- 1Key Laboratory of Respiratory Disease, People’s Hospital of Yangjiang, Yangjiang, China
- 2Guangzhou Institute of Respiratory Health, First Affiliated Hospital of Guangzhou Medical University, Guangzhou, China
Tigecycline (TGC) is currently used to treat carbapenem-resistant Acinetobacter baumannii (CRAB) infections, while eravacycline (ERV), a new-generation tetracycline, holds promise as a novel therapeutic option for these infections. However, differences in resistance mechanism between ERV and TGC against A. baumannii remain unclear. This study sought to compare the characteristics and mechanisms of ERV and TGC resistance among clinical A. baumannii isolates. A total of 492 isolates, including 253 CRAB and 239 carbapenem-sensitive A. baumannii (CSAB) isolates, were collected from hospitalized patients in China. The MICs of ERV and TGC against A. baumannii were determined by broth microdilution. Genetic mutations and expressions of adeB, adeG, adeJ, adeS, adeL, and adeN in resistant strains were examined by PCR and qPCR, respectively. The in vitro recombination experiments were used to verify the resistance mechanism of ERV and TGC in A. baumannii. The MIC90 of ERV in CRAB and CSAB isolates were lower than those of TGC. A total of 24 strains resistant to ERV and/or TGC were categorized into three groups: only ERV-resistant (n = 2), both ERV- and TGC-resistant (n = 7), and only TGC-resistant (n = 15). ST208 (75%, n = 18) was a major clone that has disseminated in all three groups. The ISAba1 insertion in adeS was identified in 66.7% (6/9) of strains in the only ERV-resistant and both ERV- and TGC-resistant groups, while the ISAba1 insertion in adeN was found in 53.3% (8/15) of strains in the only TGC-resistant group. The adeABC and adeRS expressions were significantly increased in the only ERV-resistant and both ERV- and TGC-resistant groups, while the adeABC and adeIJK expressions were significantly increased and adeN was significantly decreased in the only TGC-resistant group. Expression of adeS with the ISAba1 insertion in ERV- and TGC-sensitive strains significantly increased the ERV and TGC MICs and upregulated adeABC and adeRS expressions. Complementation of the wildtype adeN in TGC-resistant strains with the ISAba1 insertion in adeN restored TGC sensitivity and significantly downregulated adeIJK expression. In conclusion, our data illustrates that ERV is more effective against A. baumannii clinical isolates than TGC. ERV resistance is correlated with the ISAba1 insertion in adeS, while TGC resistance is associated with the ISAba1 insertion in adeN or adeS in A. baumannii.
1 Introduction
Acinetobacter baumannii is a serious hospital-acquired pathogen associated with wounds, urinary tract infections, bloodstream infections, pneumonia, and meningitis (Gerson et al., 2018). The attributable mortality of patients with A. baumannii ventilator-associated pneumonia and bloodstream infections is as high as 54% in the intensive care unit (ICU) (Alosaimy et al., 2022). In recent years, the widespread use of carbapenem antibiotics has caused the emergence and spread of carbapenem-resistant Acinetobacter baumannii (CRAB), posing a significant threat to global public health (Liu et al., 2024). Given the widespread resistance of CRAB to almost all commonly used antibiotics, available treatment options are extremely limited, with none able to effectively reduce mortality (O'Donnell et al., 2021). The antibiotics currently used to treat CRAB infection include tigecycline (TGC), polymyxins, and the β-lactamase inhibitor, sulbactam (Serapide et al., 2024). However, the antibiotics described above have some limitations in clinical application. Polymyxins can cause nephrotoxicity, which substantially narrows the therapeutic window (LaPlante et al., 2018). TGC suffers from pharmacokinetic issues, such as low plasma levels, limiting its clinical use (Isler et al., 2019). Sulbactam used as monotherapy has a high resistance rate, it is usually used in combination therapies (Deveci et al., 2012). Therefore, novel antimicrobials have been explored for the treatment of CRAB infections in recent years, including cefiderocol, new tetracycline analogues (eravacycline and TP-6076), and new β-lactamase inhibitor combinations (Sulbactam-ETX2514, meropenem- WCK 4234, and LN-1-255-carbapenem) (Isler et al., 2019). Among these novel antibiotics, ERV demonstrates superior activity against CRAB and holds promising prospects for the treatment of CRAB infections (Isler et al., 2019).
Eravacycline (ERV) is a novel fluorocycline antibacterial agent that is structurally similar to TGC with two modifications to the D-ring of the tetracycline core. Those modifications greatly improved its bioavailability and made it exhibiting more potent in vitro activity against CRAB than TGC (Zhanel et al., 2016). ERV was approved by the Food and Drug Administration (FDA) for treating complicated intra-abdominal infections in 2018 (Alosaimy et al., 2020). It has broad-spectrum activity against both gram-positive and gram-negative bacteria, including some antibiotic-resistant strains, such as multidrug-resistant A. baumannii, vancomycin-resistant Enterococcus, methicillin-resistant Staphylococcus aureus, and carbapenem-resistant Enterobacteriaceae (Sutcliffe et al., 2013; Monogue et al., 2016; Li Y. et al., 2022). As a result, ERV is currently in development as an intravenous (i.v.) and oral medication for treating serious infections caused by antibiotic-resistant bacteria (Zhanel et al., 2016). Notably, ERV is four times as effective as TGC against multidrug-resistant A. baumannii (Zhanel et al., 2016). Patients with complicated intra-abdominal CRAB infections achieved a 100% clinical and microbiologic cure following ERV treatment (Poulakou et al., 2018). Thus, ERV is a promising option for treating CRAB infections.
TGC resistance is primarily associated with overexpression of RND efflux pumps, including AdeABC, AdeFGH, and AdeIJK, that are controlled by the transcriptional regulators AdeRS, AdeL, and AdeN, respectively (Lucaßen et al., 2021b). Mutations or insertions in these regulatory genes can also promote TGC resistance by increasing efflux pump expression (Hornsey et al., 2010). In addition, other genes, including the ribosomal S10 protein-encoding gene rpsJ, MFS efflux pumps tet(A) and its regulatory factors tetR, plasmid-mediated tet(X5), antibiotic targets of action rrf, rpoB, and global regulator soxR, were also reported to be associated with TGC resistance (Beabout et al., 2015; Wang et al., 2019; Hua et al., 2021; Sun et al., 2023). Shi et al. showed that a deletion mutation in the adeS gene of induced resistant strains correlates with high AdeABC expression and increased ERV resistance (Shi et al., 2020). However, the mechanism of A. baumannii resistance to ERV and the differences between the ERV and TGC resistance mechanisms require additional clarification.
In the present study, we sought to compare the traits and resistance mechanisms in ERV- and TGC-resistant A. baumanni isolates from China. The in vitro antimicrobial activity of ERV, TGC, and other clinical agents was examined; the presence of clonality and carbapenemase resistance genes were analyzed. Furthermore, efflux pump inhibition assays, molecular sequencing, quantitative real-time PCR (qRT-PCR) and functional verification experiments were performed to explore various resistance mechanism of ERV and TGC in A. baumanni.
2 Materials and methods
2.1 Bacterial isolates, antibiotics, and growth conditions
A total of 492 non-duplicate clinical A. baumannii isolates, including 253 CRAB and 239 CSAB, were collected from patients who were admitted to Yangjiang People’s Hospital, a 2,000-bed tertiary hospital in Guangdong Province of China from January 2018 to December 2022. The A. baumannii isolates were identified using a VITEK 2 compact system (BioMérieux, Marcy l’Etoile, France) and further confirmed by VITEK mass spectrometry (BioMérieux, Marcy l’Etoile, France). All strains were cultured in Mueller-Hinton (MH) (Oxoid, Basingstoke, UK) or Luria-Bertani (LB) broth (Oxoid, Basingstoke, UK) at 37°C. Escherichia coli ATCC25922 was used as a quality control strain. All procedures performed were approved by the Ethical Committee of Yangjiang People’s Hospital and were in accordance with the 1964 Helsinki Declaration and its later amendments. ERV and TGC were obtained from MedChem Express (Shanghai, China), and the other antimicrobials were purchased from Meilunbio (Dalian, China).
