- 1College of Plant Protection and Key Laboratory of Integrated Management of Crop Diseases and Pests, Nanjing Agricultural University, Nanjing, China
- 2Key Laboratory of Plant Quarantine Pests Monitoring and Control, Ministry of Agriculture and Rural Affairs, Nanjing, China
- 3Institute of Botany, Jiangsu Province and Chinese Academy of Sciences (Nanjing Botanical Garden Mem. Sun Yat-sen), Nanjing, China
- 4Plant Protection and Quarantine Station of Province, Urumqi, China
- 5Plant Protection and Quarantine Station of Province, Nanjing, China
- 6Key Laboratory of Integrated Pest Management on Crops in Northwestern Oasis, Ministry of Agriculture and Rural Affairs, Xinjiang Key Laboratory of Agricultural Bio-safety, Institute of Plant Protection, Xinjiang Academy of Agricultural Sciences, Urumqi, Xinjiang, China
ClpA is a widely conserved protease in bacteria that plays a key role in virulence. To investigate its specific mechanism of action in the pathogenicity of Paracidovorax citrulli (formerly Acidovorax citrulli), we constructed a ClpA deletion mutant, ΔClpA. The ΔClpA mutant of P. citrulli displayed reduced virulence on melon seedlings, and reduced motility, swarming ability, and antioxidant capacity. On the other hand, the ClpA deletion of P. citrulli mutant reduced the resistance to elevated temperature and enhanced biofilm formation ability. Using qRT-PCR, we observed that ClpA negatively regulates the expression of the virulence-related genes virB, pilR, pilA, and fliM, while positively regulating hrpG, hrcQ, and trbC. Bacterial double hybrid and Glutathione-S-transferase pulldown (GST-pulldown) results showed that ClpA interacts directly with RepA, and negatively regulates the expression of RepA. After deletion of the RepA gene, the pathogenicity of P. citrulli was lost, biofilm formation ability was enhanced, and the expression of hrpG, pilR, and trbC was positively regulated. These results indicate that ClpA plays a key role in the regulation of several virulence traits of P. citrulli, paving the way for future studies to better elucidate the virulence mechanisms of this bacterial plant pathogen.
Introduction
Bacterial fruit blotch (BFB) is a plant disease that occurs worldwide (Kan et al., 2023) that can be transmitted by infecting the host or non-host plants, such as solanaceous plants (Chalupowicz et al., 2020), and has a devastating impact on species in the Cucurbitaceae family (Schaad et al., 2003; Burdman and Walcott, 2012). Paracidovorax citrulli (formerly Acidovorax citrulli), the causal agent of BFB (Schaad et al., 1978, 2009; Willems et al., 1992; Du et al., 2023), can be spread via contaminated seeds (Hopkins and Thompson, 2002). Therefore, most BFB control measures focus on seed treatment to prevent the seed transmission of BFB. However, this approach alone cannot completely prevent BFB disease epidemics (Dutta et al., 2012). Studying the virulence mechanisms of P. citrulli can help us understand how the bacterium infects Cucurbitaceae host plants, provide a basis for management of BFB outbreaks, and facilitate the selection of specific pesticides or host resistance strategies.
Paracidovorax citrulli has multiple mechanisms for infecting plants, such as the type II secretion system (Johnson et al., 2007), the type III secretion system (Johnson et al., 2011; Ma et al., 2019; Jiménez-Guerrero et al., 2020; Ji et al., 2022), the type VI secretion system (T6SS; Tian et al., 2015), type IV pili (T4P; Bahar et al., 2009; Wang et al., 2022), flagella (Bahar et al., 2011), and quorum sensing (QS; Wang et al., 2016). Plants also have various defense mechanisms against infection, such as inducing the accumulation of reactive oxygen species to resist pathogen invasion (Zipfel, 2008; Yu et al., 2016).
As a regulatory member of the Hsp100/Clp molecular chaperone family, the ClpA protease, can bind with the ClpP protein, which has ATPase activity and acts as a catalytic subunit, to form a complex for the degradation of substrate proteins. Although ClpA and ClpP only exhibit their functions effectively when they form a complete Clp complex, these two subunits can function independently (Li and Lucius, 2013). ClpA, as an important regulatory subunit within the Hsp100/Clp molecular chaperone family, has been mainly studied in Escherichia coli. Its function is to recognize substrate proteins by utilizing energy from ATP hydrolysis to degrade the ClpP catalytic subunit (Zhang, 2018). ClpA is a key chaperone protein within the AAA ATPase superfamily and is involved in pathogenesis and normal growth processes of bacteria, although ClpA has been shown to be an important molecular chaperone that binds with ClpP in Escherichia coli (Pak and Wickner, 1997), In Xanthomonas campestris pv. malvacearum (Smith) Dye, it was found that ClpA can affect various biological phenotypes of pathogens, such as motility and colonization ability (Guo, 2018), but the functions of ClpA including how it affects the pathogenicity, drug resistance, and other biological traits of P. citrulli have not been reported.
In order to clarify the influence of ClpA on the pathogenesis of P. citrulli, ClpA deletion mutant was constructed, and its pathogenicity, locomotion ability, adhesion ability and HR were identified. The interaction between ClpA and RepA and other proteins was further studied, and the influence of RepA on P. citrulli was clarified, providing a new way of thinking for the prevention and treatment of BFB.
Materials and methods
Bacterial strains and growth conditions
Wild-type (xjL12) and mutant strains of P. citrulli were cultured in Luria-Bertani (LB) broth at 28°C with shaking at 220 rpm. Escherichia coli was cultured in LB medium, at 37°C with shaking at 220 rpm. Antibiotics were added as needed at the following concentrations: 100 ug/ml rifamycin (Rif), 50 ug/ml kanamycin (Km) and 100 ug/ml gentamicin (Gm). All bacterial strains were stored at −80°C. The specific strains and vectors used in this study are shown in Table 1.
Construction and complementation of deletion mutants of P. citrulli
Using the AAC00-1 genome sequence(CP000512.1), specific oligonucleotide primers were designed to amplify the target segments (upstream and downstream fragments and Km fragment) through PCR. The PCR amplicons were assembled into pEX18GM in the order of upstream, Km, downstream to produce the recombinant vector. The recombinant vector was transformed into P. citrulli strain xjL12, and colonies were screened on LB agar plates containing 10% (wt/vol) sucrose, Rif (100 mg/ml), and Km (50 mg/ml) to obtain mutant colonies: ΔClpA and Δ RepA. PCR verification was performed using primers ClpA-F1 and ClpA-R2 to select the mutants used.
Promoter prediction for the complementing genes of ΔClpA was conducted using the promoter prediction website1, ClpA and its promoter were included in the design, specific primers were designed for that region, and the fragment was amplified by PCR and ligated into the pBBR1-MCS-5 vector (Kovach et al., 1995) to produce the recombinant vector. The recombinant vector was then transformed into the gene deletion mutants. Colonies were screened on LB agar plates containing Gm (50 ug/ml) and Km (50 ug/ml) to obtain the ClpA complemented strain. PCR verification was performed using primers ClpA-HB-F and ClpA-HB-R to select the complemented strains (ΔClpA(pClpA)). The primer sequences used in this study are shown in Supplementary Table 1.
Effect of ClpA on P. citrulli population growth dynamics
To investigate the effects of the ClpA mutation on bacterial population growth dynamics (Kovach et al., 1995; Wang et al., 2022). wild-type P. citrulli strains, ClpA deletion mutants, and the ClpA complemented strain were cultured in LB medium at 28°C, with shaking at 220 rpm overnight. The cultured strains were diluted into 25 mL of fresh LB medium to achieve a cell concentration of optical density (OD)600 = 0.01 and incubated at 28°C, with shaking at 220 rpm. The OD600 of the bacterial culture was measured every 2 h, with the measurements continuing for 36 h. This experiment was replicated three times for each bacterial strain, with each experiment was conducted three times.
Effect of ClpA on P. citrulli virulence on melon
We use three methods to assess the role of ClpA in P. citrulli virulence, including measuring BFB symptoms on melon (cv. Huanghou) seedlings and inoculation by injection, seed-to-seedling transmission assays, and spray-inoculation on euphylla (Tian et al., 2015).
To investigate the effect of ClpA on virulence using the seedling injection assay, overnight shaking cultures of WT, ΔClpA, and ΔClpA(pClpA) were centrifuged to collect bacterial cells. Cells were resuspended in sterilized water and the concentration of bacterial cells were adjusted to OD600 = 0.3. Cells were then diluted 100,000-fold and cell suspensions were injected into the cotyledons of one-week-old melon seedlings. Ten cotyledons were injected with cell suspensions of each bacterial strain, and seedlings were incubated at 75% light intensity, 28°C and 85% humidity for four days. After incubation, BFB symptoms were observed on each cotyledon. Each strain was tested three times per experiment, with the entire set of experiments conducted three times.
To assess the role of ClpA on P. citrulli virulence using spray-inoculation, 20 mL of a bacterial suspension of each strain at OD600 = 0.3 was prepared as described above and placed in a spray bottle(LISM; Nanjing). Each strain cell suspensions were sprayed evenly on both sides of the euphylla of five melon plant,. The seedlings were then placed in plastic bags and incubated at 75% light intensity, 28°C and 85% humidity for 48 h. The bags were then removed, and the plants were incubated for four more days before observing the disease condition of the euphylla with disease index (DI) as described previously (Araújo et al., 2005), with modifications. The DI was calculated based on the formula: DI = Σ(A × B) × 100/ΣB × 5 (where A: disease class (0, 1, 2, 3, 4, 5); B: the number of seedlings in the corresponding disease class). This experiment was conducted three times.
For the seed-to-seedling transmission assays, we followed the protocol described by Tian et al. (2015). More specifically, we selected 25 germinated melon seeds of uniform growth and soaked them in 5 mL of cell suspensions (∼1 × 106 CFU) of each bacterial strain for 4 h. Germinated seeds were then air-dried at room temperature. Twenty treated seeds were planted in each pot and incubated at 28°C and 85% relative humidity for 7 days. Seeds were then observed for BFB seedling symptoms. Each strain was tested three times per experiment, with the entire set of experiments conducted three times.
