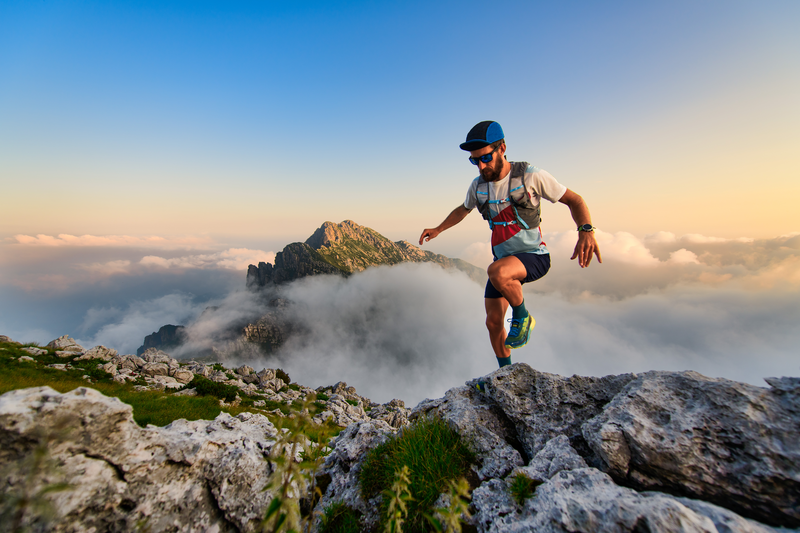
95% of researchers rate our articles as excellent or good
Learn more about the work of our research integrity team to safeguard the quality of each article we publish.
Find out more
ORIGINAL RESEARCH article
Front. Microbiol. , 08 January 2025
Sec. Microbe and Virus Interactions with Plants
Volume 15 - 2024 | https://doi.org/10.3389/fmicb.2024.1447527
As the world’s population grows, pursuing sustainable agricultural production techniques to increase crop yields is critical to ensuring global food security. The development and application of biological agents is of great significance in promoting the sustainable development of agriculture. This study aimed to investigate the role of JZ (compound microbial agent) and MZ (biological agent made from plant materials) in improving the rhizosphere microecological environment and nutrient availability for rice. This study found that JZ enriched Cyanobacteria with biological nitrogen fixation functions; spraying MZ can enrich some beneficial microbiota, such as Bradyrhizobium, playing a role in symbiotic nitrogen fixation. Meanwhile, JZ and MZ were found to affect rhizosphere soil metabolism and improve potassium and nitrogen availability. JZ may promote the degradation of fungicides in the rhizosphere soil environment. Overall, applying biological agents through optimizing rice growing environment to improve yield showed great potential.
As the world’s population grows, food production faces unprecedented challenges. By 2050, the world’s population will reach 9.6 billion, an increase of 34% from today (Tripathi et al., 2019). Pursuing sustainable agricultural production technologies to increase crop yields is critical to ensuring global food security. Traditional agricultural production has certain limitations. For example, large amounts of pesticides and chemical fertilizers are used during crop cultivation. This affects the quality of agricultural products and causes environmental pollution. Excessive chemical substances such as fertilizers and pesticides will seriously pollute water (Kumar et al., 2021), decline soil quality, and affect the ecological balance. In addition, excessive resource consumption is also a major disadvantage of the traditional agricultural model. Under this model, lower land, water, and energy use efficiency may lead to excessive consumption and waste of resources. In recent years, green agriculture has received widespread attention (Koohafkan et al., 2012; Li et al., 2021). Green agriculture focuses on increasing yields while considering the harmony between agricultural production and the natural environment. By applying more advanced production technologies and sustainable agricultural management methods, creating a high-yielding, efficient, healthy, and adaptable agricultural ecosystem can provide important scientific guarantees for solving global food security issues. With the increasing attention paid to sustainable agricultural development, the continuous innovation and optimization of crop production technology has become a research hotspot.
Rhizosphere soil contains diverse microorganisms whose importance to plant health and productivity cannot be underestimated. In the rhizosphere soil, plants interact with associated microorganisms through chemical signals produced in response to specific stimuli (Solomon et al., 2023). Many of the microbes in the rhizosphere defend plants against pathogen invasion and help acquire nutrients from the soil (Lee et al., 2021; Chepsergon and Moleleki, 2023). For example, Lactobacillus plays a crucial role in promoting plant growth by increasing soil phosphate solubilizing ability (Anzuay et al., 2013; Si et al., 2024) and stimulating root elongation (Limanska et al., 2013; Si et al., 2024). Bacillus amyloliquefaciens, an excellent biofertilizer and biocontrol agent, regulates plant cell growth and increases plant resistance to biotic and abiotic stresses (Luo et al., 2022). Lots of evidence showed that Brevibacillus laterosporus has the ability to contribute to fertility and to support plant health (Javed et al., 2020; Ruiu, 2020; Hamze and Ruiu, 2022; Wang et al., 2022). Therefore, using beneficial microorganisms to improve crop yield and conducting in-depth research on the influencing mechanisms are of great significance to promoting sustainable agricultural development. The compound microbial agent JZ used in this study contains a variety of beneficial bacteria, such as Bacillus licheniformis, Bacillus amyloliquefaciens, Lactobacillus plantarum, and Lactobacillus casei. This is different from previous studies that focus on the effects of a single bacterial species on plants. The regulation mechanism of this compound microbial agent on plants may be more complex.
