- 1Key Laboratory of Surveillance and Management of Invasive Alien Species in Guizhou Education Department, College of Biological and Environmental Engineering, Guiyang University, Guiyang, China
- 2College of Agriculture/College of Life Sciences, Guizhou University, Guiyang, China
Walnut (Juglans regia L.) is a widely grown nut plant worldwide, including in Guizhou Province, located in southwest China. The high quality and special taste make Guizhou walnuts, particularly those produced in Hezhang County, a “Chinese National Geographical Indication Product” that substantially contributes to the local economy and grower’s income. In July 2022, a serious occurrence of leaf spot disease was observed in a walnut plantation area, Shuitang Town, Hezhang County, Guizhou Province, China (27°07′67″N, 104°64′61″E). The causal agent was identified as Didymella segeticola through morphological characterization and amplification and sequencing of the internal transcribed spacer (ITS) region, beta-tubulin (TUB) gene, and glyceraldehyde-3-phosphate dehydrogenase (G3PD) gene. Koch’s postulates, including re-isolation and identification, were performed to confirm its pathogenicity on healthy leaves. To our knowledge, this is the first report of D. segeticola causing leaf spot on walnuts worldwide. Further, to determine its biological characteristics, which could be utilized for future disease management, the effects of temperature, light, and carbon and nitrogen resources on mycelial growth, conidia production, and conidia germination and the effects of humidity on conidia germination were studied. The optimum temperature for mycelial growth of representative strain D. segeticola C27 was 20°C. Increasing the light period significantly decreased conidia production and conidia germination. Maltose and beef extract were the best carbon and nitrogen sources, respectively, for the pathogen. Conidia germination was enhanced at 90% humidity. In vitro screening of effective fungicides was conducted. Among the 20 screened fungicides, difenoconazole showed the best inhibition rate, with an EC50 (concentration for 50% of the maximal effect) of 0.0007 μg/mL. Tetramycin also showed sufficient inhibitory effects against D. segeticola, with an EC50 value of 0.0009 μg/mL. Our study provides new insights into the causal agent of walnut leaf spot in Guizhou, China, as well as the first pathogen characteristics and promising candidate fungicides for its management.
1 Introduction
Walnut (Juglans regia L.), a widely distributed and important cultivated nut plant acclaimed for its timber and nutritious kernels (Bayazit et al., 2007), is among the four most consumed dried fruits globally (Fordos et al., 2023). The history of walnut traces back to Persia around 7,000 BC (Dreher et al., 1996), and presently, it is commercially cultivated across Southern Europe, North Africa, East Asia, the United States, and western South America. Notably, China has emerged as the world’s leading producer of walnuts (Vahdati et al., 2019). The widespread appreciation of walnuts stems from their popularity and extensive consumption. Prior studies underscore their role in reducing the risk of heart disease caused by polyunsaturated fatty acids (Tapsell et al., 2004; Blomhoff et al., 2006; Hama and Fitzsimmons-Thoss, 2022; Glenn et al., 2023). Moreover, the roots, barks, leaves, and green core-shell peaches of walnuts have versatile utility, for example, in the pharmaceutical and cosmetic industries (Salejda et al., 2016). As such, all of the parts of the walnut tree are recognized as rich sources of diverse chemicals that contribute to the synthesizing of compound drugs. These drugs exhibit multifaceted therapeutic properties ranging from pain relief, anti-ulcer, anti-asthma, immune regulation, and larvicidal effects, thus positively influencing human health (Jahanban-Esfahlan et al., 2019). Hence, it is imperative to cultivate and protect the walnut industry sustainably (Sagawa et al., 2020).
However, the growth of Juglans regia L. faces significant threats from plant diseases, prim caused by bacteria, viruses, and fungi. For example, walnut bark canker caused by Brenneria nigrifluens (Charkhabi et al., 2010; Razinataj et al., 2020) and bacterial black spot induced by Xanthomonas campestris and Pantoea agglomerans have been found in Gansu, China (Wang et al., 2016). Similarly, walnut fruit canker caused by Pseudomonas syringae pv. Syringae has been reported in Iran (Keshtkar et al., 2016; Ahmadi et al., 2017). Walnut bacterial wilt caused by Xanthomonas arboricola pv. Juglandis (Xaj) has been reported in Korea (Kim et al., 2021). Notably, the emergence of the Plum pox virus infecting walnut trees was first documented in 1996 (Baumgartnerová, 1996), while a walnut disease associated by the Cherry leaf roll virus (CLRV) was found in Turkey in 2008 (Ozturk et al., 2008). Further, numerous fungal infections plague walnut trees and diminish fruit quality and yield. For example, in 2013, Chen et al. (2013) reported Lasiodiplodia citricola and Neoscytalidium dimidiatum as pathogens responsible for the decrease number of walnut trees. Subsequently, walnut anthracnose caused by Colletotrichum siamense led to severe damage to yield and quality of walnuts in Shandong Province, China, in 2017 (Wang et al., 2017), while the discovery of branch canker caused by Diaporthe amygdali in 2018 further adversely affected walnut cultivation in Shandong Province (Meng et al., 2018).
In this study, we investigated walnut cultivation in Bijie City, Guizhou Province, China, and we revealed the high incidence of walnut leaf spot, especially during the rainy season from June to August. This conducive environment facilitated the rapid proliferation and dissemination of the pathogen, resulting in widespread leaf spots on walnut leaves. Notably, walnut leaf spot can be caused by various pathogenic fungi. Prior studies have attributed walnut leaf spot to Boeremia exigua infection (Cai et al., 2021; Wang et al., 2022). In addition, Xu et al. (2023) reported walnut leaf spot disease caused by Ragnhildiana diffusa in Sichuan province of China, with symptoms of initially brown lesions encircled by yellow halos, progressing to extensive leaf coverage and eventual leaf shedding. Similarly, Wang F. H. et al. (2023) identified a leaf spot disease of walnut caused by Nothophoma quercina in Sichuan Province of China. Currently, fungicides are widely screened and utilized for walnut disease control. Prior studies have highlighted the efficacy of flusilazole, fludioxonil, propiconazole, and pyraclostrobin in inhibiting Colletotrichum fioriniae mycelial growth and spore germination, thus effectively preventing and managing walnut disease (Fan R. D. et al., 2023). Further, He et al. (2019) demonstrated the high sensitivity of Colletotrichum gloeosporioides s.s. to pyraclostrobin, difenoconazole, fipronil, tebuconazole, pyrazole, and tetramycin, inhibiting mycelial growth. In addition, Zhou et al. (2023) reported the effectiveness of prochloraz in controlling the leaf spot of Carya cathayensis caused by Fusarium oxysporum, Botrytis japonica, and Botrytis cinerea. Nevertheless, research on walnut leaf spot control remains limited. Prolonged reliance on chemical sterilization can foster pathogen resistance to fungicides (Tang et al., 2022; Li et al., 2023), as evidenced by prior studies demonstrating drug resistance among walnut pathogens (Wang et al., 2020).
Hence, screening chemical pesticides that exhibit low toxicity and high efficiency is paramount for disease control. In addition, comprehending the biology of pathogenic fungal is crucial to shed light on disease development and the emergence of fungicide resistance. Therefore, exploring the biology of these pathogens is indispensable. Accordingly, the objectives of this study were to (1) isolate and characterize the pathogens responsible for severe walnut leaf spot disease in the Hezhang County area of Bijie City, Guizhou Province, China; (2) elucidate the biological characteristics of pathogenic fungal; and (3) screen for effective fungicides to mitigate disease impact.
2 Materials and methods
2.1 Sample collection and fungal isolation
In July 2022, a severe outbreak of walnut leaf blight was identified in Shuitang Town, Hezhang County, Guizhou Province, China (27°07′67″N, 104°64′61″E). Within an evaluation area spanning 0.8 hectares, disease incidence rates reached 65–75%. To isolate fungi from infected leaves, diseased leaves from various plants were collected using sterile scissors and placed in self-sealing bags using disposable gloves to prevent cross-contamination. Two diseased leaves were collected from each plant, and five plants were sampled. Collected diseased leaves were then cut into 5 × 5 mm tissue blocks at the junction of diseased and healthy leaves using sterile scissors. Subsequently, tissue blocks were soaked in 4% w/v sodium hypochlorite (NaClO) solution for 30 s, immersed in 75% v/v ethanol for 3 min, and rinsed with sterile distilled water (ddH2O) three times, with each rinse lasting more than 1 min. The treated leaves were then placed on potato dextrose agar (PDA) medium and incubated at 28°C for 5 days. Colonies grown on the medium were observed, and fungal colonies were purified by transferring single spores to obtain purified strains for further identification.
2.2 Morphological and molecular identification
Three representative fungal strains were examined microscopically, and the morphological characteristics of fungal conidia were observed using a Nikon Ni-D Microscopic System (Nikon Digital Sight 10). Sixty conidia were randomly selected, and their lengths and widths were measured and recorded. For molecular identification, genomic DNA of the isolated strains was extracted from hyphae growing on PDA medium at 28°C using Omega Fungal DNA Extraction Kit (CA, United States) following the manufacturer’s instructions. The extracted DNA was stored at −20°C. Partial sequences of the internal transcribed spacer (rDNA-ITS), beta-tubulin (TUB) gene, and glyceraldhyde-3-phosphate dehydrogenase (G3PD) gene were amplified by PCR using specific primers. The primers used were ITS1 (5′-TCCGTAGGTGAACCTGCGG-3′) and ITS4 (5′-GCTGCG TTCTTCATCGATGC-3′) (White et al., 1990), Bt2a-F (5′-GGTAACC AAATCGGTGCTGCTTTC-3′) and Bt2b-R (5′-ACCCTCAGTG TAGTGACCCTTGGC-3′) (Carbone and Kohn, 1999), and GD-F (5′-GCCGTCAACGACCCCTTCATTGA-3′) and GD-R (5′-GG GTGGAGTCGTACTTGAGCA-3′) (Templeton et al., 1992). PCR amplification was carried out using a Bio-Rad T100™ Thermal Cycler and a reaction system comprising the following for a total volume of 25 μL: 1 μL DNA template, 1 μL each of forward and reverse primers, 9.5 μL ddH2O, and 12.5 μL 2 × SanTaq PCR Mix. The PCR conditions consisted of initial denaturation at 94°C for 5 min, followed by 35 cycles of denaturation at 94°C for 30 s, annealing for 30 s at the corresponding temperatures (53°C for the ITS region, 55°C for the TUB gene, and 53.6°C for the G3PD gene), extension at 72°C for 45 s, and then a final extension at 72°C for 10 min. The PCR products obtained were sequenced by Qingke Bio (Chongqing) Technology Co., Ltd. The obtained sequences were compared and analyzed in GenBank. Subsequently, sequences were spliced according to ITS-β-tubulin-G3PD, and the phylogenetic tree was constructed using neighbor-joining clustering analysis in MEGA 7.0 software (Kumar et al., 2016). The obtained DNA sequences were uploaded to the National Center for Biotechnology Information.