Hypersensitive response assays
We investigated the influence of ClpA on P. citrulli’s ability to induce a hypersensitive response (HR) on Nicotiana tabacum. Each strain was cultured in LB medium until the cell suspension reached anOD600 = 0.3. The cell suspensions were rinsed and resuspended with sterilized water. Subsequently, 100 μL of the bacterial suspension was injected into N. tabacum leaves, which were then incubated at 28°C and 85% humidity for 72 h. N. tabacum leaves were then observed for an HR. Each strain was tested three times per experiment, with the entire set of experiments conducted three times.
Effects of ClpA on P. citrulli twitching motility, swimming motility, and biofilm formation assays and polar flagella morphology
The twitching motility assay was conducted as previously described by Bahar et al. (2009). Specifically, the concentration of bacterial suspensions was adjusted to OD600 = 0.3 with double-distilled water and diluted 10,000-fold. Then, the cell suspension was spread onto 1% nutrient agar (NA) medium and cultured at 28°C for 48 h. A stereo fluorescence microscope (Nikon) was used to observe twitching motility (wave-like ripples around a single colony).
The swimming motility assay was conducted as previously described by Liu et al. (2019). Bacterial cell suspensions were adjusted to OD600 = 0.3 using LB liquid medium, and 2 μL of the suspension was inoculated into the center of a 0.3% motility agar plate (Liu et al., 2019). After incubation at 28°C for 48 h, quantitative analysis was made by bar chart with the diameter of the swimming motility zone was measured. Each strain was tested three times per experiment, with the entire set of experiments were conducted three times.
Biofilm assays were conducted as previously described (Liu et al., 2019). Liquid LB medium was used to adjust bacterial cell suspensions to OD600 = 1.0. Then, 4 mL of LB liquid medium was added to each well of a 12-well PVC plate (Polyvinyl chloride plate; BeyoGold™ 12 Well Cell Culture Plates; Shanghai), and 40 μL of bacterial suspension was inoculated into it. The plate was incubated at 28°C for 48 h and after decanting the supernatant, the OD600 was measured. The PVC plate was then dried in an 80°C oven for 20 min. Five milliliters of 1% crystal violet was added to each well, incubated for 45 minutes, and then the purple precipitate in the well was photographed. The biofilm was dissolved with 5 mL of anhydrous ethanol and the OD590 was measured. The OD590/OD600 ratio was calculated. We used transmission electron microscopy (TEM) to visualize polar flagella of P. citrulli, as described by Bahar et al. (2009). Each strain was tested three times per experiment, with the entire set of experiments were conducted three times.
Effect of ClpA on P. citrulli H2O2 sensitivity and heat resistance
The sensitivity of P. citrulli to hydrogen peroxide (H2O2) was determined by measuring the diameter of the inhibition zones on agar plates with different concentrations of H2O2. Bacterial strains were incubated at 28°C, 220 rpm overnight to reach an OD600 = 0.3. Then, 2 mL of the adjusted bacterial culture was transferred to 98 mL of molten LB medium at 40°C, poured into Petri dishes and allowed to solidify. A sterilized filter paper disc (5 mm diameter) was placed in the center of each agar plate, and 5 μL of different concentrations (1%, 5%, 10%) of H2O2 were added to the filter paper. Filter discs were incubated at 28°C for 48 h and then the diameters of the inhibition zones were measured.
Bacterial strains were incubated at 28°C, 220 rpm overnight to reach an OD600 = 0.3 then 1 mL of the each adjusted bacterial culture was transferred to different test tubes. The test tubes were then incubated in water bath at different temperatures (35°C, 40°C, 45°C, 50°C, 55°C, 60°C), for 10 min, and 0.1 ml of bacterial suspension was re-injected into 5 ml LB medium, and shaken at 28°C for 24 h. Bacterial population growth was monitored by measuring OD600. Quantitative analysis was made by bar chart with the OD600 was measured. This experiment was conducted three times for each bacterial strain and the study was conducted three times.
RNA isolation and quantitative real-time PCR analysis
Bacterial strains were cultured at 28°C, at 220 rpm to a concentration of OD600 = 1.0, then bacterial cells were harvested by centrifugation at 1000 × g for 1 min. Total bacterial RNA was extracted using a bacterial RNA extraction kit (Pudi DP201; Shanghai). The extracted RNA was reverse transcribed using HiScript III RT SuperMix with gDNA Wiper (Vazyme; Nanjing) to obtain cDNA. The cDNA was diluted to 50ng/μL and real-time fluorescent quantitative PCR was conducted on a ABI PRISM 7500 real-time PCR machine (Applied Biosystem) using ChamQ Universal SYBR qPCR Master Mix (Vazyme; Nanjing). The reference gene was the 16S ribosomal RNA of P. citrulli. The qRT-PCR program was as follows: 95°C for 30s, 95°C for 10s, 60°C for 30s, for 40 cycles, followed by a final melting curve analysis from 60 to 95°C. Each gene had three biological replicates, and the above experiments were conducted three times. The final gene expression level changes were based on the 2–ΔΔCt value.
Bacterial two-hybrid experiments
Bacterial two-hybrid assays were used to investigate the interaction between ClpA and putative targets (Table 1). The coding region (2,352 bp) of ClpA was linked to pTRG to form the recombinant vector pTRG-ClpA. The coding regions of the putative target proteins were linked to pBT to form recombinant vectors pBT-ClpP, RepA, etc. The pTRG and pBT recombinant vectors were co-transformed into the XL1-Blue MRF′ Kan strain. When ClpA interacts with the putative target, the transformed E. coli strain will grow on the selection medium (minimum medium with 5 mm 3-amino-1,2,4-triazole, streptomycin 8 ug/ml, tetracycline 12.5 ug/ml, chloramphenicol 34 ug/ml, and Km 30 ug/ml) (Wang et al., 2018). Colony growth was observed on the medium after 48 h of incubation at 28°C. pBT and pTRG were used to co-transform strains as positive control and pBT-target with pTRG empty vector strains as negative control.
Gst-pull down assay
pET330a-RepA-His and pGEX-4T-1-ClpA protein expression vectors were constructed and transformed into E. coli. Rosetta(DE3). Cells were inoculated into LB medium containing 50 μg/mL Amp and incubated overnight at 37°C with shaking. 100 μL of the culture was transferred into 25 mL of LB medium containing 50 μg/mL Amp and shaken at 37°C until the OD600 = 0.6. IPTG was added to the culture to a final concentration of 0.5 mM, and the culture was shaken at 16°C for over 7 hours. The cells were harvested and resuspend in PBS, lysed by sonication, and centrifuged to collect the supernatant.
GST-ClpA and His-RepA proteins were mixed in a 1:1 ratio, and 50 μL of the mixture add 12.5 μL of 5 × loading buffer were added. The mixture was then boiled at 98°C for 10 min and used as the input sample. 20 μL of glutathione Sepharose 4B was added to the remaining mixture, which was then rotated and mixed at 4°C for 4 h, centrifuged at 500 × g for 5 min. to remove the supernatant, and the pellet was resuspended in 1mL of high salt PBST (250 mL phosphate-buffered saline + 1% Tween + 3 g NaCl), and mixed by rotating at 4°C for 10 min. The mixture was centrifuged at 500 × g for 5 min, the supernatant was removed, and the washing process was repeated three times. 50 μL glutathione reductase was added to the final pellet, and after mixing, the sample was centrifuged at 4°C, 500 × g for 5 min. 50 μL of the supernatant was mixed with 12.5 μL of 5 × loading buffer, boiled at 98°C for 10 min. and used as the output sample. A GST empty vector was used as the control.
The final samples were analyzed by Sodium Dodecyl Sulfate-Polyacrylamide Gel Electrophoresis (SDS-PAGE) and transferred onto polyvinylidene fluoride membranes (Millipore, Red Bank, NJ, USA) using a semi-dry protein transfer system. Membranes were blocked with 5% milk at room temperature for 1 h, washed with 5% Tween Tris-buffered saline (TBST) solution at pH 7.5, and incubated with specific anti-GST antibodies and His tags for 1 h, followed by incubation with anti-rabbit secondary antibodies for 1 h. Protein immunoblotting was performed using the HyGlo HRP ECL detection kit (MDBio Inc., Qingdao, China) and membranes were photographed using the Tanon-6600 automatic multifunctional imaging analysis system (Tanon, Shanghai, China).
Results
ClpA does not affect bacterial growth, but it attenuates the virulence of P. citrulli
The ClpA protein of P. citrulli is closely related to the protein in bacteria in the P. citrulli (WP017438384.1:1-783) (Supplementary Figure 1). However, the absence of ClpA did not affect in vitro growth of P. citrulli (Supplementary Figure 2A). To determine the impact of ClpA on the virulence of P. citrulli, we compared the severity of BFB symptoms on melon cotyledons inoculated with WT, ΔClpA, and the complementary strain, ΔClpA(pClpA).
Compared to the WT, the cotyledons of melon seedlings inoculated with the ΔClpA strain did not induce BFB symptoms (Figure 1A), and P. citrulli virulence was restored in the complemented strain. We also found that the average bacterial cell populations of P. citrulli WT, ΔClpA, and ΔClpA (pClpA) strains were approximately 1.26 × 109, 4.78 × 105, and 3.34 × 108 CFU/g of tissue, respectively, by 48 hpi (Figure 1D). In the other two virulence assays, the ClpA mutants of P. citrulli also displayed reductions of BFB symptoms (Figures 1B,C). In the seed-to-seedling transmission assays, the disease index of the negative control (NC) was 0, while that of WT, ΔClpA, and the complemented strain were 81.6, 7.3, and 41.9, respectively (Figure 1E). In the true leaf spray-inoculation experiment, the disease indices for NC, WT, ΔClpA, and the complemented strain were 0, 33.4, 14.3, and 29.8, respectively (Figure 1F). Compared to WT, the virulence of ΔClpA on the cotyledons of melon seedlings was significantly reduced. The virulence of P. citrulli ΔClpA on true leaves was also significantly decreased, as was its seed-to-seedling-transmission ability (P < 0.05).
