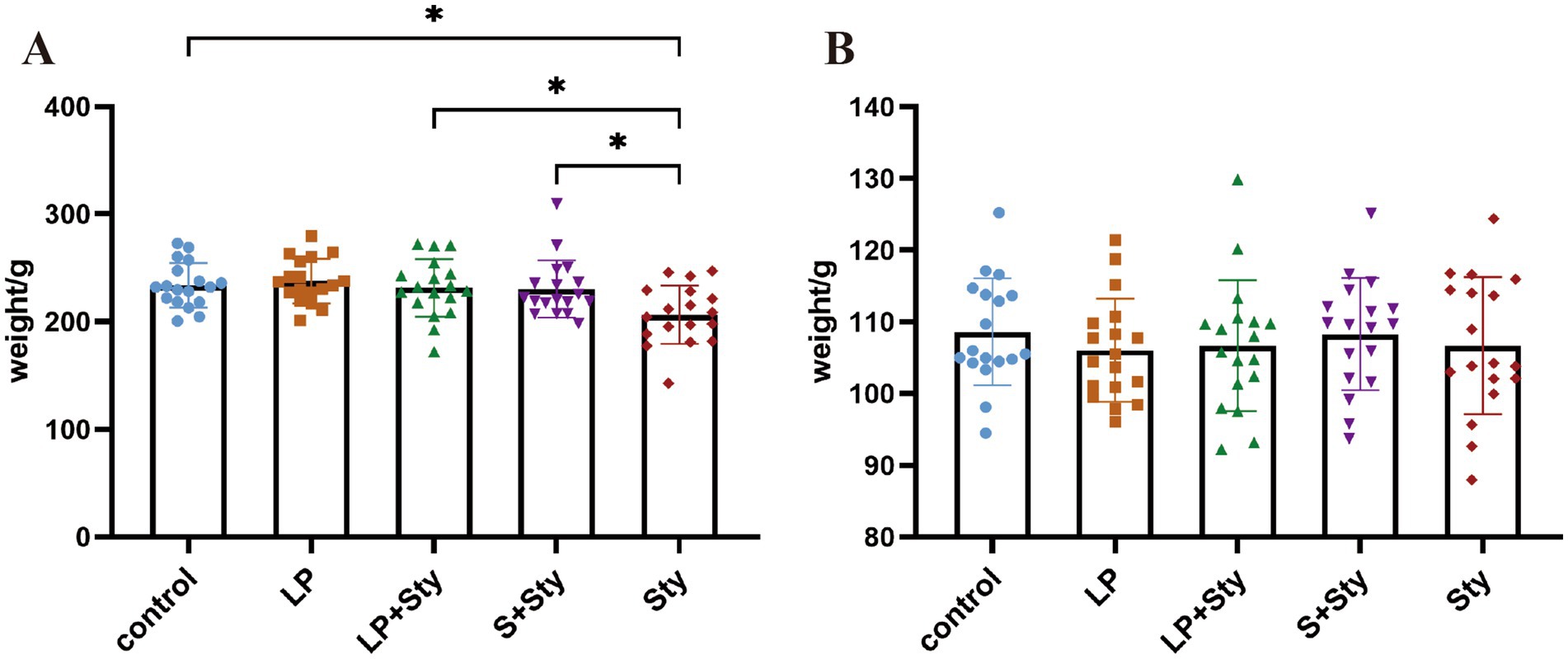
Figure 2. Body weight changes of chicks before and after S. typhimurium attack (*p < 0.05). (A) On the day of S. typhimurium attack. (B) 5 dpi. Control group: chicks were orally administered PBS on the 8th and 9th days. LP group: chicks were orally administered L. plantarum from the 1st to the 7th day and given PBS on the 8th and 9th days. LP + Sty group: chicks were orally administered L. plantarum from the 1st to the 7th day and were given S. typhimurium on the 8th and 9th days. S + Sty group: chicks were orally administered a commercial probiotic from the 1st to the 7th day and given pbs on the 8th and 9th days. sty group: chicks were orally administered S. typhimurium on the 8th and 9th days.
3.2 All immune organ indexes do not change among groups
We also tested the change in immune organ index and presented all the data in Figure 3. Compared to the control group, there were no significant differences in the immune organ weights of the thymus, spleen, and bursa of Fabricius among groups (p > 0.05).
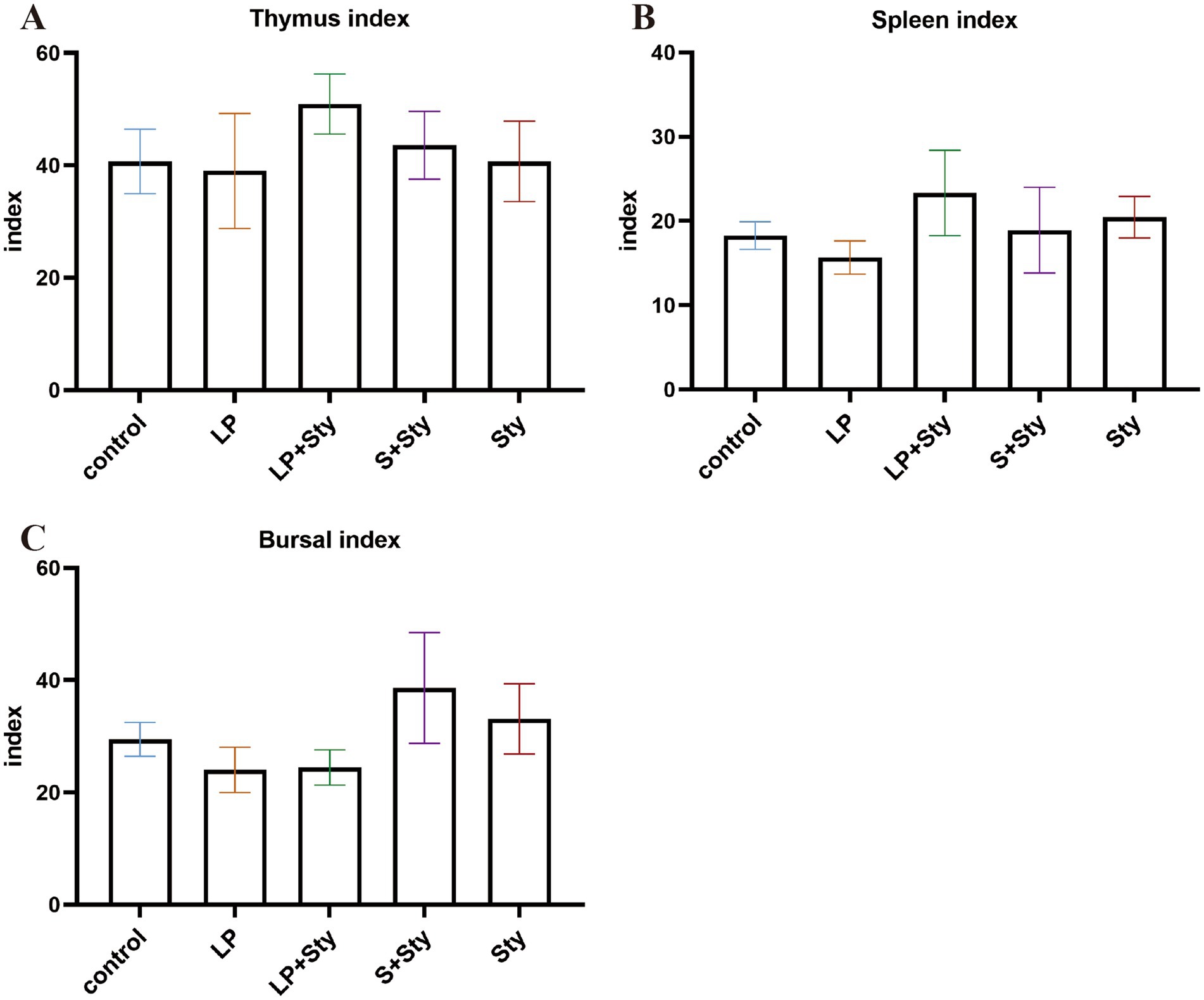
Figure 3. Comparison of immune organ indexes in different groups (A) Thymus index. (B) Spleen index. (C) Bursa of Fabricius index. Immune organ index = immune organ weight (g)/chicken weight (kg). Control group: chicks were orally administered PBS on the 8th and 9th days. LP group: chicks were orally administered L. plantarum from the 1st to the 7th day and given PBS on the 8th and 9th days. LP + Sty group: chicks were orally administered L. plantarum from the 1st to the 7th day and were given S. typhimurium on the 8th and 9th days. S + Sty group: chicks were orally administered a commercial probiotic from the 1st to the 7th day and given PBS on the 8th and 9th days. Sty group: chicks were orally administered S. typhimurium on the 8th and 9th days.
3.3 Lactiplantibacillus plantarum GX17 reduces the quantity of Salmonella typhimurium in the liver, spleen, and cecum of infected chicks
Five days post-infection, we conducted viable bacterial counts on the liver, spleen, and cecal contents of chicks. As illustrated in Figure 4, the quantities of S. typhimurium in the liver, spleen, and cecal contents of the LP + Sty and S + Sty groups were significantly lower compared to the Sty group (p < 0.05). Specifically, the concentration of S. typhimurium in the liver exceeded 106 CFU/g in the Sty group, marking a level 12.23 times and 12.36 times higher than that in the LP + Sty group and S + Sty group, respectively. In the spleen, S. typhimurium concentrations surpassed 106 CFU/g in the Sty group, which was 12.65 times and 13.38 times greater than the LP + Sty group and S + Sty group, respectively. In the cecum, S. typhimurium quantities exceeded 108 CFU/g in the Sty group, which was 11.92 times and 11.72 times the counts in the LP + Sty group and S + Sty group, respectively. These findings suggest that both L. plantarum and the commercial probiotic can significantly reduce the quantity of S. typhimurium in the liver, spleen, and cecum of chicks (p < 0.05).