In addition, a new type of biological agent MZ made from various plant monomer enzymes or plant extracts and chelated through two unique low-temperature aerobic fermentations has recently attracted the attention of many Chinese researchers. The main ingredients of this biological agent obtained by special processing of plant materials include papain, chamomile extract, bitter bark extract, etc., which has been applied to many crops, such as wheat (Sang et al., 2021), tea trees (You et al., 2021), and rice (Shao et al., 2022). Shao et al. (2022) found that the application of MZ increased the number of filled grains per panicle by 2.4 grains, the thousand-grain weight by 0.6 g, and the theoretical yield per 667 m2 by 63.5 kg, with a yield increase of 10.23% compared with the control. This means that MZ has excellent potential in increasing rice yield. Its application in agriculture is an effective means to promote production and increase income. Although MZ has shown potential in increasing rice yield, its effects on rice rhizosphere microbial diversity and soil metabolism have not been studied.
Based on previous studies, we speculated that using MZ and JZ may affect rhizosphere microecological environment and nutrient availability to promote rice production. The results of this study will provide a reference for achieving sustainable agriculture.
This study was conducted in Shenzhen-Shanwei Special Cooperation Zone, Shanwei City (20°27′-23°28′N and 114°54′-116°13′E) in 2023. According to Shenzhen Climate Bulletin, the average annual precipitation in the Shenzhen-Shanwei Special Cooperation Zone was 1928.9 mm, the maximum daily rainfall was 238.7 mm, the maximum 1-h rainfall was 80.4 mm, and the annual average temperature was 23.9°C in 2023.
The rice variety Liliangyou8022, provided by Hunan Hybrid Rice Research Center, was used in this study. JZ was provided by Inner Mongolia Ketuo Microecological Technology Development Co., Ltd. The effective strains of JZ: Bacillus licheniformis, Bacillus amyloliquefaciens, Lactobacillus plantarum, and Lactobacillus casei; the number of effective viable bacteria≥2.0 × 108 cfu·ml−1. MZ was provided by Heilongjiang Senqi Biotechnology Co., Ltd. The main ingredients of MZ are papain, chamomile extract, and bitter bark extract.
Rice seedlings were transplanted at the 4-leaf stage, with 2–3 plants per hole and a 19.98 × 26.64 cm density. The dosage of JZ per 667 m2 was 7 L aqueous solution (diluted with water to 20 times the undiluted original solution when used). JZ aqueous solution was evenly poured at the 4-leaf stage (2 days earlier than the day of transplanting), tillering stage, early heading stage, and full heading stage. The dosage of MZ per 667 m2 was 50 mL of undiluted original solution. MZ aqueous solution was sprayed on the leaves at the 4-leaf stage (2 days earlier than the day of transplanting), tillering stage, early heading stage, and full heading stage (50 mL of MZ was mixed with 15 L of water). This study set each treatment as a large plot (120 m2). There were three treatments, namely MZ, JZ, and CKZ (control). Sampling was done by randomly selecting three points in each treatment (with three replicates).
In this study, 300 kg·hm−2 of compound fertilizer (N, P, and K were all 15%) was used as base fertilizer; 225 kg·hm−2 of compound fertilizer and 75 kg·hm−2 of urea were used as tillering fertilizer; 37.5 kg·hm−2 of urea and 112.5 kg·hm−2 of potassium chloride were used as panicle fertilizer.
Determination of soil nutrient content and enzyme activity was performed by South Subtropical Crop Research Institute, China Academy of Tropical Agricultural Sciences.
Soil from 0-10 cm layer was collected at the heading stage. pH, rapidly available potassium, available phosphorus, alkaline-hydrolysable nitrogen, total nitrogen, total phosphorus, total potassium, and organic matter content were determined based on the method of Bao (2000).
The determination of sucrase (S-SC) activity was described according to the method of the kit provided by Suzhou Grace Biotechnology Co., Ltd. S-SC catalyzes the degradation of sucrose to produce reducing sugars, which further react with 3,5-dinitrosalicylic acid to generate colored amino compounds with characteristic light absorption at 540 nm. Within a certain range, the increase rate of 540 nm light absorption is proportional to S-SC activity.
Catalase (S-CAT) activity was measured according to the method described in the kit provided by Beijing Solarbio Science & Technology Co., Ltd. S-CAT plays an important role in the H2O2 scavenging system. H2O2 has a characteristic absorption peak at 240 nm. By measuring the change in absorbance of the solution after reaction with soil at this wavelength, the level of S-CAT activity can be reflected.
Urease (S-UE) activity was measured according to the method described in the kit provided by Beijing Solarbio Science & Technology Co., Ltd. S-UE can hydrolyze urea to produce ammonia and carbonic acid. The indophenol blue colorimetric method was used to determine the NH3-N produced by S-UE hydrolyzes urea.
Acid phosphatase (S-ACP) activity was measured according to the method described in the kit provided by Beijing Solarbio Science & Technology Co., Ltd. In an acidic environment, S-ACP catalyzes the hydrolysis of disodium phenyl phosphate to produce phenol and disodium hydrogen phosphate. The S-ACP activity can be calculated by measuring the amount of phenol produced.
The SPAD value was measured by SPAD-502Plus (Konica Minolta) at the heading stage. Rice yield was measured at the maturity stage.
Rhizosphere soil was collected at the heading stage, with 6 biological replicates for each treatment. Metabolome detection was performed by Shanghai Majorbio Bio-pharm Technology Co., Ltd.
