- 1Department of Applied Animal Science and Welfare, Swedish University of Agricultural Sciences, Uppsala, Sweden
- 2Department of Marine Sciences, Tjärnö, University of Gothenburg, Strömstad, Sweden
- 3Zoonosis Science Center, Uppsala University, Uppsala, Sweden
- 4Zoonosis Science Center, Department of Medical Sciences, Uppsala University, Uppsala, Sweden
Introduction: Campylobacter jejuni gastroenteritis is the most commonly reported zoonosis within the EU, with poultry products regarded as the primary source of transmission to humans. Therefore, finding strategies to reduce Campylobacter colonization in broilers holds importance for public health. Recent studies suggest that supplementation of broiler feed with brown algal extracts, particularly laminarin, can provide beneficial effects on broiler gut health, growth performance, and gut microbiota. However, its effect on gut microbiota development and subsequent reduction of Campylobacter loads in broiler caeca during the later stages of the birds' lives remains unclear.
Methods: Experimental colonization of Ross 308 broilers with two different strains of C. jejuni was conducted, with groups fed either a basal diet or the same basal diet supplemented with 725 ppm algal extract from Saccharina latissima to provide 290 ppm laminarin. Fecal samples were collected for bacterial enumeration, and caecal samples were obtained before and after the C. jejuni challenge for the determination of microbiota development.
Results and discussion: No significant differences in fecal C. jejuni concentrations between the groups fed different diets or exposed to different C. jejuni strains were observed. This suggests that both strains colonized the birds equally well and that the laminarin rich algal extract did not have any inhibitory effect on C. jejuni colonization. Notably, 16S rRNA amplicon sequencing revealed detailed data on the caecal microbiota development, likely influenced by both bird age and C. jejuni colonization, which can be valuable for further development of broiler feed formulations aimed at promoting gut health.
1 Introduction
Campylobacter jejuni (C. jejuni) is a common gram-negative bacterial pathogen associated with foodborne illnesses in humans, and broiler chickens are known to be a significant reservoir for this bacterium (EFSA, 2020). Finding strategies to reduce Campylobacter colonization in broiler flocks is important for food safety and public health.
Saccharina latissima is a brown algae belonging to the family Laminariaceae and is rich in complex polysaccharides such as alginate, laminarin, and fucoidan (Sharma et al., 2018). It has been proposed that laminarin could have prebiotic potential (Cherry et al., 2019). A study conducted on broiler chicks, where birds were fed a laminarin-rich extract from Laminaria spp. at 300 ppm, found that it exhibited promising effects on growth performance, protective immune responses, and beneficial effects on the gastrointestinal microbial profile (Venardou et al., 2021). Additionally, an increase in the expression of the cytokine interleukin 17A (IL17A) in the broiler's duodenum was observed. Interleukin 17A has been reported to be involved in the immune response against several infectious agents, including Campylobacter (Connerton et al., 2018). Sweeney and O'Doherty (2016) reported that supplementing the basal diet with laminarin at a concentration of 250 ppm during the initial stages of the chickens' life led to notable improvements in villus morphology and enhanced tight junction integrity in Ross 308 broilers. However, the same study reported no significant differences in C. jejuni colonization during experimental colonization (day three post-hatch to day 13) between broilers raised on an algal extract-supplemented diet and those raised on a conventional diet in the post-hatch period. Yet, the investigation of algal extracts' impact on the microbiota and C. jejuni bacterial load in broiler caeca during later stages of life remains unexplored in current scientific literature.
Although the epizootology of C. jejuni and its spread into broiler production is still not fully understood, it is well-known that wild birds constitute a natural reservoir for this pathogen (French et al., 2009; Waldenström et al., 2010; Griekspoor et al., 2013; Mourkas et al., 2022). Therefore, it is also likely that they play a role in the transmission of C. jejuni between wild animals, to domestic production, and further to humans. In wild birds, C. jejuni shows strong host specificity such that different bird taxa carry different genotypes of C. jejuni (Griekspoor et al., 2013). This specificity does not seem to correlate with the diet or habitat of the birds but rather with specific species associated microbiota. In a previous experiment, the relative colonization ability in mallard ducks (Anas platyrhynchos) was compared between C. jejuni strains of different host origins (Atterby et al., 2018). Not surprisingly, C. jejuni of mallard origin colonized the birds best. However, a strain from a song thrush (Turdus philomelos) was a very poor colonizer and could not establish long-term colonization in this species, whereas C. jejuni isolated from a broiler chicken could colonize the mallards. A similar experiment was previously performed on wild robins (Erithacus rubecula), where a song thrush strain of the same ST-type could colonize the birds for an average of 6.8 days whereas a strain isolated from a human could not establish colonization (Waldenström et al., 2010). These studies suggest that wild bird species might exhibit colonization resistance to C. jejuni genotypes that originate from distantly related hosts. On the other hand, there also exist generalist genotypes of C. jejuni, that can be found in many different host species (Gripp et al., 2011), and hence, colonization resistance does not seem to apply to such strains. Broiler chickens are known to harbor many different genotypes of C. jejuni and it is not known if this host species also shows colonization resistance to C. jejuni adapted to distantly related hosts.
The present study aimed to examine the impact of feed supplementation with a laminarin-rich algal extract from Saccharina latissima (AE) on the development of the caecal microbiota in broilers and assess its influence on resistance to intestinal colonization with C. jejuni upon experimental colonization. Groups of Ross 308 chickens were intraoesophagically inoculated at 17 days of age with two different C. jejuni strains, one of chicken origin and one isolated from a wild song thrush. Based on previous experiments, our hypothesis was that the C. jejuni strain isolated from chicken would exhibit superior colonization ability in broilers compared to the strain originating from song thrush and that any effect of the feed supplementation with AE would be more pronounced against the song thrush strain than the chicken strain.
2 Materials and methods
This study was conducted as part of a larger project where the effect of feeding brown algae (intact or as an AE) to broiler breeder hens was evaluated. Previously, the effect on hens' antibody response, egg quality, and the chick quality of their progeny was assessed (Ivarsson et al., 2023). In addition, the early development of hens' progenies with a main focus on early growth performance was examined (Supplementary Table 1). These progenies were subsequently utilized in the present study. The experiment was carried out at the Swedish Livestock Research Center of the Swedish University of Agricultural Sciences and was approved by the committee for animal ethics of the Uppsala region (approval number 5.8.18-10572/2019).
2.1 Housing
A total of 255 mixed-sex Ross 308 chicks were allocated into groups of 10-13 chickens in 24 experimental pens, the allocation of chicks to the rearing pens was determined prior to the hatching. The solid floor pens with dimensions 1.5 × 0.75 meters were bedded with wood shavings and equipped with feeders and nipple drinkers. The house temperature was initially set at 34°C during hatching (day 0) and then reduced to 33°C for the first 3 days. Thereafter, the temperature gradually decreased with age until reaching 23°C at day 24, remaining the same for the rest of the trial period. Lighting conditions consisted of a 24-h cycle during the first 2 days, after which the duration of light was reduced by 1 h daily until day eight, resulting in 18 h of light per day for the remaining period. All chickens were marked with color identification at hatch, which was replaced by a 1 cm × 1 cm laminated neck tag (Jolly Fine, Jolly, Italy) at 7 days of age. Two chickens from each replicate were used for the collection of fecal samples.
