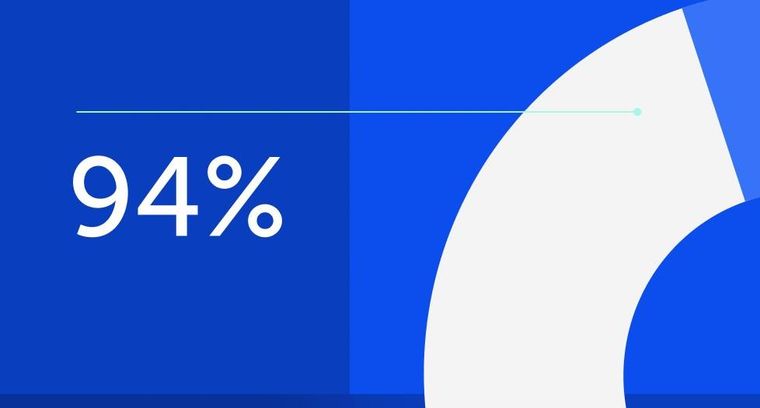
94% of researchers rate our articles as excellent or good
Learn more about the work of our research integrity team to safeguard the quality of each article we publish.
Find out more
ORIGINAL RESEARCH article
Front. Microbiol., 02 May 2024
Sec. Food Microbiology
Volume 15 - 2024 | https://doi.org/10.3389/fmicb.2024.1408013
In order to control the occurrence of ginseng root rot caused by Fusarium solani (Mart.) Sacc., the antifungal compounds of the mushroom Suillus bovinus were investigated. And three new alkaloids (1–3), named bovinalkaloid A–C, along with one known analog (4), were isolated and identified by bioassay-guided isolation and spectroscopic analyses. Compound 1 strongly inhibited the mycelial growth and spore germination of F. solani with minimum inhibitory concentration of 2.08 mM. Increases in electrical conductivity, nucleic acid, and protein contents, and decreases in lipid content showed that the membrane permeability and integrity were damaged by compound 1. Compound 1 also increased the contents of malondialdehyde and hydrogen peroxide and the activities of antioxidant enzymes, indicating that lipid peroxidation had taken place in F. solani. Compound 1 may serve as a natural alternative to synthetic fungicides for the control of ginseng root rot.
Panax ginseng C. A. Mey is a deciduous perennial herb with important medicinal and economic values (Xu et al., 2017). It is mainly distributed in the northeastern regions of China and Korea and the eastern region of Siberia (Yu et al., 2018). For thousands of years, ginseng has been widely cultivated for its medicinal value (Xiao et al., 2016). Ginseng root rot was caused by Fusarium spp., Fusarium solani was one of the most important pathogens that can reduce the yield and quality of ginseng, thereby seriously constraining the economic development of ginseng (Li et al., 2020). Currently, the disease is controlled by applying synthetic fungicides and chemical products. However, the indiscriminate use of chemical fungicides causes serious health issues, environmental pollution, and development of resistance. Therefore, it is imperative to develop new safe and effective fungicides based on natural metabolites.
Mushrooms are notable for their large and visible fruiting bodies. They are consumed for their nutritional and medicinal values (Wang et al., 2013). Besides their high contents of proteins, dietary fiber, vitamins, and minerals, and low lipid content, mushrooms also contain a variety of secondary metabolites, such as fatty acids, triterpenoids, alkaloids, sterols, nucleosides, and phenolic compounds (Wang et al., 2017). To date, several compounds isolated from mushrooms have been demonstrated to exhibit effective antifungal activity. For example, a novel peptide isolated from the fruiting bodies of the mushroom Pleurotus eryngii can inhibit mycelial growth of Mycosphaerella arachidicola and Fusarium oxysporum (Wang and Ng, 2004). A furanone derivative isolated from the edible mushroom Grifola frondosa have a broad spectrum of antifungal activity against the plant pathogens (He et al., 2016). A nerolidol mannoside isolated from the Schizophyllum commune displayed antifungal activities against the plant pathogenic fungi Botrytis cinerea, Rhizoctonia, and Alternaria solani (Woo et al., 2019).
Suillus bovinus, an edible mushroom belonging to the Suillus genus, is broadly distributed across various regions globally (Feng et al., 2022). In recent years, research about S. bovinus focus mainly on the mechanisms related to symbiotic interactions with trees (Sun et al., 2023). Additionally, the pharmacological studies indicated that S. bovinus possessed the effect of inhibiting CYP enzymes (Huang et al., 2009). However, there are no reports concerning the antifungal activity of S. bovinus. In this study, we found that the extracts of S. bovinus exhibited good antifungal activity against F. solani. Subsequently, using a bioassay-guided separation procedure to explore the antifungal compounds from S. bovinus and to elucidate the possible antifungal mechanism. This will provide an alternative method of control for ginseng root rot and provide a theoretical foundation for the disease management of ginseng.
