- 1Programa de Doctorado en Biociencias Moleculares, Universidad Andres Bello, Santiago, Chile
- 2Advanced Center for Chronic Diseases, Facultad de Ciencias Químicas y Farmacéuticas & Facultad de Medicina, Universidad de Chile, Santiago, Chile
- 3Instituto de Farmacia, Facultad de Ciencias, Universidad Austral de Chile, Valdivia, Chile
- 4Laboratorio de Microbiología Molecular, Facultad de Ciencias de la Vida, Universidad Andres Bello, Santiago, Chile
- 5Departamento de Ciencias Biológicas Animales, Facultad de Ciencias Veterinarias y Pecuarias, Universidad de Chile, Santiago, Chile
- 6Instituto de Investigación Interdisciplinar en Ciencias Biomédicas SEK (I3CBSEK), Facultad de Ciencias de la Salud, Universidad SEK, Santiago, Chile
- 7Escuela de Tecnología Médica, Facultad de Salud, Universidad Santo Tomas, Santiago, Chile
- 8Escuela de Química y Farmacia, Facultad de Medicina, Universidad Andres Bello, Santiago, Chile
The speciation of Salmonella occurred by acquisition of genomic islands from other bacterial species and continued to diverge into subspecies and serovars with diferent range of host. S. enterica serovar Typhimurium (STM) is a generalist pathogen infecting hosts that include birds, mice, and humans, whilst S. enterica serovar Typhi (STY) is a restricted-host pathogen, infecting only humans. Despite their ranges of hosts, STM and STY possess 97–98% identity. Gain of genes by horizontal transference and loss of genes by mutations, are believed essential for differentiation of Salmonella. Salmonella pathogenicity island 3 (SPI-3) is an example combining these two processes. SPI-3 encodes misL and marT, among other genes. In STM, misL is required for gut colonization. Furthermore, protein MarT, positively regulates expression of misL by binding to misL-promoter. On the other hand, in SPI-3 of STY, marT and misL are pseudogenes. Interestingly, the gene t3766 (gene involved in resistance to H2O2) is present only in STY and is negatively regulated when marTSTM is heterologously expressed in STY. Based on the view that MarT might regulate genes implicated in virulence, this work searched for new genes regulated by MarT. In silico searches for possible MarT target genes were performed, and 4 genes were selected for further analysis as they contained at least 2 copies of the consensus MarT-binding sequence in their promoters. Mutating marT in STM or heterologously expressing marTSTM in STY confirmed that MarT negatively regulates ORF STY1408 or STM14_2003, its homologue in STM. STY1408 encodes for a putative protein with homology to methyl accepting chemotaxis proteins, which participate in chemotaxis and motility. Therefore, STY1408 was named mrmI (MarT-regulated motility gene I). Motility assays confirmed that the product of mrmI modulates motility. In addition, in vitro infection of cells with STM and STY mutants in mrmI reduces association with cells at 1, 3 and 24 h post-infection. Oral infection of mice showed that a mrmI null mutant was defective in producing systemic disease. Therefore, we conclude that MarT regulated mrmI, is involved in virulence of Salmonella. While pseudogenization of marT might modulate the fitness of narrow host range STY.
1 Introduction
Salmonella is a genus consisting of only two species: Salmonella bongori which infects cold-blooded animals and Salmonella enterica which infects warm-blooded animals. The latter is divided into six subspecies, including the subspecies enterica, which includes serovars Typhimurium (S. typhimurium or STM, within the text) and Typhi (S. typhi or STY, within the text) (Boyd et al., 1993). STM is considered a generalist pathogen since it can infect birds, mice, and humans, among other hosts. In humans, STM produces self-limiting gastroenteritis, while in mice, it produces a systemic disease, resembling the fever symptoms of human typhoid. On the other hand, STY is a restricted host-range pathogen, because it only infects humans, producing the systemic disease typhoid fever which can be lethal if not treated. Despite the differences in host range and pathogenicity between both serovars, they possess at least 97% identity between their shared genes (McClelland et al., 2001; Parkhill et al., 2001). The limited differences between them could be explained by acquisition of new genes by Horizontal Gene Transfer (HGT) and the loss of genes by mutations. An example of these two genomic events is Salmonella Pathogenicity Island 3 (SPI-3), shown in Figure 1A. SPI-3 is a horizontally-acquired fragment of 17 kb localized at the selC locus of the chromosome, containing 10 functional genes in STM (Blanc-Potard et al., 1999). SPI-3 is also present in STY; however, in this serovar 3 of the 10 genes within SPI-3STM are pseudogenes (ΨmisL, ΨmarT and ΨcigR), and thus their full-length gene products are lost in STY. Furthermore, the gene t3766 (also named surV), involved in resistance to H2O2 is present only in STY SPI-3 (Tükel et al., 2007; Retamal et al., 2010; Ortega et al., 2016), in a clear example of combining gain and loss of genes, at only one genetic locus.
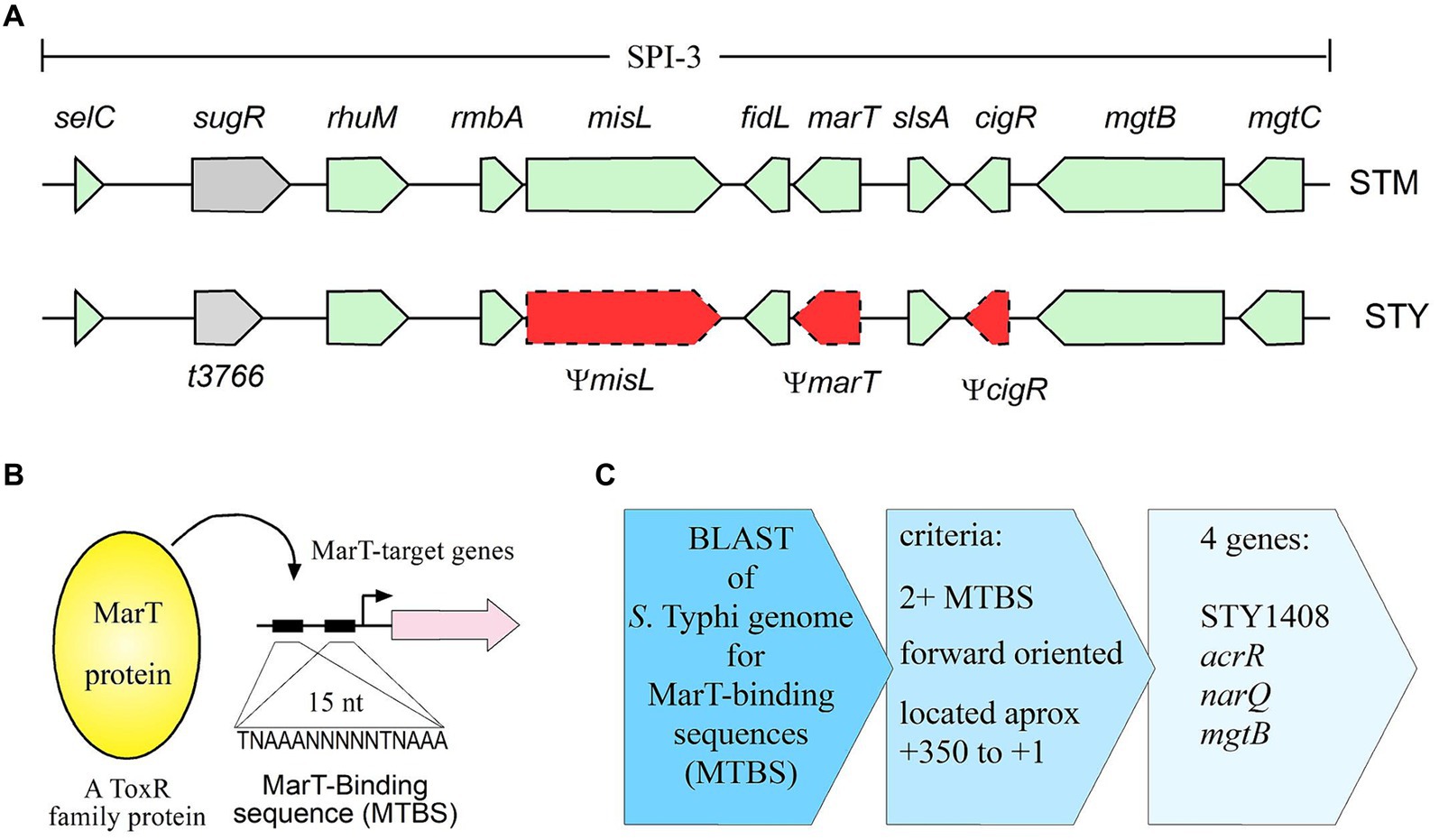
Figure 1. Genetic context of SPI-3 of S. typhimurium and S. typhi and flow chart depicting the search for MarT-binding sites in the vicinity of putative target genes. (A) Along with misL and cigR, marT is a pseudogene in S. typhi (STY), but not in S. typhimurium (STM). Gray shaded and green box, functional genes, red box with dotted lines, pseudogenes. (B) MarT is a ToxR-family transcription factor that binds to regulatory regions (MTBS) of target genes. (C) Schematic representation of the steps followed to identify genes regulated by MarT.