Soil sample: 1,000 ± 5 mg sample and a 6 mm diameter grinding bead were placed in a 2 mL centrifuge tube. Then, 1,000 μL of extraction solution was added. After grinding with a grinder for 6 min (−10°C, 50 Hz), low-temperature ultrasonic extraction was performed for 30 min (5°C, 40 KHz). The sample was placed at −20°C for 30 min and then centrifuged for 15 min (13,000 g, 4°C). The supernatant was removed and dried with nitrogen gas. Subsequently, 120 μL of reconstitution solution (acetonitrile: water = 1:1) was added to reconstitute. After vortexing and mixing for 30 s, low-temperature ultrasonic extraction was performed for 5 min (5°C, 40KHz). Finally, the supernatant was used for analysis after centrifugation for 15 min (13,000 g, 4°C).
LC–MS analysis was performed on a Thermo UHPLC-Q Exactive HF-X system.
Chromatographic conditions: the chromatographic column was ACQUITY UPLC HSS T3 (100 mm × 2.1 mm i.d., 1.8 μm; Waters, United States); mobile phase A was 95% water + 5% acetonitrile (containing 0.1% formic acid); mobile phase B was 47.5% acetonitrile + 47.5% isopropanol + 5% water (containing 0.1% formic acid); the injection volume was 3 μL and the column temperature was 40°C.
The samples were ionized by electrospray, and the mass spectrum signals were collected using positive and negative ion scanning modes. The specific parameters were shown in Table 1.
Rhizosphere soil was collected at the heading stage, with three biological replicates for each treatment. 16S rRNA sequencing and ITS sequencing were completed by Shanghai Majorbio Bio-pharm Technology Co., Ltd.
FastPure Soil DNA Isolation Kit (Shanghai Major Yuhua Bio-pharm Technology Co., Ltd); FastPfu Polymerase (TransGen Biotech Co., Ltd); T100 Thermal Cycler PCR instrument (BIO-RAD); JY600C electrophoresis apparatus (Beijing JUNYI DONGFANG Electrophoresis Co., Ltd).
The total genomic DNA of the microbial community was extracted according to the instructions of the FastPure Soil DNA Isolation Kit (Shanghai Major Yuhua Bio-pharm Technology Co., Ltd). The quality of the extracted genomic DNA was checked by 1% agarose gel electrophoresis. The DNA concentration and purity were determined using NanoDrop2000 (Thermo Scientific).
The primer design in this study was shown in Table 2.
The PCR reaction system for 338F_806R was: 5 × FastPfu Buffer (4 μL), 2.5 mM dNTPs (2 μL), 5 μM Forward Primer (0.8 μL), 5 μM Reverse Primer (0.8 μL), FastPfu Polymerase (0.4 μL), BSA (0.2 μL), and Template DNA (10 ng). ddH2O was added to make the system volume 20 μL. The amplification procedure was as follows: Pre-denaturation at 95°C for 3 min; 27 cycles (30 s at 95°C, 30 s at 55°C, 45 s at 72°C); then, the stable extension at 72°C for 10 min, and storage at 4°C (PCR instrument: ABI GeneAmp® 9,700).
The PCR reaction system for ITS1F_ITS2R was: 10 × Buffer (2 μL), 2.5 mM dNTPs (2 μL), 5 μM Forward Primer (0.8 μL), 5 μM Reverse Primer (0.8 μL), rTaq Polymerase (0.2 μL), BSA (0.2 μL), and Template DNA (10 ng). ddH2O was added to make the system volume 20 μL. The amplification procedure was as follows: Pre-denaturation at 95°C for 3 min; 35 cycles (30 s at 95°C, 30 s at 55°C, 45 s at 72°C); then, the stable extension at 72°C for 10 min, and storage at 4°C (PCR instrument: ABI GeneAmp® 9,700).
The sequencing was performed using the Illumina PE300 platform.
SPSS27 was used to perform independent samples T-test.
As shown in Supplementary Table S1, MZ and JZ significantly reduced soil pH. Rapidly available potassium and alkaline-hydrolysable nitrogen increased significantly, and available phosphorus significantly reduced after using MZ and JZ. The organic matter content was increased significantly after MZ treatment, while JZ had no significant effect on the organic matter content. In addition, both MZ and JZ could significantly increase the activities of S-CAT, S-UE, and S-ACP.
This study found that JZ and MZ positively regulated SPAD in rice leaves. The SPAD of JZ was significantly higher than that of CKZ. In addition, both JZ and MZ could significantly increase theoretical rice yield (Supplementary Table S2).
PCA, PLS-DA, and OPLS-DA results showed that the 6 samples of each treatment were distributed in the same area, and two treatments were separated (Figure 1; Supplementary Figures S1–S3). In addition, Q2 was greater than 0.5 in either positive or negative ion mode, indicating that the model had good prediction ability (Supplementary Tables S3–S6).
This study counted 172 differential metabolites, of which 110 were up-regulated, and 62 were down-regulated (Figure 2A). VIP analysis found that the differential metabolites with VIP greater than 4 were chikusetsu saponin iva, lotaustralin, and lysylthreonine, and these three metabolites were all up-regulated after the use of JZ (Figure 2B).
Figure 2. Statistics and analysis of differential metabolites in CKZ/JZ. (A) Volcano plot of differential metabolites. (B) VIP analysis. (C) KEGG annotation analysis. (D) KEGG enrichment analysis.
Further, KEGG annotation analysis found that the differential metabolites were mainly classified into lipid metabolism, carbohydrate metabolism, membrane transport, cancer: overview, biosynthesis of other secondary metabolites, and xenobiotics biodegradation and metabolism (Figure 2C). In addition, KEGG enrichment analysis found that DEGs were mainly enriched in the ABC transporters and biosynthesis of cofactors pathways (Figure 2D).
As shown in Supplementary Figure S4A, 182 differential metabolites were detected in this study, of which 116 were up-regulated and 65 were down-regulated. Momordin B had the highest VIP value, followed by O-oxalylhomoserine (Supplementary Figure S4B).
