- 1National Council of Scientific and Technological Research (CONICET)–CCT (Salta-Jujuy), Salta, Argentina
- 2Pneumonology Department, Niño Jesus Children Hospital, SIPROSA, Tucuman, Argentina
- 3Laboratory of Immunology, Faculty of Biochemistry, Chemistry and Pharmacy, National University of Tucuman, Tucuman, Argentina
- 4Laboratory of Immunobiotechnology, Reference Centre for Lactobacilli (CERELA-CONICET), Tucuman, Argentina
- 5Institute of Maternity and Gynecology “Nuestra Señora de las Mercedes”, SIPROSA, Tucuman, Argentina
- 6Rehabilitation Department of the Integrated Health Program of the Ministry of Health of the Tucuman Province, Tucuman, Argentina
- 7Hospital Centro de Salud “Zenon Santillan”, SIPROSA, Tucuman, Argentina
- 8Food and Feed Immunology Group, Laboratory of Animal Food Function, Graduate School of Agricultural Science, Tohoku University, Sendai, Japan
- 9Livestock Immunology Unit, International Education and Research Center for Food and Agricultural Immunology (CFAI), Graduate School of Agricultural Science, Tohoku University, Sendai, Japan
This study aimed to investigate the effects of the cell-free supernatant of Lactiplantibacillus plantarum ATCC® 10241TM on the biofilm-forming capacity of Pseudomonas aeruginosa strains isolated from cystic fibrosis (CF) patients. In addition, the study evaluated the in vivo potential of the cell-free supernatant to modulate inflammation and reduce lung damage in mice infected with P. aeruginosa strains or co-challenged with P. aeruginosa and the Streptococcus milleri group (SMG). The results showed that CF-derived P. aeruginosa strains can infect the respiratory tract of adult mice, inducing local inflammation and lung damage. The severity of these infections was exacerbated when P. aeruginosa was co-administered with SMG. Notably, nebulization with the cell-free supernatant of L. plantarum produced beneficial effects, reducing respiratory infection severity and inflammatory responses induced by P. aeruginosa, both alone or in combination with SMG. Reduced bacterial loads and lung damage were observed in supernatant-treated mice compared to controls. Although further mechanistic studies are necessary, the results show that the cell-free supernatant of L. plantarum ATCC® 10241TM is an interesting adjuvant alternative to treat P. aeruginosa respiratory infections and superinfections in CF patients.
Introduction
Pseudomonas aeruginosa is an opportunistic pathogen that can cause chronic airway infections in patients with cystic fibrosis (CF; Sala and Jain, 2021). The infections produced by P. aeruginosa in CF patients have been marked with the highest priority for surveillance and epidemiological research (Horcajada et al., 2019; Reynolds and Kollef, 2021; Souza et al., 2021), considering that this bacterium is a global threat because of its ability to become increasingly resistant to all available antibiotics (Tümmler, 2019; Laborda et al., 2022). P. aeruginosa infection can occur as early as the 1st year of life, is usually non-mucoid, and evolves over time, accumulating a series of adaptations to the CF lung. These adaptations include a mucoid phenotype, antibiotic resistance, and a loss of acute virulence factors, among others, and contribute to airway persistence and chronic pathogenicity (Gibson et al., 2003). The biofilm mode of life is considered important for the persistence of P. aeruginosa during long-term colonization of CF airways (Rossi et al., 2020). Biofilm synthesis and virulence factors produced by P. aeruginosa are regulated by chemical signals, including acyl-homoserine lactones (AHL) secreted by bacteria. When bacteria reach a threshold number, a concentration of signal molecules is reached, triggering the expression of the genes involved in synthesizing biofilm and virulence factors (Reading and Sperandio, 2006). Then, biofilm formation is a key event for establishing a chronic infection in the CF lung, promoting an accelerated decline of pulmonary function.
It is considered that respiratory infections in CF patients are usually polymicrobial. Historically, streptococci isolated from respiratory samples of CF patients were considered contaminants from the sampling process and were not recognized as clinically relevant. However, these bacteria are increasingly recognized as members of the CF lung microbiome and are associated with enhanced inflammation and lung damage (Sibley et al., 2008; Scott and O'Toole, 2019). Notably, the presence of the Streptococcus milleri group (SMG; also known as the anginosus group streptococci) can enhance the exacerbation and lung damage in CF patients infected with P. aeruginosa (Duan et al., 2003; Scott and O'Toole, 2019). It was reported that streptococci influence the growth and virulence of P. aeruginosa, potentiating its ability to damage lung tissue (Sibley et al., 2008; Scott and O'Toole, 2019). SGM can produce enzymes that can damage tissue and form abscesses, such as hyaluronidase, DNase, RNase, gelatinase, and collagenase, that may promote infections with other bacteria such as P. aeruginosa (Sibley et al., 2008). Given the well-documented nature of polymicrobial infections in the context of CF, strategies to prevent or treat infections with P. aeruginosa should also consider other microorganisms, such as SMG.
An attractive therapy to prevent P. aeruginosa chronic infection is bacteriotherapy, which uses beneficial harmless bacteria from the commensal microbiota, including lactobacilli. Several studies have reported the ability of lactobacilli strains to reduce P. aeruginosa growth, biofilm formation, and virulence. It was shown that culture supernatants of two human probiotic bacteria, Lacticaseibacillus casei CRL431 and Lactobacillus acidophilus CRL730, can attenuate P. aeruginosa biofilm and virulence by inhibition of the quorum sensing (Díaz et al., 2020). Similar effects were described for other lactobacilli strains (Shokri et al., 2018; Azami et al., 2022). Notably, most of the studies evaluating the effect of lactobacilli were performed with P. aeruginosa strains isolated from chronic wounds, while the interaction of lactobacilli and pathogenic bacteria isolated from CF patients was less explored. Recent studies demonstrated that some lactobacilli could inhibit the planktonic growth of P. aeruginosa, while others can reduce the pre-formed biofilm in an artificial sputum medium that mimics the CF lung microenvironment (Batoni et al., 2023; Pompilio et al., 2024). These previous studies indicate that lactobacilli and their metabolites are promising candidates as adjuvants in the antimicrobial therapy of P. aeruginosa infections in CF patients.
In a previous study, we demonstrated that the culture supernatant and the acid filtrate of Lactiplantibacillus plantarum ATCC® 10241TM grown in De Man–Rogosa–Sharpe (MRS) medium can inhibit the multiplication and viability of P. aeruginosa in vitro, reducing the synthesis of AHL, virulence factors, and the production of biofilm (Valdéz et al., 2005). Furthermore, the in vivo application of L. plantarum to burns infected with P. aeruginosa in a murine model reduced its colonization, regulating the inflammatory response and promoting tissue repair (Valdéz et al., 2005). When the culture supernatant of lactobacilli was analyzed, we found several compounds with biological activity, such as organic acids with low molecular weight (lactic, butyric, acetic, and succinic acids), H2O2, alcohols, benzoic acid, 5-methyl hydantoin, 2,5-mevalonolactone, and isobutyl piperazinedione. Many of them have proved to have growth inhibitory activity on Gram-negative bacteria and to synergize with each other in their antimicrobial action (Ramos et al., 2015). Notably, the presence of AI-2, which is considered an inhibitor of AHL, was determined in the L. plantarum supernatant by GC-MS, and its biological activity was confirmed by the Vibrio Harveyi bioassay (Ramos et al., 2015). Based on these observations, we used the supernatants of L. plantarum as clinical treatment by topical applications on infected chronic wounds, including diabetic foot ulcers, burns, and venous ulcers (Peral et al., 2009, 2010; Aybar et al., 2022). This treatment was able to reduce or eliminate P. aeruginosa, as well as diminish the amount of necrotic tissue in the wound area. Interestingly, the treatment promoted wound healing with increased production of TGF-β, IL-8, and IL-8-R (Peral et al., 2009, 2010), indicating the capacity of the culture supernatant to modulate the local immune response.
This study aimed to investigate the effect of the cell-free supernatant of L. plantarum ATCC® 10241TM on the capacity of P. aeruginosa strains isolated from CF patients to form biofilms. In addition, the study evaluated in vivo the ability of the cell-free supernatant to modulate respiratory inflammation and reduce lung damage in mice infected with the P. aeruginosa strains or challenged with P. aeruginosa and SMG.
Materials and methods
Bacterial strains
Bacterial strains were isolated from sputum samples of CF patients using standard clinical microbiological methods (Delgado et al., 2018). P. aeruginosa and SMG (S. anginosus) isolated from CF patients were used in this study. Analysis of the 16s RNA nucleotide sequence of the isolated strains showed that they were not genetically related (Delgado et al., 2018). Strains of antibiotic-sensitive P. aeruginosa (strain PaS), multi-resistant P. aeruginosa (strain PaR), and SMG were used in the subsequent experiments. The PaS strain is sensitive to ceftazidime, ciprofloxacin, gentamicin, amikacin, imipenem, meropenem, piperacillin/tazobactam, and colistine, while PaR is resistant to all the mentioned antibiotics except for colistine (Delgado et al., 2018).
In addition, the P. aeruginosa 101 strain, a qsc mutant that was a generous gift from E.P. Greenberg and K. Lee from the University of Iowa, USA, was used (Whiteley et al., 1999). The qsc mutant has random lacZ transcriptional fusions in the chromosome of a lasI–rhlI double mutant and was used to detect the production of AHL by wild-type P. aeruginosa strains. The qsc mutant strain does not produce either the autoinducer N-3-oxododecanoyl homoserine lactone (3O-C12-HSL) or the butanoyl-L-homoserine lactone (C4-HSL) but responds to the presence of these compounds by increasing the expression of β-galactosidase. P. aeruginosa 101, referred to here as Paqsc, was selectively grown in an LB medium containing gentamicin 100 mg/L (Whiteley et al., 1999). Colonies of the Paqsc strain were then cultured in LB broth without antibiotics.
Bacterial supernatants
P. aeruginosa strains were cultured in LB broth, while SMG were cultured in BHI medium. After overnight growth at 37°C, these cultures were used to obtain the supernatants of each strain by centrifugation for 30 min at 30,000 g at 10°C and the filtration of the supernatant through a millipore filter of 0.22 μm in diameter. These supernatants were kept in a refrigerator at 4°C until use. L. plantarum ATCC® 10241TM strain was cultured in MRS broth (Britania, Argentina) at 37°C. The supernatant from lactobacilli culture was obtained as described previously (Peral et al., 2010) and stored at 4°C.
Biofilm formation
A static biofilm assay was performed as described elsewhere (O'Toole and Kolter, 1998). A total of 100 μl/well (108 CFU) of the overnight culture of the different P. aeruginosa strains (PaS, PaR, or Paqsc) were placed in 96 well plates. Then, 100 μl of the supernatant from P. aeruginosa (PaS, PaR, or Paqsc), SMG, or 100 μl of cultures of the same microorganisms were added. To determine the effect of the supernatant of L. plantarum on biofilm formation and viability of P. aeruginosa strains (PaS, PaR, or Paqsc) we followed the procedure described: a total of 100 μl of P. aeruginosa strains cultures were added to each well, and then 50 μl of the supernatant from the lactic acid bacterium grown in BHI, LB, or MRS media. As a control, 100 μl of the culture media for each bacteria was used. The plates were incubated for 6 h at 37°C and then stained with crystal violet. The cell-attached dye was solubilized with ethanol at 95% v/v, and the absorbance was then measured at 540 nm. All assays were performed in triplicate.
Bacterial viability
The viability of bacteria in biofilms alone and the planktonic plus biofilm states was performed according to the assay described by Cerca et al. (2005). A total of 100 μl of overnight culture of the different P. aeruginosa strains (PaS, PaR, or Paqsc; 108 CFU) were placed in 96-well plates. Then, 50 μl of the supernatants of L. plantarum, SMG, or mixed supernatants were added. Wells with 100 μl of P. aeruginosa strain cultures in 100 μl of BHI medium were used as controls.
To determine the viability of bacteria within the biofilm, the wells were emptied and washed four times with PBS to remove planktonic cells. Then, they were refilled with 200 μl of PBS. Then, 50 μl of XTT salt solution (1 mg/ml in PBS; Promega, Madison, WI, USA) was added to each well and incubated at 37°C in the dark for 90 min. Bacterial dehydrogenase activity reduces XTT tetrazolium salt to XTT formazan, resulting in a colorimetric change that can be measured spectrophotometrically at 490 nm, which correlates with cell viability (Valdéz et al., 2005).
Production of AHL
To investigate the production of AHL by P. aeruginosa strains (PaS, PaR, or Paqsc), bacteria were grown to an OD600 of 0.7, centrifuged, and filtered. In a hemolysis tube, 500 μl of each filtrate was added to 500 μl of the Paqsc strain culture. Following incubation at 37°C for 1 h, β-galactosidase activity was measured by the Miller reaction, and the results were expressed in β-galactosidase units (Miller, 1992).
Animal model of lung infection
A model of lung infection with P. aeruginosa using BALB/c mice was developed following previous studies (Delgado et al., 2018; Brao et al., 2020). This model uses an intranasal route of infection and does not embed bacteria in foreign substances to prevent clearance, resembling a natural infection and allowing the study of P. aeruginosa in a mouse with CF-like lung disease for up to 12 days post-infection. The Institutional Animal Care and Use Committee at the University of Maryland, Baltimore, MD, approved the experiments, and it was endorsed by the Bioethics Committee of the Faculty of Biochemistry, Chemistry and Pharmacy, National University of Tucuman (Tucuman, Argentina).