NMR spectra were obtained using a Bruker DRX-600 spectrometer. HR-ESI-MS were recorded on an AB SCIE x500 Q-TOF MS spectrometer (AB Sciex Pte. Ltd., MA, United States). High-performance liquid chromatography (HPLC) was carried out using a Waters2535 chromatography system (Waters, Milford, MA, United States) equipped with a Waters 2,489 UV/visible detector with a YMC-Pack ODS-A (250 × 10 mm, 5 μm) column (YMC Co., Ltd., Kyoto, Japan). Acetonitrile and water (HPLC grade) were purchased from Fisher Scientific Ltd.
The fresh fruiting bodies of S. bovinus were collected from Chengde City, Hebei Province, China, in September 2022 and identified based on morphological characteristic and molecular analysis. The pathogenic fungus F. solani was provided by Professor Jie Gao (College of Plant Protection, Jilin Agricultural University, Changchun, China).
The fruiting bodies of S. bovinus were subjected to air-drying for 48 h followed by extraction using 95% ethanol (60 L, three times) for 24 h to obtain the crude extract weighing 2,011 g. The crude extract was suspended in 3 L of water and then sequentially partitioned with petroleum ether (3 × 3 L, 2 h each), ethyl acetate (3× 3 L, 2 h each), and n-butanol (3 × 3 L, 2 h each), to acquire a petroleum ether extract (PE, 422.2 g), an ethyl acetate extract (EA, 299.5 g), an n-butanol extract (BT, 554.3 g) and a remainder water extract (WT, 681.8 g). Antifungal assay revealed that EA showed higher activity and was selected for further isolation. Then, the EA was separated by silica gel column chromatography (200–300 mesh), eluted with a CH2Cl2/MeOH gradient (50:1–0:1), providing 10 fractions (F1–F10). The F6 which showed the best antifungal activity was further separated by Sephadex LH-20 (methanol) to obtain eight subfractions (F6.1–F6.8). The F6.4 and F6.6 exhibited much better antifungal activity than other subfractions. The F6.4 was further purified by semi-preparative RP-HPLC (YMC ODS-A column, 250 × 10 mm, 5 μm, 2.5 mL/min, acetonitrile/water, 20:80) to obtain compounds 1 (32.5 mg, tR = 20.5 min), 2 (27.2 mg, tR = 22.5 min), and 4 (19.6 mg, tR = 26.1 min). The F6.6 was further purified by semi-preparative RP-HPLC (2.5 mL/min, acetonitrile/water, 25:75) to obtain compound 3 (21.2 mg, tR = 23.5 min).
The mycelial growth inhibition assay was performed as previously described (Palacios et al., 2014; Bhutia et al., 2016), with minor modification. The samples (extracts and compounds) were diluted with pure water. Under aseptic conditions, the solution was filtered through a 0.22 μm organic filter to obtain the sterile solution. The solution was then added to sterilized PDA medium. Afterward, a seven-day-old mycelial disk (6 mm) was obtained from the periphery of a culture of F. solani and was placed in the center of the PDA culture dish. Media without samples were used as controls, and each treatment was repeated three times and incubated in the dark at 25°C. Colony diameters were measured by the cross method using a Vernier caliper after 8 days of incubation. The mycelial growth inhibition rate was calculated according to the following formula:
Where Dc and Dt are the colony diameter of the control group and treated group, respectively; Di is the initial colony diameter.
The minimal inhibitory concentration (MIC) was defined as the lowest concentration that inhibited visible growth of F. solani after 2 days of incubation in the dark at 25°C (Zhang et al., 2020).
The spore germination inhibition assay was performed as previously described (Chowdhury and Bae, 2018; Elsherbiny et al., 2021), with minor modification. Briefly, the tested F. solani strain was cultured in PDA plate medium at 25°C for 10 days. The plates were washed with 5 mL sterile water to acquire a spore suspension (1 × 106 spores/mL). Spore suspensions (10 μL, conidia) with different concentrations of compound 1 were incubated at 25°C for 24 h. The control group was given an equal amount of sterile water. Approximately 100 spores were observed with an inverted microscope (40 × magnification). Each treatment was repeated three times. Spores were germinated when the length of the germ tube was longer than the diameter of the spore. The spore germination inhibition rate was calculated according to the following formula:
Where Gc and Gt are the number of germinated spores of the control group and treated group, respectively.
Four cover glasses were inserted on a slant into the PDA medium. Simultaneously, a seven-day-old mycelial disk (6 mm) was obtained from the periphery of the culture of F. solani and was placed in the center of the PDA culture dish. When the mycelium grew to two-thirds of the area of the cover glass, cover glasses were removed, and slides were immersed in PDA medium containing compound 1 (MIC) for 24 h at 25°C. Media without compound 1 were used as controls (Chen et al., 2020). Mycelial morphology was observed under an optical microscope (Olympus CKX53, Tokyo, Japan).