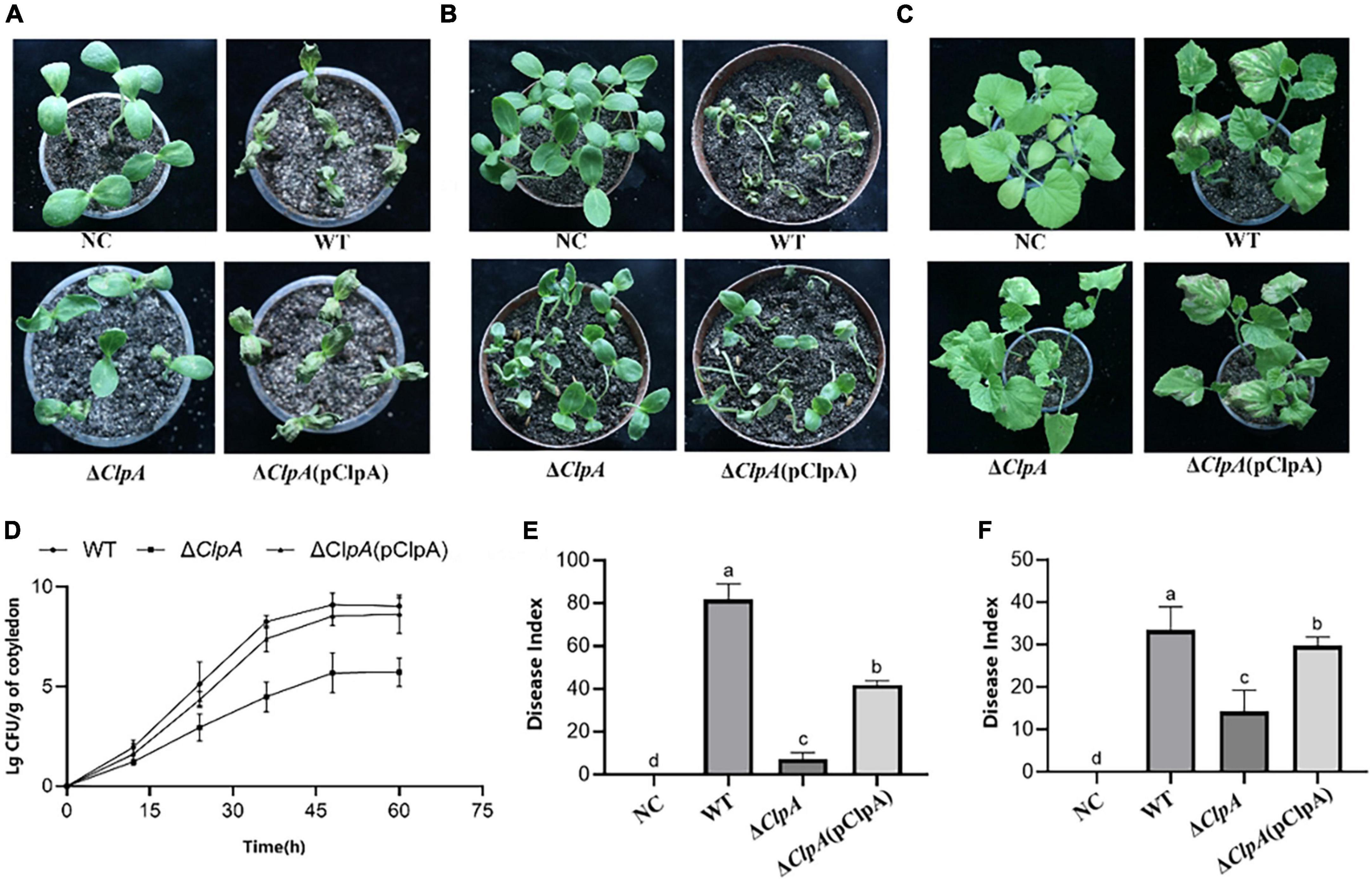
Figure 1. Role of ClpA in Paracidovorax citrulli virulence and bacterial growth in melon seedlings. (A) Melon seedling cotyledons inoculated by injection with P. citrulli wild-type (WT): xjL12, ΔClpA, and ΔClpA (pClpA) strains (∼1 × 103 CFU/ml), and double-distilled H2O as a negative control (NC). Seedlings were observed for bacterial fruit blotch symptoms at 4 days post-inoculation (dpi). (B) Melon seeds were inoculated by soaking in bacterial cell suspensions (∼1 × 106 CFU/ml) of P. citrulli WT, ΔClpA, and ΔClpA (pClpA). BFB symptoms were observed 7 days after planting. (C) Spray-inoculation of melon euphylla with P. citrulli cell suspensions (∼1 × 106 CFU/ml) of WT, ΔClpA, and ΔClpA (pClpA). (D) Bacterial suspensions (∼1 × 103 CFU/ml) of each strain were injected into cotyledons of melon seedlings and bacterial populations were quantified at 0, 12, 24, 48, and 60 h post-inoculation. (E) Melon seed were inoculated with P. citrulli strains (∼1 × 103 CFU/ml) planted under conditions conducive for BFB development and the disease index of seed-seedling transmission were determined. The letters above the bars represent significant differences (P < 0.05, LSD). (F) 7 days after spray-inoculation on euphylla and the disease index of spraying inoculation on euphylla was determined. The letters above the bars represent significant differences (P < 0.05, LSD).
ClpA attenuates P. citrulli swimming motility, twitching motility and enhances biofilm production, and positively regulates expression of genes related to flagella and pili
In the twitching motility assay, after 48 h of cultivation at 28°C, wave-like halos surrounding single colonies were visible under the stereo microscope on NA plates, however, the swarming ring of ΔClpA was less than that of the WT strain (Figure 2A). The swimming motility phenotype of ΔClpA was less than that of WT (Supplementary Figure 5A; P < 0.05), and the complemented strain did not restore the function (Figure 2B). The biofilm formation ability of ΔClpA was enhanced relative to the WT strain (Figure 2C; Supplementary Figure 4A), and using transmission electron microscopy, we observed full-length polar flagella for WT and ΔClpA (pClpA) strains, but not for ΔClpA (Figure 2D).
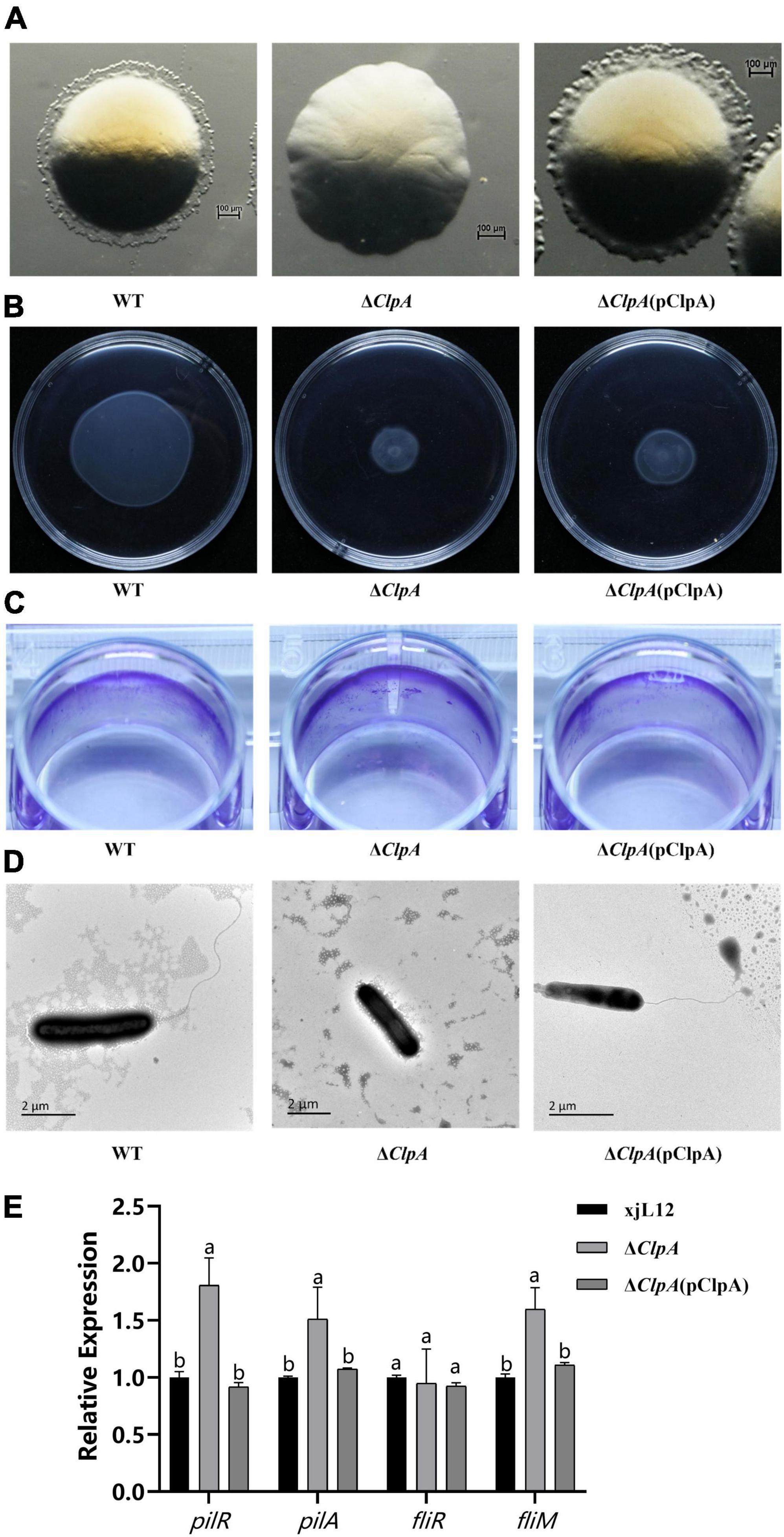
Figure 2. Role of ClpA in twitching motility, swimming motility, and biofilm formation of P. citrulli. (A) Twitching motility of P. citrulli strains including wild-type (WT): xjL12, ΔClpA, and ΔClpA (pClpA). Strains were transferred onto 1% NA medium and the resulting colonies were photographed using a stereoscope after 48 h. (B) Swimming motility of P. citrulli strains including WT: xjL12, ΔClpA, and ΔClpA (pClpA) on 0.3% agar plates at 28°C for 2 days. (C) Biofilm formation of P. citrulli strains including WT: xjL12, ΔClpA, and ΔClpA (pClpA). Biofilm production is indicated by a ring of dark purple precipitate on the inner wall of culture plate wells. (D) Transmission electron microscope verification of presence polar flagella. Full-length flagella (arrows) can be seen in WT and ΔClpA (pClpA) strains, while flagella were not visible for ΔClpA. (E) Expression level of pili and flagella-related genes pilR, pilA, fliR and fliM in P. citrulli WT, ΔClpA and ΔClpA (pClpA) were determined by qRT-PCR. Different lowercase letters indicate a significant difference between treatments. Statistically significant differences were determined by the one-way ANOVA of variance and P < 0.05.
