- 1Department of Clinical Laboratory, The Second Affiliated Hospital of Soochow University, Suzhou, Jiangsu, China
- 2Department of Blood Transfusion, The Children’s Hospital of Soochow University, Suzhou, Jiangsu, China
- 3MOE Key Laboratory of Geriatric Diseases and Immunology, The Second Affiliated Hospital of Soochow University, Suzhou, Jiangsu, China
- 4Sichuan Provincial Key Laboratory for Human Disease Gene Study, Department of Laboratory Medicine, Sichuan Provincial People’s Hospital, University of Electronic Science and Technology of China, Chengdu, China
- 5Department of Pharmacy Practice, School of Pharmacy and Pharmaceutical Sciences, University at Buffalo, Buffalo, NY, United States
Introduction: Enterobacter chengduensis was defined as a novel species in the genus. Enterobacter in 2019, however, antimicrobial resistance, such as carbapenem resistance, has rarely been described in E. chengduensis. This study described the molecular features of four carbapenem-resistant E. chengduensis strains collected from a tertiary health care hospital in Southwest China.
Methods: Whole genome sequencing (WGS) was used to determine the genome sequence of four E. chengduensis strains. The precise species of strains were identified by average nucleotide identity (ANI) and in silico DNA-DNA hybridization (isDDH). The clonal relatedness of four E. chengduensis strains and additional 15 ones from NCBI were examined through phylogenetic analysis. The molecular features of E. chengduensis and genetic structure of carbapenemase- encoding plasmids were characterized through genomic annotation and analysis.
Results: The results revealed the emergence of blaNDM–1-carrying E. chengduensis strains in China. Multilocus sequence typing (MLST) analysis showed that all 19 E. chengduensis belonged to the same sequence type of ST414. Core SNP analysis suggested the potential intrahospital clonal transmission of ST414 E. chengduensis. The carbapenemase-encoding gene blaNDM–1 was harbored by an IncC-type plasmid, which was experimentally confirmed to be able to conjugate.
Discussion This study reports the first emergence and potential clonal transmission of blaNDM–1-carrying E. chengduensis. Further surveillance should be advocated to monitor the dissemination of carbapenem-resistant E. chengduensis and blaNDM–1-harboring IncC-type plasmids in China.
Introduction
As the third most prevalent pathogenic species of Enterobacteriaceae in humans, after Escherichia and Klebsiella, the Enterobacter genus can trigger various clinical infections, including bloodstream and intra-abdominal infections (Mezzatesta et al., 2012; Davin-Regli et al., 2019). The accurate identification of species and subspecies in the genus Enterobacter has been a challenge due to the large variations in phenotypic and genotypic characteristics (Wu et al., 2021). Clinical microbial labs frequently fail to detect Enterobacter species, making it difficult to establish a clear correlation between specific species with antimicrobial resistance and clinical impact (Mezzatesta et al., 2012). Recently, Enterobacter taxonomy has been greatly improved because of the application of whole-genome sequencing (WGS) (Zong et al., 2021). To date, 24 species with known names and 21 taxa (genomospecies) without assigned names are designed in Enterobacter (Feng et al., 2021). Among them, a novel species of E. chengduensis was redefined through phenotypic and genotypic characteristics in 2019 (Wu et al., 2019). The E. chengduensis strain reported in this paper was recovered from a human blood sample in China in 2015. The whole genome sequence of this type strain exhibits an average nucleotide identity ranging from 80.48% to 93.34% when compared to the type strains of all recognized Enterobacter species. It can also be distinguished from all recognized Enterobacter species by its ability to ferment d-sorbitol, l-rhamnose and melibiose but with a negative Voges–Proskauer reaction. However, the epidemiological characteristics and molecular features of E. chengduensis haven’t been reported since its identification and remain largely unknown.
Carbapenems serve as the cornerstone in the therapeutic arsenal against multidrug-resistant Enterobacter infections (Zhang et al., 2018). However, the escalating resistance to carbapenems within Enterobacter spp., predominantly attributed to the acquisition of genes encoding carbapenemase enzymes (such as KPC, NDM, and VIM), has substantially undermined the efficacy of clinical antimicrobial treatment options (Nordmann and Poirel, 2019; Zong et al., 2021). Further investigations into the transmission mechanisms of carbapenemase-encoding genes will aid us in deciphering and limiting the spread of these genes in Enterobacter.
In our previous multicenter study (Zhu et al., 2022), we have preliminarily identified four clinical blaNDM–1-carrying E. chengduensis strain. Notably, among 98 Enterobacter strains, E. chengduensis ranked third in terms of its prevalence. To provide valuable insights on this rarely reported Enterobacter species, and clarify the potential transmission mechanisms of carbapenemase-encoding genes, we conducted a detailed genomic study on these four E. chengduensis strains. Herein, average nucleotide identity (ANI) and in silico DNA–DNA hybridization (isDDH) was used to identify the precise species of strains. The clonal relatedness of four E. chengduensis strains and additional 15 ones from NCBI were examined through phylogenetic analysis. The molecular features of E. chengduensis and genetic structure of blaNDM–1-carrying IncC plasmids were characterized through genomic annotation and analysis.
Materials and methods
Stain collection and antimicrobial susceptibility
Four carbapenem-resistant Enterobacter strains causing nosocomial infections were collected from seven tertiary health care hospitals in different provinces or cities in China between January 2017 and March 2021. Detailed bacterial strain collection was described in our previous study (Zhu et al., 2022). Clinical data were obtained from medical chart using the same standardized questionnaire in each hospital.