2.2 Experimental design and diets
The chicken facility with 24 experimental pens in total was divided into two parts, each consisting of 12 pens. Each part was split into two chick treatment groups: (1) basal diet formulated according to the nutrient requirement of Ross 308 (control - C), and (2) basal diet supplemented with 725 ppm algal extract (AE) sourced from Saccharina latissima. The supplemented diet was optimized to contain 290 ppm laminarin and was provided from the first day of the animal's life until the end of the trial. Both chick diets were pelleted and formulated according to the nutrient requirement of Ross 308 (Aviagen., 2019). The algae used in the study were cultivated at sea on longlines in the Koster archipelago, located outside Tjärnö Marine Biological Laboratory on the Swedish West Coast (Thomas et al., 2022). In the experiment, dried algae were utilized as a substrate for the AE, with the detailed processing procedures described in Ivarsson et al. (2023). The resulting AE contained 41.6 % laminarin, analyzed enzymatically by measuring the β 1,3/1,6-glucan content using the K-YBGL 12/16 assay kit from Megazyme. Additionally, the ash content of the AE was determined to be 14.5% on a dry matter (DM) basis. The non-starch polysaccharide content was analyzed by the Uppsala method (Theander et al., 1995), and amounted to a total of 45.7% on a DM basis. The specific composition of the polysaccharides was as follows: 31% glucose, 2.2% fucose, 3.0% mannose, 1.8% galactose, 0.7% xylose, and 0.5% arabinose.
2.3 Campylobacter jejuni inoculation
At 17 days of age, the bird group size was reduced to four chickens per pen, and chicks were intraoesophagically inoculated with 1 ml of a PBS solution containing ~102 CFU of C. jejuni as determined by optical density at 405 nm. Two different C. jejuni strains were used and birds inoculated with each of the strains were kept apart in two different parts of the facility, separated by a flexible wall. C. jejuni strain #65 (ST-104, in ST-21 CC) was isolated from a broiler chicken in the UK and C. jejuni strain #3926 (ST-1315 in ST-1304 CCs) was isolated from a wild song thrush captured in Sweden. The bacterial concentration of the two inoculates was confirmed by CFU counts on blood agar plates after 24 h incubation at 42°C in a microaerobic atmosphere to be 2 x 102 cfu/ml for C. jejuni strain #65 and 4 x 102 cfu/ml for strain #3926.
2.4 Fecal and caecal samples collection
Fecal samples were taken from all birds 1 day before the inoculation, to ensure that the birds were culture-negative for Campylobacter before the challenge. Subsequently, samples were collected regularly throughout the experimental period at 1 day post-infection (dpi), 2 dpi, 3 dpi, 5 dpi, 7 dpi, 14 dpi, and 19 dpi for bacterial enumeration by culture on agar plates. Focal birds from each pen were placed individually in clean boxes and sterile cotton swabs were used to collect ~100 mg of fresh fecal matter per bird. Thereafter, samples were re-suspended in 1 ml Luria-Bertani (LB) medium complemented with 20% glycerol, followed by vortexing and centrifugation (100 × g for 15 s) for sedimentation of the fecal matter. Subsequently, six 20 μl aliquots were withdrawn from each sample and added to the first column of a 96-well plate containing 180 μl of PBS per well. The six replicates were serially diluted 10-fold and six dilution steps were plated in aliquots of 10 μl on modified charcoal cefoperazone deoxycholate agar (mCCDA) plates using an 8-channel pipette according to the 6 × 6 drop plate method described by Chen et al. (2003). Plates were left to dry for 10 min and incubated for 30 h at 42°C under microaerobic conditions (Campygen, Thermo Fisher, USA). After incubation, colonies were counted and a mean value was created from the six spots from the column that contained the highest number of well separated colonies. Results are presented for four treatments; chicken strain with control feed (Ch + C), chicken strain with algal extract (Ch + AE), song thrush strain with control feed (Th + C), and song thrush strain with algal extract (Th + AE).
Caecal samples were collected from two birds per pen at day 7, day 14, and day 37 of the experiment. Euthanasia was performed by administration of sodium pentobarbital intravenously through the wing vein. An aseptic procedure was followed to obtain caecal content, which was sampled into 2.0 ml screw cap microtubes (Sarstedt AG and Co, Germany) and immediately placed in liquid nitrogen. All samples were stored at−80°C until further analysis. The microbial composition of the caecal content was investigated by 16S rRNA amplicon sequencing.
2.5 DNA extraction, sequencing, and bioinformatics analysis
The DNA extraction of caecal digesta samples was conducted as previously described (Ivarsson et al., 2023). In brief, 400 microliters of ASL lysis buffer (Qiagen, Germany) were added to the thawed sample and homogenized. Thereafter, 120 μl suspension was used for bead beating on Precellys evolution homogenizer (Bertin Technologies SAS, France) at 8000 rpm for 2 × 60 sec with 30-s pauses. After centrifugation, 120 μl of the supernatant was used for DNA extraction using the EZ1 Advanced XL instrument (Qiagen, Germany) according to the manufacturer's instructions.
The caeca sample DNA extractions were sequenced at Novogene (Cambridge, UK), using the Illumina NovaSeq 6000 PE250 platform. The primer set targeting the V4 region of 16S rRNA gene 515F (GTGCCAGCMGCCGCGGTAA) and 806R (GGACTACHVGGGTWTCTAAT) were used for amplification. PCR reactions were performed with Phusion High-Fidelity PCR Master Mix (New England Biolabs, USA). PCR products (~400–450 bp) were separated by electrophoresis on 2% agarose gel, purified with a Qiagen Gel Extraction Kit (Qiagen, Germany), and pooled at equal concentrations. Sequencing libraries were generated using the NEBNext Ultra DNA Library Prep Kit (Illumina, USA), followed by quantification using Qubit (Thermo Fisher Scientific, USA) and sequencing.
The 16S rRNA gene sequencing data processing was performed as described in Sun et al. (2022), with the following modification: (1) using the truncation length of 221 bp for both forward and reverse reads; (2) the SILVA SSU Ref NR 99 138 dataset was used for taxonomic classification (Pedregosa et al., 2011); (3) the generalized UniFrac distance matrix (alpha = 0.5) was generated using the QIIME2 diversity plugin (Bolyen et al., 2019).
2.6 Statistical analysis
All statistical analysis was performed with R (R Core Team, 2019). To investigate the effect of dietary treatment and Campylobacter strain, Quasi-Poisson regression (Ver Hoef and Boveng, 2007) was employed to analyse the plate counting data for C. jejuni, while Tukey's Honestly Significant Difference (HSD) test was utilized to conduct multiple pairwise comparisons. For sequencing data, the number of observed ASVs was calculated from rarefied ASV table. The Kruskal-Wallis rank test, followed by Dunn's test for pairwise comparisons with Benjamini and Hochberg (B–H) correction, was used to check for statistically significant differences in observed ASVs between treatment groups, using the QIIME2 q2-diversity plugin (Kruskal, 1952; Dunn, 1964; Benjamini and Hochberg, 1995). Permutational multivariate analysis of variance (PERMANOVA) of generalized UniFrac distance matrix with B-H correction was conducted to evaluate differences between the dietary and Campylobacter treatment groups, using the q2-diversity plugin (Anderson, 2001). Mixed effects linear models were fitted and analyzed using R packages lme4 (Bates et al., 2015), lmerTest (Kuznetsova et al., 2017), pbkrtest (Halekoh and Højsgaard, 2014), and emmeans (Searle et al., 1980). Age, feed treatment, and Campylobacter strain were used as fixed effects, and pen as a random effect. P < 0.05 was considered significant.