2.2 Antimicrobial susceptibility testing
The minimum inhibitory concentration (MIC) of ERV, TGC, polymyxin B, meropenem, imipenem, levofloxacin, gentamicin, cefepime, amikacin, ceftazidime, minocycline, piperacillin/tazobactam and ampicillin/sulbactam against A. baumannii isolates were determined using broth microdilution method according to the guidelines of Clinical and Laboratory Standards Institute (CLSI) M100-S34 (Clinical and Laboratory Standards Institute, 2024). Since the A. baumannii MIC breakpoints for ERV and TGC have not yet been established by CLSI and the FDA, the MIC values were categorized into three levels according to prior studies (Marchaim et al., 2014; Abdallah et al., 2015): ≤2 mg/L (susceptible), 4 mg/L (intermediate), and ≥ 8 mg/L (resistant). The susceptibility results for other antibiotics were determined based on CLSI breakpoints. In this study, CRAB strains were defined as those with an imipenem and/or meropenem MIC ≥8 mg/L, while CSAB strains were defined as those with imipenem and meropenem MICs ≤4 mg/L (Chen et al., 2023).
2.3 Polymerase chain reaction for multilocus sequence typing, carbapenemase, and genetic mutations in the resistance genes
Total DNA from ERV- and/or TGC-resistant strains were extracted using Lysis Buffer for Microorganisms to Direct PCR (Takara Bio Inc., Japan). PCR was performed using PCR Mastermix (Thermo Fisher Scientific, Waltham, MA) according to the manufacturer’s instructions. Multilocus sequence typing (MLST) of the strains was determined by PCR and sequence alignment, as described previously (Li T. et al., 2022). The carbapenemase resistance genes, blaOXA, blaKPC, blaIMP, blaVIM, blaSIM, and blaNDM-1 were detected by PCR (Ramirez et al., 2020). Genetic mutations of the RND efflux pump regulatory genes, including adeR, adeS, adeL, adeN, and other genes associated with TGC resistance, including rpsJ, tet(A), tetR, tet(X5), rrf, rpoB, and soxR (Beabout et al., 2015; Wang et al., 2019; Hua et al., 2021; Sun et al., 2023), were amplified by PCR and sequenced. Mutations in the above-mentioned genes in ERV- and TGC-resistant A. baumannii were identified by comparing them with the A. baumannii strain ATCC BAA1605 (GenBank accession number: CP058625) (Ten et al., 2021). The primers used for PCR are listed in Supplementary Tables S1–S3.
2.4 Efflux pump inhibition assays
The efflux pump activity in ERV- and/or TGC-resistant A. baumannii isolates was detected using the efflux pump inhibitors (EPIs), Phe-Arg-β-naphthylamide (PaβN; MCE, Shanghai, China) and carbonyl cyanide m-chlorophenylhydrazone (CCCP; MCE, Shanghai, China). ERV and TGC MICs were determined using the agar dilution method in the presence and absence of PAβN (50 mg/L) or CCCP (16 mg/L) in ERV- and TGC-resistant strains (Jo and Ko, 2021). Significant inhibition of the efflux pumps was determined by the reduction of MIC to a quarter (or more) of their baseline values in the presence of EPI (Zheng et al., 2018).
2.5 Quantitative real-time PCR analysis
Expression levels of the RND efflux pump genes (adeB, adeG, and adeJ) and their regulators (adeS, adeL, and adeN) were determined in ERV- and/or TGC-resistant A. baumannii using qRT-PCR. Ten ERV- and TGC-susceptible strains selected from similar sources of the resistant isolates were used as sensitive control strains. Expressions of the target genes in the three groups of resistant strains were compared with their expressions in the sensitive strains. Since adeA, adeB, and adeC are located within the same operon, adeB was considered representative of adeABC efflux pump expression in this study (Yang et al., 2019). The other genes shared the same principle. In brief, overnight cultures of the bacterial strains were diluted 1:100 in 10 mL of LB broth and incubated at 37°C with 220 rpm shaking until the growth had reached a logarithmic phase. Total bacterial RNA was extracted with RNA Extraction Kit (Vazyme, Nanjing, China), and cDNA was synthesized with a HiScriptIII-RT SuperMix for qRT-PCR kit (Vazyme, Nanjing, China). Finally, qRT-PCR was performed using a ChamQ Universal SYBR qRT-PCR Master Mix (Vazyme, Nanjing, China) in a LightCycler480II system (Roche, Basel, Switzerland), with the following parameters: 1 cycle at 95°C for 30 s, followed by 45 cycles of 95°C for 10 s and 60°C for 30 s (Li et al., 2024). Each sample was run in triplicate. Expressions of the target genes were normalized relative to the internal control gene, rpoB (Jo and Ko, 2021). Threshold cycle (Ct) numbers were confirmed with qRT-PCR system software, and the data were analyzed using the 2−ΔΔCt method. The CRAB-20 isolate, containing all the efflux pumps involved in this study and sensitive to both ERV and TGC, was used as the reference strain (expression = 1). The primers used for qRT-PCR are listed in Supplementary Table S4.
2.6 Functional verification of efflux pump gene mutations
Previous study has reported that ISAba1 is inserted within the adeS gene. The Pout promoter of the ISAba1 sequence will then replace the native promoter and drive the translation of a truncated adeS gene. The truncated AdeS protein was able to activate AdeR and then enhance the adeABC gene expression. Overexpression of AdeABC ultimately confers resistance to TGC in A. baumannii (Sun et al., 2012). To confirm the role of the ISAba1 insertion in adeS on ERV resistance, adeS with the ISAba1 insertion was amplified from the CRAB-42 isolate by PCR and integrated into the expression plasmid, pACYC184, with BamHI and ClaI cloning sites (Guo et al., 2021). Positive clones were screened with chloramphenicol and verified by PCR and sequencing. The resulting constructs (named: padeS-ISAbal) were separately transformed into five strains both susceptible to ERV and TGC by electroporation and further identified by PCR and sequencing. The pACYC184 and pACYC184 inserted with wildtype adeS (named: padeS-WT) were separately introduced into the same strains as a control. Previous research has shown that the insertion of ISAba1 within the adeN gene led to the disruption of adeN, which caused the loss of adeN repressive function on adeIJK gene and ultimately resulted in the overexpression of AdeIJK (Lucaßen et al., 2021a). To assess the impact of the ISAba1 insertion in adeN on TGC resistance, wildtype adeN with its own promoter region was amplified from the CRAB-86 isolate and cloned into the pACYC184 plasmid as described above. The complementary plasmid (named: padeN-WT) was verified by PCR and sequencing and transformed into five TGC-resistant strains with ISAba1 inserted in adeN by electroporation. The pACYC184 was transferred into the same strains as a control. The susceptibility of ERV and TGC was then evaluated in the constructed strains using broth microdilution method. Expressions of adeB, adeS, or adeJ in the constructed strains were further confirmed by qRT-PCR. The strains and primers used for the functional verification experiment are listed in Supplementary Tables S5, S6.
2.7 Statistical analysis
The Student’s t-test was used for continuous variables. All tests were performed using IBM SPSS Statistics (version 22.0; IBM, Chicago, United States). p-values <0.05 are regarded as statistically significant.