Whole-genome sequencing (WGS)
Genomic DNA of carbapenem resistant Enterobacter strains were prepared using the Omega Bio-Tek Bacterial DNA Kit (Doraville, GA, USA). The extracted DNA was detected by the agarose gel electrophoresis and quantified by Qubit (Thermo Scientific). Sequencing libraries were generated using NEBNext Ultra DNA Library Prep Kit (NEB, USA) following manufacturer’s recommendations and index codes were added to attribute sequences to each sample. At last, PCR products were purified (AMPure XP system) and libraries were analyzed for size distribution by Agilent2100 Bioanalyzer and quantified using real-time PCR. Whole-genome sequencing was performed using the Illumina NovaSeq 6000 platform (Illumina Inc., San Diego, CA, USA) with the 350 bp paired-end protocols. Reads were filtered using fastP 0.17.1, and evaluated through FastQC 0.9.1. The above methods can ensure the quantity and quality of DNA data. The qualified data were then de novo assembled to contigs using SPAdes 3.11. Further plasmid assembly was obtained by mapping contigs on reference plasmid sequence (> 99% identical), checking overlapping paired ends and gap closure by PCR and Sanger sequencing.
Species identification
Precise species identification was performed by calculating the pairwise isDDH and ANI value between the genome sequence of the query strain and the type strains of Enterobacter spp. described recently (Zong et al., 2021). The pairwise ANI with a ≥ 96% cutoff and isDDH with a ≥ 70.0% cutoff were used for precise species identification as previously suggested (Meier-Kolthoff et al., 2013; Rosselló-Móra and Amann, 2015). The isDDH analysis was performed by calculating identities/HSP length value through GGDC 3.0 (Meier-Kolthoff et al., 2022), while ANI analysis was performed by using OAT 0.93.1 (Lee et al., 2016).
Genome analysis
Open-reading frames (ORFs) and pseudogenes were predicted using RAST 2.0 (Brettin et al., 2015) combined with BLASTp/BLASTn searches. Multilocus sequence typing (MLST) as well as annotation of resistance genes, mobile elements and other features were carried out using online databases including PubMLST (Jolley et al., 2018), CARD (Alcock et al., 2020), ResFinder (Bortolaia et al., 2020), PlasmidFinder (Carattoli et al., 2014), ISfinder (Siguier et al., 2006), and INTEGRALL (Moura et al., 2009). Multiple and pairwise sequence comparisons were performed using BLASTn. Gene organization diagrams were drawn through scripts from Danmel (Wang et al., 2022), and displayed using Inkscape 1.0.1
Phylogenetic analysis
Zong et al. collected 4899 high-quality Enterobacter genome sequences within GenBank and carried out the precise species identification (Zong et al., 2021). Using the method described in this document, we further determined publicly available 15 E. chengduensis genome sequences within GenBank (excluding low-quality and duplicated sequences; accessed by January 19, 2022).
A phylogenetic analysis was performed on 19 identified E. chengduensis strains, including 15 strains from NCBI (Supplementary Table 1), and the four strains from this study. The genome sequences of E. chengduensis were aligned to the complete chromosome sequence (GenBank accession number CP004345) of E. chengduensis strain WCHECh050004, and the sequence of E. cloacae strain ATCC 13047 (GenBank accession number NC_014121) was used as an out group. The core single nucleotide polymorphisms (SNPs) were identified by Mummer 3.25 (Delcher et al., 2003). A maximum-likelihood phylogenetic tree was constructed using MEGAX 10.1.8 (Kumar et al., 2018) based on the core SNPs with a bootstrap iteration of 1000, and displayed using iTOL.2
Conjugal transfer
We conducted conjugal transfer experiments between four E. chengduensis strains (HD5030, HD7411, HD7423 and HD7427) and the recipient E. coli J53 strain (NaN3-resistant) by combining mid-log-phase cultures of the donor and recipient (in a 1:10 donor to recipient ratio). Transconjugants were selected on plates with 2 μg/ml meropenem and 200 μg/ml NaN3. The conjugants were then confirmed by PCR for blaNDM as previously described (Nordmann et al., 2011).
Growth assay
Strains were grown overnight in 3 ml of LB with shaking (200 rpm) at 37°C, and then diluted to an OD600 of 0.25. 2 μl of the solution was added to 200 μl of LB in a 96-well plate in triplicate. Culture densities were determined every 10 min by measuring the OD600 for 16 h with shaking (200 rpm) at 37°C via FLUOstar Omega (BMG Labtech, Germany). Growth curves were estimated via GraphPad Prism 5.0 (GraphPad Software, Inc.) using two-way analysis of variance (ANOVA), and p-values < 0.05 were reported as statistically significant.
Nucleotide sequence accession numbers
The draft genome sequences of four E. chengduensis strains HD5030, HD7411, HD7423 and HD7427 were submitted to GenBank under BioProject PRJNA824645. The sequences of four mapped plasmids were submitted to GenBank under accession numbers ON209151-ON209154.
Results
Emergence of E. chengduensis strains
We identified four non-duplicate E. chengduensis strains (Supplementary Table 2) from the 92 Enterobacter strains described in our previous study (Zhu et al., 2022). The four strains (HD5030, HD7411, HD7423 and HD7427) were collected from the emergency intensive care unit (ICU) or gastroenterology department in a tertiary care hospital in Southwest China (Supplementary Table 2). All strains exhibited resistance to cephalosporins (ceftazidime, cefepime, cephalothin, cefazolin, ceftriaxone etc.), carbapenems (meropenem, imipenem, ertapenem), piperacillin/tazobactam, and fluoroquinolones (ciprofloxacin, levofloxacin, moxifloxacin), while they were susceptible to amikacin, sulfamethoxazole. Antibiotic resistance gene screening through ResFinder revealed that all four E. chengduensis strains carried the blaNDM–1 gene (Supplementary Table 3).
MLST and phylogenetic analysis of E. chengduensis strains
The results of MLST analysis showed that all 19 E. chengduensis strains analyzed in this study (including 15 ones from NCBI and four ones reported in this study) belonged to ST414 (Supplementary Tables 1, 2). A total of 2066 core genome SNPs were identified from these 19 strains and were used to construct a maximum-likelihood phylogenetic tree (Figure 1). The 19 strains were divided into three clades (clade I-III). A total of seven strains carried the carbapenemase-encoding gene, of which two strains from Colombia carried the blaKPC–2 gene, while five strains from China carried the blaOXA–48 gene (n = 1) and blaNDM–1 gene (n = 4). All four blaNDM–1-carrying strains belonged to clade III, while the other three belonged to clade II.