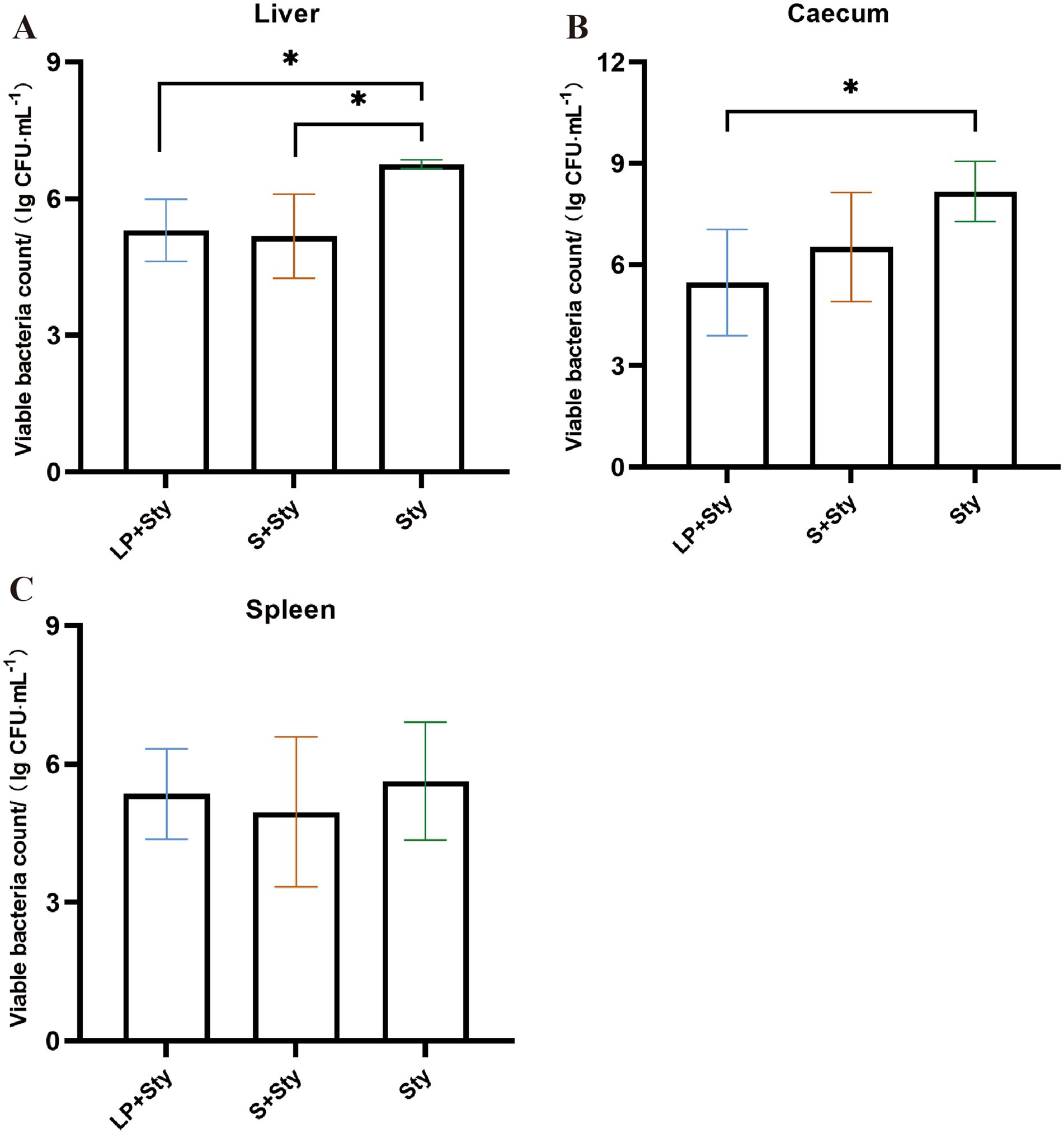
Figure 4. Determination of bacterial load in liver, spleen and cecum tissues of chicks in each group (*p < 0.05, **p < 0.01, and ***p < 0.001). (A) Liver. (B) Spleen. (C) Cecum. Control group: chicks were orally administered PBS on the 8th and 9th days. LP group: chicks were orally administered L. plantarum from the 1st to the 7th day and given PBS on the 8th and 9th days. LP + Sty group: chicks were orally administered L. plantarum from the 1st to the 7th day and were given S. typhimurium on the 8th and 9th days. S + Sty group: chicks were orally administered a commercial probiotic from the 1st to the 7th day and given PBS on the 8th and 9th days. Sty group: chicks were orally administered S. typhimurium on the 8th and 9th days.
3.4 Lactiplantibacillus plantarum GX17 mitigates the histopathological changes in infected chicks
Upon examining the liver paraffin sections of chicks (Figure 5), it is apparent that the liver structure of the LP group and control group chicks is well-defined and intact. All liver cells in this study are neatly and regularly arranged, with a distribution radiating outward from the central vein. In the Sty group (infected with S. typhimurium only), the liver tissue exhibits a blurry structure, with congestion in the blood vessels, disordered arrangement of liver cells, infiltration of red blood cells, extensive damage to cell membranes, nuclear condensation, significant hepatocyte necrosis, and severe infiltration of inflammatory cells. In the LP + Sty and S + Sty groups, the liver tissue maintains a relatively intact structure with neatly arranged liver cells compared with the Sty group. There is mild congestion, with a small amount of inflammatory cells and slight necrosis, significantly alleviating the severity of liver lesions compared to the Sty group. These experimental results indicate that L. plantarum GX17 and the commercial probiotic have a mitigating effect on liver pathology following S. typhimurium infection.
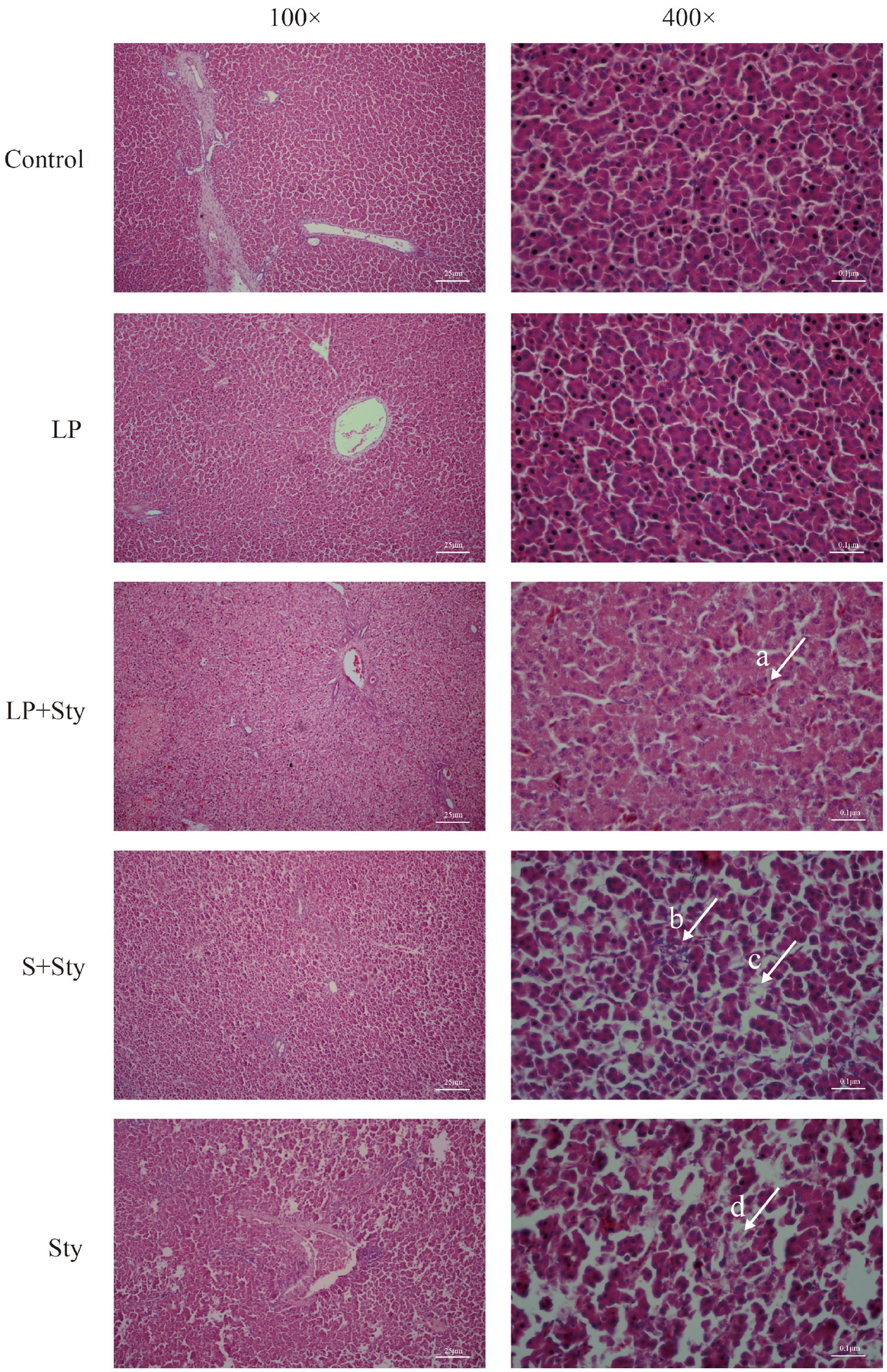
Figure 5. H&E staining results of chick liver tissues. (Arrow a) Filled with a few red blood cells. (Arrow b) Severe inflammatory cell infiltration. (Arrow c) The structure of hepatocytes is blurred. (Arrow d) Hepatocyte necrosis. Control group: chicks were orally administered PBS on the 8th and 9th days. LP group: chicks were orally administered L. plantarum from the 1st to the 7th day and given PBS on the 8th and 9th days. LP + Sty group: chicks were orally administered L. plantarum from the 1st to the 7th day and were given S. typhimurium on the 8th and 9th days. S + Sty group: chicks were orally administered a commercial probiotic from the 1st to the 7th day and given PBS on the 8th and 9th days. Sty group: chicks were orally administered S. typhimurium on the 8th and 9th days.
3.5 The α and β diversity of cecal microbiota after Salmonella typhimurium infection
The 16S rRNA sequencing generated millions of raw reads. The sample coverage indexes for all 15 samples were above 99%. Good coverage (Supplementary Figure S1A), sparsity (Supplementary Figure S1B), Shannon-Wiener index (Supplementary Figure S1C), and ASV species accumulation curve (Supplementary Figure S1D) of all samples indicate sufficient data sampling and sequencing depth, covering almost all microbial communities in the 16S rRNA database.
A total of 1,939 ASVs were obtained in this study. The number of ASVs in the LP, LP + Sty, Sty, S + Sty, and control groups were 450, 446, 426, 417, and 438, respectively. These results show that the fecal microbiota composition of the LP group was the highest, while the S + Sty group had the lowest, but the difference was not statistically significant. There were 17 ASVs shared among all five groups (Figure 6).