2.3 Pathogenicity test
The mycelium disc inoculation method described by Chen Y. et al. (2021) were employed to confirm Koch’s postulates. Healthy walnut leaves were carefully selected for the experiment. Prior to inoculation, the leaves underwent a series of sterilization steps, including being rinsed with 75% alcohol for 1 min, washed with 0.4% sodium hypochlorite solution for 30 s, and then rinsed with sterile water three times (1 min each). Subsequently, the washed leaves were dried with sterile paper and gently scratched using a sterile inoculation needle. A 5 mm diameter mycelial plug of the representative isolate D. segeticola strain C27 was used to inoculate the sterilized leaves. Leaves inoculated with sterile PDA medium cakes were used as the control group. The inoculated leaves were kept moist by covering them with sterile absorbent cotton. The leaves were then incubated in a constant temperature incubator at 28°C, with a photoperiod of 16 h/8 h and relative humidity (RH) of 75%. Disease symptoms on the leaves were observed after 5 days of incubation. Three replicates were established for each treatment group to ensure the reliability and reproducibility of the results. The pathogenicity test was conducted and repeated three times.
2.4 Effect of temperature on mycelial growth, conidia production, and conidia germination rate
To investigate the impact of different temperatures on the colony diameter and conidia yield of the pathogenic fungal, a 5 mm diameter mycelial plug of pathogen D. segeticola C27 (7-day-old) was used to inoculate the center of sterile PDA culture medium and cultured in a constant temperature incubator at temperatures ranging from 5 to 35°C at 5°C intervals. Each treatment was repeated three times. Twelve days after inoculation, the growth diameter of hyphae was recorded using the cross method (Hu et al., 2022). Simultaneously, 10 agar-mycelium plugs containing hyphae were collected from the edge of pathogen colonies cultured for 12 days using a 5 mm sterile punch and transferred to sterile centrifuge tubes containing sterile water. The centrifugal tube containing the pathogenic fungal cakes was vigorously vortexed to dissolve the conidia from the pathogenic fungal mycelium in sterile water and filtered using sterile double-layer gauze. The number of conidia per square centimeter of the colony was counted using a hemocytometer and calculated using the following formula:
where X is the dilution fold, N is the number of conidia in five squares of the hemacytometer, n is the number of perforated agar–mycelium plugs, and r is the inner diameter of the perforator (Deng et al., 2012; Cao et al., 2022).
To determine the conidia germination rate, spores from the 12-day-old colony were washed with sterile water, and the conidia concentration was adjusted to 1 × 105 conidia/mL using a hemocytometer. Subsequently, 0.2 mL of conidia suspension was added to 0.2 mL of potato broth medium (PDB) and cultured at different temperatures for 24 h. The number of germinated conidia was observed using a microscope, and the conidia germination rate was calculated accordingly.
2.5 Effect of photoperiod on mycelial growth, conidia production, and conidia germination rate
To investigate the effects of photoperiod on pathogen D. segeticola C27, the culture medium inoculated with pathogenic fungal (PDA for mycelial growth and conidia production, and PDB for conidia germination rate) was placed in a constant temperature incubator at 28°C. The following photoperiod settings were utilized: (a) 6 h light and 18 h dark; (b) 10 h light and 14 h dark; (c) 14 h light and 10 h dark; and (d) 18 h light and 6 h dark. Three replicates were established for each treatment group to ensure the reliability and reproducibility of the results.
2.6 Effect of different carbon and nitrogen sources on mycelium growth, conidia production, and conidia germination rate
The mycelium growth, conidia production, and conidia germination rate of D. segeticola C27 on various carbon and nitrogen sources were determined following methods adapted from prior research (Wu et al., 2023; Wang H. C. et al., 2023). Czapek’s medium served as the base medium, with sucrose or sodium nitrate substituted by different carbon or nitrogen sources of equal mass. Six carbon sources, namely glucose, maltose, soluble starch, D-fructose, lactose, and sucrose, were investigated alongside six nitrogen sources: NaNO3, (NH4)2SO4, beef extract, peptone, glycine, and urea. Representative fungal cakes measuring 5 mm in diameter were used to inoculate the center of Czapek’s agar plates containing different carbon and nitrogen sources on the periphery of PDA medium containing the pathogenic fungal. The plates were then cultured in a constant temperature incubator at 28°C for 12 days. Subsequently, the mycelium diameter, conidia production, and conidia germination rate were measured. Three replicates were established for each treatment group to ensure the reliability and reproducibility of the results.
2.7 Effect of humidity on conidia germination rate
To assess the impact of relative humidity on the conidia germination rate of D. segeticola C27, a suspension with a concentration of 1 × 105 conidia/mL was applied to sterile glass slides and dried using sterile air in a sterile biosafety cabinet. Subsequently, the dried glass slides were placed in sterile Petri dishes, and the relative humidity levels (50, 60, 70, 80, 90, and 100%) were evaluated in culture at 28°C in the dark. After 24 h, conidia germination was observed using a microscope to calculate the conidia germination rate. Three replicates were established for each treatment group to ensure the reliability and reproducibility of the results.
2.8 In vitro antifungal activity of fungicides on mycelial growth
The antifungal activities of 14 chemical and 6 biological fungicides against D. segeticola C27 were assessed using the mycelium growth rate method (Xin et al., 2020). Different fungicides were dissolved in water or organic solvent, with specific solvents chosen according to the fungicide type (e.g., pyraclostrobin and benzalconazole were dissolved in ethyl alcohol; thiophanate-methyl and fluazinam were dissolved in acetone; pyraclostrobin tebuconazole, prochloraz, mancozeb, difenoconazole, flusilazole, dimetachlone, jingangmycin, metalaxyl hymexazol, kasugamycin, carvacrol, zhongshengmycin, ethylicin, tetramycin, thiram, zineb, and cymoxanil were dissolved in water). These solutions were then diluted (Table 1), added to PDA culture medium, and thoroughly mixed to create the treatment group. An equal amount of the respective solvent was added to the PDA culture medium as the control group (CK). The mixture was ventilated, cooled, and solidified. Subsequently, a 5 mm cake of the representative D. segeticola C27 strain was used to inoculate the center of fungicide culture media with different concentration gradients. The inoculated plates were placed in a constant temperature incubator at 28°C and 70% humidity for 12 days. The colony diameter (mm) was measured using the crisscross method. The percent inhibition of mycelial growth (PIMG) was calculated using the following formula (Usman et al., 2022):
where F is the diameter of the fungal plug, C is the radial growth diameter of the fungus in the control, and T is the radial growth diameter of the fungus in the treatment group.
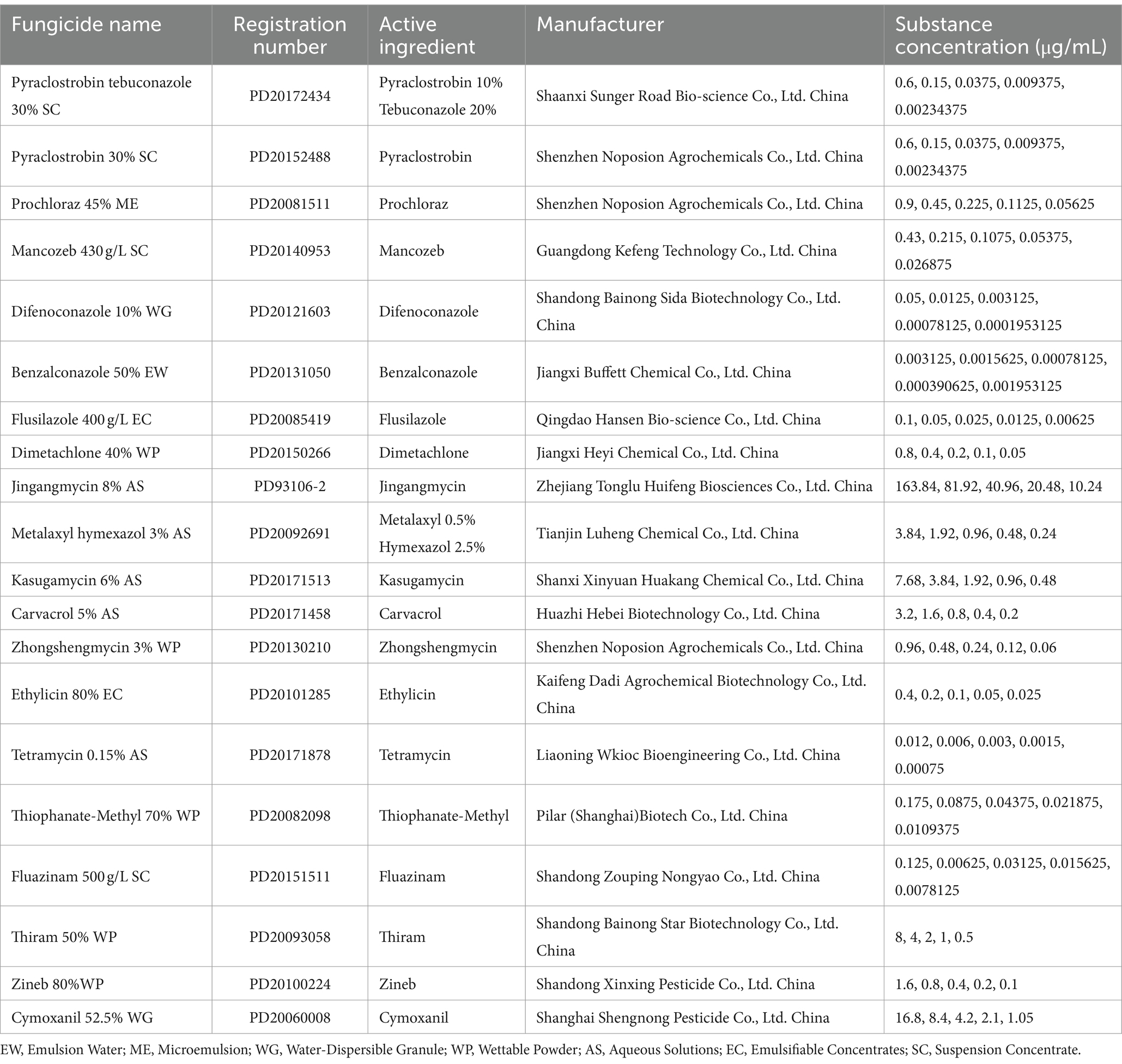
Table 1. Concentrations of substances used for fungicide sensitivity assays and their China pesticide registration numbers (http://www.chinapesticide.org.cn).