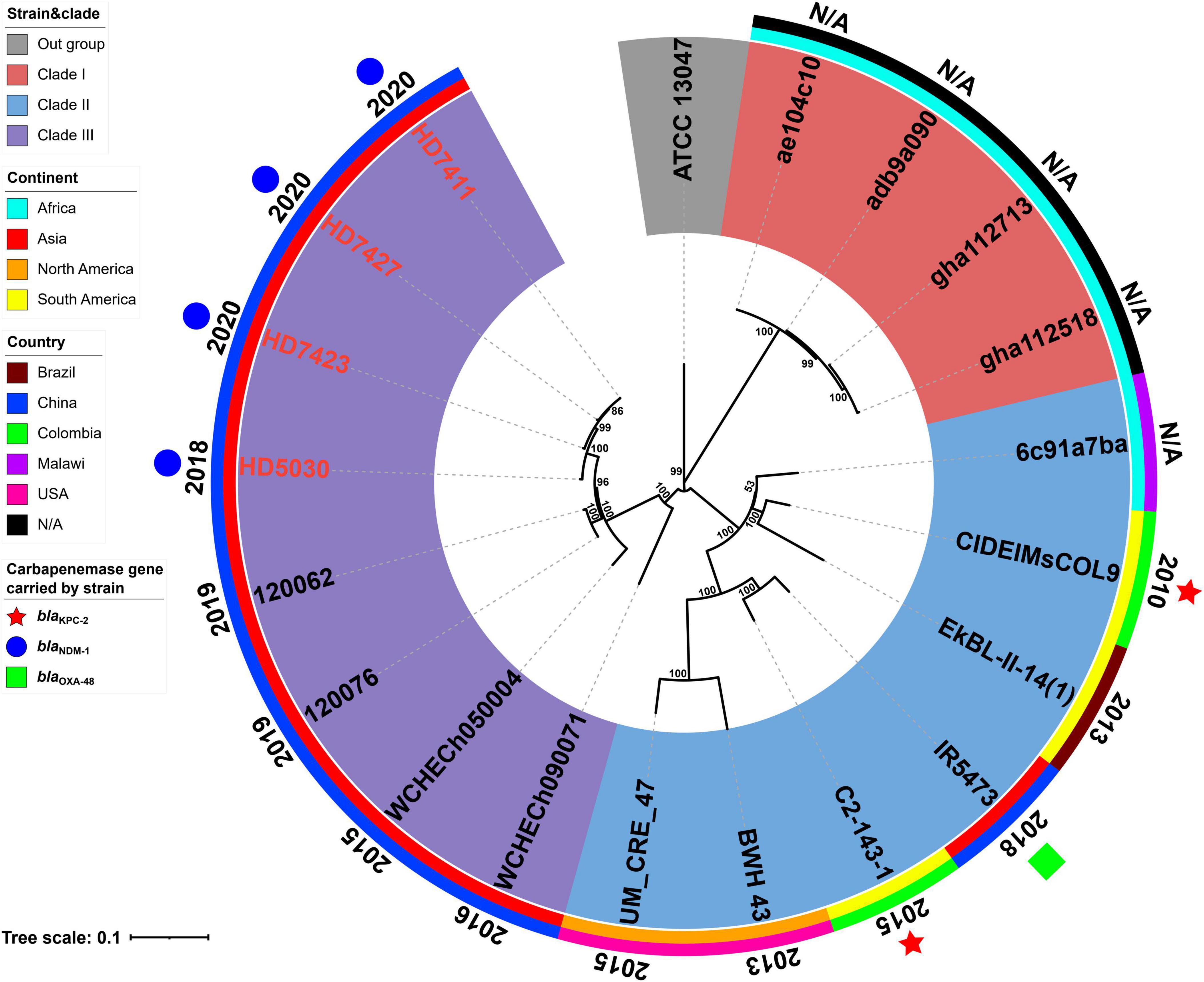
Figure 1. A Maximum-likelihood phylogenetic tree of ST414 Enterobacter chengduensis strains. A total of 19 ST414 E. chengduensis strains (including four reported in this study, and 15 from NCBI) were performed with phylogenetic analysis, while E. cloacae strain ATCC 13047 (GenBank accession number NC_014121) was used as the out group. Bar corresponds to scale of sequence divergence.
The core SNPs of 19 ST414 E. chengduensis strains were further pairwise compared to determine their clonal relatedness (Supplementary Table 4). In the nine strains from China, there were 5 to 592 SNP differences, suggesting that they did not belong to a single clone. However, the three strains in this study, HD7411, HD7423 and HD7427, only had only 5–10 SNP differences. Furthermore, these three strains were collected from different patients in two different wards in a hospital between April and June 2020. The hospitalization times of the three patients infected with these three strains overlapped, and the collection interval of these three strains was within one month (Supplementary Figure 1). These results suggest the potential intrahospital clonal transmission of ST414 E. chengduensis strains. Additionally, SNP comparison also showed that two blaKPC–2-carrying E. chengduensis strains did not belong to a single clone (having 302 SNPs difference).
