- Chongqing Key Laboratory of Plant Environmental Adaptations, College of Life Science, Chongqing Normal University, Chongqing, China
Sclerotinia sclerotiorum is a typical necrotrophic plant pathogenic fungus, which has a wide host range and can cause a variety of diseases, leading to serious loss of agricultural production around the world. It is difficult to control and completely eliminate the characteristics, chemical control methods is not ideal. Therefore, it is very important to know the pathogenic mechanism of S. sclerotiorum for improving host living environment, relieving agricultural pressure and promoting economic development. In this paper, the life cycle of S. sclerotiorum is introduced to understand the whole process of S. sclerotiorum infection. Through the analysis of the pathogenic mechanism, this paper summarized the reported content, mainly focused on the oxalic acid, cell wall degrading enzyme and effector protein in the process of infection and its mechanism. Besides, recent studies reported virulence-related genes in S. sclerotiorum have been summarized in the paper. According to analysis, those genes were related to the growth and development of the hypha and appressorium, the signaling and regulatory factors of S. sclerotiorum and so on, to further influence the ability to infect the host critically. The application of host-induced gene silencing (HIGS)is considered as a potential effective tool to control various fungi in crops, which provides an important reference for the study of pathogenesis and green control of S. sclerotiorum.
1 Introduction
Sclerotinia sclerotiorum is a typical necrotrophic nutrient fungus that belongs in taxonomic status to the Fungi, Ascomycota, Discomycetes, Helotiales, Sclerotiniaceae, Sclerotinia. An early study determined that Dictyostelium can infect more than 450 plant species in 75 families, posing a threat primarily to dicotyledonous crops such as sunflowers, soybeans, canola, edible dry beans, chickpeas, peanuts, dry beans, lentils, and a variety of vegetables, but also to monocotyledonous plants such as onions and tulips (Boland and Hall, 1994). Recent research has found that it can grow in rice, wheat, barley, oat, and corn (Tian et al., 2020).
The disease caused by S. sclerotiorum is called Sclerotinia. More than 60 names have been used to refer to diseases caused by this fungal pathogen (Purdy, 1979), including cotton rot, water soft rot, stem rot, abscission, crown rot, flower wilt, and the most common white mold. S. sclerotiorum is a representative vegetative plant pathogenic fungus with complex pathogenic mechanism. The role and mechanism of oxalic acid, cell wall degrading enzymes, and secretory proteins secreted by S. sclerotiorum in the process of infection have been the focus of reports previously. However, the molecular basis of the pathogenesis of S. sclerotiorum is imprecise and remains a subject of ongoing research. In this paper, the recent advancements in the virulence of S. sclerotiorum have been reviewed, which can serve as an important source of information for molecular research of this organism.
2 The infection process of Sclerotinia Sclerotiorum
Sclerotinia sclerotiorum (Lib.) de Bary is one of the most dangerous and common plant-killing organisms in the world. This fungus may be found all across temperate, tropical, and dry environment (Lehner et al., 2017). Sclerotia consists of three distinct layers, the thick-walled pigmented cortex, the thin-walled cortex and the white medulla (Clarkson et al., 2004). As its name indicates, the fungus produces sclerotia, which are long-lived melanized resting structures. Sclerotia can germinate in two ways, carpogenically to form apothecia from which ascospores are liberated or myceliogenically to produce hyphae. The fungus is homothallic and no asexual spores are produced (Hegedus and Rimmer, 2005). S. sclerotiorum generally exists in the form of sclerotia, and the existence of ascospores and mycelium form maintains a shorter time (Bolton et al., 2006). When environmental conditions are fit for germination, sclerotia may germinate myceliogenically to produce hyphae that infect the lower parts of plants, or germinate carpogenically to produce apothecia and release ascospores into the air (Behnam et al., 2007; Fernando et al., 2007; Zhang M. et al., 2021). When infected in the form of ascospores, only combined with multiple infection sites, can the successful infection feature be formed. For example, flowers or flower parts provide an appropriate nutritional basis for the initial colonization of ascospore inoculants. The undamaged host surface is infected by hyphae extending from the matrix (Sutton and Deverall, 1983). Once infection has been established, watery lesions with distinct edges may first appear on stems, leaves, petioles, and reproductive organs. These lesions are then followed by wilting, bleaching, and shredding. Typically on the surface of the infected tissue as well as in soft host tissue or lumen, cotton hyphae build up into pea-sized aggregates that eventually develop into hard, black sclerotia (Heffer and Johnson, 2007). When the stem is completely girdled and covered by the whitish mycelial growth all over, the plant wilts and dries. The fungus produces symptoms on all the aerial plant parts but the most destructive one is produced on the stem. Usually, the plants are attacked at flowering stage and once the pathogen is established in the field, it is very difficult to eliminate it due to the stubborn soil-borne sclerotia and wide host range (Bolton et al., 2006).
Strong pathogenicity enable S. sclerotiorum to virtually completely invade all plant tissues with its mycelium. Importantly, the fungus affects hundreds of monocotyledons and dicotyledones and is not restricted to any one host (Jahan et al., 2022). Infection can potentially begin with mycelial development from sclerotia on the soil’s surface. After colonizing dead organic debris, the germinated hyphae infect nearby living plants (Hegedus and Rimmer, 2005). Plant pathogen S. sclerotiorum is extremely destructive, and its infection can result in substantial yield loss, the drop in the quantity and quality of affected crops, and other negative economic effects (Fernando et al., 2007).
3 Pathogenicity factors
Sclerotinia sclerotiorum is a typical necrotrophic plant pathogenic fungus with complex pathogenic mechanism. At present, the main pathogenic factors are classified as plant cell-wall-degrading enzymes(CWDEs), oxalic acid and effector protein secreted by S. sclerotiorum. Firstly, S.sclerotiorum increases its colonization by secreting CWDEs, which plays an important role in the early stage of infection. Oxalic acid, a key pathogenic factor, is secreted in large quantities in the early stage and plays an important role in pathogenicity. In addition, S. Sclerotiorum also secretes some effector proteins to promote infection in its fight against plants.
3.1 Oxalic acid, a key factor in the early pathogenesis of Sclerotinia Sclerotiorum
Oxalic acid is considered to be a key factor in the early pathogenesis of many necrotrophic fungi. S. sclerotiorum involves the production and accumulation of oxalic acid at the early stage of plant susceptibility (Figure 1). Godoy et al. (1990) studied the role of oxalic acid in the pathogenicity of S. sclerotiorum in 1990, the oxalic acid-deficient mutants were obtained by UV mutagenesis and lost their pathogenicity. Moreover, the pathogenicity of the mutants was restored after the addition of sodium succinate.