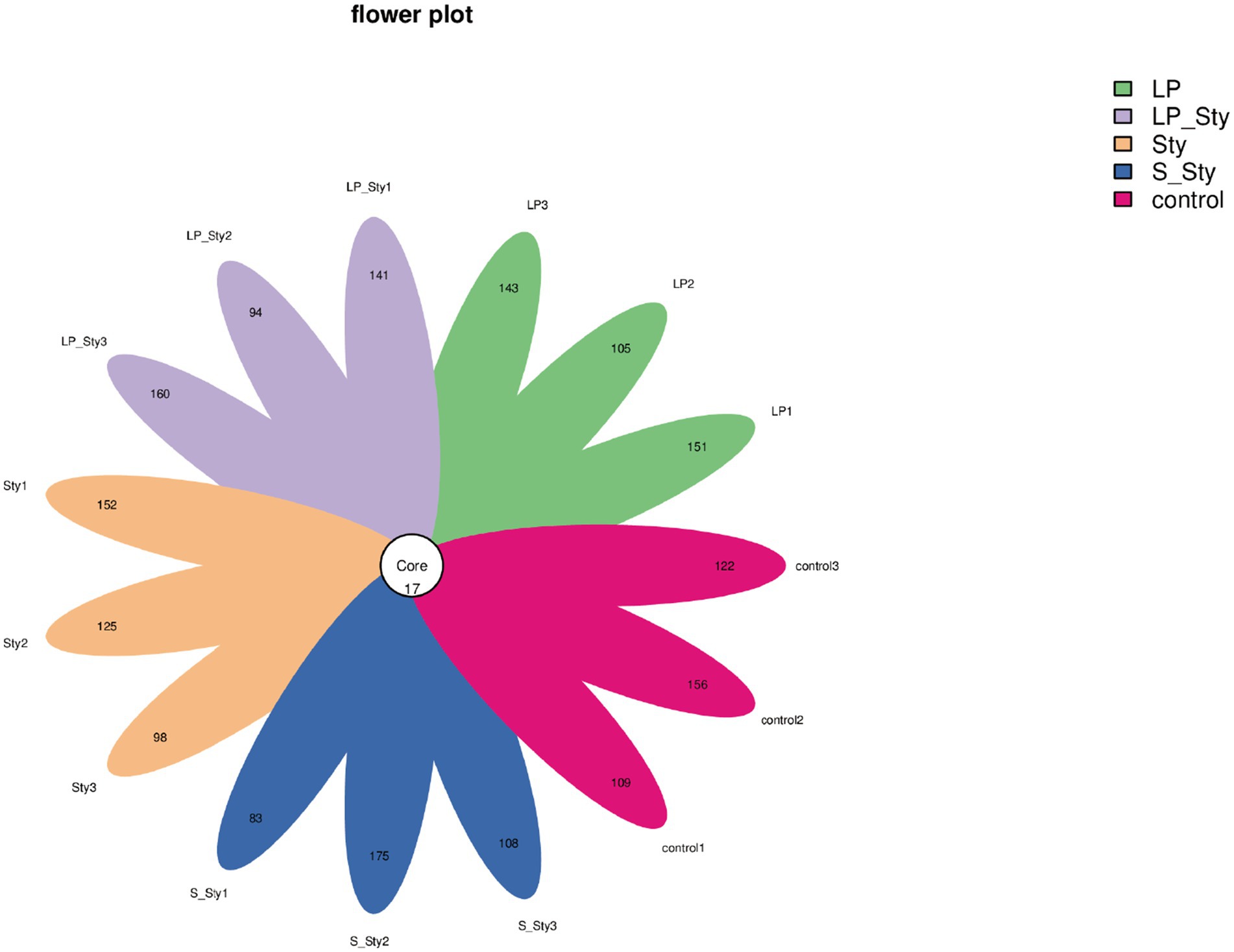
Figure 6. Flower plot of amplicon sequence variants (ASVs). This figure presents a flower plot illustrating the distribution of amplicon sequence variants (ASVs) across different samples. Each petal represents a unique ASV, with the length of the petal corresponding to the relative abundance of the ASV within the sample. The central circle denotes the core ASVs shared among all samples. Control group: chicks were orally administered PBS on the 8th and 9th days. LP group: chicks were orally administered L. plantarum from the 1st to the 7th day and given PBS on the 8th and 9th days. LP + Sty group: chicks were orally administered L. plantarum from the 1st to the 7th day and were given S. typhimurium on the 8th and 9th days. S + Sty group: chicks were orally administered a commercial probiotic from the 1st to the 7th day and given PBS on the 8th and 9th days. Sty group: chicks were orally administered S. typhimurium on the 8th and 9th days.
The Chao1 species richness estimator (Figure 7A) and observed species index (Figure 7B) were used to measure the richness of microbial species, whereas the Shannon diversity index (Figure 7C) measures their diversity. Both indexes were calculated to evaluate the α diversity in our data. There were no significant differences in alpha diversity indices among groups. PCoA showed significant differences in beta diversity among the five groups. Adonis statistical analysis yielded a p-value of 0.021 (see Figure 8).
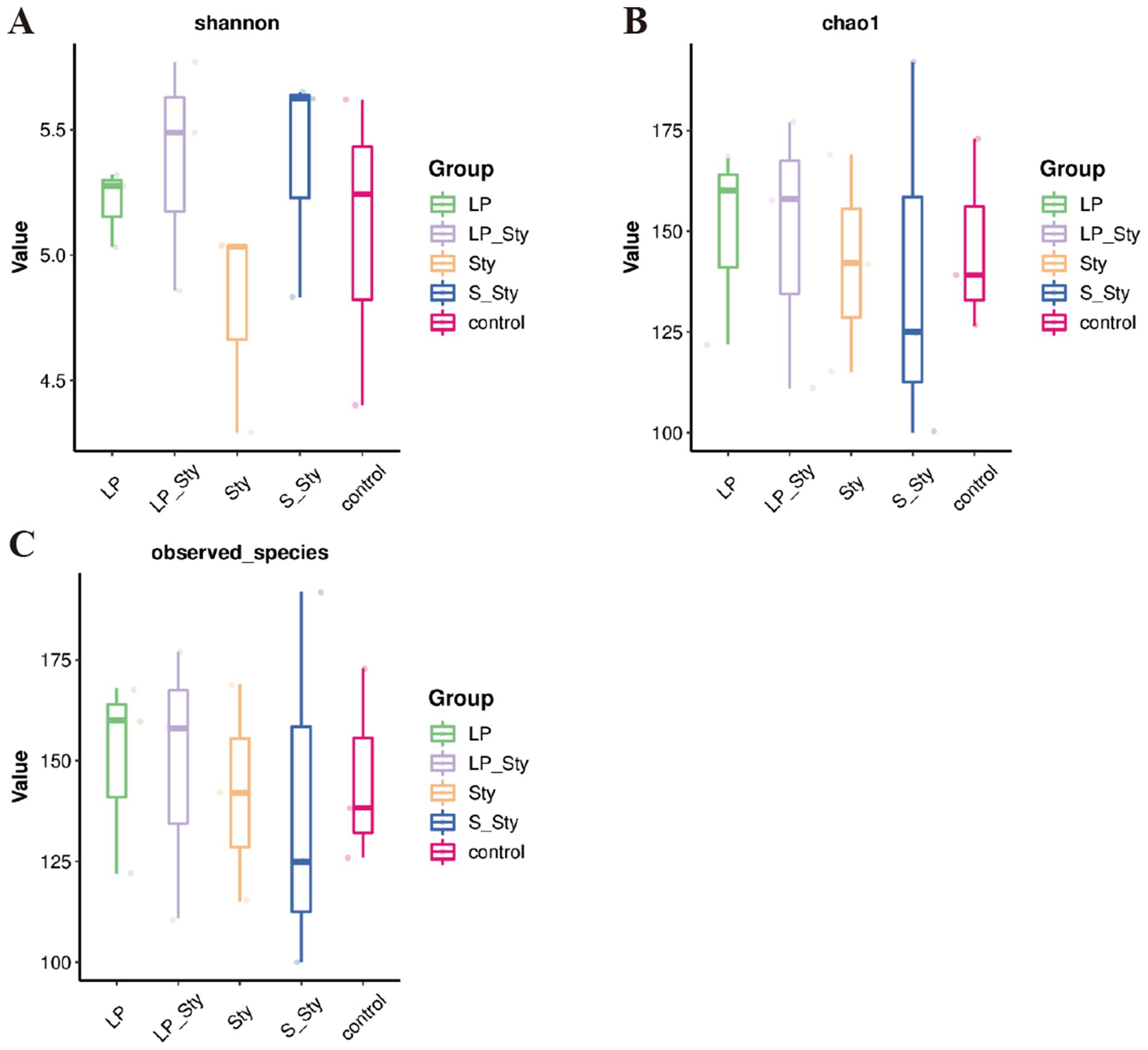
Figure 7. Alpha diversity indices. (A) Chao1—estimates species richness, accounting for rare species. (B) Observed species—reflects the actual number of distinct species observed. (C) Shannon—incorporates both species richness and evenness, providing a comprehensive measure of diversity. Control group: chicks were orally administered PBS on the 8th and 9th days. LP group: chicks were orally administered L. plantarum from the 1st to the 7th day and given PBS on the 8th and 9th days. LP + Sty group: chicks were orally administered L. plantarum from the 1st to the 7th day and were given S. typhimurium on the 8th and 9th days. S + Sty group: chicks were orally administered a commercial probiotic from the 1st to the 7th day and given PBS on the 8th and 9th days. Sty group: chicks were orally administered S. typhimurium on the 8th and 9th days.