3 Results
3.1 Characteristics and in vitro antimicrobial susceptibility of the bacterial strains
A total of 492 clinical A. baumannii isolates, including 253 isolates of CRAB and 239 isolates of CSAB, were analyzed in this study. The A. baumannii strains were isolated from sputum (44.5%), wound secretions (24.6%), urine (13.6%), blood (8.9%), and other sources (including bronchoalveolar lavage fluid, ascites, abscess, bile, cerebrospinal fluid, and pleural effusion) (8.3%) (Supplementary Table S7). CRAB was primarily found in sputum and wound secretions, while CSAB isolates were relatively dispersed. The susceptibility of 492 A. baumannii isolates to ERV, TGC, and other antibiotics is summarized in Table 1. The results show that 2.4 and 5.9% of the CRAB isolates were resistant to ERV and TGC, respectively, while only 1.3 and 2.9% of the CSAB isolates were resistant to these antibiotics, respectively. The MIC90 of ERV for CRAB and CSAB was 1 mg/L and 0.5 mg/L, respectively, which was lower than the MIC90 of TGC for CRAB (2 mg/L) and CSAB (1 mg/L), suggesting that ERV is more effective against A. baumannii than TGC. Additionally, CRAB exhibited resistance to other commonly used antibiotics, including levofloxacin, gentamicin, cefepime, amikacin, ceftazidime, piperacillin/tazobactam, and ampicillin/sulbactam; however, CSAB was sensitive to these drugs.
3.2 Comparison of the characteristics of ERV- and/or TGC-resistant isolates
A total of 24 strains, identified as resistant to ERV and/or TGC, were categorized into three groups: only ERV-resistant (n = 2), both ERV- and TGC-resistant (n = 7), and only TGC-resistant (n = 15) (Table 2). The source, clinical department, MLST, and infected populations of each group had similar characteristics (Table 2). The 24 resistant strains came from various sources, including sputum (n = 11, 45.8%), wound secretions (n = 8, 33.3%), and others (n = 5, 20.8%). They were primarily obtained from patients in the ICU (n = 11, 45.8%) and individuals >60 years of age (n = 17, 71%). Three patients infected with a resistant CRAB strain had a fatal clinical outcome (n = 3, 12.5%). Most (n = 18, 75%) of the strains belonged to ST208, and all resistant CRAB isolates contained blaOXA-23 carbapenemases. However, there were clear differences in the ERV and TGC MICs of the three groups. The ERV MICs were 2–4x higher than the TGC MICs in the only ERV-resistant group; the TGC MICs were 1–4x higher than the ERV MICs in the both ERV- and TGC-resistant group. Meanwhile, the TGC MICs were 4–64x higher than the ERV MICs in the TGC-resistant group, suggesting that the antimicrobial activity of ERV is superior to TGC.
The susceptibilities of other commonly used antibiotics in the three groups of resistant strains are summarized in Supplementary Table S8. The CRAB isolates that were resistant to ERV and/or TGC exhibited high resistance to levofloxacin, minocycline, amikacin, gentamicin, doxycycline, and cefepime, but still showed sensitivity to polymyxin B. The CSAB strains resistant to ERV and/or TGC only exhibited cross-resistance with minocycline and doxycycline, while remaining sensitive to other commonly used antibiotics.
3.3 Effect of the efflux pump inhibitor on the ERV- and/or TGC-resistant isolates
To investigate the potential role of the efflux pump in ERV- and/or TGC-resistant A. baumannii strains, the effect of EPIs on the ERV and TGC MICs of resistant strains was assessed. The addition of two EPIs to the only ERV-resistant group significantly reduced the ERV MIC by 4–32x but did not have a significant effect on the TGC MIC (Table 3). The addition of two EPIs to both ERV- and TGC-resistant group significantly reduced the MICs of both antibiotics. After adding PaβN, the ERV and TGC MICs decreased by ≥4x in 100% (n = 7) or 85.7% (n = 6) of strains, respectively, and after adding CCCP, the ERV and TGC MICs significantly decreased in 71.4% (n = 5) or 57.1% (n = 4) of strains, respectively. In the only TGC-resistant group, the TGC MIC decreased by ≥4x in the presence of PaβN or CCCP in 77.3% (n = 11) or 66.7% (n = 10) of the resistant isolates, while the ERV MIC showed no significant change. These results illustrated that EPIs can significantly reduce the ERV and TGC MICs of resistant strains, suggesting that resistance to these antibiotics may be related to the differential expression of efflux pumps.
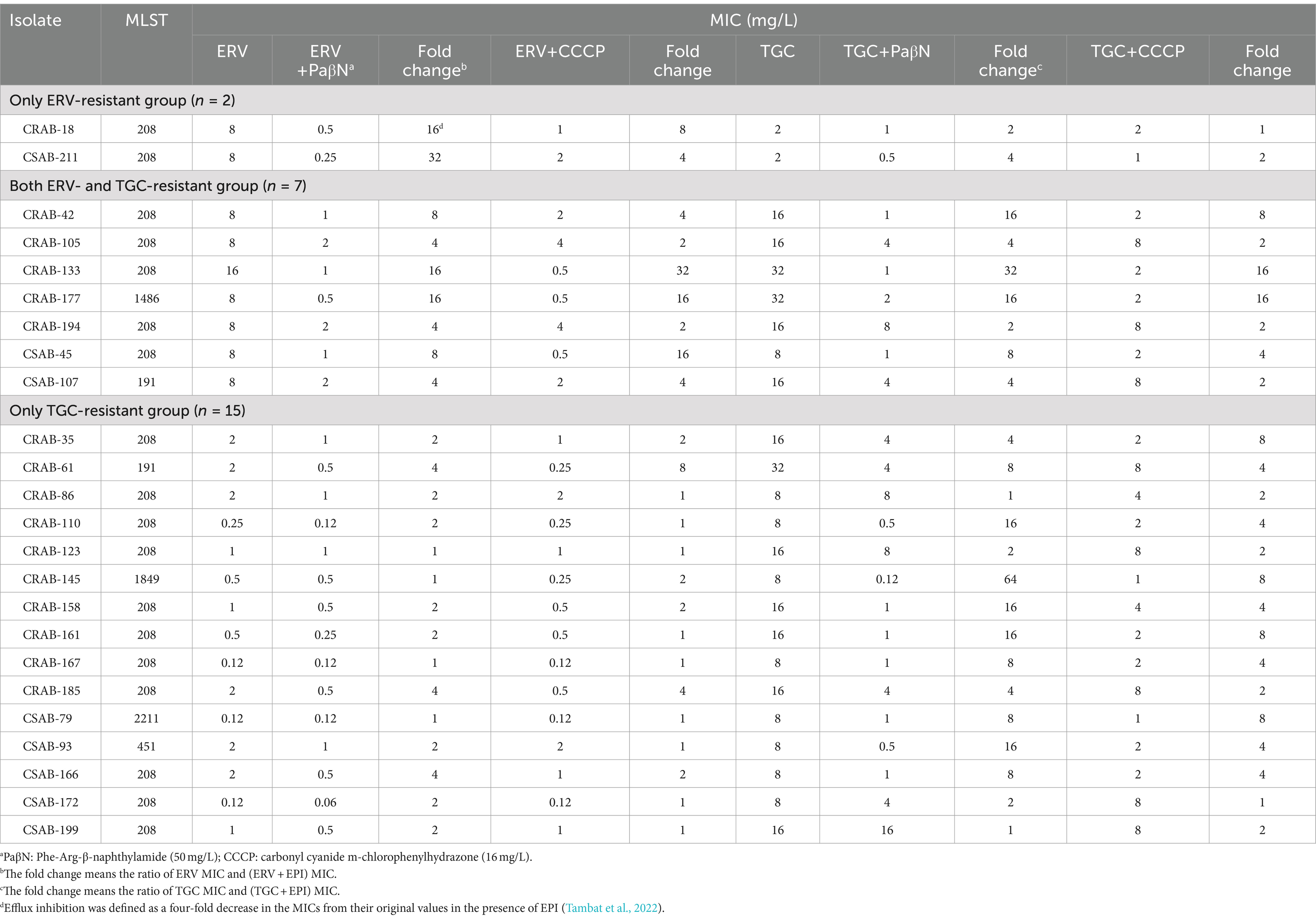
Table 3. MICs of ERV or TGC against the resistant isolates in the absence or presence of efflux pump inhibitors.