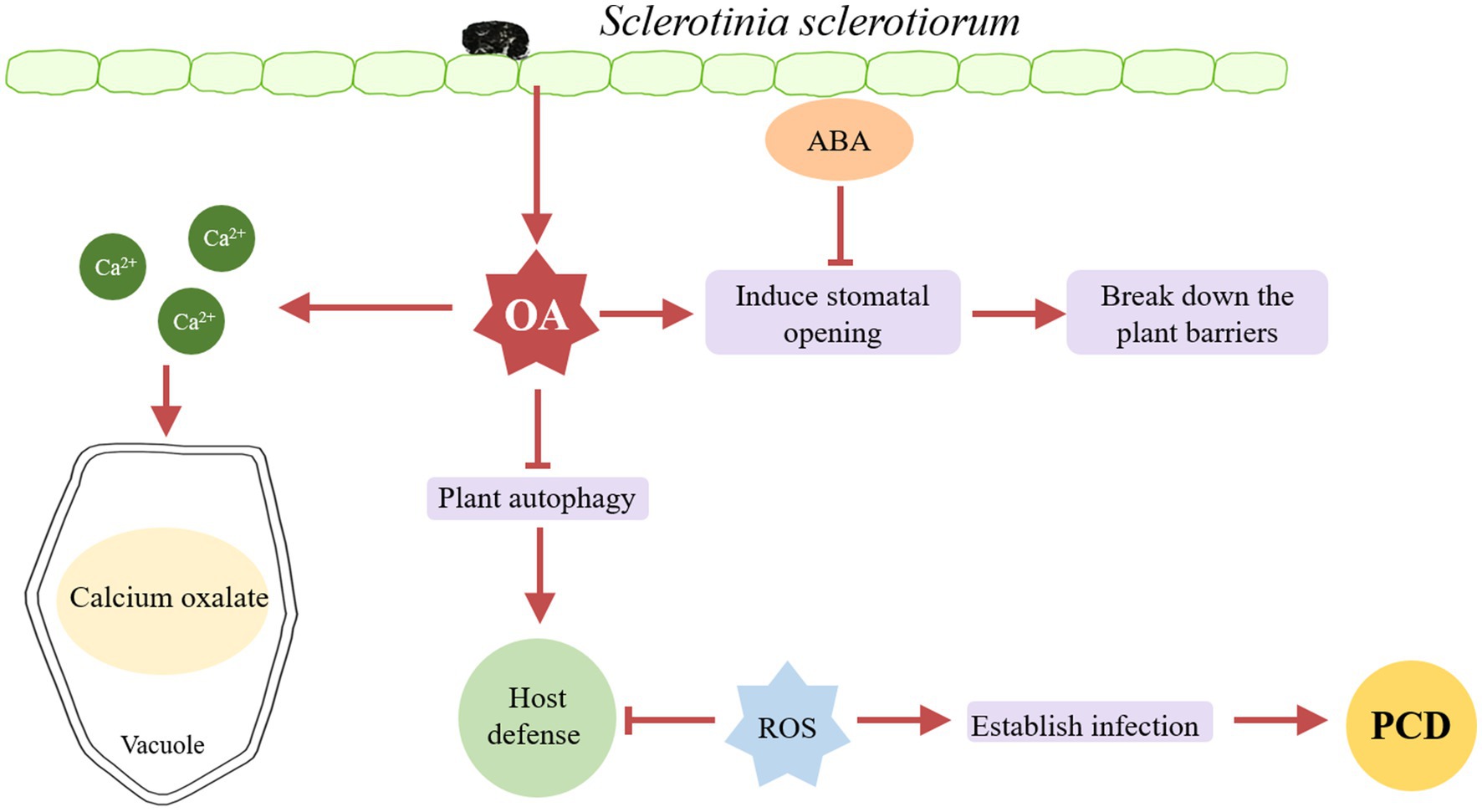
Figure 1. A model of oxalic acid (OA)-induced infection. S. sclerotiorum secretes large amounts of oxalic acid during infection. In the early stage of infection, oxalic acid blocks the closure of the plant stomata to promote infection, and then breaks down the plant barrier, at which point the host secretes ABA to shut down the plant stomata to resist infection. Oxalic acid can inhibit plant autophagy, through the outbreak of producing reactive oxygen species (ROS) in the later stage to inhibit the host defense response, and finally cause the programmed cell death (PCD) of plants. In addition, oxalic acid can preserve ca2+ generated by cell wall collapsing in the form of calcium oxalate in the vacuole, playing a detoxification role.
More studies revealed the role of oxalic acid in the pathogenesis of S. sclerotiorum. Oxalic acid can produce reactive oxygen species (ROS) and induce programmed cell death (PCD). In contrast, during the initial stage of S. sclerotiorum infection of host plants, S. sclerotiorum produces a reducing environment in host cells through oxalic acid inhibiting host defense responses, including suppression of ROS bursts and callose deposition, similar to compatible bionutrient pathogens. However, once an infection is established, oxalic acid induces ROS production in the plants, resulting PCD in the host tissue (Williams and Stelfox, 1980). Oxalic acid can regulate the PH of the environment to facilitate the infection of S. sclerotiorum. Sclerotinia sclerotiorum can cause infection and disease at least in some plants as long as the environmental pH is low enough (Bateman and Beer, 1965). Oxalic acid prevents stomatal closure in the host plant. The results showed that when the leaves of Vicia faba were infected with S. sclerotiorum mutants lacking oxalic acid, the stomata were partially closed (Guimarães and Stotz, 2004). Besides, Oxalate prevents stomatal closure caused by abscisic acid (ABA). In Arabidopsis, compared to wild type plants, mutants abi1, abi3, abi4, and aba2 are more vulnerable to oxalate-deficient S. sclerotiorum, indicating that ABA is necessary for Sclerotinia resistance (Guimarães and Stotz, 2004). Oxalic acid may be able to quench calcium ions generated during cell wall collapse to shield developing hyphae from the harmful calcium concentrations in the infection region. Heller and Witt-Geiges (2013) suggest that calcium was transferred to the older parts of the mycelium and detoxified by forming nontoxic, stable oxalate crystals and they proposed an infection model in which oxalic acid plays a detoxifying role in the late stage of infection.