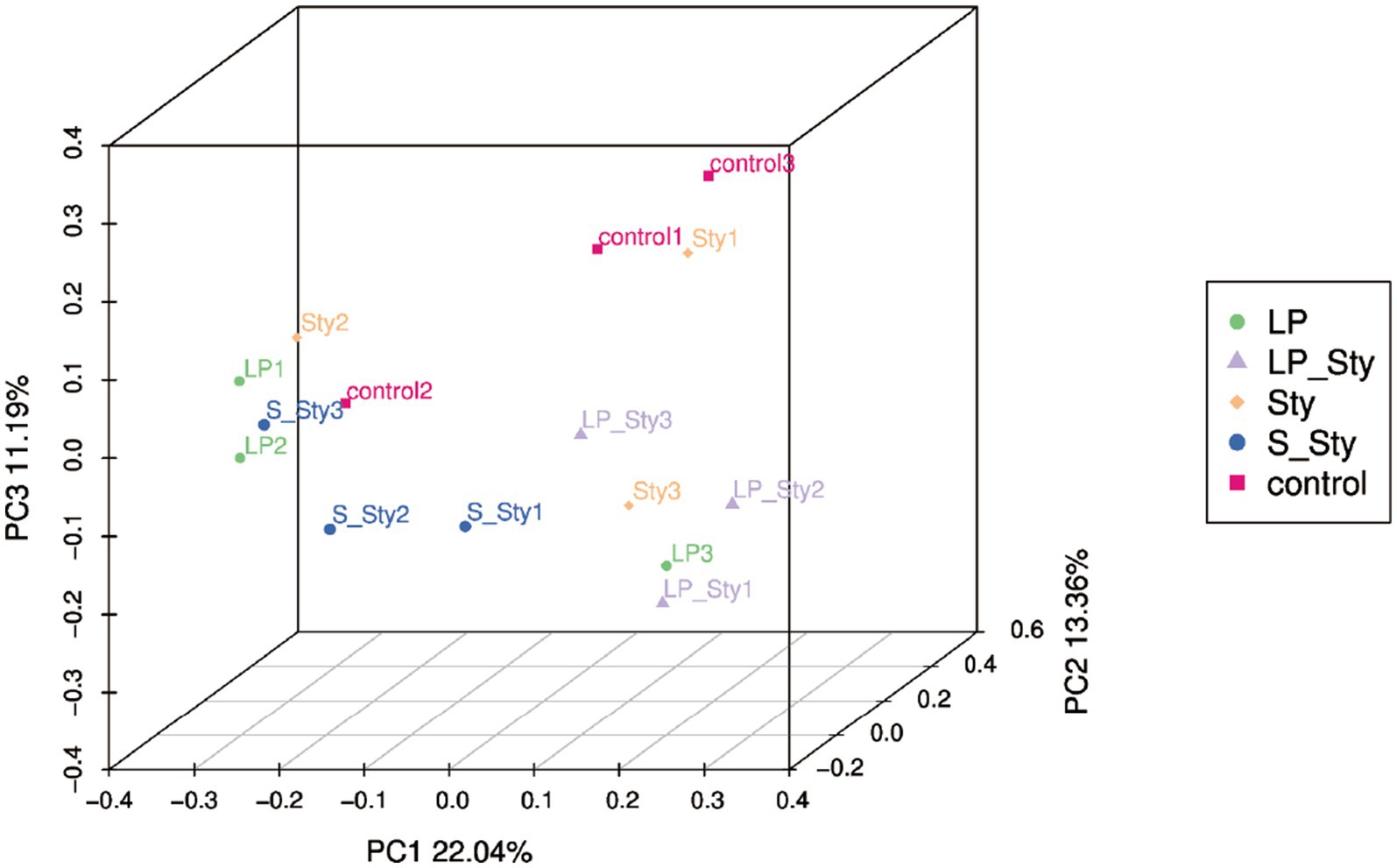
Figure 8. Principal coordinates analysis of microbial community composition. Control group: chicks were orally administered PBS on the 8th and 9th days. LP group: chicks were orally administered L. plantarum from the 1st to the 7th day and given PBS on the 8th and 9th days. LP + Sty group: chicks were orally administered L. plantarum from the 1st to the 7th day and were given S. typhimurium on the 8th and 9th days. S + Sty group: chicks were orally administered a commercial probiotic from the 1st to the 7th day and given PBS on the 8th and 9th days. Sty group: chicks were orally administered S. typhimurium on the 8th and 9th days.
3.6 Bacterial classification in the cecum of chicks
To elucidate the effects of S. typhimurium infection and probiotic supplementation on the composition of the cecal microbiota, we analyzed the bacteria at the phylum and genus levels to characterize the dynamics of microbial distribution. At the phylum level, except for the control group, where Firmicutes and Bacteroidetes were dominant, accounting for 70.37% and 26.33%, respectively, the cecal microbiota in the other groups were primarily composed of Firmicutes and Proteobacteria. Compared to the control group, the other groups had higher proportions of Proteobacteria and lower proportions of Bacteroidetes (Figure 9A). We further compared the bacterial composition in the cecum at the genus level. The relative abundance of the Clostridia genus was 18.83, 18.91, 37.48, 9.71, and 13.05% in the LP, LP + Sty, Sty, S + Sty, and control groups, respectively. These data suggest a significant Clostridia enrichment during S. typhimurium infection (Figure 9B).
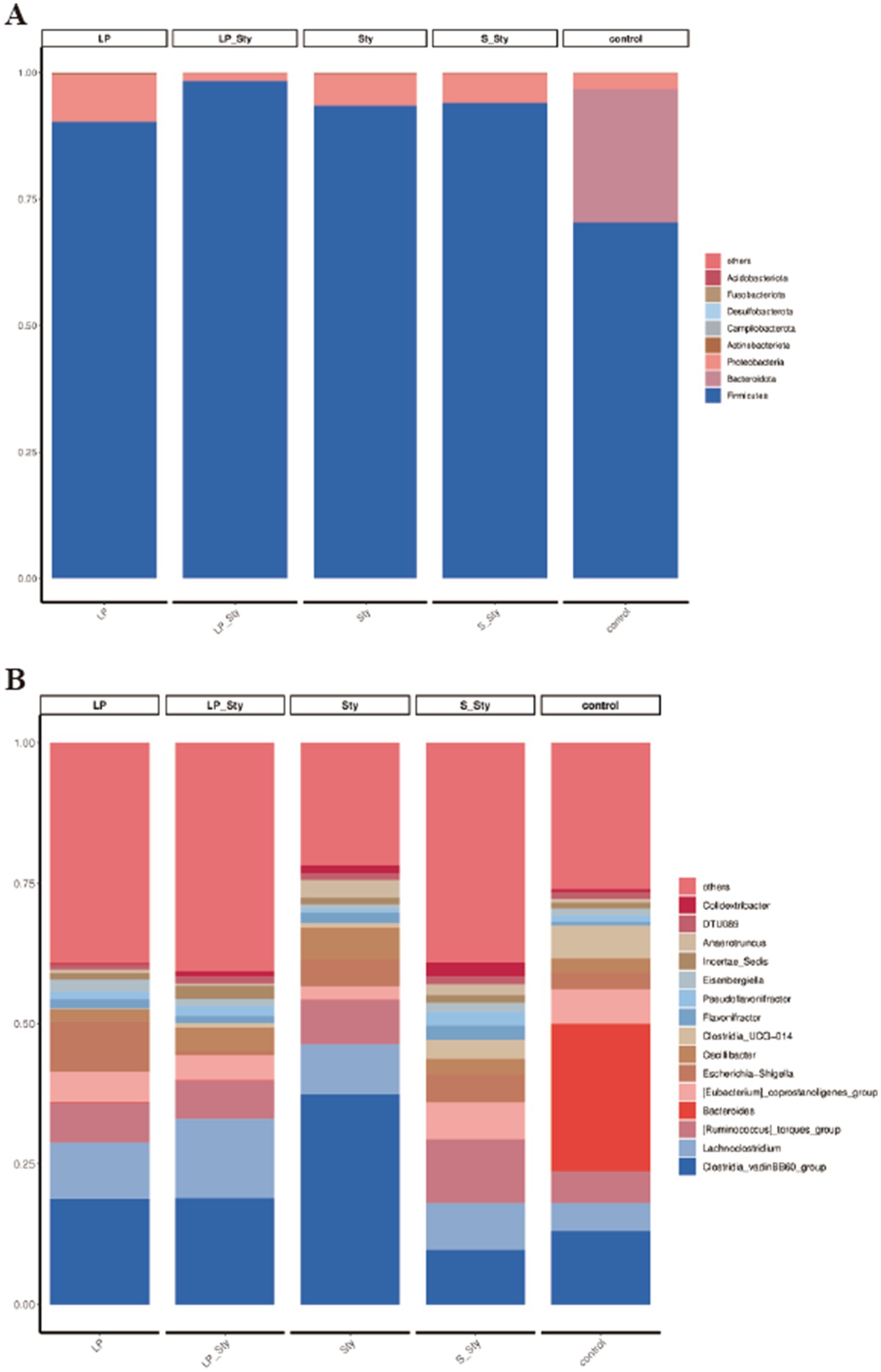
Figure 9. Comparative analysis of microbial community diversity. (A) Phylum level. (B) Genus level. Control group: chicks were orally administered PBS on the 8th and 9th days. LP group: chicks were orally administered L. plantarum from the 1st to the 7th day and given PBS on the 8th and 9th days. LP + Sty group: chicks were orally administered L. plantarum from the 1st to the 7th day and were given S. typhimurium on the 8th and 9th days. S + Sty group: chicks were orally administered a commercial probiotic from the 1st to the 7th day and given PBS on the 8th and 9th days. Sty group: chicks were orally administered S. typhimurium on the 8th and 9th days.
ANOVA analysis (Figure 10) revealed that the relative abundance of the Anaerotruncus genus was significantly higher in the Sty group compared to the LP, LP + Sty, and control groups, indicating that S. typhimurium infection significantly increased the relative abundance of Anaerotruncus (p < 0.05). The relative abundance of the Colidextribacter genus was significantly higher in the S + Sty group compared to the LP, LP + Sty, and control groups, suggesting that the addition of the commercial probiotic to the drinking water significantly increased the relative abundance of Colidextribacter (p < 0.05). Compared to the control group, the relative abundance of the Lactobacillus genus was significantly higher in the LP, LP + Sty, and S + Sty groups, indicating that the addition of L. plantarum GX17 and the commercial probiotic to the drinking water significantly increased the relative abundance of Lactobacillus (p < 0.05).