Based on qRT-PCR results, we found that the expression levels of pilR and pilA, two genes related to pili, can affect the pathogenicity of P. citrulli (Yang et al., 2023), were significantly upregulated. This is consistent with the enhanced biofilm formation observed in the biofilm assays. However, the expression levels of the gene associated with flagellum of P. citrulli (Zhang, 2018), fliM, were significantly upregulated, and there was no difference in expression of fliR (Figure 2E).
ClpA deletion weakened the antioxidative capacity and reduced the resistance to elevated temperature of P. citrulli
To elucidate the role of ClpA in the antioxidative capacity of P. citrulli, the inhibition zone method was used to measure sensitivity of WT, ΔClpA, and complemented strains to different concentrations of hydrogen peroxide. Compared to WT, the diameter of the inhibition zone of ΔClpA was significantly larger (Figure 3B; P < 0.05), indicating increased sensitivity to H2O2 (Figure 3A).
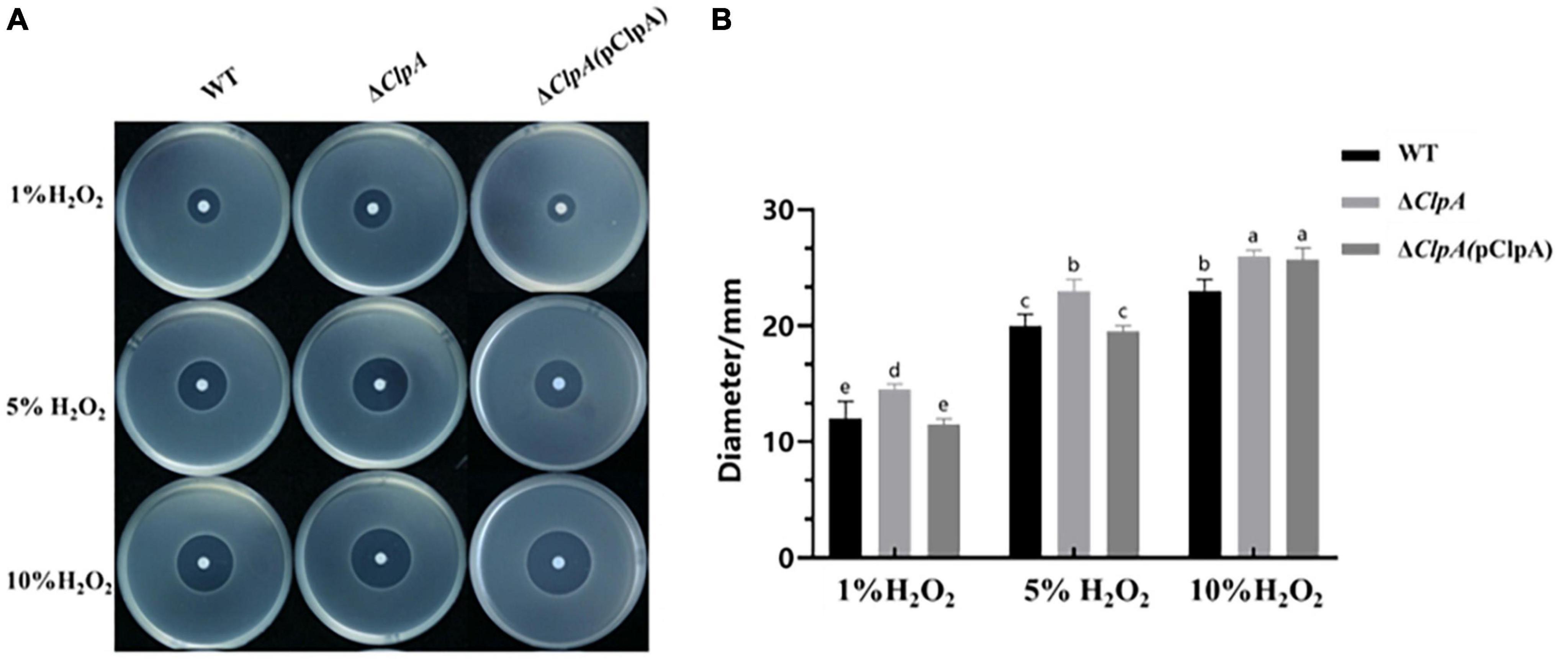
Figure 3. Sensitivity of WT, ΔClpA and ΔClpA(pClpA) strains to H2O2. (A) 2 μL of different concentrations (1, 5, and 10%) of H2O2 were dropped in the center of the plates. The H2O2inhibition zones were observed and measured after incubation at 28°C for 48 h. (B) The diameter of the zone of bacterial growth inhibition. Experiments were performed in triplicate and were repeated three times with similar results. Data represent the means of three replicates ± standard deviations (error bars). Different lowercase letters indicate a significant difference between treatments. Statistically significant differences were determined by the one-way ANOVA and P < 0.05.
In the high-temperature tolerance experiment, we found that at 35°C, there was no significant difference in the number of surviving bacteria cells between the WT and ΔClpA mutant. However, at 40°C, the WT had significantly more surviving bacterial cells than the ΔClpA mutant. The WT strain and ΔClpA (pClpA) strain survived at 45°C and the number of bacterial cells increased, while the ΔClpA mutant did not survive. After incubating at 50°C, 55°C, and 60°C for 10 min, WT, ΔClp and ΔClpA (pClpA) was unable to grow. This indicates that ClpA affects P. citrulli’s sensitivity to high temperatures (Supplementary Figure 3), ClpA deletion reduced the resistance to elevated temperature of P. citrulli.
ClpA does not influence the ability of P. citrulli to induce HR on N. tabacum, but positively regulates expression of genes related to pathogenicity
This study investigated the expression levels of related pathogenic genes in ΔClpA to clarify how ClpA impacts pathogenicity of P. citrulli, hrcQ, hrpG and hrpX has been reported in P. citrulli, which is an important T3SS regulatory gene (Bahar and Burdman, 2010; Burdman and Walcott, 2012; Yu, 2018). trbC and virB is the gene that affects the assembly of pili in T4SS (Christie et al., 2017; Shala-Lawrence et al., 2018). The results showed that after the deletion of ClpA, we found that the expression levels of hrpG (T3SS gene), hrcQ (T3SS gene), and trbC (T4SS), were significantly downregulated, while virB(T4SS gene) was upregulated (Figure 4B). ΔClpA and ΔClpA(pClpA) did not influence the ability of P. citrulli to induce a HR on Nicotiana tabacum (Figure 4A).
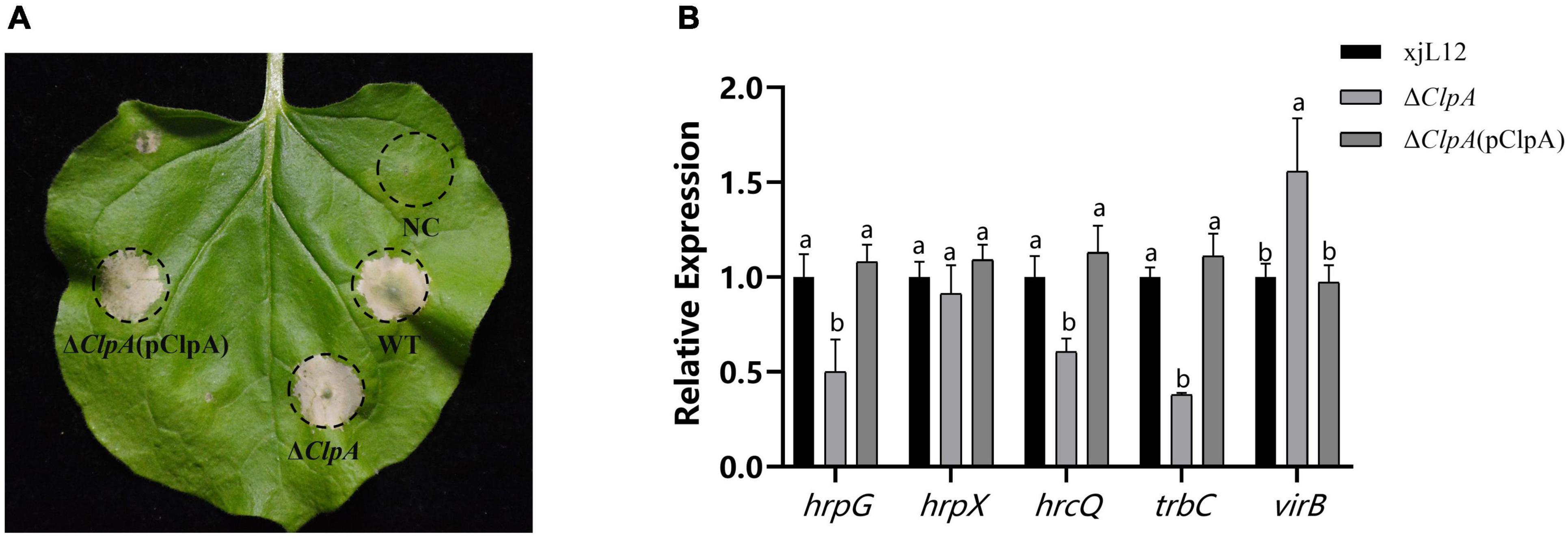
Figure 4. Effects of ClpA on Nicotiana tabacum hypersensitive response (HR) induction by P. citrulli strains. (A) Tobacco HR induction. Approximately 100 μl of bacterial suspension (OD600 = 0.3) was infiltrated at each inoculation site on a tobacco leaf and the plant was incubated at 28°C. N. tabacum was grown under greenhouse conditions at 24°C and observed for HR at 24 to 72 h post-infiltration(hpi). Negative Control: double-distilled H2O; WT: wild-type xjl12; ΔClpA; ΔClpA(pClpA). (B) Expression level of hrpG, hrpX, hrcQ, trbC and virB genes in WT, ΔClpA and ΔClpA(pClpA) strains by qRT-PCR. Experiments were conducted in triplicate and repeated three times with similar results. Data shown represent the means of three replicates ± standard deviations. Error bars indicate standard deviations. Different lowercase letters indicate a significant difference between strains. Statistically significant differences were determined by the one-way Analysis of variance (ANOVA) and P < 0.05.