3.2 Cell wall degrading enzymes play a vital role in pathogenic fungi invading host
Plant cell wall is an important place for interaction between host and pathogenic fungi, and plays a vital role in the process of plant pathogenic fungi invading host. The pathogenic fungi secrete a series of cell wall degrading enzymes during the process of infecting host plants. They can not only take up nutrition, but also degrade host tissue during the pathogenic process of pathogenic fungi. Sclerotinia sclerotiorum releases hydrolases, which degrade the cuticle, the middle layer, and the primary and secondary cell walls in turn. It was found that the cuticle-stripped leaves infected more quickly, so the cuticle could act as a barrier against Sclerotinia infection.
Sclerotinia sclerotiorum can secrete different cell wall degrading enzymes, especially Polygalacturonases (PGs), when it infects host plants. According to reports, four endo-polygalacturonase (PG) genes (SsPG1, SsPG3, SsPG5 and SsPG6) and two exo-PGs genes (SsXPG1 and SsXPG2) were identified in B. napus during S. Sclerotiorum infection (Li et al., 2004). The factors affecting the regulation of genes encoding polygalacturonase 1(SsPG1) and a newly identified keratinase (SsCUTA) were studied. In vitro, SsCUTA transcripts were detected within 1 h after leaf inoculation, and their expression was mainly controlled by the contact of mycelium with solid surface. The expression level of the keratinase-encoding gene SsCUTA of S. sclerotiorum was significantly up-regulated at 1 h after infection. Expression of SsPG1 was moderately induced by contact with solid surfaces, including leaves, and as infection progresses, expression of SsPG1 was limited to the extended margins of the lesion (Dallal Bashi et al., 2012). Both SsPG3 and SsPG6 induced light-dependent necrosis in Arabidopsis thaliana leaves (Dallal Bashi et al., 2012).
3.3 Effector proteins, critical components in the effective pathogenesis of Sclerotinia Sclerotiorum
In the process of antagonism between plant and pathogen, the pathogen will secrete some proteins into plant cells. These proteins are called “Effector proteins,” which can make the pathogen successfully infect the plant. These “Effector proteins,” have been found in a variety of plant pathogenic fungi and exhibit many different functions according to the life style of the fungi.
SsITL encodes a protein containing 302 amino acid residues, which belongs to the integrin-terminal domain superfamily. The expression of SsITL gene increased sharply during the early stage of infection and after the gene silencing, the pathogenicity of S. sclerotiorum decreased. The host plants overexpressing SsITL gene were more susceptible. Further studies showed that SsITL protein was involved in the inhibition of JA/ET signaling pathway-mediated local and systemic disease resistance in S. sclerotiorum. Therefore, SsITL played a similar role as an effector in S. Sclerotiorum pathogenesis (Wang et al., 2009). Two genes, SsNEP1 and SsNEP2, encoding necrotic and ethylene-induced polypeptides (NEPs), were identified in S. sclerotiorum. During infection, the expression of SsNEP1 was increased. The expression of SsNEP2 was induced by contact with solid surfaces and occurs in necrotic areas and the leading edge of infection. Expression of SsNEP2 was dependent on calcium and cyclic AMP signaling, instead, compounds that affected these pathways reduced or eliminated SsNEP1 expression with partial or complete loss of virulence (Dallal Bashi et al., 2010). A small cysteine-rich protein, SsCVNH, has been shown to be crucial for virulence and sclerotium development (Lyu et al., 2015a). Another small cysteine-rich protein SsSSVP1 in S. sclerotiorum has been identified as a secreted protein and its targeted silencing resulted in reduced virulence (Lyu et al., 2016).
4 Reported genes regulating virulence in Sclerotinia Sclerotiorum
The virulence of S. Sclerotiorum is related to the growth and development of its appressorium and its ability to infect plants. Studies on the virulence of S. sclerotiorum to date have been shown as follows (Table 1). In the regulation of pathogenicity in S. sclerotiorum, several genes play pivotal roles. SsTrx1 contributes to enhancing the pathogenicity of the fungus and its tolerance to oxidative stress (Rana et al., 2021), while overexpression of SsYCP1 promotes S. sclerotiorum infection and enhances its pathogenicity (Fan et al., 2021a). SsNACα regulates the expression of polygalacturonase, thus impacting the pathogenicity of S. sclerotiorum (Li et al., 2015). Additionally, SsERP1 modulates the virulence of S. sclerotiorum by regulating the ethylene pathway (Fan et al., 2021b). Moreover, SsSte12 influences fungal hyphal growth, adhesion structure formation, and virulence regulation (Xu et al., 2018). SsPDE2 plays a regulatory role in oxalate accumulation, adhesion structure formation, and virulence (Xu et al., 2023). Furthermore, SsXyl1, encoding endo-β-1,4-xylanase, plays a crucial role in sclerotium formation and infection processes (Yu et al., 2016). SsNsd1 regulates the morphology of S. sclerotiorum hyphae, appressorium structures, sclerotium, and pathogenicity (Li et al., 2018).
The signaling and regulatory factors of S. sclerotiorum involve the regulation of multiple genes. SsTOR is responsible for controlling cell wall integrity and virulence, and it participates in the rapamycin target signaling pathway (Jiao et al., 2023). SsCat2 regulates catalase activity, affecting aspects such as cell membrane dryness and pathogenicity (Huang et al., 2021). SsPKA2 and SsPKAR are involved in cAMP signaling, playing roles in growth and virulence (Yu and Rollins, 2022). Moreover, SsAMS2 contains a GATA-box domain, regulating hyphal growth, adhesion structure formation, and virulence (Liu et al., 2018). Lastly, SsSm1 participates in the MAPK signaling pathway, influencing aspects such as hyphal growth and pathogenicity (Pan et al., 2018).
These genes play critical roles in the metabolic regulation of S. sclerotiorum. Ssoah1 regulates oxalic acid content, affecting the toxicity of S. sclerotiorum (Liang et al., 2015a; Rana et al., 2022). Next, SsSOD1 is responsible for regulating the clearance capacity of ROS, directly impacting the pathogenicity of S. sclerotiorum. Additionally, SsODC1 and SsODC2 are involved in oxalic acid metabolism regulation, playing crucial regulatory roles in forming infection cushions and virulence during the infection process (Xu and Chen, 2013).
In the regulation of cellular structure and function in S. sclerotiorum, SsAGM1 participates in chitin synthesis, influencing cell wall structure and sclerotia formation (Zhang et al., 2022). Subsequently, SsGSR1 encodes glutathione sulfurtransferase, regulating cell wall integrity and the pathogenicity of S. sclerotiorum (Hu et al., 2023). SsCP1 encodes the Cerato-platanin protein, affecting cell death and invasion ability during infection (Yang et al., 2018). Furthermore, as a small secreted protein, SsSSVP1 influences plant cell death and the infection of S. sclerotiorum (Lyu et al., 2016).