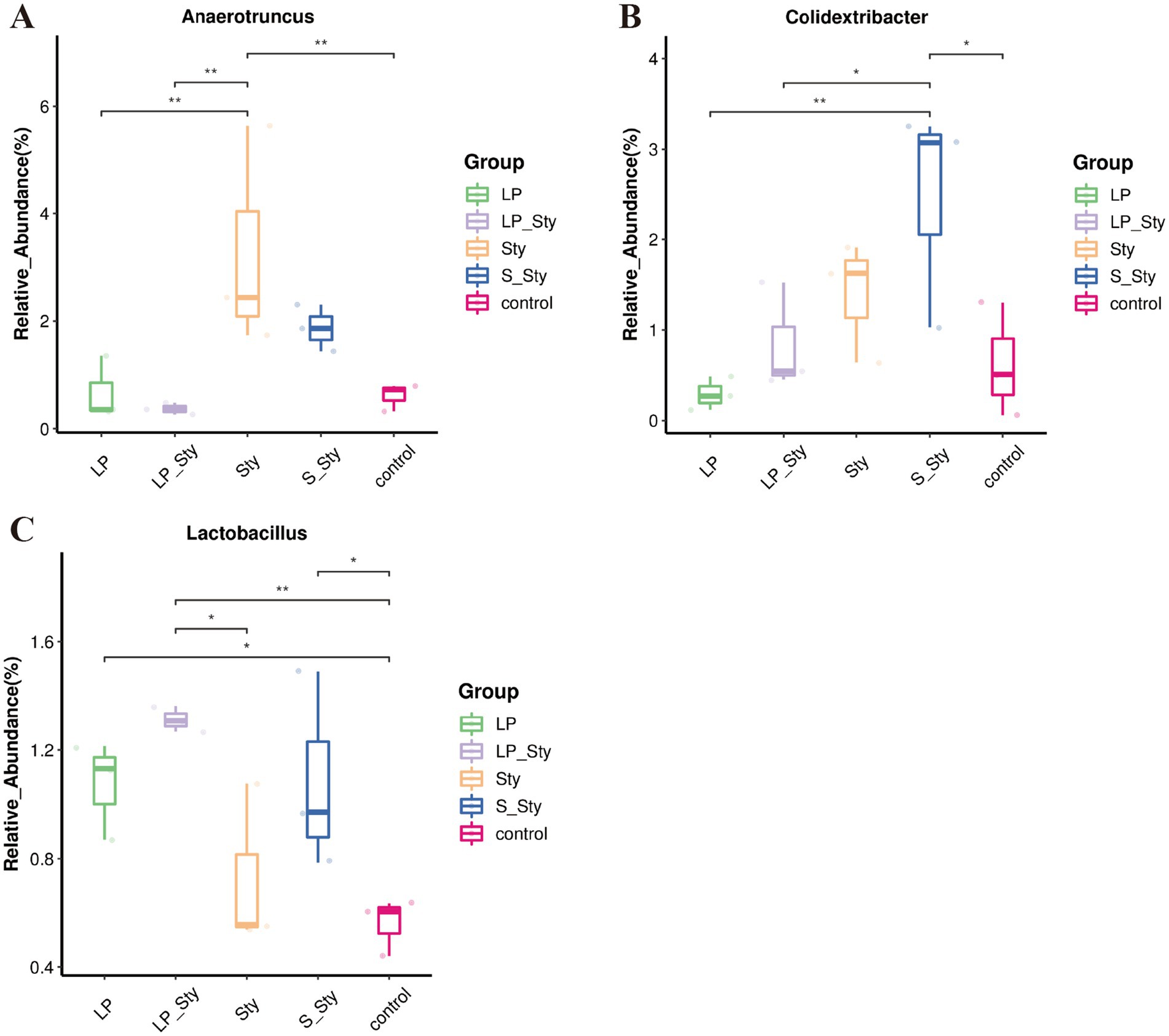
Figure 10. Boxplot analysis of genus-level species abundance (*p < 0.05 and **p < 0.01). (A) Anaerotruncus. (B) Colidextribacter. (C) Lactobacillus. This figure presents a boxplot analysis to compare the species abundance of select genera within the microbial community. Control group: chicks were orally administered PBS on the 8th and 9th days. LP group: chicks were orally administered L. plantarum from the 1st to the 7th day and given PBS on the 8th and 9th days. LP + Sty group: chicks were orally administered L. plantarum from the 1st to the 7th day and were given S. typhimurium on the 8th and 9th days. S + Sty group: chicks were orally administered a commercial probiotic from the 1st to the 7th day and given PBS on the 8th and 9th days. Sty group: chicks were orally administered S. typhimurium on the 8th and 9th days.
4 Discussion
S. typhimurium is among the most perilous pathogens of infectious diseases, significantly impacting the poultry industry and causing substantial economic losses. Marcq et al. (2011) discovered that Salmonella infection could dramatically reduce the growth performance of broiler chickens. Many studies have also shown that probiotics can prevent weight losses caused by Salmonella infection in the livestock and poultry industries. Gong et al. (2015) found that the inclusion of Bacillus subtilis in the diet mitigated the growth retardation induced by Salmonella infection in broiler chickens. Similarly, Zhu et al. (2021) reported that when broiler chickens were orally administered Bacillus subtilis BC66 during an infection, both their weight loss and feed conversion ratio improved, suggesting the preventive and health-promoting effects of probiotics. However, there are fewer studies focused on the Lactobacillus strain group. In our research, broiler chickens fed with water contain either L. plantarum GX17 or a commercial probiotic. Both types of probiotics exhibited a significant improvement in the body weight of participants compared to the infected controls. Moreover, treated chickens might recover to a similar level as uninfected individuals. These results are consistent with other studies in the field, indicating that the addition of L. plantarum GX17 significantly mitigates weight loss caused by S. typhimurium. The thymus, spleen, and bursa of Fabricius have crucial immune functions during the development of chicks, and the immune organ index serves as a set of important indicators of immune functions. The bursa of Fabricius facilitates B cell differentiation and maturation (Masteller et al., 1997), while the spleen, which systemically regulates B cells and macrophages, plays a vital role in humoral immunity (Jia and Pamer, 2009). In this study, no statistically significant variations were observed in the sizes of the thymus, spleen, and bursa of Fabricius across the treatment groups, suggesting that infection and the following inflammation reactions of S. typhimurium were suppressed by the treatment of probiotics. Similar findings have been reported in experiments using compound probiotics for the prevention and treatment of Salmonella infection in broiler chickens (Neveling et al., 2020). Our results align with those studies: the L. plantarum GX17 and commercial probiotics used in this study did not lead to immune organ enlargement. Therefore, it can be inferred that the consumption of the probiotics used in this study may alleviate the symptoms of Salmonella infection, along with having a minor immunostimulatory effect on the immune organs of the chicks.
Salmonella can cause significant decline in broiler growth performance. The main reason for the decline in growth performance is the reduced feed intake of broilers due to mucosal damage, diarrhea and systemic infection (Vandeplas et al., 2009). Studies have found that L. plantarum significantly increased the levels of IL-10, while significantly reducing the levels of IL-4, IFN-γ, and TNF-α (Zhao et al., 2021). Another study showed that L. plantarum LS/07 can promote colon IL-10 production in inflammatory rats is used to treat inflammatory diseases (Štofilová et al., 2017), and studies have also shown that L. plantarum synergistically regulates M1 macrophage polarization to resist S. typhimurium infection (Duan et al., 2022). Therefore, the process by which L. plantarum GX17 alleviates the decline in growth performance may be related to its participation in activating the body’s immune defense function and alleviating the body’s inflammatory response.
Chicken meat is an important source of high-quality protein for humans, but it is also considered a major source of foodborne diseases, especially enteritis-causing Salmonella and typhoid-causing Salmonella. Therefore, reducing the contamination of live poultry with S. typhimurium can help mitigate the food safety risks faced by consumers. Studies have shown that the use of various probiotics in preventive treatments can control and reduce the colonization of Salmonella in the gastrointestinal tract of broiler chickens (Neveling et al., 2020). Additionally, research has demonstrated that treatment with probiotics based on lactic acid bacteria can significantly reduce the Salmonella count in chicks after infection (Menconi et al., 2011). Chen et al. (2017) found that adding L. plantarum Z01 to the diet significantly reduced the quantity of S. typhimurium in the cecum. In this study, adding L. plantarum GX17 and a commercial probiotic to the drinking water of newly hatched chicks significantly reduced the colonization of S. typhimurium in the tissues. Both L. plantarum GX17 and the commercial probiotic significantly decreased the content of S. typhimurium in the liver, spleen, and cecum tissues compared to the chicks without probiotic supplementation. The protective effect of probiotics may be attributed to the production of organic acids such as lactic acid, which has antimicrobial properties. The antimicrobial effects of organic acids are generally attributed to their ability to affect pH, intracellular osmotic pressure, cell membrane permeability, substance transport, cell signaling, energy metabolism, or biomacromolecule synthesis (Hauser et al., 2016). The anti-Salmonella mode of action of L-phenyllactic acid produced by L. plantarum is attributed to cell membrane disruption and genomic DNA-binding (Zhou et al., 2020). The antibacterial activity of L. plantarum ACA-DC287 against S. typhimurium SL1344 is due to the lactic acid it produces (Makras et al., 2006). Probiotics may also produce adhesion inhibitors like hydrogen peroxide and bacteriocins to reduce the chances of pathogens entering epithelial cells (Ohland and Macnaughton, 2010). Most lactic acid bacterial bacteriocins act by disrupting the cytoplasmic membrane through pore formation or by degrading the cell wall (Pérez-Ramos et al., 2021). BMP11, a new bacteriocin produced by L. crustorum against Listeria monocytogenes, has been shown to disrupt cell membrane integrity and increase membrane permeability (Cavicchioli et al., 2017). Bacteriocins produced by L. plantarum PTCC1745 significantly inhibited biofilm formation in Acinetobacter baumannii, reducing the expression of biofilm-related bap genes by 52% (Javadi et al., 2024). Therefore, it is speculated that the inhibition of S. typhimurium by L. plantarumm GX17 in this study may be caused by the production of antibacterial substances such as organic acids, hydrogen peroxide, and bacteriocins, but its specific destructive mechanism remains to be studied.
Salmonella enters the body through a gastrointestinal infection and spreads to other organs through the digestive tract or bloodstream (Beristain-Covarrubias et al., 2018). Studies have shown that Salmonella infection often leads to severe liver damage (Wu et al., 2014). In this study, the colonization level of Salmonella in the liver confirmed the protective effect of L. plantarum GX17 and the commercial probiotic on liver tissue. Feeding probiotics significantly reduced the content of S. typhimurium in the liver compared to chicks without probiotic supplementation. Furthermore, L. plantarum GX17 and the commercial probiotic alleviated the pathological damage caused by S. typhimurium in the liver. Similar results were also observed by Xing et al. (2021), which align with the findings of this study. The translocation of S. typhimurium is mainly due to the destruction of the intestinal barrier, which causes it to translocate to the spleen and liver. Studies have shown that the administration of L. plantarum LTC-113 can eliminate the increased permeability and bacterial translocation caused by S. typhimurium, which also reduces the damage to the spleen and liver of chicks (Wang et al., 2018). Therefore, the low bacterial load and less damage in the liver in this study may be due to the fact that L. plantarum GX17 alleviated the intestinal barrier damage caused by S. typhimurium, thereby reducing the number of S. typhimurium transferred from the intestine to the liver.
In both animal and human studies, the gut microbiota plays a significant role in maintaining various aspects of health, including immunity, metabolism, and neurobehavioral characteristics (Valdes et al., 2018). Research from the 1950s indicated that disrupting the microbiota increased susceptibility to infection (Bohnhoff et al., 1954). The advancement of sequencing technologies in the early 21st century enabled the analysis of microbial communities (Kamada et al., 2012; Barman et al., 2008; Stecher et al., 2007), and subsequent studies demonstrated that virulence factors can manipulate the microbial composition by targeting the host’s environment (Kamdar et al., 2016; Lopez et al., 2016; Winter et al., 2010). S. typhimurium, an important human pathogen, is often studied as a representative species (Ao et al., 2015; Majowicz et al., 2010). By inducing inflammation, S. typhimurium creates its own niche in the intestines, altering the composition of the gut microbiota and nutrient availability to favor its colonization and expansion (Ducarmon et al., 2019). The cecum of mice is known to have a high abundance of S. typhimurium (Carter and Collins, 1974), representing the largest microbial community in the body.