The EC50 (concentration for 50% of the maximum effect) of different fungicides was calculated using toxicity regression analysis with DPS V7.05 software (Pasche et al., 2004). Three replicates were established for each treatment group to ensure the reliability and reproducibility of the results.
2.9 Data analysis
Data were analyzed using one-way analysis of variance and a least significant difference test in SPSS 27.0 software (IBM Corp., United States), and GraphPad Prism 9.0 software was used to create figures.
3 Results
3.1 Morphological characterization
A total of 15 strains of Didymella-like fungi (Figure 1A) were successfully isolated from 10 diseased walnut leaves. Initially, the hyphae exhibited a grayish-white and the colonies had dense aerial hyphae. Over time, the colony became dark brown from the center outward, the colonies had woolly aerial hyphae, forming a distinct circular pattern, white to gray eventually (Figures 1B,C). Microscopic observation of three representative isolates revealed that the conidia were solitary, ellipsoidal, or obovate in shape, with rounded ends but varying sizes ranging from 4.2 to 6.8 μm × 2.5 to 3.1 μm (n = 50; Figures 1D,E). These morphological characteristics are consistent with the description of Didymella spp. (Chen et al., 2015).
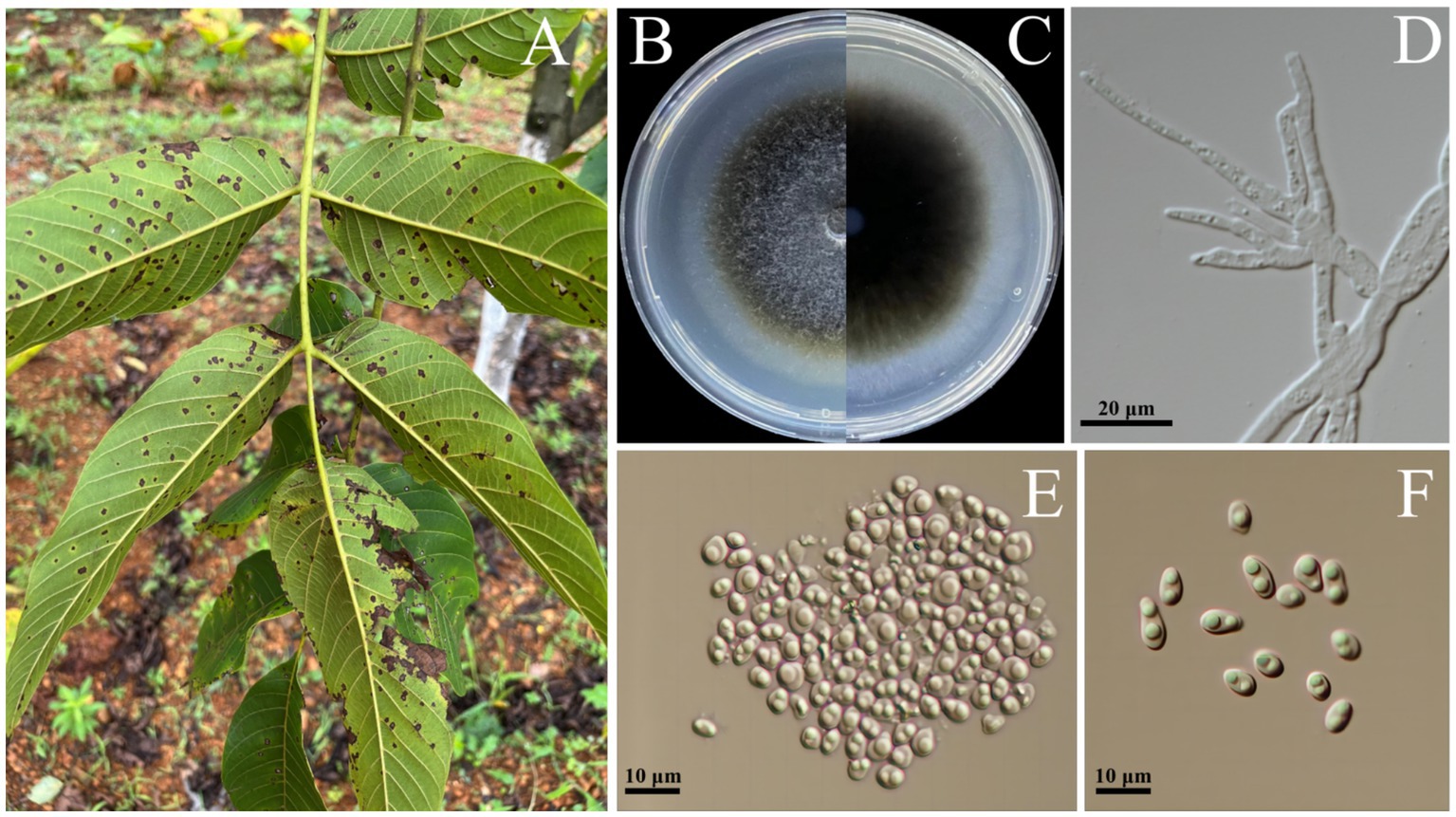
Figure 1. Leaf spot of walnut caused by Didymella segeticola. (A) Diseased leaf of walnut in field. (B,C) D. segeticola representative strain C27 colonies cultured on Potato Dextrose Agar medium for 12 days, front and back of Petri dish pictured. (D) Mycelium of D. segeticola C27 observed under the microscope. (E,F) Conidia of D. segeticola C27 observed under the microscope.
3.2 Molecular characterizations
The obtained DNA sequences were compared with existing sequences available in GenBank through alignment. BLAST analysis of the sequenced fragments revealed the closest match to D. segeticola sequences, with high levels of identity: 99.44% for ITS (PP159078.1); 97.52% for TUB (KP330399.1); and 100% for G3PD (MZ844660.1). DNA sequences of representative strains were deposited in GenBank as follows: C21 (ITS: PP564883, TUB: PP592363, G3PD: PP592360), C27 (ITS: PP526746, TUB: PP592364, G3PD: PP592361), and C29 (ITS: PP565363, TUB: PP592365, G3PD: PP592362). Further, utilizing the sequences of the ITS region, TUB, and G3PD genes, a phylogenetic tree was constructed for the three representative strains using MEGA 7.0 software and the proximity method (Figure 2). The results showed that strains C21, C27, and C29 were clustered with D. segeticola.
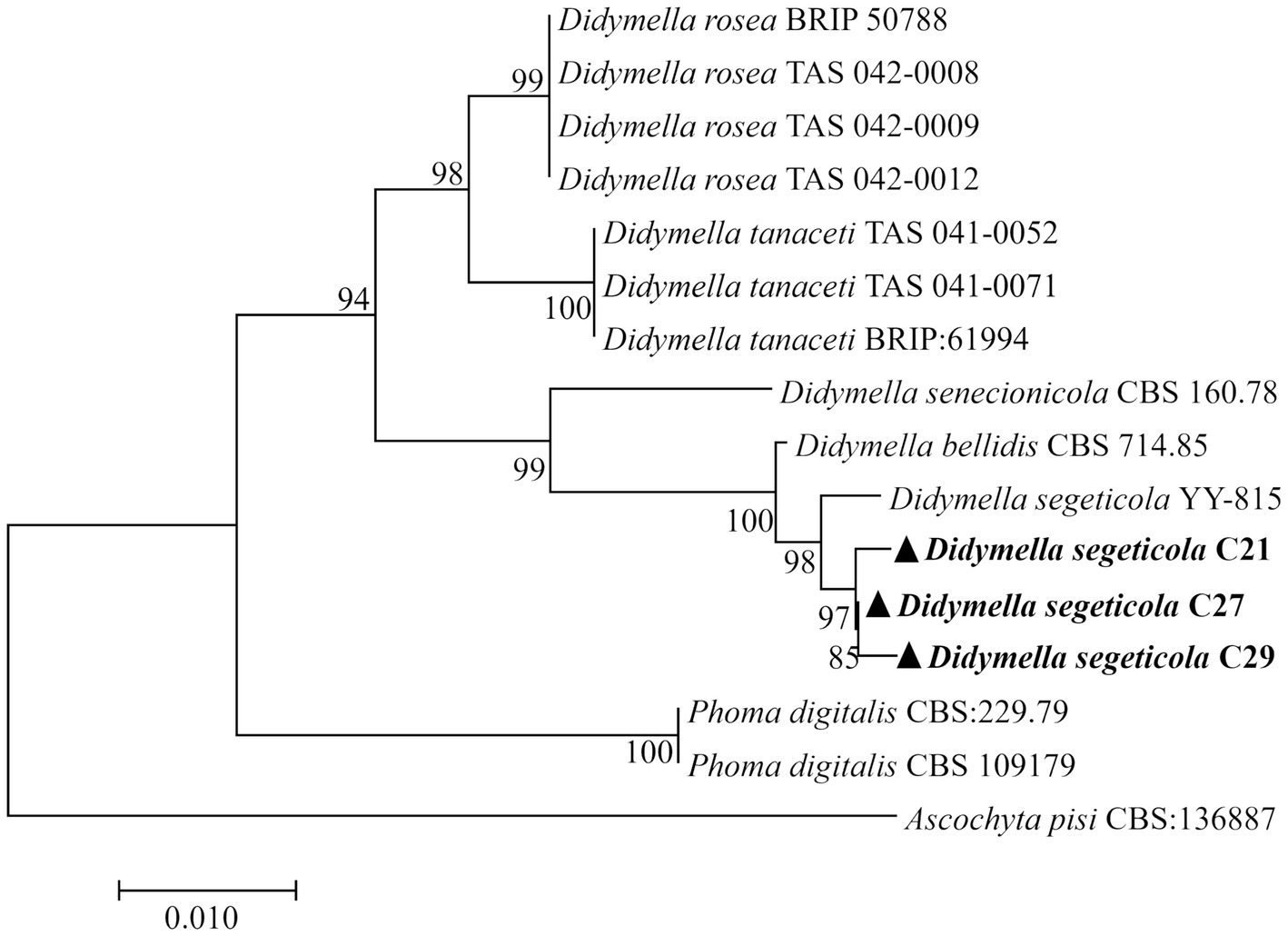
Figure 2. The neighbor-joining method (1,000 bootstrap iterations) was used to analyze concatenated sequences from the ITS region, β-tubulin, and glyceraldhyde-3-phosphate dehydrogenase genes from Didymella segeticola C21, D. segeticola C27 and D. segeticola C29 samples. Ascochyta pisi CBS:136887 was utilized as the outgroup. Bootstrap values are indicated next to the corresponding branches.