3.4 Resistance mechanisms of ERV- and/or TGC-resistant strains
The results described above suggest that efflux pumps may be involved in ERV and TGC resistance. To further evaluate this, PCR and sequencing were used to identify mutations in the regulator genes of the RND efflux pump. An ISAba1 insertion in adeS was detected in both strains from the only ERV-resistant group, while no mutations were detected in other genes. Four strains (57.1%, 4/7) in both ERV- and TGC-resistant group had an ISAba1 insertion in adeS, and one had a Q91K mutation in adeN. Meanwhile, eight strains (53.3%, 8/15) in the only TGC-resistant group had an ISAba1 insertion mutation in adeN, one had a double point mutation (T18R and A153D) in adeN, one had a double point mutation (L29R and K131N) in adeS, and two had mutations in adeR, V14G and A91V, respectively (Table 4). In addition, we further assessed the effect of various resistance mechanisms other than RND-type efflux pumps on ERV and TGC resistance, including rpsJ, tet(A), tetR, soxR, tet(X5), rrf, and rpoB. The results showed that a N27P mutation of TetR was detected in CRAB-86 from the only TGC-resistant group, a M247S mutation of Tet(A) in CRAB-194 and a V57I mutation of rpsJ in CRAB-105 were detected from both ERV- and TGC-resistant group, while no mutations were detected in other genes. Moreover, tet(X5) was not identified in all the 24 strains (Supplementary Table S9).
Relative expressions of the RND efflux pump genes were then compared between the resistant and sensitive strains. The expressions of adeB and adeS were significantly higher in both strains from the only ERV-resistant group than in the sensitive strains. No significant differences were found in the expression of adeG, adeL, adeJ, and adeN. The expressions of adeB and adeS were significantly higher in 100% (n = 7) and 57.1% (n = 4) of strains from the both ERV- and TGC-resistant group than in the sensitive strains, respectively, but no significant differences were found in the expression of other efflux pump genes. The expressions of adeB and adeJ were significantly higher in 46.7% (n = 7) and 60.0% (n = 9) of strains from the only TGC-resistant group, respectively, while the expression of adeN was significantly lower in 53.3% (n = 8) of the strains. The expression of adeS, adeG, and adeL did not change significantly (Figures 1A–D, Supplementary Figures S1A,B).
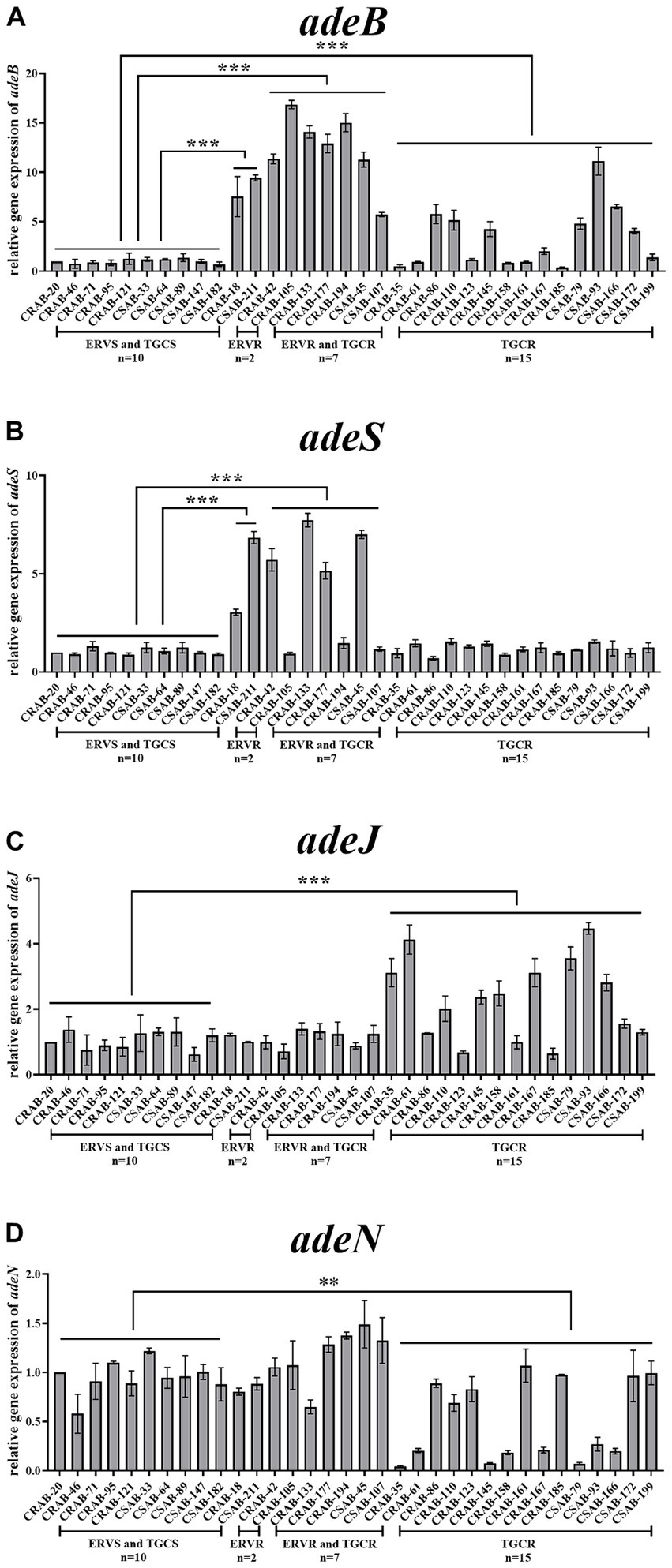
Figure 1. Comparison of the relative expressions of efflux pump genes in ERV- and/or TGC-resistant isolates. Relative expression of adeB (A), adeS (B), adeJ (C), and adeN (D) were assessed by qRT-PCR analysis. The housekeeping gene, rpoB, was used as the endogenous reference gene. CRAB-20 was used as the reference strain (expression = 1.0). All qRT-PCRs were carried out in triplicate. ∗∗∗p < 0.01, ∗∗p < 0.05. ERVS and TGCS: strains sensitive to both ERV and TGC; ERVR: strains only resistant to ERV; ERVR and TGCR: strains resistant to both ERV and TGC; TGCR: strains only resistant to TGC.
3.5 Validation of the role of the ISAba1 insertion in adeS or adeN on ERV and/or TGC antibiotic resistance
The results described above revealed significant differences in the gene mutations observed in the three groups of resistant strains. The ISAba1 insertion in adeS was identified in 66.7% (6/ 9) of strains in the only ERV-resistant and both ERV- and TGC-resistant groups, while the ISAba1 insertion in adeN was found in 53.3% (8/15) of strains in the only TGC-resistant group. These findings suggest that the mechanisms of A. baumannii resistance to ERV and TGC may differ. To evaluate the impact of the ISAba1 insertion in adeS on ERV resistance, adeS with the ISAba1 insertion was cloned into the expression plasmid, pACYC184, and transformed into five strains that were sensitive to both ERV and TGC. Changes in the ERV and TGC MICs were then measured in the constructed strains. Strains transformed with padeS-ISAba1 exhibited an 8–16x increase in ERV MIC and a 4–16x increase in TGC MIC. All strains became resistant to ERV by acquiring padeS-ISAba1, and 80% (4/5) of the strains became resistant to TGC. No significant change in ERV and TGC MIC was found in strains transformed with padeS-WT or pACYC184 (Table 5). This result suggests that the ISAba1 insertion in adeS can confer resistance to ERV and TGC in A. baumannii. Expression levels of adeB and adeS in the transformed strains were further confirmed by qRT-PCR. The expressions of adeB and adeS were significantly upregulated in strains transformed with padeS-ISAba1, while the expression of them in strains transformed with padeSWT or pACYC184 showed no significant change (Figures 2A,B). This finding indicates that the ISAba1 insertion in adeS can enhance the expression of the efflux pump genes, adeABC, and its regulators, adeRS.