Adult BALB/c mice (6 weeks) from the random-bred colony kept in the Department of Immunology at the Institute of Microbiology of the Tucuman University (Tucuman, Argentina) were randomized in experimental groups of six mice each. Mice were housed with food and water ad libitum and a 12 h light/dark cycle. Animals were anesthetized by intraperitoneal injection of ketamine hydrochloride (75 mg/kg) and medetomidine hydrochloride (1 mg/kg) to be infected or sacrificed.
To produce lung infection, mice were instilled intranasally with 50 μl of P. aeruginosa strains (PaS, PaR, or Paqsc) suspensions (106 CFU) for two consecutive days. In this schedule, mice infected with PaS, PaR, or Paqsc strains displayed signs of disease in the first 3 days of infection, including ruffled fur and hunched posture. After 6, 8, or 10 days, mice were anesthetized and sacrificed to obtain samples of bronchoalveolar lavages (BAL) and lungs for quantitative bacteriology (Figure 1A).
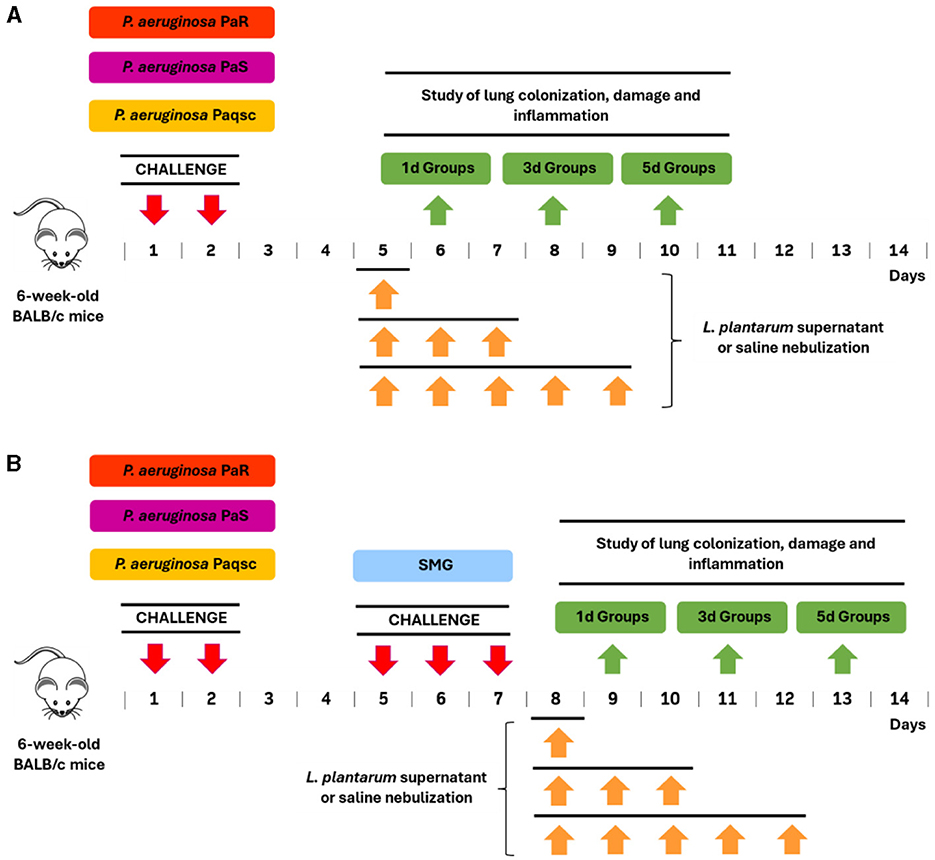
Figure 1. Experimental protocols were used to evaluate the effect of L. plantarum supernatant on respiratory infections produced by CF-derived pathogens. (A) The effects of L. plantarum supernatant on respiratory infections produced by different P. aeruginosa strains (Paqsc, PaS, and PaR) were evaluated 5 days after the challenges with the pathogens and for 1, 3, or 5 days through nebulization. (B) The effect of L. plantarum supernatant on respiratory superinfections produced by different P. aeruginosa strains (Paqsc, PaS, and PaR) and the Streptococcus milleri group (SMG) was evaluated 1 day after the challenge with SMG and for 1, 3, or 5 days through nebulization. Mice nebulized with saline solution were used as controls.
For bacterial co-infection experiments, mice were intranasally challenged with different P. aeruginosa strains (PaS, PaR, or Paqsc) for 2 consecutive days as described above and, 5 days later, infected with the 25 μl of SMG (2.5 × 104 CFU) for 3 consecutive days (Figure 1B). Control groups were mice instilled only with P. aeruginosa strains or saline. Moreover, 1, 3, or 5 days after the last SMG challenge (days 9, 11, and 13), animals were anesthetized and sacrificed to obtain samples of BAL and lungs for quantitative bacteriology and histopathology lung studies (Figure 1B).
L. plantarum treatment
Mice infected with the different P. aeruginosa strains (PaS, PaR, or Paqsc) were nebulized with L. plantarum culture supernatant from day 5 and for 1, 3, or 5 days (Figure 1A). Mice infected with P. aeruginosa strains and coinfected with SMG were nebulized with L. plantarum culture supernatant from day 8 and for 1, 3, or 5 days (Figure 1B). Control groups were mice instilled with saline during P. aeruginosa or SMG challenges and nebulized with L. plantarum supernatant. Nebulizations were performed for 5 min per day. The nebulization with L. plantarum culture supernatant and saline was conducted by a compressor/nebulizer (OMROM NE-C801) connected by a tube to a hermetically sealed plastic container. The mice to be nebulized were placed into this container.
Quantitative lung bacteriology
To determine bacterial cell counts in the respiratory tract of infected mice, the whole lungs of animals were aseptically excised, weighed, and homogenized in 5 ml of sterile 0.9% saline. Serial dilutions were immediately plated on Levin, BCSA agar, and Sodium Azide agar (Biomeriex, Argentina), and the numbers of viable bacteria were determined following overnight incubation at 37°C. The different culture mediums were used because they are selective for the pathogens studied (Sibley et al., 2008).
Studies in BAL
BAL samples were collected as described before (Tonetti et al., 2023). Briefly, 0.5 ml of saline containing 10 U/ml of heparin was injected and aspirated thrice through the tracheostomy tube. The total volume of BAL fluid was pooled. Then, BAL cells were counted in a hemocytometer, and the differential cell types were determined by smears stained with Giemsa. Hemoglobin was determined by spectrophotometry. The determination of elastase activity was carried out following the procedures of Gambello and Iglewski (1991). Briefly, 500 μl of elastin-congo red solution (20 mg of congo red elastin in 1 ml of 10 mM Na2HPO4, pH = 7) was used as a substrate. Samples (500 μl of BAL) were incubated overnight with shaking for 24 h at 37°C. The pellet was removed by centrifugation, and the absorbance of the supernatant was read on a spectrophotometer at OD 495 nm.
Histopathology
After the sacrifice of mice, the lungs were instilled with 1 ml of 10% formaldehyde using a tracheal catheter. The whole lung was removed and processed for routine histological hematoxylin-eosin staining. The degree of edema, necrosis, hemorrhage, congestion, alveolar inflammatory infiltrate, and interstitial inflammatory infiltrate was scored on scales from 0 to +++: absence (0), mild focal (+), moderate to severe focal (++), and severe throughout the lung (+++). Histopathology evaluation was performed blindly.
Statistical analysis
All assays were performed at least in triplicate, and the results were expressed as mean values with standard deviations. Since the lungs of infected mice were used for histology, quantitative bacteriology, and the evaluation of parameters in BAL samples, and it is not possible to perform all these determinations in the same lung, different experiments were performed in each case. The number of repetitions is incorporated into each figure. Statistical analyses were performed using GraphPad Prism 8 software (La Jolla, CA, USA), and a t-test (for pairwise comparisons of the means) was used to test for differences between the groups. Differences were considered significant at a p-value of <0.05.
Results
Effect of L. plantarum supernatant on P. aeruginosa biofilm formation in vitro
The ability of three P. aeruginosa strains (PaR, PaS, and Paqsc) to form biofilms and the effect of L. plantarum supernatant were evaluated in different culture mediums (Table 1, Figure 2), taking into consideration that the metabolites produced by the bacteria that are involved in the antimicrobial activities depend on the medium that they grow (Valdéz et al., 2005; Ramos et al., 2015). The cultures of P. aeruginosa strains in the LB and BHI mediums showed significantly higher biofilm production than in the MRS medium. It was observed that PaR and PaS did not have significant differences in biofilm formation between them, and both showed greater biofilm production capacity than the Paqcs strain (Table 1, Figure 2). These differences were observed in the three mediums evaluated. The addition of culture supernatants from PaS and Paqsc strains to P. aeruginosa PaR culture in LB medium reduced the production of biofilm, while supernatants from PaR and Paqsc strains to P. aeruginosa PaS culture did not affect the biofilm production (Table 1, Figure 2). It was also observed a tendency for higher levels of biofilm production in the Paqsc cultures treated with the supernatants of the PaR and PaS strains. However, the differences were not statistically significant.
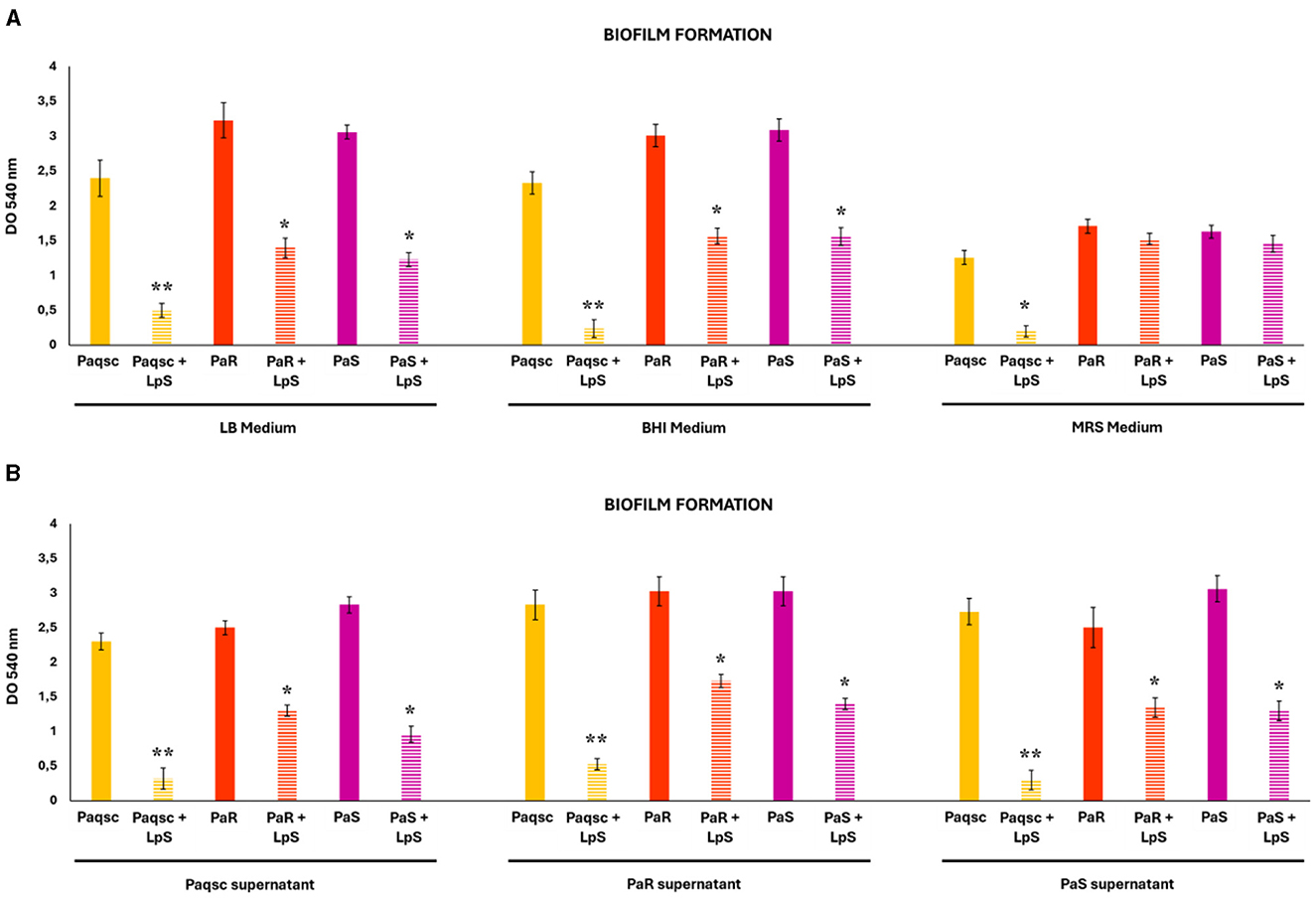
Figure 2. Effect of L. plantarum supernatants on P. aeruginosa biofilms. (A) Different P. aeruginosa strains (PaR, PaS, and Paqsc) were grown in LB, BHI, and MRS mediums, and the absorbance values (OD540) obtained from the cultures using the crystal violet method were used to evaluate biofilms. (B) The effects of L. plantarum and P. aeruginosa strain-conditioned mediums on biofilm formation are also shown. Data represent the mean ± SD of triplicated experiments (n = 3 per experiment). Asterisks show statistical significance between L. plantarum treated and the respective control groups without lactobacilli treatment *p < 0.05 and **p < 0.01.