3 Results
3.1 Campylobacter colonization not influenced by AE
All birds were Campylobacter jejuni negative prior to the challenge and successful C. jejuni colonization in both strains was achieved after the challenge at 1 dpi (18 days of age). There was no significant effect of feed supplementation with AE on the colonization dynamics of either of the C. jejuni strains, as determined by CFU counts on agar plates (Figure 1). The levels of C. jejuni in birds that received AE in their diet (Ch + AE and Th + AE) were similar to C. jejuni levels in groups fed with the C diet (Ch + C and Th + C) at all sampling points. No significant differences were observed between the chicken and song thrush C. jejuni strains in their ability to colonize the broilers' gut, and similar colonization levels were observed from dpi 1 to dpi 19 in both C and AE-fed birds. C. jejuni colonization progressed rapidly from dpi 1 to dpi 2, and the peak was observed 3 days after the challenge (3 dpi) with ~107 CFU/g of feces. Thereafter, the colonization intensity for both strains remained at similar levels of 106 CFU/g of feces on average until the end of the experiment.
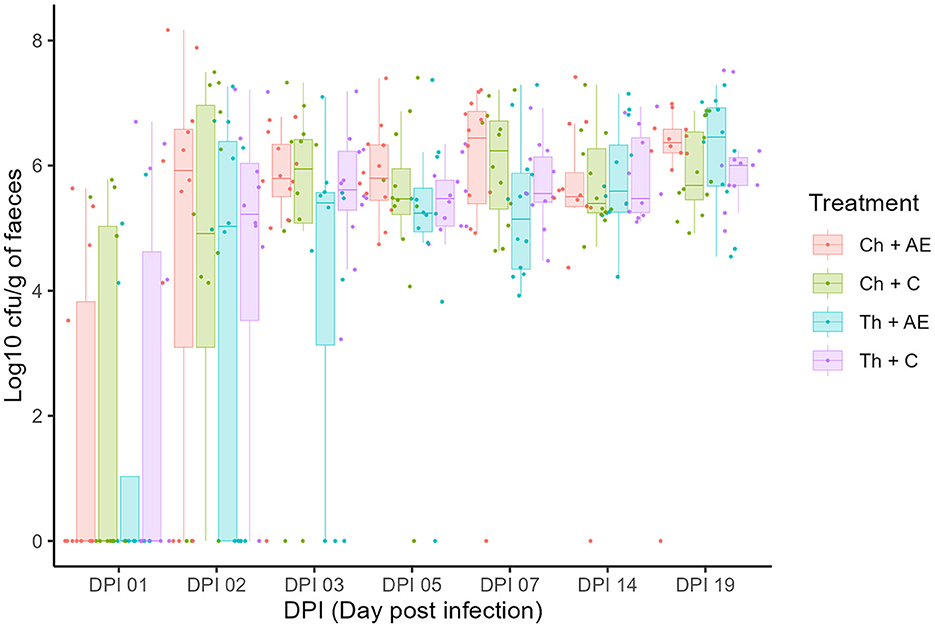
Figure 1. Colony forming unit counts of Campylobacter jejuni colonization in fecal samples at different dpi (days post-infection). Dots (data points) represent 10-log (CFU/ml) in individual fecal samples based on mean values of six replicate dots per plate at a given sampling point. Ch, chicken strain of C. jejuni; Th, song thrush strain of C. jejuni; C, control; AE, algal extract. Red = Ch + AE, Green = Ch + C, Blue = Th + AE, Purple = Th + C.
3.2 Caecal microbiota composition shifts
Rarefaction curves of observed amplicon sequencing variants (ASVs) in caecal samples revealed sufficient sequencing depth to capture species richness at days 7, 14, and 37 after hatching (Supplementary Figure 1). The alpha diversity, as measured by the observed number of ASVs had the lowest count at day 7, followed by day 14, and the highest number of ASVs was present at day 37.
Principal coordinate analysis (PCoA) was used to display differences in the generalized UniFrac distance matrix at different sampling points after hatching (day 7, day 14, and day 37). The results demonstrate a distinct ASV clustering according to time after hatching (Kruskal-Wallis, p < 0.001) (Figure 2). The differences between sampling points day 7 and day 14 indicate an effect of age, whereas the microbiota composition at day 37 could be affected by age, cage effect or C. jejuni colonization (PERMANOVA, p = 0.001). No clear separation between groups fed with AE supplemented diet in comparison to the C groups was observed, indicating that the caecal microbiota composition was not significantly affected by supplementation with AE.
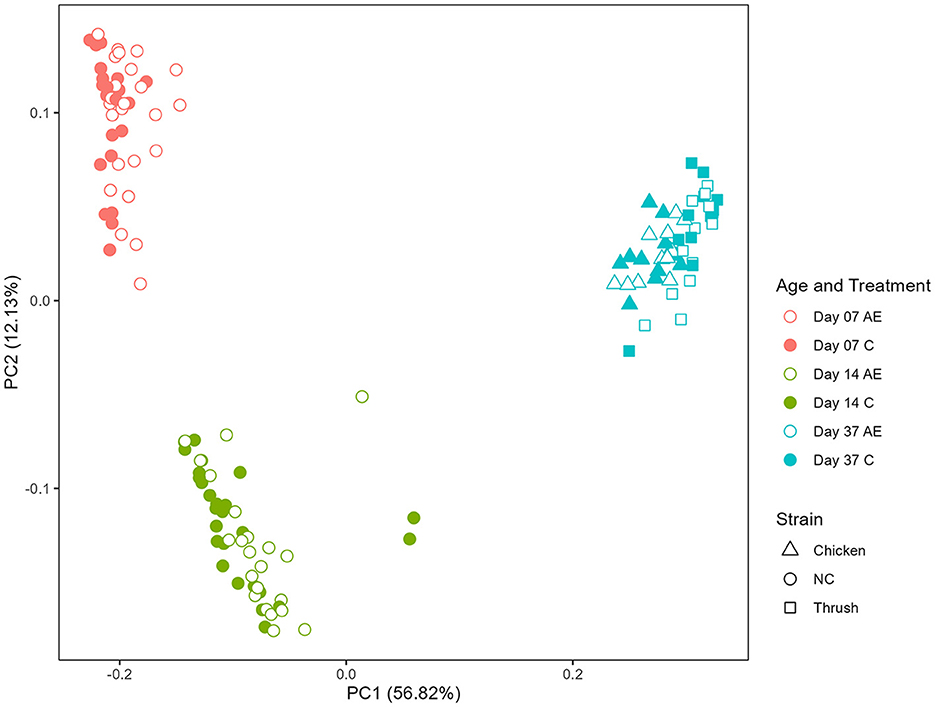
Figure 2. Principal coordinate analysis (PCoA) plot showing differences in generalized UniFrac distance matrix at different sampling points after hatching (day 7, day 14, and day 37). AE, algal extract; C, control; NC, non-challenged.
The relative abundance (RA) of the top 30 most prevalent ASVs in the caeca (Figure 3) accounted for ~80% of the total sequencing reads at day 7 and day 14. At day 37, this figure had dropped down to ~50%, concurrent with a substantial increase in the number of low abundant ASVs in the minor group; from 89 on day 14 to 285 and 297 on day 37 for the group infected with the thrush strain or the chicken strain, respectively. This minor group describes the group of ASVs, with each having an abundance lower than 0.55%. Since the caecal microbiota development was not significantly affected by supplementation with AE, the treatments were pooled for further analysis. In general, there was considerable individual variation in the caecal microbiota among the birds observed.