KEGG annotation analysis found that differential metabolites were mainly classified into membrane transport, cancer: overview, amino acid metabolism, biosynthesis of other secondary metabolites, carbohydrate metabolism, lipid metabolism, xenobiotics biodegradation and metabolism, and digestive system (Supplementary Figure S5B). Further, KEGG enrichment analysis found that the differential metabolites were mainly enriched in the ABC transporters, galactose metabolism, nucleotide metabolism, and purine metabolism pathways (Supplementary Figure S5A).
The results of this study showed that the dilution curve tended to be flat; the sequencing data had basically reached saturation, and it could cover most species in the sample (Figure 3B; Supplementary Figure S6). This study used Ace, Chao, and Sobs indices to reflect community richness and Shannon index to reflect community diversity.
Figure 3. Dilution curve analysis and alpha diversity analysis in CKZ/MZ. (A) alpha diversity analysis; (B) dilution curve analysis.
Alpha diversity analysis found no significant differences in community richness and community diversity between CKZ and JZ (Supplementary Table S7). Likewise, there was no significant change between CKZ and MZ (Figure 3A).
At the phylum level, the dominant microbial communities of CKZ and JZ treatments were Firmicutes, Chloroflexi, Actinobacteriota, Proteobacteria, and Acidobacteriota. Compared to CKZ, the percent of community abundance of Firmicutes and Actinobacteriota in JZ decreased, and that of Chloroflexi increased. At the genus level, the percent of community abundance of Bacillus in JZ increased, and that of unclassified_f__Planococcaceae decreased (Supplementary Figure S7).
A total of 5 dominant microbial communities were detected at the phylum level, namely Firmicutes, Chloroflexi, Proteobacteria, Actinobacteriota, and Acidobacteriota. Compared with CKZ, the percent of community abundance of Firmicutes and Actinobacteriota decreased in MZ treatment; that of Chloroflexi, Proteobacteria, and Acidobacteriota increased. At the genus level, the percent of community abundance of unclassified_f__Planococcaceae decreased in MZ treatment compared with CKZ (Figure 4).
PCA, PCOA, and NMDS analysis showed a clear separation between the two treatments, indicating differences in microbial community composition between the two treatments (Figure 5; Supplementary Figure S8).
In CKZ/JZ, this study detected 182 differential communities at multiple levels, of which 96 were significantly enriched in JZ, and 86 were significantly enriched in CKZ (LDA threshold>2) (Supplementary Figure S9). In CKZ/MZ, 48 differential communities at multiple levels were obtained, of which 46 were significantly enriched in MZ, and 2 were significantly enriched in CKZ (LDA threshold>3) (Supplementary Table S8).
The results of this study showed that the dilution curve tended to be flat; the sequencing data had basically reached saturation, and it could cover most species in the sample (Supplementary Figures S10, S11B).
Alpha diversity analysis found no significant differences in community richness and community diversity between the CKZ and JZ (Supplementary Table S9). In addition, Ace, Sobs, and Chao indexes showed no significant changes after using MZ; the Shannon index decreased significantly after using MZ (Supplementary Figure S11A). These results indicated that the community diversity of MZ was lower compared to CKZ.
At the phylum level, the percent of community abundance of Ascomycota and Basidiomycota in JZ was higher compared with CKZ; that of unclassified_k__Fungi, Rozellomycota, and Mortierellomycota was lower. In addition, at the genus level, the percent of community abundance of Sporidiobolus, Gibberella, and Penicillium was higher in JZ; that of unclassified_k__Fungi, Rozellomycota, Westerdykella, Saitozyma, Talaromyces, and Sordariomycetes was lower compared to CKZ (Supplementary Tables S10, S11).
A total of 6 dominant communities were detected at the phylum level, namely Ascomycota, unclassified_k__Fungi, Rozellomycota, Basidiomycota, Mortierellomycota, and Chytridiomycota. Compared with CKZ, the percent of community abundance of unclassified_k__Fungi, Rozellomycota, Basidiomycota, Mortierellomycota, and Chytridiomycota decreased in MZ treatment; that of Ascomycota increased. At the genus level, the percent of community abundance of Gibberella, Penicillium, and Mortierella increased in MZ treatment compared with CKZ; that of unclassified_k__Fungi, unclassified_p__Rozellomycota, unclassified_p__Rozellomycota, Westerdykella, Saitozyma, unclassified_c__Sordariomycetes, and Talaromyces decreased (Supplementaryt Figure S12).
PCA, PCOA, and NMDS analysis results showed the differences in the community composition between the two treatments (Figure 6A; Supplementary Figure S13). The LDA discriminant histogram showed 110 differential communities in CKZ/JZ, of which 68 were significantly enriched in CKZ and 42 were significantly enriched in JZ (LDA threshold >2) (Figure 6B). In addition, 50 differential communities were screened in CKZ/MZ, of which 17 were significantly enriched in MZ, and 33 were significantly enriched in CKZ (LDA threshold >3) (Supplementary Figure S14).
Figure 6. Lefse multilevel species difference discriminant analysis, PCA, PCOA, and NMDS analysis in CKZ/JZ. (A) PCA, PCOA, and NMDS analysis. (B) Lefse multilevel species difference discriminant analysis.