Electrolyte leakage, nucleic acid content, and protein content were determined as previously described (Chen et al., 2020; Souza et al., 2020), with slight modification. Compound 1 was added to the fungal suspension at a final concentration of MIC and incubated for 0, 2, 4, 6, 8, 10, 15, and 20 h. The control group was prepared without compound 1. Subsequently, the suspension was centrifuged at 12,000 rpm for 3 min, and the supernatant was collected. The electrical conductivity was evaluated using a conductivity meter (SevenMulti, Shanghai Mettler Toledo Co., Ltd., Shanghai, China). Nucleic acid and protein contents were measured at wavelengths of 260 and 280 nm, respectively, using a Shimadzu UV-2600 spectrophotometer (Shimadzu UV-2600, Tokyo, Japan).
Total lipid content was determined as previously described (Helal et al., 2007), with minor modification. Compound 1 was added to the fungal suspension at a final concentration of MIC and incubated for 0, 2, 4, 6, 8, 10, 15, and 20 h Subsequently, the mycelia (1 g, dry weight) were extracted using a methanol-chloroform-water (2:1:1, v/v) mixture. Saline solution (0.9% NaCl, 0.2 mL) was added to the lower phase containing lipids, shaken, and then centrifuged at 5000 rpm for 5 min. The lower phase containing lipids was oven dried at 65°C to constant weight. After drying, 0.5 mL 12.4 mol/L H2SO4 was added to the extracted lipids, and the mixture was heated in a boiling water bath for 5 mins and left to cool down at room temperature. Then, 3 mL phosphovanillin was added and the mixture was shaken. The mixture was allowed to stand for 10 min at room temperature, and the absorbance at 520 nm was measured to determine total lipid content from a standard curve obtained using cholesterol.
The malondialdehyde (MDA) and hydrogen peroxide (H2O2) contents were measured using commercial MDA and H2O2 assay kits (A003-1, A064-1 Jiancheng Bioengineering Institute, Nanjing, China) respectively.
The catalase (CAT) activity, peroxidase (POD) activity, and superoxide dismutase (SOD) activity were measured using commercial CAT, POD, and SOD assay kits (A007-1, A084-3, A001-1, Jiancheng Bioengineering Institute, Nanjing, China), respectively.
Data were analyzed with Origin 8.5 software (OriginLab Corporation, Hampton, MA, United States), and all data were expressed as means ± standard deviation. Means were compared by Duncan’s new multiple range test. p < 0.05 was used to identify statistical significance.
To screen for the compounds with antifungal activity against F. solani, a 95% aqueous ethyl alcohol crude extract of S. bovinus was sequentially partitioned with petroleum ether, ethyl acetate, and n-butanol to yield four solvent-soluble fractions, PE, EA, BT, and WT. Then, the antifungal activities of the four fractions were tested at concentrations of 2 mg/mL. As shown in Figure 1A, the EA (61.72%) showed higher antifungal activity than PE (14.33%), BT (23.42%), and WT (28.71%). The results indicated the EA may contain the antifungal compounds. Afterward, bioassay-guided separation of EA led to the isolation of four compounds (1–4) (Figure 2).
Figure 1. Antifungal activity of crude extracts (A), and isolated compounds (B) from Suillus bovinus. The data were expressed as means ± standard deviation of mean. a, b, c, d indicates statistically significant differences (p < 0.05).
Compound 1 possessed a molecular formula of C13H10N2O3 by the negative HR-ESI-MS (m/z 241.0619 C13H9N2O3−, calcd. 241.0619), implying 10 degrees of unsaturation. The 1H NMR spectrum (Table 1) in DMSO-d6 of 1 showed one exchangeable proton signal at δH 11.19 (1H, s, NH), three aromatic protons at δH 7.93 (1H, d, J = 8.5 Hz, H-5), 7.02 (1H, d, J = 1.9 Hz, H-8) and 6.64 (1H, dd, J = 8.5, 1.9 Hz, H-6) assignable to a 1,2,4-trisubstituted benzene ring, one isolated aromatic singlet at δH 7.46 (1H, s, H-4), as well as one methyl singlet at δH 2.68 (3H, s, H-2′). The 13C NMR spectrum (Table 1) showed one conjugated ketone carbon at δC 200.5 (s, C-1′), 11 sp2 carbon signals (4 × CH and 7 × C) in the range from 97.8—159.4 ppm, as well as one aliphatic methyl carbon at δC 25.9 (q, C-2′). The above NMR features were very similar to those of arenarine D (1-acetyl-7-hydroxy-β-carboline; Wu et al., 1989), and the signal differences were only from the pyridine ring moiety. In consideration of the molecular formula and the presence of an isolated aromatic singlet, a phenolic hydroxy group should be substituted at C-3 or C-4 of the pyridine ring. The observable HMBC correlations (Figure 3) from the aromatic singlet at δH 7.46 (1H, s) to C-4b [δC 112.2 (s)] and C-9a [δC 131.2 (s)] revealed the existence of a phenolic hydroxy group at C-3. The resulting structure was further verified by the detailed 2D NMR analysis. Therefore, the structure of 1 was established as 1-acetyl-3,7-dihydroxy-β-carboline and named as bovinalkaloid A, shown in Figure 2.