ClpA interacts with RepA but not with ClpP
Bacterial two-hybrid assays were conducted targeting proteins closely related to ClpA and pathogenic genes to elucidate the interaction targets of ClpA and understand how it impacts P. citrulli pathogenicity. Based on to Pak’s study (Pak and Wickner, 1997) and our preliminary results, we selected six targets: SsrA: the polypeptide translated from truncated mRNAs that are marked by a short peptide, known as SsrA tag, at their C-termini and directed to the specific endogenous proteases for C-terminal proteolysis; RepA: A protein that recognizes the origin of replication (ori) in the plasmid; ClpX: Chaperone protein; ClpP: Catalytic subunit; DnaJ: Heat shock associated chaperone proteins; and GrpE: Essential protein for resistance to high temperature stress. Using a two-hybrid assay between ClpA and potential targets, we found that in P. citrulli, there is an interaction between RepA, ClpX with ClpA (Figures 5B, E), but ClpA does not interact with SsrA, DnaJ, GrpE (Figures 5A, D, F). Although ClpA exerts its full function by forming a complex with ClpP in many bacteria, in P. citrulli, ClpA does not interact with ClpP (Figure 5C). Therefore, we assumed that ClpA directly interacts with RepA. To further validate the regulatory relationship between ClpA and its interaction targets, Gst pull-down experiments were performed. (Figure 5G), this result showed that ClpA directly interacts with RepA, but ClpA does not directly interact with ClpX(Figure 5H). qRT-PCR results indicated that in ΔClpA, the expression levels of RepA were significantly upregulated, meaning that ClpA negatively regulates RepA(Figure 6F).
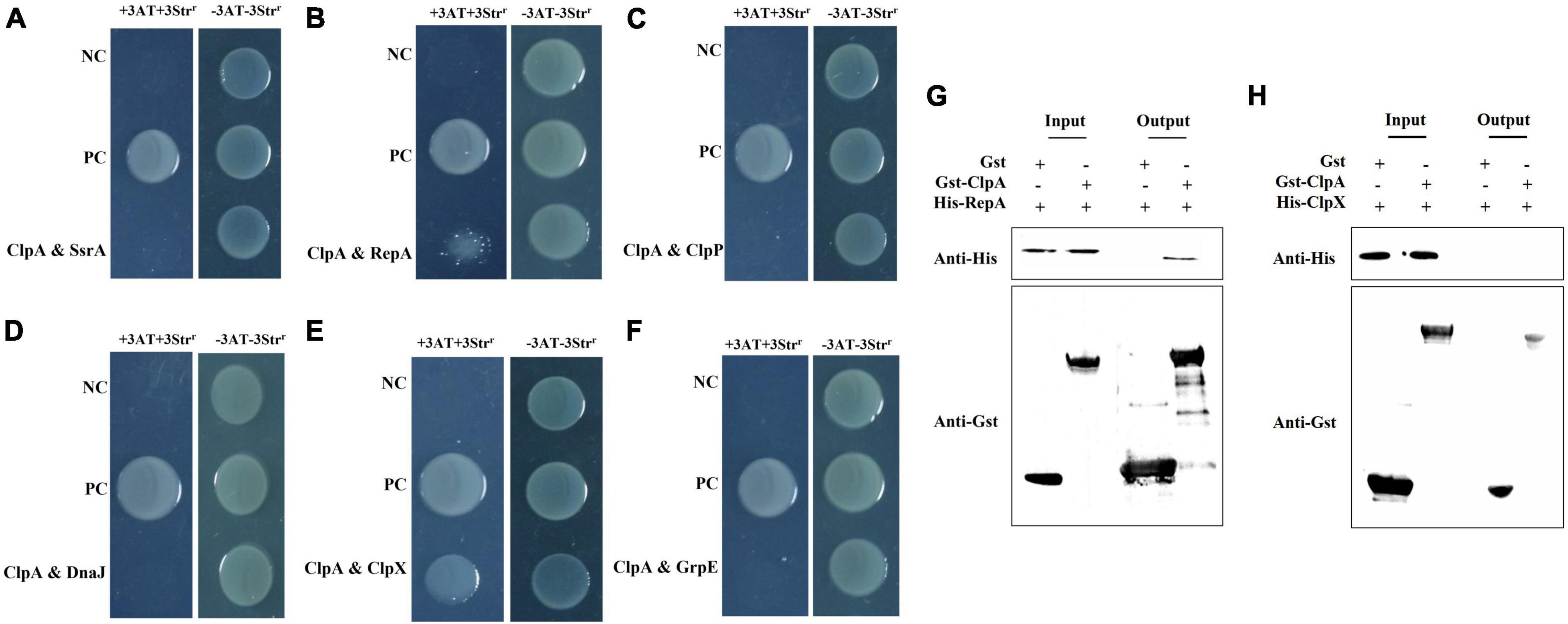
Figure 5. Bacterial two-hybrid assays: pTRG-GacSand pBT-GacS: co transformant containing pTRG-GacS and pBT-GacS serves as a positive control; 3AT-Strr: no selective LB medium plate; and +3AT + Strr: M9-based selective medium plate. (A) ClpA and SsrA displayed no interaction as verified by bacterial two-hybrid assay. ssrA was cloned into vector pTRG, and ClpA were cloned into vector pBT, respectively. (B) ClpA and RepA interaction verified by bacterial two-hybrid. RepA was cloned into vector pTRG, and ClpA were cloned into vector pBT, respectively. (C) ClpA and ClpP showed no interaction as verified by bacterial two-hybrid assay. ClpP was cloned into vector pTRG, and ClpA were cloned into vector pBT, respectively. (D) ClpA and DnaJ no interaction verified by bacterial two-hybrid. DnaJ was cloned into vector pTRG, and ClpA were cloned into vector pBT, respectively. (E) ClpA and ClpX interaction verified by bacterial two-hybrid. ClpX was cloned into vector pTRG, and ClpA were cloned into vector pBT, respectively. (F) ClpA and GrpE no interaction verified by bacterial two-hybrid. GrpE was cloned into vector pTRG, and ClpA were cloned into vector pBT, respectively. (G) Interaction between P. citrulli ClpA and RepA is indicated by a GST pull-down assay. The purified GST-ClpA was incubated with His-RepA and pulled down with GST beads. (H) Interaction between P. citrulli ClpA and ClpX is indicated by a GST pull-down assay. The purified GST-ClpA was incubated with His-ClpX and pulled down with GST beads.
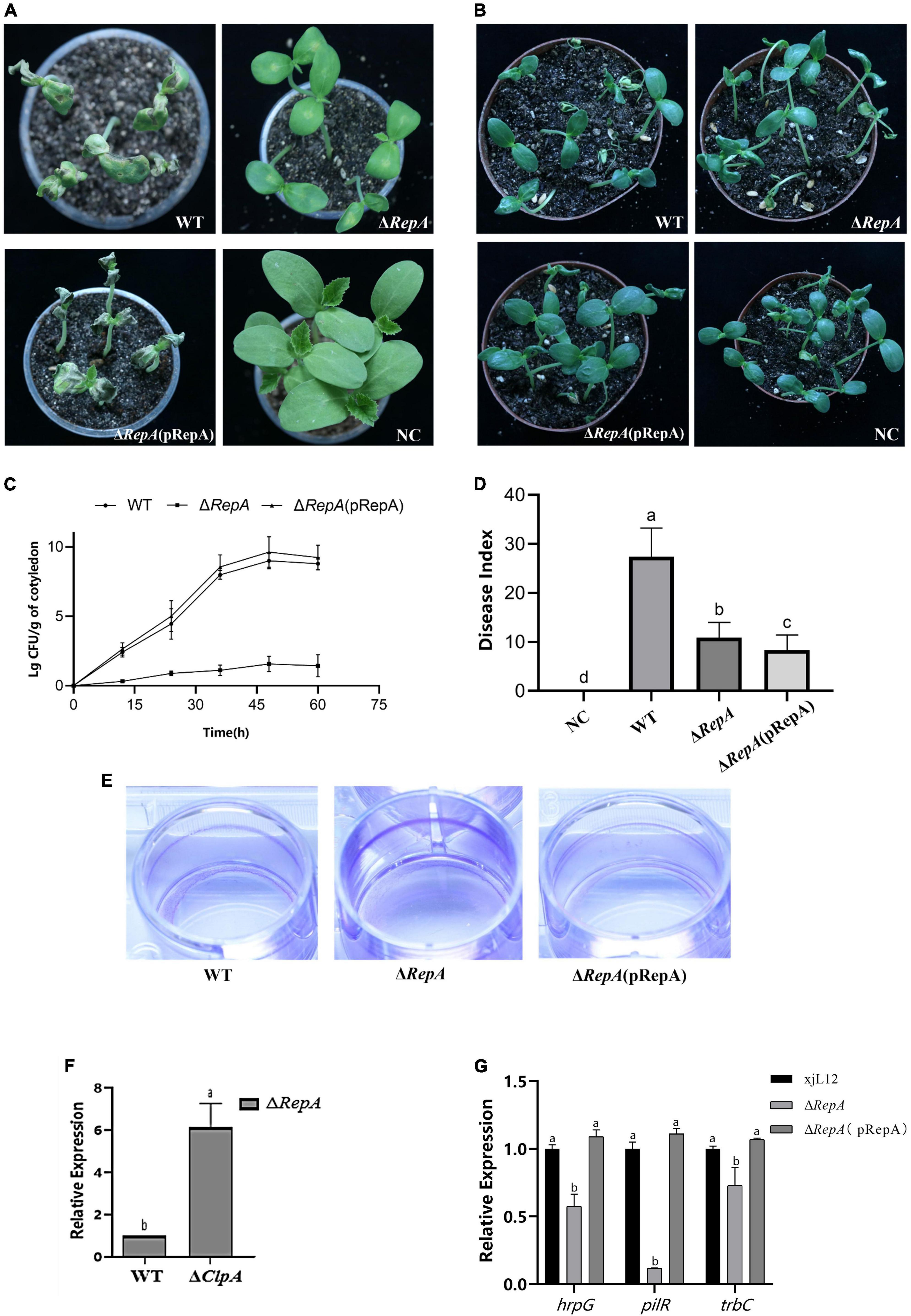
Figure 6. Role of RepA in P. citrulli virulence and bacterial growth in melon seedlings. (A) Melon seedling cotyledons inoculated by injection with P. citrulli wild-type (WT): xjL12, ΔRepA and ΔRepA(pRepA) strains (∼1 × 103 CFU/ml), and double-distilled H2O (ddH2O) as a negative control (NC). Seedlings were observed for bacterial fruit blotch symptoms at 4 days post-inoculation (dpi). (B) Melon seeds were inoculated by soaking in bacterial cell suspensions (∼1 × 106 CFU/ml) of P. citrulli WT, ΔRepA,ΔRepA(pRepA). BFB symptoms were observed 7 days after planting. (C) Bacterial suspensions (∼1 × 103 CFU/ml) of each strain were injected into cotyledons of melon seedlings and populations were quantified at 0, 12, 24, 48, and 60 h post-inoculation. (D) Melon seeds were inoculated with P. citrulli strains (∼1 × 103 CFU/ml) planted under conditions conducive for BFB development and the disease index of seed-seedling transmission were calculated. The letters above the bars represent Least Significant Differences (P < 0.05, LSD). (E) Biofilm formation of P. citrulli strains including wild-type (WT): xjL12, ΔRepA and ΔRepA(pRepA). Biofilm production is indicated by a ring of dark purple precipitate on the inner wall of culture plate wells. (F) Expression level of RepA between P. citrulli WT and ΔClpA were determined by qRT-PCR. Different lowercase letters indicate a significant difference between treatments. Statistically significant differences were determined by the one-way ANOVA of variance and P < 0.05. (G) Expression level of pathogenicity-related genes hrpG, pilR, trbC between P. citrulli WT, ΔRepA and ΔRepA(pRepA)were determined by qRT-PCR. Different lowercase letters indicate a significant difference between treatments. Statistically significant differences were determined by the one-way analysis of variance and P < 0.05.