In the adaptation to environmental changes and stress responses in S. sclerotiorum, SsNE2, as a novel necrosis-inducing protein, influences the fungal growth, pathogenicity, and ability to respond to environmental stress (Seifbarghi et al., 2020). As we know, thioredoxin reductases play crucial roles in maintaining cellular redox homeostasis. SsTrr1, encoding thioredoxin reductase, participates in oxidative stress responses, thereby affecting the pathogenicity of S. sclerotiorum (Zhang et al., 2019). Additionally, SsEmp24 and SsErv25, as early secretory pathway-related proteins, regulate the protein secretion process, playing important roles in the pathogenicity of S. sclerotiorum. Their absence leads to abnormal fungal growth, sclerotium formation, formation of appressorium, and lower virulence in host plants (Xie et al., 2021).
5 Host-induced gene silencing of virulence-related genes of Sclerotinia Sclerotiorum
Most eukaryotic organisms possess the RNA interference (RNAi) pathway. In this process, double-stranded RNA (dsRNA) can be processed into small interfering RNA (siRNA), which can induce gene silencing. As RNA can be transferred from plant hosts to related eukaryotic pathogens, the RNAi pathway has been utilized for HIGS to control pathogens. In this scenario, hosts are engineered to express dsRNAs targeting crucial pathogen genes, thus disrupting their successful lifecycle. It is considered to be a potentially effective tool for controlling various fungi in crops (Chen et al., 2016; Zhu et al., 2017; Qi et al., 2018).
With the discovery of more and more virulence-related genes of S. sclerotiorum, many genes can be used to control S. sclerotiorum through HIGS. In tobacco, the authors tested whether the MAPK cascade consisting of SsSte50-SsSte11-SsSte7-Smk1 could serve as a target for disease control in HIGS. SsSte50 is used to make hairpin RNAi constructs. Compared with no-load, the lesion area was significantly reduced. The data showed that the HIGS of SsSte50 was heritable and could be used as a good target for controlling S. sclerotiorum (Tian et al., 2024). Besides, a gene was found to encode a cAMP phosphodiesterase (SsPDE2). The author introduced the construction of HIGS targeting SsPDE2 into N.benthamiana, observing a significant reduction in virulence against S. sclerotiorum. In summary, SsPDE2 may serve as a HIGS target to control stem rot in the field (Xu et al., 2023). Transient silencing of Sscnd1 gene by HIGS mediated by tobacco rattle virus (TRV) can significantly reduce the occurrence of tobacco diseases. Three transgenic Arabidopsis lines with HIGS gene showed a high level of resistance to S. sclerotiorum and reduced the expression of Sscnd1 (Ding et al., 2021). Similarly, a putative protein kinase SsCak1, silencing by TRV-HIGS can reduce virulence and enhance host resistance to S. sclerotiorum (Qin et al., 2023). In rapeseed, three disease-causing genes, the endo-polygalacturonase gene (SsPG1), cellobiohydrolase gene (SsCBH), and oxaloacetate acetylhydrolase gene (SsOAH1), were selected as the targets of HIGS, which significantly reduced the transcript levels of the target genes (Wu et al., 2022). A RAS-GTPase activating protein SsGAP1, which plays an important role in sclerotia formation, showed complex appressorium production and virulence. The author observed reduced virulence when they introduced HIGS constructs targeting SsGAP1, SsRAS1, and SsRAS2 in Arabidopsis, as well as in tobacco (Xu et al., 2024). Likewise, S. sclerotiorum thioredoxin 1 gene (SsTrx1) has also been identified as a potential HIGS target through the disease resistance analysis in above two plants (Rana et al., 2021).
6 Conclusion
The extremely dangerous soil-borne pathogen S. sclerotiorum poses a serious risk to crops used in agricultural production. S. sclerotiorum can not be eradicated in the field due to the lack of resistant breeding and the resistance of S. sclerotiorum strains to fungicides. On the other hand, the negative effects of pesticide residues and environmental pollution are also readily apparent, as they can result in the emergence of drug-resistant strains in the field and the death of beneficial organisms. Thus, it will be helpful to regulate S. sclerotiorum to comprehend the pathophysiology of the organism as well as to create and employ disease-resistant genes in plants. For instance, oxalic acid is a pathogenic component of S. sclerotiorum. Hence, lowering the pathogenicity of S. sclerotiorum can be achieved by reducing oxalic acid production. Moreover, targeting silencing of some proteins, such as Ss-Rhs1, can lead to aberrant colony formation and decreased pathogenicity to the host plant, hence improving host plant resistance to S. sclerotiorum. However, for all control methods, a successful strategy should be based on an in-depth understanding of the pathogenesis of S.sclerotiorum. The application of HIGS targets of known virulence-related genes can effectively provide host resistance to S. sclerotiorum. This may be an effective way to control sclerotinia in the future, providing a new idea for the green control of this broad host range plant pathogen.
Author contributions
YZ: Writing – original draft. CW: Writing – original draft. YD: Writing – original draft. WY: Writing – original draft. TZ: Writing – original draft. JL: Writing – review & editing, Writing – original draft.
Funding
The author(s) declare that no financial support was received for the research, authorship, and/or publication of this article.
Conflict of interest
The authors declare that the research was conducted in the absence of any commercial or financial relationships that could be construed as a potential conflict of interest.
Publisher’s note
All claims expressed in this article are solely those of the authors and do not necessarily represent those of their affiliated organizations, or those of the publisher, the editors and the reviewers. Any product that may be evaluated in this article, or claim that may be made by its manufacturer, is not guaranteed or endorsed by the publisher.