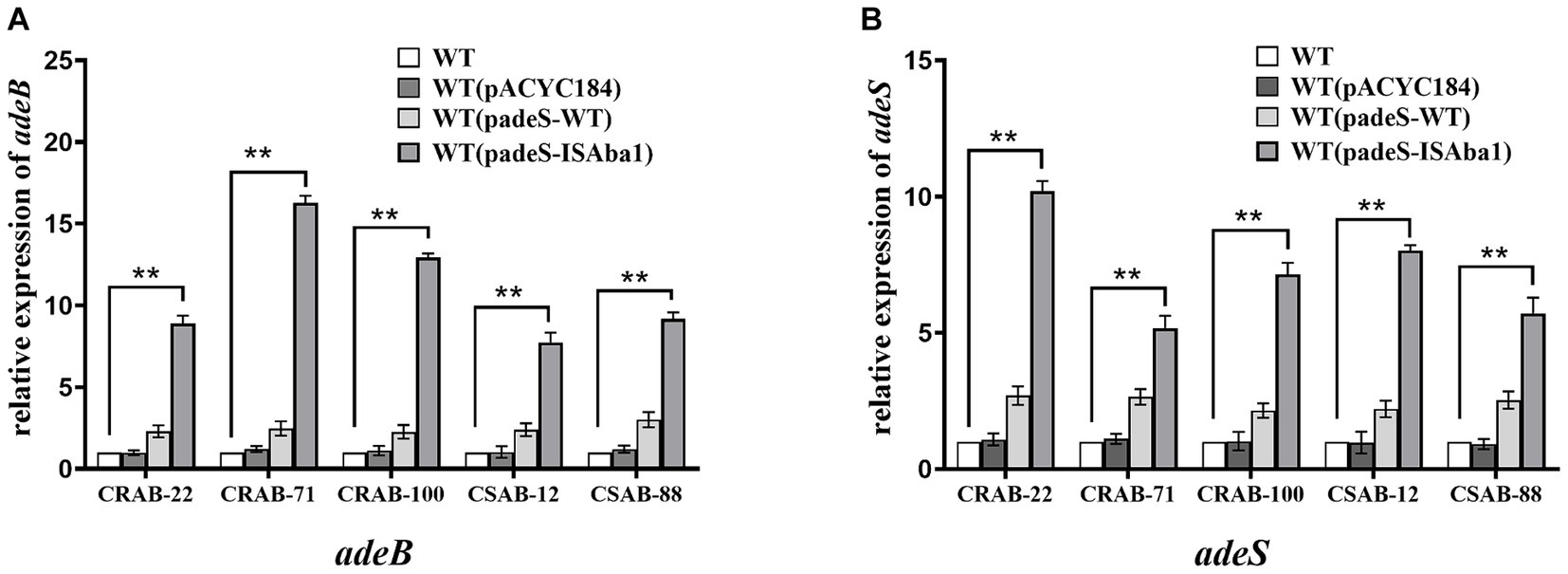
Figure 2. Expression level of adeB and adeS in strains transformed with padeS-ISAba1. Relative expression of adeB (A) and adeS (B) were assessed by qRT-PCR analysis. The housekeeping gene, rpoB, was used as an endogenous reference gene. The wildtype strain was used as the reference strain (expression = 1.0). All qRT-PCR experiments were carried out in triplicate. ** p < 0.05. WT: wildtype strain. WT (pACYC184): wildtype strain transformed with pACYC184. WT (padeS-WT): wildtype strain transformed with the padeS-WT. WT (padeS-ISAba1): wildtype strain transformed with the padeS-ISAba1.
To assess the role of the ISAba1 insertion in adeN on TGC resistance, the wildtype adeN was integrated into pACYC184 and transformed into five TGC-resistant strains with the ISAba1 insertion in adeN. The TGC and ERV MICs were then measured in the transformed strains. The TGC MIC of the complementary strains decreased by 4–16x after transformation with padeN-WT. Most (4/5, 80%) of the complementary strains had restored TGC sensitivity, while there was no significant change in ERV MIC for the complementary strains (Table 6). This result suggests that the expression of wildtype adeN can decrease the TGC MIC in resistant strains, and the disruption of adeN with the ISAba1 insertion confers TGC resistance. The expression of adeJ was significantly downregulated in all complementary strains transformed with padeN-WT (Supplementary Figure S2), indicating that the expression of wildtype adeN in strains with the ISAba1 insertion in adeN can repress adeIJK transcription.
4 Discussion
A. baumannii is a common pathogen associated with nosocomial infections that have posed a serious threat to public health for decades. CRAB is resistant to almost all commonly used antibiotics, leaving few available treatment options. TGC is one of the primary drugs used alone or in combination to treat CRAB infections, however, TGC resistance has gradually increased in recent years (Jo and Ko, 2021). ERV, a new-generation tetracycline, is shown to confer excellent antimicrobial activity against CRAB. Previous studies have compared the in vitro activity of ERV and TGC (Liu et al., 2022), while the different resistance mechanisms of these drugs against A. baumannii have not been explained. The current study sought to compare the characteristics and mechanisms of ERV and TGC resistance in A. baumannii to inform the clinical use of ERV in the treatment of CRAB infections.
CRAB exhibited an alarmingly high resistance rate to traditional tetracyclines, including doxycycline and minocycline (Xiong et al., 2023). ERV and TGC have been proven to overcome the main resistance mechanisms of tetracyclines, such as tetracycline-specific efflux Tet(A) and tetracycline resistance ribosomal protection protein Tet(M), which effectively elevated their antimicrobial activity to CRAB (Grossman et al., 2012; Yaghoubi et al., 2022). In this study, both ERV and TGC exhibited good antibacterial activity against CRAB, with MIC90 values of 1 mg/L and 2 mg/L, respectively, which was consistent with previous studies (Scheetz et al., 2007; Cruz-López et al., 2022). The ERV MIC50 and MIC90 of CRAB and CSAB isolates were 2x lower than those of TGC. Significantly fewer strains were resistant to ERV alone (n = 2) than to TGC alone (n = 15). Moreover, among strains that were resistant to both drugs, the ERV MIC was lower than the TGC MIC, indicating that ERV is a more effective drug against A. baumannii. Besides, high-dose regimens are required when TGC is used as monotherapy due to its low bioavailability (Cai et al., 2016). ERV had two unique changes compared to TGC structure, which greatly improved its bioavailability and antibacterial activity (Alosaimy et al., 2022). The MIC50 and MIC90 for ERV were higher in the CRAB than in the CSAB isolates, which was similar to the findings of Livermore et al. on carbapenem-resistant Klebsiella pneumoniae (Livermore et al., 2016). These data together suggest that the sensitivity of ERV is lower in carbapenem-resistant than in carbapenem-sensitive strains.
CRAB strains resistant to TGC and other tetracyclines primarily belong to the ST208 lineage in China (Deng et al., 2014; Jiang et al., 2019). In the current study, 77.8% (7/9) of the ERV-resistant strains were ST208 sequence types, suggesting that ST208 was also the major prevalent clone in ERV-resistant strain. Importantly, ERV- and/or TGC-resistant A. baumannii strains were primarily found to infect individuals >60 years of age and originate from the ICU, where patients are often immunocompromised. Strains resistant to both ERV and TGC may pose a serious clinical threat. While polymyxin B still exhibits antibacterial activity against ERV- and/or TGC-resistant CRAB isolates, this antibiotic is associated with severe nephrotoxicity and is not suitable for elderly patients with renal dysfunction, further limiting treatment options. Thus, there is a critical need to enhance the prevention and control of ERV and TGC-resistant strains in clinical practice. ERV- and/or TGC-resistant CSAB strains had high rates of resistance to minocycline and doxycycline, indicating the presence of cross-resistance among clinical A. baumannii strains. These data highlight the importance of regular monitoring and detection to prevent bacterial cross-resistance and treatment failure.