Compound 2, obtained as yellow amorphous powder, had a molecular formula of C15H10N2O2 by the negative HR-ESI-MS (m/z 249.0669 C15H9N2O2−, calcd. 249.0670), indicating 12 indices of hydrogen deficiency. The 1H NMR spectrum (Table 2) in DMSO-d6 of 2 exhibited one phenolic hydroxy signal at δH 10.51 (1H, br s), a pair of mutually coupled heterocyclic aromatic doublets at δH 8.71 (1H, d, J = 5.0 Hz, H-2) and 8.07 (1H, d, J = 5.0 Hz, H-1), three aromatic protons at δH 8.15 (1H, d, J = 8.5 Hz, H-11), 8.01 (1H, d, J = 2.1 Hz, H-8) and 6.99 (1H, dd, J = 8.5, 2.1 Hz, H-10) assignable to a 1,2,4-trisubstituted benzene ring, one isolated olefinic or aromatic signal at δH 8.02 (1H, br s, H-4), as well as one olefinic methyl singlet at δH 2.31 (3H, br s) in the up-field region. The 13C NMR spectrum (Table 2) showed a total of 15 carbon resonances, including 14 sp2 carbon signals (6 × CH and 8 × C) in the range from 103.1—160.5 ppm, as well as one aliphatic methyl carbon at δC 17.4 (q). The aforementioned NMR features and its characteristic UV curve suggested that 2 should be a canthin-6-one alkaloid, structurally similar to 9-hydroxycanthin-6-one (Leonardus et al., 1991). A careful comparison of the NMR data of 2 with those of 9-hydroxycanthin-6-one revealed that the signal differences were only from the lactam ring moiety. According to the observable HMBC correlations (Figure 3) from the methyl proton signal to C-4 [δC 135.9 (d)] and C-6 [δC 159.8 (s)], and from H-4 [δH 8.02 (1H, br s)] to C-14 [δC 130.9 (s)], the newly emerging aliphatic methyl group was positioned at C-5. The resulting structure was further rechecked by the detailed 2D NMR analysis. Therefore, the structure of 2 was established as 9-hydroxy-5-methylcanthin-6-one and named as bovinalkaloid B, shown in Figure 2.
Compound 3, yellow amorphous powder, possessed a molecular formula of C16H12N2O2 by the negative HR-ESI-MS (m/z 263.0821 C16H11N2O2−, calcd. 263.0826), which was 14 Da (CH2) more than that of 2. The 1H NMR spectrum (Table 2) in DMSO-d6 of 3 also showed a pair of characteristic heterocyclic aromatic doublets at δH 8.74 (1H, d, J = 5.0 Hz, H-2) and 8.09 (1H, d, J = 5.0 Hz, H-1), three aromatic protons assignable to a 1,2,4-trisubstituted benzene ring, as well as two olefinic methyl singlets in the up-field region. The 13C NMR spectrum (Table 2) showed 14 sp2 carbon signals (5 × CH and 9 × C) in the range from 103.0—160.4 ppm, as well as two methyl carbons at δC 13.3 (q) and 12.9 (q). The above NMR features were very similar to those of 2, and allowed us to infer that the structure 3 should be a 4,5-dimethylated derivative of 9-hydroxycanthin-6-one. The HMBC correlations (Figure 3) from the methyl proton signal at δH 2.60 (3H, s) to C-5 [δC 132.3 (s)] and C-16 [δC 135.7 (s)], and from the other methyl signal at δH 2.27 (3H, s) to C-4 [δC 144.3 (s)] and C-6 [δC 159.4 (s)] confirmed the two methyl groups at C-4 and C-5, respectively. Thus, the structure of 3 was established as 9-hydroxy-4,5-dimethylcanthin-6-one and named as bovinalkaloid A, shown in Figure 2.
By comparing with data reported in the literature, the known compound 4 was identified as 9-hydroxycanthin-6-one (Jiang and Zhou, 2008). All of the isolated compounds (1–4) were evaluated for their in vitro antifungal activity against F. solani (Figure 1B). At the concentration of 1 mg/mL, the compounds 1–4 exhibited some degree of antifungal activity, with inhibition rates of 100.00%, 22.31%, 37.59%, and 59.29%, respectively. Compound 1 had significant antifungal activity against F. solani. For a better understanding of the antifungal activity and mechanisms of compound 1, a further in-depth study was required.
Compound 1 showed excellent antifungal activity against F. solani (Table 3). The inhibitory effect increased with increasing concentration. For compound 1 at a comparatively low concentration of 0.52 mM, the mycelial growth inhibition rate was 42.11%. When the concentration reached 1.04 mM, the mycelial growth inhibition rate was greater than 70%. In particular, the mycelial growth of F. solani on PDA medium was entirely inhibited by compound 1 at the concentration of 4.16 mM. The MIC of compound 1 are illustrated in Figure 4. Compound 1 at 2.08 mM or higher concentrations entirely inhibited visible growth of F. solani after 2 days of incubation in the dark at 25°C. Thus, the MIC of compound 1 was 2.08 mM.