3.3 Pathogenicity test
Ten days post-inoculation, all of the healthy walnut leaves inoculated with the pathogen exhibited typical leaf spot symptoms (Figure 3), confirming the pathogenicity of the isolated strains. In contrast, the control leaves showed no disease symptoms. The pathogenic fungal were also successfully re-isolated from the inoculated leaves, confirming their role in causing the observed symptoms. Morphological characterization and molecular biology identification (utilizing ITS region, TUB, and G3PD gene sequences) confirmed that isolated strains C21, C27, and C29 belonged to the species D. segeticola. Subsequent experiments were conducted using pathogen C27 due to its consistent pathogenic behavior in the pathogenicity test.
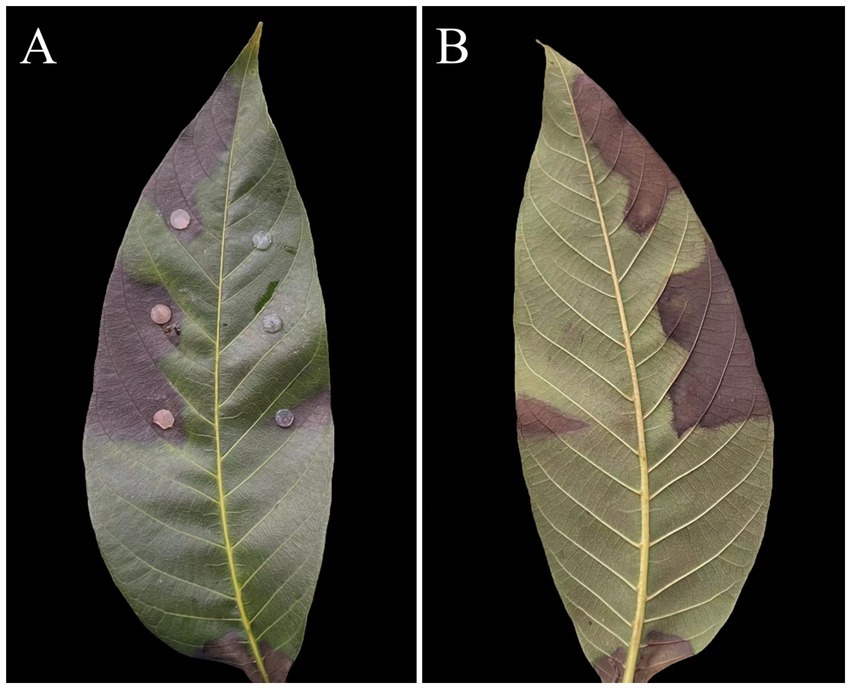
Figure 3. Symptoms of leaf spot caused by Didymella segeticola C27 used to inoculate walnut leaves: front side (A); reverse side (B).
3.4 Effects of temperature
Didymella segeticola C27 exhibited growth within a temperature range of 5–30°C. Notably, optimal growth conditions were observed at 20°C, with the highest mycelium growth rate recorded compared to other temperature treatments (Figure 4A). However, no mycelium or conidia growth was observed at 35°C. Regarding conidial production, the pathogen exhibited higher yields at 30°C compared to the other temperatures (Figure 4B). In addition, the conidia germination rate was highest at 30°C, exhibiting a significant difference compared to temperatures of 10, 15, 20, 25, and 35°C (Figure 4C).
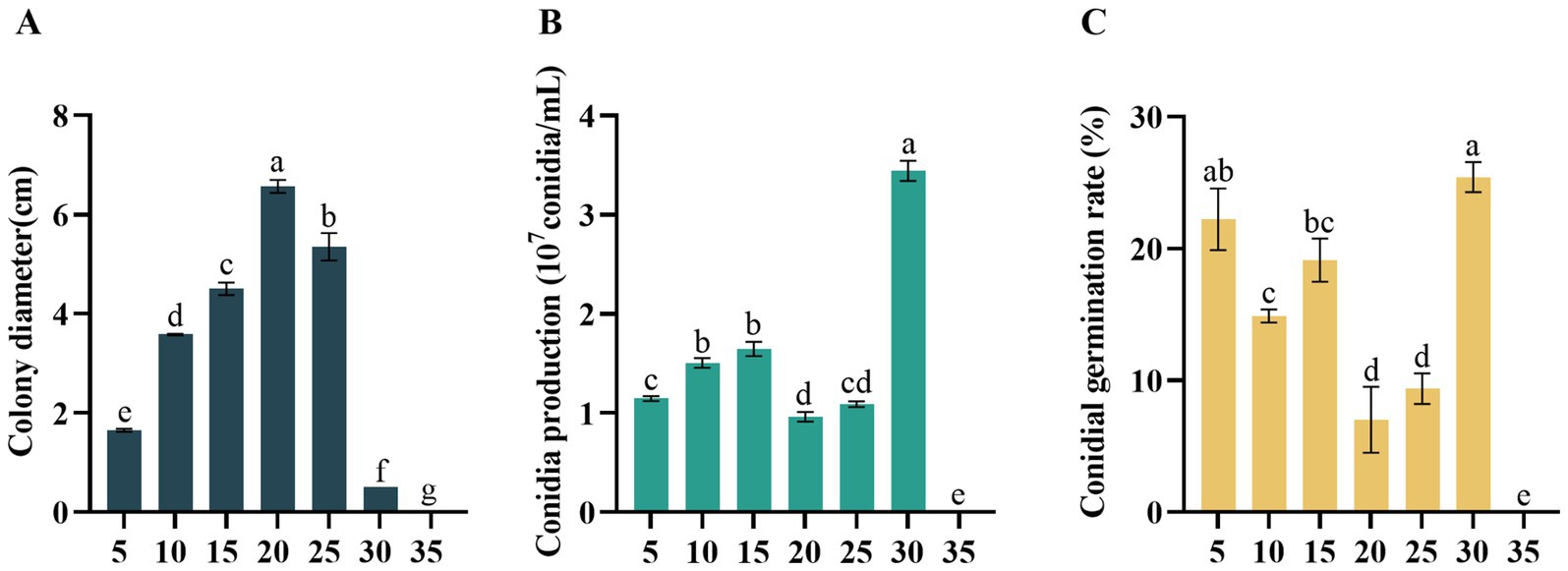
Figure 4. Effects of temperature (°C) on (A) mycelial growth, (B) conidia production, and (C) conidia germination rate of Didymella segeticola C27. Data are presented as the means ± standard error. Different lowercase letters indicate significant differences (p < 0.05).
3.5 Effects of photoperiod
Varying photoperiods had a discernible impact on the growth of D. segeticola C27. Specifically, the increase in mycelium growth was observed under photoperiods of 6/18 h, 14/10 h, and 18/6 h (Figure 5A). Notably, when the photoperiod was set to 6/18 h, the pathogen exhibited the highest number of conidia and the highest germination rate (Figures 5B,C). This photoperiod was particularly conducive to spore production and germination, and this effect was significantly different from the other treatment groups.
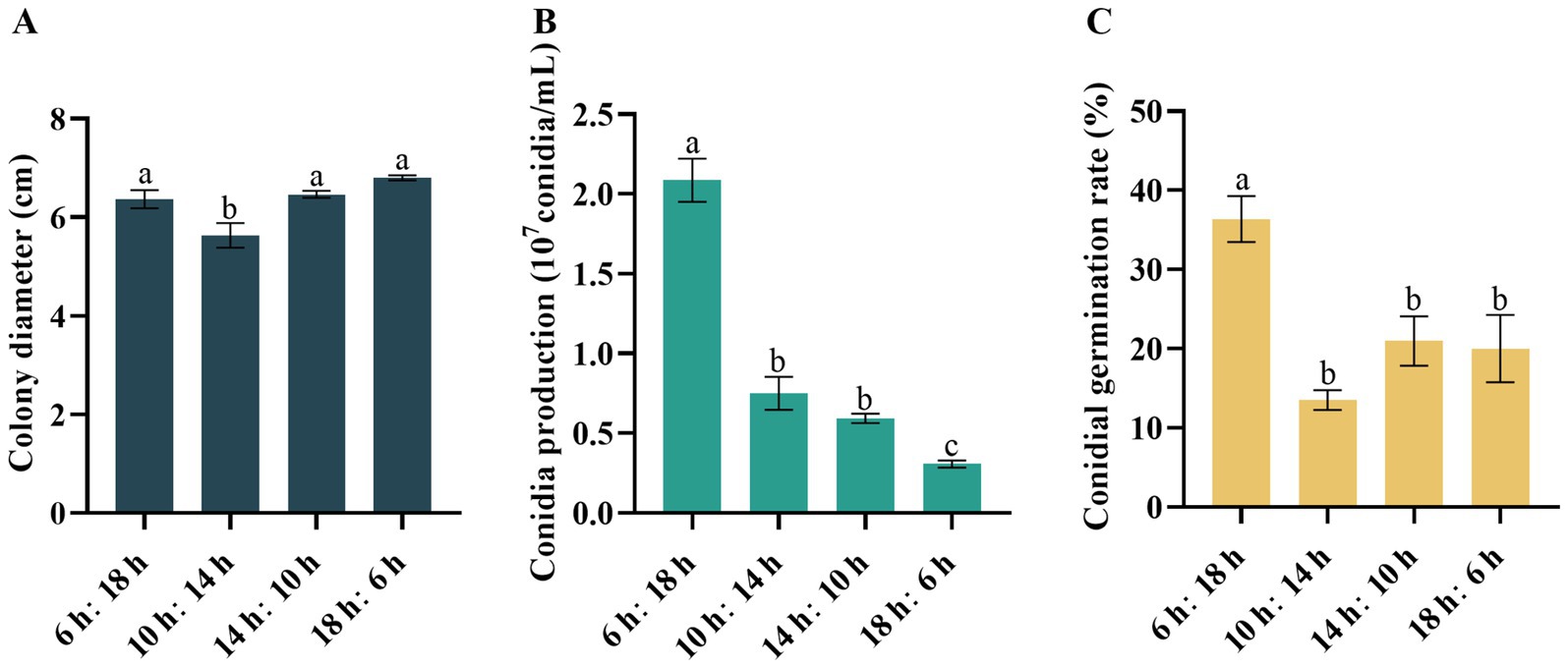
Figure 5. Effects of photoperiod on (A) mycelial growth, (B) conidia production, and (C) conidia germination rate of Didymella segeticola C27. Data are presented as the means ± standard error. Different lowercase letters indicate significant differences (p < 0.05).