Overexpression of RND efflux pumps and mutations in their regulatory genes are the main mechanisms of A. baumannii resistance to TGC (Salehi et al., 2021). To determine the role of the RND efflux pump and its regulators in ERV- and/or TGC-resistant strains, the effect of EPIs on the ERV and TGC MICs was assessed. ERV and TGC MICs were significantly reduced in resistant strains following the addition of EPIs, suggesting that expression of the efflux pump may be associated with ERV and TGC resistance. Meanwhile, significant differences in genetic mutations and resistance gene expressions were identified among the three groups of resistant strains. The ISAba1 insertion in adeS was commonly found in the only ERV-resistant group and the both ERV- and TGC-resistant group, while the ISAba1insertion in adeN was common in the only TGC-resistant group. The expressions of adeABC and adeRS were significantly increased in the only ERV-resistant group and in the both ERV- and TGC-resistant group, while adeABC and adeIJK expressions were significantly increased and adeN expression was significantly decreased in the only TGC-resistant group. Sun et al. found that an ISAba1 insertion in the adeS gene enhanced expression of the N-terminal truncated AdeS protein and further activated AdeR, leading to overexpression of adeABC and A. baumannii resistance to TGC (Sun et al., 2012). Lucaßen et al. found that the ISAba1 insertion could disrupt the function of AdeN, resulting in overexpression of adeIJK and contributing to TGC resistance in A. baumannii (Lucaßen et al., 2021a). Differences in gene mutations and expressions in the three groups of resistant strains identified in the current study indicate that the resistance mechanisms of ERV and TGC in A. baumannii may be various. Other resistance mechanisms apart from RND-type efflux pumps on ERV and TGC resistance have been reported previously. Wang et al. found that plasmid-mediated tet(X5) gene confers resistance to TGC and ERV in a clinical A. baumannii isolate (Wang et al., 2019). Hua et al. demonstrated that mutations in the genes of adeS, rpoB and rrf are associated with TGC resistance in A. baumannii (Hua et al., 2021). Other mutations in rpsJ, tet(A), tetR, and soxR were also reported to be associated with TGC resistance (Beabout et al., 2015; Linkevicius et al., 2016; Li et al., 2017; Zhang et al., 2021). However, few of the aforementioned resistance mechanisms were identified in the resistant strains of our study.
Functional validation experiments were performed to confirm the roles of ISAba1 insertion in adeS on ERV resistance and ISAba1 insertion in adeN on TGC resistance. Strains transformed with padeS-ISAba1 significantly increased the MICs of ERV and TGC and upregulated the expressions of AdeABC and AdeRS. These results suggest that the ISAba1 insertion in adeS promotes high AdeABC expression and contributes to both ERV and TGC resistance, similar to the TGC resistance mechanism reported by Sun et al. (2012). Previous studies have shown that ISAba1 insertion in adeS was rarely found in TGC resistance (Lucaßen et al., 2021a), but it is the primary mechanism conferring resistance to ERV in current study. Moreover, the TGC MICs of all adeN mutant strains complemented with padeN-WT were reduced to sensitive or intermediate levels, and the expression of adeJ in all complementary strains was significantly downregulated. This finding suggests that the expression of wildtype adeN can restore the TGC sensitivity of resistant strains by repressing adeIJK and the disruption of adeN by the ISAba1 insertion can increase AdeIJK expression and lead to TGC resistance, a result consistent with those of Rosenfeld et al. (2012). Notably, TGC resistant strains with ISAba1 insertion in adeN remained sensitive to ERV in our study and the ERV MIC did not show significant change in complementary strains transformed with padeN-WT. This suggests that ERV susceptibility is not affected by the ISAba1 insertion in adeN.
In conclusion, ERV exhibited excellent in vitro activity against both CRAB and CSAB and surpassed the efficacy of TGC. A major sequence type, ST208, was found in ERV- and/or TGC-resistant strains. Moreover, the ISAba1 insertion in adeS was shown to play a critical role in ERV resistance in A. baumannii, while the TGC resistance was mainly associated with the ISAba1 insertion in adeN.
Data availability statement
The original contributions presented in the study are included in the article/Supplementary material, further inquiries can be directed to the corresponding authors.
Ethics statement
All procedures performed were approved by the Ethical Committee of Yangjiang People’s Hospital and were in accordance with the 1964 Helsinki Declaration and its later amendments.
Author contributions
XC: Investigation, Methodology, Writing – original draft, Writing – review & editing. YLi: Methodology, Validation, Writing – review & editing. YLin: Methodology, Writing – review & editing. YG: Formal analysis, Writing – review & editing. GH: Methodology, Resources, Writing – review & editing. XW: Software, Writing – review & editing. MW: Investigation, Writing – review & editing. JX: Investigation, Writing – review & editing. MS: Methodology, Writing – review & editing. XT: Supervision, Writing – review & editing. CZ: Supervision, Writing – review & editing. ZL: Funding acquisition, Supervision, Writing – review & editing.
Funding
The author(s) declare that financial support was received for the research, authorship, and/or publication of this article. This work was supported by the Guangdong Basic and Applied Basic Research Foundation [grant numbers 2022A1515010643 and 2021A1515220090], Yangjiang Health Technology Project [grant number SF2023021], the Scientific Research Fund of People’s Hospital of Yangjiang [grant numbers G2020005, G2021003, and 2021001].
Conflict of interest
The authors declare that the research was conducted in the absence of any commercial or financial relationships that could be construed as a potential conflict of interest.
Publisher’s note
All claims expressed in this article are solely those of the authors and do not necessarily represent those of their affiliated organizations, or those of the publisher, the editors and the reviewers. Any product that may be evaluated in this article, or claim that may be made by its manufacturer, is not guaranteed or endorsed by the publisher.
Supplementary material
The Supplementary material for this article can be found online at: https://www.frontiersin.org/articles/10.3389/fmicb.2024.1417237/full#supplementary-material
Abbreviations
CCCP, carbonyl cyanide m-chlorophenylhydrazone; CLSI, clinical and laboratory standardization institute; CRAB, carbapenem-resistant Acinetobacter baumannii; CSAB, carbapenem-sensitive Acinetobacter baumannii; EPI, efflux pump inhibitor; ERV, eravacycline; FDA, food and drug administration; ICU, intensive care unit; LB, Luria-Bertani; MH, Mueller-Hinton; MIC, minimum inhibitory concentrations; MLST, multilocus sequence typing; PCR, polymerase chain reaction; PaβN, phe-arg-β-naphthylamide; qRT-PCR, quantitative real-time PCR; TGC, tigecycline.
References
Abdallah, M., Olafisoye, O., Cortes, C., Urban, C., Landman, D., and Quale, J. (2015). Activity of eravacycline against Enterobacteriaceae and Acinetobacter baumannii, including multidrug-resistant isolates, from new York City. Antimicrob. Agents Chemother. 59, 1802–1805. doi: 10.1128/aac.04809-14
Alosaimy, S., Abdul-Mutakabbir, J. C., Kebriaei, R., Jorgensen, S. C. J., and Rybak, M. J. (2020). Evaluation of Eravacycline: a novel Fluorocycline. Pharmacotherapy 40, 221–238. doi: 10.1002/phar.2366
Alosaimy, S., Morrisette, T., Lagnf, A. M., Rojas, L. M., King, M. A., Pullinger, B. M., et al. (2022). Clinical outcomes of Eravacycline in patients treated predominately for Carbapenem-resistant Acinetobacter baumannii. Microbiol. Spectr. 10:e0047922. doi: 10.1128/spectrum.00479-22
Beabout, K., Hammerstrom, T. G., Perez, A. M., Magalhães, B. F., Prater, A. G., Clements, T. P., et al. (2015). The ribosomal S10 protein is a general target for decreased tigecycline susceptibility. Antimicrob. Agents Chemother. 59, 5561–5566. doi: 10.1128/aac.00547-15
Cai, Y., Bai, N., Liu, X., Liang, B., Wang, J., and Wang, R. (2016). Tigecycline: alone or in combination? Infect. Dis. 48, 491–502. doi: 10.3109/23744235.2016.1155735
Chen, C. L., Janapatla, R. P., Dudek, A., Chen, Y. R., Lee, H. Y., and Chiu, C. H. (2023). Functional characterization and clinical implication of a novel epidemic carbapenem-resistant Acinetobacter baumannii genetic marker. Int. J. Antimicrob. Agents 62:106879. doi: 10.1016/j.ijantimicag.2023.106879
Clinical and Laboratory Standards Institute (2024). Performance standard for antimicrobial susceptibility testing: Thirty-one informational supplement M100-S34. Wayne: CLSI.