Interestingly, when cultures of P. aeruginosa strains were performed in the presence of L. plantarum supernatant, a significant inhibition of biofilm formation was observed for the three strains in both LB and BHI mediums (Figure 2). The reduction of biofilm formation in the MRS medium by L. plantarum supernatant was only detected for the Paqsc strain. The cell-free supernatant of L. plantarum was also capable of reducing biofilm formation of the three P. aeruginosa strains in the experiments in which the bacteria were cultured in the presence of the supernatants of the PaR, PaS, and Paqsc strains (Figure 2). The viability of P. aeruginosa strains within the biofilms was also evaluated (Table 2). The three P. aeruginosa strains showed higher viability in LB and BHI than in the MRS medium. In addition, it was detected that L. plantarum supernatant significantly reduced the viability of the PaR, PaS, and Paqsc strains within the biofilm produced in the LB medium (Table 2).
We also evaluated AHL production in P. aeruginosa PaR and PaS cultures by measuring β-galactosidase activity as described in materials and methods (Figure 3). The study of the AHL production revealed that the PaS strain (UE = 38.71) had a higher activity than P. aeruginosa PaR (UE = 26.54). The cell-free supernatant of L. plantarum could also reduce the AHL production in both P. aeruginosa PaR and PaS cultures (Figure 3).
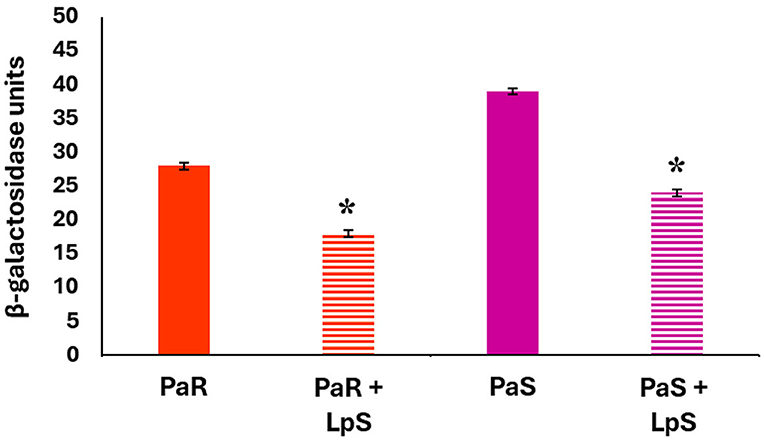
Figure 3. Effect of L. plantarum supernatant on P. aeruginosa AHL production. The effects of L. plantarum supernatant on P. aeruginosa strains (PaR, PaS) acyl-homoserine lactone (AHL) production are shown. AHL production is measured as β-galactosidase units. Data represent the mean ± SD of triplicated experiments (n = 3 per experiment). Asterisks show statistical significance between L. plantarum treated and the respective control groups (PaR and PaS) without lactobacilli treatment, *p < 0.05.
Effect of L. plantarum supernatant on P. aeruginosa respiratory infection in vivo
Considering the ability of L. plantarum supernatant to affect the viability and biofilm production of the three P. aeruginosa strains, we next aimed to evaluate whether this supernatant could influence the outcome of respiratory infections caused by opportunistic pathogens. Then, we first assessed the abilities of PaR, PaS, and Paqsc to colonize the lungs of mice nasally challenged with the bacteria (Figure 4A). It was observed that the three P. aeruginosa strains were able to colonize the lower respiratory tract of mice. However, the lung bacterial cell counts in mice challenged with PaR and PaS were significantly higher than those observed in the group infected with the Paqsc strain. The elastase release in BAL samples was also determined to indirectly measure P. aeruginosa strain virulence. Elastase is under the detection limit in BAL samples of non-infected mice, while the challenges with PaR, PaS, or Paqsc significantly increased the values of this parameter in infected animals. The elastase release in mice challenged with P. aeruginosa Paqsc was significantly lower than observed in the groups infected with PaR or PaS strains (Figure 4B). The results indicate that PaR and PaS would be more virulent than the mutant Paqsc strain that is not able to produce AHL, an important pathogenicity factor. Furthermore, elastase release was higher in the BAL of animals infected with PaR than those challenged with P. aeruginosa PaS (Figure 4B).
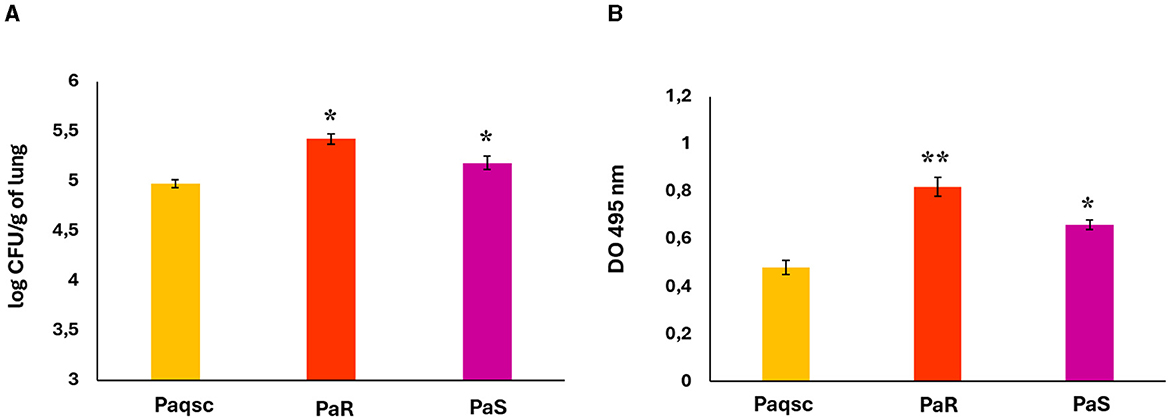
Figure 4. Abilities of P. aeruginosa strains to produce respiratory infections. Mice were nasally challenged with different P. aeruginosa strains (PaR, PaS, and Paqsc) and (A) the bacterial counts in the lungs and (B) the elastase activity in bronchoalveolar lavages (BAL) samples were determined. Data represent the mean ± SD of triplicated experiments (n = 3 per experiment). Asterisks show statistical significance compared to Paqsc, *p < 0.05, **p < 0.01.
The effect of L. plantarum supernatant on P. aeruginosa respiratory infections was evaluated after 1, 3, and 5 days of nebulizations (Figure 5). Lung bacterial cell counts after the nebulizations with L. plantarum supernatant for 1 or 3 days were not different from controls for strains PaR, PaS, and Paqsc (Figure 5A). In contrast, L. plantarum supernatant significantly reduced lung bacterial cell counts after 5 days of nebulizations in the three groups of mice infected with the P. aeruginosa strains (Figure 5A). When bacterial loads in the lower respiratory tract of infected mice were compared, no differences were detected between the strains after 3 days of nebulization with L. plantarum supernatant. In contrast, lung bacterial cell counts in mice infected with P. aeruginosa PaR and treated with L. plantarum supernatant for 5 days were significantly higher than the values detected in animals infected with PaS and Paqsc strains (Figure 5A).
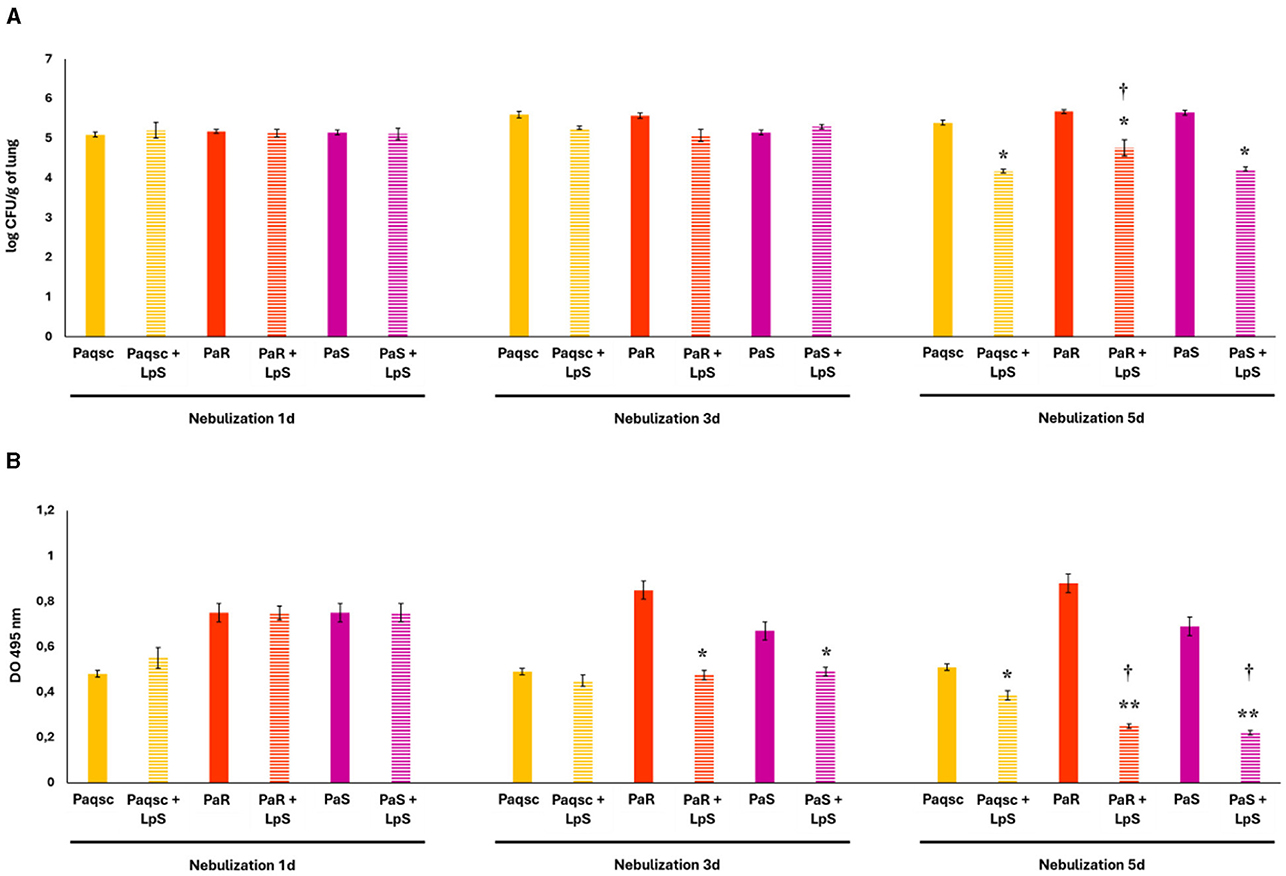
Figure 5. Effect of L. plantarum supernatant on respiratory infections produced by P. aeruginosa strains. The effects of L. plantarum supernatant on respiratory infections produced by different P. aeruginosa strains (Paqsc, PaS, and PaR) are shown. (A) The bacterial counts in the lungs and (B) elastase release in bronchoalveolar lavages (BAL) were determined. Data represent the mean ± SD of triplicated experiments (n = 3 per experiment). Asterisks show statistical significance compared to the respective non-supernatant-treated control, *p < 0.05, **p < 0.01. Symbols show statistical significance compared with the Paqsc group, †p < 0.05.
The effect of L. plantarum supernatant on elastase release in BAL samples was also determined for the three P. aeruginosa strains (Figure 5B). The nebulization of mice infected with PaR and PaS with L. plantarum supernatant significantly reduced the levels of elastase release in BAL after 3 and 5 days, making the effect more remarkable on day 5. The elastase release in BAL samples of mice infected with P. aeruginosa Paqsc and treated with L. plantarum supernatant differed from control only at day 5 (Figure 5B).
We also evaluated the number of leukocytes in BAL samples to measure the inflammatory response (Table 3). The challenge of mice with P. aeruginosa strains significantly increased the number of BAL leukocytes compared to basal levels, the most remarkable effect observed in animals infected with the PaR strain. The treatment with L. plantarum supernatant significantly reduced the levels of BAL leukocytes after 1, 3, and 5 days for the three P. aeruginosa strains. The lowest number of leucocytes in mice infected with PaR, PaS, and Paqsc strains and treated with L. plantarum supernatant were observed at day 5 (Table 3). However, these values did not reach the numbers observed in non-infected animals. When the different types of leukocytes were analyzed in BAL samples, it was observed that in mice infected with P. aeruginosa Paqsc, 90% corresponded to neutrophils, 5% to macrophages, and 5% to lymphocytes. In animals challenged with the PaS strains, the BAL leukocytes were composed of 30% of neutrophils and 70% of macrophages, while in PaR-infected mice, the composition was 5% of neutrophils and 95% of macrophages (Supplementary Figure 1).