The marT gene (intact in STM, but inactivated in STY) encodes for a transcriptional regulator possessing 41% similarity with ToxR, a transcription factor of Vibrio cholerae which regulates the expression of cholera toxin, colonization factors and porins (Blanc-Potard et al., 1999). Transcription factors in the ToxR-family recognize the consensus sequence TNAAANNNNNTNAAA in the promoter of target genes (Goss et al., 2013). MarT positively regulates misL expression in STM by directly-binding to the sequence TNAAANNNNNTNAAA (Tükel et al., 2007). The misL gene, located in SPI-3, encodes for an adhesin required for the efficient colonization of birds and mice (Morgan et al., 2004; Dorsey et al., 2005). These observations suggest that MarT regulates the expression of genes involved in STM virulence. Transcriptomic analyses of STM showed that marTSTM expression is 10-times higher in bacteria harbored in macrophages (Eriksson et al., 2003) reinforcing the idea that marT is involved in the virulence of STM.
In silico analyses indicate that MarT is a protein of 260 amino acids, with a conserved DNA-binding domain between residues 32 and 180 (Tükel et al., 2007). However, marTSTY presents 12 nucleotide substitutions compared with marTSTM resulting in nonsense mutations and a truncated MarTSTY protein. The 60 nt truncated MarTSTY partially loses the DNA binding domain and the regulatory functions (Tükel et al., 2007; Ortega et al., 2016). Heterologous expression of marT downregulates surV, a gene located only in SPI-3 of STY that is involved in resistance to hydrogen peroxide (Ortega et al., 2016), again suggesting that marT is involved in virulence, by modulating transcription of target effector-genes. Therefore, considering that marT is a functional gene in STM, but is a pseudogene in STY, that MarT positively regulates misL expression in STM, and that heterologous expression of marT negatively regulates surV expression in STY, the goals of this study are to search for and to characterize new genes regulated by MarT in S. enterica and to discover how pseudogenization of marT contributes to the evolution of S. enterica in becoming a pathogen restricted to human hosts.
2 Materials and methods
2.1 Cellular cultures and strains
All the strains used in this work were routinely cultured at 37°C with aeration in LB-medium (NaCl, 5 g/L; yeast extract, 5 g/L and peptone 10 g/L; prepared in phosphate buffer pH 7.0 all reagents obtained from Sigma-Aldrich). When needed, LB was solidified by adding agar 15 g/L (Sigma-Aldrich). For microaerophilic conditions, mineral oil was added on top of LB broth cultures and bacteria used to infect eukaryotic cells and mice.
2.2 In silico analyses
Using Basic Local Alignment Search Tool (BLAST) (Altschul et al., 1990), the consensus sequence TNAAANNNNNTNAAA was aligned against the Salmonella Typhi CT18 reference genome. The classical parameters were used, searching for a 100% match between the consensus sequence and the reference genome (considering the degenerated nucleotides N).
2.3 RNA isolation, reverse transcription, and real-time PCR
Total RNA from saturated bacterial cultures was extracted using phenol (Invitrogen) at 65°C. The nucleic acids were precipitated using absolute ethanol (Merck) at −80°C overnight and resuspended in nuclease-free water (Thermo Fisher Scientific). After the samples were treated with Turbo RNase Free DNase (Ambion) to remove the DNA, the RNA was quantified by spectrophotometry. Integrity of RNA was determined by electrophoresis using agarose gels (agarose 1% w/v; hypochlorite 1% v/v and ethidium bromide 0.5 μg/mL) with 500 ng per sample. Reverse transcription was performed using 1 μg of DNase-treated RNA with Superscript II RT (Invitrogen) at 50°C for 50 min. Relative quantification of each mRNA was performed using the Brilliant II SYBR Green QPCR Master Reagent Kit (Agilent). Real Time PCR was undertaken in a volume of 10 μL containing 1 μL of diluted cDNA (1:1000) and specific primers to detect each gene (Supplementary Table S1) in an Eco™ Real-Time PCR System (Illumina). Amplification efficiency was calculated from a standard curve constructed by amplifying serial dilutions of RT-PCR products. Normalization of expression was achieved against expression of 16S rRNA as described (Pfaffl, 2001; Hidalgo et al., 2016). Experiments were performed with at least three biological replicates with technical triplicates.
2.4 Construction of mutant strains
All mutants were derived from either Salmonella enterica serovar Typhi strain STH2370 or Salmonella enterica serovar Typhimurium strain 14028s. STY1408 mutant strains were obtained by facilitated allelic exchange using the Red-Swap method (Datsenko and Wanner, 2000). Briefly, 60 bp primers (Supplementary Table S1), whose 40 bp at the 5′ end exhibited 100% identity to target genes (STM14_2003 or STY1408), while the 20 bp at the 3′ end aligned to plasmids pKD3 or pKD4 were used to PCR amplify the cam or kan cassettes. Amplicons were electroporated into electrocompetent S. typhimurium ATCC14028s or S. typhi STH2370 harboring pKD46. Recombinant bacteria were selected on LB-agar + kan 50 μg/mL or LB-agar + cam 20 μg/mL. PCR amplification from colonies was carried out using primers RT-STY1408R + RT-STY1408F to verify mutations. Strains and their phenotypes are summarized in Table 1. In addition, a modification of the Red-Swap method (Datsenko and Wanner, 2000) was used to replace the STM14_2003 promoter with the tetRA cassette. Briefly, 60 bp primers (Supplementary Table S1), whose 40 bp at the 5′ end exhibited 100% identity to target gene STM14_2003, while the 20 bp at the 3′ end aligned to the terRA cassette present in the S. typhimurium 14028s yabB :: tetRA mutant previously described (Hidalgo et al., 2004, 2016). Ones the STM14_2003 promoter was replaced with the tetRA cassette, tetA gene was replaced with the kan cassette as described above. Finally, the kan cassette was scised to produce a mutant containing the gene STM14_2003 under control of the tetracycline dependent promoter PtetA. We have successfully created this kind of genetic constructions previously (Millanao et al., 2020). Details of the production of this mutant and the promoter sequences interchanged can be found in Supplementary Figures S1, S2. kanamycin, chloramphenicol and tetracycline were obtained from Sigma.
2.5 Motility assay
Two microliter of bacteria grown to OD600 of 0.4, equivalent to 3×105 CFU, were inoculated at the center of a Petri plates with 20 mL of semi-solid LB-agar (0.3% agar). The plates were dried for 30 min, incubated overnight at 37°C, and the diameter of bacterial growth was measured.
2.6 In vitro cell infection
The gentamicin protection protocol was carried out as previously described (Contreras et al., 1997). Briefly, bacteria were grown in microaerophilic conditions to OD600 of 0.2. Then, 5×104 HEp2 cells (5×104) were plated in each well of a 96-well plate using DMEM +10%FBS at 37°C and 5% CO2. Cells monolayers were infected next day with a bacteria-to-cell multiplicity of infection (MOI) of 100. One hour after infection, a group of cells monolayer were washed 3 times with 100 μL PBS and lysed in 100 μL of deoxycholate 0.5% p/v. Lysates were serially diluted and 5 μL plated on LB-agar. The plates were incubated for 16 h at 37°C before counting the CFUs. The same procedure was repeated for the other groups of cells infected, only that after the first hour of infection, the cells were maintained in medium with gentamicine at 50 μg/mL until lysis at 3 and 24 h postinfection. All culture medium, FBS, PBS and trypsin were acquired from Thermo Fisher Scientific. Plastic material was provided by Falcon.
2.7 In vivo infection
Microaerophilic cultures were grown as in the in vitro cell infection protocol, and 5×104 bacteria were inoculated in each BALB/c mouse. In the case of orally infected mice, they were inoculated with a volume of 200 μL using a straight irrigation cannula (0.6 mm) with an oval tip on a tuberculin syringe. Orally infected mice were euthanized 5 days post-infection. In the case of Intraperitoneally infected mice, mice were inoculated with 100 μL using a tuberculin syringe (Cranberry). Intraperitoneally infected mice were euthanized 1 day post-infection. Weight of mice was measured each day during the assay. After sacrificed, mice were dissected to extract the liver and the spleen which were homogenized in 5 mL per every 1 g of tissue and 1 mL per every 0.1g of tissue, respectively. After serial dilution, 5 μL of each dilution was seeded on LB agar plates and incubated overnight at 37°C. Finally, CFU were counted to compare colonization of mutants versus wild-type (WT) strains. Experiments using the S. typhimurium mrmITD mutant were performed by oral infection as described above, with the addition that a group of mice started treatment with anhydrotetracycline (AHT, Thermo Fisher Scientific) at a concentration of 4 mg/mL in their drinking water. Experiments were conducted using protocols approved by the institutional bioethics committee at Universidad Andres Bello as described in the approval certificate 023/2015.
2.8 Statistical analyses
Two-tailed Student t-tests with α = 0.05 were employed, using GraphPad Prism 5.00.288.