References
Bateman, D. F., and Beer, S. V. (1965). Simultaneous production and synergistic action of oxalic acid and polygalacturonase during pathogenesis by Sclerotium rolfsii. Phytopathology 55, 204–211
Behnam, S., Ahmadzadeh, M., Sharifi Tehrani, A., Hedjaroude, G. A., and Farzaneh, M. (2007). Biological control of Sclerotinia sclerotiorum (lib.) de Bary, the causal agent of white mold, by pseudomonas species on canola petals. Commun. Agric. Appl. Biol. Sci. 72, 993–996
Boland, G. J., and Hall, R. (1994). Index of plant hosts of Sclerotinia sclerotiorum. Can. J. Plant Pathol. 16, 93–108. doi: 10.1080/07060669409500766
Bolton, M. D., Thomma, B. P. H. J., and Nelson, B. D. (2006). Sclerotinia sclerotiorum (lib.) de Bary: biology and molecular traits of a cosmopolitan pathogen. Mol. Plant Pathol. 7, 1–16. doi: 10.1111/j.1364-3703.2005.00316.x
Chen, W., Kastner, C., Nowara, D., Oliveira-Garcia, E., Rutten, T., Zhao, Y., et al. (2016). Host-induced silencing of fusarium culmorum genes protects wheat from infection. J. Exp. Bot. 67, 4979–4991. doi: 10.1093/jxb/erw263
Chen, J., Ullah, C., Reichelt, M., Beran, F., Yang, Z.-L., Gershenzon, J., et al. (2020). The phytopathogenic fungus Sclerotinia sclerotiorum detoxifies plant glucosinolate hydrolysis products via an isothiocyanate hydrolase. Nat. Commun. 11:3090. doi: 10.1038/s41467-020-16921-2
Clarkson, J. P., Phelps, K., Whipps, J. M., Young, C. S., Smith, J. A., and Watling, M. (2004). Forecasting sclerotinia disease on lettuce: toward developing a prediction model for carpogenic germination of sclerotia. Phytopathology 94, 268–279. doi: 10.1094/PHYTO.2004.94.3.268
Cong, J., Xiao, K., Jiao, W., Zhang, C., Zhang, X., Liu, J., et al. (2022). The coupling between Cell Wall integrity mediated by MAPK kinases and SsFkh1 is involved in Sclerotia formation and pathogenicity of Sclerotinia sclerotiorum. Front. Microbiol. 13:816091. doi: 10.3389/fmicb.2022.816091
Dallal Bashi, Z., Hegedus, D. D., Buchwaldt, L., Rimmer, S. R., and Borhan, M. H. (2010). Expression and regulation of Sclerotinia sclerotiorum necrosis and ethylene-inducing peptides (NEPs). Mol. Plant Pathol. 11, 43–53. doi: 10.1111/j.1364-3703.2009.00571.x
Dallal Bashi, Z., Rimmer, S. R., Khachatourians, G. G., and Hegedus, D. D. (2012). Factors governing the regulation of Sclerotinia sclerotiorum cutinase a and polygalacturonase 1 during different stages of infection. Can. J. Microbiol. 58, 605–616. doi: 10.1139/w2012-031
Ding, Y., Chen, Y., Wu, Z., Yang, N., Rana, K., Meng, X., et al. (2022). SsCox17, a copper chaperone, is required for pathogenic process and oxidative stress tolerance of Sclerotinia sclerotiorum. Plant Sci. 322:111345. doi: 10.1016/j.plantsci.2022.111345
Ding, Y., Chen, Y., Yan, B., Liao, H., Dong, M., Meng, X., et al. (2021). Host-induced gene silencing of a multifunction gene Sscnd1 enhances plant resistance against Sclerotinia sclerotiorum. Front. Microbiol. 12:693334. doi: 10.3389/fmicb.2021.693334
Erental, A., Harel, A., and Yarden, O. (2007). Type 2A phosphoprotein phosphatase is required for asexual development and pathogenesis of Sclerotinia sclerotiorum. Mol. Plant-Microbe Interact. 20, 944–954. doi: 10.1094/MPMI-20-8-0944
Fan, H., Yang, W., Nie, J., Lin, C., Wu, J., Wu, D., et al. (2021a). Characterization of a secretory YML079-like Cupin protein that contributes to Sclerotinia sclerotiorum pathogenicity. Microorganisms 9:2519. doi: 10.3390/microorganisms9122519
Fan, H., Yang, W., Nie, J., Zhang, W., Wu, J., Wu, D., et al. (2021b). A novel effector protein SsERP1 inhibits plant ethylene Signaling to promote Sclerotinia sclerotiorum infection. J. Fungi 7:825. doi: 10.3390/jof7100825
Fan, H., Yu, G., Liu, Y., Zhang, X., Liu, J., Zhang, Y., et al. (2017). An atypical forkhead-containing transcription factor SsFKH1 is involved in sclerotial formation and is essential for pathogenicity in Sclerotinia sclerotiorum. Mol. Plant Pathol. 18, 963–975. doi: 10.1111/mpp.12453
Fernando, W. G. D., Nakkeeran, S., Zhang, Y., and Savchuk, S. (2007). Biological control of Sclerotinia sclerotiorum (lib.) de Bary by pseudomonas and bacillus species on canola petals. Crop Prot. 26, 100–107. doi: 10.1016/j.cropro.2006.04.007
Godoy, G., Steadman, J. R., Dickman, M. B., and Dam, R. (1990). Use of mutants to demonstrate the role of oxalic acid in pathogenicity of Sclerotinia sclerotiorum on Phaseolus vulgaris. Physiol. Mol. Plant Pathol. 37, 179–191. doi: 10.1016/0885-5765(90)90010-U
Gong, Y., Fu, Y., Xie, J., Li, B., Chen, T., Lin, Y., et al. (2022). Sclerotinia sclerotiorum SsCut1 modulates virulence and Cutinase activity. J. Fungi 8:526. doi: 10.3390/jof8050526
Guimarães, R. L., and Stotz, H. U. (2004). Oxalate production by Sclerotinia sclerotiorum deregulates guard cells during infection. Plant Physiol. 136, 3703–3711. doi: 10.1104/pp.104.049650
Heffer, L. V., and Johnson, K. B. (2007). White mold (Sclerotinia). The Plant Health Instructor. doi: 10.1094/PHI-I-2007-0809-01
Hegedus, D. D., and Rimmer, S. R. (2005). Sclerotinia sclerotiorum: when “to be or not to be” a pathogen? FEMS Microbiol. Lett. 251, 177–184. doi: 10.1016/j.femsle.2005.07.040
Heller, A., and Witt-Geiges, T. (2013). Oxalic acid has an additional, detoxifying function in Sclerotinia sclerotiorum pathogenesis. PLoS One 8:e72292. doi: 10.1371/journal.pone.0072292