Plasmid carrying the blaNDM–1 gene
The blaNDM–1 genes of four strains were all carried by IncC plasmids (Table 1). Each IncC plasmid could be divided into the backbone and two or three accessory modules, which resulted from exogenous DNA region insertion at different sites of the backbone (Figure 2). The backbones of the four plasmids were almost identical (Table 1) to the IncC reference plasmid p5_SCLZS62 (GenBank accession number CP082173), while these five plasmids were mainly different in accessory modules (Figures 3, 4). First, compared with p5_SCLZS62 and the other three plasmids, the plasmid pHD5030-NDM was interrupted by a 27.6-kb inserted regionorf2454 containing several inserted sequences (IS) (Figure 4A). Second, the four plasmids and reference plasmid p5_SCLZS62 all carried unit transposon Tn6358 variants (Figure 4B). Among them, p5_SCLZS62 harbored Tn6358b, whereas the four plasmids in this study harbored Tn6358c. Tn6358b and Tn6358c were inserted within the orf240 gene from the backbone of IncC plasmids and were flanked by 5-bp direct repeats (DRs). Moreover, Tn6358b and Tn6358c differed from Tn6358a (GenBank accession number JX141473) by exchanging integron In834 for truncated integron In7. However, truncated integron In7 from Tn6358b harbored three resistance loci: gene cassette array (GCA) aadB (VR1: variable region 1), ISCR1–truncated Tn125 (carrying blaNDM–1) region (VR2), and ISCR1–qnrA1 unit (VR3), while truncated integron In7 from Tn6358c further lost a 4.3-kb region including the VR3, a truncated qacED1 gene and a sul1 gene. In addition, the four plasmids all harbored the 15.1-kb inserted regionuvrD (Figure 4C), which was inserted within uvrD from backbone of IncC plasmid and was flanked by 6-bp DRs. The inserted regionuvrD carried a predicted efflux RND transporter (acrAB-tolC-like) and its function was not clear.
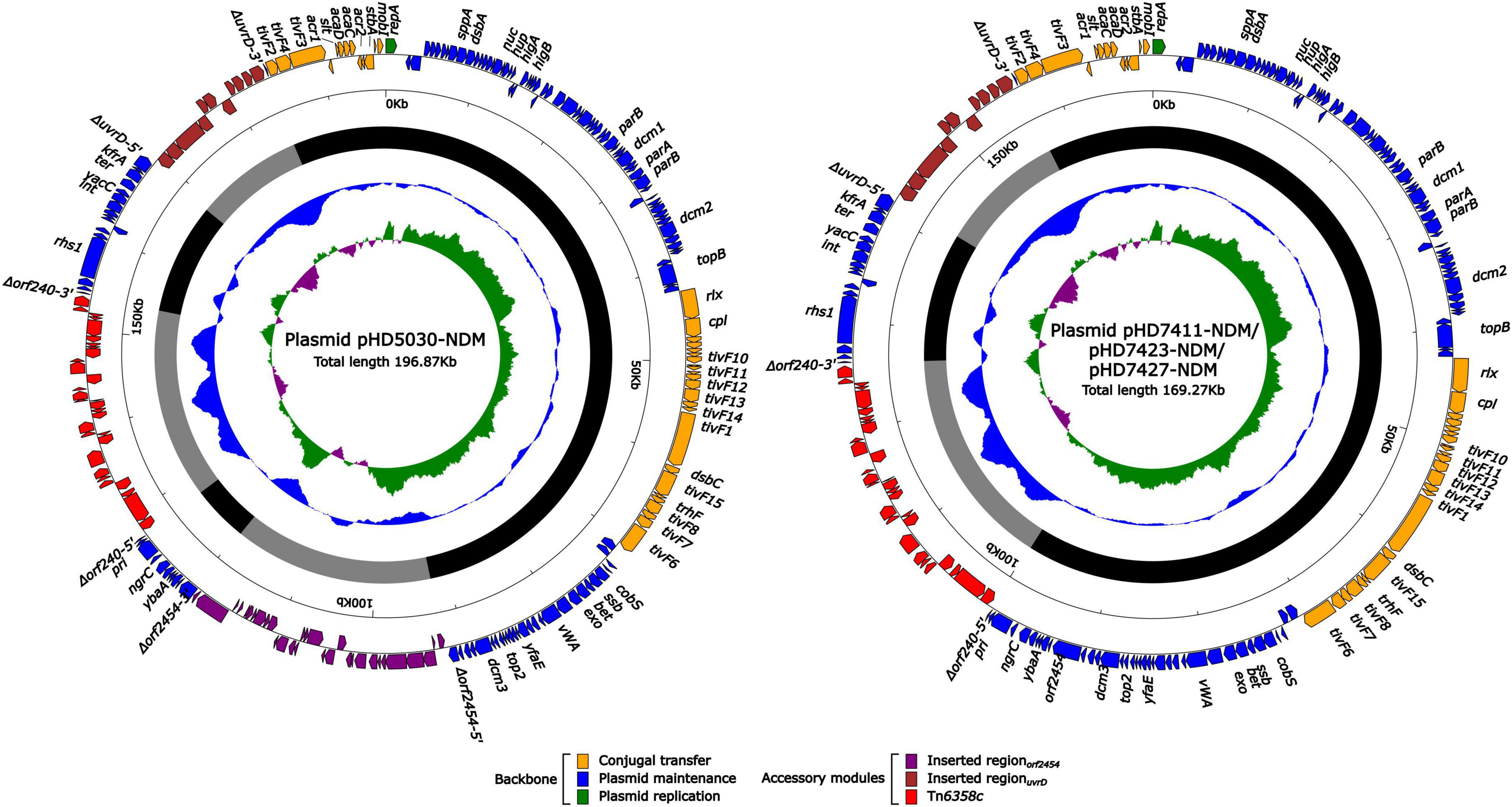
Figure 2. Schematic diagram of IncC-type plasmids assembled in this study. Genes of different functions are denoted by arrows and presented in various colors. The circles show (from outside to inside): predicted coding sequences, scale in 10 kb, backbone (black) and accessory module (gray) regions, GC content and GC skew [(G–C)/(G + C)].