3.6 Effects of carbon resource
All six of the carbon sources tested supported the growth, conidial sporulation, and conidia germination of the pathogenic fungal D. segeticola C27. However, maltose notably stood out as a carbon source, demonstrating significant promotion of both mycelial growth and conidia germination rates compared to other carbon sources (p < 0.05) (Figures 6A,C). Further, the utilization of lactose as a carbon source resulted in increased conidia production (Figure 6B).
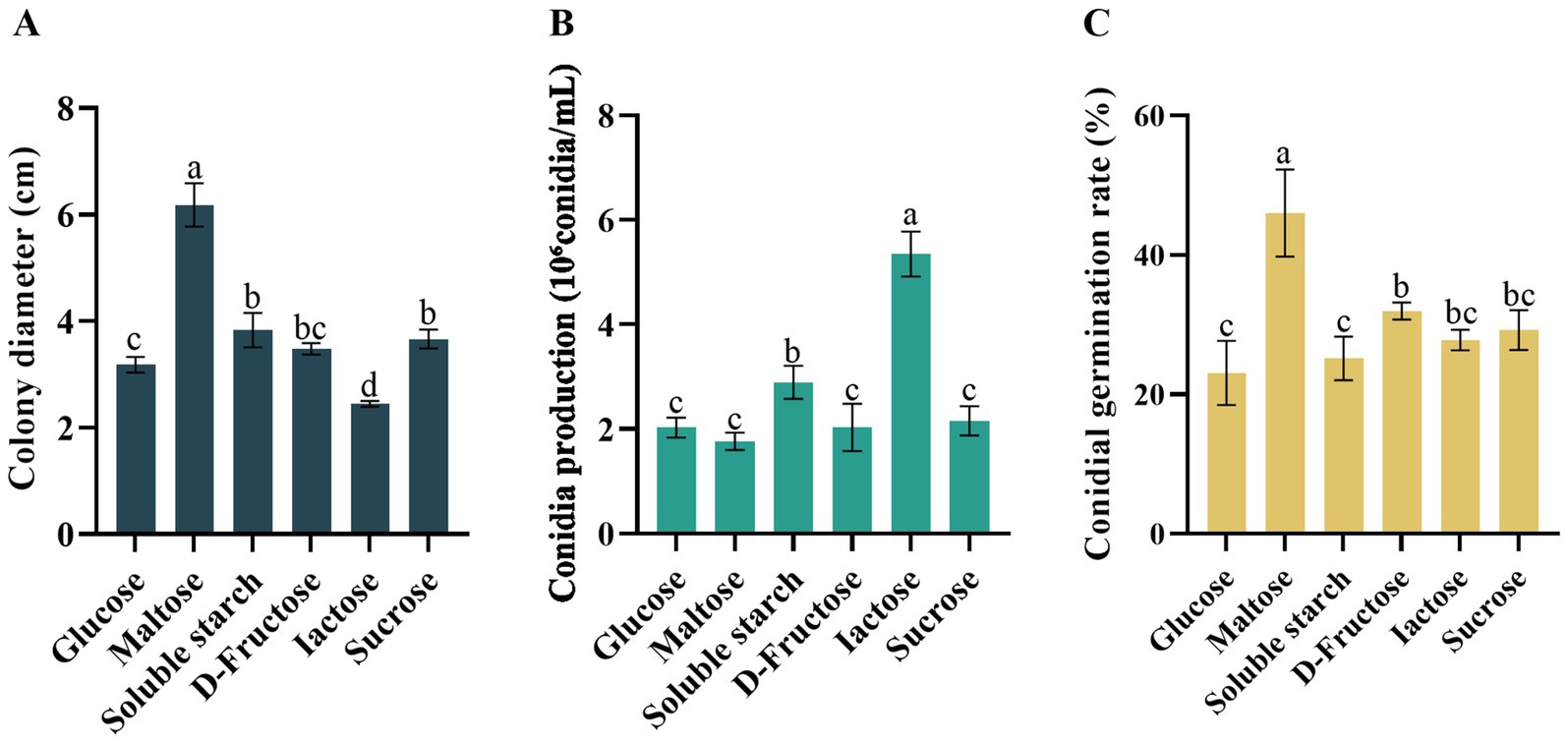
Figure 6. Effects of carbon resource on (A) mycelial growth, (B) conidia production, and (C) conidia germination rate of Didymella segeticola C27. Data are presented as the means ± standard error. Different lowercase letters indicate significant differences (p < 0.05).
3.7 Effects of nitrogen resource
The growth rate, conidia production, and conidia germination rate varied significantly under different nitrogen sources. Notably, the mycelium of D. segeticola C27 exhibited the fastest growth when beef extract was utilized as the nitrogen source, a difference that was significant compared to other nitrogen sources (p < 0.05) (Figure 7A). Further, the pathogen demonstrated enhanced conidia production when (NH4)2SO4 was employed as the nitrogen source (Figure 7B). In addition, the conidia germination rate was notably improved when the pathogen utilized NaNO3, beef extract, glycine, and urea as nitrogen sources, a difference that was statistically significant compared to (NH4)2SO4 and peptone nitrogen sources (p < 0.05) (Figure 7C).
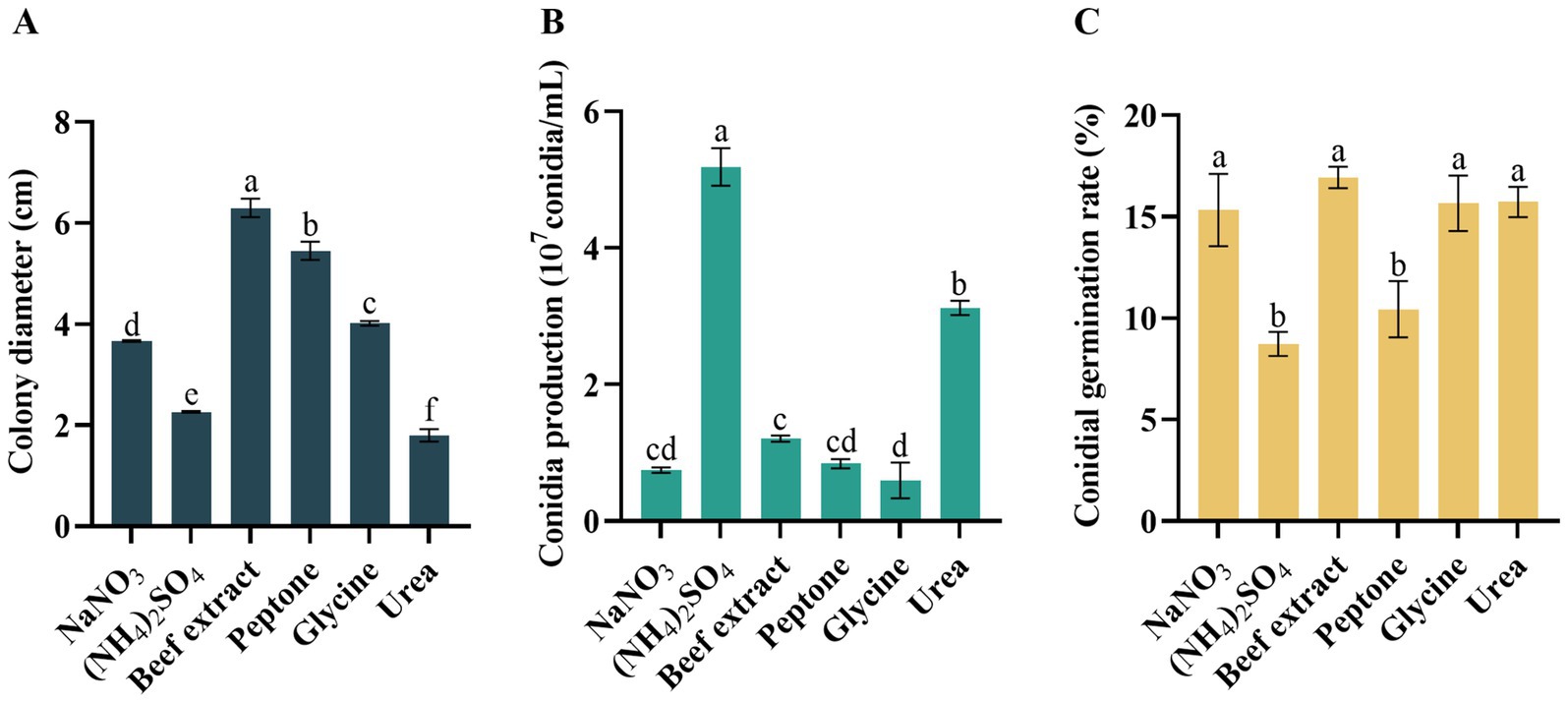
Figure 7. Effects of nitrogen resource on (A) mycelial growth, (B) conidia production, and (C) conidia germination rate of Didymella segeticola C27. Data are presented as the means ± standard error. Different lowercase letters indicate significant differences (p < 0.05).