In this study, the rapidly available potassium and alkaline-hydrolysable nitrogen contents increased significantly after applying JZ and MZ. These are essential active nutrients in the soil and directly affect the growth and development of rice. The significant increase in S-UE activity in this study is an essential contributor to the increase in alkaline-hydrolysable nitrogen because this enzyme plays a role in urea degradation (Cordero et al., 2019). In addition, soil pH dropped significantly after JZ and MZ application. In this acidic soil environment, S-ACP plays an efficient role in organic P mineralization in soil (Huang et al., 2011). In this study, S-ACP activity significantly increased under JZ and MZ treatment conditions, which is beneficial for catalysing the decomposition of organic phosphorus. However, an unexpected result was that the available phosphorus in the JZ and MZ treatment was significantly reduced compared to that in CKZ. Here, we speculated that the decrease in available phosphorus content may be affected by the decrease in pH; in this soil environment, phosphoric acid may form a delayed effect state with calcium, iron or aluminum, reducing its effectiveness.
Furthermore, the results showed that using JZ and MZ significantly increased S-CAT activity. This antioxidant enzyme plays a role in antioxidant in the soil, indicating that JZ and MZ can prevent soil microorganisms and plants from oxidative damage.
There are various ecological relationships between plants and microorganisms in the rhizosphere, such as competition, exploitation, commensalism, and mutualism, which affect plant growth and the overall function of the ecosystem (Solomon et al., 2023). Acidobacteria is one of the bacterial taxa in soil associated with the decomposition of soil organic matter (Banerjee et al., 2016; Kalam et al., 2020) and is structural and functional keystones in plant–soil microbiomes and agroecosystems (Kalam et al., 2020). In this study, c__Acidobacteriae and o__Acidobacteriales were significantly enriched in JZ treatment, indicating that JZ may play a role in the decomposition of soil organic matter. f__unclassified_p__Cyanobacteria, o__unclassified_p__Cyanobacteria, c__unclassified_p__Cyanobacteria, and g__unclassified_p__Cyanobacteria were also representatives significantly enriched in JZ treatment. According to report, Cyanobacteria play a major role in soil nitrogen fixation in agricultural fields and are widely used in rice fields (Joshi et al., 2020). Previous study showed that Cyanobacteria fix nitrogen up to 30%, produce some phytohormones, vitamins, amino acids, and organic acids, and increase rice productivity (Purwani et al., 2021). Therefore, the enrichment of Cyanobacteria in the JZ treatment may have contributed to the elevation of alkaline-hydrolysable nitrogen in this study, as it fixed nitrogen from the air and transformed it into the soil.
Additionally, ITS sequencing found that a total of 42 differential microbial communities, were significantly enriched in JZ, including g__Cladosporium and g__Plectosphaerella. Cladosporium species can secrete beneficial secondary metabolites to improve the ability of plants to adapt to new habitats and to sustain plant health and performance (Răut et al., 2021). The nematophagous fungus Plectosphaerella cucumerina has been reported to have the potential to against potato cyst nematodes populations by reducing field populations by up to 60% in trials (Atkins et al., 2003).
Based on the rhizosphere soil microbial community changes, this study conducted rhizosphere soil metabolome detection. 172 differential metabolites were detected between CKZ and JZ (Supplementary Table S12), indicating that rice rhizosphere soil metabolism changed after using JZ. In this study, the VIP values of the three up-regulated differential metabolites chikusetsu saponin iva, lotaustralin, and lysylthreonine were all greater than 4, ranking in the top 3 of all differential metabolites, indicating that these three metabolites had the highest contribution to the difference between the two treatments. Furthermore, this study found that these three metabolites had the strongest correlation with g__Massilia and g__Plectosphaerella. Previous studies found that Massilia sp. WF1 and Massilia sp. WG5 can degrade phenanthrene and repair contaminated soil (Lou et al., 2016; Gu et al., 2021; Xu et al., 2023). Interestingly, g__Massilia was significantly enriched in JZ. This revealed that JZ may potentially promote soil purification by enriching g__Massilia.
To further study the functions of differential metabolites in rhizosphere soil, this study conducted KEGG annotation on all differential metabolites and found that most metabolites were classified into lipid metabolism. Among these metabolites, jasmonic acid was significantly up-regulated after JZ application. Jasmonic acid is an endogenous growth regulatory substance, and exogenous application also has a regulatory effect on plants (Wang et al., 2020). A previous study found that jasmonic acid can contribute to rice resistance against Magnaporthe oryzae (Ma et al., 2022). Exogenous application of jasmonic acid triggers the defense mechanism of rice against Rhizoctonia solani Kühn (Younis et al., 2022). Therefore, up-regulation of jasmonic acid in the rhizosphere soil is beneficial to enhance rice’s disease resistance. In addition, JZ may also play a role in reducing pesticide residues. KEGG compound classification found that tricyclazole and dodemorph were significantly down-regulated; dinotefuran was significantly up-regulated. Since tricyclazole and dodemorph belong to fungicides, we speculated that using JZ changed the rhizosphere soil environment and promoted the degradation of fungicides. Dinotefuran is an insecticide, and its up-regulation may be due to the inhibition of dinotefuran degradation after JZ application. Research on the impact of JZ on pesticide residues in rice fields needs further exploration.
The phylum Bacteroidota represents a group of bacteria, and these bacteria are dominant carbohydrate degraders in the soil (Larsbrink and McKee, 2020; Kruczyńska et al., 2023). Kruczyńska et al. (2023) found that the abundance of these beneficial microorganisms from the Bacteroidota community could potentially be connected with soil fertility, affecting crop yield (Kruczyńska et al., 2023). In this study, Bacteroidota was significantly enriched in the MZ treatment. In addition, at the genus level, Bradyrhizobium and Pseudolabrys were significantly enriched in MZ treatment. Bradyrhizobium performs essential biological functions in soil. Different representatives from the genus Bradyrhizobium were found to play essential roles in photosynthesis, symbiotic nitrogen fixation, and denitrification (Jones et al., 2016). Genus Pseudolabrys is also a beneficial biocontrol microorganism in plant rhizosphere soils (Fox et al., 2007; Tan et al., 2019).