In this study, the 16S rRNA sequencing results revealed that the most common bacterial phyla in the cecum of broiler chickens were Firmicutes, Bacteroidetes, and Proteobacteria. Firmicutes, the predominant phylum, had the highest abundance (Wei et al., 2013). Certain members of Firmicutes can inhibit the growth of opportunistic pathogens, while others participate in the degradation of complex carbohydrates (Brown et al., 2012). Previous research has also shown that adding Bacillus subtilis to broiler chicken feed can regulate the intestinal immune system and gut microbiota, providing resistance against Salmonella infection (Hayashi et al., 2018). In this study, adding L. plantarum GX17 or a commercial probiotic to the drinking water could help resist the invasion of S. typhimurium on broiler chickens by modulating the abundance of Anaerotruncus, Colidextribacter, and Lactobacillus. Clostridia, which consist of various bacterial species, are closely associated with host inflammatory responses and serve as an important pathogen for producing botulinum toxins and causing fatal infections. It has been reported that an increasing level of Anaerotruncus is directly related to the elevation of pro-inflammatory cytokines associated with aging (Konkol et al., 2019). In mice fed a high-fat/high-cholesterol diet, an increase in Anaerotruncus abundance was found to be associated with hepatocellular carcinoma related to non-alcoholic fatty liver disease (NAFLD) (Zhang et al., 2020). Adding L. plantarum GX17 or a commercial probiotic to the drinking water restored the relative abundance of Anaerotruncus in the intestinal tract of broiler chickens infected with S. typhimurium, thereby alleviating the inflammatory response caused by the infection. Furthermore, adding the commercial probiotic to the drinking water significantly increased the relative abundance of Colidextribacter in the intestinal tract of Salmonella-infected broiler chickens. Colidextribacter promotes the production of guanosine, which helps reduce the secretion of inflammatory factors (Guo et al., 2021; Mager et al., 2020) and regulates systemic inflammatory responses while maintaining intestinal mucosal integrity (Liu et al., 2022). Increasing the proportion of Lactobacillus in the gut is desirable and beneficial. Lactobacillus is significantly positively correlated with the tight junction protein in the intestines and negatively correlated with inflammation and oxidative stress (Xia et al., 2020). Researchers have found that L. plantarum PS128 can regulate microbial communities, modulate inflammation, oxidative reactions, and protect gastrointestinal integrity, thereby affecting the physiological health of athletes, particularly their adaptability to exercise (Huang et al., 2020). Previous studies have shown that oral administration of probiotics can increase the relative abundance of Lactobacillus in the chicken gut (Yu et al., 2021). In this study, adding L. plantarum GX17 or a commercial probiotic to the drinking water significantly increased the relative abundance of Lactobacillus in the chicken gut. Compared to broiler chickens infected with S. typhimurium without probiotic supplementation, the addition of L. plantarum GX17 resulted in a significant increase in the relative abundance of Lactobacillus in the intestinal tract. Alternatively, this may be attributed to the proliferation of the administered probiotic strain, which produces molecules that stimulate the growth of Lactobacillus in the intestine (Ohashi et al., 2001; Takahashi et al., 2007). When a large number of probiotics enter the intestine, they adhere to the host’s epithelial cells, thereby eliminating the possibility of Salmonella colonization on the same substrate (Khochamit et al., 2020). In this study, colonization of L. plantarum GX17 into the intestine increased the number of lactic acid bacteria, and corresponding to the cecum bacterial load, the cecum S. typhimurium bacterial load significantly decreased after the addition of probiotics. Therefore, it was concluded that the competitive colonization of L. plantarum reduced the colonization amount of Salmonella, thus regulating the intestinal flora. Improves the immune system and maintains the function of the intestinal barrier.
5 Conclusion
In general, we demonstrated that L. plantarum GX17 can prevent the damage caused by S. typhimurium infection by modulating the gut microbiota. In this study, L. plantarum GX17 showed a preventive and protective effect in the S. typhimurium infection model. Our study represents a potential anti-infection strategy that uses probiotics to prevent and resist pathogen infections, possibly by modulating the gut microbiota of the host.
Data availability statement
The datasets presented in this study can be found in online repositories. The names of the repository/repositories and accession number(s) can be found at: https://www.ncbi.nlm.nih.gov/, PRJNA943474.
Ethics statement
The animal study was approved by Institutional Animal Ethics Examination Committee of the Guangxi Veterinary Research Institute. The study was conducted in accordance with the local legislation and institutional requirements.
Author contributions
YY: Conceptualization, Formal analysis, Methodology, Writing – original draft, Writing – review & editing. HP: Conceptualization, Formal analysis, Funding acquisition, Project administration, Resources, Supervision, Writing – review & editing. HB: Data curation, Writing – review & editing. ZP: Formal analysis, Investigation, Writing – review & editing. ZC: Investigation, Methodology, Writing – review & editing. CM: Investigation, Methodology, Software, Writing – review & editing. MZ: Investigation, Methodology, Writing – review & editing. JL: Data curation, Software, Writing – review & editing. CL: Investigation, Software, Writing – review & editing. YG: Funding acquisition, Investigation, Methodology, Writing – review & editing. LW: Data curation, Formal analysis, Software, Writing – review & editing. LT: Formal analysis, Resources, Supervision, Writing – review & editing. ZQ: Investigation, Methodology, Writing – review & editing. JZ: Software, Visualization, Writing – review & editing. TW: Conceptualization, Formal analysis, Project administration, Resources, Supervision, Writing – review & editing. YL: Data curation, Formal analysis, Methodology, Software, Writing – review & editing.
Funding
The author(s) declare that financial support was received for the research, authorship, and/or publication of this article. This research was supported by Guangxi Key Research and Development Program, Grant Nos. AB23075145, AB21238003, and AB21220005-4L; Laibing District Key Research and Development Program, Grant Nos. 240110 and 240113; Special Project for the Construction of National Waterfowl Industry Technical System, Grant No. CARS-42-55; Jiangnan Key Research and Development Program, Grant No. 20220620-2; Qingxiu Key Research and Development Program, Grant No. 2022004; Guangxi Department of Agriculture and Rural Affairs Science and Technology Self-Raised Project, Grant No. Z202214; Guangxi Academy of Agricultural Sciences Stable Funding Research Team Project, Grant No. 2021YT109; Science and Technology Project of Guangxi Agriculture and Rural Department, Grant Nos. Z202218 and Z202219; Guangxi Scientific Research Project, Grant Nos. 22-5, XKJ2325, and XKJ2335; Guangxi Innovation Team Construction Project of National Modern Agricultural Industry Technology System, Grant No. nycytxgxcxtd-2021-09; National College Student Innovation and Entrepreneurship Training Program, Grant No. 202210593024; Special Project for the Construction of National Beef Cattle Industry Technical System, Grant No. GZCYTX-03; Guizhou Key Research and Development Program, Grant Nos. [2020]1Y075 and NY [2018]016.
Conflict of interest
The authors declare that the research was conducted in the absence of any commercial or financial relationships that could be construed as a potential conflict of interest.
Publisher’s note
All claims expressed in this article are solely those of the authors and do not necessarily represent those of their affiliated organizations, or those of the publisher, the editors and the reviewers. Any product that may be evaluated in this article, or claim that may be made by its manufacturer, is not guaranteed or endorsed by the publisher.
Supplementary material
The Supplementary material for this article can be found online at: https://www.frontiersin.org/articles/10.3389/fmicb.2024.1450690/full#supplementary-material
SUPPLEMENTARY FIGURE S1 | Comprehensive analysis of microbial diversity. (A) Good’s coverage analysis: this panel depicts the sequencing coverage of the sample data, indicating the relationship between the sequencing depth and the estimated species richness. (B) Dilution curve: the dilution curve illustrates the species richness as a function of the number of sequences sampled. This curve is used to estimate the total number of species present in the sample and to determine if the sampling effort is sufficient to capture the majority of the species diversity. (C) Accumulation curve: the accumulation curve shows the increase in the number of observed species with the addition of more samples or sequences. It is a graphical representation of species richness over the sampling effort, helping to identify if the sampling is exhaustive or if more samples are needed to achieve a comprehensive understanding of the microbial community’s diversity. Control group: chicks were orally administered PBS on the 8th and 9th days. LP group: chicks were orally administered L. plantarum from the 1st to the 7th day and given PBS on the 8th and 9th days. LP + Sty group: chicks were orally administered L. plantarum from the 1st to the 7th day and were given S. typhimurium on the 8th and 9th days. S + Sty group: chicks were orally administered a commercial probiotic from the 1st to the 7th day and given PBS on the 8th and 9th days. Sty group: chicks were orally administered S. typhimurium on the 8th and 9th days.
References
Abudabos, A. M., Alyemni, A. H., Dafalla, Y. M., and Khan, R. U. (2018). The effect of phytogenics on growth traits, blood biochemical and intestinal histology in broiler chickens exposed to Clostridium perfringens challenge. J. Appl. Anim. Res. 46, 691–695. doi: 10.1080/09712119.2017.1383258
Abudabos, A. M., Yehia, H. M., Alotybi, M. N., Garelnabi, A. R., and Alyemni, A. H. (2014). Effects of direct-fed probiotics on broiler performance and susceptibility to oral Salmonella enteritidis challenge. J. Food Agric. Environ. 1212, 30–34.
Agyare, C., Boamah, V. E., Zumbi, C. N., and Osei, F. B. (2019). “Antibiotic use in poultry production and its effects on bacterial resistance” in Antimicrobial resistance—a global threat (London: IntechOpen).
Ao, T. T., Feasey, N. A., Gordon, M. A., Keddy, K. H., Angulo, F. J., and Crump, J. A. (2015). Global burden of invasive nontyphoidal Salmonella disease, 2010(1). Emerg. Infect. Dis. 21, 941–949. doi: 10.3201/eid2106.140999
Apajalahti, J., Kettunen, A., and Graham, H. (2004). Characteristics of the gastrointestinal microbial communities, with special reference to the chicken. Worlds Poult. Sci. J. 60, 223–232. doi: 10.1079/WPS20040017
Assem, A. H., Lena, K., and Sofia, B. (2021). COVID-19, livestock systems and food security in developing countries: a systematic review of an emerging literature. Pathogens 10:586. doi: 10.3390/pathogens10050586
Baikui, W., Yuanhao, Z., Yulong, M., Li, G., Xiang, L., Shujie, X., et al. (2021). Dietary supplementation with Lactobacillus plantarum ameliorates compromise of growth performance by modulating short-chain fatty acids and intestinal dysbiosis in broilers under Clostridium perfringens challenge. Front. Nutr. 8:706148. doi: 10.3389/fnut.2021.706148
Barman, M., Unold, D., Shifley, K., Amir, E., Hung, K., Bos, N., et al. (2008). Enteric salmonellosis disrupts the microbial ecology of the murine gastrointestinal tract. Infect. Immun. 76, 907–915. doi: 10.1128/IAI.01432-07
Beristain-Covarrubias, N., Perez-Toledo, M., Flores-Langarica, A., Zuidscherwoude, M., Hitchcock, J. R., Channell, W. M., et al. (2018). Salmonella-induced thrombi in mice develop asynchronously in the spleen and liver and are not effective bacterial traps. Blood 133, 600–604. doi: 10.1182/blood-2018-08-867267
Bohnhoff, M., Drake, B. L., and Miller, C. P. (1954). Effect of streptomycin on susceptibility of intestinal tract to experimental Salmonella infection. Proc. Soc. Exp. Biol. Med. 86:132. doi: 10.3181/00379727-86-21030
Bolyen, E., Rideout, J. R., Dillon, M. R., Bokulich, N. A., Abnet, C. C., Al-Ghalith, G. A., et al. (2019). Reproducible, interactive, scalable and extensible microbiome data science using qiime 2. Nat. Biotechnol. 37, 852–857. doi: 10.1038/s41587-019-0209-9
Boone, D. R., Castenholz, R. W., and Garrity, G. M. (2001). Bergey’s manual of systematic bacteriology. 2nd Edn. New York: Springer.