3.8 Effect of different humidities
The findings revealed that under 90% humidity, the germination rate of D. segeticola C27 was highest. This observed germination rate was significantly higher compared to other humidity conditions (p < 0.05) (Figure 8).
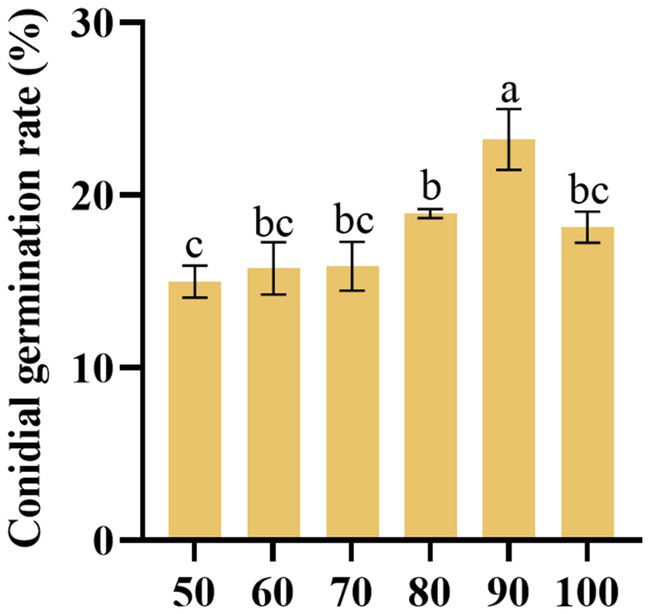
Figure 8. Effects of humidity (%) on the conidia germination rate of Didymella segeticola C27. Data are presented as the means ± standard error. Different lowercase letters indicate significant differences (p < 0.05).
3.9 Fungicide assays
The sensitivity of the representative pathogen D. segeticola C27 to 20 selected fungicides was assessed (Table 2). All of the tested fungicides demonstrated inhibition of the mycelial growth of D. segeticola C27, as shown in Supplementary Figure S1. Among the 20 tested fungicides, the EC50 values of 16 fungicides were < 1 μg/mL. Difenoconazole had the best inhibitory effect, with an EC50 value of 0.0007 μg/mL, followed by tetramycin with an EC50 value of 0.0009 μg/mL. In addition, benzalconazole, flusilazole, pyraclostrobin tebuconazole, fluazinam, pyraclostrobin, prochloraz, ethylicin, mancozeb, thiophanate-methyl, dimetachlone, carvacrol, zineb, zhongshengmycin, and hymexazol also exhibited effective inhibitory effects against D. segeticola C27, with EC50 values ranging from 0.0036 to 0.6607 μg/mL. In contrast, kasugamycin, thiram, cymoxanil, kasugamycin, and jingangmycin displayed EC50 values larger than 1 μg/mL, with EC50 values of 1.3270, 1.6192, 7.2928, and 41.3217 μg/mL, respectively.
4 Discussion
Walnut leaf spot stands out as a significant challenge in walnut cultivation, exerting detrimental effects on yield and quality (Wang F. H. et al., 2023). Prior investigations have underscored the havoc wreaked by Didymella bryoniae, inducing vine blight in melons and leading to substantial yield losses in 2004 (Sudisha et al., 2004). Similarly, Phoma segeticola was implicated in leaf spot disease in Tibetan thistle in 2015 (Chen et al., 2015), while D. segeticola was found to be a causal agent of leaf spot disease in tobacco, impairing tobacco quality (Guo et al., 2020). Further, its involvement in leaf spot disease in Zanthoxylum bungeanum, resulting in extensive leaf shedding and affecting seed yield and quality, was documented in 2022 (Yang et al., 2022). This study successfully identified the causal agent responsible for walnut leaf spot through comprehensive morphological, molecular, and pathogenicity assessments conducted in Hezhang County, Guizhou Province, China. To our knowledge, this is the first report of D. segeticola as the causal agent behind walnut leaf spot, providing crucial insights for policymakers to devise targeted control strategies.
It is pivotal to clarify the biological characteristics of plant pathogens for devising effective disease control strategies (Fan R. D. et al., 2023). Our study unveiled crucial insights into the biology of the walnut leaf spot fungus, D. segeticola, revealing its temperature and photoperiod preferences and its utilization of different carbon and nitrogen sources. Notably, we found that D. segeticola mycelium thrives within a temperature range of 5–30°C, with optimal growth observed between 20 and 25°C, aligning with prior findings in tobacco leaf spot disease (Wang H. C. et al., 2023). Further, maximal mycelial spore production was observed at 30°C, while the highest conidia germination rates were recorded at 5 and 30°C. Consistent with physiological analyses of tea leaf spot disease in Guizhou, we observed that D. segeticola failed to grow at 35°C (Cheng et al., 2022). Thus, for future walnut breeding, it is necessary to cultivate or select cultivars with high resistance to high temperature.
Photoperiod is an important environmental factor influencing the biological characteristics and pathogenesis of plant pathogens (Costa et al., 2021; Macioszek et al., 2021). In our study, we observed the fastest growth of pathogenic mycelium under photoperiods of 6:18 h (light: dark), 14:12 h, and 18:6 h, contrasting significantly with the 10:14 h photoperiod. Specifically, the 6:18 h photoperiod facilitated maximal spore production and germination rates, differing significantly from other photoperiod conditions. Hence, we suggest that the planting time of walnuts should be adjusted when planting walnuts in Guizhou, China.
Carbon source availability significantly impacts pathogen growth and spore germination. Our findings highlight maltose as the optimal carbon source, fostering the fastest mycelial growth and highest spore germination rates compared to other carbon sources, echoing results from research on tea spot caused by D. segeticola in Guizhou Province of China (Cheng et al., 2022). In addition, we observed maximal spore production when lactose served as the carbon source. Regarding nitrogen sources, beef extract emerged as the most conducive to mycelial growth, significantly differing from other nitrogen sources, in contrast to results observed in tea leaf spot caused by Didymella bellidis (Wu et al., 2023). Notably, (NH4)2SO4 supplementation resulted in the highest conidia production by pathogen C27, indicating its ability to thrive on diverse nitrogen sources. Moreover, NaNO3, beef extract, glycine, and urea resulted in the highest conidia germination rates, significantly differing from ammonium sulfate and peptone.
In addition, our study demonstrated that a humidity level of 90% facilitated the highest germination rate of pathogenic conidia, similar to the local humidity conditions in Bijie City, Guizhou Province, China, as reported by the China Meteorological Data Network. By monitoring the environmental humidity, an optimal drainage system can be established to prevent the soil from becoming excessively moist, and chemical agents under high humidity can also be utilized to effectively prevent and control diseases. These findings underscore the intricate interplay between environmental factors and the biology of D. segeticola, offering insights into tailored disease management strategies. The occurrences of plant disease caused by pathogenic fungi are closely related to the nutritional and environmental factors that when they providing feasible conditions for the pathogens to grow and spread (Tang et al., 2022). On the opposite, regulating nutritional and environmental conditions to establish the conditions that could adversely affect the growth of pathogens, may reduce the development and severity of plant disease (Fan K. et al., 2023). Therefore, these findings in our study on the new walnut leaf spot causal agent, Didymella bryoniae, could give new insights into the occurrences mechanisms of the disease, as well as provide biological and ecological basis for its effective control.
Chemical control remains the primary method for controlling fungal diseases, particularly those caused by the genus Subspora (Zhan et al., 2024). However, there are few studies on preventing and treating diseases caused by D. segeticola. Therefore, our study focused on screening fungicides against D. segeticola C27 in vitro. Among the 20 fungicides tested, comprising 14 chemical and 6 biological fungicides, the EC50 values of 3 fungicides were ≤ 0.01 μg/mL, while those of 16 fungicides were ≤ 1 μg/mL. The indoor toxicity test results revealed that difenoconazole was the most effective in controlling D. segeticola, with an EC50 value of 0.0007 μg/mL, deviating from prior research results, possibly due to differing hosts (Yang et al., 2022). Tetracycline followed with an EC50 value of 0.0009 μg/mL. Tetracycline is notable for its broad activity spectrum and low toxicity and is known to enhance plant disease resistance by inducing phenylalanine ammonia lyase (PAL), peroxidase (POD), and polyphenol oxidase (PPO) activities (Gao et al., 2018; Ma et al., 2018; Alqahtani et al., 2022). In addition, difenoconazole propiconazol demonstrated effective control, with an EC50 value of 0.0036 ug/mL. Notably, triazole fungicides effectively inhibited pathogenic fungal growth by interfering with ergosterol demethylation on the cell membrane, impeding membrane formation, and restraining pathogen growth (Vazquez et al., 2022). Conversely, jingangmycin exhibited weak inhibitory activity against D. segeticola C27, with an EC50 value of 41.3217 μg/mL. Nevertheless, prolonged and extensive use of chemical fungicides can adversely impact the ecological environment and food safety (Chen X. Y. L. et al., 2021). Hence, alternating between chemical and biological fungicides during control processes can mitigate pathogen resistance.
In summary, our study elucidated the biological characteristics, pathology, and effect fungicides for a walnut leaf spot pathogen in Guizhou Province of China. These findings could provide a foundation for the sustainable and effective management of walnut leaf spot caused by D. segeticola in Guizhou Province, facilitating informed decision-making for future disease control strategies.
Data availability statement
The datasets presented in this study can be found in online repositories. The names of the repository/repositories and accession number(s) can be found in the article/Supplementary material.
Author contributions
XA: Data curation, Investigation, Writing – original draft. TS: Investigation, Methodology, Writing – original draft. WY: Funding acquisition, Project administration, Supervision, Writing – review & editing. HO: Formal analysis, Investigation, Writing – original draft. RF: Formal analysis, Investigation, Writing – original draft. JS: Investigation, Writing – original draft. CW: Formal analysis, Investigation, Writing – original draft. ZL: Investigation, Writing – original draft. SD: Investigation, Writing – original draft. XC: Conceptualization, Resources, Writing – review & editing.