Cruz-López, F., Martínez-Meléndez, A., Morfin-Otero, R., Rodriguez-Noriega, E., Maldonado-Garza, H. J., and Garza-González, E. (2022). Efficacy and in vitro activity of novel antibiotics for infections with Carbapenem-resistant gram-negative pathogens. Front. Cell. Infect. Microbiol. 12:884365. doi: 10.3389/fcimb.2022.884365
Deng, M., Zhu, M. H., Li, J. J., Bi, S., Sheng, Z. K., Hu, F. S., et al. (2014). Molecular epidemiology and mechanisms of tigecycline resistance in clinical isolates of Acinetobacter baumannii from a Chinese university hospital. Antimicrob. Agents Chemother. 58, 297–303. doi: 10.1128/aac.01727-13
Deveci, A., Coban, A. Y., Acicbe, O., Tanyel, E., Yaman, G., and Durupinar, B. (2012). In vitro effects of sulbactam combinations with different antibiotic groups against clinical Acinetobacter baumannii isolates. J. Chemother. 24, 247–252. doi: 10.1179/1973947812y.0000000029
Gerson, S., Nowak, J., Zander, E., Ertel, J., Wen, Y., Krut, O., et al. (2018). Diversity of mutations in regulatory genes of resistance-nodulation-cell division efflux pumps in association with tigecycline resistance in Acinetobacter baumannii. J. Antimicrob. Chemother. 73, 1501–1508. doi: 10.1093/jac/dky083
Grossman, T. H., Starosta, A. L., Fyfe, C., O'Brien, W., Rothstein, D. M., Mikolajka, A., et al. (2012). Target- and resistance-based mechanistic studies with TP-434, a novel fluorocycline antibiotic. Antimicrob. Agents Chemother. 56, 2559–2564. doi: 10.1128/aac.06187-11
Guo, Y., Liu, N., Lin, Z., Ba, X., Zhuo, C., Li, F., et al. (2021). Mutations in porin LamB contribute to ceftazidime-avibactam resistance in KPC-producing Klebsiella pneumoniae. Emerg. Microb. Infect. 10, 2042–2051. doi: 10.1080/22221751.2021.1984182
Hornsey, M., Ellington, M. J., Doumith, M., Thomas, C. P., Gordon, N. C., Wareham, D. W., et al. (2010). AdeABC-mediated efflux and tigecycline MICs for epidemic clones of Acinetobacter baumannii. J. Antimicrob. Chemother. 65, 1589–1593. doi: 10.1093/jac/dkq218
Hua, X., He, J., Wang, J., Zhang, L., Zhang, L., Xu, Q., et al. (2021). Novel tigecycline resistance mechanisms in Acinetobacter baumannii mediated by mutations in adeS, rpoB and rrf. Emerg. Microb. Infect. 10, 1404–1417. doi: 10.1080/22221751.2021.1948804
Isler, B., Doi, Y., Bonomo, R. A., and Paterson, D. L. (2019). New treatment options against Carbapenem-resistant Acinetobacter baumannii infections. Antimicrob. Agents Chemother. 63:1128. doi: 10.1128/AAC.01110-18
Jiang, L., Liang, Y., Yao, W., Ai, J., Wang, X., and Zhao, Z. (2019). Molecular epidemiology and genetic characterisation of carbapenem-resistant Acinetobacter baumannii isolates from Guangdong Province, South China. J. Glob. Antimicrob. Resist. 17, 84–89. doi: 10.1016/j.jgar.2018.11.002
Jo, J., and Ko, K. S. (2021). Tigecycline Heteroresistance and resistance mechanism in clinical isolates of Acinetobacter baumannii. Microbiol. Spectr. 9:e0101021. doi: 10.1128/Spectrum.01010-21
LaPlante, K., Cusumano, J., and Tillotson, G. (2018). Colistin for the treatment of multidrug-resistant infections. Lancet Infect. Dis. 18, 1174–1175. doi: 10.1016/S1473-3099(18)30611-X
Li, Y.-T., Chen, X.-D., Guo, Y.-Y., Lin, S.-W., Wang, M.-Z., Xu, J.-B., et al. (2024). Emergence of eravacycline heteroresistance in carbapenem-resistant Acinetobacter baumannii isolates in China. Front. Cell. Infect. Microbiol. 14:1356353. doi: 10.3389/fcimb.2024.1356353
Li, Y., Cui, L., Xue, F., Wang, Q., and Zheng, B. (2022). Synergism of eravacycline combined with other antimicrobial agents against carbapenem-resistant Enterobacteriaceae and Acinetobacter baumannii. J. Glob. Antimicrob. Resist. 30, 56–59. doi: 10.1016/j.jgar.2022.05.020
Li, H., Wang, Q., Wang, R., Zhang, Y., Wang, X., and Wang, H. (2017). Global regulator SoxR is a negative regulator of efflux pump gene expression and affects antibiotic resistance and fitness in Acinetobacter baumannii. Medicine 96:e7188. doi: 10.1097/md.0000000000007188
Li, T., Yang, Y., Yan, R., Lan, P., Liu, H., Fu, Y., et al. (2022). Comparing core-genome MLST with PFGE and MLST for cluster analysis of carbapenem-resistant Acinetobacter baumannii. J. Glob. Antimicrob. Resist. 30, 148–151. doi: 10.1016/j.jgar.2022.06.014
Linkevicius, M., Sandegren, L., and Andersson, D. I. (2016). Potential of tetracycline resistance proteins to evolve Tigecycline resistance. Antimicrob. Agents Chemother. 60, 789–796. doi: 10.1128/aac.02465-15
Liu, P. Y., Ko, W. C., Lee, W. S., Lu, P. L., Chen, Y. H., Cheng, S. H., et al. (2022). In vitro activity of cefiderocol, cefepime/enmetazobactam, cefepime/zidebactam, eravacycline, omadacycline, and other comparative agents against carbapenem-non-susceptible Pseudomonas aeruginosa and Acinetobacter baumannii isolates associated from bloodstream infection in Taiwan between 2018-2020. J. Microbiol. Immunol. Infect. 55, 888–895. doi: 10.1016/j.jmii.2021.08.012
Liu, H., Moran, R. A., Doughty, E. L., Hua, X., Snaith, A. E., Zhang, L., et al. (2024). Changes to an intensive care unit Acinetobacter baumannii population following COVID-19 disruptions and targeted infection prevention interventions. medRxiv. 24302174. doi: 10.1101/2024.02.02.24302174
Livermore, D. M., Mushtaq, S., Warner, M., and Woodford, N. (2016). In vitro activity of Eravacycline against Carbapenem-resistant Enterobacteriaceae and Acinetobacter baumannii. Antimicrob. Agents Chemother. 60, 3840–3844. doi: 10.1128/aac.00436-16
Lucaßen, K., Müller, C., Wille, J., Xanthopoulou, K., Hackel, M., Seifert, H., et al. (2021a). Prevalence of RND efflux pump regulator variants associated with tigecycline resistance in carbapenem-resistant Acinetobacter baumannii from a worldwide survey. J. Antimicrob. Chemother. 76, 1724–1730. doi: 10.1093/jac/dkab079
Lucaßen, K., Xanthopoulou, K., Wille, J., Wille, T., Wen, Y., Hua, X., et al. (2021b). Characterization of amino acid substitutions in the two-component regulatory system AdeRS identified in multidrug-resistant Acinetobacter baumannii. mSphere 6:e0070921. doi: 10.1128/msphere.00709-21
Marchaim, D., Pogue, J. M., Tzuman, O., Hayakawa, K., Lephart, P. R., Salimnia, H., et al. (2014). Major variation in MICs of tigecycline in gram-negative bacilli as a function of testing method. J. Clin. Microbiol. 52, 1617–1621. doi: 10.1128/jcm.00001-14
Monogue, M. L., Thabit, A. K., Hamada, Y., and Nicolau, D. P. (2016). Antibacterial efficacy of Eravacycline in vivo against gram-positive and gram-negative organisms. Antimicrob. Agents Chemother. 60, 5001–5005. doi: 10.1128/aac.00366-16