Table 3. Effect of different concentrations of compound 1 on mycelial growth and spore germination of Fusarium solani.
Figure 4. The inhibitory effect of different concentrations of compound 1 against the mycelial growth of Fusarium solani.
We also researched the effect of compound 1 on the germination of F. solani spores (Table 3). The spore germination was significantly inhibited by compound 1 in a dose-dependent manner. When the concentration at 4.16 mM, the spore germination inhibition rate was 94.37%, less than 10% of F. solani spores germinated.
The potential antifungal mechanisms of compound 1 against F. solani were investigated by light microscopy. Figure 5 shows the mycelial morphology in the control and compound 1 treated groups. The control F. solani mycelia were regular and complete, and the surface of the mycelium was smooth and without cracks (Figure 5A). However, the mycelia treated with compound 1 displayed a damaged morphology. As shown in Figure 5B, mycelia appeared collapsed, malformed, and atrophied, and their surface structure became fractured and rough.
Figure 5. The effect of compound 1 on mycelial morphology of Fusarium solani. Normal mycelial morphology (A); Mycelia treated with MIC of compound 1 (B).
Examination of the morphological changes in F. solani mycelia before and after being treated with compound 1 was important for discovering the targets upon which compound 1 act. Obviously, compound 1 treatment resulted in surface structure damage to F. solani mycelia. This implied that compound 1 might destroy the structure of the membrane system to change its permeability, inducing the leakage of intracellular substances. Similar research has demonstrated that with chelerythrine treatment, the mycelia of Ustilaginoidea virens collapse and become twisted, and the membrane becomes thin and rough (Wei et al., 2020). Based on the above analysis, we studied the effects of compound 1 on cell membrane permeability.
The cell membrane is a semipermeable protective structure that can regulate the homeostasis of internal and external environments of fungi. When the membrane is stimulated by externally applied compounds, the electrical conductivity increases due to damage to cell membranes, consequently resulting in solute leakage (Cai et al., 2019). In the present study, electrolyte leakage, nucleic acid content, and protein content of the supernatant were used to determine the membrane permeability. The results (Figures 6A–C) indicated that after compound 1 treatment, the electric conductivity, nucleic acid content, and protein content were significantly higher than in the control group. After incubation for 20 h, the electric conductivity of the compound 1 treated group (121.24 μs/cm) was approximately 2.65-fold higher than that of the control group (45.78 μs/cm); the nucleic acid content (OD260) of the compound 1 treated group (0.493) was approximately 2.26-fold higher than that of the control group (0.218), and the protein content (OD280) of the compound 1 treated group (0.592) was approximately 1.79-fold higher than that of the control group (0.331).
Figure 6. The effect of compound 1 on electrical conductivity (A), nucleic acid content (B), protein content (C), and lipid content (D) of Fusarium solani.
These results further demonstrated that compound 1 might damage the cell membrane of F. solani, leading to leakage of the nucleic acids, proteins, and electrolytes. Similar results have been observed in Botrytis cinerea (Ji et al., 2020) and Penicillium roqueforti (Ju et al., 2020). After confirming that the membrane permeability of F. solani was affected by compound 1, the main constituents of the membrane were measured. The integrity of the cell membrane is critical for F. solani growth.
Lipids are essential components of cell membranes, and they are vitally important for F. solani structural integrity (Lu et al., 2019). As shown in Figure 6D, the total lipid content of the control group remained largely the same at 80.23 ± 3.25 mg/g. In contrast, the lipid content of the compound 1 treated group was lower than that of the control group, and the content followed a gradually decreasing tend, reaching a minimum of 29.62 ± 2.89 mg/g at 20 h. The reduction of the total lipid content indicated the disruption of cell membrane integrity.
Numerous studies have reported that lipid peroxidation is one of the main causes of damage to cell membranes (Liu et al., 2020). MDA is a terminal product of lipid peroxidation, and it can cause the cross-linking polymerization of nucleic acids, proteins, and other biological macromolecules. We measured the content of MDA in mycelia of F. solani as an indicator of lipid peroxidation. As shown in Figure 7A, the MDA level of compound 1 treated group was consistently higher than that of the control group. Especially at 10 h, the MDA content of compound 1 treated group (39.83 μmol/g) was approximately 2.79-fold higher than that of the control group (14.23 μmol/g), indicating that lipid peroxidation had taken place in F. solani.
Lipid peroxidation is generally caused by excess reactive oxygen species (ROS) such as superoxide and H2O2 that are necessary products of aerobic metabolism (Li et al., 2018). If the generated ROS are not scavenged in a timely manner, they can cause oxidative damage to lipids, proteins, and DNA. To examine this, we measured H2O2 levels in F. solani and found that the H2O2 content in the compound 1 treated group was markedly higher than in the control group (Figure 7B). These results suggest that compound 1 could induce ROS generation and increase lipid peroxidation.