Hu, Y., Gong, H., Lu, Z., Zhang, P., Zheng, S., Wang, J., et al. (2023). Variable tandem glycine-rich repeats contribute to cell death-inducing activity of a glycosylphosphatidylinositol-anchored Cell Wall protein that is associated with the pathogenicity of Sclerotinia sclerotiorum. Microbiol. Spectr. 11:e0098623. doi: 10.1128/spectrum.00986-23
Huang, Z., Lu, J., Liu, R., Wang, P., Hu, Y., Fang, A., et al. (2021). SsCat2 encodes a catalase that is critical for the antioxidant response, QoI fungicide sensitivity, and pathogenicity of Sclerotinia sclerotiorum. Fungal Genet. Biol. 149:103530. doi: 10.1016/j.fgb.2021.103530
Jahan, R., Siddique, S. S., Jannat, R., and Hossain, M. M. (2022). Cosmos white rot: first characterization, physiology, host range, disease resistance, and chemical control. J. Basic Microbiol. 62, 911–929. doi: 10.1002/jobm.202200098
Jiao, W., Ding, W., Rollins, J. A., Liu, J., Zhang, Y., Zhang, X., et al. (2023). Cross-talk and multiple control of target of rapamycin (TOR) in Sclerotinia sclerotiorum. Microbiol. Spectr. 11:e0001323. doi: 10.1128/spectrum.00013-23
Jiao, W., Yu, H., Chen, X., Xiao, K., Jia, D., Wang, F., et al. (2022a). The SsAtg1 activating autophagy is required for Sclerotia formation and pathogenicity in Sclerotinia sclerotiorum. J. Fungi (Basel) 8:1314. doi: 10.3390/jof8121314
Jiao, W., Yu, H., Cong, J., Xiao, K., Zhang, X., Liu, J., et al. (2022b). Transcription factor SsFoxE3 activating SsAtg8 is critical for sclerotia, compound appressoria formation, and pathogenicity in Sclerotinia sclerotiorum. Mol. Plant Pathol. 23, 204–217. doi: 10.1111/mpp.13154
Jurick, W. M., and Rollins, J. A. (2007). Deletion of the adenylate cyclase (sac1) gene affects multiple developmental pathways and pathogenicity in Sclerotinia sclerotiorum. Fungal Genet. Biol. 44, 521–530. doi: 10.1016/j.fgb.2006.11.005
Kim, Y. T., Prusky, D., and Rollins, J. A. (2007). An activating mutation of the Sclerotinia sclerotiorum pac1 gene increases oxalic acid production at low pH but decreases virulence. Mol. Plant Pathol. 8, 611–622. doi: 10.1111/j.1364-3703.2007.00423.x
Lehner, M. S., de Paula Júnior, T. J., Del Ponte, E. M., Mizubuti, E. S. G., and Pethybridge, S. J. (2017). Independently founded populations of Sclerotinia sclerotiorum from a tropical and a temperate region have similar genetic structure. PLoS One 12:e0173915. doi: 10.1371/journal.pone.0173915
Li, X., Guo, M., Xu, D., Chen, F., Zhang, H., Pan, Y., et al. (2015). The nascent-polypeptide-associated complex alpha subunit regulates the polygalacturonases expression negatively and influences the pathogenicity of Sclerotinia sclerotiorum. Mycologia 107, 1130–1137. doi: 10.3852/14-250
Li, M., Liang, X., and Rollins, J. A. (2012). Sclerotinia sclerotiorum γ-glutamyl transpeptidase (Ss-Ggt1) is required for regulating glutathione accumulation and development of sclerotia and compound appressoria. Mol. Plant-Microbe Interact. 25, 412–420. doi: 10.1094/MPMI-06-11-0159
Li, W., Lu, J., Yang, C., Arildsen, K., Li, X., and Xia, S. (2022). An amidase contributes to full virulence of Sclerotinia sclerotiorum. Int. J. Mol. Sci. 23:11207. doi: 10.3390/ijms231911207
Li, J., Mu, W., Veluchamy, S., Liu, Y., Zhang, Y., Pan, H., et al. (2018). The GATA-type IVb zinc-finger transcription factor SsNsd1 regulates asexual-sexual development and appressoria formation in Sclerotinia sclerotiorum. Mol. Plant Pathol. 19, 1679–1689. doi: 10.1111/mpp.12651
Li, R., Rimmer, R., Buchwaldt, L., Sharpe, A. G., Séguin-Swartz, G., and Hegedus, D. D. (2004). Interaction of Sclerotinia sclerotiorum with Brassica napus: cloning and characterization of endo- and exo-polygalacturonases expressed during saprophytic and parasitic modes. Fungal Genet. Biol. 41, 754–765. doi: 10.1016/j.fgb.2004.03.002
Li, T., Xiu, Q., Wang, J., Duan, Y., and Zhou, M. (2021). A putative MAPK kinase kinase gene Ssos4 is involved in mycelial growth, virulence, osmotic adaptation, and sensitivity to Fludioxonil and is essential for SsHog1 phosphorylation in Sclerotinia sclerotiorum. Phytopathology 111, 521–530. doi: 10.1094/PHYTO-07-20-0292-R
Li, J., Zhu, F., and Li, J. (2019). Expression of the histidine kinase gene Sshk correlates with Dimethachlone resistance in Sclerotinia sclerotiorum. Phytopathology 109, 395–401. doi: 10.1094/PHYTO-05-18-0156-R
Liang, X., Liberti, D., Li, M., Kim, Y.-T., Hutchens, A., Wilson, R., et al. (2015a). Oxaloacetate acetylhydrolase gene mutants of Sclerotinia sclerotiorum do not accumulate oxalic acid, but do produce limited lesions on host plants. Mol. Plant Pathol. 16, 559–571. doi: 10.1111/mpp.12211
Liang, X., Moomaw, E. W., and Rollins, J. A. (2015b). Fungal oxalate decarboxylase activity contributes to Sclerotinia sclerotiorum early infection by affecting both compound appressoria development and function. Mol. Plant Pathol. 16, 825–836. doi: 10.1111/mpp.12239
Liberti, D., Rollins, J. A., and Dobinson, K. F. (2013). Peroxysomal carnitine acetyl transferase influences host colonization capacity in Sclerotinia sclerotiorum. Mol. Plant-Microbe Interact. 26, 768–780. doi: 10.1094/MPMI-03-13-0075-R
Liu, L., Wang, Q., Zhang, X., Liu, J., Zhang, Y., and Pan, H. (2018). Ssams2, a gene encoding GATA transcription factor, is required for Appressoria formation and chromosome segregation in Sclerotinia sclerotiorum. Front. Microbiol. 9:3031. doi: 10.3389/fmicb.2018.03031