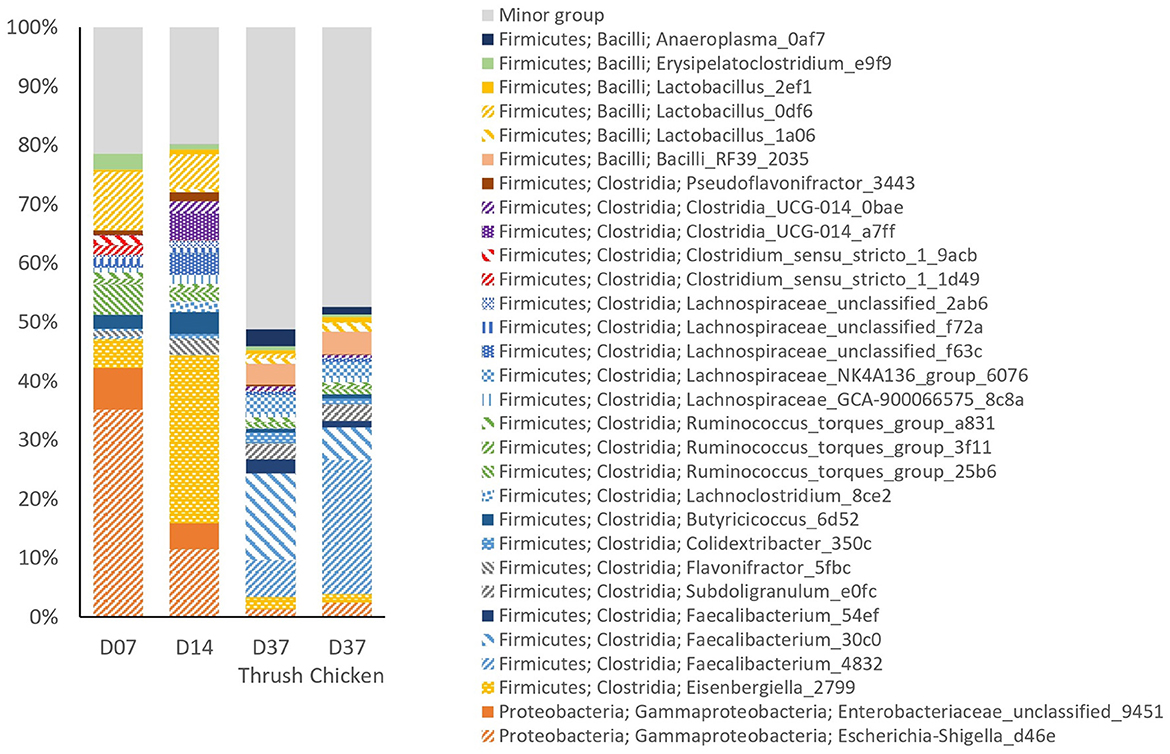
Figure 3. The relative abundance (%) of the top 30 most abundant ASVs in caecal samples at different ages (day 7, day 14, and day 37) and Campylobacter strains (song thrush and chicken).
Examination of taxonomic composition revealed shifts in the caecal microbiota composition between the sampling points. Comparison of pre-C. jejuni challenge samples from day 7 and day 14 showed a considerable decrease in the RA of ASV Escherichia-Shigella_d46e at day 14, while Eisenbergiella_2799 showed a pronounced increase. At day 37 (post-challenge samples), the RA of both Escherichia-Shigella_d46e and Eisenbergiella_2799 had decreased to low percentages. In contrast, Faecalibacterium_4832 and Faecalibacterium_30c0 emerged as the dominant ASVs at day 37, with a minor representation at days 7 and 14. Unclassified Enterobacteriaceae_9451 showed the highest RA at day 7, with a decrease at day 14, and minimal presence at day 37. ASVs Clostridium_sensu_stricto_1_1d49 and Clostridium_sensu_stricto_1_9acb were present at day 7 and thereafter their RA was minimal. Conversely, ASVs Clostridia_UCG-014_a7ff and Clostridia_UCG-014_0bae were the most abundant at day 14 and diminished at day 37. The most abundant ASV classified as Lactobacillus was Lactobacillus_0df6, which had the highest RA at day 7, decreased by day 14, and was minimally present at day 37. Between day 14 and day 37, there was a distinct increase in the abundance of Faecalibacterium_54ef, Bacilli_RF39_2035, and Anaeroplasma_0af7. These ASVs were not present among the top 30 most abundant ASVs at day 7. The ASV Subdoligranulum_e0fc exhibited minor presence at days 7 and 14, with a considerable increase in its RA at day 37. A reduction in the RA of Butyricicoccus_6d52 was noted at day 37 in both groups (chicken and thrush strain), in contrast to its moderate abundance at days 7 and 14.
A PCoA plot of caecal samples obtained at day 37 after hatching revealed a significant clustering according to the C. jejuni strain used for the challenge (PERMANOVA, p = 0.001) (Figure 4). No effect of diet was observed at day 37.
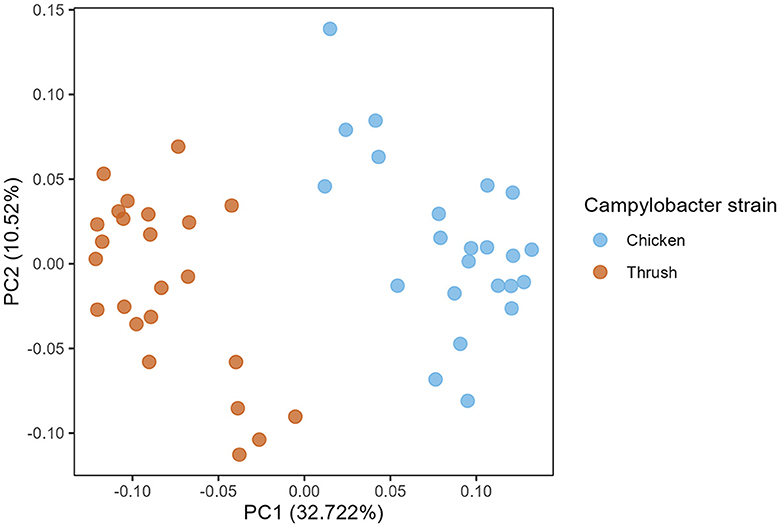
Figure 4. Principal coordinate analysis (PCoA) plot showing differences in generalized UniFrac distance matrix between different Campylobacter strains (chicken and song thrush origin) at age day 37.
At day 37, a significant difference (p < 0.05) in the RA of several ASVs was detected in caecal samples obtained from broilers challenged with the two different C. jejuni strains. The most abundant ASV within the group challenged with the song thrush strain was Faecalibacterium_30c0, exhibiting a significantly higher RA compared to the chicken strain samples (Figure 5). In contrast, the dominant ASV found in the samples from birds challenged with the chicken strain was Faecalibacterium_4832. Furthermore, a significantly higher abundance of ASVs Anaeroplasma_0af7 and Faecalibacterium_54ef was observed in the thrush strain samples than in the chicken strain samples (Figure 5). We cannot exclude that these differences were due to environmental factors as the birds challenged with the two different strains were caged in different parts of the stable, separated by a flexible wall.