3 Results
3.1 Identification of MarT target genes
The alignment of MarT binding sites (MTBS) in the STY CT18 reference genome identified 106 potential candidate target genes, all of which contain at least part of the canonical TNA3N5TNA3 sequence in their promoters. To filter and identify the best candidates, the promoters of confirmed MarT-target genes, such as misL and surV, were analyzed to define the most-characteristic parameters, such as position (distance from initial ATG), orientation, multiplicity, and whether the full or half of the consensus sequence is present (Supplementary Figure S3). In the misL promoter, two complete consensus sequences were found. The most-distal consensus sequence was located aproximately 360 nt upstream of the initial ATG of the misL-coding sequence (Supplementary Figure S3A). It is important to point out that although in vitro binding of MarT at the misL promoter is observed (along with biological effects after overexpressing misL) (Tükel et al., 2007), there is no significant expression under several conditions, as it can be found the the Salcom web site from Hinton’s Lab (Kröger et al., 2013).
For the surV promoter, only half MTBSs (TNAAA) were found 70, 110 and 140 nt upstream from initial GTG of the surV-coding sequence (Supplementary Figure S3B). Therefore, we searched for MTBS sequences in the forward orientation, while either the presence of a complete consensus sequence or just half of the consensus sequence were considered. After filtering the initial global search (Figures 1B,C), only 25 harbored MTBS located 350 upstream from the initial codon, yet only 4 presented two or more repeats (considering at least half the binding site). Thus, only 4 genes complied with all established MTBS parameters (Table 2 and Figure 1C). Out of the four genes identified, mgtB, narQ, and acrR have known functions (Snavely et al., 1991; Villagra et al., 2008), while the remaining gene (STY1408 and its STM homologous STM14_2003) have only been assigned putative functions based on sequence homology (Table 2).
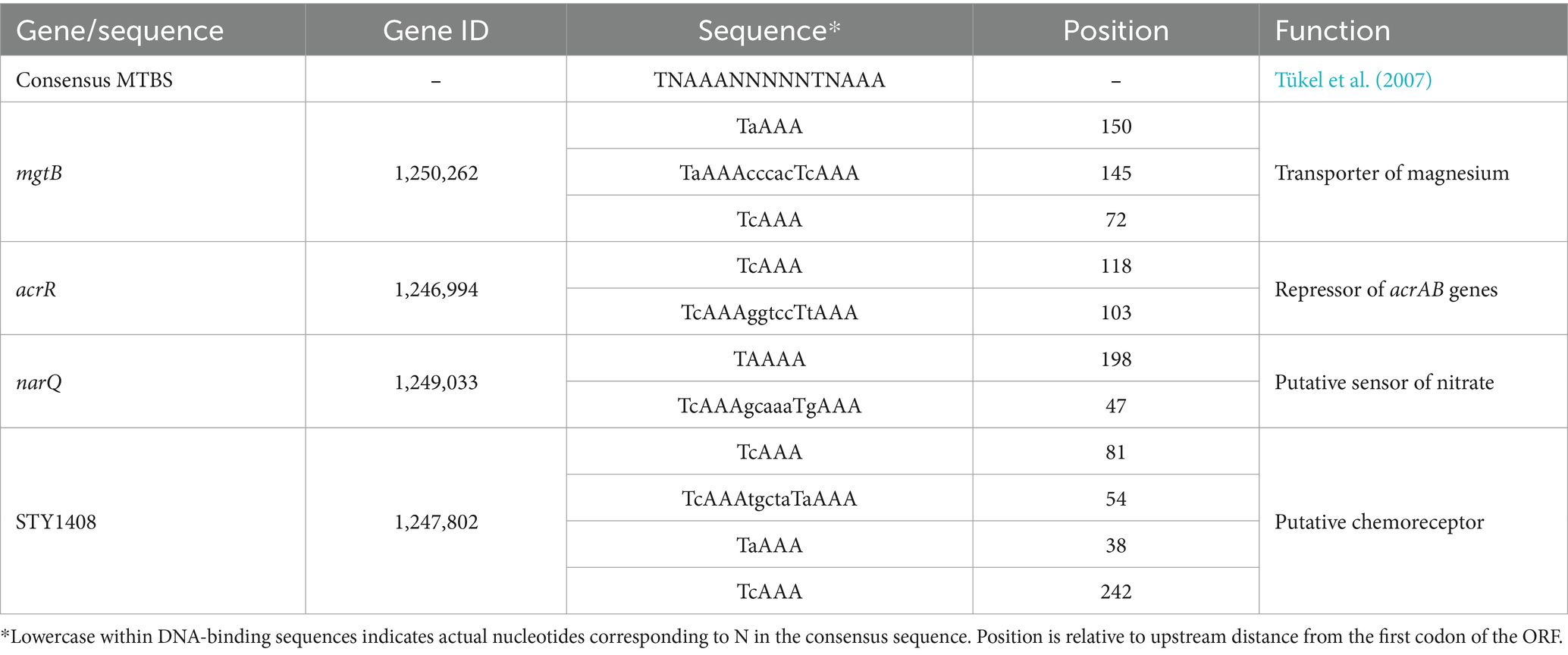
Table 2. Putative MarT-target genes and their DNA-binding sequences found in the S. typhi CT18 genome.
To empirically confirm the regulation of the 4 final target candidates by MarT, their expression was studied in STM, STM ΔmarT, STY STH2370 and STY STH2370 ΔΨmarT::marTSTM. RNA isolated from stationary phase cultures was used to measure expression of genes mgtB, acrR, narQ and STY1408 (or its STM homologous gene STM14_2003). Expression of the four genes changed significantly in STM ΔmarT compared with the STM WT strain (gray versus black bars in Figure 2). For genes mgtB and acrR, MarT upregulates their expression, as expression was significantly decreased in the MarT null mutant. For narQ and STM14_2003 (STM homologous of STY1408), downregulation by MarT was observed, as the marT deletion increased the expression of both genes. Analogously, expression of the four genes was examined in STY WT which does not express a full-length marT gene and was compared with expression in STY heterologously expressing marTSTM. In STY, only STY1408 suffered changes in expression as a function of marT expression. In STY, the heterologous expression of marTSTM reduced expression of STY1408. This is consistent with the expression of STM14_2003 in STM which is diminished when marT is intact. In conclusion, a new gene downregulated by MarT in STM and STY was discovered, and the subsequent experiments were designed to understand the phenotypes associated with STY1408 and STM14_2003, in STY and STM, respectively.
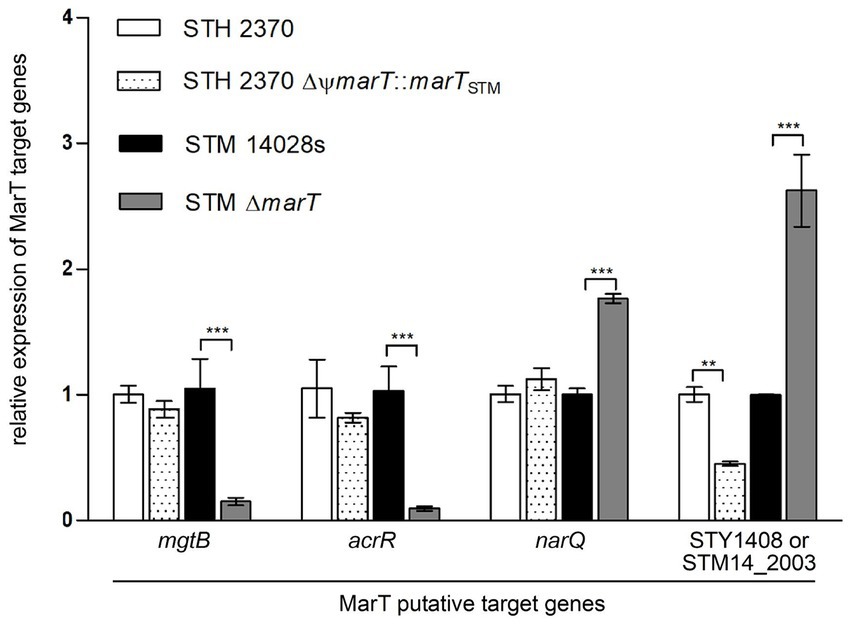
Figure 2. Quantitative PCR of putative MarT-target genes. Expression of the four genes selected was assessed in S. typhi after restoring marT with marTSTM. Analogously, expression of the four genes was assessed in S. typhimurium after inactivating endogenous marT. A representative assay of 2 independent biological replicates is shown. **p < 0.005, ***p < 0.0005.
3.2 Genetic and phenotypic characterization of STY1408 mutants
As mentioned above, the function of STY1408 is unknown, although the analyses of the nucleotide and predicted amino acid sequences indicate high identity and similarity with MCP-family chemosensors, such as Tsr, CheD and Aer. The identity, similarity and coverage of their sequences is summarized in Table 3. More details of aligned sequences can be found in Supplementary Figure S4, for both DNA-coding sequences and aminoacidic sequences. MCP stands for Methyl-accepting chemotaxis protein since proteins in this family participate in chemotaxis and have a methylated glutamate residue in the C-terminal domain. Biochemical and structural modeling studies suggest all proteins in the MCP-family form homodimers, although they might form superstructures by combining 2 or 3 dimeric structures (Derr et al., 2006; Alexander and Zhulin, 2007). This type of protein detects nutrients and other molecules to transduce signals that in turn modulate bacterial motility (Alexander and Zhulin, 2007). Prediction of transmembrane domains using three different on-line platforms indicate that STY1408 may span the inner membrane twice (Sonnhammer et al., 1998; Ikeda et al., 2003, p. 20; Blum et al., 2021) (Figure 3).