Lyu, X., Shen, C., Fu, Y., Xie, J., Jiang, D., Li, G., et al. (2015a). Comparative genomic and transcriptional analyses of the carbohydrate-active enzymes and secretomes of phytopathogenic fungi reveal their significant roles during infection and development. Sci. Rep. 5:15565. doi: 10.1038/srep15565
Lyu, X., Shen, C., Fu, Y., Xie, J., Jiang, D., Li, G., et al. (2015b). The microbial opsin homolog Sop1 is involved in Sclerotinia sclerotiorum development and environmental stress response. Front. Microbiol. 6:1504. doi: 10.3389/fmicb.2015.01504
Lyu, X., Shen, C., Fu, Y., Xie, J., Jiang, D., Li, G., et al. (2016). A small secreted virulence-related protein is essential for the necrotrophic interactions of Sclerotinia sclerotiorum with its host plants. PLoS Pathog. 12:e1005435. doi: 10.1371/journal.ppat.1005435
Pan, Y., Wei, J., Yao, C., Reng, H., and Gao, Z. (2018). SsSm1, a Cerato-platanin family protein, is involved in the hyphal development and pathogenic process of Sclerotinia sclerotiorum. Plant Sci. 270, 37–46. doi: 10.1016/j.plantsci.2018.02.001
Purdy, L. H. (1979). Sclerotinia sclerotiorum: history, diseases and symptomatology, host range, geographic distribution, and impact. Phytopathology 69, 875–880. doi: 10.1094/Phyto-69-875
Qi, T., Zhu, X., Tan, C., Liu, P., Guo, J., Kang, Z., et al. (2018). Host-induced gene silencing of an important pathogenicity factor PsCPK1 in Puccinia striiformis f. sp. tritici enhances resistance of wheat to stripe rust. Plant Biotechnol. J. 16, 797–807. doi: 10.1111/pbi.12829
Qin, L., Nong, J., Cui, K., Tang, X., Gong, X., Xia, Y., et al. (2023). SsCak1 regulates growth and pathogenicity in Sclerotinia sclerotiorum. Int. J. Mol. Sci. 24:12610. doi: 10.3390/ijms241612610
Qu, X., Yu, B., Liu, J., Zhang, X., Li, G., Zhang, D., et al. (2014). MADS-box transcription factor SsMADS is involved in regulating growth and virulence in Sclerotinia sclerotiorum. Int. J. Mol. Sci. 15, 8049–8062. doi: 10.3390/ijms15058049
Rana, K., Ding, Y., Banga, S. S., Liao, H., Zhao, S., Yu, Y., et al. (2021). Sclerotinia sclerotiorum Thioredoxin1 (SsTrx1) is required for pathogenicity and oxidative stress tolerance. Mol. Plant Pathol. 22, 1413–1426. doi: 10.1111/mpp.13127
Rana, K., Yuan, J., Liao, H., Banga, S. S., Kumar, R., Qian, W., et al. (2022). Host-induced gene silencing reveals the role of Sclerotinia sclerotiorum oxaloacetate acetylhydrolase gene in fungal oxalic acid accumulation and virulence. Microbiol. Res. 258:126981. doi: 10.1016/j.micres.2022.126981
Rollins, J. A. (2003). The Sclerotinia sclerotiorum pac1 gene is required for sclerotial development and virulence. Mol. Plant-Microbe Interact. 16, 785–795. doi: 10.1094/MPMI.2003.16.9.785
Sang, H., Chang, H.-X., and Chilvers, M. I. (2019). A Sclerotinia sclerotiorum transcription factor involved in Sclerotial development and virulence on pea. mSphere 4, e00615–e00618. doi: 10.1128/mSphere.00615-18
Seifbarghi, S., Borhan, M. H., Wei, Y., Ma, L., Coutu, C., Bekkaoui, D., et al. (2020). Receptor-like kinases BAK1 and SOBIR1 are required for necrotizing activity of a novel Group of Sclerotinia sclerotiorum necrosis-inducing effectors. Front. Plant Sci. 11:1021. doi: 10.3389/fpls.2020.01021
Sutton, D. C., and Deverall, B. J. (1983). Studies on infection of bean (Phaseolus vulgaris) and soybean (Glycine max) by ascospores of Sclerotinia sclerotiarum. Plant Pathol. 32, 251–261. doi: 10.1111/j.1365-3059.1983.tb02832.x
Tian, L., Li, J., Xu, Y., Qiu, Y., Zhang, Y., and Li, X. (2024). A MAP kinase cascade broadly regulates the lifestyle of Sclerotinia sclerotiorum and can be targeted by HIGS for disease control. Plant J. 118, 324–344. doi: 10.1111/tpj.16606
Tian, B., Xie, J., Fu, Y., Cheng, J., Li, B., Chen, T., et al. (2020). A cosmopolitan fungal pathogen of dicots adopts an endophytic lifestyle on cereal crops and protects them from major fungal diseases. ISME J. 14, 3120–3135. doi: 10.1038/s41396-020-00744-6
Wang, X., Li, Q., Niu, X., Chen, H., Xu, L., and Qi, C. (2009). Characterization of a canola C2 domain gene that interacts with PG, an effector of the necrotrophic fungus Sclerotinia sclerotiorum. J. Exp. Bot. 60, 2613–2620. doi: 10.1093/jxb/erp104
Wang, Y., Xu, Y., Wei, J., Zhang, J., Wu, M., Li, G., et al. (2023). Sclerotinia sclerotiorum agglutinin modulates Sclerotial development, pathogenicity and response to abiotic and biotic stresses in different manners. J. Fungi (Basel) 9:737. doi: 10.3390/jof9070737
Wei, J., Yao, C., Zhu, Z., Gao, Z., Yang, G., and Pan, Y. (2023). Nitrate reductase is required for sclerotial development and virulence of Sclerotinia sclerotiorum. Front. Plant Sci. 14:1096831. doi: 10.3389/fpls.2023.1096831
Williams, J. R., and Stelfox, D. (1980). Influence of farming practices in Alberta on germination and apothecium production of sclerotia of Sclerotinia sclerotiorum. Can. J. Plant Pathol. 2, 169–172. doi: 10.1080/07060668009501435
Wu, J., Yin, S., Lin, L., Liu, D., Ren, S., Zhang, W., et al. (2022). Host-induced gene silencing of multiple pathogenic factors of Sclerotinia sclerotiorum confers resistance to Sclerotinia rot in Brassica napus. Crop J. 10, 661–671. doi: 10.1016/j.cj.2021.08.007