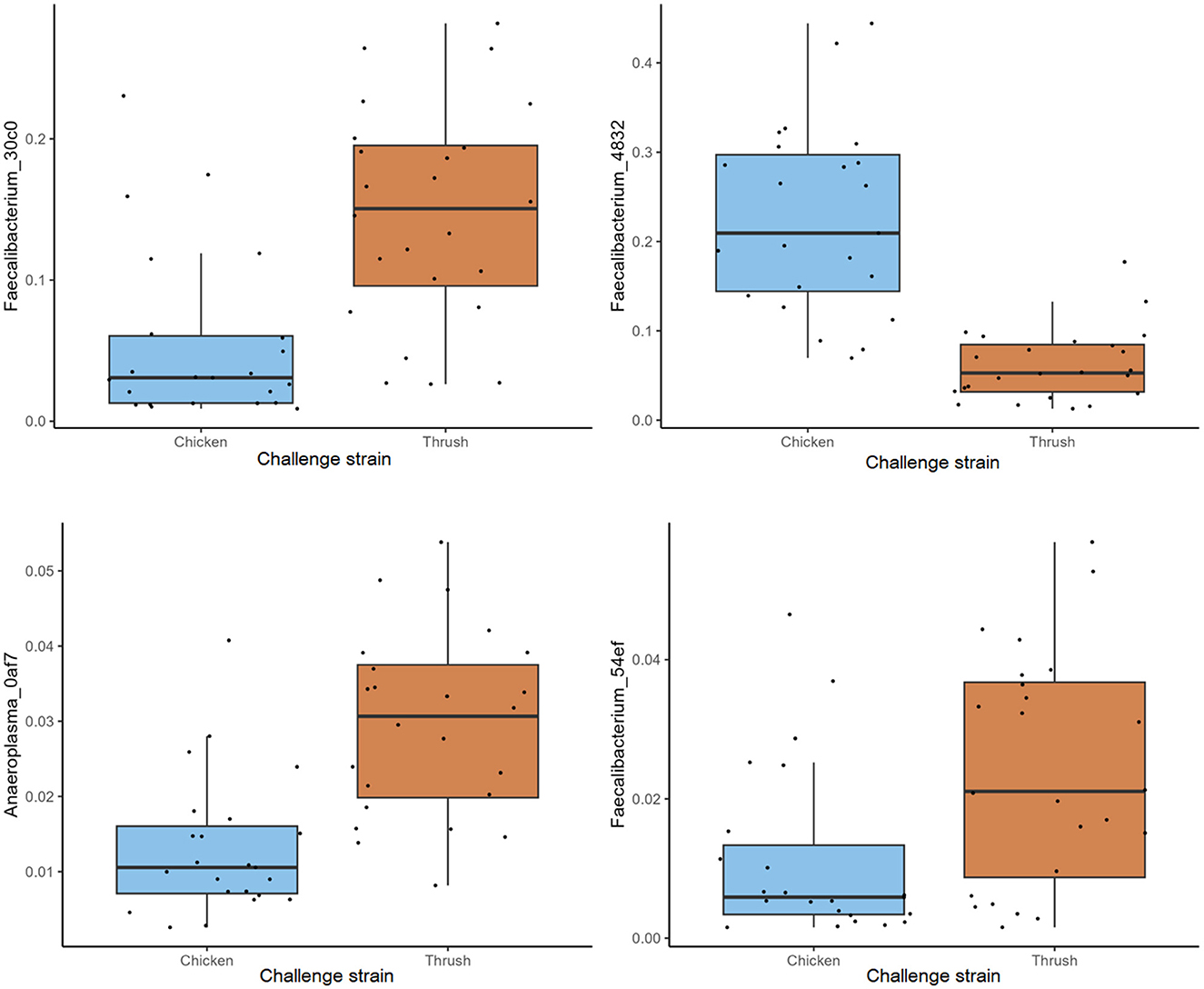
Figure 5. The relative abundance (%) of ASVs in caecal samples at day 37 with a significant difference (p < 0.05).
4 Discussion
Identifying ways to reduce Campylobacter jejuni colonization in broilers' gut is desirable to minimize the risk of meat contamination during the slaughter process and thereby enhance the safety of poultry products for human consumption. Feed supplementation with prebiotics and probiotics may be an important component of the complex solution to this challenge.
Our study's results revealed that supplementation with 290 ppm laminarin had no significant effect on the colonization dynamics of two distinct strains of C. jejuni: the chicken origin strain #65 and the song thrush origin strain #3926. No significant differences in bacterial loads could be observed between the groups that received laminarin supplementation compared to the control groups at any time point during the study (Figure 1). These findings are in line with an earlier study by Sweeney and O'Doherty (2016), where the addition of purified laminarin extract (250 ppm) to the diet of broilers showed no significant impact on the suppression of C. jejuni during the initial post-hatch period. Taken together, this suggests that laminarin-rich algal extracts have a limited direct antimicrobial effect on reducing C. jejuni colonization in broiler caeca.
The chicken and song thrush origin C. jejuni strains exhibited comparable colonization dynamics during the whole experiment (Figure 1), indicating that the Ross 308 hybrid used in this study displayed similar susceptibility to colonization by both C. jejuni strains. This observation was in contrast to the study by Atterby et al. (2018) where dramatic differences in colonization patterns were observed in mallard ducks inoculated with either the chicken strain #65 or the song thrush strain #3926, both used in this study. In wild birds, C. jejuni shows strong host association that might be due to colonization resistance to specific C. jejuni genotypes (Griekspoor et al., 2013; Atterby et al., 2018). Our findings suggest that such colonization resistance against specific C. jejuni genotypes might be weaker in broiler chickens. However, further investigation utilizing a broader range of C. jejuni strains from diverse bird host origins is necessary to verify this. The fast establishment and continuous persistence of colonization observed in both strains highlight the efficiency with which C. jejuni establishes itself in the broilers' caeca. Colonization levels remained high from 3 dpi until the end of the experiment. These observations are in agreement with the results reported by Connerton et al. (2018), who documented the onset of C. jejuni colonization in broilers infected at 20 days of age within 2 days of exposure, with colonization persistence until the end of the study at day 35.
The caeca are known to be the most bacteria-dense part of the broiler's gastrointestinal tract and an important site for carbohydrate fermentation contributing to the birds' intestinal health and nutrition (Waite and Taylor, 2014). To evaluate the impact of diet supplementation with AE on broiler microbiota development, caecal samples were analyzed by 16S rRNA amplicon sequencing and investigated at the ASV level. Principal Coordinate Analysis (Figure 2) used to visualize the dissimilarities within the generalized UniFrac distance matrix across different sampling points (day 7, day 14, and day 37) did not show any clear clustering of the groups that received feed supplemented with AE compared to the control groups. This suggests that the supplementation did not significantly influence the composition of the caecal microbiota in the current experiment. Yet, Venardou et al. (2021) found that supplementing the basal diet with 300 ppm of laminarin for 35 days led to an increase in both the relative and absolute abundance of Bifidobacterium in the caeca of Ross 308 broiler chickens, proposing this dosage to promote a beneficial gastrointestinal microbiota profile. However, it is worth mentioning that in that study broilers were not exposed to experimental Campylobacter challenge and that the influence of dietary supplements can vary based on diverse factors such as host physiological state, application pattern, housing effect, and dosage, which can yield different outcomes in their activity (Marco and Tachon, 2013; Salami et al., 2016). Additionally, pronounced individual variation observed in the caecal microbiota composition among the birds on all sampling points in the present study highlights the complexity of avian gut microbial communities (Stanley et al., 2013).