Further, ITS sequencing found Penicillium was significantly enriched in MZ treatment at the genus level. Previous studies have reported that some Penicillium species can promote plant growth by production of PGP phytohormones, solubilization of minerals, or antagonism to phytopathogens (Wakelin et al., 2006; Nath et al., 2012; Radhakrishnan et al., 2013; Babu et al., 2015). Endophytic Penicillium spp. has been investigated for agricultural purposes to reduce the pollution of agricultural farms by chemical pesticides and heavy metals (Toghueo and Boyom, 2020).
In addition, 4-hydroxybutyric acid was up-regulated in the rhizosphere soil after application of MZ (Supplementary Table S13). 4-hydroxybutyrate together with threonine resulted in a significant increase in nitrogen and phosphorus content in root tissue under greenhouse conditions (Pantigoso et al., 2023).
The results of this study demonstrated that JZ and MZ can optimize the rice rhizosphere microecological environment by enriching beneficial microorganisms, adjusting rhizosphere soil metabolism, and improving nitrogen and potassium availability, effectively increasing rice yield, which provided a new agricultural management strategy. In the future, further research can be conducted into the long-term effects of JZ and MZ on rice growth under different environmental conditions, as well as how to optimize their use methods to maximize benefits in rice production.
16S rRNA and ITS sequencing raw data were deposited in the Genome Sequence Archive in National Genomics Data Center, China National Center for Bioinformation/Beijing Institute of Genomics, Chinese Academy of Sciences (GSA: CRA020197 and CRA020200) that were publicly accessible at https://ngdc.cncb.ac.cn/gsa. Metabolome raw data was deposited in the OMIX, China National Center for Bioinformation/Beijing Institute of Genomics, Chinese Academy of Sciences: https://ngdc.cncb.ac.cn/omix with accession no. OMIX007778.
HZ: Writing – original draft, Writing – review & editing. KY: Methodology, Supervision, Writing – review & editing. LN: Conceptualization, Investigation, Methodology, Visualization, Writing – review & editing. LL: Conceptualization, Writing – review & editing. JZ: Methodology, Supervision, Writing – review & editing. KW: Conceptualization, Investigation, Writing – review & editing. HY: Methodology, Supervision, Writing – review & editing. ZW: Methodology, Supervision, Writing – review & editing.
The author(s) declare that financial support was received for the research, authorship, and/or publication of this article. The financial support in this study was from Hainan Province Major Science and Technology Plan (ZDKJ2021002) and National Key Laboratory Independent Project (2022MS15).
The authors declare that the research was conducted in the absence of any commercial or financial relationships that could be construed as a potential conflict of interest.
All claims expressed in this article are solely those of the authors and do not necessarily represent those of their affiliated organizations, or those of the publisher, the editors and the reviewers. Any product that may be evaluated in this article, or claim that may be made by its manufacturer, is not guaranteed or endorsed by the publisher.
The Supplementary material for this article can be found online at: https://www.frontiersin.org/articles/10.3389/fmicb.2024.1447527/full#supplementary-material
Anzuay, M. S., Frola, O., Angelini, J. G., Ludueña, L. M., Fabra, A., and Taurian, T. (2013). Genetic diversity of phosphate-solubilizing peanut (Arachis hypogaea L.) associated bacteria and mechanisms involved in this ability. Symbiosis 60, 143–154. doi: 10.1007/s13199-013-0250-2
Atkins, S., Clark, I., Sosnowska, D., Hirsch, P., and Kerry, B. (2003). Detection and quantification of Plectosphaerella cucumerina, a potential biological control agent of potato cyst nematodes, by using conventional PCR, real-time PCR, selective media, and baiting. Appl. Environ. Microbiol. 69, 4788–4793. doi: 10.1128/AEM.69.8.4788-4793.2003
Babu, A., Kim, S., Yadav, D., Hyum, U., Adhikari, M., and Lee, Y. (2015). Penicillium menonorum: a novel fungus to promote growth and nutrient management in cucumber plants. Mycobiology 43, 49–56. doi: 10.5941/MYCO.2015.43.1.49
Banerjee, S., Kirkby, C. A., Schmutter, D., Bissett, A., Kirkegaard, J. A., and Richardson, A. E. (2016). Network analysis reveals functional redundancy and keystone taxa amongst bacterial and fungal communities during organic matter decomposition in an arable soil. Soil Biol. Biochem. 97, 188–198. doi: 10.1016/j.soilbio.2016.03.017
Chepsergon, J., and Moleleki, L. N. (2023). Rhizosphere bacterial interactions and impact on plant health. Curr. Opin. Microbiol. 73:102297. doi: 10.1016/j.mib.2023.102297
Cordero, I., Snell, H., and Bardgett, R. D. (2019). High throughput method for measuring urease activity in soil. Soil Biol. Biochem. 134, 72–77. doi: 10.1016/j.soilbio.2019.03.014