RepA attenuates the pathogenicity of P. citrulli, enhances biofilm production and reduces the expression of pathogenicity-related genes.
Preliminary verification of the effect of RepA on the pathogenicity of P. citrulli was obtained through virulence assays on melon seedlings. In both pathogenicity assays, the mutant did not cause BFB symptoms. Compared to the WT, the cotyledons of melon seedlings inoculated with the ΔRepA strain showed significantly reduced BFB symptoms, and the complementary strain ΔRepA(pRepA) displayed symptoms similar to the WT strain (Figure 6A). The average bacterial cell populations for P. citrulli WT, ΔRepA(pRepA) and ΔRep strains were approximately 1 × 109, 4 × 109 and 37 CFU/g of tissue, respectively, by 48 hpi (Figure 6C). In the seedling transmission test, the disease indexes for seeds inoculated with NC, WT, ΔRepA(pRepA) and ΔRepA were 0, 27.4, 8.3 and 10.9 (Figure 6D), respectively, after 7 days of cultivation. Compared to WT, ΔRepA showed significantly reduced virulence on melon cotyledons and a significant reduction in seedling transmission (P < 0.05; Figure 6B), and the complementary strain ΔRepA(pRepA) did not recover pathogenicity. These results suggest that RepA is the key to P. citrulli pathogenicity.
This study determined the role of RepA by measuring the biofilm production and swimming motility of P. citrulli. In the motility assay, the diameter of ΔRepA was not different from that of the WT strain (Supplementary Figure 5C). However, in the biofilm assay, the biofilm formation ability of ΔRepA significantly increased (Figure 6E), which was similar to ΔClpA. These results suggest that RepA is the key to P. citrulli biofilm formation. ClpA can regulate the expression of RepA (Figure 6F). To clarify how RepA affects the pathogenicity of P. citrulli, we quantified the expression of pathogenicity-related genes in ΔRepA and found that the expression levels of hrpG, pilR, trbC were significantly downregulated (Figure 6G). In contrast, the expression levels of RepA was significantly upregulated, while pilA, fliM, virB were not changed (Supplementary Figure 5D). These results suggest that RepA is important for positively regulating the expression levels of the T3SS gene (hrpG), pili gene (pilR) and quorum gene (trbC) of P. citrulli (Figure 6G). So RepA is the key to the pili, T3SS and quorum sensing.
Discussion
The AAA ATPase superfamily in P. citrulli has a key impact on bacterial virulence, such as the deletion of genes ClpP, which also leads to a significant reduction in pathogenicity of P. citrulli (Yu, 2023). As a member of the Hsp100/Clp family, ClpA is responsible for preparing protein substrates to be degraded by ClpP (Butler et al., 2006). In this study, by constructing a ClpA deletion mutant strain of P. citrulli and its corresponding complemented strain, we found that although ClpA is essential for normal bacterial life (Gottesman, 1996), its absence does not affect the growth of P. citrulli. However, ΔClpA displayed significantly reduced pathogenicity in cotyledon-injection assays, true leaf spray-inoculation assays, and seedling transmission assays. P. citrulli pathogenicity involves systems such as T3SS (Johnson et al., 2011), quorum sensing (Wang et al., 2016) and flagella (Bahar et al., 2011). The melon host plant’s PTI/ETI also impedes bacterial infection by inducing ROS bursts (Dodds and Rathjen, 2010; Block and Alfano, 2011). Therefore, in this study, the in vitro motility, adhesion ability, and antioxidant capacity of various strains were studied. The results showed that ΔClpA motility halo disappeared, and the complemented strain’s halo was restored. While the mutant lost swimming motility, the complemented strain did not fully recover his phenotype. These results are similar to reports on ClpX and ClpP (Yu, 2023). This study validated the changes of high-temperature resistance of ΔClpA and found that bacterial resistance to high temperatures was weakened after the deletion of the ClpA gene. This result is in accordance with earlier study which showed that ClpA is essential for bacterial survival under high-temperature conditions (Lo et al., 2022). In order to identify potential interacting targets for ClpA, we chose GrpE and DnaJ, which are proteins related to bacterial response to high temperatures (Thomas and Baneyx, 2000), for bacterial two-hybrid validation. However, there are no interaction between ClpA and GrpE, DnaJ. Therefore, we assumed that ClpA could play an independent role in P. citrulli, and further experiments will be conducted to verify this hypothesis. In in vitro adhesion ability assays, the biofilm formation ability of ΔClpA was significantly enhanced, and the biofilm formation phenotype of the complemented strain was restored. Research has indicated that bacterial biofilms play a role as virulence factors in bacterial pathogenicity (Jain and Agarwal, 2009). Li’s study was observed that there is a significant difference in bacterial pathogenicity between biofilm of Pseudomonas aeruginosa and planktonic forms of Pseudomonas aeruginosa, with gene expression related to pathogenicity being approximately 30 times lower in biofilm bacteria compared to the planktonic counterparts, this study also revealed that the formation of bacterial biofilms affects the release of virulence factors by planktonic bacteria, resulting in reduced pathogenicity. Furthermore, it was discovered that biofilms possess inherent immunity against host responses, which could contribute to this decrease in bacterial toxicity (Li et al., 2014), however, the real reason for this phenomenon in P. citrulli still needs to be verified by further experiments. ClpA is a key gene in P. citrulli pathogenicity and it may have complex interactions with neighboring genes. This may explain why the complemented strain could not fully restore the biofilm phenotype.
The enhanced biofilm formation ability of ΔClpA in this study is opposite to the ClpX deletion in P. citrulli and ClpP deletion in P. citrulli (Yu, 2023), so we speculate that ClpA may not regulate in the traditional binding form to form a complex with ClpP in P. citrulli. To clarify the regulation of virulence by ClpA in P. citrulli, we performed real-time quantitative PCR assays to measure the expression of related pathogenic genes in ΔClpA and found that the expression levels of pilR, pilA, fliM, and virB genes were significantly upregulated, while hrpG, hrcQ, and trbC were significantly downregulated. There was no significant change in the expression levels of hrpX and fliR. Similar results were observed with the deletion of ClpA in Aac5 (Yu, 2023); so ClpA is involved in the regulation of pathogenic genes’ expression in P. citrulli. To further clarify how ClpA affects the expression of related genes, we screened for potential interaction targets of ClpA. Through bacterial two-hybrid assays, we confirmed the interaction of ClpA with RepA, ClpA may has indirect interaction with ClpX(further experimental verification is required), and the absence of interaction with the catalytic subunit ClpP. This observation agrees with our previous conclusion, but in P. citrulli, ClpA can regulate the expression of ClpP (Yu, 2023). We further speculate that ClpA plays a role in protein degradation independently, and can regulate RepA to influence the degradation of ClpP and the other proteins in P. citrulli.
Using GST-pulldown assays we found that ClpA directly interacts with RepA as reported by Pak and Wicker (Pak and Wickner, 1997). We also observed that the expression levels of RepA were significantly upregulated in ΔClpA. We observed that RepA’s expression level is significantly enhanced, but Pak and Wickner showed that ClpA can activate RepA in E.coli (Pak and Wickner, 1997), which may be different to P. citrulli. Research has shown that ClpA can remodel the RepA dimer of bacteriophage P1 into a monomer, activating the potential DNA binding activity of RepA (Wickner et al., 1994; Pak and Wickner, 1997). When independent of ClpP, ClpA functions as an ATP-dependent chaperone protein to activate the P1 plasmid replication initiation protein RepA in vitro, similar to DnaJ and GrpE (Wickner et al., 1994). Although it can highly activate specific DNA binding sites for RepA, it is not part of the RepA-specific DNA complex (Wickner et al., 1991). ClpA also protects RepA from heat-induced inactivation and prevents destructive thermal denaturation outside of luciferase bodies (Wickner et al., 1994). Therefore, we constructed a RepA deletion mutant to investigate the impact of RepA on P. citrulli virulence and to determine whether ClpA influences the pathogenicity by interacting with RepA. The results showed that the growth ability of ΔRepA did not change significantly, but its virulence, including symptoms on melon seedlings in injection assays, and seed-to-seedling transmission assays, was reduced, which is consistent with the phenotype of ΔClpA. Hou et al. showed that the resistance response induced by RepA in N. tabacum is temperature-sensitive (Hou, 2015), and this was true for ΔClpA and ΔRepA.