O'Donnell, J. N., Putra, V., and Lodise, T. P. (2021). Treatment of patients with serious infections due to carbapenem-resistant Acinetobacter baumannii: how viable are the current options? Pharmacotherapy 41, 762–780. doi: 10.1002/phar.2607
Poulakou, G., Lagou, S., Karageorgopoulos, D. E., and Dimopoulos, G. (2018). New treatments of multidrug-resistant gram-negative ventilator-associated pneumonia. Ann. Transl. Med. 6:423. doi: 10.21037/atm.2018.10.29
Ramirez, M. S., Bonomo, R. A., and Tolmasky, M. E. (2020). Carbapenemases: transforming Acinetobacter baumannii into a yet more dangerous menace. Biomol. Ther. 10:720. doi: 10.3390/biom10050720
Rosenfeld, N., Bouchier, C., Courvalin, P., and Périchon, B. (2012). Expression of the resistance-nodulation-cell division pump AdeIJK in Acinetobacter baumannii is regulated by AdeN, a TetR-type regulator. Antimicrob. Agents Chemother. 56, 2504–2510. doi: 10.1128/aac.06422-11
Salehi, B., Ghalavand, Z., Yadegar, A., and Eslami, G. (2021). Characteristics and diversity of mutations in regulatory genes of resistance-nodulation-cell division efflux pumps in association with drug-resistant clinical isolates of Acinetobacter baumannii. Antimicrob. Resist. Infect. Control 10:53. doi: 10.1186/s13756-021-00924-9
Scheetz, M. H., Qi, C., Warren, J. R., Postelnick, M. J., Zembower, T., Obias, A., et al. (2007). In vitro activities of various antimicrobials alone and in combination with tigecycline against carbapenem-intermediate or-resistant Acinetobacter baumannii. Antimicrob. Agents Chemother. 51, 1621–1626. doi: 10.1128/aac.01099-06
Serapide, F., Guastalegname, M., Gullì, S. P., Lionello, R., Bruni, A., Garofalo, E., et al. (2024). Antibiotic treatment of Carbapenem-resistant Acinetobacter baumannii infections in view of the newly developed β-lactams: a narrative review of the existing evidence. Antibiotics 13:506. doi: 10.3390/antibiotics13060506
Shi, Y., Hua, X., Xu, Q., Yang, Y., Zhang, L., He, J., et al. (2020). Mechanism of eravacycline resistance in Acinetobacter baumannii mediated by a deletion mutation in the sensor kinase adeS, leading to elevated expression of the efflux pump AdeABC. Infect. Genet. Evol. 80:104185. doi: 10.1016/j.meegid.2020.104185
Sun, J. R., Perng, C. L., Chan, M. C., Morita, Y., Lin, J. C., Su, C. M., et al. (2012). A truncated AdeS kinase protein generated by ISAba1 insertion correlates with tigecycline resistance in Acinetobacter baumannii. PLoS One 7:e49534. doi: 10.1371/journal.pone.0049534
Sun, C., Yu, Y., and Hua, X. (2023). Resistance mechanisms of tigecycline in Acinetobacter baumannii. Front. Cell. Infect. Microbiol. 13:1141490. doi: 10.3389/fcimb.2023.1141490
Sutcliffe, J. A., O'Brien, W., Fyfe, C., and Grossman, T. H. (2013). Antibacterial activity of eravacycline (TP-434), a novel fluorocycline, against hospital and community pathogens. Antimicrob. Agents Chemother. 57, 5548–5558. doi: 10.1128/aac.01288-13
Tambat, R., Mahey, N., Chandal, N., Verma, D. K., Jangra, M., Thakur, K. G., et al. (2022). A microbe-derived efflux Pump inhibitor of the resistance-nodulation-cell division protein restores antibiotic susceptibility in Escherichia Coli and Pseudomonas aeruginosa. ACS Infect. Dis. 8, 255–270. doi: 10.1021/acsinfecdis.1c00281
Ten, K. E., Md Zoqratt, M. Z. H., Ayub, Q., and Tan, H. S. (2021). Characterization of multidrug-resistant Acinetobacter baumannii strain ATCC BAA1605 using whole-genome sequencing. BMC. Res. Notes 14:83. doi: 10.1186/s13104-021-05493-z
Wang, L., Liu, D., Lv, Y., Cui, L., Li, Y., Li, T., et al. (2019). Novel plasmid-mediated tet(X5) gene conferring resistance to Tigecycline, Eravacycline, and Omadacycline in a clinical Acinetobacter baumannii isolate. Antimicrob. Agents Chemother. 64:1326. doi: 10.1128/aac.01326-19
Xiong, L., Deng, C., Yang, G., Shen, M., Chen, B., Tian, R., et al. (2023). Molecular epidemiology and antimicrobial resistance patterns of carbapenem-resistant Acinetobacter baumannii isolates from patients admitted at ICUs of a teaching hospital in Zunyi, China. Front. Cell. Infect. Microbiol. 13:1280372. doi: 10.3389/fcimb.2023.1280372
Yaghoubi, S., Zekiy, A. O., Krutova, M., Gholami, M., Kouhsari, E., Sholeh, M., et al. (2022). Tigecycline antibacterial activity, clinical effectiveness, and mechanisms and epidemiology of resistance: narrative review. Eur. J. Clin. Microbiol. Infect. Dis. 41, 1003–1022. doi: 10.1007/s10096-020-04121-1
Yang, Y. S., Chen, H. Y., Hsu, W. J., Chou, Y. C., Perng, C. L., Shang, H. S., et al. (2019). Overexpression of AdeABC efflux pump associated with tigecycline resistance in clinical Acinetobacter nosocomialis isolates. Clin. Microbiol. Infect. 25, 512.e1–512.e6. doi: 10.1016/j.cmi.2018.06.012
Zhanel, G. G., Cheung, D., Adam, H., Zelenitsky, S., Golden, A., Schweizer, F., et al. (2016). Review of Eravacycline, a novel Fluorocycline antibacterial agent. Drugs 76, 567–588. doi: 10.1007/s40265-016-0545-8
Zhang, Y., Wang, X., Wang, Q., Chen, H., Li, H., Wang, S., et al. (2021). Emergence of Tigecycline nonsusceptible and IMP-4 Carbapenemase-producing K2-ST65 Hypervirulent Klebsiella pneumoniae in China. Microbiol. Spectr. 9:e0130521. doi: 10.1128/Spectrum.01305-21
Keywords: Acinetobacter baumannii , eravacycline, tigecycline, antimicrobial activity, resistance mechanism, ISAba1 insertion
Citation: Chen X, Li Y, Lin Y, Guo Y, He G, Wang X, Wang M, Xu J, Song M, Tan X, Zhuo C and Lin Z (2024) Comparison of antimicrobial activities and resistance mechanisms of eravacycline and tigecycline against clinical Acinetobacter baumannii isolates in China. Front. Microbiol. 15:1417237. doi: 10.3389/fmicb.2024.1417237
Edited by:
Sulagna Basu, National Institute of Cholera and Enteric Diseases (ICMR), IndiaReviewed by:
Saranya Vijayakumar, Kansas State University, United StatesSubhasree Roy, National Institute of Cholera and Enteric Diseases (ICMR), India
Copyright © 2024 Chen, Li, Lin, Guo, He, Wang, Wang, Xu, Song, Tan, Zhuo and Lin. This is an open-access article distributed under the terms of the Creative Commons Attribution License (CC BY). The use, distribution or reproduction in other forums is permitted, provided the original author(s) and the copyright owner(s) are credited and that the original publication in this journal is cited, in accordance with accepted academic practice. No use, distribution or reproduction is permitted which does not comply with these terms.
*Correspondence: Zhiwei Lin, NDIyMTU2MzIxQHFxLmNvbQ==; Chao Zhuo, Y2hhb19zaGVlcEAyNjMubmV0; Xixi Tan, NDY4MjEyMjU1QHFxLmNvbQ==
†These authors have contributed equally to this work