Brown, K., Decoffe, D., Molcan, E., and Gibson, D. L. (2012). Diet-induced dysbiosis of the intestinal microbiota and the effects on immunity and disease. Nutrients 4, 1095–1119. doi: 10.3390/nu4081095
Callahan, B. J., Mcmurdie, P. J., Rosen, M. J., Han, A. W., and Holmes, S. P. (2016). DADA2: high resolution sample inference from amplicon data. Nat. Methods 13, 581–583. doi: 10.1038/nmeth.3869
Carter, P. B., and Collins, F. M. (1974). The route of enteric infection in normal mice. J. Exp. Med. 139, 1189–1203. doi: 10.1084/jem.139.5.1189
Cavicchioli, V. Q., Camargo, A. C., Todorov, S. D., and Nero, L. A. (2017). Novel bacteriocinogenic Enterococcus hirae and Pediococcus pentosaceus strains with antilisterial activity isolated from Brazilian artisanal cheese. J. Dairy Sci. 100, 2526–2535. doi: 10.3168/jds.2016-12049
Celi, P., Cowieson, A. J., Fru-Nji, F., Steinert, R. E., Kluenter, A. M., and Verlhac, V. (2017). Gastrointestinal functionality in animal nutrition and health: new opportunities for sustainable animal production. Anim. Feed Sci. Technol. 234, 88–100. doi: 10.1016/j.anifeedsci.2017.09.012
Chao, A., and Bunge, J. (2015). Estimating the number of species in a stochastic abundance model. Biometrics 58, 531–539. doi: 10.1111/j.0006-341x.2002.00531.x
Chen, Q., Tong, C., Ma, S., Zhou, L., Zhao, L., and Zhao, X. (2017). Involvement of microRNAs in probiotics-induced reduction of the cecal inflammation by Salmonella typhimurium. Front. Immunol. 8:704. doi: 10.3389/fimmu.2017.00704
Dragana, S., Geier, M. S., Hughes, R. J., Denman, S. E., Moore, R. J., and Forster, R. J. (2013). Highly variable microbiota development in the chicken gastrointestinal tract. PLoS One 8:e84290. doi: 10.1371/journal.pone.0084290
Duan, B., Liu, R., Shi, Y., Sun, A., Tang, Z., Wang, C., et al. (2022). Lactobacillus plantarum synergistically regulates m1 macrophage polarization in resistance against Salmonella enterica serovar typhimurium infection. Front. Microbiol. 13:933550. doi: 10.3389/fmicb.2022.933550
Ducarmon, Q. R., Zwittink, R. D., Hornung, B., Schaik, W. V., and Kuijper, E. J. (2019). Gut microbiota and colonization resistance against bacterial enteric infection. Microbiol. Mol. Biol. Rev. 83, e7–e19. doi: 10.1128/MMBR.00007-19
Eng, S., Pusparajah, P., Mutalib, N. A., Ser, H., Chan, K., and Lee, L. (2015). Salmonella: a review on pathogenesis, epidemiology and antibiotic resistance. Front. Life Sci. 8, 284–293. doi: 10.1080/21553769.2015.1051243
Ferrari, R. G., Rosario, D., Neto, A. C., Borges, S., and Junior, C. (2019). Worldwide epidemiology of Salmonella serovars in animal-1 based foods: a meta-analysis. Appl. Environ. Microbiol. 85, 591–619. doi: 10.1128/AEM.00591-19
Gong, X. Y., Wei, M., Jiang, Q. F., Liu, S. D., Wang, Z., and de Timary, P. (2015). Effects of Bacillus coagulans on growth performance and antioxidant function of broilers infected with Salmonella enteritidis. Chin. J. Anim. Husb. 51:7. doi: 10.3382/ps/pew254
Guo, M., Li, M., Zhang, C., Zhang, X., and Wu, Y. (2020). Dietary administration of the Bacillus subtilis enhances immune responses and disease resistance in chickens. Front. Microbiol. 11:1768. doi: 10.3389/fmicb.2020.01768
Guo, W., Xiang, Q., Mao, B., Tang, X., and Chen, W. (2021). Protective effects of microbiome-derived inosine on lipopolysaccharide-induced acute liver damage and inflammation in mice via mediating the TLR4/nf-κb pathway. J. Agric. Food Chem. 69, 7619–7628. doi: 10.1021/acs.jafc.1c01781
Hauser, C., Thielmann, J., and Muranyi, P. (2016). “Organic acids: usage and potential in antimicrobial packaging” in Antimicrobial food packaging (Amsterdam: Elsevier).
Hayashi, R. M., Lourenço, M. C., Kraieski, A. L., Araujo, R. B., Gonzalez-Esquerra, R., Leonardecz, E., et al. (2018). Effect of feeding Bacillus subtilis spores to broilers challenged with Salmonella enterica serovar Heidelberg Brazilian strain ufpr1 on performance, immune response, and gut health. Front. Vet. Sci. 5:13. doi: 10.3389/fvets.2018.00013
Herlemann, D. P., Labrenz, M., Jürgens, K., Bertilsson, S., Waniek, J. J., and Andersson, A. F. (2011). Transitions in bacterial communities along the 2000 km salinity gradient of the Baltic Sea. ISME J. 5, 1571–1579. doi: 10.1038/ismej.2011.41
Hill, T. C. J., Walsh, K. A., Harris, J. A., and Ett, B. F. M. (2002). Using ecological diversity measures with bacterial communities. FEMS Microbiol. Ecol. 43, 1–11. doi: 10.1111/j.1574-6941.2003.tb01040.x
Huang, W. C., Pan, C. H., Wei, C. C., and Huang, H. Y. (2020). Lactobacillus plantarum ps128 improves physiological adaptation and performance in triathletes through gut microbiota modulation. Nutrients 12:2315. doi: 10.3390/nu12082315
Javadi, K., Emadzadeh, M. R., Mohammadzadeh, H. M. S., Halaji, M., Parsian, H., Rajabnia, M., et al. (2024). Anti-biofilm and antibacterial effect of bacteriocin derived from Lactobacillus plantarum on the multidrug-resistant Acinetobacter baumannii. Protein Expr. Purif. 226:106610. doi: 10.1016/j.pep.2024.106610
Jia, T., and Pamer, E. G. (2009). Immunology. Dispensable but not irrelevant. Science 325, 549–550. doi: 10.1126/science.1178329
Jiangrang, L., Umelaalim, I., Barry, H., Charles, H., Maurer, J. J., and Lee, M. D. (2003). Diversity and succession of the intestinal bacterial community of the maturing broiler chicken. Appl. Environ. Microbiol. 69, 6816–6824. doi: 10.1128/AEM.69.11.6816-6824.2003
Jinfeng, H., Yongwei, W., Dan, S., Zixian, L., Zhenglin, D., Haijiang, M., et al. (2018). Effects of Clostridium butyricum and Lactobacillus plantarum on growth performance, immune function and volatile fatty acid level of caecal digesta in broilers. Food Agric. Immunol. 29, 797–807. doi: 10.1080/09540105.2018.1457013
Kamada, N., Kim, Y. G., Sham, H. P., Vallance, B. A., Puente, J. L., Martens, E. C., et al. (2012). Regulated virulence controls the ability of a pathogen to compete with the gut microbiota. Science 336, 1325–1329. doi: 10.1126/science.1222195
Kamdar, K., Khakpour, S., Chen, J., Leone, V., Brulc, J., Mangatu, T., et al. (2016). Genetic and metabolic signals during acute enteric bacterial infection alter the microbiota and drive progression to chronic inflammatory disease. Cell Host Microbe 19, 21–31. doi: 10.1016/j.chom.2015.12.006
Khan, R. U., and Naz, S. (2013). The applications of probiotics in poultry production. Worlds Poult. Sci. J. 69, 621–632. doi: 10.1017/S0043933913000627
Khochamit, N., Siripornadulsil, S., Sukon, P., and Siripornadulsil, W. (2020). Bacillus subtilis and lactic acid bacteria improve the growth performance and blood parameters and reduce Salmonella infection in broilers. Vet. World. 13, 2663–2672. doi: 10.14202/vetworld.2020.2663-2672
Konkol, Y., Keskitalo, A., Vuorikoski, H., Pietil, S., Elo, L. L., Munukka, E., et al. (2019). Chronic nonbacterial prostate inflammation in a rat model is associated with changes of gut microbiota that can be modified with a galactoglucomannan-rich hemicellulose extract in the diet. BJU Int. 123, 899–908. doi: 10.1111/bju.14553
Lin, L., and Zhang, J. (2017). Role of intestinal microbiota and metabolites on gut homeostasis and human diseases. BMC Immunol. 18:2. doi: 10.1186/s12865-016-0187-3
Liu, Y., Wang, Q., Liu, H., Niu, J. I., Jiao, N., Huang, L., et al. (2022). Effects of dietary bopu powder supplementation on intestinal development and microbiota in broiler chickens. Front. Microbiol. 13:1019130. doi: 10.3389/fmicb.2022.1019130
Lopez, C. A., Miller, B. M., Rivera-Chavez, F., Velazquez, E. M., Byndloss, M. X., Chavez-Arroyo, A., et al. (2016). [research article] virulence factors enhance Citrobacter rodentium expansion through aerobic respiration. Science 353, 1249–1253. doi: 10.1126/science.aag3042
Lutful, K. S. (2009). The role of probiotics in the poultry industry. Int. J. Mol. Sci. 10, 3531–3546. doi: 10.3390/ijms10083531
Madsen, K., Cornish, A., Soper, P., McKaigney, C., Jijon, H., Yachimec, C., et al. (2001). Probiotic bacteria enhance murine and human intestinal epithelial barrier function. Gastroenterology 121, 580–591. doi: 10.1053/gast.2001.27224
Maeve, H., Maria, H., Maria, M. A., Mark, F., and Brijesh, T. (2017). Future protein supply and demand: strategies and factors influencing a sustainable equilibrium. Foods 6:53. doi: 10.3390/foods6070053
Mager, L. F., Burkhard, R., Pett, N., Cooke, N., Brown, K., Ramay, H., et al. (2020). Microbiome-derived inosine modulates response to checkpoint inhibitor immunotherapy. Science 369, 1481–1489. doi: 10.1126/science.abc3421
Majowicz, S. E., Musto, J., Scallan, E., Angulo, F. J., Kirk, M., O’Brien, S. J., et al. (2010). The global burden of nontyphoidal Salmonella gastroenteritis. Clin. Infect. Dis. 50, 882–889. doi: 10.1086/650733
Makras, L., Triantafyllou, V., Fayol-Messaoudi, D., Adriany, T., Zoumpopoulou, G., Tsakalidou, E., et al. (2006). Kinetic analysis of the antibacterial activity of probiotic lactobacilli towards Salmonella enterica serovar typhimurium reveals a role for lactic acid and other inhibitory compounds. Res. Microbiol. 157, 241–247. doi: 10.1016/j.resmic.2005.09.002
Marcq, C., Cox, E., Szalo, I. M., Thewis, A., and Beckers, Y. (2011). Salmonella typhimurium oral challenge model in mature broilers: bacteriological, immunological, and growth performance aspects. Poult. Sci. 90, 59–67. doi: 10.3382/ps.2010-01017
Markowiak, P., and Śliżewska, K. (2017). Effects of probiotics, prebiotics, and synbiotics on human health. Nutrients 9:1021. doi: 10.3390/nu9091021
Masteller, E. L., Pharr, G. T., Funk, P. E., and Thompson, C. B. (1997). Avian B cell development. Int. Rev. Immunol. 15, 185–206. doi: 10.3109/08830189709068176
Menconi, A., Wolfenden, A. D., Shivaramaiah, S., Terraes, J. C., Urbano, T., Kuttel, J., et al. (2011). Effect of lactic acid bacteria probiotic culture for the treatment of Salmonella enterica serovar Heidelberg in neonatal broiler chickens and Turkey poults. Poult. Sci. 90, 561–565. doi: 10.3382/ps.2010-01220
Neveling, D. P., van Emmenes, L., Ahire, J. J., Pieterse, E., Smith, C., and Dicks, L. (2020). Effect of a multi-species probiotic on the colonisation of Salmonella in broilers. Probiotics Antimicrob. Proteins 12, 896–905. doi: 10.1007/s12602-019-09593-y
Norma, H., and Santos, G. (2018). Animals as sources of food-borne pathogens: a review. Anim. Nutr. 4, 250–255. doi: 10.1016/j.aninu.2018.04.006
OECD, Food and Agriculture Organization of the United Nations (2016). OECD-FAO agricultural outlook 2016–2025. Paris: OECD Publishing.