ROS production and scavenging maintain a dynamic balance in the normal cellular systems. Cells normally protect themselves against ROS damage by activating antioxidant defense systems (Li et al., 2012). CAT, POD, and SOD play crucial roles in antioxidant systems that scavenge ROS by transforming H2O2 into water (Yang et al., 2020). Therefore, it was necessary to measure the respective antioxidant enzyme activities of F. solani mycelia. As shown in Figure 8, CAT, POD, and SOD activities of the control group remained essentially unchanged during 0–20 h, while the antioxidant enzymes activities in the compound 1 treated group exhibited an initial increasing trend and then decreased.
Figure 8. The effect of compound 1 on CAT activity (A), POD activity (B), and SOD activity (C) of Fusarium solani.
Based on the results, it was supposed that in the early stages of compound 1 treatment, the defense system of F. solani up-regulated the levels of these antioxidant enzymes in response to increased ROS. However, with increasing compound 1 treatment time, the production of ROS overwhelmed the scavenging capacity of antioxidant enzymes, thereby disrupting the balance of ROS production and scavenging in F. solani. Immediately after, the antioxidant enzymes were damaged by excessive ROS, leading to a reduction in the activities of antioxidant enzymes and incrementing production of ROS. The accumulated ROS reacted with polyunsaturated fatty acids to produce MDA (lipid peroxidation) and eventually led to cell death. This further confirmed that compound 1 could induce ROS generation that caused lipid peroxidation, eventually resulting in cell membrane damage.
In the present study, four alkaloids including three new compounds (1–3), were isolated and identified by bioassay-guided isolation and spectroscopic analyses from mushroom S. bovinus. Compound 1 strongly inhibited the mycelial growth and spore germination of F. solani, the causal fungus of ginseng root rot. Furthermore, we investigated the antifungal mechanisms of compound 1. Compound 1 could induce ROS generation that causes lipid peroxidation, resulting in cell membrane injury, solute and electrolyte leakage, and subsequent cell death. We believe that these findings will provide a theoretical foundation for the application of compound 1 as a potential natural fungicide for the control of ginseng root rot.
The original contributions presented in the study are included in the article/Supplementary material, further inquiries can be directed to the corresponding authors.
MX: Formal analysis, Investigation, Writing – original draft. XY: Investigation, Supervision, Validation, Writing – review & editing. LY: Investigation, Validation, Visualization, Writing – original draft. ZL: Data curation, Project administration, Software, Visualization, Writing – review & editing. JZ: Formal analysis, Funding acquisition, Investigation, Validation, Writing – original draft. JL: Conceptualization, Funding acquisition, Project administration, Resources, Writing – original draft.
The author(s) declare that financial support was received for the research, authorship, and/or publication of this article. Science Research Start-up Fund for Doctor of Hebei Normal University, grant numbers L2023B22 and L2024B16.
The authors declare that the research was conducted in the absence of any commercial or financial relationships that could be construed as a potential conflict of interest.
All claims expressed in this article are solely those of the authors and do not necessarily represent those of their affiliated organizations, or those of the publisher, the editors and the reviewers. Any product that may be evaluated in this article, or claim that may be made by its manufacturer, is not guaranteed or endorsed by the publisher.
The Supplementary material for this article can be found online at: https://www.frontiersin.org/articles/10.3389/fmicb.2024.1408013/full#supplementary-material
Bhutia, D., Zhimo, V. Y., Kole, R., and Saha, J. (2016). Antifungal activity of plant extracts against Colletotrichum musae, the postharvest anthracnose pathogen of banana cv. Martaman. Nutr. Food Sci. 46, 2–15. doi: 10.1108/NFS-06-2015-0068
Cai, M., Lv, H., Cao, C., Zhang, L., Cao, R., and Xu, B. (2019). Evaluation of antimicrobial activity of Pterocarpus extracts. Ind. Crop Prod. 140:111668. doi: 10.1016/j.indcrop.2019.111668