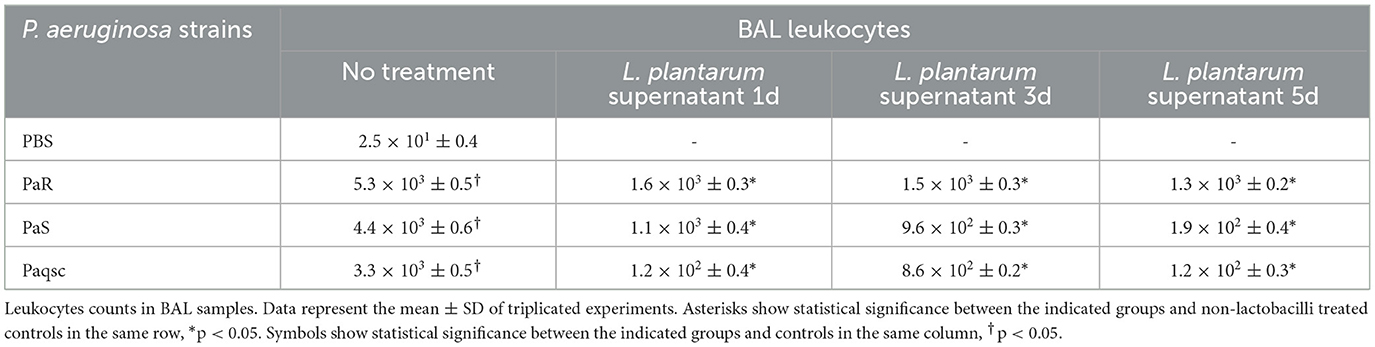
Table 3. Effect of L. plantarum supernatant on respiratory infections produced by P. aeruginosa strains.
Lung histology was also studied to evaluate the tissue damage induced by P. aeruginosa infections (Figure 6 and Table 4). The three P. aeruginosa strains could induce lung tissue injury compared to non-infected controls (Supplementary Figure 2), although with a different degree of severity. The PaR strain induced hemorrhage, edema, congestion, and inflammatory infiltrates both in the alveolar and the interstitial compartments (Figure 6A). Animals challenged with P. aeruginosa PaS showed edema, moderate congestion, hemorrhage, and scant inflammatory infiltrate in the lung interstitial tissue (Figure 6B). The histopathological analysis also showed that the infection produced by the mutant Paqsc strain was much milder when compared to the strains of clinical origin, PaR and PaS (Figure 6 and Table 4). When the effect of L. plantarum supernatant was evaluated, it was determined that after 1 or 3 days of treatments, there were no changes in lung tissue damage compared to the respective controls for any of the P. aeruginosa strains, as shown in the microscopic observations (Figure 6) and the scores (Table 4). However, the nebulization with L. plantarum supernatant for 5 days significantly decreased lung damage in animals infected with any of the three P. aeruginosa strains.
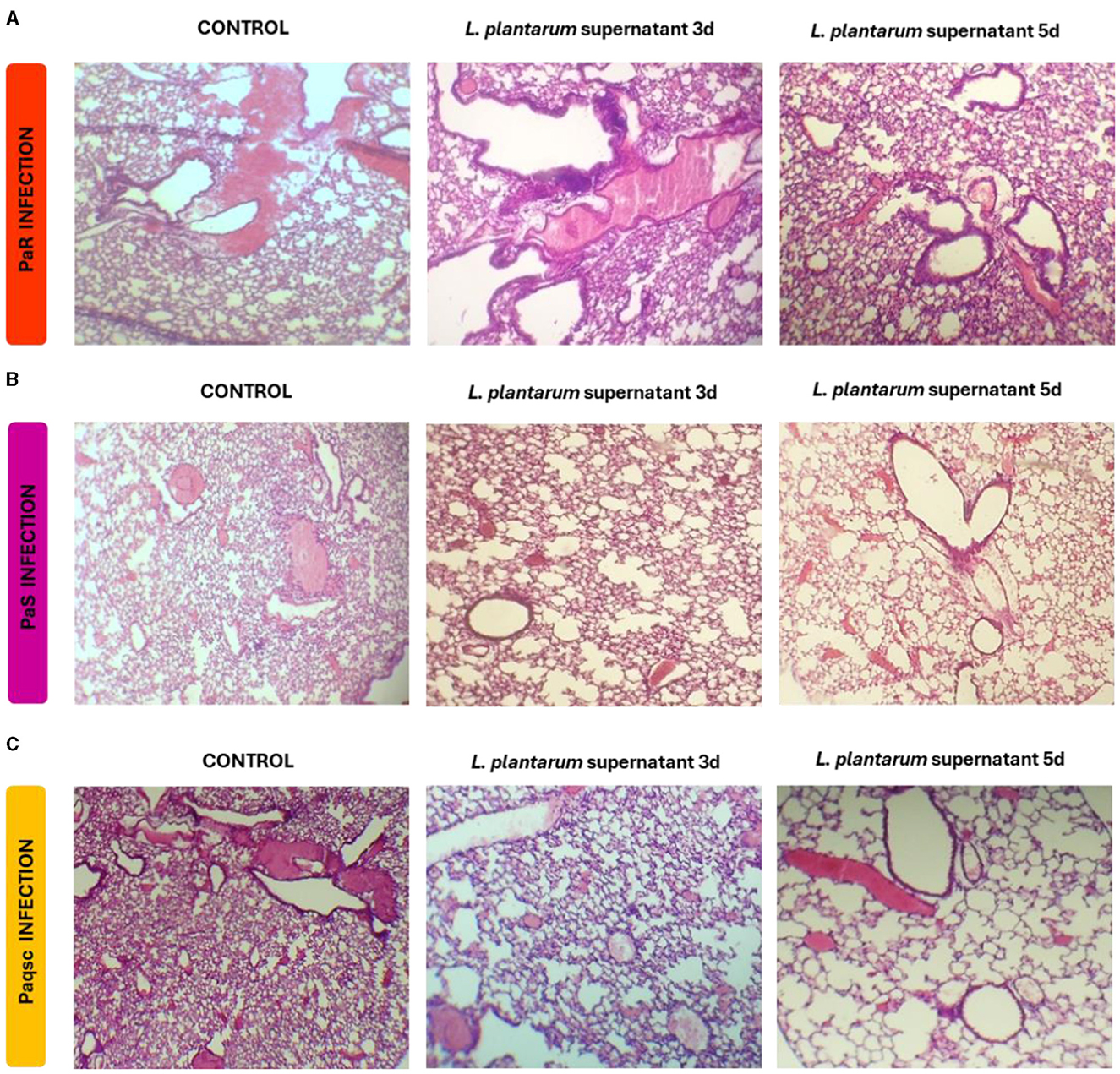
Figure 6. Effect of L. plantarum supernatant on respiratory infections produced by P. aeruginosa strains. The effects of L. plantarum supernatant on respiratory infections produced by different P. aeruginosa strains (Paqsc, PaS, and PaR) are shown. The histology of the lungs was determined. (A–C) Lung histopathological analysis at different points post-infection and treatment with L. plantarum supernatant for PaR, PaS, and Paqsc, respectively. Data were obtained from duplicated experiments (n = 3 per experiment).
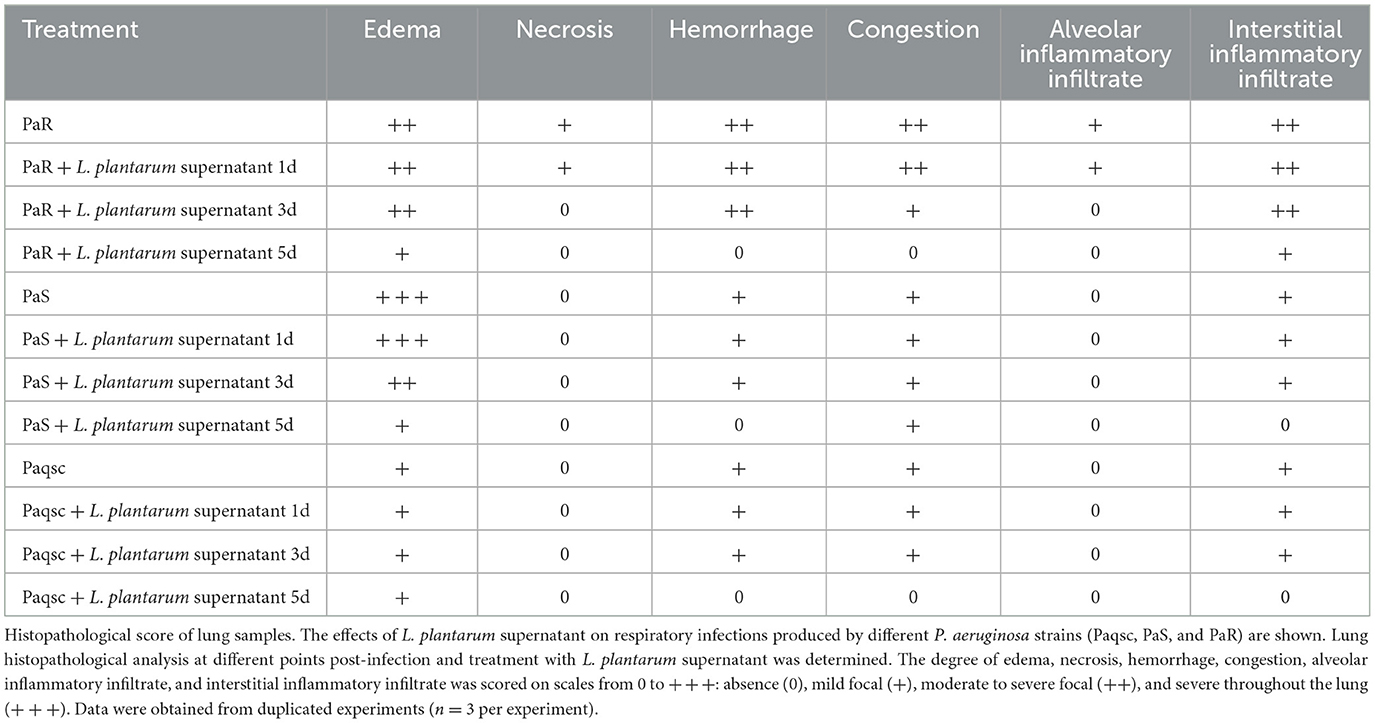
Table 4. Effect of L. plantarum supernatant on respiratory infections produced by P. aeruginosa strains.
Effect of L. plantarum supernatant on P. aeruginosa and SMG biofilm formation in vitro
Subsequently, we aimed to evaluate the interaction of the three P. aeruginosa strains (PaR, PaS, and Paqsc) with SGM and the potential beneficial effect of L. plantarum supernatant on that interaction. The effect of SMG and SMG supernatants in the ability to form biofilms was evaluated in different culture mediums (Table 5). Both SMG and SMG supernatants significantly reduced the capacity of P. aeruginosa strains PaR, PaS, and Paqsc to form biofilms. The cell-free supernatant of L. plantarum could also reduce the biofilm formation of the three P. aeruginosa strains cocultured with SMG or SMG supernatants (Table 5).
The viability of P. aeruginosa strains within the biofilms in coculture with SMG supernatant was also evaluated (Table 6). When the SMG supernatant was added, the three P. aeruginosa strains showed lower viability in biofilms. In addition, it was observed that L. plantarum supernatant significantly reduced the viability of the PaR, PaS, and Paqsc strains within the biofilm produced in LB medium coculture with SMG supernatant (Table 6).
Effect of L. plantarum supernatant on P. aeruginosa and SMG respiratory infection in vivo
Considering the ability of L. plantarum supernatant to affect the viability and biofilm production of the three P. aeruginosa strains in coculture with SMG supernatant, we next aimed to evaluate whether this supernatant could be used to influence the outcome of respiratory infections caused by P. aeruginosa and SMG. Then, we evaluated the abilities of PaR, PaS, and Paqsc to colonize the lungs of mice nasally challenged with the bacteria and infected 5 days later with SMG (Figure 7A). The lung bacterial counts for the three strains in experiments with SMG challenge were slightly higher than that observed when P. aeruginosa was used alone: PaR 5.8 vs. 5.4 log CUF/g of the lung and PaS 5.7 vs. 5.3 log CUF/g of the lung (Figure 7A). However, the lung bacterial cell counts in mice challenged with PaR and PaS were significantly higher than those observed in the group infected with the Paqsc strain (data not shown).