Fox, J. E., Gulledge, J., Engelhaupt, E., Burow, M. E., and McLachlan, J. A. (2007). Pesticides reduce symbiotic efficiency of nitrogen-fixing rhizobia and host plants. Proc. Natl. Acad. Sci. 104, 10282–10287. doi: 10.1073/pnas.0611710104
Gu, H., Yan, K., You, Q., Chen, Y., Pan, Y., Wang, H., et al. (2021). Soil indigenous microorganisms weaken the synergy of Massilia sp. WF1 and Phanerochaete chrysosporium in phenanthrene biodegradation. Sci. Total Environ. 781:146655. doi: 10.1016/j.scitotenv.2021.146655
Hamze, R., and Ruiu, L. (2022). Brevibacillus laterosporus as a natural biological control agent of soil-dwelling nematodes. Agronomy 12:2686. doi: 10.3390/agronomy12112686
Huang, W., Liu, J., Zhou, G., Zhang, D., and Deng, Q. (2011). Effects of precipitation on soil acid phosphatase activity in three successional forests in southern China. Biogeosciences 8, 1901–1910. doi: 10.5194/bg-8-1901-2011
Javed, K., Javed, H., and Qiu, D. (2020). Biocontrol potential of purified elicitor protein PeBL1 extracted from Brevibacillus laterosporus strain A60 and its capacity in the induction of defense process against cucumber aphid (Myzus persicae) in cucumber (Cucumis sativus). Biology 9:179. doi: 10.3390/biology9070179
Jones, F. P., Clark, I. M., King, R., Shaw, L. J., Woodward, M. J., and Hirsch, P. R. (2016). Novel European free-living, non-diazotrophic Bradyrhizobium isolates from contrasting soils that lack nodulation and nitrogen fixation genes–a genome comparison. Sci. Rep. 6:25858. doi: 10.1038/srep25858
Joshi, H., Shourie, A., and Singh, A. (2020). “Cyanobacteria as a source of biofertilizers for sustainable agriculture”, in eds. P. K. Singh, A. Kumar, V. K. Singh, and A. K. Shrivastava Adv. Cyanobacterial Biol. (Academic Press), 385–396. doi: 10.1016/B978-0-12-819311-2.00025-5
Kalam, S., Basu, A., Ahmad, I., Sayyed, R., El-Enshasy, H. A., Dailin, D. J., et al. (2020). Recent understanding of soil acidobacteria and their ecological significance: a critical review. Front. Microbiol. 11:580024. doi: 10.3389/fmicb.2020.580024
Koohafkan, P., Altieri, M. A., and Gimenez, E. H. (2012). Green agriculture: foundations for biodiverse, resilient and productive agricultural systems. Int. J. Agric. Sustain. 10, 61–75. doi: 10.1080/14735903.2011.610206
Kruczyńska, A., Kuźniar, A., Podlewski, J., Słomczewski, A., Grządziel, J., Marzec-Grządziel, A., et al. (2023). Bacteroidota structure in the face of varying agricultural practices as an important indicator of soil quality–a culture independent approach. Agric. Ecosyst. Environ. 342:108252. doi: 10.1016/j.agee.2022.108252
Kumar, N., Kumar, A., Marwein, B. M., Verma, D. K., Jayabalan, I., Kumar, A., et al. (2021). Agricultural activities causing water pollution and its mitigation—a review. Int. J. Modern Agric. 10, 590–609.
Larsbrink, J., and McKee, L. S. (2020). Bacteroidetes bacteria in the soil: glycan acquisition, enzyme secretion, and gliding motility. Adv. Appl. Microbiol. 110, 63–98. doi: 10.1016/bs.aambs.2019.11.001
Lee, S.-M., Kong, H. G., Song, G. C., and Ryu, C.-M. (2021). Disruption of Firmicutes and Actinobacteria abundance in tomato rhizosphere causes the incidence of bacterial wilt disease. ISME J. 15, 330–347. doi: 10.1038/s41396-020-00785-x
Li, Y., Fan, Z., Jiang, G., and Quan, Z. (2021). Addressing the differences in farmers’ willingness and behavior regarding developing green agriculture—a case study in Xichuan County, China. Land 10:316. doi: 10.3390/land10030316
Limanska, N., Ivanytsia, T., Basiul, O., Krylova, K., Biscola, V., Chobert, J.-M., et al. (2013). Effect of Lactobacillus plantarum on germination and growth of tomato seedlings. Acta Physiol. Plant. 35, 1587–1595. doi: 10.1007/s11738-012-1200-y
Lou, J., Gu, H., Wang, H., An, Q., and Xu, J. (2016). Complete genome sequence of Massilia sp. WG5, an efficient phenanthrene-degrading bacterium from soil. J. Biotechnol. 218, 49–50. doi: 10.1016/j.jbiotec.2015.11.026
Luo, L., Zhao, C., Wang, E., Raza, A., and Yin, C. (2022). Bacillus amyloliquefaciens as an excellent agent for biofertilizer and biocontrol in agriculture: An overview for its mechanisms. Microbiol. Res. 259:127016. doi: 10.1016/j.micres.2022.127016
Ma, J., Morel, J.-B., Riemann, M., and Nick, P. (2022). Jasmonic acid contributes to rice resistance against Magnaporthe oryzae. BMC Plant Biol. 22:601. doi: 10.1186/s12870-022-03948-4
Nath, R., Sharma, G., and Barooah, M. (2012). Efficiency of tricalcium phosphate solubilization by two different endophytic Penicillium sp. isolated from tea (Camellia sinensis L.). Eur. J. Exp. Biol. 2, 1354–1358.
Pantigoso, H. A., Manter, D. K., Fonte, S. J., and Vivanco, J. M. (2023). Root exudate-derived compounds stimulate the phosphorus solubilizing ability of bacteria. Sci. Rep. 13:4050. doi: 10.1038/s41598-023-30915-2
Purwani, J., Pratiwi, E., and Sipahutar, I., (2021). The effect of different species of cyanobacteria on the rice yield and nitrogen use efficiency under different levels of nitrogen fertilizer on alluvial West Java. IOP conference series: Earth and environmental science. IOP Publishing.