Funding
The author(s) declare that financial support was received for the research, authorship, and/or publication of this article. This study was supported by Program for Forestry Science and Technology Research of Guizhou Province (QLKH[2021]04), Program of Excellent Innovation Talents in Guizhou Province (GCC[2023]071), Program for Natural Science Research in Guizhou Education Department (QJJ-[2023]-024), Program of Postgraduate Research Fund in Guiyang University (GYU-YJS-SH[2022]-08), and Discipline and Master’s Site Construction Project of Guiyang University by Guiyang City Financial Support Guiyang University (2022-xk12).
Acknowledgments
The authors gratefully acknowledge Zeirui Feng, Huliang Teng, and Benlin Yi for laboratory assistant work.
Conflict of interest
The authors declare that the research was conducted in the absence of any commercial or financial relationships that could be construed as a potential conflict of interest.
Publisher’s note
All claims expressed in this article are solely those of the authors and do not necessarily represent those of their affiliated organizations, or those of the publisher, the editors and the reviewers. Any product that may be evaluated in this article, or claim that may be made by its manufacturer, is not guaranteed or endorsed by the publisher.
Supplementary material
The Supplementary material for this article can be found online at: https://www.frontiersin.org/articles/10.3389/fmicb.2024.1439487/full#supplementary-material
References
Ahmadi, S., Harighi, B., and Abdollahzadeh, J. (2017). Phylogenetic relationships of fluorescent pseudomonad isolates associated with bacterial canker of stone fruit trees in Kurdistan Province, Iran. Eur. J. Plant Pathol. 150, 679–689. doi: 10.1007/s10658-017-1316-4
Alqahtani, A., Marrez, D. A., Aleraky, M., Fagir, N. A., Alqahtani, O., Othman, S., et al. (2022). Characterization and isolation of the major biologically active metabolites isolated from ficus retusa and their synergistic effect with tetracycline against certain pathogenic-resistant bacteria. Pharmaceuticals Basel. 15:1473. doi: 10.3390/ph15121473
Baumgartnerová, H. (1996). First findings of plum pox virus in walnut trees (Juglans Regia L.). Acta Virol. 40, 59–60.
Bayazit, S., Kazan, K., Gülbitti, S., Cevik, V., Ayanoğlu, H., and Ergül, A. (2007). AFLP analysis of genetic diversity in low chill requiring walnut (Juglans regia L.) genotypes from Hatay, Turkey. Sci. Hortic. 111, 394–398. doi: 10.1016/j.scienta.2006.11.006
Blomhoff, R., Carlsen, M. H., Andersen, L. F., and Jacobs, D. R. Jr. (2006). Health benefits of nuts: potential role of antioxidants. Br. J. Nutr. 96, S52–S60. doi: 10.1017/bjn20061864
Cai, F. F., Yang, C. D., Ma, T., Jin, M. J., and Cui, L. X. (2021). First report of Boeremia exigua var. exigua causing branch blight on walnut in China. Plant Dis. 105:3291. doi: 10.1094/PDIS-02-21-0382-PDN
Cao, W. P., Zhen, W., Chen, D., Feng, S., and Song, J. (2022). Influence of plant growth regulators on sporulation and conidia characteristics of the entomopathogenic fungus Beauveria bassiana. Chin. J. Biol. Control. 38, 555–564. doi: 10.16409/j.cnki.2095-039x.2021.05.022
Carbone, I., and Kohn, L. M. (1999). A method for designing primer sets for speciation studies in filamentous ascomycetes. Mycologia 91, 553–556. doi: 10.1080/00275514.1999.12061051
Charkhabi, N. F., Shams-Bakhsh, M., and Rahimian, H. (2010). Genetic diversity among Brenneria nigrifluens strains in Iran. Eur. J. Plant Pathol. 128, 303–310. doi: 10.1007/s10658-010-9667-0
Chen, S. F., Fichtner, E., Morgan, D. P., and Michailides, T. J. (2013). First report of Lasiodiplodia citricola and Neoscytalidium dimidiatum causing death of graft union of English walnut in California. Plant Dis. 97:993. doi: 10.1094/PDIS-10-12-1000-PDN
Chen, X. Y. L., Wicaksono, W. A., Berg, G., and Cernava, T. (2021). Bacterial communities in the plant phyllosphere harbour distinct responders to a broad-spectrum pesticide. Sci. Total Environ. 751:141799. doi: 10.1016/j.scitotenv.2020.141799
Chen, Y., Yuan, S. Y., Liang, W. K., Li, Z. L., Liu, J. X., Zhang, H. L., et al. (2021). Pathogen identification and fungicides screening for grey spot of Syzygium samarangense. Chin. Plant Prot. 41, 24–29. doi: 10.3969/j.issn.1672-6820.2021.10.004
Chen, Q., Zhang, K. L., Zhang, G. Z., and Cai, L. (2015). A polyphasic approach to characterise two novel species of Phoma (Didymellaceae) from China. Phytotaxa. 197, 267–281. doi: 10.11646/phytotaxa.197.4.4
Cheng, Y. H., Huang, X. Z., Zhang, J. F., Meng, Z. H., Lu, S. J., Li, S., et al. (2022). Phylogenetic analysis and biological characteristics of tea leaf spot disease in Guizhou. Mol. Plant Breed. 20, 4468–4476. doi: 10.13271/j.mpb.020.004468
Costa, T. P. C., Rodrigues, E. M., Dias, L. P., Pupin, B., Ferreira, P. C., and Rangel, D. E. N. (2021). Different wavelengths of visible light influence the conidial production and tolerance to ultra-violet radiation of the plant pathogens Colletotrichum acutatum and fusarium fujikuroi. Eur. J. Plant Pathol. 159, 105–115. doi: 10.1007/s10658-020-02146-y
Deng, Y. Z., Qu, Z. W., He, Y. L., and Naqvi, N. I. (2012). Sorting nexin Snx41 is essential for conidiation and mediates glutathione-based antioxidant defense during invasive growth in Magnaporthe oryzae. Autophagy 8, 1058–1070. doi: 10.4161/auto.20217
Dreher, M. L., Maher, C. V., and Kearney, P. (1996). The traditional and emerging role of nuts in healthful diets. Nutr. Rev. 54, 241–245. doi: 10.1111/j.1753-4887.1996.tb03941.x
Fan, R. D., Liu, Y. J., Bin, Y. L., Huang, J. Y., Yi, B. L., Tang, X. L., et al. (2023). Identification of Colletotrichum aenigma as the new causal agent of leaf blight disease on Aucuba japonica Thunb., and screenings of effective fungicides for its sustainable management. Front. Microbiol. 14:1222844. doi: 10.3389/fmicb.2023.1222844
Fan, K., Qi, Y. K., Fu, L., Li, L., Liu, X. H., Qu, J. L., et al. (2023). Identification and fungicide screening of fungal species associated with walnut anthracnose in Shaanxi and Liaoning provinces, China. Plant Dis. 108, 599–607. doi: 10.1094/PDIS-05-23-0967-RE
Fordos, S., Abid, N., Gulzar, M., Pasha, I., Oz, F., Shahid, A., et al. (2023). Recent development in the application of walnut processing by-products (walnut shell and walnut husk). Biomass Conv. Bioref. 13, 14389–14411. doi: 10.1007/s13399-023-04778-6
Gao, Y. Y., He, L. F., Li, X. X., Lin, J., Mu, W., and Liu, F. (2018). Toxicity and biochemical action of the antibiotic fungicide tetramycin on Colletotrichum scovillei. Pestic Biochem. Physiol. 147, 51–58. doi: 10.1016/j.pestbp.2018.02.012
Glenn, A. J., Aune, D., Freisling, H., Mohammadifard, N., Kendall, C. W. C., Salas-Salvadó, J., et al. (2023). Nuts and cardiovascular disease outcomes: a review of the evidence and future directions. Nutrients 15:911. doi: 10.3390/nu15040911
Guo, Z. N., Xie, H. L., Wang, H. C., Huang, Y., Chen, Q. L., Xiang, L. G., et al. (2020). Leaf spot caused by Didymella segeticola on tobacco in China. Plant Dis. 104, 1559–1560. doi: 10.1094/pdis-11-19-2398-pdn
Hama, J. R., and Fitzsimmons-Thoss, V. (2022). Determination of unsaturated fatty acids composition in walnut (Juglans regia L.) oil using NMR spectroscopy. Food Anal. Methods 15, 1226–1236. doi: 10.1007/s12161-021-02203-0
He, L. F., Li, X. X., Gao, Y. Y., Li, B. X., Mu, W., and Liu, F. (2019). Characterization and fungicide sensitivity of Colletotrichum spp. from different hosts in Shandong, China. Plant Dis. 103, 34–43. doi: 10.1094/PDIS-04-18-0597-RE
Hu, J. N., Chang, R. K., Yuan, Y. J., Li, Z. R., and Wang, Y. H. (2022). Identification of key residues essential for the activation of plant immunity by subtilisin from Bacillus velezensis LJ02. Front. Microbiol. 13:869596. doi: 10.3389/fmicb.2022.869596
Jahanban-Esfahlan, A., Ostadrahimi, A., Tabibiazar, M., and Amarowicz, R. (2019). A comparative review on the extraction, antioxidant content and antioxidant potential of different parts of walnut (Juglans regia L.) fruit and tree. Molecules 24:2133. doi: 10.3390/molecules24112133
Keshtkar, A. R., Khodakaramian, G., and Rouhrazi, K. (2016). Isolation and characterization of Pseudomonas syringae pv. Syringae which induce leaf spot on walnut. Eur. J. Plant Pathol. 146, 837–846. doi: 10.1007/s10658-016-0962-2
Kim, H. S., Cheon, W., Lee, Y., Kwon, H. T., Seo, S. T., Balaraju, K., et al. (2021). Identification and characterization of Xanthomonas arboricola pv. Juglandis causing bacterial blight of walnuts in Korea. Plant Pathol. J. 37, 137–151. doi: 10.5423/PPJ.OA.12.2020.0217