Chen, C. J., Li, Q. Q., Zeng, Z. Y., Duan, S. S., Wang, W., Xu, F. R., et al. (2020). Efficacy and mechanism of Mentha haplocalyx and Schizonepeta tenuifolia essential oils on the inhibition of Panax notoginseng pathogens. Ind. Crop Prod. 145:112073. doi: 10.1016/j.indcrop.2019.112073
Chowdhury, M. D. E. K., and Bae, H. (2018). Bacterial endophytes isolated from mountain-cultivated ginseng (Panax ginseng Mayer) have biocontrol potential against ginseng pathogens. Biol. Control 126, 97–108. doi: 10.1016/j.biocontrol.2018.08.006
Elsherbiny, E. A., Dawood, D. H., and Safwat, N. A. (2021). Antifungal action and induction of resistance by β-aminobutyric acid against Penicillium digitatum to control green mold in orange fruit. Pestic. Biochem. Physiol. 171:104721. doi: 10.1016/j.pestbp.2020.104721
Feng, W., Sun, X., and Ding, G. (2022). Morphological and transcriptional characteristics of the symbiotic interaction between Pinus massoniana and Suillus bovinus. J. Fungi 8:1162. doi: 10.3390/jof8111162
He, X., Du, X., Zang, X., Dong, L., Gu, Z., Cao, L., et al. (2016). Extraction, identification and antimicrobial activity of a new furanone, grifolaone a, from Grifola frondosa. Nat. Prod. Res. 30, 941–947. doi: 10.1080/14786419.2015.1081197
Helal, G. A., Sarhan, M. M., Abu Shahla, A. N., and Abou El-Khair, E. K. (2007). Effects of Cymbopogon citratus L. essential oil on the growth, morphogenesis and aflatoxin production of aspergillus flavus ML2-strain. J. Basic Microbiol. 47, 5–15. doi: 10.1002/jobm.200610137
Huang, Y. T., Onose, J., Abe, N., and Yoshikawa, K. (2009). In vitro inhibitory effects of pulvinic acid derivatives isolated from chinese edible mushrooms, boletus calopus and Suillus bovinus, on cytochrome p450 activity. Biosci. Biotechnol. Biochem. 73, 855–860. doi: 10.1271/bbb.80759
Ji, J. Y., Yang, J., Zhang, B. W., Wang, S. R., Zhang, G. C., and Lin, L. N. (2020). Sodium pheophorbide a controls cherry tomato gray mold (Botrytis cinerea) by destroying fungal cell structure and enhancing disease resistance-related enzyme activities in fruit. Pestic. Biochem. Physiol. 166:104581. doi: 10.1016/j.pestbp.2020.104581
Jiang, M. X., and Zhou, Y. J. (2008). Canthin-6-one alkaloids from Picrasma quassioides and their cytotoxic activity. J. Asian Nat. Prod. Res. 10, 1009–1012. doi: 10.1080/10286020802277956
Ju, J., Xie, Y., Yu, H., Guo, Y., Cheng, Y., Zhang, R., et al. (2020). Major components in lilac and Litsea cubeba essential oils kill Penicillium roqueforti through mitochondrial apoptosis pathway. Ind. Crop Prod. 149:112349. doi: 10.1016/j.indcrop.2020.112349
Leonardus, B. S. K., Angerhofer, G. K., Tsauri, S., Padmawinata, K., Pezzuto, J. M., and Kinghorn, A. D. (1991). Cytotoxic and antimalarial constituents of the roots of Eurycoma longifolia. J. Nat. Prod. 54, 1360–1367. doi: 10.1021/np50077a020
Li, T., Kim, J. H., Jung, B., Ji, S., Seo, M. W., Han, Y. K., et al. (2020). Transcriptome analyses of the ginseng root rot pathogens Cylindrocarpon destructans and fusarium solani to identify radicicol resistance mechanisms. J. Ginseng Res. 44, 161–167. doi: 10.1016/j.jgr.2018.11.005
Li, M., Xu, T., Zhou, F., Wang, M., Song, H., Xiao, X., et al. (2018). Neuroprotective effects of four phenylethanoid glycosides on H₂O₂-induced apoptosis on PC12 cells via the Nrf2/ARE pathway. Int. J. Mol. Sci. 19:1135. doi: 10.3390/ijms19041135
Li, Y., Zhang, W., Niu, J., and Chen, Y. (2012). Mechanism of photogenerated reactive oxygen species and correlation with the antibacterial properties of engineered metal-oxide nanoparticles. ACS Nano 6, 5164–5173. doi: 10.1021/nn300934k
Liu, Q., Kong, W., Hu, S., Kang, Y., Zhang, Y., and Ng, T. B. (2020). Effects of Oudemansiella radicata polysaccharide on postharvest quality of oyster mushroom (Pleurotus ostreatus) and its antifungal activity against Penicillium digitatum. Postharvest Biol. Technol. 166:111207. doi: 10.1016/j.postharvbio.2020.111207
Lu, S., Liu, H., Jin, C., Li, Q., and Guo, L. (2019). An efficient and comprehensive plant glycerolipids analysis approach based on high-performance liquid chromatography-quadrupole time-of-flight mass spectrometer. Plant Direct. 3:e00183. doi: 10.1002/pld3.183
Palacios, S., Casasnovas, F., Ramirez, M. L., Reynoso, M. M., and Torres, A. M. (2014). Impact of water potential on growth and germination of fusarium solani soilborne pathogen of peanut. Braz. J. Microbiol. 45, 1105–1112. doi: 10.1590/s1517-83822014000300046