Ohashi, Y., Inoue, R., Tanaka, K., Matsuki, T., Umesaki, Y., and Ushida, K. (2001). Lactobacillus casei strain shirota-fermented milk stimulates indigenous lactobacilli in the pig intestine. J. Nutr. Sci. Vitaminol. 47, 172–176. doi: 10.3177/jnsv.47.172
Ohimain, E. I., and Ofongo, R. (2012). The effect of probiotic and prebiotic feed supplementation on chicken health and gut microflora: a review. Int. J. Anim. Vet. Adv. 4, 135–143.
Ohland, C. L., and Macnaughton, W. K. (2010). Probiotic bacteria and intestinal epithelial barrier function. Am. J. Physiol. Gastrointest. Liver Physiol. 298, G807–G819. doi: 10.1152/ajpgi.00243.2009
Pérez-Ramos, A., Madi-Moussa, D., Coucheney, F., and Drider, D. (2021). Current knowledge of the mode of action and immunity mechanisms of lab-bacteriocins. Microorganisms 9:2107. doi: 10.3390/microorganisms9102107
Santos, A. D., Ferrari, R. G., and Conte-Junior, C. A. (2018). Virulence factors in Salmonella typhimurium: the sagacity of a bacterium. Curr. Microbiol. 76, 762–773. doi: 10.1007/s00284-018-1510-4
Stecher, B., Robbiani, R., Walker, A. W., Westendorf, A. M., Barthel, M., Kremer, M., et al. (2007). Salmonella enterica serovar typhimurium exploits inflammation to compete with the intestinal microbiota. PLoS Biol. 5, 2177–2189. doi: 10.1371/journal.pbio.0050244
Štofilová, J., Langerholc, T., Botta, C., Treven, P., Gradišnik, L., Salaj, R., et al. (2017). Cytokine production in vitro and in rat model of colitis in response to Lactobacillus plantarum ls/07. Biomed. Pharmacother. 94, 1176–1185. doi: 10.1016/j.biopha.2017.07.138
Takahashi, S., Egawa, Y., Simojo, N., Tsukahara, T., and Ushida, K. (2007). Oral administration of Lactobacillus plantarum strain lq80 to weaning piglets stimulates the growth of indigenous lactobacilli to modify the lactobacillal population. J. Gen. Appl. Microbiol. 53, 325–332. doi: 10.2323/jgam.53.325
Valdes, A. M., Walter, J., Segal, E., and Spector, T. D. (2018). Re: role of the gut microbiota in nutrition and health. BMJ 361, k2179–k2122. doi: 10.1136/bmj.k2179
Vandeplas, S., Dauphin, R. D., Thiry, C., Beckers, Y., Welling, G. W., Thonart, P., et al. (2009). Efficiency of a Lactobacillus plantarum-xylanase combination on growth performances, microflora populations, and nutrient digestibilities of broilers infected with Salmonella typhimurium. Poult. Sci. 88, 1643–1654. doi: 10.3382/ps.2008-00479
Wang, L., Li, L., Lv, Y., Chen, Q., Feng, J., and Zhao, X. (2018). Lactobacillus plantarum restores intestinal permeability disrupted by Salmonella infection in newly-hatched chicks. Sci. Rep. 8:2229. doi: 10.1038/s41598-018-20752-z
Wei, S., Morrison, M., and Yu, Z. (2013). Bacterial census of poultry intestinal microbiome. Poult. Sci. 92, 671–683. doi: 10.3382/ps.2012-02822
Wenguang, X., Yulin, W., Yongxue, S., Liping, M., Qinglin, Z., Xiaotao, J., et al. (2018). Antibiotic-mediated changes in the fecal microbiome of broiler chickens define the incidence of antibiotic resistance genes. Microbiome 6:34. doi: 10.1186/s40168-018-0419-2
Wilson, K. M., Bourassa, D. V., Davis, A. J., Freeman, M. E., and Buhr, R. J. (2016). The addition of charcoals to broiler diets did not alter the recovery of Salmonella typhimurium during grow-out. Poult. Sci. 95, 694–704. doi: 10.3382/ps/pev371
Winter, S. E., Thiennimitr, P., Winter, M. G., Butler, B. P., Huseby, D. L., Crawford, R. W., et al. (2010). Gut inflammation provides a respiratory electron acceptor for Salmonella. Nature 467, 426–429. doi: 10.1038/nature09415
Wu, D., Teng, D., Wang, X., Dai, C., and Wang, J. (2014). Saccharomyces boulardii prevention of the hepatic injury induced by Salmonella enteritidis infection. Can. J. Microbiol. 60, 681–686. doi: 10.1139/cjm-2014-0259
Xia, T., Zhang, B., Li, S., Fang, B., Duan, W., Zhang, J., et al. (2020). Vinegar extract ameliorates alcohol-induced liver damage associated with the modulation of gut microbiota in mice. Food Funct. 11, 2898–2909. doi: 10.1039/c9fo03015h
Xing, J. H., Li, Q. Y., Zhao, W., Yang, G. L., and Wang, C. F. (2021). Bacillus subtilis BSH has a protective effect on Salmonella infection by regulating the intestinal flora structure in chickens. Microb. Pathog. 155:104898. doi: 10.1016/j.micpath.2021.104898
Yin, Y., Pan, Y., Li, X., Li, J., Liao, Y., Li, C., et al. (2023). Study on biological characteristics and antibacterial activity in vitro of a Lactobacillus plantarum strain. China Anim. Husb. Vet. Med. 50, 666–673. doi: 10.16431/j.cnki.1671-7236.2023.02.024
Yu, M., Cheng, B., Zhang, Z. G., and Yang, R. F. (2021). Effects of compound probiotics on growth performance, gut microbiota and immune function of broilers. Mod. Anim. Husb. Vet. Med. 39–42.
Zhang, X., Coker, O. O., Chu, E. S., Fu, K., and Yu, J. (2020). Dietary cholesterol drives fatty liver-associated liver cancer by modulating gut microbiota and metabolites. Gut 70, 761–774. doi: 10.1136/gutjnl-2019-319664
Zhao, W., Peng, C., Sakandar, H. A., Kwok, L. Y., and Zhang, W. (2021). Meta-analysis: randomized trials of Lactobacillus plantarum on immune regulation over the last decades. Front. Immunol. 12:643420. doi: 10.3389/fimmu.2021.643420
Zhou, Q., Gu, R., Li, P., Lu, Y., Chen, L., and Gu, Q. (2020). Anti-Salmonella mode of action of natural l-phenyl lactic acid purified from Lactobacillus plantarum zj316. Appl. Microbiol. Biotechnol. 104, 5283–5292. doi: 10.1007/s00253-020-10503-4
Keywords: probiotics, yellow-feathered broiler, growth performance, intestine tissue, gut health, immune response
Citation: Yin Y, Peng H, Bai H, Pei Z, Chen Z, Ma C, Zhu M, Li J, Li C, Gong Y, Wang L, Teng L, Qin Z, Zhou J, Wei T and Liao Y (2024) Enhancing resistance to Salmonella typhimurium in yellow-feathered broilers: a study of a strain of Lactiplantibacillus plantarum as probiotic feed additives. Front. Microbiol. 15:1450690. doi: 10.3389/fmicb.2024.1450690
Edited by:
Jack Wong, Saint Francis University, ChinaReviewed by:
Anand Kumar, Los Alamos National Laboratory (DOE), United StatesSooyeon Song, Jeonbuk National University, Republic of Korea
Gang Liu, Qingdao Agricultural University, China
Copyright © 2024 Yin, Peng, Bai, Pei, Chen, Ma, Zhu, Li, Li, Gong, Wang, Teng, Qin, Zhou, Wei and Liao. This is an open-access article distributed under the terms of the Creative Commons Attribution License (CC BY). The use, distribution or reproduction in other forums is permitted, provided the original author(s) and the copyright owner(s) are credited and that the original publication in this journal is cited, in accordance with accepted academic practice. No use, distribution or reproduction is permitted which does not comply with these terms.
*Correspondence: Hao Peng, aHBlbmcyMDA2QDE2My5jb20=; Tianchao Wei, dGN3ZWk4OEAxMjYuY29t; Yuying Liao, TGlhb3l1eWluZ0AxMjYuY29t
†These authors have contributed equally to this work and share first authorship