When testing the biofilm formation ability of ΔRepA, we observed an increased biofilm formation ability, which also aligns with the phenotype of ΔClpA. Therefore, we conducted quantitative analyses of expression of virulence-related genes that showed significant changes in ΔClpA and discovered in ΔRepA, genes such as hrpG, pilR, and trbC were significantly downregulated. This indicated that RepA positively regulates the expression of genes related to virulence and pili formation. We observed that the change in expression of hrpG, trbC in ΔRepA was similar for ΔClpA. However, the change in expression of pilR, which is downregulated in ΔRepA, is upregulated in ΔClp. Therefore, ClpA negatively regulates the expression of pilR and RepA positively regulates the expression of pilR. In other words, ClpA can regulate RepA to change the expression of pilR, then change the pili and pathogenicity. In contrast, the biofilm formation ability of ΔRepA increased; it may be regulated by more genes than a single pilR. More research is needed to better understand this phenomenon.
In conclusion, we observed that ClpA plays a key role in the regulation of many pathogenic factors in P. citrulli, such as in vitro motility, in vitro adhesion capacity, antioxidant activity, and T3SS. We also showed that in P. citrulli, ClpA does not interact with ClpP, but may has a direct interaction with RepA. ClpA negatively regulates RepA. RepA is a protein that influences key pathogenicity factors such as pili, T3SS, and virulence proteins in P. citrulli, making it a crucial virulence gene (Figure 7). Therefore, ΔClpA affects the expression of virulence factors, such as pili (pilR), thus impacting the pathogenicity of P. citrulli through its interaction with RepA. Follow-up studies will delve into the ClpA regulation by to further regulate these complex regulatory mechanisms.
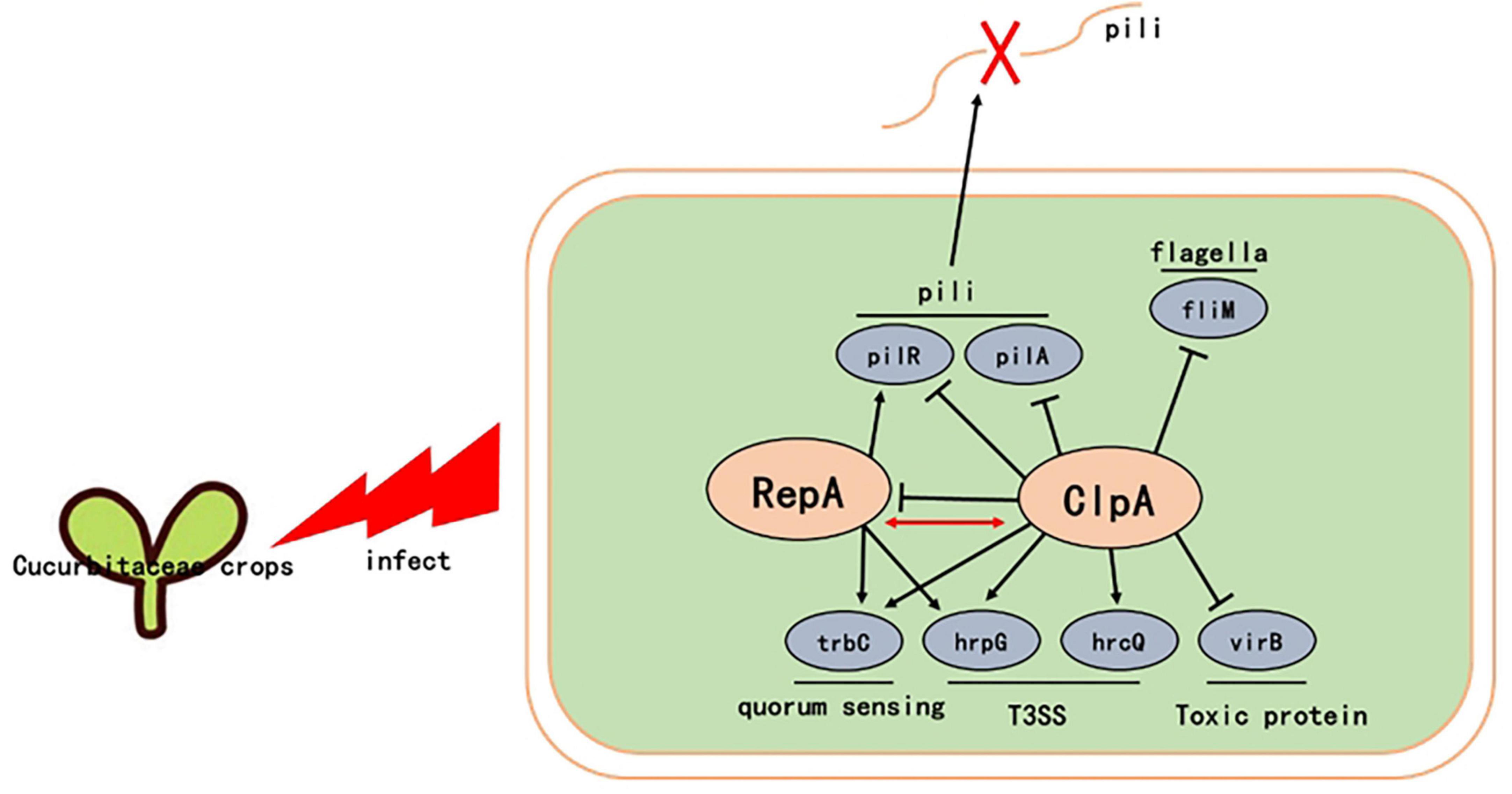
Figure 7. Proposed model illustrating the global effect of ClpA in P. citrulli. ↓, positive regulation; ⊥, negative regulation; T3SS: type III secretion system. Regulatory steps in the model are mainly at the transcriptional level.
Data availability statement
The original contributions presented in the study are included in the article/Supplementary material, further inquiries can be directed to the corresponding author.
Author contributions
SZ: Data curation, Formal analysis, Writing–original draft. ZY: Conceptualization, Formal analysis, Methodology, Writing–review and editing. WS: Funding acquisition, Methodology, Software, Writing–original draft. CL: Data curation, Project administration, Writing–original draft. SC: Methodology, Software, Writing–original draft. WJ: Software, Writing–review and editing. GW: Validation, Visualization, Writing–review and editing. TY: Data curation, Methodology, Writing–original draft, Writing–review and editing. HB: Writing–review and editing.
Funding
The author(s) declare financial support was received for the research, authorship, and/or publication of the article. This work was supported by the Modern Agriculture Industrial Technology System Program of JiangSu, Grant/Award Number: JATS[2023]315, and the Cultivation Special Project in Xinjiang Academy of Agricultural Sciences (xjnkycxzx-2022-003).
Conflict of interest
The authors declare that the research was conducted in the absence of any commercial or financial relationships that could be construed as a potential conflict of interest.
Publisher’s note
All claims expressed in this article are solely those of the authors and do not necessarily represent those of their affiliated organizations, or those of the publisher, the editors and the reviewers. Any product that may be evaluated in this article, or claim that may be made by its manufacturer, is not guaranteed or endorsed by the publisher.
Supplementary material
The Supplementary Material for this article can be found online at: https://www.frontiersin.org/articles/10.3389/fmicb.2024.1431029/full#supplementary-material
Footnotes
References
Araújo, D., Mariano, R., and Michereff, S. (2005). Inoculation methods of Acidovorax avenae subsp. citrulli in melon. Summa Phytopathol, 31, 69–73.
Bahar, O., and Burdman, S. (2010). Bacterial fruit blotch: A threat to the cucurbit industry. Israel J. Plant Sci. 58, 19–31.
Bahar, O., Goffer, T., and Burdman, S. (2009). Type IV Pili are required for virulence, twitching motility, and biofilm formation of Acidovorax avenae subsp. Citrulli. Mol. Plant Microbe Interact. 22, 909–920. doi: 10.1094/MPMI-22-8-0909
Bahar, O., Levi, N., and Burdman, S. (2011). The cucurbit pathogenic bacterium Acidovorax citrulli requires a polar flagellum for full virulence before and after host-tissue penetration. Mol. Plant Microbe Interact. 24, 1040–1050. doi: 10.1094/MPMI-02-11-0041
Block, A., and Alfano, J. R. (2011). Plant targets for Pseudomonas syringae type III effectors: Virulence targets or guarded decoys? Curr. Opin. Microbiol. 14, 39–46.
Burdman, S., and Walcott, R. (2012). Acidovorax citrulli: Generating basic and applied knowledge to tackle a global threat to the cucurbit industry. Mol. Plant Pathol. 13, 805–815. doi: 10.1111/j.1364-3703.2012.00810.x
Butler, S. M., Festa, R. A., Pearce, M. J., and Darwin, K. H. (2006). Self-compartmentalized bacterial proteases and pathogenesis. Mol. Microbiol. 60, 553–562.
Chalupowicz, L., Reuven, M., Dror, O., Sela, N., Burdman, S., and Manulis-Sasson, S. (2020). Characterization of Acidovorax citrulli strains isolated from solanaceous plants. Plant Pathol.69, 1787–1797.
Christie, P. J., Gomez, V. L., and Buchrieser, C. (2017). Biological diversity and evolution of type IV secretion systems. Curr. Top. Microbiol. Immunol. 413, 1–30.
Dodds, P. N., and Rathjen, J. P. (2010). Plant immunity: Towards an integrated view of plant–pathogen interactions. Nat. Rev. Genet. 11, 539–548. doi: 10.1038/nrg2812
Du, J., Liu, Y., and Zhu, H. (2023). Genome-based analyses of the genus Acidovorax: Proposal of the two novel genera Paracidovorax gen. nov. Paenacidovorax gen. nov. and the reclassification of Acidovorax antarcticus as Comamonas antarctica comb. nov. and emended description of the genus Acidovorax. Arch. Microbiol. 205, 1–10. doi: 10.1007/s00203-022-03379-7
Dutta, B., Scherm, H., Gitaitis, R. D., and Walcott, R. R. (2012). Acidovorax citrulli seed inoculum load affects seedling transmission and spread of bacterial fruit blotch of watermelon under greenhouse conditions. Plant Dis. 96, 705–711. doi: 10.1094/PDIS-04-11-0292
Gottesman, S. (1996). Proteases and their targets in Escherichia coli. Ann. Rev. Genet. 30, 465–506.
Guo, M. (2018). Effect of Clpaatpase in Xanthomonas Campestris pv. Malvacearum on adverse stress. Kaifeng: Henan University.
Hopkins, D. L., and Thompson, C. M. (2002). Seed transmission of Acidovorax avenae subsp. citrulli in Cucurbits. HortScience 37, 924–926.