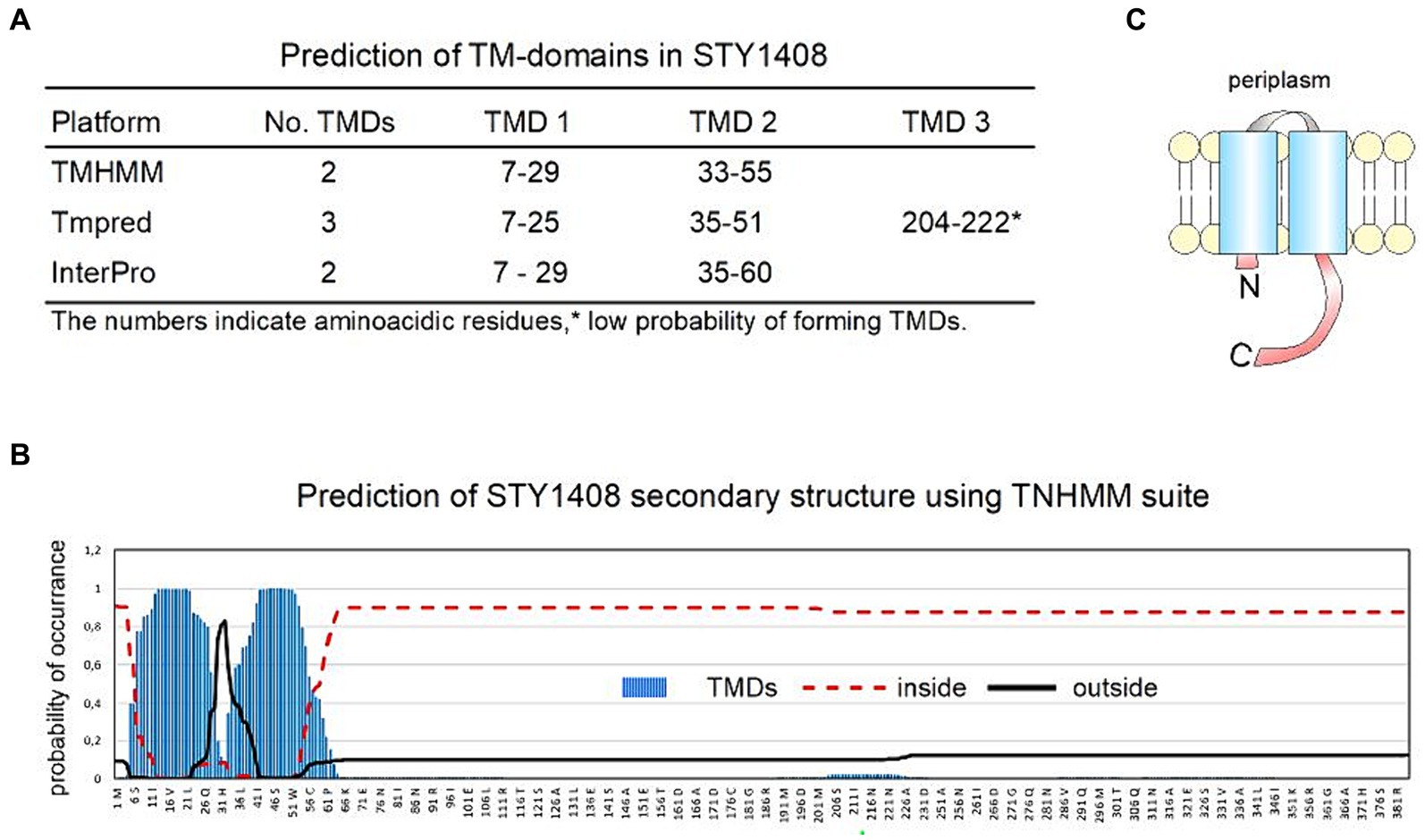
Figure 3. In silico sequence and structure analysis of the putative protein encoded by mrmI (STY1408). Sequence analysis predicts this gene encodes for a membrane protein, probably in the MCP family. (A) Three different programs (Sonnhammer et al., 1998; Ikeda et al., 2003; Blum et al., 2021) predict a membrane protein with 2 transmembrane domains (TMD). (B) Details of the probability of amino acid residues being located inside, outside or as part of a TMD according to TNHMM suite (Sonnhammer et al., 1998). (C) Topology of the predicted protein.
Therefore, we named STY1408 as “MarT-regulated motility gene I” or mrmI. Next, the motility of STM and STY mutants in the mrmI gene was evaluated. As shown in Figure 4, inactivation of mrmI reduced swimming in both serovars (by 40% for STY and by 20% for STM), when compared with their WT counterpart. The same assay was performed with strains STY ΔmarT and STY STH2370 ΔΨmarT::marTSTM; the results are consistent with mrmI regulation by MarT. Since MarT downregulates mrmI expression, inactivation of marT in STM resulted in increasing motility compared to STM WT, while heterologous expression of mrmI in STY produced a slight, yet not significant, reduction of motility (Supplementary Figure S5). With these observations, we conclude that mrmI is involved in motility, while marT is indirectly involved in this cellular function in STM.
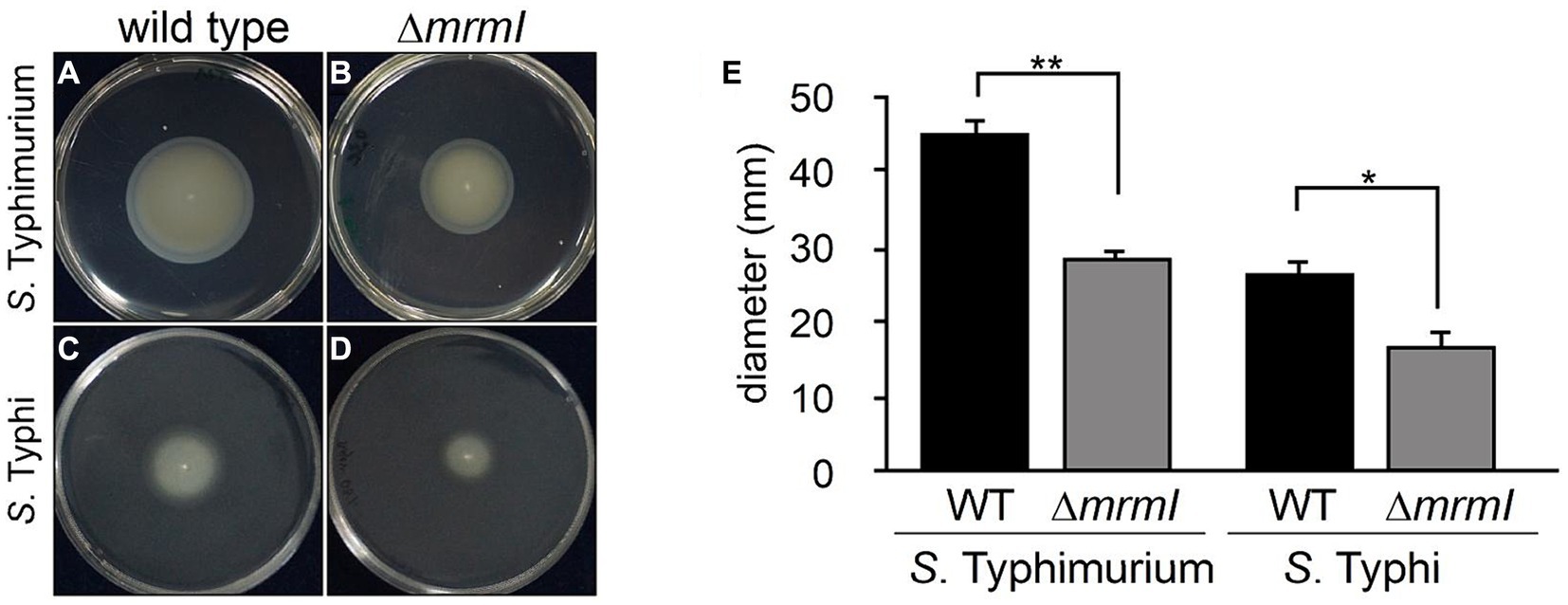
Figure 4. Soft-agar motility assay. The gene mrmI is involved in motility of S. typhimurium. Growth of STM and its derivative mutants on semi-solid agar plates. (A–D) show growth of STM WT, STM ΔmrmI, STY and STY ΔmrmI, respectively. (E) growth compared to WT strains measured as diameter in millimeters. *p < 0.05, **p ≤ 0.01. The figure shows a representative assay of 3 independent biological replicates, each with 3 technical replicates.