Souza, D. P., Pimentel, R. B. Q., Santos, A. S., Albuquerque, P. M., Fernandes, A. V., Junior, S. D., et al. (2020). Fungicidal properties and insights on the mechanisms of the action of volatile oils from Amazonian Aniba trees. Ind. Crop Prod. 143:111914. doi: 10.1016/j.indcrop.2019.111914
Sun, X., Zhao, Y., and Ding, G. (2023). Morphogenesis and metabolomics reveal the compatible relationship among Suillus bovinus, Phialocephala fortinii, and their co-host, Pinus massoniana. Microbiol. Spectr. 11:e0145323. doi: 10.1128/spectrum.01453-23
Wang, S., Bao, L., Zhao, F., Wang, Q., Li, S., Ren, J., et al. (2013). Isolation, identification, and bioactivity of monoterpenoids and sesquiterpenoids from the mycelia of edible mushroom Pleurotus cornucopiae. J. Agric. Food Chem. 61, 5122–5129. doi: 10.1021/jf401612t
Wang, H., and Ng, T. B. (2004). Eryngin, a novel antifungal peptide from fruiting bodies of the edible mushroom Pleurotus eryngii. Peptides 25, 1–5. doi: 10.1016/j.peptides.2003.11.014
Wang, Q., Wang, F., Xu, Z., and Ding, Z. (2017). Bioactive mushroom polysaccharides: a review on monosaccharide composition, biosynthesis and regulation. Molecules 22:955. doi: 10.3390/molecules22060955
Wei, Q. H., Cui, D. Z., Liu, X. F., Chai, Y. Y., Zhao, N., Wang, J. Y., et al. (2020). In vitro antifungal activity and possible mechanisms of action of chelerythrine. Pestic. Biochem. Physiol. 164, 140–148. doi: 10.1016/j.pestbp.2020.01.007
Woo, E. E., Kim, J. Y., Kim, J. S., Kwon, S. W., Lee, I. K., and Yun, B. S. (2019). Mannonerolidol, a new nerolidol mannoside from culture broth of Schizophyllum commune. J. Antibiot. 72, 178–180. doi: 10.1038/s41429-018-0130-3
Wu, F. E., Koike, K., Nikaido, T., Sakamoto, Y., Ojmoto, T., and Ikeda, K. (1989). New β-carboline alkaloids from a chinese medicinal plant, Arenaria kansuensis. Structures of Arenarines a, B, C, and D. Chem. Pharm. Bull. 37, 1808–1809. doi: 10.1248/cpb.37.1808
Xiao, C., Yang, L., Zhang, L., Liu, C., and Han, M. (2016). Effects of cultivation ages and modes on microbial diversity in the rhizosphere soil of Panax ginseng. J. Ginseng Res. 40, 28–37. doi: 10.1016/j.jgr.2015.04.004
Xu, J., Chu, Y., Liao, B., Xiao, S., Yin, Q., Bai, R., et al. (2017). Panax ginseng genome examination for ginsenoside biosynthesis. Gigascience. 6, 1–15. doi: 10.1093/gigascience/gix093
Yang, Q., Wang, J., Zhang, P., Xie, S., Yuan, X., Hou, X., et al. (2020). In vitro and in vivo antifungal activity and preliminary mechanism of cembratrien-diols against Botrytis cinerea. Ind. Crop Prod. 154:112745. doi: 10.1016/j.indcrop.2020.112745
Yu, J. S., Roh, H. S., Baek, K. H., Lee, S., Kim, S., So, H. M., et al. (2018). Bioactivity-guided isolation of ginsenosides from Korean red ginseng with cytotoxic activity against human lung adenocarcinoma cells. J. Ginseng Res. 42, 562–570. doi: 10.1016/j.jgr.2018.02.004
Keywords: Fusarium solani , Suillus bovinus , mushrooms, alkaloids, antifungal activities
Citation: Xiong M, Yang X, Yao L, Li Z, Zhang J and Lv J (2024) Bioassay-guided isolation of three new alkaloids from Suillus bovinus and preliminary mechanism against ginseng root rot. Front. Microbiol. 15:1408013. doi: 10.3389/fmicb.2024.1408013
Received: 27 March 2024; Accepted: 22 April 2024;
Published: 02 May 2024.
Edited by:
Antonio Bevilacqua, University of Foggia, ItalyReviewed by:
Baohui Lu, Jilin Agriculture University, ChinaCopyright © 2024 Xiong, Yang, Yao, Li, Zhang and Lv. This is an open-access article distributed under the terms of the Creative Commons Attribution License (CC BY). The use, distribution or reproduction in other forums is permitted, provided the original author(s) and the copyright owner(s) are credited and that the original publication in this journal is cited, in accordance with accepted academic practice. No use, distribution or reproduction is permitted which does not comply with these terms.
*Correspondence: Jinxiu Zhang, eGl1ZG91ODgyMDAzQDE2My5jb20=; Jianhua Lv, bHZqaWFuaHVhQGhlYnR1LmVkdS5jbg==
Disclaimer: All claims expressed in this article are solely those of the authors and do not necessarily represent those of their affiliated organizations, or those of the publisher, the editors and the reviewers. Any product that may be evaluated in this article or claim that may be made by its manufacturer is not guaranteed or endorsed by the publisher.
Research integrity at Frontiers
Learn more about the work of our research integrity team to safeguard the quality of each article we publish.