Radhakrishnan, R., Shim, K.-B., Lee, B.-W., Hwang, C.-D., Pae, S.-B., Park, C.-H., et al. (2013). IAA-producing Penicillium sp. NICS01 triggers plant growth and suppresses fusarium sp.-induced oxidative stress in sesame (Sesamum indicum L.). J. Microbiol. Biotechnol. 23, 856–863. doi: 10.4014/jmb.1209.09045
Răut, I., Călin, M., Capră, L., Gurban, A.-M., Doni, M., Radu, N., et al. (2021). Cladosporium sp. isolate as fungal plant growth promoting agent. Agronomy 11:392. doi: 10.3390/agronomy11020392
Ruiu, L. (2020). Plant-growth-promoting bacteria (PGPB) against insects and other agricultural pests. Agronomy 10:861. doi: 10.3390/agronomy10060861
Sang, X., You, Z., Zhou, Y., Zou, L., Shi, L., and Hu, L. (2021). Application effects and application technology of hypersensitive protein complex enzyme on wheat. Shanghai Agric. Sci. Technol. 4, 106–107.
Shao, W., Yao, S., Zhou, Y., Zou, L., and Shi, L. (2022). Brief report on the effects of applying hypersensitive protein complex enzyme to rice seedbeds. Shanghai Agricultural Science and Technology 5, 112–114.
Si, T., Lu, J., Cao, Y., Tang, Z., Ci, D., Yu, X., et al. (2024). Physiological, transcriptional and metabolomic evidence for arbuscular mycorrhizal fungi and Lactobacillus plantarum in peanut resistance to salinity stress. J. Agron. Crop Sci. 210:e12672. doi: 10.1111/jac.12672
Solomon, W., Janda, T., and Molnár, Z. (2023). Unveiling the significance of rhizosphere: implications for plant growth, stress response, and sustainable agriculture. Plant Physiol. Biochem. 206:108290. doi: 10.1016/j.plaphy.2023.108290
Tan, L., Gu, S., Li, S., Ren, Z., Deng, Y., Liu, Z., et al. (2019). Responses of microbial communities and interaction networks to different management practices in tea plantation soils. Sustain. For. 11:4428. doi: 10.3390/su11164428
Toghueo, R. M. K., and Boyom, F. F. (2020). Endophytic Penicillium species and their agricultural, biotechnological, and pharmaceutical applications. 3 Boitech 10:107. doi: 10.1007/s13205-020-2081-1
Tripathi, A. D., Mishra, R., Maurya, K. K., Singh, R. B., and Wilson, D. W. (2019). “Estimates for world population and global food availability for global health” in The role of functional food security in global health. eds. R. B. Singh, R. R. Watson, and T. Takahashi (Amsterdam: Elsevier), 3–24.
Wakelin, S., Anstis, S., Warren, R., and Ryder, M. (2006). The role of pathogen suppression on the growth promotion of wheat by Penicillium radicum. Australas. Plant Pathol. 35, 253–258. doi: 10.1071/AP06008
Wang, J., Song, L., Gong, X., Xu, J., and Li, M. (2020). Functions of jasmonic acid in plant regulation and response to abiotic stress. Int. J. Mol. Sci. 21:1446. doi: 10.3390/ijms21041446
Wang, X., Zhang, J., Wang, X., An, J., You, C., Zhou, B., et al. (2022). The growth-promoting mechanism of Brevibacillus laterosporus AMCC100017 on apple rootstock Malus robusta. Hortic. Plant J. 8, 22–34. doi: 10.1016/j.hpj.2021.11.005
Xu, A., Liu, C., Zhao, S., Song, Z., and Sun, H. (2023). Dynamic distribution of Massilia spp. in sewage, substrate, plant rhizosphere/phyllosphere and air of constructed wetland ecosystem. Front. Microbiol. 14:1211649. doi: 10.3389/fmicb.2023.1211649
You, Z., Hu, L., Zhou, Y., Zou, L., and Shi, L. (2021). Application effect and application technology of hypersensitive protein complex enzyme on tea trees. Shanghai Agricultural Science and Technology 5, 98–100.
Keywords: rhizosphere microorganisms, rice, biological agents, yield, metabolome
Citation: Zhou H, Yu K, Nie L, Liu L, Zhou J, Wu K, Ye H and Wu Z (2025) Effects of biological agents on rhizosphere microecological environment and nutrient availability for rice. Front. Microbiol. 15:1447527. doi: 10.3389/fmicb.2024.1447527
Received: 11 June 2024; Accepted: 29 August 2024;
Published: 08 January 2025.
Edited by:
Puneet Singh Chauhan, National Botanical Research Institute (CSIR), IndiaReviewed by:
Sudipta Sankar Bora, Assam Agricultural University, IndiaCopyright © 2025 Zhou, Yu, Nie, Liu, Zhou, Wu, Ye and Wu. This is an open-access article distributed under the terms of the Creative Commons Attribution License (CC BY). The use, distribution or reproduction in other forums is permitted, provided the original author(s) and the copyright owner(s) are credited and that the original publication in this journal is cited, in accordance with accepted academic practice. No use, distribution or reproduction is permitted which does not comply with these terms.
*Correspondence: Zhaohui Wu, d3poQGhocnJjLmFjLmNu
†These authors have contributed equally to this work
Disclaimer: All claims expressed in this article are solely those of the authors and do not necessarily represent those of their affiliated organizations, or those of the publisher, the editors and the reviewers. Any product that may be evaluated in this article or claim that may be made by its manufacturer is not guaranteed or endorsed by the publisher.
Research integrity at Frontiers
Learn more about the work of our research integrity team to safeguard the quality of each article we publish.