3.3 The mrmI genes of Salmonella are required for infection of cells in vitro
As motility of Salmonella is directly involved in the process of infecting epithelial cells, the impact of inactivating mrmI on invasion was assessed. HEp-2 cells were infected with mrmI mutant strains of STM and STY and with their WT counterparts, to determinate whether mrmI is involved in the infective process (Olsen et al., 2013; Rivera-Chávez et al., 2013; Ryan et al., 2016). Interestingly, after 1 h of infecting the cells, STM and STY defective in mrmI presented lower (although statistically non-significant) association with HEp-2 epithelial cells, compared with their WT counterpart (Figure 5A). Impressively, after 3 h of infecting the cells, the association of STM and STY defective in mrmI was significantly impaired, compared with WT STM and WT STY, a feature that was also prevalent after 24 h (Figures 5B,C). Therefore, the results indicate that mrmI is involved in the process of infecting cells in vitro, in both STM and STY.
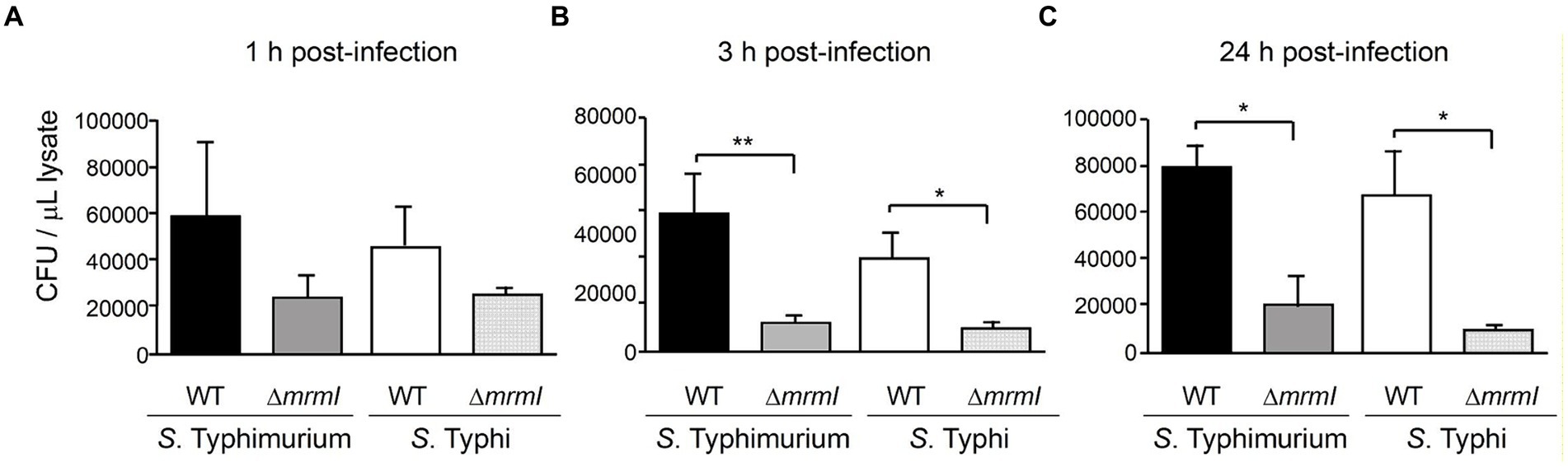
Figure 5. Gentamicin protection assay indicates mrmI mutants are defective in invasion and proliferation into cultured cells. Bacteria associated to cells 1- (A), 3- (B) and 24-h (C) post-infection were assessed. In (A), adherence is not significatively affected by deletion of mrmI in either STM or STY serovars. In (B,C), defective invasion, and proliferation of mrmI mutants, inside cultured cells, is shown. Representative assays of 3 independent biological replicates are shown *p < 0.05, **p < 0.01.
3.4 The mrmI gene of Salmonella is required to infect mice oraly
As shown above, mrmI is a new gene regulated by MarT that plays an important role in bacterial motility and in vitro infection of cells. For these reasons, we tested whether expression of mrmI is involved in the virulence of Salmonella, using the in vivo STM-mouse model of infection. Groups of mice were infected orally, and the weight of each animal was monitored daily for the next 5 days. Impaired virulence of STM ΔmrmI was observed, as less weight-loss was observed in mice infected with the mutant, compared with mice infected with STM WT; this trend was maintained throughout the 5 days of the experiment (Figure 6A). Day five post-infection, mice were euthanized, dissected, the liver and spleen macerated, and serially diluted suspensions plated on LB-agar to obtain CFU the next day. As shown in Figure 6B, STM ΔmrmI mutant was present in the liver at approximately 100 times lower levels compared to the WT strain. Similarly, STM ΔmrmI was present in the spleen at aproximately 10 times lower compared to the STM WT. However, when mice were infected intraperitoneally, deletion of mrmI only produced a marginal decrease in the infection of STM in liver and spleen (Figure 6C). To demonstrate the dependency of mrmI during the process of mice infection, oral infection was performed with the S. typhimurium mrmITD mutant (Figure 7A). In this mutant, transcription of mrmI depends on the presence of tetracycline. Therefore, a group of mice started treatment with anhydrotetracycline (AHT) at a concentration of 4 mg/mL in drinking water 24 h before oral infection. Mice were dissected 5 days after infection to study bacteria in the liver and spleen (Figure 7B). In the presence of AHT, bacterial counts in the liver and spleen were approximately 64 and 128 times higher, respectively, compared to control mice on a normal diet (Figure 7). This result indicates that the expression of mrmI increases deep organ invasion. Finally, mice were orally infected with STM ΔmarT, to study the involvement of MarT in virulence of Salmonella. Day five post-infection, liver and spleen bacteria were collected and processed as before. Unexpectedly, STM ΔmarT presented a less invasive phenotype (Supplementary Figure S6). Therefore, regulation of genes other than mrmI, might be responsible for the final phenotypes of marT null mutants. We conclude that mrmI plays a crucial role during invasion of STM via the mouse intestine.
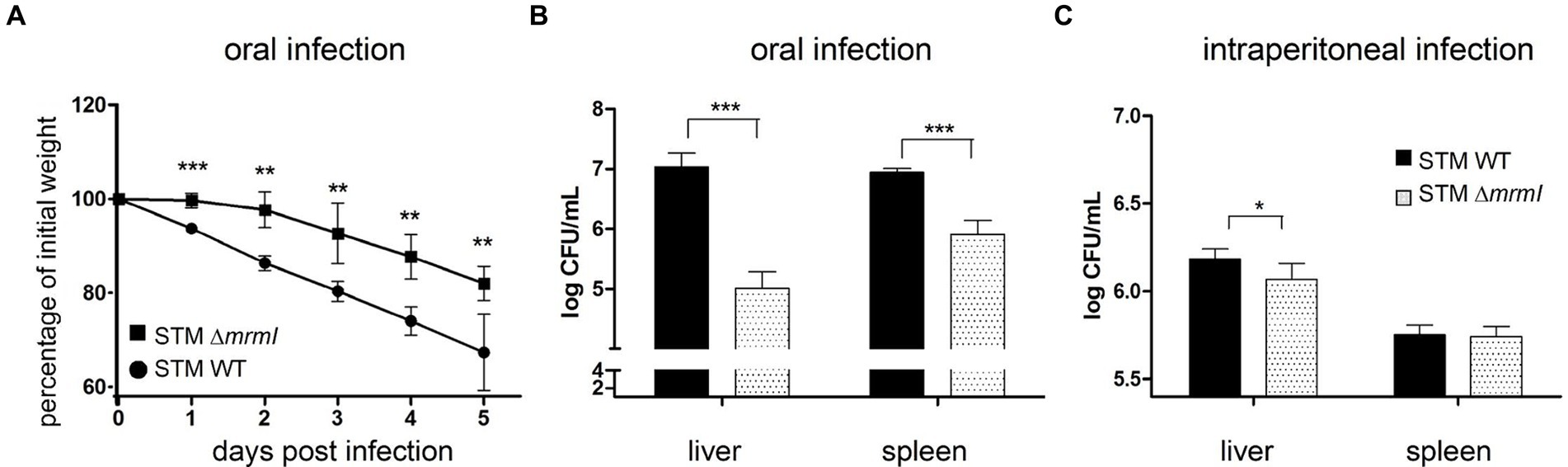
Figure 6. In vivo colonization of liver and spleen. Deletion of mrmI reduces virulence of S. typhimurium in orally infected mice. (A), Weight evolution for 5 days after oral infection. (B,C), CFU/mL of STM and STM ΔmrmI collected from oral and intraperitoneal infections, respectively, from liver and spleen. Note that the ordinate axes in panels (B,C) are in Log10 scale, in CFUx10,000. For each experiment, a representative assay of 3 biological independent replicates is shown. *p < 0.05, ***p < 0.005.