The age of the birds exerted a significant effect on the microbiota composition, as suggested by the distinct clustering of ASVs from each sampling point, correlating to the age of the birds (Figure 2). Furthermore, the rarefaction curves of observed ASVs revealed a consistent temporal increase in alpha diversity (Supplementary Figure 1). These observations find support in previous studies which have highlighted the age-dependent aspect of caecal microbiota development (Ivarsson et al., 2022; Boyner et al., 2023). Among changes in the gut microbiota solely connected to the age of the birds, a relatively high RA of ASVs Clostridium_sensu_stricto_1 as well as ASVs Escherichia-Shigella_d46e and unclassified Enterobacteriaceae_9451 (belonging to the phylum Proteobacteria), were observed at day 7, while their RA notably decreased at day 14 (Figure 3). Our observation aligns with a study conducted by Kers et al. (2020), suggesting that members of the genus Clostridium sensu stricto are among the first colonizers of the avian caeca, and that after a few days, members of the phylum Proteobacteria colonize the caeca, followed by an increase in Firmicutes later in the broiler's life.
Since the main aim of this study was to investigate if feeding broilers with AE had an effect on their resistance to C. jejuni colonization and if this could be explained by their caecal microbiota composition in contrast to birds fed control feed, we did not include a control group that was not challenged with C. jejuni. Regrettably, this fact renders us unable to draw direct conclusions on the relative effect of C. jejuni colonization vs. that of bird age on the caecal microbiota composition observed at day 37. However, this study provides valuable insights into caecal microbiota development during C. jejuni challenge, focusing on the ASV level rather than the higher taxonomic levels commonly discussed in broiler gut microbiota studies. Interestingly, the challenge with the two different C. jejuni strains at day 17 affected caecal microbiota composition differently by day 37 (Figure 5), suggesting an influence of C. jejuni colonization. ASVs classified as Faecalibacterium were pronounced at day 37, where ASV Faecalibacterium_4832 dominated in birds challenged with the chicken strain, while ASVs Faecalibacterium_30c0 and Faecalibacterium_54ef had significantly higher RA in the birds that received the song thrush strain. These findings are consistent with prior studies by Ocejo et al. (2019) and Thibodeau et al. (2015), who reported a positive association between Faecalibacterium and Campylobacter presence in broiler caeca. Moreover, similarities to our previous research (Valečková et al., 2023) were found, where Faecalibacterium emerged as the predominant genus in Ross 308 caeca 20 days after C. jejuni challenge. Furthermore, Liao et al. (2020) noted that Faecalibacterium dominated the caecal bacterial communities of Campylobacter-free broilers during the grower phase (7-14 days of age), while its prevalence decreased thereafter. In addition, in the current study, significantly higher RA of Anaeroplasma_0af7 was observed in birds that received the song thrush strain. Current reports about Anaeroplasma in broilers caeca in relation to Campylobacter presence are limited. However, Ocejo et al. (2019) identified Anaeroplasma as the main genus of the minor phyla Tenericutes, which was present at stable levels (0.002%−1.5%) throughout the productive period when analyzing the microbiome of chicken caeca in two different breeds (Ross-308 and Sasso-T451A), and management systems throughout their whole productive lifespan. It should be noted that the differences at ASV level seen at day 37 may also be due to environmental effects imposed by the fact that birds challenged with the two different C. jejuni strains were physically separated in the stable by a flexible wall (Kers et al., 2018).
Analyzing the RA of the top 30 most abundant ASVs revealed the pronounced reduction of ASV Escherichia-Shigella_d46e after the C. jejuni challenge. This observation aligns with results from Awad et al. (2016), where 14-day-old Ross 308 broilers were challenged with 1 × 108 CFU of C. jejuni NCTC 12744 and their microbial community composition was followed from day 1 to day 28 of age. The authors found that the presence of C. jejuni was associated with a significant reduction in Escherichia coli abundance compared to the non-challenged control birds. These findings may also be related to the observed decrease in RA of unclassified Enterobacteriaceae_9451 at day 37 in the present study. Furthermore, the RA of ASVs Clostridia UCG-014 decreased considerably post-challenge which aligns with the results from Pang et al. (2023), where a significant negative correlation between Campylobacter abundance and genera Clostridia was reported in caeca of 5 weeks old broilers. Additionally, a considerable increase in the RA of ASVs Subdoligranulum_e0fc and Bacilli_RF39_2035 at day 37, compared to their minimal presence prior to the challenge, along with a converse shift in the RA of Eisenbergiella_2799, Butyricicoccus_6d52, and Lactobacillus_0df6 in response to the challenge, aligns with our previous findings discussing the effect of C. jejuni presence on the broilers caeca microbial composition in Ross 308 (Valečková et al., 2023).
The observed increase in the species richness within the minor ASV group (Supplementary Figure 1) and the increased RA of ASVs within this group at day 37 (Figure 3) was likely attributed to the progressive divergence in the caecal microbiota with age (Zhou et al., 2021). Ocejo et al. (2019) identified age as the strongest factor influencing the composition of caecal microbiota when the core microbiome was compared among Ross 308 broilers raised in a conventional system for 42 days with that of a 'slow-growing breed', Sasso T451A reared in an extensive farming system with outdoor access for 86-days. Interestingly, the same study reported different microbial taxa to be either positively or negatively correlated with C. jejuni RA, suggesting that both bird age and C. jejuni colonization can affect the caecal microbiota composition. Therefore, it can be speculated that the drastic increase in species richness observed on day 37 could have been an effect of the experimental C. jejuni challenge, where the host immune response triggered by C. jejuni colonization might selectively have promoted the profusion and growth of specific ASVs, resulting in an increase in microbial diversity as suggested in a study conducted by Pang et al. (2023). In their analysis of samples collected from five-week-old birds, the researchers observed that farms with Campylobacter-positive broilers had a higher species richness in the caecal microbiota and greater phylogenetic diversity in comparison to Campylobacter-negative farms. As stated above, it is important to note that the interpretation of pre- and post-challenge observations in the current study is obscured by the considerable age difference between the two sampling occasions, specifically 23 days. Further studies are therefore required to better understand changes in caecal microbiota composition related to C. jejuni colonization in general and the presence of different C. jejuni strains in particular.
In conclusion, the present study demonstrates that the supplementation of AE in broiler diets has no significant impact on either C. jejuni colonization after experimental challenge or the development of the caecal microbiota. The results suggest that the overall changes in the gut microbiota observed at different sampling points were mainly age-dependent. Furthermore, the results do not support the initial hypothesis that the chicken origin C. jejuni strain would exhibit better colonization ability in chickens compared to the strain originating from a song thrush. Yet, this study contributes to understanding the complex interactions influencing microbial communities in the broiler gut and provides insights for future interventions aimed at enhancing resistance to Campylobacter colonization in broilers.
Data availability statement
The sequencing data are deposited in NCBI, accession number PRJNA1078836.
Ethics statement
The animal study was approved by the Uppsala Ethics Committee for Animal Research, Uppsala, Sweden approval number (5.8.18-10572/2019). The study was conducted in accordance with the local legislation and institutional requirements.