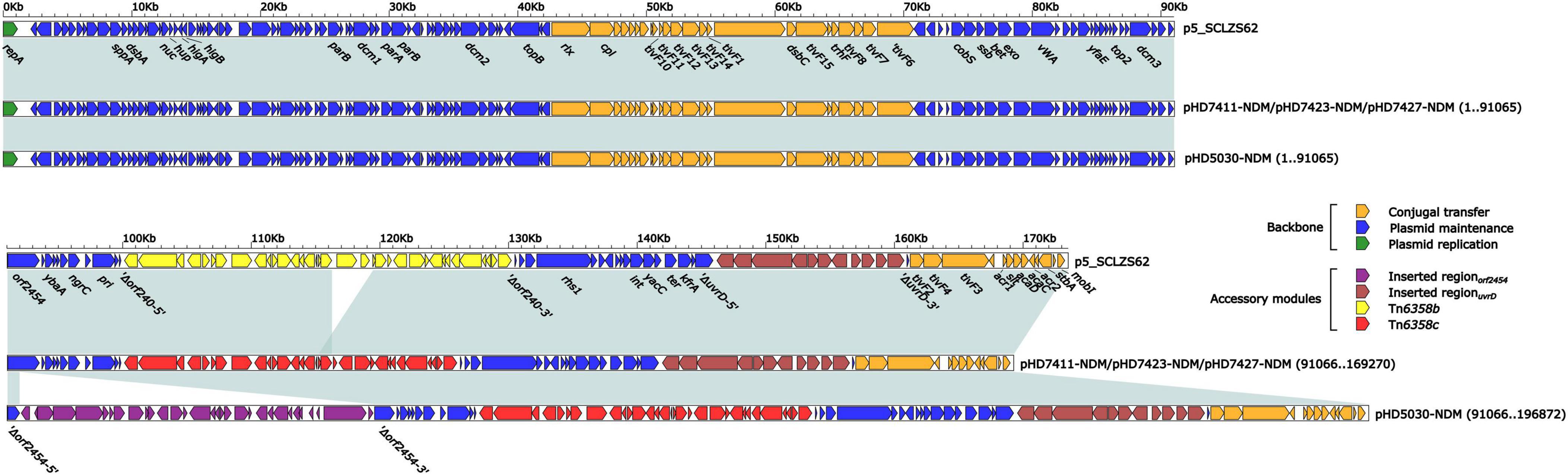
Figure 3. Linear comparison of IncC-type plasmids analyzed in this study. Genes are denoted by arrows. Genes, mobile genetic elements and other features are colored based on function classification. Shading regions denote homology of two plasmids (light blue: ≥ 99% nucleotide identity). The accession number of plasmid used as reference is CP082173.
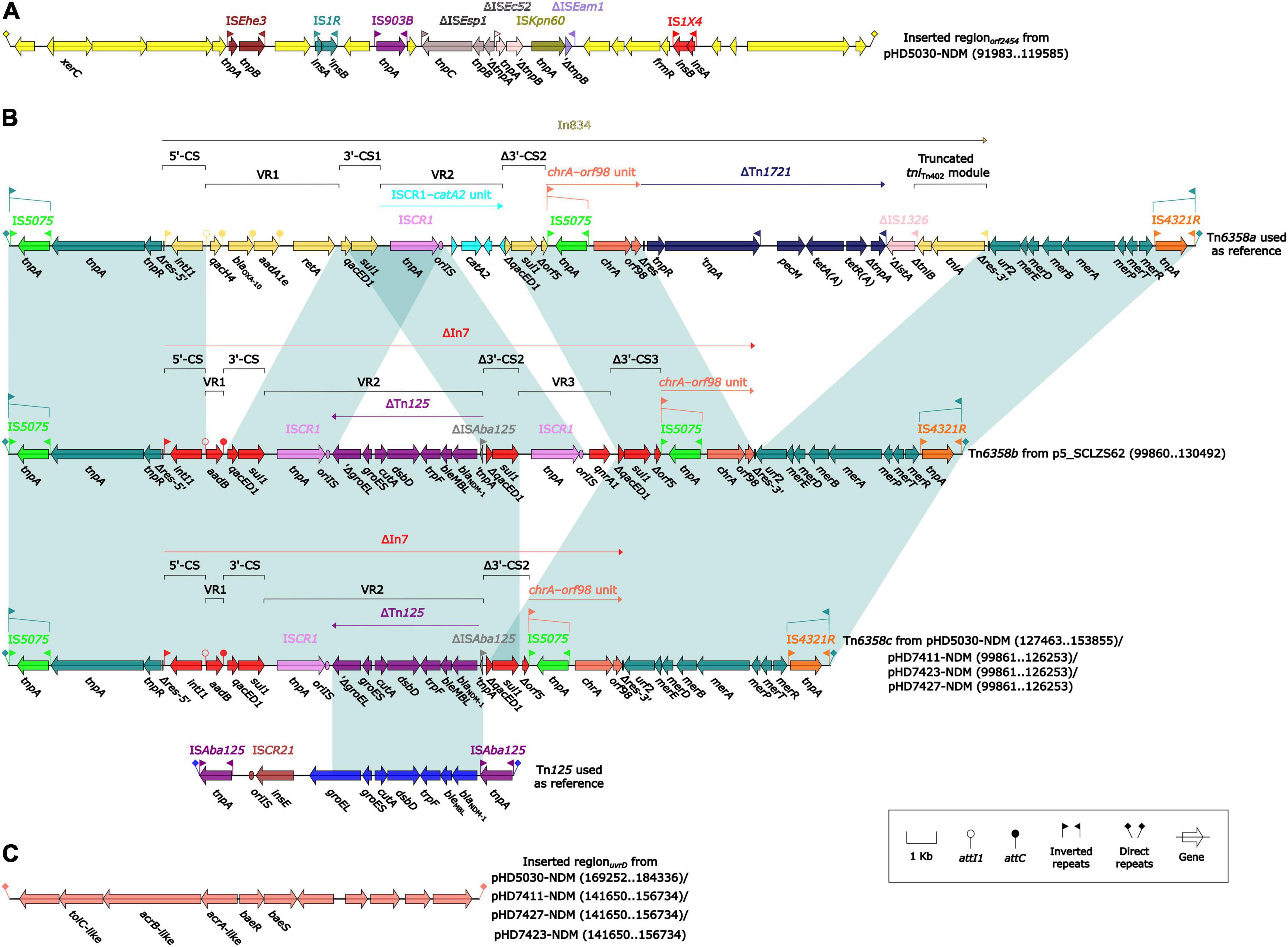
Figure 4. Organization of (A) inserted regionorf2454, (B) blaNDM regions, and (C) inserted regionuvrD from blaNDM-harboring plasmids. Genes are denoted by arrows. Genes, mobile genetic elements and other features are colored based on their functional classification. Shading denotes regions of homology (light blue: ≥ 99% nucleotide identity). The accession number of Tn6358a and Tn125 used as reference are JX141473 and JN872328, respectively.