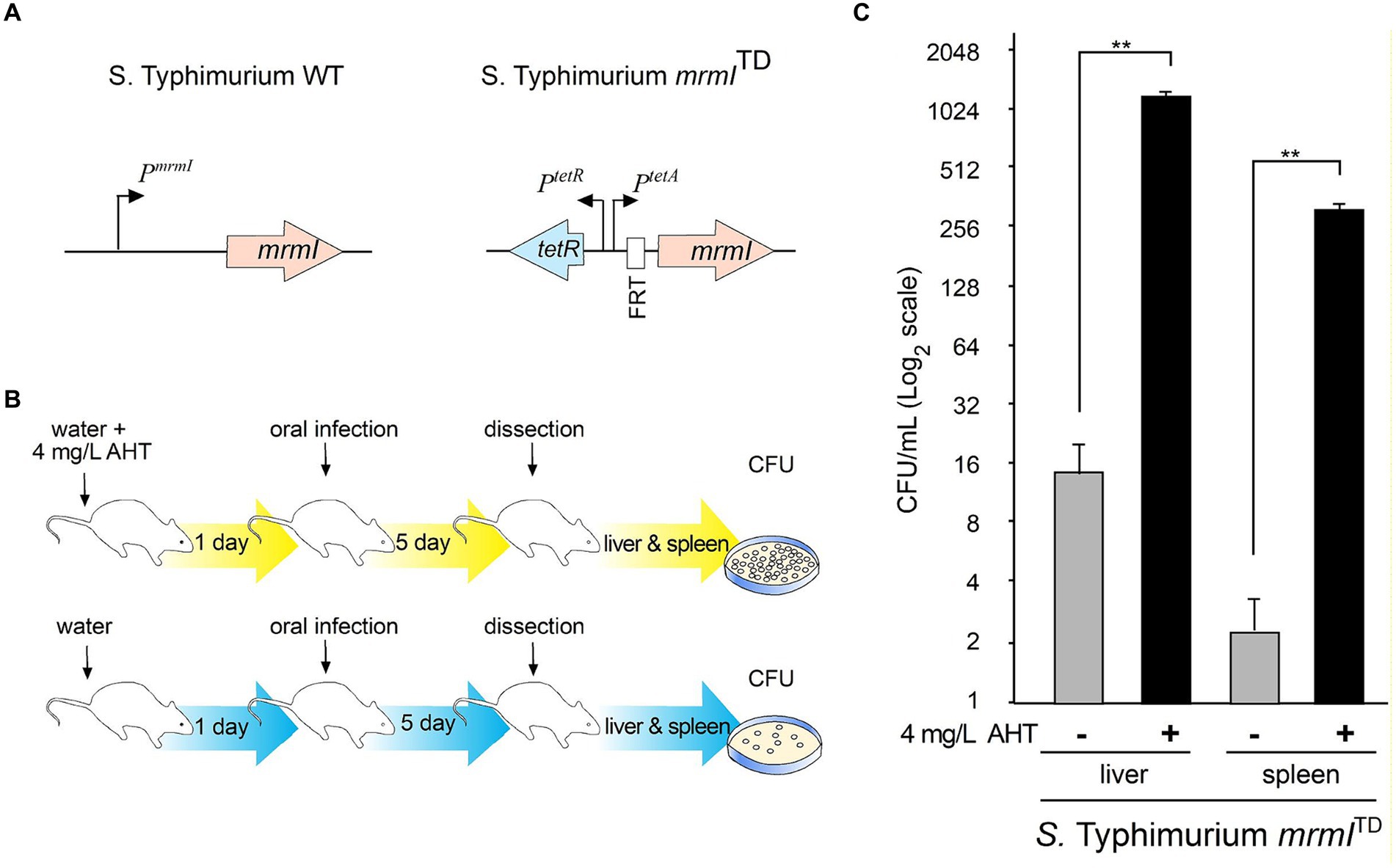
Figure 7. Complementation in cis of mrmI by using a tetracycline inducible system in vivo. The S. typhimurium mrmITD was used to orally infect mice maintained on a diet with AHT to assess the presence of bacteria in liver and mice. In (A), details of the genetic construction that allow for expression of mrmI from the tetA promoter. In (B), experimental setting to assess invasion of liver and spleen. In (C), number of CFU in liver and spleen. The plot is in log2 scale to facilitate comparing induced and control conditions, in CFUx1,000. The experiment was performed twice with 3 mice each attempt. The results shown are representative from one experiment with technical triplicates. **p < 0.01.
4 Discussion
In silico analysis identified 4 candidate genes bearing at least one complete MarT-binding sequence in their promoter region. ‘The extension of the MarT-binding sequence (15 nt), its location, and its sometimes directly repeated nature suggest that MarT could act as a dimer to exert its function as a transcriptional regulator. In addition, the 10-nucleotide per turn DNA structure of Watson and Crick (Watson and Crick, 1953) is consistent with this idea. In fact, MarT belongs to the ToxR protein family, as it shares 32% amino acid similarity with ToxR (Blanc-Potard et al., 1999; Tükel et al., 2007). In V. cholerae, ToxR forms homodimers when overexpressed, while at normal expression levels, ToxR forms heterodimers with ToxS in the periplasm (Ottemann and Mekalanos, 1996). Furthermore, ToxR mutants with the inability to dimerize show defective DNA-binding capacity with downregulation of its regulon, in V. cholerae (DiRita and Mekalanos, 1991) reinforcing the idea that MarT could exert its function as a dimer.
MarT possesses a DNA binding domain with a winged helix-turn-helix. MarT regulates expression of surV and might bind to regulatory sequences by recognizing heptameric direct repeats present in the surV promoter, as is the case of ToxR regulation of the cholera toxin, which recognizes the heptameric sequence TTTTGAT (Miller et al., 1987). Other proteins in the ToxR family recognize sequences different to MarT-binding sequences (Tükel et al., 2007). Such is the case of TcpP, which recognizes the sequence TGTAANNNNNNTGTAA (Goss et al., 2010) and PhoB which targets the TGTCANNNNNNTGTCA (Diniz et al., 2011). Thus, we speculate that the consensus MarT-binding sequence might differ from the consensus for ToxR-binding sequences. MarT-binding sequences, full direct repeats, and half direct repeats are located upstream from mgtB, acrR, narQ and mrmI. However, MarT is a positive regulator of mgtB and acrR, but a negative regulator of narQ and mrmI (Figure 2). How binding site structure, interactions and topology affect MarT as a negative or positive regulator, is unknown. The heterologous expression of marTSTM in STY adds another level of complexity to understanding the effects of MarT, as it acts as a positive regulator only of mrmI, with no significant effect on transcription of mgtB, acrR or narQ.
The mrmI gene has 98% identity with members of the MCP-protein family, which are implicated in the sensing of sugars and motility. Deficient motility was observed in mrmI mutants of both STM and STY, possibly determined by a defective detection of sugars, which is characteristic of the chemotaxis function of MCPs (Lux et al., 1995). MCPs sense sugars including maltose, ribose and galactose (Parkinson, 1976).
Reports show motility or chemotaxis related genes are essential for the STM infective cycle, since their participation in the colonization process is crucial, both in vitro and in vivo. This was shown as mutants in motility and chemotaxis related genes are less invasive (Olsen et al., 2013). In fact, a study in S. enterica serovar Enteritidis shows that pathogenic strains express higher levels of virulence transcriptional regulators and virulence-involved genes, such as motility and fimbriae genes, compared to Low-Pathogenic strains (Barbosa et al., 2017).
The result of in vitro infection indicates that both serovars Typhi and Typhimurium defective in mrmI gene presented a poor association with cultured cells 1, 3 and 24 h post infection. Further characterization of S. typhimurium mrmI in the murine infection model revealed that mrmI is important in the invasion via the intestinal barrier, as only orally infected mice showed important defects in liver and spleen invasion. On the other hand, colonization of liver and spleen changed only slightly after intraperitoneal infection with S. typhimurium mrmI. In addition, the preliminary results of intraperitoneally infected mice with 1,000 CFU of Salmonella Typhimurium mrmI show no significant differences in liver and spleen invasion compared to the wild-type counterpart 5 days post-infection. These results highlight the importance of mrmI and chemotaxis for invading and crossing the intestinal barrier. In support of these findings, it has been reported that motility genes such as flagellar structural components, flagellar synthesis machinery and flagellar export components, play no part in systemic infections of STM in mice macrophages (Schmitt et al., 2001) Nevertheless, colonization of organs other than liver and spleen via different administration routes requires further analysis. Therefore, we propose that mrmI participates in intestinal phase of infection, but it loses importance during the systemic cycle, where the bacteria are phagocytized and disseminated by phagocytic cells (Hurley et al., 2014).
Given that mrmI is involved in motility of STM and STY and that mrmI expression is regulated (directly or indirectly) by MarT, it was found that MarT is involved in motility, as the S. typhimurium ΔmarT mutant displayed increased motility (Supplementary Figure S5). Therefore, MarT might regulate motility at least in part by negatively regulating mrmI. Our results indicate that MarT is a negative regulator of mrmI, and for this reason, we predicted that deletion of marT would increase expression of mrmI and increase invasion, to liver and spleen, as a consequence. However, STM ΔmarT presented a less invasive phenotype, compared to STM WT in mice (Supplementary Figure S6). Therefore, regulation of genes other than mrmI, might be responsible for the final phenotypes of marT mutants. Studying the regulon of marT by using global search approaches will elucidate the contribution of other genes to final phenotypes of marT mutants.
As mentioned above, MarT belongs to the ToxR family protein (Blanc-Potard et al., 1999; Tükel et al., 2007). These proteins are bound to the inner membrane of Gram-negative bacteria, where they sense particular compounds and conditions to produce a genomic response (Auger et al., 1989; DiRita and Mekalanos, 1991). One example is CadC, which harbors 41% identity with MarT (Blanc-Potard et al., 1999). At pH 5.8 and a rich lysine environment, CadC positively regulates the operon cadBA in E. coli (Auger et al., 1989). cadBA encodes proteins that responsible for lysine catabolization and the expulsion of cadaverine resulting from lysine decarboxylation (Supplementary Figure S7) (Dell et al., 1994; Cserzö et al., 1997; Tetsch et al., 2008). In silico analyses of MarT reveal a transmembrane domain (Supplementary Figure S7) suggesting that MarT might act through mechanisms similar to those used by CadC.