Author contributions
EE: Writing – review & editing, Writing – original draft, Visualization, Investigation. LS: Writing – review & editing, Writing – original draft, Visualization, Validation, Software, Methodology, Formal analysis, Data curation. GC: Writing – review & editing, Validation, Resources. HP: Writing – review & editing, Resources, Funding acquisition. GT: Writing – review & editing, Validation, Methodology, Investigation, Data curation. PE: Writing – review & editing, Writing – original draft, Validation, Supervision, Resources, Methodology, Investigation, Funding acquisition, Conceptualization. EI: Writing – review & editing, Supervision, Project administration, Investigation, Funding acquisition, Conceptualization.
Funding
The author(s) declare that financial support was received for the research, authorship, and/or publication of this article. The work was supported by FORMAS, a Swedish Research Council for Sustainable Development (Grant number: 2016-00365).
Acknowledgments
The authors wish to thank Dr. Annica Andersson and Professor Roger Andersson at the Department of Molecular Sciences, SLU, for great assistance with fiber analysis.
Conflict of interest
The authors declare that the research was conducted in the absence of any commercial or financial relationships that could be construed as a potential conflict of interest.
Publisher's note
All claims expressed in this article are solely those of the authors and do not necessarily represent those of their affiliated organizations, or those of the publisher, the editors and the reviewers. Any product that may be evaluated in this article, or claim that may be made by its manufacturer, is not guaranteed or endorsed by the publisher.
Supplementary material
The Supplementary Material for this article can be found online at: https://www.frontiersin.org/articles/10.3389/fmicb.2024.1396949/full#supplementary-material
References
Anderson, M. J. (2001). A new method for non-parametric multivariate analysis of variance. Austr. Ecol. 26, 32–46. doi: 10.1111/j.1442-9993.2001.01070.pp.x
Atterby, C., Mourkas, E., Meric, G., Pascoe, B., Wang, H., Waldenström, J., et al. (2018). The potential of isolation source to predict colonization in avian hosts: a case study in Campylobacter jejuni strains from three bird species. Front. Microbiol. 9:591. doi: 10.3389/fmicb.2018.00591
Aviagen. (2019). Ross Nutrition Specifications. Available olnline aa: https://www.cipa.com.co/wp-content/uploads/2019/11/RossBroilerNutritionSpecs2019-EN.pdf (accessed November 24, 2023).
Awad, W. A., Mann, E., Dzieciol, M., Hess, C., Schmitz-Esser, S., Wagner, M., et al. (2016). Age-related differences in the luminal and mucosa-associated gut microbiome of broiler chickens and shifts associated with Campylobacter jejuni infection. Front. Cell. Infect. Microbiol. 6:154. doi: 10.3389/fcimb.2016.00154
Bates, D., Mächler, M., Bolker, B., and Walker, S. (2015). Fitting linear mixed-effects models using lme4. J. Stat. Soft. 67, 1–48. doi: 10.18637/jss.v067.i01
Benjamini, Y., and Hochberg, Y. (1995). Controlling the false discovery rate: a practical and powerful approach to multiple testing. J. R. Stat. Soc. Ser. B Stat. Methodol. 57, 289–300. doi: 10.1111/j.2517-6161.1995.tb02031.x
Bolyen, E., Rideout, J. R., Dillon, M. R., Bokulich, N. A., Abnet, C. C., Al-Ghalith, G. A., et al. (2019). Reproducible, interactive, scalable and extensible microbiome data science using QIIME 2. Nat. Biotechnol. 37, 852–857. doi: 10.1038/s41587-019-0209-9
Boyner, M., Ivarsson, E., Wattrang, E., Sun, L., Wistedt, A., Wall, H., et al. (2023). Effects of access to feed, water, and a competitive exclusion product in the hatcher on some immune traits and gut development in broiler chickens. Br. Poult. Sci. 12, 1–13. doi: 10.1080/00071668.2022.2163152
Chen, C.-Y., Nace, G. W., and Irwin, P. L. (2003). A 6 × 6 drop plate method for simultaneous colony counting and MPN enumeration of Campylobacter jejuni, Listeria monocytogenes, and Escherichia coli. J. Microbiol. Methods. 55, 475–479. doi: 10.1016/S0167-7012(03)00194-5
Cherry, P., Yadav, S., Strain, C. R., Allsopp, P. J., McSorley, E. M., Ross, R. P., et al. (2019). Prebiotics from seaweeds: An ocean of opportunity? Mar. Drugs 17:327. doi: 10.3390/md17060327
Connerton, P., Flaujac Lafontaine, G., O'Kane, P., Ghaffar, N., Cummings, N., Smith, D., et al. (2018). The effect of the timing of exposure to Campylobacter jejuni on the gut microbiome and inflammatory responses of broiler chickens. Microbiome 6:88. doi: 10.1186/s40168-018-0477-5
Core Team, R. (2019). R: A Language and Environment for Statistical Computing. Vienna: R Foundation for Statistical Computing.
Dunn, O. J. (1964). Multiple comparisons using rank sums. Technometrics, 6, 241–252. doi: 10.1080/00401706.1964.10490181
EFSA (2020). Update and review of control options for Campylobacter in broilers at primary production. EFSA J. 18:e06090. doi: 10.2903/j.efsa.2020.6090
French, N. P., Midwinter, A., Holland, B., Collins-Emerson, J., Pattison, R., Colles, F., et al. (2009). Molecular epidemiology of Campylobacter jejuni isolates from wild-bird fecal material in children's playgrounds. Appl. Environ. Microbiol. 75, 779–783. doi: 10.1128/AEM.01979-08
Griekspoor, P., Colles, F. M., McCarthy, N. D., Hansbro, P. M., Ashhurst-Smith, C., Olsen, B., et al. (2013). Marked host specificity and lack of phylogeographic population structure of Campylobacter jejuni in wild birds. Mol. Ecol. 22, 1463–1472. doi: 10.1111/mec.12144
Gripp, E., Hlahla, D., Didelot, X., Kops, F., Maurischat, S., Tedin, K., et al. (2011). Closely related Campylobacter jejuni strains from different sources reveal a generalist rather than a specialist lifestyle. BMC Genom. 12, 1–21. doi: 10.1186/1471-2164-12-584
Halekoh, U., and Højsgaard, S. (2014). A kenward-roger approximation and parametric bootstrap methods for tests in linear mixed models–the R package pbkrtest. J. Stat. Softw. 59, 1–32. doi: 10.18637/jss.v059.i09
Ivarsson, E., Wall, H., Boyner, M., Cervin, G., Pavia, H., Wattrang, E., et al. (2023). Effects of algal supplementation in feed to broiler breeders on transfer of nutrients and antibodies to chicks and quality of hatchlings. Animal 17:101020. doi: 10.1016/j.animal.2023.101020
Ivarsson, E., Wattrang, E., Sun, L., Cervin, G., Pavia, H., Wall, H., et al. (2022). Evaluation of early feed access and algal extract on growth performance, organ development, gut microbiota and vaccine-induced antibody responses in broiler chickens. Animal 16:100522. doi: 10.1016/j.animal.2022.100522
Kers, J. G., de Oliveira, J. E., Fischer, E. A., Tersteeg-Zijderveld, M. H., Konstanti, P., Stegeman, J. A., et al. (2020). Associations between phenotypic characteristics and clinical parameters of broilers and intestinal microbial development throughout a production cycle: a field study. Microbiologyopen 9:e1114. doi: 10.1002/mbo3.1114
Kers, J. G., Velkers, F. C., Fischer, E. A., Hermes, G. D., and Stegeman, J. A. (2018). Host and environmental factors affecting the intestinal microbiota in chickens. Front. Microbiol. 9:322066. doi: 10.3389/fmicb.2018.00235
Kruskal, W. H. (1952). Use of ranks in one-criterion variance analysis. J. Am. Stat. Assoc. 47, 583–621. doi: 10.1080/01621459.1952.10483441
Kuznetsova, A., Brockhoff, P. B., and Christensen, R. H. B. (2017). lmerTest package: tests in linear mixed effects models. J. Stat. Softw. 82:13. doi: 10.18637/jss.v082.i13
Liao, X., Shao, Y., Sun, G., Yang, Y., Zhang, L., Guo, Y., et al. (2020). The relationship among gut microbiota, short-chain fatty acids, and intestinal morphology of growing and healthy broilers. Poult. Sci. 99, 5883–5895. doi: 10.1016/j.psj.2020.08.033
Marco, M. L., and Tachon, S. (2013). Environmental factors influencing the efficacy of probiotic bacteria. Curr. Opin. Biotechnol. 24, 207–213. doi: 10.1016/j.copbio.2012.10.002
Mourkas, E., Yahara, K., Bayliss, S. C., Calland, J. K., Johansson, H., Mageiros, L., et al. (2022). Host ecology regulates interspecies recombination in bacteria of the genus Campylobacter. Elife 11:e73552. doi: 10.7554/eLife.73552.sa2
Ocejo, M., Oporto, B., and Hurtado, A. (2019). 16S rRNA amplicon sequencing characterization of caecal microbiome composition of broilers and free-range slow-growing chickens throughout their productive lifespan. Sci. Rep. 9:2506. doi: 10.1038/s41598-019-39323-x
Pang, J., Looft, T., Zhang, Q., and Sahin, O. (2023). Deciphering the association between Campylobacter colonization and microbiota composition in the intestine of commercial broilers. Microorganisms 11:1724. doi: 10.3390/microorganisms11071724
Pedregosa, F., Varoquaux, G., Gramfort, A., Michel, V., Thirion, B., Grisel, O., et al. (2011). Scikit-learn: machine learning in Python. J. Mach. Learn. Res. 12, 2825–2830.