Conjugal transfer experiment showed that all four blaNDM–1-carrying IncC-type plasmids could be transferred from host strains into the recipient E. coli J53. To assess the impact of the IncC-type plasmid on the growth of the strain, the growth of E. coli J53 was compared with the growth of its corresponding transformant carrying the IncC-type plasmid pHD5030-NDM. The growth curves of E. coli J53 and J53/pHD5030-NDM showed no significant difference (p > 0.05; Supplementary Figure 2). These results indicated that the IncC-type plasmid was self-transmissible, and had little impact on the growth of the E. coli J53 strain in LB.
Discussion
Studies on epidemiological characteristics and molecular features in E. chengduensis are very limited since this species was first reported in 2019 (Wu et al., 2019). Only one review paper identified 12 E. chengduensis strains from 4899 Enterobacter genomes (accessed by September 30, 2020) from the NCBI database through isDDH analysis (Zong et al., 2021). However, this review paper didn’t provide a detailed description of the characteristics of E. chengduensis. In order to clarify epidemiological characteristics and molecular features of E. chengduensis, we retrieved 15 E. chengduensis genomes from NCBI (accessed by January 19, 2022; Supplementary Table 1), and included four clinical E. chengduensis strains (Supplementary Table 2) from 98 Enterobacter strains collected in our previous study (Zhu et al., 2022) for further research. Interestingly, MLST analysis showed that all 19 E. chengduensis strains belonged to ST414. These results suggested that the current spread of E. chengduensis was still uncommon, and ST414 might be the predominant ST in E. chengduensis.
Nevertheless, the raise of carbapenem-resistance in E. chengduensis strains may spur the further dissemination of this species, and cause a significant threat to public health, which has been demonstrated in other Enterobacter species (Nordmann and Poirel, 2019; Zong et al., 2021). Among 15 E. chengduensis strains from NCBI, only three strains carried carbapenemase-encoding genes, including two carrying blaKPC–2 and one carrying blaOXA–48. However, all four E. chengduensis strains reported in this study carried blaNDM–1 gene (Supplementary Table 3). Phylogenetic analysis showed that these four E. chengduensis strains have relatively close homology (Figure 1). Furthermore, potential intrahospital clonal transmission of three strains among them was also detected (Supplementary Table 4 and Supplementary Figure 1). In view of this, blaNDM–1-carrying E. chengduensis should be closely monitored in China.
Horizontal transfer is one of the main mechanisms for carbapenemase-encoding gene transfer. In this study, we found that the blaNDM–1-harboring IncC-type plasmid was the only carbapenemase-encoding plasmid detected in the strains sequenced herein (Figure 2 and Table 1). These four plasmids shared highly identical backbone region (Figure 3 and Table 1), demonstrating their high level of homology. The IncC-type plasmid was conjugative, which was confirmed in this study and previous reports (Ambrose et al., 2018; Zheng et al., 2020; Fernandez et al., 2022). From the limited data in this study, E. chengduensis does not appear to be the natural host of the blaNDM gene. Thus, the self-transferability and low fitness cost (Supplementary Figure 2) of the blaNDM–1-harboring IncC-type plasmid may in part contribute to the emergence of blaNDM–1-carrying E. chengduensis. Additionally, IncC-type plasmid and its derived hybrid plasmid have been reported as an important vector for novel resistance genes, such as blaVMB–1 (Zheng et al., 2020), and tmexCD3-toprJ3 (Hirabayashi et al., 2021), which is cause for concern.
All blaNDM–1 genes reported in this study were carried by truncated Tn125 (Figure 4B). Tn125, a composite transposon bordered by two copies of ISAba125 (Poirel et al., 2012), plays a critical role in the initial spread of the blaNDM gene to plasmid (Acman et al., 2022). The truncated Tn125 element in this study was captured by the truncated integron In7 as a variable region, and subsequently integrated into the unit transposon Tn6358 (Wang et al., 2022). Although the mobility of Tn6358 has not been experimentally confirmed, the genetic element that can integrate various resistance genes after sophisticated events of transposition and homologous recombination needs to be cautious.
This study has some limitations. Firstly, the sample size of E. chengduensis in this study was small. The small sample size was in part because we focused on non-duplicated and high-quality sequences. Nevertheless, this dataset represents relatively comprehensive E. chengduensis genomic resources currently available and the data provide valuable insights on this rarely reported Enterobacter species. Secondly, our analysis on clonal relatedness of ST414 E. chengduensis strains, and function of IncC plasmid are not comprehensive, which necessitate further in-depth investigations.
In conclusion, this study reports the first emergence and potential clonal transmission of blaNDM–1-carrying E. chengduensis. ST414 might be the predominant ST in E. chengduensis. The IncC-type plasmid serves as the main vector of blaNDM–1 in this rarely reported Enterobacter species. Further surveillance should be advocated to monitor the dissemination of ST414 E. chengduensis and blaNDM–1-harboring IncC-type plasmids in China.
Data availability statement
The datasets presented in this study can be found in online repositories. The names of the repository/repositories and accession number(s) can be found in the article/Supplementary material.