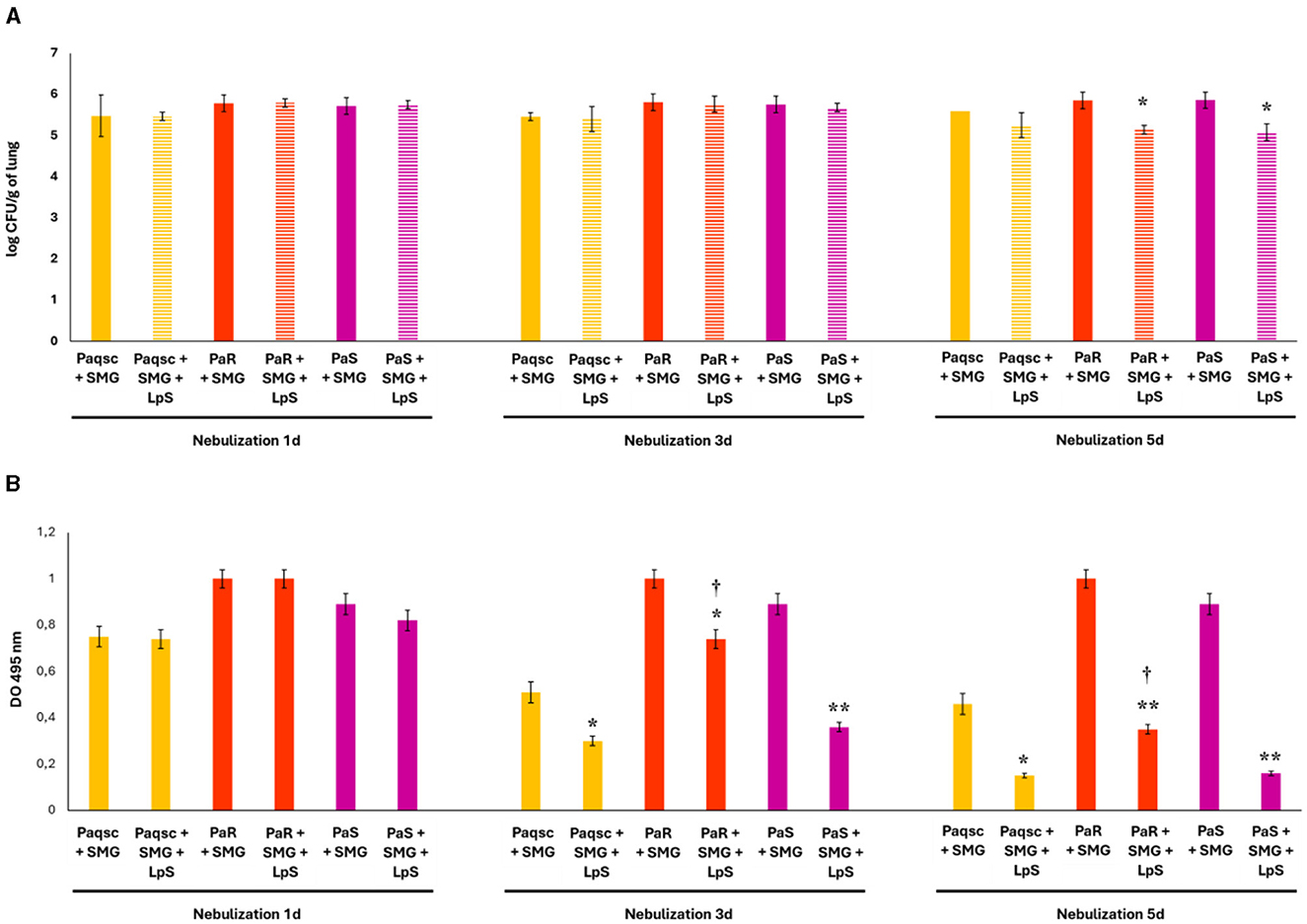
Figure 7. Effect of L. plantarum supernatant on respiratory superinfections produced by P. aeruginosa strains and SMG. The effects of L. plantarum supernatant on respiratory superinfections produced by different P. aeruginosa strains (Paqsc, PaS, and PaR) and SMG are shown. (A) The bacterial counts in the lungs and (B) elastase release in bronchoalveolar lavages (BAL) were determined. Data represent the mean ± SD of triplicated experiments (n = 3 per experiment). Asterisks show statistical significance compared to the respective non-supernatant-treated control, *p < 0.05, **p < 0.01. Symbols show statistical significance compared with the Paqsc group, †p < 0.05.
The effect of L. plantarum supernatant on P. aeruginosa and SMG respiratory infections was evaluated after 1, 3, and 5 days of nebulizations. Lung bacterial cell counts after the nebulizations with L. plantarum supernatant for 1 or 3 days were not different from controls for strains PaR, PaS, and Paqsc (Figure 7A). L. plantarum supernatant significantly reduced lung bacterial cell counts after 5 days of nebulizations in the groups of mice infected with the P. aeruginosa strains PaR and PaS, while no differences were noticed for Paqsc. When bacterial loads in the lower respiratory tract of infected mice were compared, significant differences were detected between the strains after 1 or 3 (Figure 7A) days of nebulizations with L. plantarum supernatant. The counts of PaR and PaS were higher than Paqsc. In contrast, lung bacterial cell counts in mice treated with L. plantarum supernatant for 5 days were not different when PaR, PaS, and Paqsc strains were compared (Figure 7A).
The elastase release in BAL samples was also determined in the infections produced by P. aeruginosa strains and SMG (Figure 7B). The release of elastase in mice infected with the three strains in experiments with SMG challenge was slightly higher than the observed when P. aeruginosa was used alone: PaR 1.12 vs. 0.83, PaS 0.87 vs. 0.65, and Paqsc 0.76 vs. 0.48 (Figure 7B), indicating an increased virulence of P. aeruginosa strains in the presence of SMG. The elastase release in Paqsc-challenged mice was significantly lower than that observed in the groups infected with the PaR or PaS strains, and it was higher in the BAL of animals infected with PaR than in those challenged with P. aeruginosa PaS (Figure 7B).
The effect of L. plantarum supernatant on elastase release in BAL samples was also determined for the three P. aeruginosa strains administered with SMG. The nebulization of mice infected with PaR, PaS, and Paqsc (Figure 7B) with L. plantarum supernatant for 1 day did not induce changes in the elastase levels in BAL samples. However, significantly reduced levels of elastase release in BAL were found after 3 and 5 days of L. plantarum supernatant treatment, with the effect more remarkable on day 5.
The number of leukocytes in BAL samples to measure the inflammatory response was also evaluated in the infections produced with the three P. aeruginosa strains administered with SMG (Table 7). The challenge of mice with P. aeruginosa strains significantly increased the number of BAL leukocytes compared to basal levels, the most remarkable effect was observed in animals infected with PaR strain and SMG. As observed with other parameters, the BAL leucocyte counts in mice infected with the PaR and PaS strains in experiments with SMG challenge were higher than the observed when P. aeruginosa was used alone: PaR 8.3 × 103 vs. 5.3 × 103 and PaS 5.2 × 103 vs. 4.4 × 103. In contrast, no differences were detected in BAL leucocytes in mice infected with the Paqsc strain with (3.5 × 103) or without (3.3 × 103) SMG.
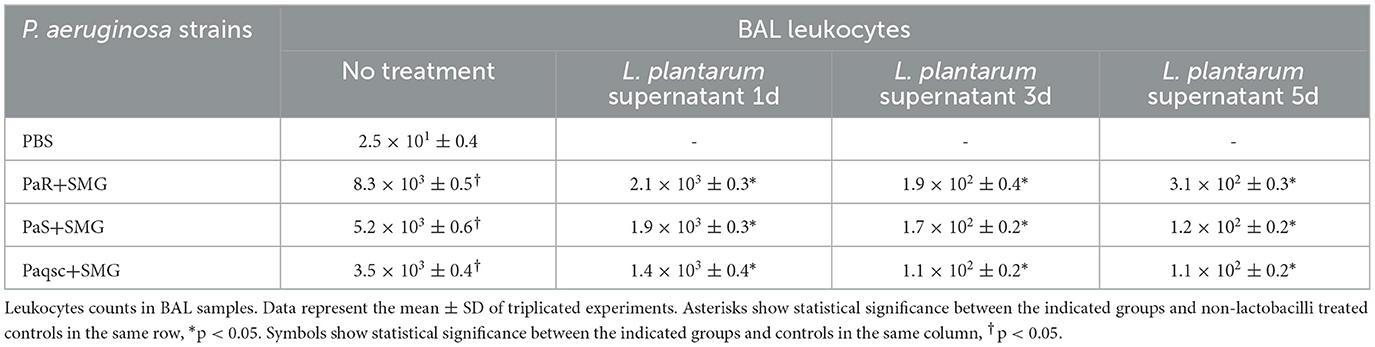
Table 7. Effect of L. plantarum supernatant on respiratory infections produced by P. aeruginosa strains and SMG.
The treatment with L. plantarum supernatant significantly reduced the levels of BAL leukocytes after 1, 3, and 5 days for the three P. aeruginosa strains administered with SMG. The lowest number of leucocytes in mice infected with PaR, PaS, and Paqsc strains and treated with L. plantarum supernatant was observed on day 5 (Table 7). The analysis of the different populations of BAL leucocytes showed that for PaR and SMG-infected mice, 5% were neutrophils, while 95% corresponded to macrophages. In animals challenged with PaS and SMG, BAL samples contained 20% neutrophils and 80% macrophages, while in mice infected with Paqsc and SMG, the samples contained 80% neutrophils and 20% macrophages. The treatment with L. plantarum supernatant significantly changed the proportion of neutrophils and macrophages in BAL in both PaR and SMG and PaS and SMG-infected mice.
A total of 60% neutrophils and 40% macrophages were found in the first group, and 70% neutrophils and 30% macrophages were found in the latter group. Notably, the treatment with L. plantarum supernatant did not induce any changes in the proportion of neutrophils and macrophages in the BAL of mice infected with P. aeruginosa Paqsc and SMG.
Lung histology was also studied to evaluate the tissue damage induced by P. aeruginosa and SMG infections (Figure 8 and Table 8). The three strains administered with SMG could produce higher lung tissue injury compared to infections with P. aeruginosa alone. It was also detected that PaR, PaS, and Paqsc administered with SMG produced different degrees of lung injury severity (Table 8). The PaR strain with SMG-induced hemorrhage, edema, and necrosis was accompanied by intense congestion and inflammatory infiltrates both in the alveolar and interstitial compartments (Figure 8A). Animals challenged with P. aeruginosa PaS and SMG also showed edema, congestion, hemorrhage, and inflammatory infiltrate in the alveolar and lung interstitial compartment that were lower than the observed in PaR and SMG-challenged mice (Figure 8B). The histopathological analysis also revealed that the infection produced by the mutant Paqsc strain was much milder when compared to the PaR and SGM infection but similar to the PaS and SMG challenge in some parameters such as edema, hemorrhage, and interstitial inflammatory infiltrate (Figure 8C and Table 8). When the effect of L. plantarum supernatant was evaluated, it was determined that after 1 day of treatments, there were no changes in lung tissue damage compared to the respective controls for any of the P. aeruginosa strains, as shown in the microscopic observations (Figure 8) and the scores (Table 8). However, the nebulization with L. plantarum supernatant for 3 or 5 days significantly decreased lung damage in animals infected with any of the three P. aeruginosa strains and SMG. The most notorious effect was found within 5 days of treatment.
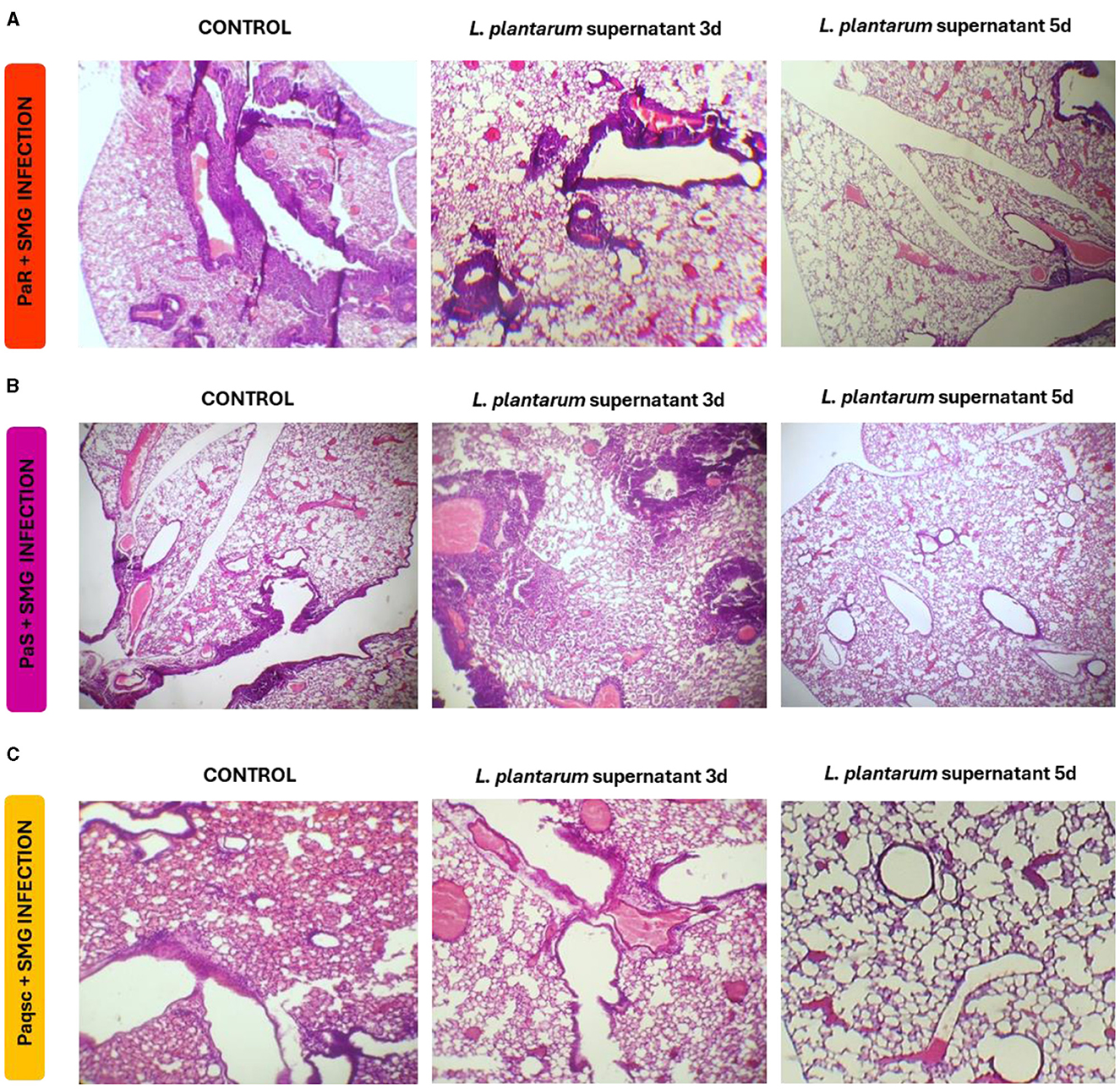
Figure 8. Effect of L. plantarum supernatant on respiratory superinfections produced by P. aeruginosa strains and SMG. The effects of L. plantarum supernatant on respiratory infections produced by different P. aeruginosa strains (Paqsc, PaS, and PaR) and SMG are shown. The histology of the lungs was determined. (A–C) Lung histopathological analysis at different points post-infection and treatment with L. plantarum supernatant for PaR, PaS, and Paqsc plus SMG, respectively. Data were obtained from duplicated experiments (n = 3 per experiment).