Xiao, X., Xie, J., Cheng, J., Li, G., Yi, X., Jiang, D., et al. (2014). Novel secretory protein Ss-Caf1 of the plant-pathogenic fungus Sclerotinia sclerotiorum is required for host penetration and normal sclerotial development. Mol. Plant-Microbe Interact. 27, 40–55. doi: 10.1094/MPMI-05-13-0145-R
Xie, C., Shang, Q., Mo, C., Xiao, Y., Wang, G., Xie, J., et al. (2021). Early secretory pathway-associated proteins SsEmp24 and SsErv25 are involved in morphogenesis and pathogenicity in a filamentous Phytopathogenic fungus. MBio 12:e0317321. doi: 10.1128/mBio.03173-21
Xu, L., and Chen, W. (2013). Random T-DNA mutagenesis identifies a cu/Zn superoxide dismutase gene as a virulence factor of Sclerotinia sclerotiorum. Mol. Plant-Microbe Interact. 26, 431–441. doi: 10.1094/MPMI-07-12-0177-R
Xu, T., Li, J., Yu, B., Liu, L., Zhang, X., Liu, J., et al. (2018). Transcription factor SsSte12 was involved in mycelium growth and development in Sclerotinia sclerotiorum. Front. Microbiol. 9:2476. doi: 10.3389/fmicb.2018.02476
Xu, Y., Qiu, Y., Zhang, Y., and Li, X. (2023). A cAMP phosphodiesterase is essential for sclerotia formation and virulence in Sclerotinia sclerotiorum. Front. Plant Sci. 14:1175552. doi: 10.3389/fpls.2023.1175552
Xu, Y., Tan, J., Lu, J., Zhang, Y., and Li, X. (2024). RAS signalling genes can be used as host-induced gene silencing targets to control fungal diseases caused by Sclerotinia sclerotiorum and Botrytis cinerea. Plant Biotechnol. J. 22, 262–277. doi: 10.1111/pbi.14184
Yajima, W., Liang, Y., and Kav, N. N. V. (2009). Gene disruption of an arabinofuranosidase/beta-xylosidase precursor decreases Sclerotinia sclerotiorum virulence on canola tissue. Mol. Plant-Microbe Interact. 22, 783–789. doi: 10.1094/MPMI-22-7-0783
Yang, C., Li, W., Huang, X., Tang, X., Qin, L., Liu, Y., et al. (2022). SsNEP2 contributes to the virulence of Sclerotinia sclerotiorum. Pathogens 11:446. doi: 10.3390/pathogens11040446
Yang, G., Tang, L., Gong, Y., Xie, J., Fu, Y., Jiang, D., et al. (2018). A cerato-platanin protein SsCP1 targets plant PR1 and contributes to virulence of Sclerotinia sclerotiorum. New Phytol. 217, 739–755. doi: 10.1111/nph.14842
Yang, C., Tang, L., Qin, L., Zhong, W., Tang, X., Gong, X., et al. (2023). mRNA turnover protein 4 is vital for fungal pathogenicity and response to oxidative stress in Sclerotinia sclerotiorum. Pathogens 12:281. doi: 10.3390/pathogens12020281
Yu, Y., Du, J., Wang, Y., Zhang, M., Huang, Z., Cai, J., et al. (2019). Survival factor 1 contributes to the oxidative stress response and is required for full virulence of Sclerotinia sclerotiorum. Mol. Plant Pathol. 20, 895–906. doi: 10.1111/mpp.12801
Yu, P.-L., and Rollins, J. A. (2022). The cAMP-dependent protein kinase a pathway perturbs autophagy and plays important roles in development and virulence of Sclerotinia sclerotiorum. Fungal Biol. 126, 20–34. doi: 10.1016/j.funbio.2021.09.004
Yu, Y., Xiao, J., Du, J., Yang, Y., Bi, C., and Qing, L. (2016). Disruption of the gene encoding Endo-β-1, 4-xylanase affects the growth and virulence of Sclerotinia sclerotiorum. Front. Microbiol. 7:1787. doi: 10.3389/fmicb.2016.01787
Zhang, H., Li, Y., Lai, W., Huang, K., Li, Y., Wang, Z., et al. (2021). SsATG8 and SsNBR1 mediated-autophagy is required for fungal development, proteasomal stress response and virulence in Sclerotinia sclerotiorum. Fungal Genet. Biol. 157:103632. doi: 10.1016/j.fgb.2021.103632
Zhang, M., Liu, Y., Li, Z., She, Z., Chai, M., Aslam, M., et al. (2021). The bZIP transcription factor GmbZIP15 facilitates resistance against Sclerotinia sclerotiorum and Phytophthora sojae infection in soybean. iScience 24:102642. doi: 10.1016/j.isci.2021.102642
Zhang, J., Wang, Y., Du, J., Huang, Z., Fang, A., Yang, Y., et al. (2019). Sclerotinia sclerotiorum Thioredoxin reductase is required for oxidative stress tolerance, virulence, and Sclerotial development. Front. Microbiol. 10:233. doi: 10.3389/fmicb.2019.00233
Zhang, J., Xiao, K., Li, M., Hu, H., Zhang, X., Liu, J., et al. (2022). SsAGM1-mediated uridine diphosphate-N-Acetylglucosamine synthesis is essential for development, stress response, and pathogenicity of Sclerotinia sclerotiorum. Front. Microbiol. 13:938784. doi: 10.3389/fmicb.2022.938784
Keywords: virulence, life cycle, oxalic acid, cell wall degrading enzymes, effector proteins, host-induced gene silencing
Citation: Zhu Y, Wu C, Deng Y, Yuan W, Zhang T and Lu J (2024) Recent advances in virulence of a broad host range plant pathogen Sclerotinia sclerotiorum: a mini-review. Front. Microbiol. 15:1424130. doi: 10.3389/fmicb.2024.1424130
Edited by:
Daolong Dou, Nanjing Agricultural University, ChinaReviewed by:
Minhui Li, South China Agricultural University, ChinaCong Jiang, Northwest A&F University, China
Copyright © 2024 Zhu, Wu, Deng, Yuan, Zhang and Lu. This is an open-access article distributed under the terms of the Creative Commons Attribution License (CC BY). The use, distribution or reproduction in other forums is permitted, provided the original author(s) and the copyright owner(s) are credited and that the original publication in this journal is cited, in accordance with accepted academic practice. No use, distribution or reproduction is permitted which does not comply with these terms.
*Correspondence: Junxing Lu, junxlu@163.com
†These authors have contributed equally to this work