Ethics statement
The studies involving humans were approved by the Second Affiliated Hospital of Soochow University. The studies were conducted in accordance with the local legislation and institutional requirements. The human samples used in this study were acquired from primarily isolated as part of your previous study for which ethical approval was obtained. Written informed consent for participation was not required from the participants or the participants’ legal guardians/next of kin in accordance with the national legislation and institutional requirements.
Author contributions
HF: Data curation, Formal analysis, Investigation, Validation, Visualization, Writing–original draft. ZZ: Data curation, Formal analysis, Investigation, Validation, Visualization, Writing–original draft. XW: Data curation, Resources, Writing–original draft. JL: Investigation, Resources, Writing–review and editing. JZ: Investigation, Validation, Writing–original draft. LC: Conceptualization, Methodology, Writing–review and editing. HY: Project administration, Resources, Supervision, Writing–review and editing. HD: Conceptualization, Funding acquisition, Project administration, Resources, Supervision, Writing–review and editing.
Funding
The author(s) declare financial support was received for the research, authorship, and/or publication of the article. This study was supported by the Science Foundation of Jiangsu Province Health Department (ZDB2020014); the Science Foundation of Suzhou Health Department (LCZX202106); the Science and Technology Program of Suzhou (SLJ2022003, 2022SS41); the Discipline Construction Program of the Second Affiliated Hospital of Soochow University (XKTJ-TD202001) and the Natural Science Fund of China (82002204).
Conflict of interest
The authors declare that the research was conducted in the absence of any commercial or financial relationships that could be construed as a potential conflict of interest.
Publisher’s note
All claims expressed in this article are solely those of the authors and do not necessarily represent those of their affiliated organizations, or those of the publisher, the editors and the reviewers. Any product that may be evaluated in this article, or claim that may be made by its manufacturer, is not guaranteed or endorsed by the publisher.
Supplementary material
The Supplementary Material for this article can be found online at: https://www.frontiersin.org/articles/10.3389/fmicb.2024.1404996/full#supplementary-material
Supplementary Figure 1 | Schematic diagram of hospitalization of patients. Blue lines represent admission date of patients; green lines represent discharge date of patients; and red lines represent collection date of Enterobacter chengduensis strains.
Supplementary Figure 2 | Growth curves of J53 and its transformant J53/pHD5030-NDM. Optical densities of E. coli J53 and its transformant J53/pHD5030-NDM in LB without antibiotic.
Footnotes
References
Acman, M., Wang, R., Van Dorp, L., Shaw, L. P., Wang, Q., Luhmann, N., et al. (2022). Role of mobile genetic elements in the global dissemination of the carbapenem resistance gene blaNDM. Nat. Commun. 13, 1131. doi: 10.1038/s41467-022-28819-2
Alcock, B. P., Raphenya, A. R., Lau, T. T. Y., Tsang, K. K., Bouchard, M., Edalatmand, A., et al. (2020). CARD 2020: antibiotic resistome surveillance with the comprehensive antibiotic resistance database. Nucleic Acids Res. 48, D517–D525.
Ambrose, S. J., Harmer, C. J., and Hall, R. M. (2018). Evolution and typing of IncC plasmids contributing to antibiotic resistance in Gram-negative bacteria. Plasmid 99, 40–55. doi: 10.1016/j.plasmid.2018.08.001
Bortolaia, V., Kaas, R. S., Ruppe, E., Roberts, M. C., Schwarz, S., Cattoir, V., et al. (2020). ResFinder 4.0 for predictions of phenotypes from genotypes. J. Antimicrob. Chemother. 75, 3491–3500.
Brettin, T., Davis, J. J., Disz, T., Edwards, R. A., Gerdes, S., Olsen, G. J., et al. (2015). RASTtk: a modular and extensible implementation of the RAST algorithm for building custom annotation pipelines and annotating batches of genomes. Sci. Rep. 5:8365. doi: 10.1038/srep08365
Carattoli, A., Zankari, E., García-Fernández, A., Voldby Larsen, M., Lund, O., Villa, L., et al. (2014). In silico detection and typing of plasmids using PlasmidFinder and plasmid multilocus sequence typing. Antimicrob. Agents Chemother. 58, 3895–3903.
Davin-Regli, A., Lavigne, J. P., and Pagès, J. M. (2019). Enterobacter spp.: Update on taxonomy, clinical aspects, and emerging antimicrobial resistance. Clin. Microbiol. Rev. 32, e00002–e19. doi: 10.1128/CMR.00002-19
Delcher, A. L., Salzberg, S. L., and Phillippy, A. M. (2003). Using MUMmer to identify similar regions in large sequence sets. Curr. Protoc. Bioinformatics 10, 11–18. doi: 10.1002/0471250953.bi1003s00
Feng, Y., Hu, Y., and Zong, Z. (2021). Reexamining the Association of AmpC Variants with Enterobacter Species in the Context of Updated Taxonomy. Antimicrob. Agents Chemother. 65, e0159621. doi: 10.1128/AAC.01596-21
Fernandez, J. E., Seth-Smith, H. M. B., Nordmann, P., Egli, A., Endimiani, A., and Perreten, V. (2022). Intra- and interspecies spread of a novel conjugative multidrug resistance IncC Plasmid Coharboring blaOXA–181 and armA in a Cystic Fibrosis Patient. Microbiol. Spectr. 10, e0312122. doi: 10.1128/spectrum.03121-22
Hirabayashi, A., Dao, T. D., Takemura, T., Hasebe, F., Trang, L. T., Thanh, N. H., et al. (2021). A Transferable IncC-IncX3 Hybrid Plasmid Cocarrying blaNDM–4, tet(X), and tmexCD3-toprJ3 Confers Resistance to Carbapenem and Tigecycline. mSphere 6:e0059221. doi: 10.1128/mSphere.00592-21
Jolley, K. A., Bray, J. E., and Maiden, M. C. J. (2018). Open-access bacterial population genomics: BIGSdb software, the PubMLST.org website and their applications. Wellcome Open Res. 3:124. doi: 10.12688/wellcomeopenres.14826.1
Kumar, S., Stecher, G., Li, M., Knyaz, C., and Tamura, K. (2018). MEGA X: molecular evolutionary genetics analysis across computing platforms. Mol. Biol. Evol. 35, 1547–1549. doi: 10.1093/molbev/msy096
Lee, I., Ouk Kim, Y., Park, S. C., and Chun, J. (2016). OrthoANI: An improved algorithm and software for calculating average nucleotide identity. Int. J. Syst. Evol. Microbiol. 66, 1100–1103.