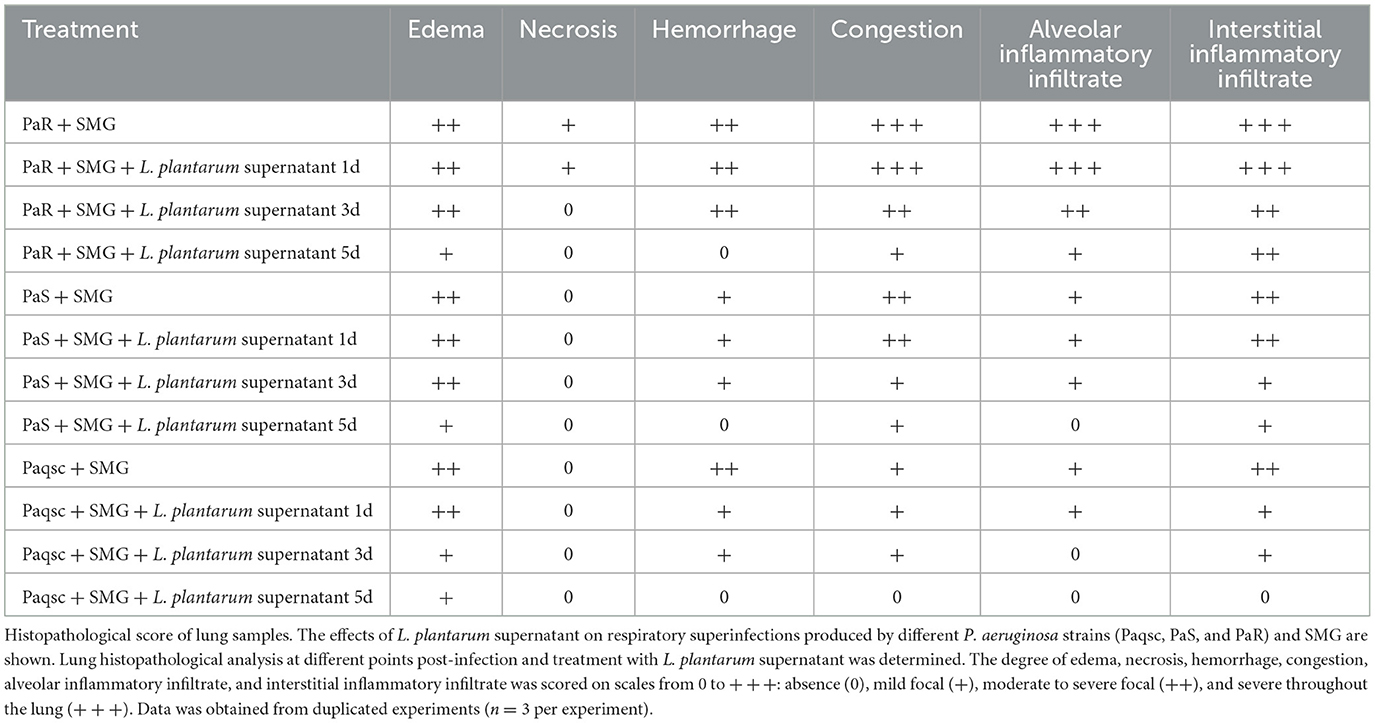
Table 8. Effect of L. plantarum supernatant on respiratory infections produced by P. aeruginosa strains and SMG.
Discussion
The bacterium P. aeruginosa is a ubiquitous microorganism encountered in the environment that usually does not cause problems to human health. However, P. aeruginosa is also a formidable opportunistic pathogen that can cause severe infections in susceptible hosts such as those with chronic lung diseases, including CF patients (Faure et al., 2018). Respiratory infections by P. aeruginosa in CF patients can be produced by phenotypically distinct strains that vary in the virulence factors and biofilm expressions as well as in their antibiotic resistance (Rossi et al., 2018, 2020). Although it is generally assumed that multi-drug-resistant P. aeruginosa strains are less frequently associated with infection, inflammatory responses, and mortality than susceptible strains (Horcajada et al., 2019), it was suggested that some resistance mutations are not associated with fitness/virulence reduction (Skurnik et al., 2013; Pacheco et al., 2017). Furthermore, it was demonstrated that multi-drug-resistant P. aeruginosa strains could develop compensatory mutations that allow them to preserve their virulence (Suárez et al., 2010). In line with these studies, we demonstrated here that both PaR and PaS isolated from CF patients, when administered by the nasal route, can produce infections in the respiratory tract of mice, triggering inflammatory responses and inducing lung damage. Notably, the capacity of PaR to induce inflammation and lung damage (Figure 9A) was superior to the observed for PaS (Figure 9B). In addition, the respiratory infection caused by the Paqsc strain was less severe than the one induced by PaR and PaS. These results were expected since it was shown that AHL signaling is an important virulence factor in P. aeruginosa (Whiteley et al., 1999; Schuster et al., 2003). There are two AHL quorum-sensing systems in this bacterium, LasI-LasR and RhlI-RhlR. The LasI is responsible for synthesizing 3O-C12-HSL, while RhlI is responsible for synthesizing C4-HSL. The production of these two molecules, which are regulators of gene expression and virulence, is not produced by Paqsc. Then, we conducted experiments using three P. aeruginosa strains that produce respiratory infections with a different degree of severity.
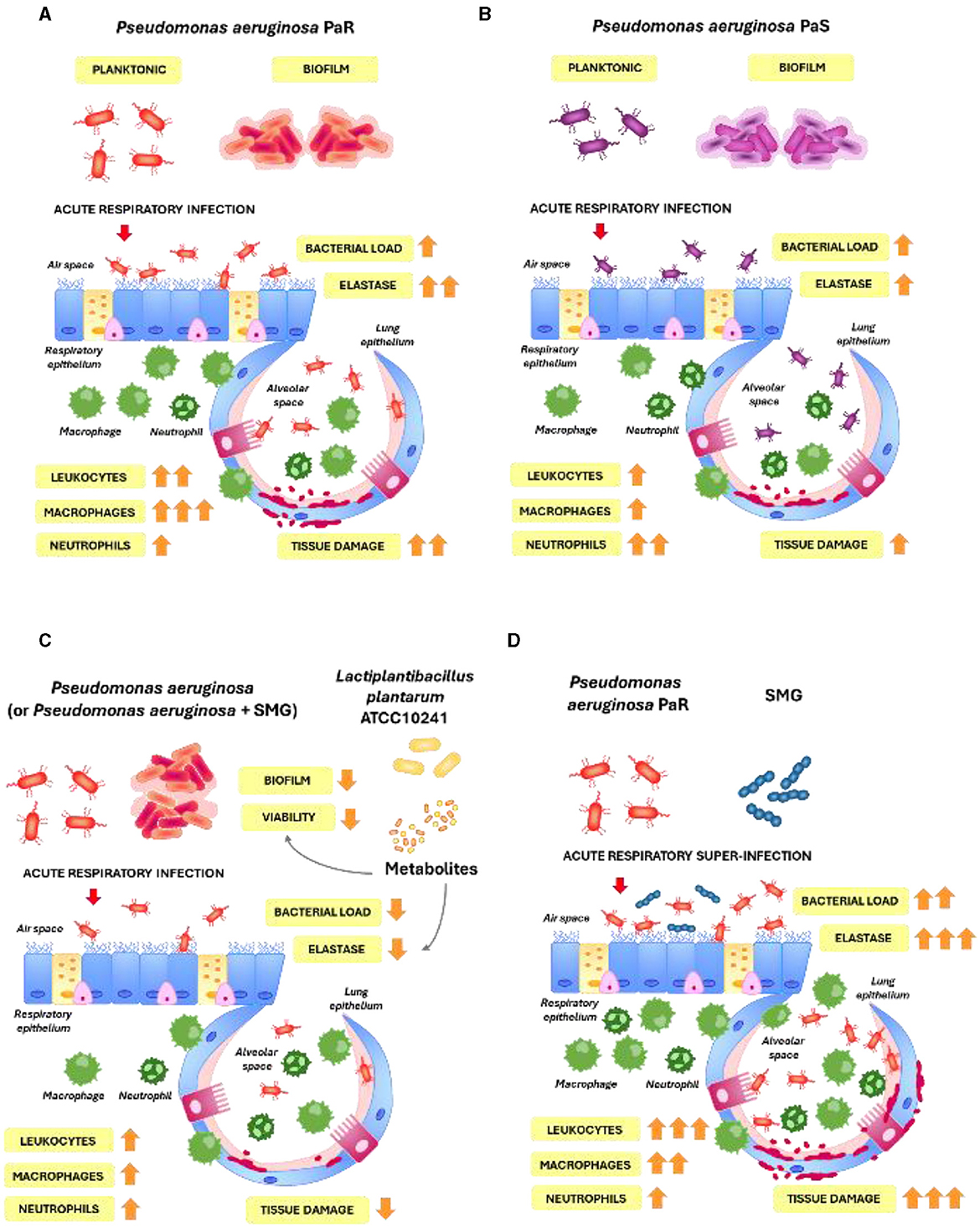
Figure 9. Ability to form biofilms and respiratory infections produced by P. aeruginosa PaR (A) and PaS (B). Effect of L. plantarum supernatant on biofilm formation and respiratory infections produced by P. aeruginosa (C). Respiratory superinfections produced by P. aeruginosa and SMG (D).
It should be considered that the differences in the virulence of P. aeruginosa in patients with CF are given by the moment the pathogens are studied. Increased antibiotic resistance and decreased virulence are generally observed during chronicity (Faure et al., 2018). In our experimental model, infections with PaR and PaS occurred acutely, thus it is expected that the microorganisms would not present the transcriptional changes induced in the CF lung during chronicity, allowing them to cause acute infections. In this regard, it was reported that the expressions of some virulence factors, such as proteases and secretion systems, in bacteria growing in biofilms, are maintained at similar levels to those found in free-living cells (Rossi et al., 2018; Kordes et al., 2019), whereas other virulence factor genes experience a reversion of regulation when the environmental conditions of chronic states are eliminated (Bartell et al., 2019). These results indicate that both PaR and PaS are strains with intrinsic virulence capable of inducing both acute infections, as demonstrated in the in vivo experiments in this study, and chronic infections, as observed in the patients from which they were isolated (Delgado et al., 2018). It is also possible to conclude that PaR would be intrinsically more virulent than PaS. This statement is supported by our results showing that PaR produced higher levels of elastase in the respiratory tract of infected mice than PaS. P. aeruginosa produces several secreted proteases, including the elastase, that allow the bacteria to interact with a wide range of molecules in the host, causing direct tissue damage. Therefore, elastase is considered a key virulence factor in the pathogenesis of acute infections (Tang et al., 1996; Le Berre et al., 2011; Faure et al., 2018).
In addition, we evaluated whether the respiratory administration of the cell-free supernatant of L. plantarum ATCC® 10241TM induced some beneficial effect in the infection and inflammatory response of PaR, PaS, and Paqsc in vivo, considering our previous data demonstrating its potential to inhibit the multiplication, viability and, AHL synthesis of P. aeruginosa strains isolated from wounds (Valdéz et al., 2005). These results were confirmed and extended by in vitro studies in this study, which used pathogen strains isolated from the respiratory tract of CF patients. Although clinically controlled trials demonstrated that probiotic supplementation could diminish pulmonary exacerbations and enhance the quality of life of children with CF (reviewed in Neri et al., 2019), the mechanism of micro-microbe and microbe-host interaction in this context has not been explored in depth. Notably, most of the studies investigating the potential of lactobacilli as an alternative antimicrobial therapy against P. aeruginosa in CF were performed in in vitro studies, while fewer were explored in vivo. In addition, most of the studies evaluated orally administered probiotic strains that would exert their beneficial effects on the respiratory tract through the modulation of the gut–lung axis (Neri et al., 2019; Villena and Kitazawa, 2020). However, it seems more rational that the beneficial effect of probiotics might be potentiated by directly administering them into the respiratory tract. In this way, bacteria and/or their metabolites would have the possibility to directly act on the lung pathogens and/or modulate the local immune responses. In this study, we offer solid data to support this statement by demonstrating that the nebulization with L. plantarum supernatant can reduce the susceptibility of mice to respiratory infections induced by P. aeruginosa strains isolated from CF patients. In our study, the administration of L. plantarum supernatant reduced the lung bacterial cell counts, diminished lung tissue damage, and differentially regulated the respiratory immune response in mice infected with PaR or PaS as well as the less virulent strain Paqsc (Figure 9C).