Salami, S., Guinguina, A., Agboola, J., Omede, A., Agbonlahor, E., Tayyab, U., et al. (2016). In vivo and postmortem effects of feed antioxidants in livestock: a review of the implications on authorization of antioxidant feed additives. Animal 10, 1375–1390. doi: 10.1017/S1751731115002967
Searle, S. R., Speed, F. M., and Milliken, G. A. (1980). Population marginal means in the linear model: an alternative to least squares means. Am. Stat. 34, 216–221. doi: 10.1080/00031305.1980.10483031
Sharma, S., Neves, L., Funderud, J., Mydland, L. T., Øverland, M., Horn, S. J., et al. (2018). Seasonal and depth variations in the chemical composition of cultivated Saccharina latissima. Algal Res. 32, 107–112. doi: 10.1016/j.algal.2018.03.012
Stanley, D., Geier, M. S., Hughes, R. J., Denman, S. E., and Moore, R. J. (2013). Highly variable microbiota development in the chicken gastrointestinal tract. PLoS ONE 8:e84290. doi: 10.1371/journal.pone.0084290
Sun, L., Lundh, Å., Höjer, A., Bernes, G., Nilsson, D., Johansson, M., et al. (2022). Milking system and premilking routines have a strong effect on the microbial community in bulk tank milk. J. Dairy Sci. 105, 123–139. doi: 10.3168/jds.2021-20661
Sweeney, T., and O'Doherty, J. (2016). Marine macroalgal extracts to maintain gut homeostasis in the weaning piglet. Domest. Anim. Endocrinol. 56, S84–S89. doi: 10.1016/j.domaniend.2016.02.002
Theander, O., Åman, P., Westerlund, E., Andersson, R., and Pettersson, D. (1995). Total dietary fiber determined as neutral sugar residues, uronic acid residues, and Klason lignin (the Uppsala method): collaborative study. J. AOAC Int. 78, 1030–1044. doi: 10.1093/jaoac/78.4.1030
Thibodeau, A., Fravalo, P., Yergeau, É., Arsenault, J., Lahaye, L., Letellier, A., et al. (2015). Chicken caecal microbiome modifications induced by Campylobacter jejuni colonization and by a non-antibiotic feed additive. PLoS ONE 10:e0131978. doi: 10.1371/journal.pone.0131978
Thomas, J. B., Sterner, M., Nylund, G. M., Albers, E., Edlund, U., Undeland, I., et al. (2022). The effects of cultivation deployment-and harvest-timing, location and depth on growth and composition of Saccharina latissima at the Swedish west coast. Aquaculture 559:738443. doi: 10.1016/j.aquaculture.2022.738443
Valečková, E., Sun, L., Wang, H., Dube, F., Ivarsson, E., Kasmaei, K. M., et al. (2023). Intestinal colonization with Campylobacter jejuni affects broiler gut microbiota composition but is not inhibited by daily intake of Lactiplantibacillus plantarum. Front. Microbiol. 14:1205797. doi: 10.3389/fmicb.2023.1205797
Venardou, B., O'Doherty, J., Vigors, S., O'Shea, C., Burton, E., Ryan, M., et al. (2021). Effects of dietary supplementation with a laminarin-rich extract on the growth performance and gastrointestinal health in broilers. Poult. Sci. 100:101179. doi: 10.1016/j.psj.2021.101179
Ver Hoef, J. M., and Boveng, P. L. (2007). Quasi-Poisson vs. negative binomial regression: how should we model overdispersed count data? Ecol. 88, 2766–2772. doi: 10.1890/07-0043.1
Waite, D. W., and Taylor, M. W. (2014). Characterizing the avian gut microbiota: membership, driving influences, and potential function. Front. Microbiol. 5:223. doi: 10.3389/fmicb.2014.00223
Waldenström, J., Axelsson-Olsson, D., Olsen, B., Hasselquist, D., Griekspoor, P., Jansson, L., et al. (2010). Campylobacter jejuni colonization in wild birds: results from an infection experiment. PLoS ONE 5:e9082. doi: 10.1371/journal.pone.0009082
Keywords: brown algae, Saccharina latissima, laminarin, Campylobacter jejuni, host specificity, microbiota, broiler
Citation: Eliasson E, Sun L, Cervin G, Pavia H, Tällberg G, Ellström P and Ivarsson E (2024) No colonization resistance to Campylobacter jejuni in broilers fed brown algal extract-supplemented diets. Front. Microbiol. 15:1396949. doi: 10.3389/fmicb.2024.1396949
Received: 06 March 2024; Accepted: 06 June 2024;
Published: 27 June 2024.
Edited by:
Karolina Skonieczna-Żydecka, Pomeranian Medical University, PolandReviewed by:
Egil Andreas Joor Fischer, Utrecht University, NetherlandsCristiane Cantiga-Silva, São Paulo State University (UNESP), Brazil
Copyright © 2024 Eliasson, Sun, Cervin, Pavia, Tällberg, Ellström and Ivarsson. This is an open-access article distributed under the terms of the Creative Commons Attribution License (CC BY). The use, distribution or reproduction in other forums is permitted, provided the original author(s) and the copyright owner(s) are credited and that the original publication in this journal is cited, in accordance with accepted academic practice. No use, distribution or reproduction is permitted which does not comply with these terms.
*Correspondence: Eliška Eliasson, eliska.valeckova@slu.se
†These authors have contributed equally to this work and share first authorship