5 Conclusion
According to our results, we conclude that MarT is involved in downregulating expression of mrmI in STM, while marT is pseudogenized in STY. In addition, decreased expression of mrmI when marTSTM is restored in STY further supports the evidence that MarT downregulates mrmI. Furthermore, it was found that mrmI possesses homology and high levels of similarity with methyl accepting chemotaxis proteins such as Tsr, CheD, and Aer. the mrmI gene is involved in the motility of both STM and STY and might play a crucial role in host invasion through the intestinal barrier. We speculate that STY evolved in such a way to silently cross the intestinal barrier to avoid alerting the immune system, thus favoring systemic invasion.
Data availability statement
The datasets generated for this study are available on request from the corresponding author, AH.
Ethics statement
The animal study was approved by institutional bioethics committee at Universidad Andres Bello. The study was conducted in accordance with the local legislation and institutional requirements.
Author contributions
SJ: Conceptualization, Data curation, Formal analysis, Investigation, Validation, Writing – original draft, Methodology, Software. ArM: Data curation, Formal analysis, Investigation, Methodology, Resources, Validation, Writing – review & editing. AnM: Methodology, Writing – review & editing, Formal analysis, Investigation, Resources. CS: Data curation, Methodology, Writing – review & editing, Validation, Visualization. SB: Formal analysis, Investigation, Resources, Writing – review & editing, Data curation, Methodology. GM: Resources, Supervision, Writing – review & editing, Funding acquisition, Methodology, Project administration. NV: Conceptualization, Data curation, Formal analysis, Project administration, Resources, Supervision, Writing – review & editing. AH: Conceptualization, Data curation, Formal analysis, Investigation, Validation, Writing – review & editing, Funding acquisition, Project administration, Resources, Supervision, Visualization, Writing – original draft.
Funding
The author(s) declare that financial support was received for the research, authorship, and/or publication of this article. This work was supported by the Fondo Nacional de Ciencia y Tecnología (FONDECYT/ANID, Government of Chile) Grants 11150588 (AH), 1151393 (GM), and 1210633 (CS); UNAB Regular Grants DI-15-19/RG (AH) and DI-4-17/RG (NV); and ECOS-CONICYT grant C16B04 (AH). SJ was supported by ANID predoctoral fellowship ANID21210879.
Acknowledgments
Thanks to Mr. Víctor H. Ahumada for the technical assistant. A part of the results included in this manuscript were presented during the XXXVIII and XXXIX Meetings of the Chilean Society for Microbiology.
Conflict of interest
The authors declare that the research was conducted in the absence of any commercial or financial relationships that could be construed as a potential conflict of interest.
Publisher’s note
All claims expressed in this article are solely those of the authors and do not necessarily represent those of their affiliated organizations, or those of the publisher, the editors and the reviewers. Any product that may be evaluated in this article, or claim that may be made by its manufacturer, is not guaranteed or endorsed by the publisher.
Supplementary material
The Supplementary material for this article can be found online at: https://www.frontiersin.org/articles/10.3389/fmicb.2024.1430982/full#supplementary-material
References
Alexander, R. P., and Zhulin, I. B. (2007). Evolutionary genomics reveals conserved structural determinants of signaling and adaptation in microbial chemoreceptors. PNAS 104, 2885–2890. doi: 10.1073/pnas.0609359104
Altschul, S. F., Gish, W., Miller, W., Myers, E. W., and Lipman, D. J. (1990). Basic local alignment search tool. J. Mol. Biol. 215, 403–410. doi: 10.1016/S0022-2836(05)80360-2
Auger, E. A., Redding, K. E., Plumb, T., Childs, L. C., Meng, S. Y., and Bennett, G. N. (1989). Construction of lac fusions to the inducible arginine- and lysine decarboxylase genes of Escherichia coli K12. Mol. Microbiol. 3, 609–620. doi: 10.1111/j.1365-2958.1989.tb00208.x
Blanc-Potard, A.-B., Solomon, F., Kayser, J., and Groisman, E. A. (1999). The SPI-3 Pathogenicity Island Ofsalmonella enterica. J. Bacteriol. 181, 998–1004. doi: 10.1128/JB.181.3.998-1004.1999
Blum, M., Chang, H.-Y., Chuguransky, S., Grego, T., Kandasaamy, S., Mitchell, A., et al. (2021). The InterPro protein families and domains database: 20 years on. Nucleic Acids Res. 49, D344–D354. doi: 10.1093/nar/gkaa977
Boyd, E. F., Wang, F.-S., Beltran, P., Plock, S. A., Nelson, K., and Selander, R. K. Y. (1993). Salmonella reference collection B (SARB): strains of 37 serovars of subspecies I. Microbiology 139, 1125–1132. doi: 10.1099/00221287-139-6-1125
Contreras, I., Toro, C. S., Troncoso, G., and Mora, G. C. Y. (1997). Salmonella typhi mutants defective in anaerobic respiration are impaired in their ability to replicate within epithelial cells. Microbiology 143, 2665–2672. doi: 10.1099/00221287-143-8-2665
Cserzö, M., Wallin, E., Simon, I., von Heijne, G., and Elofsson, A. (1997). Prediction of transmembrane alpha-helices in prokaryotic membrane proteins: the dense alignment surface method. Protein Eng. 10, 673–676. doi: 10.1093/protein/10.6.673
Datsenko, K. A., and Wanner, B. L. (2000). One-step inactivation of chromosomal genes in Escherichia coli K-12 using PCR products. PNAS 97, 6640–6645. doi: 10.1073/pnas.120163297
Barbosa, F. D. O., Freitas, O. C. D., Batista, D. F. A., Almeida, A. M. D., Rubio, M. D. S., Alves, L. B. R., et al. (2017). Contribution of flagella and motility to gut colonisation and pathogenicity of Salmonella Enteritidis in the chicken. Braz. J. Microbiol. 48, 754–759. doi: 10.1016/j.bjm.2017.01.012
Dell, C. L., Neely, M. N., and Olson, E. R. (1994). Altered pH lysine signalling mutants of cadC, a gene encoding a membrane-bound transcriptional activator of the Escherichia coli cadBA operon. Mol. Microbiol. 14, 7–16. doi: 10.1111/j.1365-2958.1994.tb01262.x
Derr, P., Boder, E., and Goulian, M. (2006). Changing the specificity of a bacterial chemoreceptor. J. Mol. Biol. 355, 923–932. doi: 10.1016/j.jmb.2005.11.025
Diniz, M. M. P., Goulart, C. L., Barbosa, L. C., Farache, J., Lery, L. M. S., Pacheco, A. B. F., et al. (2011). Fine-tuning control of phoBR expression in Vibrio cholerae by binding of PhoB to multiple pho boxes. J. Bacteriol. 193, 6929–6938. doi: 10.1128/JB.06015-11
DiRita, V. J., and Mekalanos, J. J. (1991). Periplasmic interaction between two membrane regulatory proteins, ToxR and ToxS, results in signal transduction and transcriptional activation. Cell 64, 29–37. doi: 10.1016/0092-8674(91)90206-E
Dorsey, C. W., Laarakker, M. C., Humphries, A. D., Weening, E. H., and Bäumler, A. J. (2005). Salmonella enterica serotype typhimurium MisL is an intestinal colonization factor that binds fibronectin. Mol. Microbiol. 57, 196–211. doi: 10.1111/j.1365-2958.2005.04666.x
Eriksson, S., Lucchini, S., Thompson, A., Rhen, M., and Hinton, J. C. D. (2003). Unravelling the biology of macrophage infection by gene expression profiling of intracellular Salmonella enterica. Mol. Microbiol. 47, 103–118. doi: 10.1046/j.1365-2958.2003.03313.x
Goss, T. J., Morgan, S. J., French, E. L., and Krukonis, E. S. (2013). ToxR recognizes a direct repeat element in the toxT, ompU, ompT, and ctxA promoters of Vibrio cholerae to regulate transcription. Infect. Immun. 81, 884–895. doi: 10.1128/IAI.00889-12
Goss, T. J., Seaborn, C. P., Gray, M. D., and Krukonis, E. S. (2010). Identification of the TcpP-binding site in the toxT promoter of vibrio cholerae and the role of ToxR in TcpP-mediated activation. Infect. Immun. 78, 4122–4133. doi: 10.1128/IAI.00566-10
Hidalgo, A. A., Trombert, A. N., Castro-Alonso, J. C., Santiviago, C. A., Tesser, B. R., Youderian, P., et al. (2004). Insertions of Mini-Tn10 transposon T-POP in Salmonella enterica sv. Typhi. Genetics 167, 1069–1077. doi: 10.1534/genetics.104.026682