L. plantarum supernatant treatment reduced the inflammatory damage and differentially regulated the number and proportions of leucocytes in the respiratory tract after the infection with PaR, PaS, and Paqsc. Notably, the elastase from P. aeruginosa has been associated with augmented neutrophilic inflammation and lung damage during acute infection in mice (Qu et al., 2018). It was reported that mice infected with high elastase-producing strains have elevated inflammation and damage in the respiratory tract associated with high levels of MPO, IL-1β, IL-6, and KC (Zupetic et al., 2021). Furthermore, high elastase activity is common in P. aeruginosa strains isolated from severely ill patients (Zupetic et al., 2021). In mice infected with PaR, higher levels of elastase and lung damage were observed, but a lower proportion of neutrophils were detected. The smaller amounts of neutrophils in the respiratory tract of PaR-infected mice compared with animals receiving PaS or Paqsc could be associated with higher cellular necrosis induced by the most virulent strain. It was shown that rhamnolipids produced by pathogenic P. aeruginosa cause the lysis of several cellular human immune cells, including polymorphonuclear leukocytes (Van Gennip et al., 2009). Furthermore, in a pulmonary model of P. aeruginosa infections in mice, it was demonstrated that bacteria with a reduced ability to produce rhamnolipids did not cause the necrotic death of neutrophils and were cleared more efficiently from the lungs of infected mice.
The respiratory administration of the cell-free supernatant of L. plantarum ATCC® 10241TM was not only effective in increasing the resistance of mice to the challenge with P. aeruginosa but also reduced the lung bacterial loads and the inflammatory damage in mice infected with P. aeruginosa and SMG. In an in vitro biofilm model, it was shown that the coculture of P. aeruginosa DWW2 with S. anginosus 3a, both pathogens isolated from CF patients, resulted in significantly higher numbers of streptococci than the observed in monocultures (Waite et al., 2017). In addition, P. aeruginosa grown in the presence of streptococci had increased expression of virulence factors (pyocyanin) and caused a higher pathogenicity in Galleria mellonella. In line with these previous studies, we showed here that the co-infection of mice with P. aeruginosa and SMG significantly augmented the bacterial loads in the lungs and potentiated the inflammatory response, resulting in greater damage of the lung tissue than the observed in animals infected only with P. aeruginosa (Figure 9D). The SMG (S. anginosus) isolated from CF patients exacerbated the respiratory infections induced by the PaR, PaS, and Paqsc strains. Notably, the in vitro studies performed in this study showed an opposite effect when the interaction of PaR, PaS, and Paqcs with SMG was evaluated. SMG inhibited the biofilm formation of P. aeruginosa strains, and in in vitro, bacteria grow together and cannot be isolated in separate niches for a short period of time. Then, a direct inhibition is easier than when these interactions occur in vivo, in which longer interactions are expected, and other bacteria from the local microbiota and host factors are present. Studies of P. aeruginosa biofilm formation on CF-derived airway cells showed that Streptococcus constellatus induced no effect in the in vitro model. However, these streptococci are associated with the onset of pulmonary exacerbations in CF patients (Price et al., 2015). Thus, these data indicate that in vitro studies evaluating the interactions of CF pathogens should be interpreted in context since a similar effect will not necessarily be observed in vivo, in which a complex network of microbial and host interactions occurs.
Few studies reported the beneficial effects of probiotics on respiratory superinfections. Our previous studies demonstrated that the nasal administration of Lacticaseibacillus rhamnosus CRL1505 (Clua et al., 2017, 2020) or Ligilactobacillus salivarius FFIG58 (Elean et al., 2023) to infant and adult immunocompetent mice can modulate the local immune response and increase the resistance to a virus-bacteria respiratory superinfection. We demonstrated the benefits of nasally administered probiotics in respiratory superinfection by showing a higher resistance to primary infection with respiratory syncytial virus (RSV) and secondary infection with Streptococcus pneumoniae. These beneficial effects were associated with the higher capacity of probiotic-treated animals to limit the growth of pathogens and efficiently regulate TLR3-mediated lung inflammation (Clua et al., 2020; Elean et al., 2023). To the best of our knowledge, the potential beneficial effects of nasally administered probiotics and/or their metabolites to improve the resistance to a bacterial respiratory superinfection have not been reported before. Then, the studies presented here show for the first time the ability of an L. plantarum supernatant treatment to favorably impact the P. aeruginosa and SMG respiratory superinfection, allowing the reduction of bacterial loads in the lungs, a more controlled inflammation, and lower damage to the lung tissue. Although the nasal administration of probiotic strains, such as L. rhamnosus CRL1505 (Clua et al., 2020) or L. salivarius FFIG58 (Elean et al., 2023), could have beneficial effects on P. aeruginosa and SMG respiratory superinfection, it should be considered that the treatment will be applied to CF patients in which the administration of viable microorganisms could represent a risk. Then, L. plantarum supernatant can offer a safer alternative. Another point of contrast with our previous publications is that the CRL1505 and FFIG58 strains were administered preventively before the induction of RSV and S. pneumoniae superinfection and were associated with a differential modulation of alveolar macrophages that coordinated an improved innate respiratory immune response against both pathogens (Clua et al., 2020; Elean et al., 2023). Here, L. plantarum supernatant was administered after the challenges with the pathogens. The results allow to speculate that the L. plantarum supernatant, which contains a complex mixture of compounds with antimicrobial capacities including lactic, butyric, acetic, and succinic acids, H2O2, benzoic acid, 5-methyl hydantoin, 2,5-mevalonolactone, and isobutyl piperazinedione, would exert antagonistic effects directly on P. aeruginosa and SMG, allowing a reduction of their multiplication and biofilm formation and indirectly to a more efficient performance of the local immune system.
The limitations of our study open the doors for future investigations. An interesting question for future research is to determine whether the effect of the supernatant on lung inflammatory damage is due only to its ability to inhibit the growth and biofilm formation of the PaR and PaS strains or whether it can also exert a direct immunomodulatory effect. Kinetic studies of different immune cell populations, cytokines, and chemokines in the respiratory tract could provide valuable information. In addition, it is necessary to test whether the L. plantarum supernatant can exert a beneficial effect on chronic P. aeruginosa infections in in vivo models. These are studies that we intend to explore in the immediate future. Furthermore, clinical studies in CF patients that demonstrate both the efficacy and the security of L. plantarum supernatant are necessary.
Conclusion
Alternative or adjuvant therapies that minimize direct bacterial damage to the host and enhance protective responses can improve infection outcomes in CF patients. Such therapies are particularly needed considering the alarming rise in drug resistance in chronic pulmonary infections. In this study, we provided evidence supporting the potential use of the cell-free supernatant of L. plantarum ATCC® 10241TM to induce beneficial effects of respiratory infections and the inflammatory response by P. aeruginosa, either alone or in combination with SMG. Although further mechanistic studies are necessary, our results show that the cell-free supernatant of L. plantarum ATCC® 10241TM presents a promising adjuvant alternative for treating P. aeruginosa respiratory infections and superinfections in CF patients.
Data availability statement
The original contributions presented in the study are included in the article/Supplementary material, further inquiries can be directed to the corresponding authors.
Ethics statement
The animal study was approved by Bioethics Committee of the Faculty of Biochemistry, Chemistry and Pharmacy, National University of Tucuman. The study was conducted in accordance with the local legislation and institutional requirements.
Author contributions
CA: Writing – original draft, Methodology, Investigation, Formal analysis, Conceptualization. SOro: Writing – review & editing, Methodology, Investigation, Data curation, Conceptualization. JA: Writing – review & editing, Methodology, Investigation, Formal analysis. LA: Writing – review & editing, Validation, Methodology, Formal analysis, Data curation. AV: Writing – review & editing, Methodology, Investigation. LP: Writing – review & editing, Methodology, Investigation. SOrt: Writing – review & editing, Methodology, Investigation. KN: Writing – review & editing, Methodology, Investigation, Formal analysis. JCV: Writing – original draft, Project administration, Funding acquisition, Conceptualization. HK: Writing – review & editing, Supervision, Project administration, Funding acquisition. JV: Writing – original draft, Supervision, Resources, Project administration, Funding acquisition, Conceptualization. NG: Writing – review & editing, Validation, Supervision, Resources, Funding acquisition.
Funding
The author(s) declare financial support was received for the research, authorship, and/or publication of this article. This study was supported by the ANPCyT–FONCyT grants: PICT-2016-0410 and PICT-2021-I-A-00705 awarded to JV and by the PIUNT Project 26/D473 from the Research Council of the National University of Tucuman to NG. Additionally, the study received support from a Grant-in-Aid for Scientific Research (A) (23H00354), a Challenging Research (Exploratory, 23K18072) from the Japan Society for the Promotion of Science (JSPS), the Research Program on Development of Innovative Technology grants (JPJ007097) from the Project of the Bio-oriented Technology Research Advancement Institution (BRAIN), the Japan Racing Association, and AMED grant number: JP21zf0127001, all awarded to HK.
Conflict of interest
The authors declare that the research was conducted in the absence of any commercial or financial relationships that could be construed as a potential conflict of interest.
Publisher's note
All claims expressed in this article are solely those of the authors and do not necessarily represent those of their affiliated organizations, or those of the publisher, the editors and the reviewers. Any product that may be evaluated in this article, or claim that may be made by its manufacturer, is not guaranteed or endorsed by the publisher.
Supplementary material
The Supplementary Material for this article can be found online at: https://www.frontiersin.org/articles/10.3389/fmicb.2024.1440090/full#supplementary-material
References
Aybar, J. N. A., Ortiz Mayor, S., Olea, L., Garcia, J. J., Nisoria, S., Kolling, Y., et al. (2022). Topical administration of Lactiplantibacillus plantarum accelerates the healing of chronic diabetic foot ulcers through modifications of infection, angiogenesis, macrophage phenotype and neutrophil response. Microorganisms 10:634. doi: 10.3390/microorganisms10030634
Azami, S., Arefian, E., and Kashef, N. (2022). Postbiotics of Lactobacillus casei target virulence and biofilm formation of Pseudomonas aeruginosa by modulating quorum sensing. Archiv. Microbiol. 204:8. doi: 10.1007/s00203-022-02770-8
Bartell, J. A., Sommer, L. M., Haagensen, J. A. J., Loch, A., Espinosa, R., Molin, S., et al. (2019). Evolutionary highways to persistent bacterial infection. Nat. Commun. 10, 1–13. doi: 10.1038/s41467-019-08504-7
Batoni, G., Catelli, E., Kaya, E., Pompilio, A., Bianchi, M., Ghelardi, E., et al. (2023). Antibacterial and antibiofilm effects of lactobacilli strains against clinical isolates of Pseudomonas aeruginosa under conditions relevant to cystic fibrosis. Antibiotics 12:71158. doi: 10.3390/antibiotics12071158
Brao, K. J., Wille, B. P., Lieberman, J., Ernst, R. K., Shirtliff, M. E., and Harro, J. M. (2020). Scnn1b-Transgenic BALB/c mice as a model of Pseudomonas aeruginosa infections of the cystic fibrosis lung. Infect. Immun. 88:20. doi: 10.1128/IAI.00237-20
Cerca, N., Martins, S., Cerca, F., Jefferson, K. K., Pier, G. B., Oliveira, R., et al. (2005). Comparative assessment of antibiotic susceptibility of coagulase-negative staphylococci in biofilm versus planktonic culture as assessed by bacterial enumeration or Rapid XTT Colorimetry. J. Antimicrob. Chemother. 56, 331–336. doi: 10.1093/jac/dki217
Clua, P., Kanmani, P., Zelaya, H., Tada, A., Humayun Kober, A. K. M., Salva, S., et al. (2017). Peptidoglycan from immunobiotic Lactobacillus rhamnosus improves resistance of infant mice to respiratory syncytial viral infection and secondary pneumococcal pneumonia. Front. Immunol. 8:266735. doi: 10.3389/fimmu.2017.00948
Clua, P., Tomokiyo, M., Tonetti, F. R., Islam, M. A., Castillo, V. G., Marcial, G., et al. (2020). The role of alveolar macrophages in the improved protection against respiratory syncytial virus and pneumococcal superinfection induced by the peptidoglycan of Lactobacillus rhamnosus CRL1505. Cells 9:1653. doi: 10.3390/cells9071653
Delgado, G., Valdez, J. C., Gobbato, N., Orosco, S., Saguir, P., Silva, C., et al. (2018). Bacteria-bacteria and host-bacteria interaction of bacteria isolated from a patient with cystic fibrosis. Int. J. Sci. Res. 7, 63–65. doi: 10.36106/IJSR
Díaz, M. A., González, S. N., Alberto, M. R., and Arena, M. E. (2020). Human probiotic bacteria attenuate Pseudomonas aeruginosa biofilm and virulence by quorum-sensing inhibition. Biofouling 36, 597–609. doi: 10.1080/08927014.2020.1783253
Duan, K., Dammel, C., Stein, J., Rabin, H., and Surette, M. G. (2003). Modulation of Pseudomonas aeruginosa gene expression by host microflora through interspecies communication. Mol. Microbiol. 50, 1477–1491. doi: 10.1046/j.1365-2958.2003.03803.x
Elean, M., Raya Tonetti, F., Fukuyama, K., Arellano-Arriagada, L., Namai, F., Suda, Y., et al. (2023). Immunobiotic Ligilactobacillus salivarius FFIG58 confers long-term protection against Streptococcus pneumoniae. Int. J. Mol. Sci. 24:15773. doi: 10.3390/ijms242115773
Faure, E., Kwong, K., and Nguyen, D. (2018). Pseudomonas aeruginosa in chronic lung infections: how to adapt within the host? Front. Immunol. 9:2416. doi: 10.3389/fimmu.2018.02416
Gambello, M. J., and Iglewski, B. H. (1991). Cloning and characterization of the Pseudomonas aeruginosa LasR Gene, a transcriptional activator of elastase expression. J. Bacteriol. 173:3000. doi: 10.1128/jb.173.9.3000-3009.1991
Gibson, R. L., Burns, J. L., and Ramsey, B. W. (2003). Pathophysiology and management of pulmonary infections in cystic fibrosis. Am. J. Respirat. Crit. Care Med. 168, 918–951. doi: 10.1164/rccm.200304-505SO
Horcajada, J. P., Montero, M., Oliver, A., Sorlí, L., Luque, S., Gómez-Zorrilla, S., et al. (2019). Epidemiology and treatment of multidrug-resistant and extensively drug-resistant Pseudomonas aeruginosa infections. Clin. Microbiol. Rev. 32:19. doi: 10.1128/CMR.00031-19
Kordes, A., Preusse, M., Willger, S. D., Braubach, P., Jonigk, D., Haverich, A., et al. (2019). Genetically diverse Pseudomonas aeruginosa populations display similar transcriptomic profiles in a cystic fibrosis explanted lung. Nat. Commun. 10, 1–10. doi: 10.1038/s41467-019-11414-3
Laborda, P., Hernando-Amado, S., Martínez, J. L., and Sanz-García, F. (2022). Antibiotic resistance in pseudomonas. Adv. Exp. Med. Biol. 1386, 117–143. doi: 10.1007/978-3-031-08491-1_5
Le Berre, R. L., Nguyen, S., Nowak, E., Kipnis, E., Pierre, M., Quenee, L., et al. (2011). Relative contribution of three main virulence factors in Pseudomonas aeruginosa pneumonia. Crit. Care Med. 39, 2113–2120. doi: 10.1097/CCM.0b013e31821e899f
Miller, J. H. (1992). A Short Course in Bacterial Genetics: A Laboratory Manual and Handbook for Escherichia coli and Related Bacteria. Plainview, NY: Cold Spring Harbor Laboratory Press.