Meier-Kolthoff, J. P., Auch, A. F., Klenk, H. P., and Göker, M. (2013). Genome sequence-based species delimitation with confidence intervals and improved distance functions. BMC Bioinformatics 14:60. doi: 10.1186/1471-2105-14-60
Meier-Kolthoff, J. P., Carbasse, J. S., Peinado-Olarte, R. L., and Göker, M. (2022). TYGS and LPSN: a database tandem for fast and reliable genome-based classification and nomenclature of prokaryotes. Nucleic Acids Res. 50, D801–D807. doi: 10.1093/nar/gkab902
Mezzatesta, M. L., Gona, F., and Stefani, S. (2012). Enterobacter cloacae complex: clinical impact and emerging antibiotic resistance. Future Microbiol. 7, 887–902. doi: 10.2217/fmb.12.61
Moura, A., Soares, M., Pereira, C., Leitão, N., Henriques, I., and Correia, A. (2009). INTEGRALL: a database and search engine for integrons, integrases and gene cassettes. Bioinformatics 25, 1096–1098. doi: 10.1093/bioinformatics/btp105
Nordmann, P., and Poirel, L. (2019). Epidemiology and Diagnostics of Carbapenem Resistance in Gram-negative Bacteria. Clin. Infect. Dis. 69, S521–S528.
Nordmann, P., Poirel, L., Carrër, A., Toleman, M. A., and Walsh, T. R. (2011). How to detect NDM-1 producers. J. Clin. Microbiol. 49, 718–721.
Poirel, L., Bonnin, R. A., Boulanger, A., Schrenzel, J., Kaase, M., and Nordmann, P. (2012). Tn125-related acquisition of blaNDM-like genes in Acinetobacter baumannii. Antimicrob. Agents Chemother. 56, 1087–1089.
Rosselló-Móra, R., and Amann, R. (2015). Past and future species definitions for Bacteria and Archaea. Syst. Appl. Microbiol. 38, 209–216. doi: 10.1016/j.syapm.2015.02.001
Siguier, P., Perochon, J., Lestrade, L., Mahillon, J., and Chandler, M. (2006). ISfinder: the reference centre for bacterial insertion sequences. Nucleic Acids Res. 34, D32–D36.
Wang, P., Jiang, X., Mu, K., Jing, Y., Yin, Z., Cui, Y., et al. (2022). DANMEL: A manually curated reference database for analyzing mobile genetic elements associated with bacterial drug resistance. mLife 1, 460–464. doi: 10.1002/mlf2.12046
Wu, W., Feng, Y., and Zong, Z. (2019). Characterization of a strain representing a new Enterobacter species, Enterobacter chengduensis sp. nov. Antonie Van Leeuwenhoek 112, 491–500. doi: 10.1007/s10482-018-1180-z
Wu, W., Wei, L., Feng, Y., Xie, Y., and Zong, Z. (2021). Precise Species Identification by Whole-Genome Sequencing of Enterobacter Bloodstream Infection, China. Emerg. Infect. Diseases 27, 161–169. doi: 10.3201/eid2701.190154
Zhang, Y., Wang, Q., Yin, Y., Chen, H., Jin, L., Gu, B., et al. (2018). Epidemiology of Carbapenem-Resistant Enterobacteriaceae Infections: Report from the China CRE Network. Antimicrob. Agents Chemother. 62, e1882–e1817. doi: 10.1128/AAC.01882-17
Zheng, Z., Cheng, Q., Chan, E. W., and Chen, S. (2020). Genetic and Biochemical Characterization of VMB-1, a Novel Metallo-β-Lactamase Encoded by a Conjugative, Broad-Host Range IncC Plasmid from Vibrio spp. Adv. Biosyst. 4, e1900221.
Zhu, Z., Xie, X., Yu, H., Jia, W., Shan, B., Huang, B., et al. (2022). Epidemiological characteristics and molecular features of carbapenem-resistant Enterobacter strains in China: A multicenter genomic study. Emerg. Microb. Infect. 1, 228. doi: 10.1080/22221751.2022.2148562
Keywords: Enterobacter chengduensis, carbapenemase, blaNDM–1, ST414, IncC
Citation: Fu H, Zhu Z, Wang X, Lv J, Zhu J, Chen L, Yu H and Du H (2024) Emergence of blaNDM–1-carrying Enterobacter chengduensis in China. Front. Microbiol. 15:1404996. doi: 10.3389/fmicb.2024.1404996
Received: 22 March 2024; Accepted: 31 July 2024;
Published: 14 August 2024.
Edited by:
Tomas Vinar, Comenius University, SlovakiaReviewed by:
Sangita Dixit, Siksha O Anusandhan University, IndiaYujie Hu, China National Center for Food Safety Risk Assessment, China
Adam Valcek, Vrije University Brussel, Belgium
Copyright © 2024 Fu, Zhu, Wang, Lv, Zhu, Chen, Yu and Du. This is an open-access article distributed under the terms of the Creative Commons Attribution License (CC BY). The use, distribution or reproduction in other forums is permitted, provided the original author(s) and the copyright owner(s) are credited and that the original publication in this journal is cited, in accordance with accepted academic practice. No use, distribution or reproduction is permitted which does not comply with these terms.
*Correspondence: Hong Du, aG9uZ19kdUAxMjYuY29t
†These authors have contributed equally to this work