Kumar, S., Stecher, G., and Tamura, K. (2016). MEGA7: molecular evolutionary genetics analysis version 7.0 for bigger datasets. Mol. Biol. Evol. 33, 1870–1874. doi: 10.1093/molbev/msw054
Li, F. X., Chen, J. W., Chen, Q., Liu, Z. Y., Sun, J. Y., Yan, Y. T., et al. (2023). Identification, pathogenicity, and sensitivity to fungicide of Colletotrichum species that causes walnut anthracnose in Beijing. Agronomy 13:214. doi: 10.3390/agronomy13010214
Ma, D. C., Zhu, J. M., Jiang, J. G., Zhao, Y. H., Li, B. X., Mu, W., et al. (2018). Evaluation of bioactivity and control efficacy of tetramycin against Corynespora cassiicola. Pestic. Biochem. Physiol. 152, 106–113. doi: 10.1016/j.pestbp.2018.09.009
Macioszek, V. K., Sobczak, M., Skoczowski, A., Oliwa, J., Michlewska, S., Gapi’nska, M. A., et al. (2021). The effect of photoperiod on necrosis development, photosynthetic efficiency and ‘Green Islands’ formation in Brassica juncea infected with Alternaria brassicicola. Int. J. Mol. Sci. 22:8435. doi: 10.3390/ijms22168435
Meng, L., Yu, C. L., Wang, C., and Li, G. (2018). First report of Diaporthe amygdali causing walnut twig canker in Shandong Province of China. Plant Dis. 102:1859. doi: 10.1094/pdis-01-18-0192-pdn
Ozturk, M. O., Sipahioğlu, H. M., Ocak, M., and Usta, M. (2008). Cherry leafroll virus in Juglans regia in the Lake Van basin of Turkey. J. Plant Pathol. 90, 75–79. doi: 10.4454/jpp.v90i1.594
Pasche, J. S., Wharam, C. M., and Gudmestad, N. C. (2004). Shift in sensitivity of Alternaria solani in response to QoI fungicides. Plant Dis. 88, 181–187. doi: 10.1094/PDIS.2004.88.2.181
Razinataj, M., Aeini, M., Khademlou, A., and Sadeghi, K. (2020). Isolation and characterization of Brenneria nigrifluens causing bacterial shallow bark canker of walnut trees in Golestan Province, Iran. J. Genet. Resour. 6, 148–156. doi: 10.22080/jgr.2020.18940.1188
Sagawa, C. H. D., Assis, R. D. B., Zaini, P. A., Wilmarth, P. A., Phinney, B. S., Moreira, L. M., et al. (2020). Proteome analysis of walnut bacterial blight disease. Int. J. Mol. Sci. 21:7453. doi: 10.3390/ijms21207453
Salejda, A. M., Janiewicz, U., Korzeniowska, M., Kolniak-Ostek, J., and Krasnowska, G. (2016). Effect of walnut green husk addition on some quality properties of cooked sausages. Lwt-Food Sci. Technol. 65, 751–757. doi: 10.1016/j.lwt.2015.08.069
Sudisha, J., Kumar, T. V., Niranjana, S. R., and Shetty, H. S. (2004). First report of gummy stem blight caused by Didymella bryoniae on muskmelon (Cucumis melo) in India. Plant Pathol. 53:533. doi: 10.1111/j.1365-3059.2004.01031.x
Tang, X. L., Yangjing, G., Zhuoma, G., Guo, X. F., Cao, P. X., Yi, B. L., et al. (2022). Biological characterization and in vitro fungicide screenings of a new causal agent of wheat fusarium head blight in Tibet, China. Front. Microbiol. 13:941734. doi: 10.3389/fmicb.2022.941734
Tapsell, L. C., Gillen, L. J., Patch, C. S., Batterham, M., Owen, A., Baré, M., et al. (2004). Including walnuts in a low-fat/modified-fat diet improves HDL cholesterol-to-total cholesterol ratios in patients with type 2 diabetes. Diabetes Care 27, 2777–2783. doi: 10.2337/diacare.27.12.2777
Templeton, M. D., Rikkerink, E. H., Solon, S. L., and Crowhurst, R. N. (1992). Cloning and molecular characterization of the glyceraldehyde-3-phosphate dehydrogenase-encoding gene and cDNA from the plant pathogenic fungus Glomerella cingulata. Gene 122, 225–230. doi: 10.1016/0378-1119(92)90055-t
Usman, H. M., Tan, Q., Fan, F., Karim, M. M., Yin, W. X., Zhu, F. X., et al. (2022). Sensitivity of Colletotrichum nymphaeae to six fungicides and characterization of fludioxonil-resistant isolates in China. Plant Dis. 106, 165–173. doi: 10.1094/PDIS-05-21-0993-RE
Vahdati, K., Arab, M. M., Sarikhani, S., Sadat-Hosseini, M., Leslie, C. A., and Brown, P. J. (2019). “Advances in Persian walnut (Juglans regia L.) breeding strategies” in Advances in plant breeding strategies: Nut and beverage crops, vol. 4. eds. J. Al-Khayri, S. Jain, and D. Johnson (Springer), 401–472.
Vazquez, A. E. G., Hockemeyer, K., McConville, M., Remucal, C. K., and Koch, P. L. (2022). Assessment of temperature and time following application as predictors of propiconazole translocation in Agrostis stolonifera. J. Agric. Sci. Technol. 2, 592–602. doi: 10.1021/acsagscitech.2c00022
Wang, F. F., Dun, C. Y., Tang, T., Duan, Y. Y., Guo, X. L., and You, J. M. (2022). Boeremia exigua causes leaf spot of walnut trees (Juglans regia) in China. Plant Dis. 106:1993. doi: 10.1094/PDIS-10-21-2304-PDN
Wang, Q. H., Fan, K., Li, D. W., Han, C. M., Qu, Y. Y., Qi, Y. K., et al. (2020). Identification, virulence and fungicide sensitivity of Colletotrichum gloeosporioides s.s. responsible for walnut anthracnose disease in China. Plant Dis. 104, 1358–1368. doi: 10.1094/PDIS-12-19-2569-RE
Wang, Q. H., Fan, K., Li, D. W., Niu, S. G., Hou, L. Q., and Wu, X. Q. (2017). Walnut anthracnose caused by Colletotrichum siamense in China. Australas. Plant Pathol. 46, 585–595. doi: 10.1007/s13313-017-0525-9
Wang, H. C., Huang, Y., Huang, Y., Cai, L. T., Meng, J. Y., Song, G. L., et al. (2023). Identification and characterization of the pathogen Didymella segeticola causing tobacco leaf spot. J. Plant Protect. Res. 50, 757–766. doi: 10.13802/j.cnki.zwbhxb.2023.2021171
Wang, F. H., Liu, C., Zeng, Q., Zhou, Y. J., Liu, F., Xu, X. L., et al. (2023). Identification and pathogenicity analysis of leaf brown spot of Juglans regia in China. Sci. Rep. 13:6599. doi: 10.1038/s41598-023-33853-1
Wang, H., Wang, R. J., Tian, F. M., Zhao, S. L., He, J. J., Li, Z. H., et al. (2016). Identification and pathogenicity assay of pathogen of blight disease on walnut trees in Longnan. Fujian J. Agric. Sci. 31, 1086–1090. doi: 10.19303/j.issn.1008-0384.2016.10.016
White, T. J., Bruns, T., Lee, S., J., W. T., and Taylor, J. (1990). “Amplification and direct sequencing of fungal ribosomal RNA genes for phylogenetics,” in PCR protocols: A guide to methods and applications. eds. M. A. Innis, D. H. Gelfand, J. Sninsky, and T. J. White (New York), 315–322.
Wu, S., Yang, Z. Y., Yang, R., Zhang, Y., Dharmasena, D. S. P., Jiang, S. L., et al. (2023). Identification and growth characteristics of tea leaf spot pathogenic. J. Mt. Agric. Biol. 42, 10–17. doi: 10.15958/j.cnki.sdnyswxb.2023.04.002
Xin, W. J., Mao, Y. S., Lu, F., Li, T., Wang, J. X., Duan, Y. B., et al. (2020). In vitro fungicidal activity and in planta control efficacy of coumoxystrobin against Magnaporthe oryzae. Pestic. Biochem. Physiol. 162, 78–85. doi: 10.1016/j.pestbp.2019.09.004
Xu, X. L., Huang, Q. Y., Gao, L. P., Chen, F., Wang, B. X., Zeng, Z., et al. (2023). Leaf spot disease on Juglans regia caused by Ragnhildiana diffusa in China. Plant Dis. 107:2524. doi: 10.1094/PDIS-02-23-0205-PDN
Yang, J. Z., Chen, C. X., Yin, X. H., Xu, H. Y., Long, H. J., Gu, G. F., et al. (2022). Didymella Segeticola is a new pathogen causing leaf spot disease on Zanthoxylum bungeanum. N. Z. J. Crop Hortic. 51, 694–703. doi: 10.1080/01140671.2022.2080235
Zhan, H. X., Yang, Y. F., Song, W. T., Tom, H., Liu, L. P., and Gao, J. (2024). Advances in knowledge of fungi in the genus Didymella. J. Fungi. 22, 1–33. doi: 10.13341/j.jfr.2024.7888
Keywords: Juglans regia, leaf spot, Didymella segeticola, biological characterization, fungicides screening
Citation: Ao X, Shi T, Yang W, Ouyang H, Fan R, Siddiqui JA, Wu C, Lv Z, Deng S and Chen X (2024) Biological characterization and in vitro fungicide screening of a new causal agent of walnut leaf spot in Guizhou Province, China. Front. Microbiol. 15:1439487. doi: 10.3389/fmicb.2024.1439487
Edited by:
Ravinder Kumar, Indian Agricultural Research Institute (ICAR), IndiaReviewed by:
Md Aktaruzzaman, University of Georgia, United StatesLiming Shi, Chinese Academy of Agricultural Sciences, China
Beibei Ge, Chinese Academy of Agricultural Sciences, China
Copyright © 2024 Ao, Shi, Yang, Ouyang, Fan, Siddiqui, Wu, Lv, Deng and Chen. This is an open-access article distributed under the terms of the Creative Commons Attribution License (CC BY). The use, distribution or reproduction in other forums is permitted, provided the original author(s) and the copyright owner(s) are credited and that the original publication in this journal is cited, in accordance with accepted academic practice. No use, distribution or reproduction is permitted which does not comply with these terms.
*Correspondence: Wenjia Yang, eWFuZ3dlbmppYTEwQDEyNi5jb20=; Xiaoyulong Chen, Y2hlbnhpYW95dWxvbmdAc2luYS5jbg==
†These authors have contributed equally to this work