Neri, L. D. C. L., Taminato, M., and Da Silva Filho, L. V. R. F. (2019). Systematic review of probiotics for cystic fibrosis patients: moving forward. J. Pediatr. Gastroenterol. Nutr. 68, 394–399. doi: 10.1097/MPG.0000000000002185
O'Toole, G. A., and Kolter, R. (1998). Initiation of biofilm formation in Pseudomonas fluorescens WCS365 proceeds via multiple, convergent signalling pathways: a genetic analysis. Mol. Microbiol. 28, 449–461. doi: 10.1046/j.1365-2958.1998.00797.x
Pacheco, J. O., Alvarez-Ortega, C., Rico, M. A., and Martínez, J. L. (2017). Metabolic compensation of fitness costs is a general outcome for antibiotic-resistant Pseudomonas aeruginosa mutants overexpressing efflux pumps. MBio 8:17. doi: 10.1128/mBio.00500-17
Peral, M. C., Huaman Martinez, M. A., and Valdez, J. C. (2009). Bacteriotherapy with Lactobacillus plantarum in burns. Int. Wound J. 6:73. doi: 10.1111/j.1742-481X.2008.00577.x
Peral, M. C., Rachid, M. M., Gobbato, N. M., Huaman Martinez, M. A., and Valdez, J. C. (2010). Interleukin-8 production by polymorphonuclear leukocytes from patients with chronic infected leg ulcers treated with Lactobacillus plantarum. Clin. Microbiol. Infect. 16, 281–286. doi: 10.1111/j.1469-0691.2009.02793.x
Pompilio, A., Kaya, E., Lupetti, V., Catelli, E., Bianchi, M., Maisetta, G., et al. (2024). cell-free supernatants from lactobacillus strains exert antibacterial, antibiofilm, and antivirulence activity against Pseudomonas aeruginosa from cystic fibrosis patients. Microbes Infect. 2024:105301. doi: 10.1016/j.micinf.2024.105301
Price, K. E., Naimie, A. A., Griffin, E. F., Bay, C., and O'Toole, G. A. (2015). Tobramycin-treated Pseudomonas aeruginosa PA14 enhances Streptococcus constellatus 7155 biofilm formation in a cystic fibrosis model system. J. Bacteriol. 198, 237–247. doi: 10.1128/JB.00705-15
Qu, Y., Olonisakin, T., Bain, W., Zupetic, J., Brown, R., Hulver, M., et al. (2018). Thrombospondin-1 protects against pathogen-induced lung injury by limiting extracellular matrix proteolysis. JCI Insight 3:96914. doi: 10.1172/jci.insight.96914
Ramos, A. N., Sesto Cabral, M. E., Arena, M. E., Arrighi, C. F., Arroyo Aguilar, A. A., and Valdéz, J. C. (2015). Compounds from Lactobacillus plantarum culture supernatants with potential pro-healing and anti-pathogenic properties in skin chronic wounds. Pharmaceut. Biol. 53, 350–358. doi: 10.3109/13880209.2014.920037
Reading, N. C., and Sperandio, V. (2006). Quorum sensing: the many languages of bacteria. FEMS Microbiol. Lett. 254, 1–11. doi: 10.1111/j.1574-6968.2005.00001.x
Reynolds, D., and Kollef, M. (2021). The epidemiology and pathogenesis and treatment of Pseudomonas aeruginosa infections: an update. Drugs 81, 2117–2131. doi: 10.1007/s40265-021-01635-6
Rossi, E., Falcone, M., Molin, S., and Johansen, H. K. (2018). High-resolution in situ transcriptomics of Pseudomonas aeruginosa unveils genotype independent patho-phenotypes in cystic fibrosis lungs. Nat. Commun. 9, 1–13. doi: 10.1038/s41467-018-05944-5
Rossi, E., La Rosa, R., Bartell, J. A., Marvig, R. L., Haagensen, J. A. J., Sommer, L. M., et al. (2020). Pseudomonas aeruginosa adaptation and evolution in patients with cystic fibrosis. Nat. Rev. Microbiol. 19, 331–342. doi: 10.1038/s41579-020-00477-5
Sala, M. A., and Jain, M. (2021). Pseudomonas aeruginosa and children with cystic fibrosis. Clin. Infect. Dis. 73, e2529–e2530. doi: 10.1093/cid/ciaa765
Schuster, M., Lostroh, C. P., Ogi, T., and Greenberg, E. P. (2003). Identification, timing, and signal specificity of Pseudomonas aeruginosa quorum-controlled genes: a transcriptome analysis. J. Bacteriol. 185, 2066–2079. doi: 10.1128/JB.185.7.2066-2079.2003
Scott, J. E., and O'Toole, G. A. (2019). The yin and yang of streptococcus lung infections in cystic fibrosis: a model for studying polymicrobial interactions. J. Bacteriol. 201:19. doi: 10.1128/JB.00115-19
Shokri, D., Khorasgani, M. R., Mohkam, M., Fatemi, S. M., Ghasemi, Y., and Taheri-Kafrani, A. (2018). The inhibition effect of lactobacilli against growth and biofilm formation of Pseudomonas aeruginosa. Probiot. Antimicrob. Proteins 10, 34–42. doi: 10.1007/s12602-017-9267-9
Sibley, C. D., Parkins, M. D., Rabin, H. R., Duan, K., Norgaard, J. C., and Surette, M. G. (2008). A polymicrobial perspective of pulmonary infections exposes an enigmatic pathogen in cystic fibrosis patients. Proc. Natl. Acad. Sci. U. S. A. 105, 15070–15075. doi: 10.1073/pnas.0804326105
Skurnik, D., Roux, D., Cattoir, V., Danilchanka, O., Lu, X., Yoder-Himes, D. R., et al. (2013). enhanced in vivo fitness of carbapenem-resistant OprD mutants of Pseudomonas aeruginosa revealed through high-throughput sequencing. Proc. Natl. Acad. Sci. U. S. A. 110, 20747–20752. doi: 10.1073/pnas.1221552110
Souza, G. H. d. A., Rossato, L., Brito, G. T., Dos Santos Bet, G. M., and Simionatto, S. (2021). Carbapenem-resistant Pseudomonas aeruginosa strains: a worrying health problem in intensive care units. Revista Do Instituto de Medicina Tropical de Sao Paulo 63:71. doi: 10.1590/s1678-9946202163071
Suárez, C., Peña, C., Gavaldà, L., Tubau, F., Manzur, A., Angeles Dominguez, M., et al. (2010). Influence of carbapenem resistance on mortality and the dynamics of mortality in Pseudomonas aeruginosa bloodstream infection. Int. J. Infect. Dis. 14(Suppl.3), e73–e78. doi: 10.1016/j.ijid.2009.11.019
Tang, H. B., Dimango, E., Bryan, R., Gambello, M., Iglewski, B. H., Goldberg, J. B., et al. (1996). Contribution of specific Pseudomonas aeruginosa virulence factors to pathogenesis of pneumonia in a neonatal mouse model of infection. Infect. Immun. 64, 37–43. doi: 10.1128/iai.64.1.37-43.1996
Tonetti, F. R., Tomokiyo, M., Fukuyama, K., Elean, M., Ortiz Moyano, R., Yamamuro, H., et al. (2023). Post-immunobiotics increase resistance to primary respiratory syncytial virus infection and secondary pneumococcal pneumonia. Beneficial Microbes 14, 209–221. doi: 10.3920/BM2022.0118
Tümmler, B. (2019). Emerging therapies against infections with Pseudomonas aeruginosa. F1000Research 8:1. doi: 10.12688/f1000research.19509.1
Valdéz, J. C., Peral, M. C., Rachid, M., Santana, M., and Perdigón, G. (2005). Interference of Lactobacillus plantarum with Pseudomonas aeruginosa in vitro and in infected burns: the potential use of probiotics in wound treatment. Clin. Microbiol. Infect. 11, 472–479. doi: 10.1111/j.1469-0691.2005.01142.x
Van Gennip, M., Christensen, L. D., Alhede, M., Phipps, R., Jensen, P. Ø., Christophersen, L., et al. (2009). Inactivation of the RhlA gene in Pseudomonas aeruginosa prevents rhamnolipid production, disabling the protection against polymorphonuclear leukocytes. APMIS 117, 537–546. doi: 10.1111/j.1600-0463.2009.02466.x
Villena, J., and Kitazawa, H. (2020). The modulation of mucosal antiviral immunity by immunobiotics: could they offer any benefit in the SARS-CoV-2 pandemic? Front. Physiol. 11:699. doi: 10.3389/fphys.2020.00699
Waite, R. D., Qureshi, M. R., and Whiley, R. A. (2017). Modulation of behaviour and virulence of a high alginate expressing Pseudomonas aeruginosa strain from cystic fibrosis by oral commensal bacterium Streptococcus anginosus. PLoS ONE 12:e0173741. doi: 10.1371/journal.pone.0173741
Whiteley, M., Lee, K. M., and Greenberg, E. P. (1999). Identification of genes controlled by quorum sensing in Pseudomonas aeruginosa. Proc. Natl. Acad. Sci. U. S. A. 96, 13904–13909. doi: 10.1073/pnas.96.24.13904
Keywords: Lactiplantibacillus plantarum, supernatant, respiratory infection, Pseudomonas aeruginosa, cystic fibrosis
Citation: Abán CL, Orosco S, Argañaraz Aybar JN, Albarracín L, Venecia A, Perret L, Ortiz Mayor S, Nishiyama K, Valdéz JC, Kitazawa H, Villena J and Gobbato N (2024) Effect of Lactiplantibacillus plantarum cell-free culture on bacterial pathogens isolated from cystic fibrosis patients: in vitro and in vivo studies. Front. Microbiol. 15:1440090. doi: 10.3389/fmicb.2024.1440090
Received: 28 May 2024; Accepted: 06 August 2024;
Published: 16 September 2024.
Edited by:
Michal Letek, University of León, SpainReviewed by:
Jose Ramos-Vivas, Universidad Europea del Atlántico, SpainAna Motos, Hospital Clinic of Barcelona, Spain
Copyright © 2024 Abán, Orosco, Argañaraz Aybar, Albarracín, Venecia, Perret, Ortiz Mayor, Nishiyama, Valdéz, Kitazawa, Villena and Gobbato. This is an open-access article distributed under the terms of the Creative Commons Attribution License (CC BY). The use, distribution or reproduction in other forums is permitted, provided the original author(s) and the copyright owner(s) are credited and that the original publication in this journal is cited, in accordance with accepted academic practice. No use, distribution or reproduction is permitted which does not comply with these terms.
*Correspondence: Nadia Gobbato, bmFkaWFnb2JiYXRvJiN4MDAwNDA7aG90bWFpbC5jb20=; Julio Villena, amN2aWxsZW5hJiN4MDAwNDA7Y2VyZWxhLm9yZy5hcg==; Haruki Kitazawa, aGFydWtpLmtpdGF6YXdhLmM3JiN4MDAwNDA7dG9ob2t1LmFjLmpw