Hou, H. (2015). Molecular mechanism of hypersensitive response induced by RepA protein encoded by Oat dwarf viurs on tobacco plants. [Ph.D. thesis]. Hangzhou: Zhejiang University.
Jain, A., and Agarwal, A. (2009). Biofilm production, a marker of pathogenic potential of colonizing and commensal staphylococci. J. Microbiol. Methods 76, 88–92. doi: 10.1016/j.mimet.2008.09.017
Ji, W., Zhao, M., Fei, N., Yang, L., Qiao, P., Walcott, R., et al. (2022). Essential Acidovorax citrulli virulence gene hrpE activates host immune response against pathogen. Int. J. Mol. Sci. 23:9144. doi: 10.3390/ijms23169144
Jiménez-Guerrero, I., Pérez-Montaño, F., Da Silva, G., Wagner, N., Shkedy, D., Zhao, M., et al. (2020). Show me your secret(ed) weapons: A multifaceted approach reveals a wide arsenal of type III-secreted effectors in the cucurbit pathogenic bacterium Acidovorax citrulli and novel effectors in the Acidovorax genus. Mol. Plant Pathol. 21, 17–37. doi: 10.1111/mpp.12877
Johnson, K. L., Minsavage, G. V., Le, T., Jones, J. B., and Walcott, R. R. (2011). Efficacy of a nonpathogenic Acidovorax citrulli strain as a biocontrol seed treatment for bacterial fruit blotch of cucurbits. Plant Disease 95, 697–704. doi: 10.1094/PDIS-09-10-0660
Johnson, T. L., Scott, M. E., and Sandkvist, M. (2007). Mapping critical interactive sites within the periplasmic domain of the Vibrio cholerae type II secretion protein EpsM. J. Bacter. 189, 9082–9089.
Kan, Y., Zhang, Y., Lin, W., and Dong, T. (2023). Differential plant cell responses to Acidovorax citrulli T3SS and T6SS reveal an effective strategy for controlling plant-associated pathogens. mBio 14:e0045923. doi: 10.1128/mbio.00459-23
Kovach, M. E., Elzer, P. H., Steven Hill, D., Robertson, G. T., Farris, M. A., Roop, R. M., et al. (1995). Four new derivatives of the broad-host-range cloning vector pBBR1MCS, carrying different antibiotic-resistance cassettes. Gene 166, 175–176. doi: 10.1016/0378-1119(95)00584-1
Li, T., and Lucius, A. L. (2013). Examination of the polypeptide substrate specificity for Escherichia coli ClpA. Biochemistry 52, 4941–4954. doi: 10.1021/bi400178q
Li, Y., Petrova, O. E., Su, S. C., Gee, W., Warunya, P., Renuka, N., et al. (2014). Bdla, DipA and induced dispersion contribute to acute virulence and chronic persistence of Pseudomonas aeruginosa. PLoS Pathog. 10:e1004168. doi: 10.1371/journal.ppat.1004168
Liu, J., Tian, Y., Zhao, Y., Zeng, R., Chen, B., Hu, B., et al. (2019). Ferric uptake regulator (FurA) is required for Acidovorax citrulli virulence on watermelon. Phytopathology 109, 1997–2008. doi: 10.1094/PHYTO-05-19-0172-R
Lo, H. H., Chang, H. C., Liao, C. T., and Hsiao, Y. M. (2022). Expression and function of clpS and clpA in Xanthomonas campestris pv. campestris. Antonie Van Leeuwenhoek 115, 589–607. doi: 10.1007/s10482-022-01725-9
Ma, Y. N., Chen, L., Si, N. G., Jiang, W. J., Zhou, Z. G., Liu, J. L., et al. (2019). Identification of benzyloxy carbonimidoyl dicyanide derivatives as novel type III secretion system inhibitors via high-throughput screening. Front. Plant Sci. 10:1059. doi: 10.3389/fpls.2019.01059
Pak, M., and Wickner, S. (1997). Mechanism of protein remodeling by ClpA chaperone. Proc. Natl. Acad. Sci. U.S.A. 94, 4901–4906.
Schaad, N. W., Postnikova, E., and Randhawa, P. (2003). “Emergence of Acidovorax avenae subsp. Citrulli as a crop threatening disease of watermelon and melon,” in Pseudomonas syringae and related pathogens, eds N. S. Iacobellis, A. Collmer, S. W. Hutcheson, J. W. Mansfield, C. E. Morris, J. Murillo, et al. (Dordrecht: Kluwer Academic Publishers), 573–581.
Schaad, N. W., Postnikova, E., Sechler, A., Claflin, L., Vidaver, A., Jones, J., et al. (2009). Reclassification of subspecies of Acidovorax avenae as A. Avenae (Manns 1905) emend., A. cattleyae (Pavarino, 1911) comb. Nov., A. citrulli Schaad et al., 1978) comb. Nov., and proposal of A. oryzae sp. Nov. Syst. Appl. Microbiol. 31, 434–446. doi: 10.1016/j.syapm.2008.09.003
Schaad, N. W., Sowell, G., Goth, R. W., Colwell, R. R., and Webb, R. E. (1978). Pseudomonas pseudoalcaligenes subsp. Citrulli subsp. Nov. Int. J. Syst. Bacteriol. 28, 117–125.
Shala-Lawrence, A., Bragagnolo, N., Nowroozi-Dayeni, R., Kheyson, S., and Audette, G. F. (2018). The interaction of TraW and TrbC is required to facilitate conjugation in F-like plasmids. Biochem. Biophys. Res. Commun. 503, 2386–2392. doi: 10.1016/j.bbrc.2018.06.166
Thomas, J. G., and Baneyx, F. (2000). ClpB and HtpG facilitate de novo protein folding in stressed Escherichia coli cells. Mol Microbiol.8 36, 1360–1370. doi: 10.1046/j.1365-2958.2000.01951.x
Tian, Y., Zhao, Y., Wu, X., Liu, F., Hu, B., and Walcott, R. R. (2015). The type VI protein secretion system contributes to biofilm formation and seed-to-seedling transmission of A Cidovorax citrulli on melon. Mol. Plant Pathol. 16, 38–47. doi: 10.1111/mpp.12159
Wang, B., Wu, G., Zhang, Y., Qian, G., and Liu, F. (2018). Dissecting the virulence-related functionality and cellular transcription mechanism of a conserved hypothetical protein in Xanthomonas oryzae pv. Oryzae. Mol. Plant Pathol. 19, 1859–1872. doi: 10.1111/mpp.12664
Wang, J., Liu, J., Zhao, Y., Sun, M., Yu, G., Fan, J., et al. (2022). OxyR contributes to virulence of Acidovorax citrulli by regulating anti-oxidative stress and expression of flagellin FliC and type IV pili PilA. Front. Microbiol. 13:977281. doi: 10.3389/fmicb.2022.977281
Wang, T., Guan, W., Huang, Q., Yang, Y., Yan, W., Sun, B., et al. (2016). Quorum-sensing contributes to virulence, twitching motility, seed attachment and biofilm formation in the wild type strain Aac-5 of Acidovorax citrulli. Microb. Pathog. 100, 133–140. doi: 10.1016/j.micpath.2016.08.039
Wickner, S., Gottesman, S., Skowyra, D., Hoskins, J., McKenney, K., and Maurizi, M. R. (1994). A molecular chaperone, ClpA, functions like DnaK and DnaJ. Proc. Natl. Acad. Sci. U.S.A. 91, 12218–12222.
Wickner, S., Hoskins, J., and McKenney, K. (1991). Function of DnaJ and DnaK as chaperones in origin-specific DNA binding by RepA. Nature 350, 165–167. doi: 10.1038/350165a0
Willems, A., Goor, M., Thielemans, S., Gillis, M., Kersters, K., and De Ley, J. (1992). Transfer of several phytopathogenic Pseudomonas Species to Acidovorax as Acidovorax avenae subsp. Avenae subsp. Nov., comb. Nov., Acidovorax avenae subsp. Citrulli, Acidovorax avenae subsp. Cattleyae, and Acidovorax konjaci. Int. J. Syst. Evol. Microbiol. 42, 107–119. doi: 10.1099/00207713-42-1-107
Yang, Y., Ji, W., Qiao, P., Fei, N., Yang, L., Guan, W., et al. (2023). Acidovorax citrulli type IV PilI PilR Interacts with PilS and regulates the expression of the pilA gene. Horticulturae 9:1296.
Yu, C. (2018). Functional analyses of hrcQ, abmR and abmK in Acidovorax citrulli. Beijing: Chinese Academy of Agricultural Sciences.
Yu, C. (2023). Function analysis of major genes in the Clp family in Acidovorax citrulli. Shenyang: Shenyang Agricultural University.
Yu, C., Wang, N., Wu, M., Tian, F., Chen, H., Yang, F., et al. (2016). OxyR-regulated catalase CatB promotes the virulence in rice via detoxifying hydrogen peroxide in Xanthomonas oryzae pv. Oryzae. BMC Microbiol. 16:269. doi: 10.1186/s12866-016-0887-0
Zhang, M. (2018). Functions of stress related genes mediated by ClpA ATPase in Xanthomonas campestris pv. Malvacearum. Kaifeng: Henan University.
Keywords: Paracidovorax citrulli, ClpA, RepA, virulence, regulate
Citation: Ziye S, Yuqiang Z, Shitong W, Ling C, Chenchao S, Jun W, Weirong G, Yanli T and Baishi H (2024) ClpA affects the virulence of Paracidovorax citrulli on melon by regulating RepA. Front. Microbiol. 15:1431029. doi: 10.3389/fmicb.2024.1431029
Received: 11 May 2024; Accepted: 20 June 2024;
Published: 23 July 2024.
Edited by:
Gang Yu, Shanghai Jiao Tong University, ChinaReviewed by:
Xiaoxiao Zhang, Guangxi University, ChinaWang Peihong, Shanghai Jiao Tong University, China
Copyright © 2024 Ziye, Yuqiang, Shitong, Ling, Chenchao, Jun, Weirong, Yanli and Baishi. This is an open-access article distributed under the terms of the Creative Commons Attribution License (CC BY). The use, distribution or reproduction in other forums is permitted, provided the original author(s) and the copyright owner(s) are credited and that the original publication in this journal is cited, in accordance with accepted academic practice. No use, distribution or reproduction is permitted which does not comply with these terms.
*Correspondence: Tian Yanli, tianyanli@njau.edu.cn