Hidalgo, A. A., Villagra, N. A., Jerez, S. A., Fuentes, J. A., and Mora, G. C. (2016). A conditionally lethal mutant of Salmonella Typhimurium induces a protective response in mice. Biochem. Biophys. Res. Commun. 470, 313–318. doi: 10.1016/j.bbrc.2016.01.058
Hurley, D., McCusker, M. P., Fanning, S., and Martins, M. (2014). Salmonella-host interactions - modulation of the host innate immune system. Front. Immunol. 5:481. doi: 10.3389/fimmu.2014.00481
Ikeda, M., Arai, M., Okuno, T., and Shimizu, T. (2003). TMPDB: a database of experimentally-characterized transmembrane topologies. Nucleic Acids Res. 31, 406–409. doi: 10.1093/nar/gkg020
Kröger, C., Colgan, A., Srikumar, S., Händler, K., Sivasankaran, S. K., Hammarlöf, D. L., et al. (2013). An Infection-Relevant Transcriptomic Compendium for Salmonella enterica Serovar Typhimurium. Cell Host Microbe 14, 683–695. doi: 10.1016/j.chom.2013.11.010
Lux, R., Jahreis, K., Bettenbrock, K., Parkinson, J. S., and Lengeler, J. W. (1995). Coupling the phosphotransferase system and the methyl-accepting chemotaxis protein-dependent chemotaxis signaling pathways of Escherichia coli. PNAS 92, 11583–11587. doi: 10.1073/pnas.92.25.11583
McClelland, M., Sanderson, K. E., Spieth, J., Clifton, S. W., Latreille, P., Courtney, L., et al. (2001). Complete genome sequence of Salmonella enterica serovar Typhimurium LT2. Nature 413, 852–856. doi: 10.1038/35101614
Millanao, A. R., Mora, A. Y., Saavedra, C. P., Villagra, N. A., Mora, G. C., and Hidalgo, A. A. (2020). Inactivation of glutamine Synthetase-coding gene glnA increases susceptibility to quinolones through increasing outer membrane protein F in Salmonella enterica Serovar Typhi. Front. Microbiol. 11:428. doi: 10.3389/fmicb.2020.00428
Miller, V. L., Taylor, R. K., and Mekalanos, J. J. (1987). Cholera toxin transcriptional activator ToxR is a transmembrane DNA binding protein. Cell 48, 271–279. doi: 10.1016/0092-8674(87)90430-2
Morgan, E., Campbell, J. D., Rowe, S. C., Bispham, J., Stevens, M. P., Bowen, A. J., et al. (2004). Identification of host-specific colonization factors of Salmonella enterica serovar typhimurium. Mol. Microbiol. 54, 994–1010. doi: 10.1111/j.1365-2958.2004.04323.x
Olsen, J. E., Hoegh-Andersen, K. H., Casadesús, J., Rosenkrantz, J. T., Chadfield, M. S., and Thomsen, L. E. (2013). The role of flagella and chemotaxis genes in host pathogen interaction of the host adapted Salmonella enterica serovar Dublin compared to the broad host range serovar S.Typhimurium. BMC Microbiol. 13:67. doi: 10.1186/1471-2180-13-67
Ortega, A. P., Villagra, N. A., Urrutia, I. M., Valenzuela, L. M., Talamilla-Espinoza, A., Hidalgo, A. A., et al. (2016). Lose to win: marT pseudogenization in Salmonella enterica serovar Typhi contributed to the surV-dependent survival to H2O2, and inside human macrophage-like cells. Infect. Genet. Evol. 45, 111–121. doi: 10.1016/j.meegid.2016.08.029
Ottemann, K. M., and Mekalanos, J. J. (1996). The ToxR protein of Vibrio cholerae forms homodimers and heterodimers. J. Bacteriol. 178, 156–162. doi: 10.1128/jb.178.1.156-162.1996
Parkhill, J., Dougan, G., James, K. D., Thomson, N. R., Pickard, D., Wain, J., et al. (2001). Complete genome sequence of a multiple drug resistant Salmonella enterica serovar Typhi CT18. Nature 413, 848–852. doi: 10.1038/35101607
Parkinson, J. S. (1976). cheA, cheB, and cheC genes of Escherichia coli and their role in chemotaxis. J. Bacteriol. 126, 758–770. doi: 10.1128/jb.126.2.758-770.1976
Pfaffl, M. W. (2001). A new mathematical model for relative quantification in real-time RT–PCR. Nucleic Acids Res. 29:e45, 45e–445e. doi: 10.1093/nar/29.9.e45
Retamal, P., Castillo-Ruiz, M., Villagra, N. A., Morgado, J., and Mora, G. C. (2010). Modified intracellular-associated phenotypes in a recombinant Salmonella Typhi expressing S. typhimurium SPI-3 sequences. PLoS One 5:e9394. doi: 10.1371/journal.pone.0009394
Rivera-Chávez, F., Winter, S. E., Lopez, C. A., Xavier, M. N., Winter, M. G., Nuccio, S.-P., et al. (2013). Salmonella uses energy taxis to benefit from intestinal inflammation. PLoS Pathog. 9:e1003267. doi: 10.1371/journal.ppat.1003267
Ryan, D., Ojha, U. K., Jaiswal, S., Padhi, C., and Suar, M. (2016). The small RNA DsrA influences the acid tolerance response and virulence of Salmonella enterica Serovar typhimurium. Front. Microbiol. 7:599. doi: 10.3389/fmicb.2016.00599
Santiviago, C. A., Toro, C. S., Bucarey, S. A., and Mora, G. C. (2001). A chromosomal region surrounding the ompD porin gene marks a genetic difference between Salmonella typhi and the majority of Salmonella serovars. Microbiology 147, 1897–1907. doi: 10.1099/00221287-147-7-1897
Schmitt, C. K., Ikeda, J. S., Darnell, S. C., Watson, P. R., Bispham, J., Wallis, T. S., et al. (2001). Absence of all components of the flagellar export and synthesis machinery differentially alters virulence of Salmonella enterica Serovar typhimurium in models of typhoid fever, survival in macrophages, tissue culture invasiveness, and calf enterocolitis. Infect. Immun. 69, 5619–5625. doi: 10.1128/iai.69.9.5619-5625.2001
Snavely, M. D., Gravina, S. A., Cheung, T. T., Miller, C. G., and Maguire, M. E. (1991). Magnesium transport in Salmonella typhimurium. Regulation of mgtA and mgtB expression. J. Biol. Chem. 266, 824–829. doi: 10.1016/S0021-9258(17)35247-X
Sonnhammer, E. L., von Heijne, G., and Krogh, A. (1998). A hidden Markov model for predicting transmembrane helices in protein sequences. Proc. Int. Conf. Intell. Syst. Mol. Biol. 6, 175–182
Tetsch, L., Koller, C., Haneburger, I., and Jung, K. (2008). The membrane-integrated transcriptional activator CadC of Escherichia coli senses lysine indirectly via the interaction with the lysine permease LysP. Mol. Microbiol. 67, 570–583. doi: 10.1111/j.1365-2958.2007.06070.x
Tükel, Ç., Akçelik, M., de Jong, M. F., Şimşek, Ö., Tsolis, R. M., and Bäumler, A. J. (2007). MarT activates expression of the MisL autotransporter protein of Salmonella enterica serotype typhimurium. J. Bacteriol. 189, 3922–3926. doi: 10.1128/JB.01746-06
Valenzuela, C., Ugalde, J. A., Mora, G. C., Álvarez, S., Contreras, I., and Santiviago, C. A. (2014). Draft genome sequence of Salmonella enterica Serovar Typhi strain STH2370. Genome Announc. 2, e00104–e00114. doi: 10.1128/genomeA.00104-14
Villagra, N. A., Hidalgo, A. A., Santiviago, C. A., Saavedra, C. P., and Mora, G. C. (2008). SmvA, and not AcrB, is the major efflux pump for acriflavine and related compounds in Salmonella enterica serovar typhimurium. J. Antimicrob. Chemother. 62, 1273–1276. doi: 10.1093/jac/dkn407
Keywords: Salmonella typhi , Salmonella typhimurium , marT , pseudogene, MCP-family chemosensor
Citation: Jerez SA, Mora AY, Millanao AR, Saavedra CP, Bucarey SA, Mora GC, Villagra NA and Hidalgo AA (2024) Transcriptional regulator MarT negatively regulates MarT-regulated motility gene I, a new gene involved in invasion and virulence of Salmonella enterica. Front. Microbiol. 15:1430982. doi: 10.3389/fmicb.2024.1430982
Edited by:
Laurent Aussel, Aix-Marseille Université, FranceReviewed by:
James M. Slauch, University of Illinois at Urbana-Champaign, United StatesJohid Malik, University of Nebraska Medical Center, United States
Copyright © 2024 Jerez, Mora, Millanao, Saavedra, Bucarey, Mora, Villagra and Hidalgo. This is an open-access article distributed under the terms of the Creative Commons Attribution License (CC BY). The use, distribution or reproduction in other forums is permitted, provided the original author(s) and the copyright owner(s) are credited and that the original publication in this journal is cited, in accordance with accepted academic practice. No use, distribution or reproduction is permitted which does not comply with these terms.
*Correspondence: Alejandro A. Hidalgo, alejandro.hidalgo@unab.cl
†These authors have contributed equally to this work and share first authorship